- 1Chaire industrielle CRSNG-UQAT-UQÀM en aménagement forestier durable, Institut de recherche sur les forêts, Université du Québec en Abitibi-Témiscamingue, Rouyn-Noranda, QC, Canada
- 2Département de biologie, chimie et géographie, Université du Québec à Rimouski, Rimouski, QC, Canada
Settlement of eastern North America has generated significant modifications in forest composition. In regions highly influenced by human activity, historical ecology can be used to reconstruct pre-settlement forest composition. In this study, we reconstruct the composition of the pre-settlement (1909–1937) forest of a 4,134 km2 sector of the boreal mixedwood forest using early land survey archives. The pre-settlement composition was compared with modern composition using recent eco-forest inventories (1980–2008), and the influence of surficial deposits on compositional changes assessed. During the pre-settlement period, the landscape was primarily dominated by spruce, which was evenly distributed across surficial deposit types. Trembling aspen, although widespread, rarely dominated stands. In contrast, the present-day landscape is dominated by trembling aspen, notably on clay and till deposits. In general, conifers have undergone a severe reduction in frequency. Spruce and pine forests are today mainly restricted to organic and sandy surficial deposits, respectively, compared to their historical frequencies. Composition changes observed in the boreal mixedwood forest of western Quebec are essentially the results of fires and forest harvesting, but surficial deposits have affected the current abundance and spatial distribution of the different taxa. In the context of sustainable forest management, considerable effort should be deployed to restore conifer dominance in the region, notably on the fertile deposits that appear particularly susceptible to composition changes.
Introduction
Human activity in North America has modified species distribution and composition of vegetation communities (Nowacki and Abrams, 2015). Settlement and industrialisation have been accompanied by changes in disturbance regimes and in land use which have caused important shifts in forest composition and landscape homogenisation in many regions (Whitney, 1994; Foster et al., 1998; Lorimer, 2001; Hanberry et al., 2012). Current remnants of natural forests are therefore rare and hardly representative of landscape-scale composition (Nowak, 1993; Abrams et al., 1995; Foster et al., 1996). In such regions, historical ecology can be the most viable option for reconstructing past forest composition and determining changes that have occurred over the last centuries (Morgan et al., 1994; Swetnam et al., 1999), as a reference to develop ecosystem-based forest management and restoration.
In the field of historical ecology, survey archives have been frequently used to reconstruct pre- settlement or pre-industrial forest composition (Bourdo, 1956; Egan and Howell, 2001), and to understand the impact of recent human activities on changes in forest composition (Nowacki and Abrams, 2015; Danneyrolles et al., 2016). Although archives only offer a portrait of ecosystems at a certain moment in time, they do have the advantage of providing spatially explicit observations of disturbances and vegetation with reasonably good spatial (generally a few dozen meters) and taxonomic resolution (species or genus). These kinds of archival records have been used notably to quantitatively describe and compare pre-settlement and modern-day forest compositions (e.g., Schulte et al., 2007; Pinto et al., 2008), to reconstruct pre-settlement disturbance regimes (e.g., Canham and Loucks, 1984; Whitney, 1986), and to determine the influence of edaphic factors on pre-settlement composition (e.g., Whitney, 1982; Barrett et al., 1995). This said, little attention has been given to understanding the influence of edaphic conditions on compositional changes (but see Whitney and DeCant, 2003), most notably in the boreal forest.
Edaphic conditions in the boreal forest of western Quebec play an important role in the distribution of species (Gauthier et al., 2000; Brassard and Chen, 2006). First, they influence species establishment (Royer-Tardif and Bradley, 2011; Lafleur et al., 2015) and, therefore in a direct way, stand composition. Secondly, they influence fire regime, in particular fire severity and frequency (Johnson, 1992; Mansuy et al., 2010; Portier et al., 2019). In this region, mixed stands are abundant on mesic sites and are characterized by balsam fir (Abies balsamea), white spruce (Picea glauca), white birch (Betula papyrifera) and trembling aspen (Populus tremuloides, referred hereafter as aspen). Xeric and hydric sites, however, support conifer stands characterized by jack pine (Pinus banksiana) and black spruce (Picea mariana), respectively (Bergeron and Bouchard, 1984). Given the influence of soils on forest composition and disturbance regime, our principal objective is to document vegetation changes over time and to verify if edaphic conditions contributed to these changes in the boreal forest of eastern Canada.
To answer these questions we (1) reconstructed using early land survey archives, the pre-settlement composition of a sector of the boreal mixedwood forest situated in the Claybelt of western Quebec (administrative region of West-Abitibi), (2) compared pre-settlement forest composition with current composition established from recent eco-forest inventories, and (3) determined whether observed forest composition changes differed among surficial deposits. The Claybelt region is ideal for determining if surfical deposits influenced compositional changes because productive deposits (i.e., clays and tills) are abundant and the presence of aspen on these sites make them particularly susceptible to composition changes due to changes in the disturbance regime (see Laquerre et al., 2009). Several ecological issues related to changes in forest composition are anticipated in the boreal mixedwood forest of western Quebec, notably the reduction of white spruce and Eastern white cedar (Thuja occidentalis), and intolerant hardwood expansion or encroachment (Grondin and Cimon, 2003; Jetté et al., 2013). We assume that expansion of intolerant hardwoods, particularly aspen, occurred in the study area, especially on the more productive surficial deposits where conifer-intolerant hardwood mixtures were most frequent during pre-settlement.
Materials and Methods
Study Area
The study area covers an area of 4,134 km2 in northwestern Quebec, eastern Canada (between 48°25′ and 49°01′N, 78°38′ and 79°31′W) (Figure 1). The study area corresponds to the Quebec administrative region of West-Abitibi. The climate is subpolar-subhumid, with average temperature and precipitation (1981–2010) of 0.8°C and 886 mm at Lac Berry (70 km east of the study area), and 1°C and 985 mm in Mont Brun (45 km south-east of the study area) (Environment Canada, 2019). Regional topography is generally flat with mean elevation in the order of 300 m (Blouin and Berger, 2002). The southern part of the study area does include some hillier areas that reach up to 570 m in elevation.
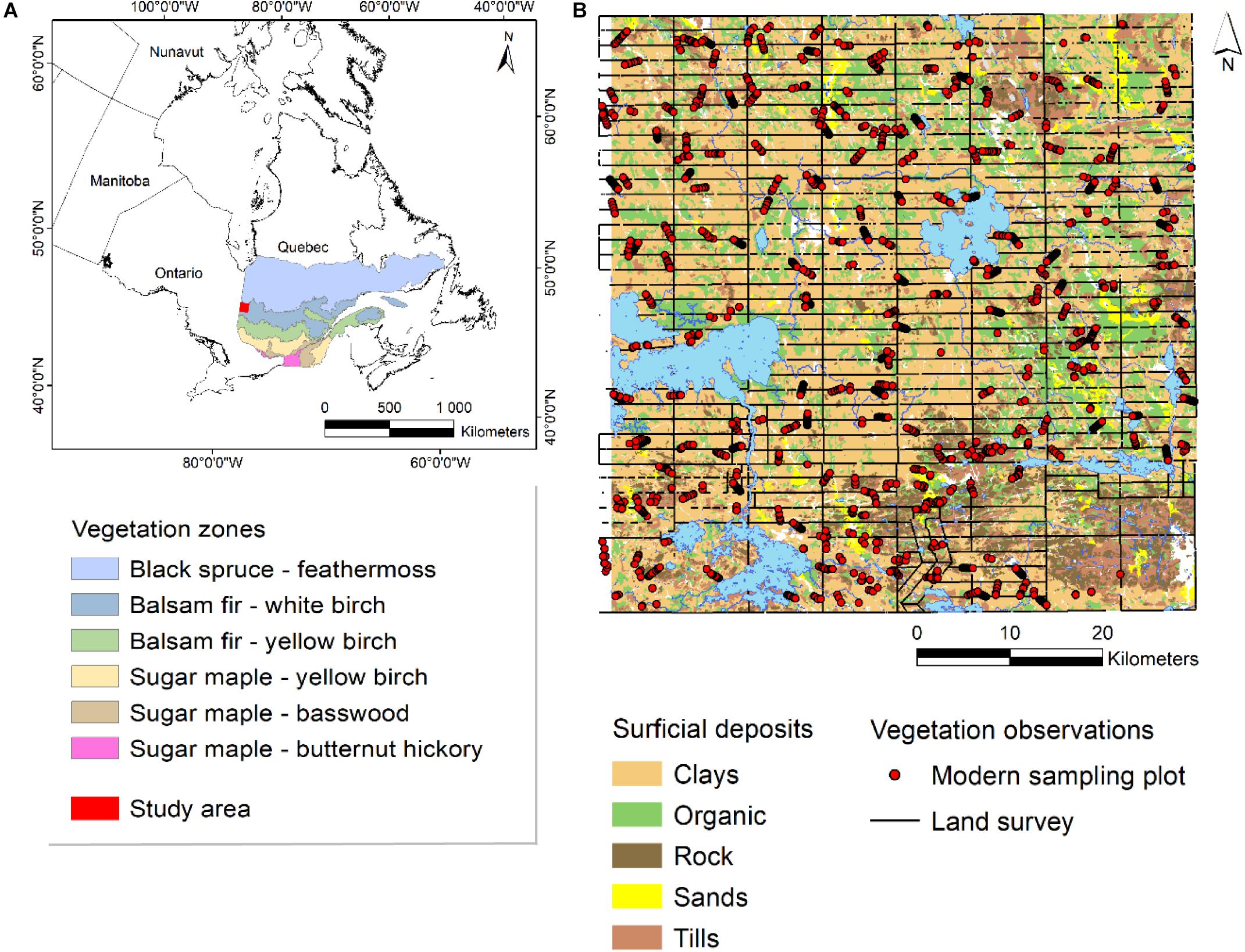
Figure 1. Location of the study area according to the ecological land classification of the Ministère des ressources naturelles du Québec (Saucier et al., 2009) (A). Study area and distribution of surficial deposits, land survey data and modern sampling plots (B).
The study area is situated in a physiographic zone known as the Claybelt (Blanchard, 1954; Bergeron et al., 1982), that covers a wide band of land of approximately 125,000 km2 in Ontario and Quebec. The Claybelt is characterized by the presence of a thick layer of clay deposits (55% of the study area). These sediments were deposited in the depths of pro-glacial Lake Ojibway that covered almost the entire region 9,000 years ago (Veillette, 1994). Higher hilly zones that were not submerged during this period are characterized by the presence of tills (11% of the study area), whereas lower hills which were in contact with the pro-glacial lake have outcrops of metamorphic Precambrian bedrock (8% of the study area). Sandy deposits (3% of the study area) are partly of fluvial-glacial origin, and partly established in sub-littoral zones during the progressive decline of the lake water level. Since the complete retreat of the waters around 8,000 years ago, organic deposits have accumulated on poorly drained clays (21% of the study area) (Thibaudeau and Veillette, 2005).
The study area is situated in the southeastern part of the boreal mixedwood forest, which corresponds to the Thermoboreal bioclimatic subdivision of the Canadian Boreal Biome (Baldwin et al., 2012). This forest is characterized by a complex mosaic of forest types which contain variable proportions of conifer and intolerant broadleaved species (Bergeron et al., 2014). The southern part of the study area belongs to Rowe (1972) Missinaibi-Cabonga forest section and the northern part to the Northern Clays section. The principal tree species present are black and white spruce, balsam fir, white birch and aspen. Jack pine generally dominates on xeric sites whereas black spruce is characteristic of hydric sites (Ecoregions Working Group, 1989).
Land Use History
Archeological evidence of the Abitibiwinnis places their presence in the region to ca. 5,000 years before present (Vincent, 1995). These nomadic hunter-gatherers used different tree species such as white birch, balsam fir, black and white spruce and white cedar for construction of shelter, canoes, etc., as well as for heating and cooking. The Abitibiwinnis (estimated population 300–500 up until the settlement period, Statistique Canada, 2015) used the land extensively, so their impact on the forest composition is believed to have been relatively low. Beginning in 1912, the region was opened up to Euro-Canadian settlement and forest exploitation. Up until 1930, this impact was concentrated along the corridor of the Transcontinental railway and waterways used for floating logs to sawmills (Vincent, 1995). Starting in 1935, demand for wood increased substantially following a major rise in population as settlement plans were implemented (Barrette, 1972; Asselin, 1982), and the mining industry developed. This increase in wood demand, associated with mechanization of forest harvesting operations beginning in the 1940s, resulted in the expansion and intensification of forest exploitation in the region. To illustrate, 538,500 m3 were cut in 1925 in the Abitibi region compared to 1,370,573 m3 in 1948 (Vincent, 1995), and the number of sawmills increased from 43 in 1919 (Trudelle, 1938) to 122 in 1948 (Blanchard, 1949). Up to the end of the 1950s, the industry essentially harvested fir, spruce and pine (Vincent, 1995); aspen has been industrially harvested since the 1960s. Clear-cut harvesting was systematically replaced by “clear-cut with protection of advance regeneration and soils” (coupe avec protection de la régénération et des sols (CPRS), in French) in the 1990s. CPRS is applied systematically today and harvesting is concentrated on spruce and aspen.
Data Sources
Pre-settlement data (1909–1937) were derived from survey notebooks housed in the Registry of the Surveyor General of Quebec. Early land surveys divided the public lands into townships, lines and lots of uniform areas, under the authority of the Surveyor General. The surveyors have provided spatially explicit observations of the vegetation and disturbances at this time. These observations were associated with township lines and lot boundaries and their precise positions were noted (location from the beginning to the end of the observation, i.e., observation length). Vegetation observations could take the form of a taxonomic list, or as a cover type (alder swamp, mixed species…). Several lines of evidences from all regions of the province indicate that taxa where consistently listed in descending order of importance. First, a direct comparison with an early forest inventory (number of stems per taxa per DBH class) indicates that ranks of taxa in lists correspond directly to their relative basal area in stands (Terrail et al., 2014). Second, a comparison between archive types (i.e., taxa lists vs. stems regularly selected along range lines) indicates that the most frequent line trees correspond to the taxa listed first (Fortin, 2018). Third, several surveyors inverted taxa pairs between consecutive observations but used expressions such as ‘ditto’ or ‘same as before’ to indicate identical successive stands (Dupuis et al., 2011). Fourth, taxa are often increasingly shifted to the right as they are listed one below the other to indicate a decreasing importance. Sources for “modern” data were temporary sampling plots (TSP) of the last three eco-forest inventories undertaken by the Government of Quebec (1980, 1990, 2000) (Boudreau and Philibert, 2011). These inventories use 400 m2 circular sampling plots distributed over an area stratified by productive forest stand types. The number of plots in each stand type is based on its relative area on the land base. In each plot, the diameter of all stems >9 cm at breast height (DBH) is measured and the diameter of all stems >1 and ≤9 cm DBH is measured in a 40 m2 sub-plot. Stems are identified to species allowing calculation of basal area by species. All stems >1 cm DBH are used in the modern-day database.
Databases
The pre-settlement database contains 3,194 taxonomic lists, or 2,063 km of surveyed lines (mean length = 650 m) from 44 of the 80 survey notebooks available for the study area. The retained notebooks cover the period from 1909 to 1937. The choice of notebooks maximized the proportion of the area surveyed, while minimizing surveyed lines associated with anthropic disturbances. Because the initial surveying was undertaken prior to settlement, primitive notebooks were systematically preferred to resurvey notebooks. Some resurvey entries were nonetheless used when no disturbance occurred between observations documented in the primitive survey and resurvey and the latter provided a more precise vegetation description, in ecological and/or spatial terms. Because surveyors did not systematically distinguish the different species belonging to Picea and Pinus genera, these taxa were grouped to the genus level. This said, Pinus represents essentially jack pine, given that white (P. strobus) and red (P. resinosa) are at their northern limit in the south of our study area. A rank was attributed to taxa according to the order in which they were registered in survey entries; hereafter, we refer to a first-ranked taxon as being a dominant taxon. All observations were georeferenced using a modern digital cadastral map (original scale 1:20,000).
The modern-day database contains 2,496 plots available for the study area and covering the period of 1980–2008. For each plot, spruce and pine measurements were grouped into their respective genera to permit comparison with the pre-settlement data set. American mountain ash (Sorbus americana) and pin cherry (Prunus pensylvanica) were aggregated into the category “Other.” Basal area by taxon was then calculated and taxa representing less than 5% of plot total basal area were excluded. This choice was made to match pre-settlement and contemporary taxa number in lists. Indeed, in modern plots, all taxa occurring have been recorded, whereas the surveyors may have overlooked the less important taxa at several sites. Lower cut-offs of 0% (i.e., including all taxa) and 10% were also tested. Once these data were excluded, a final ranking was attributed to each taxon in decreasing order of basal area.
Data Analyses
Forest compositions for pre-settlement and modern-day periods were compared using two indices calculated for each database. First, a general frequency, that is, the relative frequency of occurrence expressed as a percentage of all observations (i.e., surveyed lines or plots) made in the study area, was calculated at the taxon level. Second, a rank frequency was calculated for the four first ranks of the taxon lists using the following formula (Scull and Richardson, 2007):
where L(Xr) is the total length of observations in which taxon X is registered to rank r in the list of taxa and Lr is the total length of observations with at least r taxa noted in the taxonomic lists.
General frequencies of taxa were compared spatially and statistically between the pre-settlement and modern periods using a grid of 256 16-km2 cells. Several sizes of cells were tested. Surveyed lines and retained sampling plots were situated ≤1.6 km from each other to not compare observations too distant; this distance corresponded to the longest surveyed lines (i.e., weakest spatial precision). Each cell contained at least four surveyed lines and four TSPs. Ultimately, 109 cells containing four to 32 survey lines (mean = 12) and four to 52 TSPs (mean = 14) were retained. The cells’ number has been mostly reduced by the criterion of minimum number of observations per cell. The frequency index was recalculated by taxon for each cell. A Wilcoxon signed-rank test for paired samples was done using the R Stats library (version 3.1.2, R Foundation for Statistical Computing, Vienna, AT). Under H0, no significant difference of taxon frequencies exists between the pre-settlement and modern-day periods.
Taxonomic distribution on the principal surficial deposits was examined for the two periods using contingency tables. Only taxa with a general frequency >5% were included in this analysis. In order to determine the type of surficial deposit associated with each observation, survey lines and TSPs were overlaid on maps of the fourth (most recent) provincial inventory. Surficial deposit information integrated in these maps is derived from 1:15,000 scale aerial photos (Lord et al., 2009). If a survey line crossed several deposit types, the survey line was “cut” in as many observations (i.e., lines) as there are types of surface deposits. For plots, a unique deposit type is already attributed in eco-forest inventories. Contingency tables were built for each combination of the surficial deposit – taxon variables (Strahler, 1978). A G-test with Williams’ correction was employed to test the independence between taxon distribution and surficial deposit type (Sokal and Rohlf, 2012). For taxa that presented a significant (α = 0.05) G statistic, namely white birch, aspen, balsam fir, spruces and pines, corrected standardized residuals were calculated according to the method established by Haberman (1973). A positive residual indicated an over-representation of a taxon on a given deposit type relative to a random distribution, and a negative residual indicates an under-representation.
Results
During the pre-settlement period (Table 1), spruces and balsam fir were the most frequent taxa (87.3 and 50.9%, respectively) and the most dominant in the study area (67.7 and 15.0%, respectively). White birch, aspen and pine also had a relatively high frequency (39.7–31.6%), but their dominance was relatively low (6.1–4.5%). White birch and aspen were respectively most frequent of the third and fourth ranks of taxon lists, which indicates that these two taxa were essentially companion species.
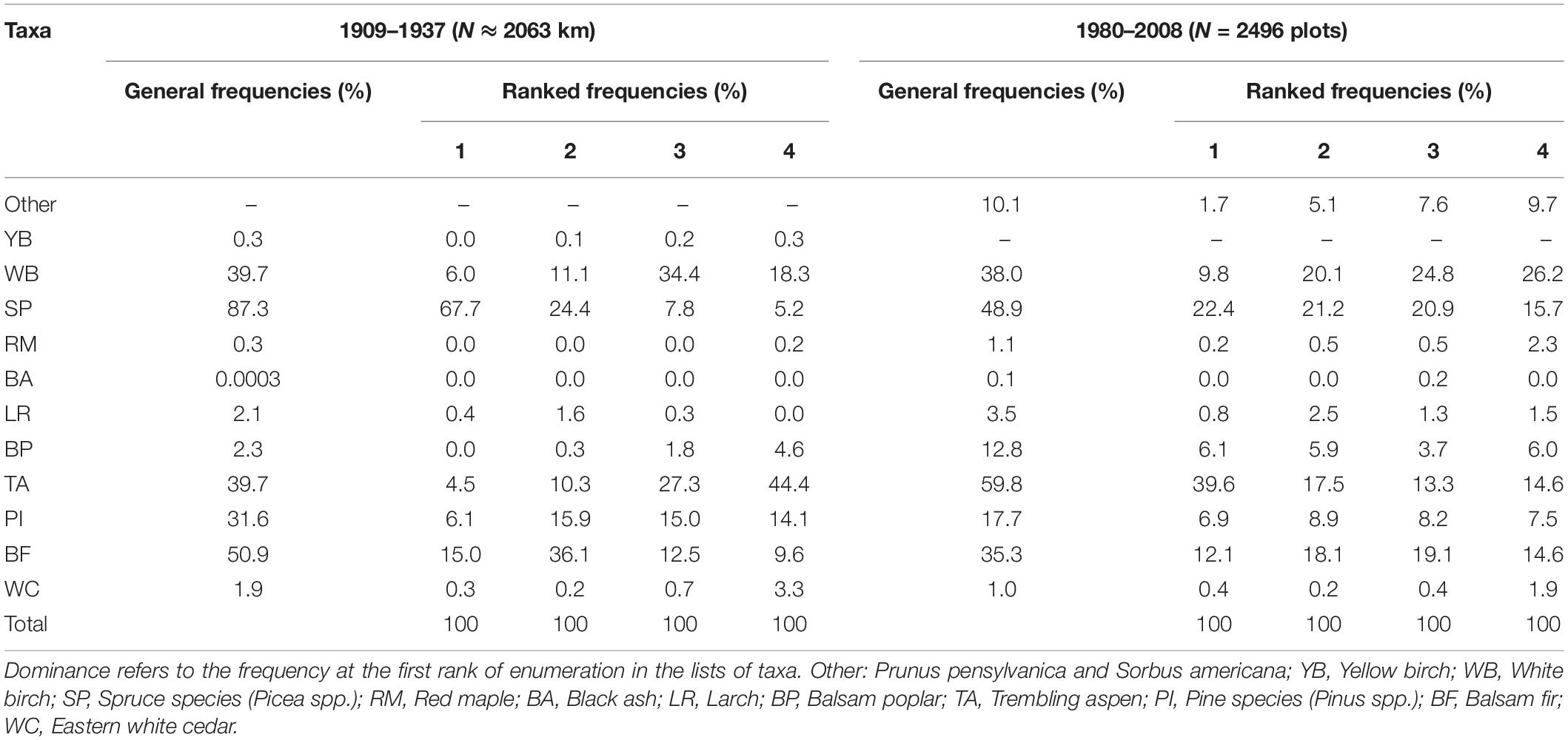
Table 1. General frequencies and ranked frequencies of taxa during pre-settlement (1909–1937) and modern (1980–2008) periods.
Comparison of pre-settlement and modern forest compositions indicates important composition changes (Table 1). Aspen registered a marked expansion in the study area, with frequency and dominance increased by a factor of 1.5 and 8.8, respectively. At the same time, spruces, balsam fir and pines registered a marked frequency decrease (by a factor of 1.8, 1.5, and 1.5 respectively), and the spruces dominance was reduced by a factor of 3. The frequency of eastern white cedar was also severely reduced (by a factor of 2).
Frequency differences in cells between the pre-settlement and modern periods were statistically significant for all taxa (α = 0.01) (Figure 2). Spruces showed decreases in frequency in practically all cells, whereas variations in frequency of white birch, pines, balsam fir and aspen were spatially more heterogeneous. Spruces virtually disappeared in a large number of cells. The increase in frequency of aspen was most often between 10 and 20%, but numerous cells present higher frequency increases (40–70%).
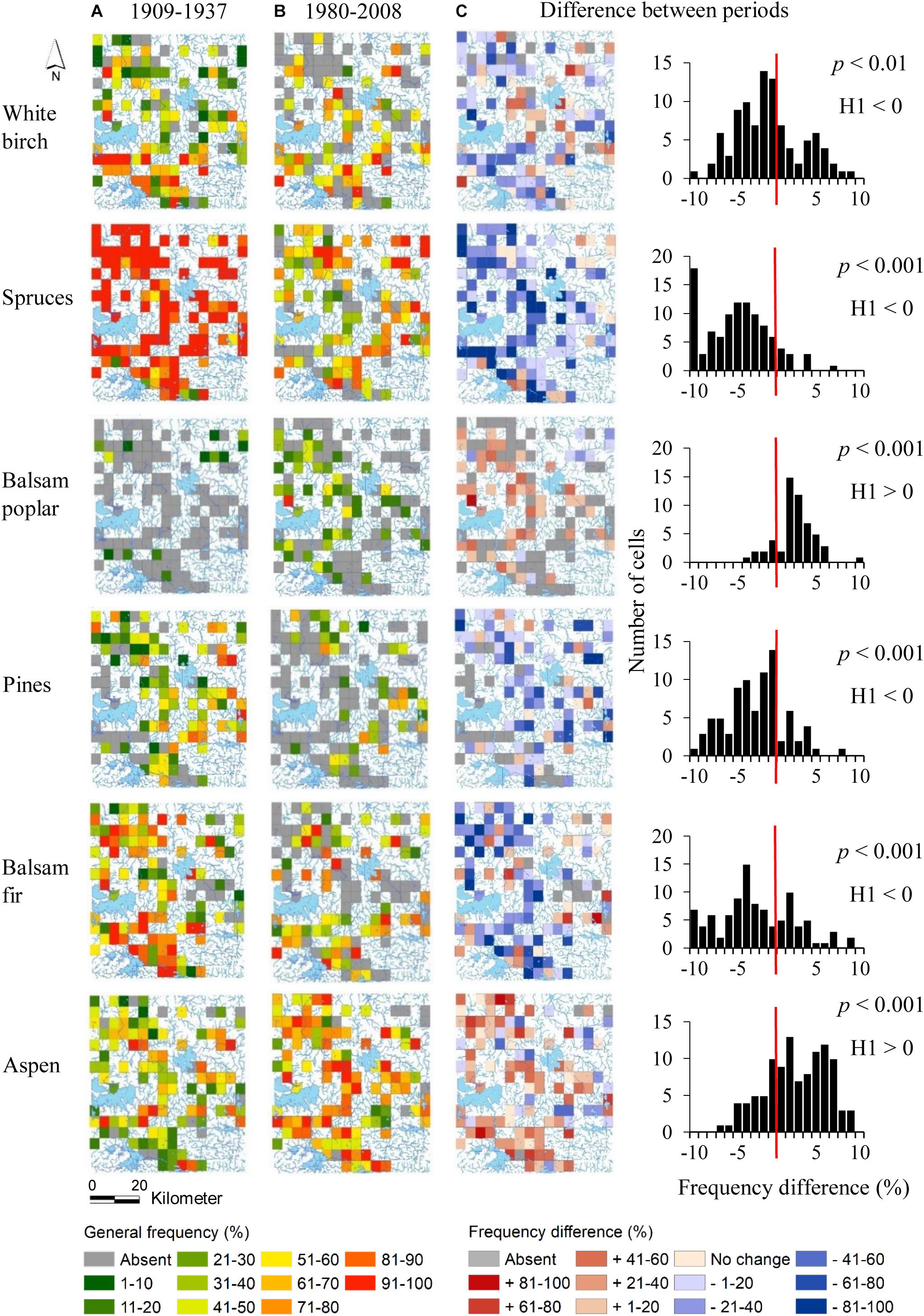
Figure 2. General frequency maps of the principal taxa during pre-settlement (A) and modern (B) periods, and of frequency distribution differences between the two periods (C). A positive difference indicates that the taxon is more frequent in the modern period than in the pre-settlement period. P values for Wilcoxon signed-rank tests are also indicated. Under H0, no significant difference of taxon frequencies exists between the pre-settlement and modern-day periods. H1 indicates the direction of the tests. X axes on graphs by frequency classes of 10%. For example, for the value of 10, the corresponding frequency class is +91–100%. Aspen refers to trembling aspen. Pines and spruces are grouped to genus level.
Taxon distribution on the different surficial deposit types changed between the pre-settlement and modern periods (Figure 3). Spruce and pine distributions are now more restricted to organic deposits, and sands and rocky outcrops, respectively, than they were during the pre-settlement period. Moreover, spruces and pines are the most under-represented taxa on clay deposits in the modern forest, while aspen is the most over-represented. White birch is currently the most over-represented taxon on tills as well as, with pines, the most over-represented taxon on rocky outcrops.
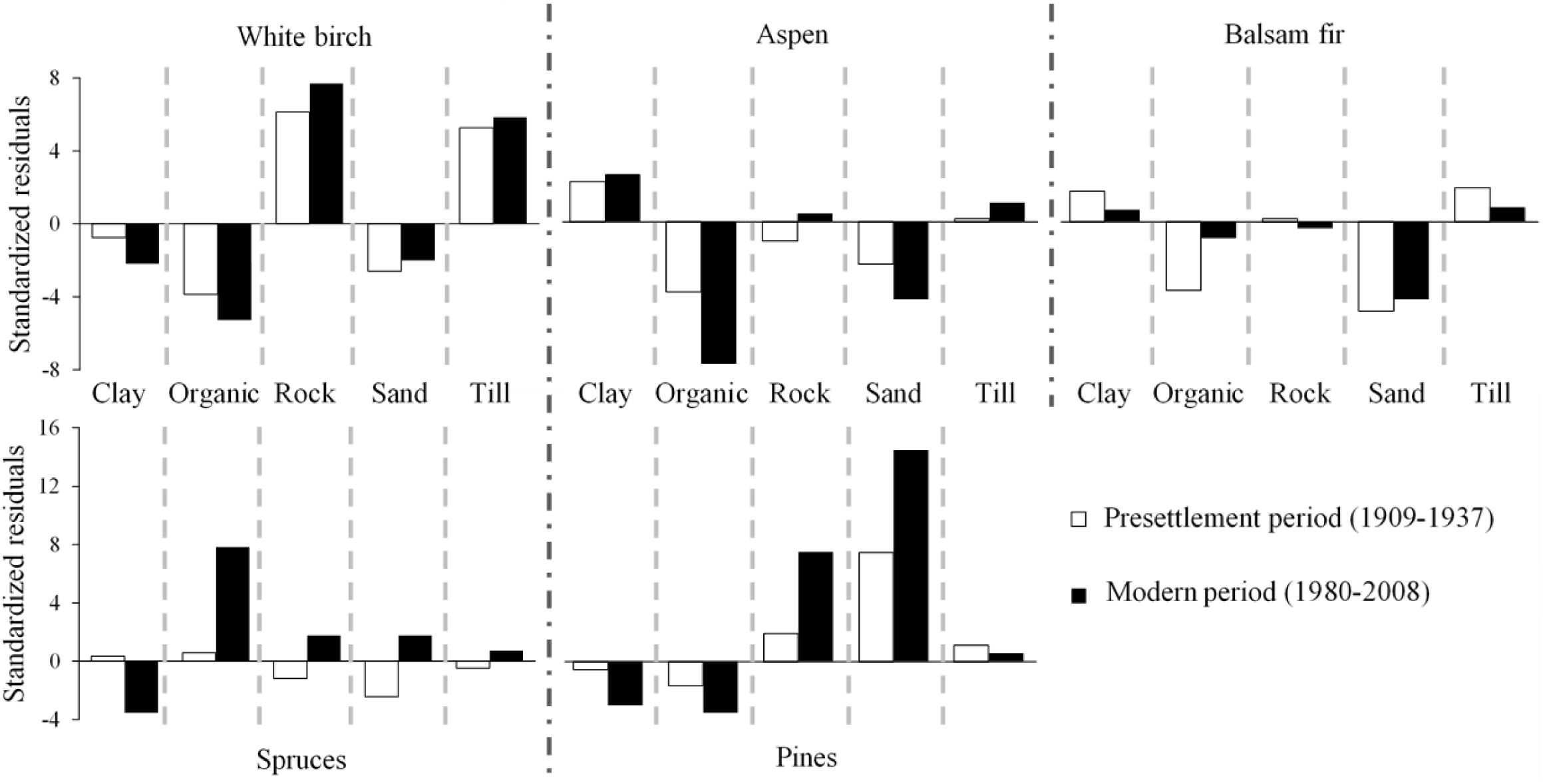
Figure 3. Corrected standardized residuals by type of surficial deposits during pre-settlement (1909–1937) and modern (1980–2008) periods, for taxa that presented a significant (α = 0.05) G statistic. A positive value indicates an over-representation of a taxon relative to a random distribution. A negative value indicates an under-representation. Y axes scale differ between the graphs. Aspen refers to trembling aspen. Spruces and Pines are grouped to genus level.
Discussion
Composition Changes and Their Causes
Significant composition changes occurred in the study area between the pre-settlement and modern periods (Table 1 and Figure 2). Balsam poplar (Populus balsamifera) and aspen registered particularly large frequency increases whereas spruces, balsam fir, and pines have decreased. Aspen effectively replaced spruces on the modern landscape, shifting from spruce (68%) dominance in the past to aspen (40%) today. Similar compositional changes were found in the boreal forests of northern Minnesota (Friedman and Reich, 2005; Nowacki and Abrams, 2015). Immediately south of our study area, Danneyrolles et al. (2016) also observed a landscape-level transition from an historic domination by spruces (47%) and balsam fir (21%) to a modern-day landscape dominated by aspen and balsam poplar (29%) and spruces (19%). Significant increases in dominance of aspen, to the detriment of conifer taxa has also been observed in several other regions of Québec (e.g., Fortin, 2008; Dupuis et al., 2011), and elsewhere in eastern North America (e.g., White and Mladenoff, 1994; Weir and Johnson, 1998; Jackson et al., 2000; Schulte et al., 2007; Pinto et al., 2008).
Increases in aspen frequency and dominance in the study area are essentially due to the combination of fires that occurred during the settlement period (Lefort et al., 2003) and mechanized clear-cutting. Aspen is effectively able to establish on sites that have been recently burned (e.g., Baker, 1925; Stahelin, 1943) and/or harvested (e.g., Doucet, 1979; Bartos and Mueggler, 1982) by sexual and asexual reproduction (Peterson and Peterson, 1992), and to become dominant despite relatively synchronous establishment of several other species (Gutsell and Johnson, 2002; Johnstone et al., 2004). This dominance is possible because of its initial superior height growth compared to competing species, especially slow-growing, shade-tolerant trees (Bergeron, 2000; Gutsell and Johnson, 2002; Cardona, 2014), notably in the case of asexual reproduction where young ramets exploit the root system of parent trees (Barnes, 1966).
Because our historical portrait of disturbances is incomplete, we were unable to discriminate the proportion of the increase in aspen frequency and dominance that was due to fire vs. cutting. Nonetheless, fires that occurred during settlement (Lefort et al., 2003) probably played an important role, as has been demonstrated in the boreal forest of the Saguenay region (Boucher et al., 2016). In effect, a large part of the study area was burned during the settlement period (Bergeron et al., 2001) and post-fire dynamics result generally in the dominance of shade-intolerant aspen, most notably on mesic sites (Bergeron and Charron, 1994; Bergeron, 2000). Moreover, considerable increases in abundance are sometimes possible even when aspen constitutes only a minor component of the prior stand (Chen et al., 2009; Ilisson and Chen, 2009), as was the case in many pre-settlement forests. The presence of a few scattered individuals can effectively allow aspen to dominate a stand following a severe disturbance due to the persistence of a well-developed root system (Lavertu et al., 1994) and presence of seed trees. Given the extensive spatial distribution of aspen during the pre-settlement period (Figure 2A), an important increase in frequency and dominance probably occurred following fires via both sexual and asexual reproduction. Indeed, pre-settlement spatial distribution was clearly a key element in its post-settlement expansion.
In areas where aspen is absent, it can establish in certain stands by seeding following fires (e.g., Kay, 1993; Quinn and Wu, 2001) or mechanical clear-cutting (e.g., Landhäusser et al., 2010). Aspen seeds can be wind-dispersed over many kilometers (Perala, 1990; Turner et al., 2003), thereby allowing colonization of distant sites where good germination beds, particularly those characterized by a thin layer of residual organic matter (Johnstone and Chapin, 2006; Greene et al., 2007; Gewehr et al., 2014; Lafleur et al., 2015), and adequate soil moisture (Faust, 1936; Perala, 1990), are available. Colonization of new sites also can be facilitated by the fact that continuous recruitment can occur over several years following a severe disturbance (Quinn and Wu, 2001; Romme et al., 2005; Landhäusser et al., 2010). Once established, aspen seedlings can sucker from the age of one or two years (Day, 1944; Perala, 1990). Therefore, if a new severe disturbance such as a clear-cut occurred following establishment by seed, aspen would be able to expand its presence by suckering. Clear-cuts by mechanical harvesting that occurred after fires associated with the settlement period must therefore have allowed the aspen to maintain – indeed expand – its frequency and dominance by suckering (see Weir and Johnson, 1998; Laquerre et al., 2009).
Frequency and dominance variations in spruces, pines and balsam fir could also be explained by the occurrence of fires and cutting. Fire must have at least temporarily reduced the frequency and dominance of balsam fir and white spruce, given their dependence on living seed trees to recolonize burned sites (Galipeau et al., 1997), and the short dispersal distance of their seeds (Dobbs, 1976; Sims et al., 1990), resulting in a relatively slow recolonization following large, severe fires (Greene et al., 1999). White spruce establishment could also have been limited if fires occurred during years of low seed production (Purdy et al., 2002; Peters et al., 2005). Moreover, with the opening of the Abitibi Power and Paper mill in 1915, balsam fir, white and black spruce were targeted for harvesting (Vincent, 1995), which reduced the availability of seed trees; this likely limited their capacity to re-establish following fire. Mechanized harvesting also caused considerable direct and indirect mortality (i.e., via soil compaction) of advanced conifer regeneration (Harvey and Bergeron, 1989), again limiting the re-establishment capacity of these taxa. The decrease in frequency of pines in the region was largely the result of their harvesting to supply sawmills (Vincent, 1995).
Differences Between Surficial Deposits
Composition changes observed in the study region differed between types of surficial deposits. The transition from a spruce-fir dominance to an aspen dominance was more marked on the more productive sites, where the species mix was greater during the pre-settlement period. The increase in frequency and dominance of aspen occurred essentially on clays and tills (Supplementary Figures 1, 2), as observed in other studies undertaken in the region (Bergeron and Dubuc, 1989; Bergeron and Charron, 1994; Laquerre et al., 2009). These sites appear to be particularly sensitive to compositional changes following severe disturbances. Aspen regeneration following fire or harvesting is, in effect, generally strongly related to its basal area prior to disturbance (Graham et al., 1963; Greene and Johnson, 1999; Chen et al., 2009; Ilisson and Chen, 2009), and to site index in the case of harvesting (Frey et al., 2003). Moreover, stands dominated by balsam fir and white spruce are particularly vulnerable to transitioning toward aspen dominance following fire (Chen et al., 2009). As they occur in the boreal mixedwood forest, these types of stands are characteristically found on productive surficial deposits (Blanchard, 1949; Bergeron and Bouchard, 1984).
On organic deposits, aspen currently remains strongly under-represented (Figure 3), whereas spruces maintained their frequency and dominance (Supplementary Figures 1, 2). Organic matter thickness is recognized as the primary factor limiting the capacity of aspen to establish and grow, as much for sexual as asexual reproduction (Anyomi et al., 2013, 2014; Gewehr et al., 2014; Lafleur et al., 2015). Thick organic deposits can limit aspen presence by inducing low soil temperatures, high soil moisture and/or limiting access to the mineral soil (Van Cleve et al., 1983; Simard et al., 2007). A soil temperature of 8°C or less can inhibit sucker emergence (Landhäusser et al., 2006), while a temperature under 5°C can inhibit root growth and limit photosynthesis, water absorption and leaf growth (Landhäusser and Lieffers, 1998; Wan et al., 1999, 2001; Landhäusser et al., 2001). Soil water saturation is also known to limit initiation of aspen suckers (Schier et al., 1985; Frey et al., 2003) but does not appear to impact negatively on seed germination (Faust, 1936) or seedling growth (Landhäusser et al., 2003).
On sandy sites, pines (likely mostly jack pine) increased their dominance, presumably following fires in the decades of 1910 and 1920 (Lefort et al., 2003), whereas aspen remained under-represented (Figure 3 and Supplementary Figure 2). Aspen under-representation could be the result of high fire severities on these surficial deposits. The quantity of organic matter consumed during a fire is effectively a function of soil moisture (Johnson, 1992). Fires occurring on these dry deposits could therefore consume the organic matter to greater depths and consequently damage the aspen root system, thus limiting its capacity to sucker (Horton and Hopkins, 1964; Wang, 2003). Stands dominated by jack pine appear to be more resistant to composition changes following fires (Chen et al., 2009).
Consequences for Forest Ecosystem Management
Forest ecosystem management aims to maintain the principal attributes of ecosystems within the limits of natural variability (Sustainable forest development act, SFDA 2010, A-18.1, c. 3, s. 4). In this context, considerable effort should be deployed to restore conifer dominance in the region. The frequency of intolerant hardwood-dominated mixedwoods and pure intolerant hardwood stands has increased between the pre-settlement and modern periods (from 9 to 26% and from 2 to 32%, respectively), whereas conifer-dominated mixedwoods decreased (from 52 to 21%). Moreover, particular attention should be paid to several taxa, particularly aspen which is probably in expansion (sensu Grondin and Cimon, 2003). Aspen’s current frequency and dominance appear to exceed observable proportions relative to natural dynamics, given the large area burned during the settlement period compared to the previous era (Bergeron et al., 2001), and the additive effect of forest harvesting on its increase in dominance (Weir and Johnson, 1998). In addition, while survey data did not allow distinction of black and white spruce, the reduction of white spruce, an ecological issue identified in Quebec’s forest ecosystem management framework (Jetté et al., 2013), likely occurred in the study area. In effect, white spruce was particularly sought after by the forest industry during settlement. Its decrease in frequency would have been markedly important on clays and tills because it is known to grow on productive mesic to sub-hydric deposits (Blanchard, 1949; Bergeron and Bouchard, 1984). Particular attention also should be paid to eastern white cedar whose frequency was halved between the pre-settlement and modern periods.
The fire cycle (i.e., time needed to burn an area equivalent to the studied territory) has lengthened considerably over recent decades in our study region (Bergeron et al., 2001), and few large fires have been observed since the 1940s (Lefort et al., 2003). Over the long term, this should favor the return toward fire-sensitive conifer dominance in certain stands (Bergeron, 2000), although transitional trajectories are highly variable (Bergeron et al., 2014). The elongation in fire cycle should, however, negatively affect jack pine recruitment, although the species has some capacity to maintain itself in the absence of fire via non-serotinous cones (Gauthier et al., 1993). Certain pure stands of aspen could also present a particular problem to conifer revival; during the summer field season of 2016 (data not presented), numerous aspen stands were noted to have no seedling or sapling conifer regeneration. In these stand types, the delay in the return to conifer dominance could be extremely long and (under-) planting may be necessary.
In the context of climate change, a return to pre-settlement forest composition could however, be unrealistic if climatic conditions and the disturbance regime change beyond the limits of natural variability (see Boulanger et al., 2019). In our study region, climate change will likely induce an increase in fire occurrence which, nonetheless, should not exceed the limits of natural variability between now and the end of the 21st century (Bergeron et al., 2010). Finally, reduction in the differences in composition with the pre-settlement forest still appears to be relevant, but considerable effort should be devoted to the functional restoration of these ecosystems (Boulanger et al., 2019), notably on the fertile deposits that have been particularly susceptible to composition changes.
Data Availability Statement
The datasets generated for this study are available on request to the corresponding author.
Author Contributions
YB and DA designed and supervised the project, critically reviewed the manuscript. MM collected and analyzed the data, interpreted the results, and drafted the manuscript. All authors read and approved the submitted version.
Funding
This project was financially supported by the Natural Sciences and Engineering Research Council of Canada (Grant number NSERC: 471469), by the Fonds de recherche du Québec nature et technologies (Grant number FQRNT: 188460), and by a practical field grant (BMP Innovation) in collaboration with the company Norbord Inc. and the lake Duparquet Research and teaching forest.
Conflict of Interest
The authors declare that this study received funding from the company Norbord Inc. The funder was not involved in the study design, collection, analysis, interpretation of data, the writing of this article or the decision to submit it for publication.
Acknowledgments
We thank Sébastien Dupuis for his help during data treatment, Alain Shink for providing information concerning aspen harvesting and management in Abitibi, Félix Guay for his support inside Norbord, Brian Harvey for comments the translation of this article and Victor Danneyrolles as well as Elias Ganivet for their help during data sampling.
Supplementary Material
The Supplementary Material for this article can be found online at: https://www.frontiersin.org/articles/10.3389/fevo.2020.00126/full#supplementary-material
References
Abrams, M. D., Orwig, D. A., and Demeo, T. E. (1995). Dendroecological analysis of successional dynamics for a presettlement-origin white-pine-mixed-oak forest in the southern Appalachians, USA. J. Ecol. 83, 123–133. doi: 10.2307/2261156
Anyomi, K. A., Raulier, F., Bergeron, Y., and Mailly, D. (2013). The predominance of stand composition and structure over direct climatic and site effects in explaining aspen (Populus tremuloides Michaux) site index within boreal and temperate forests of western Quebec, Canada. For. Ecol. Manage. 302, 390–403. doi: 10.1016/j.foreco.2013.03.035
Anyomi, K. A., Raulier, F., Bergeron, Y., Mailly, D., and Girardin, M. P. (2014). Spatial and temporal heterogeneity of forest site productivity drivers: a case study within the eastern boreal forests of Canada. Landsc. Ecol. 29, 905–918. doi: 10.1007/s10980-014-0026-y
Asselin, M. (1982). La Colonisation de l’Abitibi, «un projet géopolitique». Rouyn-Noranda: Collège du Nord-Ouest.
Baker, F. S. (1925). Aspen in the Central Rocky Mountain Region. Washington, DC: United States Department of Agriculture.
Baldwin, K. A., MacKenzie, W. H., Pfalz, J., Meades, W. J., Meidinger, D. V., Robitaille, A., et al. (2012). Level 4 map, version 1, Canadian Component of the Circumboreal Vegetation Map (CBVM). Canadian Regional Team of the Circumboreal Vegetation Map Project. Marie, ON: Natural Resources Canada.
Barnes, B. V. (1966). The clonal growth habit of American aspens. Ecology 47, 439–447. doi: 10.2307/1932983
Barrett, L. R., Liebens, J., Brown, D. G., Schaetzl, R. J., Zuwerink, P., Cate, T. W., et al. (1995). Relationships between soils and presettlement forests in Baraga County, Michigan. Am. Midl. Nat. 264–285. doi: 10.2307/2426297
Barrette, R. (1972). “Le plan Vautrin et l’Abitibi-Témiscamingue 1934-1936,” in L’Abbittibbi et le Témiskaming Hier et Aujourd’hui, eds M. Asselin and B. Beaudry Gourd (Rouyn: Université du Québec), 92–155.
Bartos, D., and Mueggler, W. (1982). Early succession following clearcutting of aspen communities in Northern Utah. J. Range Manage. 35, 764–768. doi: 10.2307/3898260
Bergeron, Y. (2000). Species and stand dynamics in the mixed woods of Quebec’s southern boreal forest. Ecology 81, 1500–1516.
Bergeron, Y., and Bouchard, A. (1984). Use of ecological groups in analysis and classification of plant communities in a section of western Quebec. Vegetatio 56, 45–63. doi: 10.1007/BF00036136
Bergeron, Y., Camiré, C., Bouchard, A., and Gangloff, P. (1982). Analyse et classification des sols pour une étude écologique intégrée d’un secteur de l’Abitibi, Québec. Géogr. Phys. Quat. 36, 291–305. doi: 10.7202/032482ar
Bergeron, Y., and Charron, D. (1994). Postfire stand dynamics in a southern boreal forest (Québec): a dendroecological approach. Ecoscience 1, 173–184. doi: 10.1080/11956860.1994.11682241
Bergeron, Y., Chen, H. Y., Kenkel, N. C., Leduc, A. L., and Macdonald, S. E. (2014). Boreal mixedwood stand dynamics: ecological processes underlying multiple pathways. For. Chron. 90, 202–213. doi: 10.5558/tfc2014-039
Bergeron, Y., Cyr, D., Girardin, M. P., and Carcaillet, C. (2010). Will climate change drive 21st century burn rates in Canadian boreal forest outside of its natural variability: collating global climate model experiments with sedimentary charcoal data. Int. J. Wildl. Fire 19, 1127–1139. doi: 10.1071/WF09092
Bergeron, Y., and Dubuc, M. (1989). Succession in the southern part of the Canadian boreal forest. Vegetatio 79, 51–63. doi: 10.1007/BF00044848
Bergeron, Y., Gauthier, S., Kafka, V., Lefort, P., and Lesieur, D. (2001). Natural fire frequency for the eastern Canadian boreal forest: consequences for sustainable forestry. Can. J. For. Res. 31, 384–391. doi: 10.1139/x00-178
Blanchard, R. (1949). “Etudes canadiennes (Troisième série). IV, L’Abitibi-Témiscamingue” in Revue de Géographie Alpine (n°3: tome 37), eds R. Blanchard and P. Veyret (Grenoble, FR: Institut de Géographie Alpine, Université de Grenoble), 421–551.
Blanchard, R. (1954). L’Ouest du Canada français: Les Pays de l’Ottawa,” L’Abitibi-Témiscamingue”. Montréal, QC: Librairie Beauchemin.
Blouin, J., and Berger, J. (2002). Guide de Reconnaissance des Types Écologiques de la Région Écologique 5a - Plaine de l’Abitibi. Québec, QC: Ministère des Ressources Naturelles du Québec.
Boucher, Y., Auger, I., Noël, J., Grondin, P., and Arseneault, D. (2016). Fire is a stronger driver of forest composition than logging in the boreal forest of eastern Canada. J. Veg. Sci. 28, 57–68. doi: 10.1111/jvs.12466
Boudreau, J., and Philibert, Y. (2011). Normes D’inventaire Ecoforestier, Placettes-Echantillons Permanentes. Québec, QC: Ministère des Forêts de la Faune et des Parcs.
Boulanger, Y., Arseneault, D., Boucher, Y., Gauthier, S., Cyr, D., Taylor, A. R., et al. (2019). Climate change will affect the ability of forest management to reduce gaps between current and presettlement forest composition in southeastern Canada. Landsc. Ecol. 34, 159–174. doi: 10.1007/s10980-018-0761-6
Bourdo, E. A. (1956). A review of the General Land Office survey and of its use in quantitative studies of former forests. Ecology 37, 754–768.
Brassard, B. W., and Chen, H. Y. (2006). Stand structural dynamics of North American boreal forests. Crit. Rev. Plant Sci. 25, 115–137. doi: 10.1080/07352680500348857
Canham, C. D., and Loucks, O. L. (1984). Catastrophic windthrow in the presettlement forests of Wisconsin. Ecology 803–809. doi: 10.2307/1938053
Cardona, L. (2014). Dynamique des Peuplements de Peuplier Faux-Tremble dans la Forêt Boréale de L’ouest du Québec. Québec, QC: Université Laval.
Chen, H. Y., Vasiliauskas, S., Kayahara, G. J., and Ilisson, T. (2009). Wildfire promotes broadleaves and species mixture in boreal forest. For. Ecol. Manage. 257, 343–350. doi: 10.1016/j.foreco.2008.09.022
Danneyrolles, V., Arseneault, D., and Bergeron, Y. (2016). Pre-industrial landscape composition patterns and post-industrial changes at the temperate–boreal forest interface in western Quebec, Canada. J. Veg. Sci. 27, 470–481. doi: 10.1111/jvs.12373
Dobbs, R. (1976). White spruce seed dispersal in central British Columbia. For. Chron. 52, 225–228. doi: 10.5558/tfc52225-5
Doucet, R. (1979). Méthodes de coupe et de préparation du terrain pour favoriser la régénération naturelle de quelques tremblaies de l’est du Québec. For. Chron. 55, 133–136. doi: 10.5558/tfc55133-4
Dupuis, S., Arseneault, D., and Sirois, L. (2011). Change from pre-settlement to present-day forest composition reconstructed from early land survey records in eastern Québec, Canada. J. Veg. Sci. 22, 564–575. doi: 10.1111/j.1654-1103.2011.01282.x
Ecoregions Working Group (1989). Ecoclimatic Regions of Canada: First Approximation. Ecological Land Classification Series, No. 23. Ottawa: Environment Canada.
Egan, D., and Howell, E. A. (2001). The Historical Ecology Handbook : A Restorationist’s Guide to Reference Ecosystems. Washington, DC: Island Press.
Environment Canada (2019). Normales climatiques canadiennes: Normales et moyennes climatiques de 1981-2010. Service Météorologique du Canada. Available online at: http://climat.meteo.gc.ca/climate_normals/index_f.htm (accessed septembre 16, 2019).
Faust, M. E. (1936). Germination of Populus grandidentata and P. tremuloides, with particular reference to oxygen consumption. Bot. Gaz. 97, 808–821. doi: 10.1086/334605
Fortin, G. (2018). Transformation de la Composition de la Forêt de la Péninsule Gaspésienne au Cours du XXe siècle. Ph. D. thesis, Université du Québec à Rimouski, Rimouski, QC.
Fortin, S. (2008). Expansion Postcoloniale du Tremble (Populus tremuloides) dans le Bassin de la Rivière York, en Gaspésie. Ph. D. thesis, Université du Québec à Chicoutimi, Chicoutimi, QC.
Foster, D. R., Motzkin, G., and Slater, B. (1998). Land-use history as long-term broad-scale disturbance: regional forest dynamics in central New England. Ecosystems 1, 96–119. doi: 10.1007/s100219900008
Foster, D. R., Orwig, D., and McLachlan, J. (1996). Ecological and conservation insights from reconstructive studies of temperate old-growth forests. Trends Ecol. Evol. 11, 419–424. doi: 10.1016/0169-5347(96)10047-1
Frey, B. R., Lieffers, V. J., Landhäusser, S. M., Comeau, P. G., and Greenway, K. J. (2003). An analysis of sucker regeneration of trembling aspen. Can. J. For. Res. 33, 1169–1179. doi: 10.1139/x03-053
Friedman, S. K., and Reich, P. B. (2005). Regional legacies of logging: departure from presettlement forest conditions in northern Minnesota. Ecol. Appl. 15, 726–744. doi: 10.1890/04-0748
Galipeau, C., Kneeshaw, D. D., and Bergeron, Y. (1997). White spruce and balsam fir colonization of a site in the southeastern boreal forest as observed 68 years after fire. Can. J. For. Res. 27, 139–147. doi: 10.1139/x96-148
Gauthier, S., De Grandpré, L., and Bergeron, Y. (2000). Differences in forest composition in two boreal forest ecoregions of Quebec. J. Veg. Sci. 11, 781–790. doi: 10.2307/3236548
Gauthier, S., Gagnon, J., and Bergeron, Y. (1993). Population age structure of Pinus banksiana at the southern edge of the Canadian boreal forest. J. Veg. Sci. 4, 783–790. doi: 10.2307/3235615
Gewehr, S., Drobyshev, I., Berninger, F., and Bergeron, Y. (2014). Soil characteristics mediate the distribution and response of boreal trees to climatic variability. Can. J. For. Res. 44, 487–498. doi: 10.1139/cjfr-2013-0481
Graham, S. A., Harrison, R. P. Jr., and Westell, C. E. Jr. (1963). Aspens, Phoenix Trees of the Great Lakes Region. Ann Arbor, MI: University of Michigan Press.
Greene, D., and Johnson, E. (1999). Modelling recruitment of Populus tremuloides, Pinus banksiana, and Picea mariana following fire in the mixedwood boreal forest. Can. J. For. Res. 29, 462–473. doi: 10.1139/x98-211
Greene, D. F., Macdonald, S. E., Haeussler, S., Domenicano, S., Noel, J., Jayen, K., et al. (2007). The reduction of organic-layer depth by wildfire in the North American boreal forest and its effect on tree recruitment by seed. Can. J. For. Res. 37, 1012–1023. doi: 10.1139/X06-245
Greene, D. F., Zasada, J. C., Sirois, L., Kneeshaw, D., Morin, H., Charron, I., et al. (1999). A review of the regeneration dynamics of North American boreal forest tree species. Can. J. For. Res. 29, 824–839. doi: 10.1139/x98-112
Grondin, P., and Cimon, A. (2003). Les Enjeux de Biodiversité Relatifs à la Composition Forestière. Québec, QC: Gouvernement du Québec.
Gutsell, S., and Johnson, E. (2002). Accurately ageing trees and examining their height-growth rates: implications for interpreting forest dynamics. J. Ecol. 90, 153–166. doi: 10.1046/j.0022-0477.2001.00646.x
Haberman, S. J. (1973). The analysis of residuals in cross-classified tables. Biometrics 29, 205–220. doi: 10.2307/2529686
Hanberry, B. B., Palik, B. J., and He, H. S. (2012). Comparison of historical and current forest surveys for detection of homogenization and mesophication of Minnesota forests. Landsc. Ecol. 27, 1495–1512. doi: 10.1007/s10980-012-9805-5
Harvey, B. D., and Bergeron, Y. (1989). Site patterns of natural regeneration following clear-cutting in northwestern Quebec. Can. J. For. Res. 19, 1458–1469. doi: 10.1139/x89-222
Ilisson, T., and Chen, H. Y. (2009). The direct regeneration hypothesis in northern forests. J. Veg. Sci. 20, 735–744. doi: 10.1111/j.1654-1103.2009.01066.x
Jackson, S. M., Pinto, F., Malcolm, J. R., and Wilson, E. R. (2000). A comparison of pre-European settlement (1857) and current (1981-1995) forest composition in central Ontario. Can. J. For. Res. 30, 605–612. doi: 10.1139/x99-242
Jetté, J., Leblanc, M., Bouchard, M., and Villeneuve, N. (2013). Intégration des Enjeux Écologiques dans les Plans D’aménagement Forestier Intégré, Partie I—Analyse des Enjeux. Québec, QC: Gouvernement du Québec.
Johnson, E. A. (1992). Fire and Vegetation Dynamics: Studies from the North American Boreal Forest. Cambridge: Cambridge University Press.
Johnstone, J. F., and Chapin, F. S. III (2006). Effects of soil burn severity on post-fire tree recruitment in boreal forest. Ecosystems 9, 14–31. doi: 10.1007/s10021-004-0042-x
Johnstone, J. F., Chapin Iii, F., Foote, J., Kemmett, S., Price, K., and Viereck, L. (2004). Decadal observations of tree regeneration following fire in boreal forests. Can. J. For. Res. 34, 267–273. doi: 10.1139/x03-183
Kay, C. E. (1993). Aspen seedlings in recently burned areas of Grand Teton and Yellowstone National Parks. Northwest Sci. 67, 94–104.
Lafleur, B., Cazal, A., Leduc, A., and Bergeron, Y. (2015). Soil organic layer thickness influences the establishment and growth of trembling aspen (Populus tremuloides) in boreal forests. For. Ecol. Manage. 347, 209–216. doi: 10.1016/j.foreco.2015.03.031
Landhäusser, S. M., Deshaies, D., and Lieffers, V. J. (2010). Disturbance facilitates rapid range expansion of aspen into higher elevations of the Rocky Mountains under a warming climate. J. Biogeogr. 37, 68–76. doi: 10.1111/j.1365-2699.2009.02182.x
Landhäusser, S. M., DesRochers, A., and Lieffers, V. J. (2001). A comparison of growth and physiology in Picea glauca and Populus tremuloides at different soil temperatures. Can. J. For. Res. 31, 1922–1929. doi: 10.1139/x01-129
Landhäusser, S. M., and Lieffers, V. J. (1998). Growth of Populus tremuloides in association with Calamagrostis canadensis. Can. J. For. Res. 28, 396–401. doi: 10.1139/x98-006
Landhäusser, S. M., Lieffers, V. J., and Mulak, T. (2006). Effects of soil temperature and time of decapitation on sucker initiation of intact Populus tremuloides root systems. Scand. J. For. Res. 21, 299–305. doi: 10.1080/02827580600813313
Landhäusser, S. M., Silins, U., Lieffers, V. J., and Liu, W. (2003). Response of Populus tremuloides, Populus balsamifera, Betula papyrifera and Picea glauca seedlings to low soil temperature and water-logged soil conditions. Scand. J. For. Res. 18, 391–400. doi: 10.1080/02827580310015044
Laquerre, S., Leduc, A., and Harvey, B. D. (2009). Augmentation du couvert en peuplier faux-tremble dans les pessières noires du nord-ouest du Québec après coupe totale. Ecoscience 16, 483–491. doi: 10.2980/16-4-3252
Lavertu, D., Mauffette, Y., and Bergeron, Y. (1994). Effects of stand age and litter removal on the regeneration of Populus tremuloides. J. Veg. Sci. 5, 561–568. doi: 10.2307/3235983
Lefort, P., Gauthier, S., and Bergeron, Y. (2003). The influence of fire weather and land use on the fire activity of the Lake Abitibi area, eastern Canada. For. Sci. 49, 509–521. doi: 10.1093/forestscience/49.4.509
Lord, G., Faucher, A., and Berger, J.-P. (2009). Normes de Cartographie Écoforestière: Troisième Inventaire Écoforestier. Québec, QC: Gouvernement du Québec.
Lorimer, C. G. (2001). Historical and ecological roles of disturbance in eastern North American forests: 9, 000 years of change. Wildl. Soc. Bull. 29, 425–439.
Mansuy, N., Gauthier, S., Robitaille, A., and Bergeron, Y. (2010). The effects of surficial deposit–drainage combinations on spatial variations of fire cycles in the boreal forest of eastern Canada. Int. J. Wildl. Fire 19, 1083–1098. doi: 10.1071/WF09144
Morgan, P., Aplet, G. H., Haufler, J. B., Humphries, H. C., Moore, M. M., and Wilson, W. D. (1994). Historical range of variability: a useful tool for evaluating ecosystem change. J. Sustaina. For. 2, 87–111. doi: 10.1300/J091v02n01_04
Nowacki, G. J., and Abrams, M. D. (2015). Is climate an important driver of post-European vegetation change in the Eastern United States? Glob. Change Biol. 21, 314–334. doi: 10.1111/gcb.12663
Nowak, D. J. (1993). Historical vegetation change in Oakland and its implications for urban forest management. J. Arboric. 19, 313–319.
Perala, D. A. (1990). “Populus tremuloides Michx. quaking aspen,” in Silvics of North America: 2. Hardwoods, eds R. M. Burns and B. H. Honkala (Washington, DC: United States Department of Agriculture), 555–569.
Peters, V. S., Macdonald, S. E., and Dale, M. R. (2005). The interaction between masting and fire is key to white spruce regeneration. Ecology 86, 1744–1750. doi: 10.1890/03-0656
Peterson, E., and Peterson, N. (1992). Ecology, Management, and Use of Aspen and Balsam Poplar in the Prairie Provinces. Edmonton: Forestry Canada.
Pinto, F., Romaniuk, S., and Ferguson, M. (2008). Changes to preindustrial forest tree composition in central and northeastern Ontario, Canada. Can. J. For. Res. 38, 1842–1854. doi: 10.1139/X08-034
Portier, J., Gauthier, S., and Bergeron, Y. (2019). Spatial distribution of mean fire size and occurrence in eastern Canada: influence of climate, physical environment and lightning strike density. Int. J. Wildl. Fire 28, 927–940. doi: 10.1071/WF18220
Purdy, B. G., Macdonald, S. E., and Dale, M. R. (2002). The regeneration niche of white spruce following fire in the mixedwood boreal forest. Silva Fenn. 36, 289–306. doi: 10.7939/R3BK16W29
Quinn, R. D., and Wu, L. (2001). “Quaking aspen reproduce from seed after wildfire in the mountains of southeastern Arizona,” in Sustaining Aspen in Western Landscapes: Symposium Proceedings, eds W. D. Shepperd, D. Binkley, D. L. Bartos, D. J. Stohlgren, and L. G. Eskew (Grand Junction, CO: United States Department of Agriculture).
Romme, W. H., Turner, M. G., Tuskan, G. A., and Reed, R. A. (2005). Establishment, persistence, and growth of aspen (Populus tremuloides) seedlings in Yellowstone National Park. Ecology 86, 404–418.
Royer-Tardif, S., and Bradley, R. L. (2011). Evidence that soil fertility controls the mixing of jack pine with trembling aspen. For. Ecol. Manage. 262, 1054–1060.
Saucier, J., Grondin, P., Robitaille, A., Gosselin, J., Morneau, C., Richard, P., et al. (2009). “Écologie forestière - Chapitre 4,” in Manuel de Foresterie, eds Ordre des Ingénieurs Forestiers du Québec (Québec, QC: Éditions MultiMondes), 165–315.
Schier, G. A., Jones, J. R., and Winokur, R. P. (1985). “Vegetative regeneration,” in Aspen Ecology and Management in the Western United States, eds N. V. DeByle and R. P. Winokur (Fort Collins, CO: United States Department of Agriculture), 29–33.
Schulte, L. A., Mladenoff, D. J., Crow, T. R., Merrick, L. C., and Cleland, D. T. (2007). Homogenization of northern US Great Lakes forests due to land use. Landsc. Ecol. 22, 1089–1103.
Scull, P., and Richardson, J. L. (2007). A method to use ranked timber observations to perform forest composition reconstructions from land survey data. Am. Midl. Nat. 158, 446–460.
Simard, M., Lecomte, N., Bergeron, Y., Bernier, P. Y., and Paré, D. (2007). Forest productivity decline caused by successional paludification of boreal soils. Ecol. Appl. 17, 1619–1637. doi: 10.1890/06-1795.1
Sims, R., Kershaw, H. M., and Wickware, G. (1990). The Autecology of Major Tree Species in the North Central Region of Ontario. Sault Ste. Marie, ON: Forestry Canada.
Stahelin, R. (1943). Factors influencing the natural restocking of high altitude burns by coniferous trees in the central Rocky Mountains. Ecology 24, 19–30. doi: 10.2307/1929857
Statistique Canada (2015). Les Autochtones : Rapport spécial de la chambre des communes, 1857. Recensements du Canada 1665 à 1871. Available online at: https://www150.statcan.gc.ca/n1/pub/98-187-x/4151278-fra.htm (accessed septembre 16, 2019).
Strahler, A. H. (1978). Binary discriminant analysis: a new method for investigating species-environment relationships. Ecology 59, 108–116. doi: 10.2307/1936636
Swetnam, T. W., Allen, C. D., and Betancourt, J. L. (1999). Applied historical ecology: using the past to manage for the future. Ecol. Appl. 9, 1189–1206.
Terrail, R., Arseneault, D., Fortin, M. J., Dupuis, S., and Boucher, Y. (2014). An early forest inventory indicates high accuracy of forest composition data in pre-settlement land survey records. J. Veg. Sci. 25, 691–702. doi: 10.1111/jvs.12142
Thibaudeau, P., and Veillette, J. (2005). Géologie des Formations en Surface et Histoire Glaciaire. Lac Chicobi, QC: Commission géologique du Canada.
Turner, M. G., Romme, W. H., Reed, R. A., and Tuskan, G. A. (2003). Post-fire aspen seedling recruitment across the Yellowstone (USA) landscape. Landsc. Ecol. 18, 127–140. doi: 10.1023/A:1024462501689
Van Cleve, K., Dyrness, C., Viereck, L., Fox, J., Chapin, F. III, and Oechel, W. (1983). Taiga ecosystems in interior Alaska. Bioscience 33, 39–44. doi: 10.2307/1309243
Veillette, J. (1994). Evolution and paleohydrology of glacial lakes Barlow and Ojibway. Quat. Sci. Rev. 13, 945–971. doi: 10.1016/0277-3791(94)90010-8
Vincent, O. F. (1995). Histoire de l’Abitibi-Témiscamingue. Québec, QC: Institut québécois de recherche sur la culture.
Wan, X., Landhäusser, S. M., Zwiazek, J. J., and Lieffers, V. J. (1999). Root water flow and growth of aspen (Populus tremuloides) at low root temperatures. Tree Physiol. 19, 879–884. doi: 10.1093/treephys/19.13.879
Wan, X., Zwiazek, J. J., Lieffers, V. J., and Landhäusser, S. M. (2001). Hydraulic conductance in aspen (Populus tremuloides) seedlings exposed to low root temperatures. Tree Physiol. 21, 691–696. doi: 10.1093/treephys/21.10.691
Wang, G. G. (2003). Early regeneration and growth dynamics of Populus tremuloides suckers in relation to fire severity. Can. J. For. Res. 33, 1998–2006. doi: 10.1139/x03-123
Weir, J., and Johnson, E. (1998). Effects of escaped settlement fires and logging on forest composition in the mixedwood boreal forest. Can. J. For. Res. 28, 459–467. doi: 10.1139/x98-001
White, M. A., and Mladenoff, D. J. (1994). Old-growth forest landscape transitions from pre-European settlement to present. Landsc. Ecol. 9, 191–205. doi: 10.1007/BF00134747
Whitney, G. G. (1982). Vegetation-site relationships in the presettlement forests of northeastern Ohio. Bot. Gaz. 143, 225–237. doi: 10.1086/337294
Whitney, G. G. (1986). Relation of Michigan’s presettlement pine forests to substrate and disturbance history. Ecology 67, 1548–1559. doi: 10.2307/1939085
Whitney, G. G. (1994). From Coastal Wilderness to Fruited Plain: A History of Environmental Change in Temperate North America from 1500 to the Present. Cambridge: Cambridge University Press.
Keywords: early land surveys, Euro-Canadian settlement, historical ecology, surficial deposits, pre-settlement forest, mixedwood boreal forest, Picea spp., Populus tremuloides
Citation: Marchais M, Arseneault D and Bergeron Y (2020) Composition Changes in the Boreal Mixedwood Forest of Western Quebec Since Euro-Canadian Settlement. Front. Ecol. Evol. 8:126. doi: 10.3389/fevo.2020.00126
Received: 17 January 2020; Accepted: 17 April 2020;
Published: 15 May 2020.
Edited by:
Tuomas Aakala, University of Helsinki, FinlandReviewed by:
James Dyer, Ohio University, United StatesGregory Jay Nowacki, United States Forest Service (USDA), United States
Copyright © 2020 Marchais, Arseneault and Bergeron. This is an open-access article distributed under the terms of the Creative Commons Attribution License (CC BY). The use, distribution or reproduction in other forums is permitted, provided the original author(s) and the copyright owner(s) are credited and that the original publication in this journal is cited, in accordance with accepted academic practice. No use, distribution or reproduction is permitted which does not comply with these terms.
*Correspondence: Mathilde Marchais, Mathilde.Marchais@uqat.ca