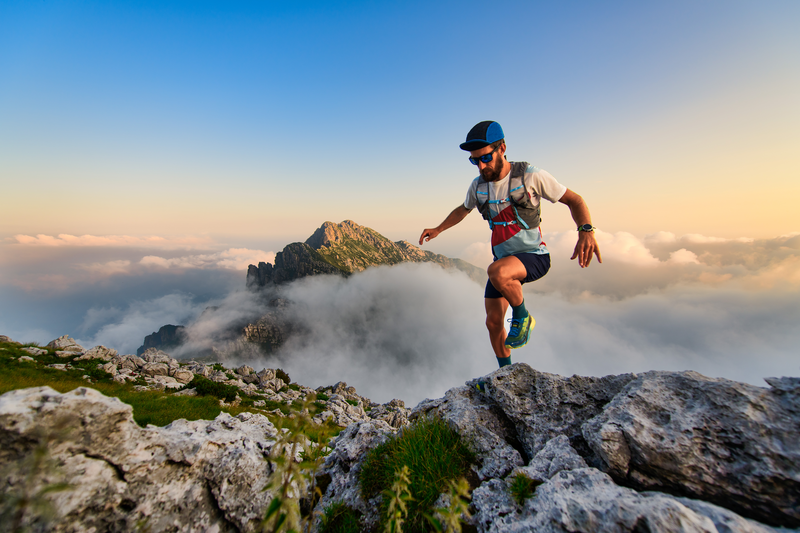
94% of researchers rate our articles as excellent or good
Learn more about the work of our research integrity team to safeguard the quality of each article we publish.
Find out more
ORIGINAL RESEARCH article
Front. Ecol. Evol. , 05 May 2020
Sec. Behavioral and Evolutionary Ecology
Volume 8 - 2020 | https://doi.org/10.3389/fevo.2020.00107
This article is part of the Research Topic Advances in Ungulate Ecology View all 22 articles
How animals respond to a changing environment is a key question in ecological research. Animals living at higher latitudes are exposed to pronounced seasonal differences in both climate and in resource availability. Endotherms living in those environments have the ability to maintain a constant high body temperature (Tb), over a wide range of ambient temperatures (Ta). Nonetheless, many endotherms display seasonal shifts in metabolic rate (MR). Here, we studied the annual and circadian cycle of Tb and heart rate (HR) in female moose (Alces alces) in relation to activity and Ta. HR also can be used as a proxy of MR to calculate energy budgets. We deployed biologgers to 12 free-ranging female moose; a temperature sensor in the rumen, a HR logger subcutaneously, and a GPS collar equipped with acceleration and Ta sensors. We documented seasonal differences in Tb, HR and activity of moose, with lower levels during winter and higher values during summer. The highest daily mean Tb and HR were 38.64°C (10 July) and 71.9 beats per minute (bpm; 26 June), whereas the lowest daily mean Tb and HR were 38.03°C (17 March) and 40.5 bpm (6 March). High-resolution Tb and activity data allowed us to detect circadian and ultradian rhythmicity throughout the year. Based on previous calibration studies, MR decreased by 60% from the highest to the lowest point. Our results demonstrate hypometabolism including lower Tb and HR during winter as a strategy to reduce energy expenditure during periods with colder climate and limited availability of resources.
Animals living in environments with ambient temperatures (Ta) below freezing are exposed to pronounced seasonal differences in both climate and resource availability. Nevertheless, endotherms living in these environments can maintain a constant high body temperature (Tb), over a wide range of Ta. Maintaining homeostasis in cold temperatures with limited food availability is energetically costly, and endotherms have adapted to seasonal differences through several strategies including increased insulation, hibernation, seasonal hypometabolism, and daily torpor (Geiser, 2004; Heldmaier et al., 2004; Lovegrove, 2005; Boyles et al., 2011). Hibernation in mammals usually lasts for months, during which animals rely on energy stores and reduce Tb and metabolic rate (MR). The basal MR for hibernating mammals is on average reduced by about 95% during hibernation (Geiser, 2004; Heldmaier et al., 2004; Ruf and Geiser, 2015).
A reduction in MR hypometabolism, is not limited to organisms entering hibernation or torpor, as other endotherms also can exhibit hypometabolism. The reason for entering this stage is not only to adapt to a colder climate, but also to cope with limited energy supplies as evidenced by endotherms in tropical climates (Heldmaier et al., 2004). Seasonal variations of MR, with hypometabolism during winter, occur in a variety of both captive and free-ranging ungulates living in temperate climates, including moose (Alces alces; Regelin et al., 1985; Kochan, 2007), red deer (Cervus elaphus; Arnold et al., 2004; Turbill et al., 2011), Przewalski horse (Equus ferus przewalskii; Arnold et al., 2006), Svalbard reindeer (Rangifer tarandus platyrhynchus; Arnold et al., 2018), alpine ibex (Capra ibex ibex; Signer et al., 2011), and llama (Lama glama; Riek et al., 2019).
Circadian variations of MR also are reported in ungulates, e.g., as episodes of nocturnal hypometabolism independent of activity levels in alpine ibex and red deer (Arnold et al., 2004; Signer et al., 2011). A circadian rhythm is a biological process that displays an endogenous oscillation of about 24 h. Circadian organization in the activity pattern of different ungulate species have been reported previously (Arnold et al., 2004; Lowe et al., 2010; Signer et al., 2011; Pagon et al., 2013; Ensing et al., 2014). Studies of reindeer (Rangifer tarandus tarandus) living above the Arctic Circle, including animals on the Svalbard archipelago, reported only weak circadian organization of activity during constant darkness or light (Van Oort et al., 2005; van Oort et al., 2007). A more recent study of Svalbard reindeer, using biologgers, reported circadian rhythmicity in behavior, HR and Tb (Arnold et al., 2018). Circadian rhythmicity in moose could be caused by some combined adaptations to predator and/or human activity, Ta, light-dark cycles, food availability, feeding, and rumination (Van Ballenberghe and Miquelle, 1990; Gillingham and Klein, 1992; Scheibe et al., 1999; Klassen and Rea, 2008).
Several mammal species exhibit a significant correlation between heart rate (HR) and MR, which is attributed to the correlation between the rate of oxygen consumption (VO2) and HR, when animals are in a metabolic steady state with aerobic metabolism (Green, 2011). Because of the correlation between HR and MR, HR measurements make it possible to calculate energy expenditures of animals under free-ranging circumstances (Renecker and Hudson, 1985). Calculations of energy expenditure are important when discussing potential stressful situations, especially in capital breeders (i.e., organisms relying on stored resources for reproduction) such as moose because increased MR could result in weight loss due to increased energy consumption and decreased time spent foraging. This relationship could further result in lower survival and reproduction rates.
Biologgers have made it possible to obtain data for characterization of the physiology of an animal in their environmental setting, and their reactions to humans over long periods of time (Rutz and Hays, 2009). In this study we used ruminal implants to obtain Tb. Ruminal and vaginal transmitters have previously been used in long-term (>1 year) studies of moose, and rumen temperature successfully predicts core Tb when censoring and filtering out drinking events (Herberg et al., 2018; Thompson et al., 2019). The few studies on moose using biologgers to monitor HR are short-term (weeks-months), usually with a limited number of animals in semi-captive conditions (Renecker and Hudson, 1985; Langvatn, 1992; Roshchevsky et al., 1999). In this study, we used the same type of HR loggers, as previously deployed in a variety of large mammals including domestic sheep (Ovis aries; Fuchs et al., 2019a), and Asiatic black bears (Ursus thibetanus) and Eurasian brown bears (Ursus arctos; Fuchs et al., 2019b).
There are relatively few long-term studies using biologgers that reports simultaneous measurements of Tb and HR in ungulates living in harsh climates (Arnold et al., 2006; Signer et al., 2011; Turbill et al., 2011; Arnold et al., 2018; Riek et al., 2019). Similar studies of moose are important, because similar to reindeer and caribou (Rangifer tarandus spp.), moose are a key herbivore in northern ecosystems that are ecologically, economically, and culturally important. Currently, we still lack knowledge on the physiological ability of moose to respond to ambient temperatures that are likely to come with changing climatic conditions. Previous studies have shown that moose are easily heat stressed with respiration rate, HR and energy expenditure rising with increasing Ta (Renecker and Hudson, 1986; Roshchevsky et al., 1999; McCann et al., 2013). In captive moose the respiration rate started to increase at 14°C and at 20°C they began open-mouthed panting (Renecker and Hudson, 1986). Thompson et al. (2019) suggested several other factors that also should be considered, including core Tb and daily variations of core Tb, in addition to the influence of body condition and season, when evaluating heat stress. Climatic changes resulting in increased Ta can be expected to result in habitat changes, decreased food availability and, therefore, poorer body condition (van Beest et al., 2012; van Beest and Milner, 2013).
In the present study, we used biologgers and global positioning system (GPS) collars to study the annual and circadian rhythms of HR and Tb, in relation to activity and Ta, in free-ranging female moose in Sweden. The obtained values and patterns are relevant background for evaluating the behavioral and physiological effects of potential stressors. Moreover, because HR can be a proxy for MR, values of HR also can be used to calculate energy budgets.
We hypothesized that moose would exhibit physiological and behavioral changes related to the dramatic seasonal differences present at northern latitudes. Firstly, we predicted lower Tb and HR in winter (Regelin et al., 1985; Langvatn, 1992; Kochan, 2007; Thompson et al., 2019). Secondly, we predicted a circadian rhythmicity in the HR, Tb, and activity of moose, as previously described in ungulates because of adaptations to feeding and rumination or environmental factors such as food availability, Ta and light-dark cycles, predation, and human activities (Scheibe et al., 1999; Arnold et al., 2004; Lowe et al., 2010; Signer et al., 2011; Pagon et al., 2013; Ensing et al., 2014). We also expected a change in these rhythms over the year (Klassen and Rea, 2008; Arnold et al., 2018). Thirdly, we predicted Tb to increase with increasing Ta because of the poor tolerance of moose to heat-stress (Renecker and Hudson, 1986; McCann et al., 2013; Thompson et al., 2019).
The study was conducted in the Nordmaling municipality (63°34′00′′ N, 19°30′00′′ E) in the county of Västerbotten in Sweden. The study area is characterized by boreal forests dominated by Scots pine (Pinus silvestris), Norway spruce (Picea abies) and birches (Betula pendula and B. pubescens). Yearly average Ta is 3°C and there are >150 days with snow cover from November to May. Mean and maximum daily snow depth from 1 November to 30 April was 0.15 and 0.5 m in 2016–2017 and 0.53 and 0.98 m in 2017–2018 (SMHI, 2019). The length of the growing season is between 150 and 160 days from late April to mid-October (SMHI, 2019).
We immobilized 12 female moose (>1.5 years old) in February 2017. The project was approved by the Animal Care Committee for Northern Sweden in Umeå (Dnr A3/2016 2016–02-26) and was conducted in accordance with Swedish laws concerning animal research ethics. Experienced veterinarians, pilots and field personnel were responsible for captures, monitoring, handling, collaring and surgeries. All personnel were trained and certified according to the standards of the Swedish Animal Welfare Agency and the Swedish Board of Agriculture.
We fitted moose with collars including a GPS receiver, a temperature recorder, an activity sensor, a mortality sensor, a very high frequency (VHF) transmitter, and a Global System for Mobile communication (GSM) modem (Vectronic-Aerospace, Berlin, Germany). Activity sensors measured acceleration in three orthogonal directions six to eight times per second. For each direction activity values were averaged for a recording interval of 5 min. To present overall activity, values of two of the three orthogonal directions (x and y) were summed, resulting in values ranging from 0–510, with 0 representing no activity and 510 highest activity (Gervasi et al., 2006). The GPS was scheduled to record positions from 1 min to 3 h depending on the ongoing studies, and together with the recorded Ta (every 5 min), those readings were sent using the GSM network to WRAM (Wireless Remote Animal Movement) database for storage (Dettki et al., 2014). Moose also were equipped with a mortality implant transmitter (MIT, Vectronic Aerospace GmbH, Berlin, Germany) in their rumen, which recorded Tb at 5-min intervals and then transmitted information to a collar unit, where it was archived. The MIT is a cylindrical device with a diameter of 21 mm, length 72 mm, and weight ca 100 g (VectronicAerospace, 2017). The most recently stored Tb was sent together with the GPS position message and the remaining data downloaded upon collar retrieval.
Additionally, we fitted moose with subcutaneous HR loggers (DST centi HRT; Star Oddi, Gardabaer, Iceland), which can simultaneously record long-term HR and subcutaneous Tb. The DST centi HRT is a cylindrical device with dimensions of 46 × 15 mm and weighs 19 grams. HR is automatically calculated from a 4 s electrocardiogram (ECG) strip with a 150 Hz measurement frequency and stored along with a quality index of signal clarity and the R-R interval regularity. The logger can store up to 699,051 HR and temperature measurements or 2,785 ECG buffer measurements and, according to the manufacturer, has a battery life of up to 19 month (StarOddi, 2017). We programmed the DST centi HRTs to record HR every second hour from the beginning of the study until 6 August; thereafter, they were programmed to record HR every second minute.
We immobilized moose from a helicopter using a CO2 powered rifle (Dan-Inject, Børkop, Denmark) with the drug combination of 50 mg xylazine (Rompun Dry Substance, Bayer AG, Leverkusen, Germany) and 4.5 mg etorphine (Etorphine® HCl 9.8 mg/mL, Vericore Veterinary Products, Novartis Animal Health UK Ltd., Litlington, United Kingdom), according to previously described procedures (Evans et al., 2012; Lian et al., 2014). Pregnancy status was determined by rectal palpation (Solberg et al., 2003). Number of offspring (zero, single or twins) was verified the following spring by field observations. Biologgers were sterilized with ethylene oxide gas (Anprolene AN74i 60 L, Andersen Europe, Kortrijk, Belgium) before surgical implantation. Prior to surgery, moose were given analgesics, meloxicam (Metacam®, Boehringer Ingelheim Vetmedica GmBH, Ingelheim am Rhein, Germany) subcutaneously at a dose of 0.5 mg/kg. The DST centi HRT was implanted surgically at the right side of the most rostral part of the sternum, with the moose placed in lateral recumbency with the left side up. A cell phone device (KardiaMobile EKG Monitor, AliveCor Inc., Mountain View, CA, United States) and a corresponding application Kardia App (AliveCor Inc.; AliveCor, 2018), were used to find the best ECG signal, and the most appropriate site to implant the DST centi HRT. Surgery was performed according to best-practice guidelines (Fiorello et al., 2016) and a local anesthetic, bupivacaine (Marcain 5 mg/mL, AstraZeneca, Cambridge, United Kingdom), was administered at a total dose of 2.0–3.0 mg/moose. A 2-cm long skin incision was made and the DST centi HRT was placed between the subcutaneous fat and the muscle with the electrodes facing away from the muscle. The incision was closed with 2-0 monofilament absorbable suture PDS® II (polydioxanone) suture (Ethicon, Johnson & Johnson, New Brunswick, NJ, United States). After surgical implantation, we reversed the effects of xylazine with 5 mg atipamezole (Antisedan® 5 mg/mL, Orion Pharma Animal Health, Turku, Finland) injected intramuscularly or intravenously, to inhibit a swallowing reflex. We deployed MIT as previously described (Minicucci et al., 2018), before we reversed the immobilization with 50 mg naltrexone (Naltrexonhydroklorid vet. APL 50 mg/mL; Apotek Produktion och Laboratorier, Kungens Kurva, Sweden) intramuscularly or intravenously. There were no mortalities or known morbidities associated with the immobilization procedure. Two moose were shot during the study period, one during the annual moose hunt (September 2017) and one as a special action by the local government because of local damage to forests (February 2018). In both instances, the loggers were collected and downloaded. We recaptured the remaining 10 moose 1 year after instrumentation (February 2018). The DST centi HRT was surgically removed, and data were downloaded, with the Mercury software program and a Communication Box (StarOddi, 2017). We downloaded all collar data and data from MITs stored in the collar unit in the field during recaptures.
One of the instrumented moose was not pregnant the first season; the remainder gave birth in May–June 2017 (mean calving date 28 May, range 17 May to 10 June), as determined from GPS clustering and subsequent visual verification in the field. The following season two moose were not pregnant. We excluded the non-pregnant moose in the first season from the beginning of the study until 15 September 2017, and the non-pregnant moose in the second season from 15 September 2017 until the end of the study. We chose 15 September because of reports of mid-September as the beginning of oestrus in female moose in Sweden (Malmsten et al., 2014).
A strong positive correlation between temperature recorded by GPS collars on moose and Ta recorded by weather stations was previously reported, therefore collar temperatures are regarded as a reliable index for Ta (Ericsson et al., 2015). We used the offset for latitude 64°N and month reported by Ericsson et al. (2015) to correct the collar temperatures for each individual moose. Drinking and snow intake have been shown to influence ruminal temperature obtained by MITs (Herberg et al., 2018). We used the R package “anomalize” (Dancho and Vaughan, 2018) to detect outliers associated with water intake (low Tb values). To facilitate optimal outlier detection for Tb time series including visual inspection and filtering, we used the twitter method to detect outliers, set trend and frequency to 6 h and allowed for a total of 20% of outlier detection (Dancho and Vaughan, 2018).
We programmed the Star Oddi device to record raw ECG signals every second hour of which a subsample of 150 ECG strips were used to manually calculate HR. The manual ECG based HR was compared with the HR measurements automatically calculated by the algorithm of the Star Oddi device. Because of insufficient automatic R-R interval detection, the algorithm was adjusted by StarOddi and rerun on the entire raw ECG data set. Bjarnason et al. (2019) provides a more detailed description of the HR calculation. Ultimately only recalculated HR measurements with quality index 0 or 1 were included in the analysis. Limitations on data storage resulted in a decision to not record raw ECG data for the 2-min detailed HR data after 6 August. Because of the high noise level and insufficient automatic R-R interval detection, HR data were not reliable after that date.
One of the recaptured moose had lost the DST centi-HRT logger and was therefore excluded from the HR analysis. Data from two moose were excluded during the entire period because of high noise level in the ECG raw data and unsuccessful HR calculation. Days of capture and recapture were excluded from the analysis, because the capture event and the anesthetics were expected to affect HR, Tb, and activity (Kreeger and Arnemo, 2018).
To investigate the seasonal patterns of Tb, HR, and activity, we fitted separate generalized additive mixed models (GAMM) with the function “bam” on daily mean Tb, HR activity and Ta as response variables (Wood, 2017). We added a fitted smooth term for day since capture, added a random intercept and slope for moose ID and an autoregressive model (AR1) structure to account for detected residual temporal autocorrelation (Pinheiro and Bates, 2000). We applied the “gam.check” function to choose adequate basis dimensions of the parameter k (Wood, 2017). We calculated the first derivatives of the smooth term for day since capture with the “fderiv” function, to determine periods of significant increase or decrease in daily mean Tb, HR and activity (Simpson, 2018).
We used Lomb-Scargle periodogram analysis to investigate circadian rhythms in Tb, HR, and activity (Ruf, 1999). We performed analyses on the raw data on a 15-day rolling window and tested for presence of circadian rhythms between 0.5 and 30 h. Significant periodicity was detected when peaks in the Lomb-Scargle periodogram exceeded the 95% confidence limit.
We visually investigated the intensity of Tb and activity variation over the year using the R package “ggplot2” to plot actograms of raw data of Tb and activity (Wickham, 2016). We calculated times for sunrise, sunset, dusk, and dawn at the following coordinates within the study area (Bivand and Lewin-Koh, 2017). We added the times to the actograms and defined sunrise and sunset as the time when the top edge of the sun reaches the horizon. Dusk and dawn were defined as the onset and end of civil twilight.
Daily energy expenditure was calculated on the days with the lowest and highest mean daily HR based on a pooled exponential equation:
where y is the MR and x is the HR normalised to BM–0.25 (body mass). The equation was developed from a study of captive moose (Renecker and Hudson, 1985).
From February to September 2017, we obtained Tb data from eleven moose, and from September 2017 to February 2018, we obtained Tb data from nine moose. We had valid HR data from eight moose from February until the beginning of August 2017.
Female moose exhibited seasonal variation in Tb, HR and activity with higher levels in June-July and lower levels in January–March (Table 1 and Figure 1). The seasonal trends followed the same patterns for all parameters throughout the year. Significantly increasing periods of activity, HR and Tb occurred between April and June and significantly decreasing periods occurred between July and November.
Table 1. Overview of dates, daily mean values (Mean) and 95% CI of the daily mean (95% CI) for dates with lowest and highest values and start and stop for increasing and decreasing periods of body temperature (Tb), heart rate (HR), collar activity (activity), and ambient temperature (Ta) for female moose in Sweden.
Figure 1. Daily mean body temperature, collar activity, heart rate, and ambient temperature for 12 female moose in Sweden. Solid line represents daily mean body temperature, collar activity, heart rate, and ambient temperature, respectively, dashed lines represents 95% confidence interval of the daily mean. Significantly increasing and decreasing periods are presented as red and blue, respectively, of the solid line.
Estimated mean daily energy expenditure, for all individuals, for the days with the lowest and highest daily mean HR were 377 kJ/kg0.75 and 935 kJ/kg0.75, respectively, which represented a 60% decrease in energy expenditure from summer to winter (calculations in Supplementary Appendix A).
Lomb-Scargle periodogram analyses detected circadian rhythmicity in Tb and activity, the schedule with HR measurements only every second hour was not adequate to detect circadian patterns in HR. Percentages of the displayed rhythms, based on the results of the periodograms for Tb and activity for one representative individual in January and September are presented in Figure 2 (for the remainder of individuals, percentages of the displayed rhythms for each month are included in Supplementary Appendix B). A period length (τ) of 24 h of Tb was dominate during most of the year, whereas ultradian rhythms with τ of 3–8 h were more frequent in December and January, and to a small extent in June and July. Activity displayed ultradian rhythmicity with period length τ of 2–8 h throughout the year. Actograms of raw data of Tb and activity for the same individual in January and September are presented in Figure 3 (for actograms for all individuals throughout the study period, see Supplementary Appendix C). A visual analysis of the actograms shows higher intensity of Tb and activity in September compared to January, as demonstrated in Figure 1.
Figure 2. Percentages of displayed rhythms based on Lomb-Scargle periodograms for body temperature (top) and activity (lower) for one representative moose in January and September. Vertical axis represents percentage of displayed rhythm and horizontal axis represents the rhythmicity in hours.
Figure 3. Actograms for body temperature (Tb) and activity for one representative moose in January and September. Vertical axis represents the date and horizontal axis represents time of the day. The area between the black lines represents the twilight. Lightest color represents lowest Tb and activity, and darker color represents higher Tb and activity.
Seasonal patterns displayed in the moose with low levels of Tb, HR and activity during winter compared with summer are similar to those reported in moose and other ungulates including alpine ibex, red deer, and Svalbard reindeer (Signer et al., 2011; Turbill et al., 2011; Arnold et al., 2018; Thompson et al., 2019). The interval from the lowest to the highest Tb recorded by vaginal implant transmitters in moose was 0.5°C (Thompson et al., 2019) compared with 0.61°C in our study using MITs, this is likely occurred because of the different types of biologgers used. The seasonal changes in Tb and HR are smaller than those reported for alpine ibex and Svalbard reindeer, which may be due to species differences, physiological state (i.e., pregnancy status, body-condition and mass, age, and sex), seasonal adaptations, the relatively mild coastal conditions and the unusually small amount of snow during the first winter of our study (mean and maximum daily snow depth was 0.15 and 0.5 m; Signer et al., 2011; Arnold et al., 2018). Maximum snow depths recorded from 2007/2008 to 2018/2019 ranged from 0.4 to 1.1 m (mean 0.66 m) and the mean snow depths from 0.13 to 0.53 m (mean of means 0.29 m; SMHI, 2019).
We calculated a 60% decrease in MR from summer to winter by decreasing HR. The calculation is based on an equation from Renecker and Hudson (1985). Mean daily MR was 377 kJ/kg0.75 in winter and 935 kJ/kg0.75 in summer. Calculated MR in this study is comparable to MRs obtained in earlier studies, and the decreased MR from summer to winter in these studies varies from 33–76% (Regelin et al., 1985; Renecker and Hudson, 1986, 1989). Differences between studies are likely related to dissimilarities in methods used to measure energy expenditure, study designs, and individual and environmental variation.
Voluntarily reduced food intake in winter, was demonstrated previously in moose receiving ad libitum high-quality food (Schwartz et al., 1984; Regelin et al., 1985). Differences in quality and intake of digestible nutrients could result in seasonal variations of HR and Tb, but do not result from food availability alone (Mesteig et al., 2000; Theil et al., 2004; Kochan, 2007; Signer et al., 2011). Decreased food intake could result in a decrease in both endogenous heat production from fermentation and metabolism in addition to reduced blood perfusion in abdominal organs, which results in lower HR and Tb (Mesteig et al., 2000; Signer et al., 2011). Seasonal variation in organ sizes corresponding to seasonal fluctuations in food and water intake occur in Sand gazelles (Gazella subgutturosa; Ostrowski et al., 2006), and it is assumed that this is a common feature in other ungulates as well (Arnold et al., 2006; Signer et al., 2011). A reduction in organ size could result from lower oxygen consumption with subsequent lower HR during winter.
The period with lowest levels of HR and Tb (March-April) coincides with the time of the year we would expect least natural food availability. Shortly after reaching the lowest values in mid-March, Tb started to rise, while HR was stable at its lowest level for a longer period. A similar relationship with Tb starting to rise prior to HR also was demonstrated in hibernating brown bears prior to den exit (Evans et al., 2016). Those authors suggested that the bears experienced passive rewarming with increasing Ta, before they experienced increased activity of the sympathetic nervous system, decreased activity of the parasympathetic nervous system or a combination thereof, resulting in an increase in HR. Passive warming from basking in the sun, was also offered as a strategy in ungulates, by using exogenous heat, i.e., the sun, for thermoregulation and to reduce endogenous energy production (Signer et al., 2011). The earlier increase in Tb compared with HR and activity (and hence feeding behavior) after the lowest levels of those measurements during winter, in our study, might result from passive rewarming by the sun. Even if the Ta did not exhibit a significant increase during the period where Tb started to increase significantly, an increase in Ta was visible in the graph in March and April (Figure 1). Short days and cold nights might account for the lack of a significant increase in Ta.
Growing season, wind, light-dark cycle, snowfall, and snow cover are other external cues that influence HR and Tb in ungulates (Signer et al., 2011; Arnold et al., 2018). HR started to increase significantly at approximately the same time as the start of the growing season (mean daily Ta > 5°C; SMHI, 2019), likely because of increased nutrition content in the food (Brosh, 2007). The last month of pregnancy could also influence the increase in HR (Mauget et al., 1997).
Activity, measured in this study with motion sensitive collars, includes head movements in addition to locomotion; consequently, feeding behavior increases activity (Gervasi et al., 2006). A rise in activity before the start of the growing season, as demonstrated in this study, could reflect increased feeding behavior because of increased food availability when snow is melting. Another moose study in the same study area reported that about 80% of the animals were migratory and that the timing of the migration differed between years, and varied with food availability and calving time (Singh et al., 2012). We did not investigate the migratory patterns in this study, but migration likely affected the seasonal patterns we observed.
We demonstrated, based on our model, a break in the increasing period of HR from 31 May until 3 June, which coincides with the calving. Nonetheless, further investigation will be necessary to determine if this break in HR resulted from calving. Reproductive status influence Tb patterns in several wild mammals. Reports exist of improved homeothermy in African lions (Panthera leo; Trethowan et al., 2016), wolverines (Gulo gulo; Thiel et al., 2019), muskox (Ovibos moschatus; Schmidt et al., 2020), and brown bears (Friebe et al., 2014) during gestation. Schmidt et al. (2020) also reported differences in seasonal Tb pattern between pregnant and non-pregnant muskoxen. The effect of reproductive status was not possible to detect in our study, because we only studied pregnant moose. MR in roe deer (Capreolus capreolus) increased during the last 2 months of gestation and during the first month of lactation, by 15 and 27%, respectively (Mauget et al., 1997). Regelin et al. (1985) documented no differences between sexes in MR in adult moose, when excluding last trimester of gestation and lactation. Thus, we would expect differences in Tb, HR and MR between pregnant and non-pregnant moose at the end of the gestation and the lactation. The skewed ratio of pregnant and non-pregnant females did not allow us to test for effects of pregnancy status on seasonal variation in HR and Tb.
Circadian rhythmicity in activity was demonstrated in this study, as previously reported in both moose (Van Ballenberghe and Miquelle, 1990; Gillingham and Klein, 1992) and other ungulates (Arnold et al., 2004; Lowe et al., 2010; Signer et al., 2011; Pagon et al., 2013; Ensing et al., 2014). In addition, we were able to demonstrate circadian organization of Tb, which has also been shown in Svalbard reindeer (Arnold et al., 2018). Tb displayed rhythms with τ of 24 h for most of the year, except December and January, where ultradian rhythms with τ 3–8 h were more dominate. In June and July, the months with the longest daylight periods, the 24 h rhythmicity was not as dominant as in the other months. This result is similar to the pattern reported in Svalbard reindeer by Arnold et al. (2018), who documented that during the periods with midnight sun and polar night the 24-h patterns were not as dominant as during the rest of the year. Even if there are no periods with constant daylight or darkness in our study area, the differences within the year could be affected by changes in the light-dark cycle. Other reasons for deviation from the 24-h cycle could be food availability, predation, and human disturbance (Ensing et al., 2014). We observed the same trend in the ultradian rhythms in activity as in moose in Alaska and Svalbard reindeer, with shorter periods in summer compared with winter (Van Ballenberghe and Miquelle, 1990; Arnold et al., 2018). Oscillation of rumination is demonstrated to be a regulatory function of activity, so the ultradian rhythmicity of activity is likely results from feeding behavior and ruminating (Scheibe et al., 1999). Diseases and stressful situations result in impairment of the ultradian rhythmicity of activity, and periodogram analysis could be used to detect irregular patterns (Scheibe et al., 1999; Berger, 2011).
Global climate change is an important concern regarding higher energetic cost with both fluctuating and increasing Ta (Boyles et al., 2011). The threshold for heat stress cannot only be explained by the Ta, but is also affected by factors such as wind speed, solar radiation, winter fur (i.e., in spring), physiological differences (e.g.,: age, sex, pregnancy status, body mass, and fitness), and immune status (Renecker and Hudson, 1986; McCann et al., 2013; Thompson et al., 2019). In this study, the highest mean daily Ta was 22.4°C, and >2 months had a mean daily Ta > 14°C, one reported threshold for heat stress (Renecker and Hudson, 1986); thus, the highest mean MR calculated in our study possibly represents a MR under heat stress and hypermetabolism. As a response to the increased MR, and to reduce heat generated from feeding, ruminants reduce dry matter intake under warm conditions (Beatty et al., 2008). Increasing Ta also could result in decreased availability of high-quality forage because of changes in vegetation phenology, habitat changes, and a shortened period with forage of high nutritional quality (Monteith et al., 2015). Cumulatively, loss of body mass and failure to accumulate fat will result in lower reproduction and survival, and increased infection risk (Lenarz et al., 2009; van Beest et al., 2012; McCann et al., 2013; van Beest and Milner, 2013).
In this study, Tb started to decline significantly 1 day after Ta peaked in July. Heat stress with voluntarily reduced food intake or habitat changes to habitats with forage of poorer quality could result in decreased endogenous heat production and thereby explain the decrease in Tb (Beatty et al., 2008; Monteith et al., 2015). The lack of HR data from the beginning of August, prevent us from commenting on the HR fluctuations from summer to winter. We strongly recommend performing pilot studies when using a HR detection algorithm on new species because validation is crucial to get reliable results.
Herein we documented the daily and annual pattern of Tb and HR of moose in northern Sweden. Annual variations of Tb and HR followed the same patterns with lowest levels in March and the highest levels from late June to early July. The decrease in HR from the highest to the lowest daily mean represents a 60% decrease in MR from summer to winter. The daily pattern of Tb varies throughout the year, with 24-h cycles as the predominant pattern in September, compared with ultradian cycles with τ 2–6 h as the predominant pattern in January. Our results indicate that hypometabolism is a vital strategy for energy saving with limited resources available in large are capital breeder in northern environment, with complexly regulated by both physiological and environmental factors. We hypothesize that hypermetabolism is also a strategy for gaining weight during summer.
The datasets generated for this study are available on request to the corresponding author.
The animal study was reviewed and approved by Animal Care Committee for Northern Sweden in Umeå (Dnr A3/2016 2016–02–26).
AE, JA, GE, WN, and NS designed and initiated the study. AG, AE, FS, JA, BF, and AT performed the data collection; data management was done by BF and AT. AG and AT drafted the manuscript. AT and AG performed the statistical analysis with advice from BF and NS. All authors participated in writing the manuscript and approved the submitted version.
The study was funded by the Norwegian Environmental Agency, the Inland Norway University of Applied Science, and the Swedish University of Agricultural Sciences (SLU) through the Beyond Moose program (financed by the Swedish EPA, the Kempe foundation, and the County Board of Västerbotten). The funders had no role in study design, data collection and analysis, decision to publish, or preparation of the manuscript.
The authors declare that the research was conducted in the absence of any commercial or financial relationships that could be construed as a potential conflict of interest.
We acknowledge the collaboration with the research programs Beyond Moose and Governance in cooperation with local landowners and hunters, the county board of Västerbotten, and the Västerbotten chapter of the Swedish Association of Hunting and Wildlife Management. We will also thank Ada Viljanen and Helle Bernsdorf Hydeskov for excellent help during fieldwork, and Olivier Devineau for statistical advice.
The Supplementary Material for this article can be found online at: https://www.frontiersin.org/articles/10.3389/fevo.2020.00107/full#supplementary-material
APPENDIX A | Energy calculations for the day with the lowest and highest daily mean heart rate.
APPENDIX B | Percentages of displayed rhythms based on Lomb-Scargle periodograms for Tb and activity for all individuals in all months.
APPENDIX C | Actograms for Tb and activity for each individual during the study.
Arnold, W., Ruf, T., and Kuntz, R. (2006). Seasonal adjustment of energy budget in a large wild mammal, the Przewalski horse (Equus ferus przewalskii) II. Energy expenditure. J. Exp. Biol. 209, 4566–4573. doi: 10.1242/jeb.02536
Arnold, W., Ruf, T., Loe, L. E., Irvine, R. J., Ropstad, E., Veiberg, V., et al. (2018). Circadian rhythmicity persists through the Polar night and midnight sun in Svalbard reindeer. Sci. Rep. 8:14466. doi: 10.1038/s41598-018-32778-4
Arnold, W., Ruf, T., Reimoser, S., Tataruch, F., Onderscheka, K., and Schober, F. (2004). Nocturnal hypometabolism as an overwintering strategy of red deer (Cervus elaphus). Am. J. Physiol. Regul. Integr. Compar. Physiol. 286, R174–R181. doi: 10.1152/ajpregu.00593.2002
Beatty, D., Barnes, A., Taylor, E., and Maloney, S. (2008). Do changes in feed intake or ambient temperature cause changes in cattle rumen temperature relative to core temperature? J. Ther. Biol. 33, 12–19. doi: 10.1016/j.jtherbio.2007.09.002
Berger, A. (2011). Activity patterns, chronobiology and the assessment of stress and welfare in zoo and wild animals. Int. Zoo Yearb. 45, 80–90. doi: 10.1111/j.1748-1090.2010.00121.x
Bivand, R., and Lewin-Koh, N. (2017). Maptools: Tools for Reading and Handling Spatial Objects. R package version 0.9–2.
Bjarnason, A., Gunnarsson, A., Arnason, T., Oddgeirsson, M., Sigmarsson, A. B., and Gunnarsson, A. (2019). Validation of ECG-derived heart rate recordings in Atlantic cod (Gadus morhua L.) with an implantable data logging system. Anim. Biotelemetr. 7, 1–10. doi: 10.1186/s40317-019-0176-4
Boyles, J. G., Seebacher, F., Smit, B., and McKechnie, A. E. (2011). Adaptive thermoregulation in endotherms may alter responses to climate change. Integr. Compar. Biol. 51, 676–690. doi: 10.1093/icb/icr053
Brosh, A. (2007). Heart rate measurements as an index of energy expenditure and energy balance in ruminants: a review. J. Anim. Sci. 85, 1213–1227. doi: 10.2527/jas.2006-298
Dettki, H., Brode, M., Giles, T., and Hallgren, J. (2014). “Wireless remote animal monitoring (WRAM)–A new international database e-infrastructure for management and sharing of telemetry sensor data from fish and wildlife,” in Proceedings of the International Congress on Environmental Modelling and Software, Colorado.
Ensing, E. P., Ciuti, S., de Wijs, F. A., Lentferink, D. H., ten Hoedt, A., Boyce, M. S., et al. (2014). GPS based daily activity patterns in European red deer and North American elk (Cervus elaphus): indication for a weak circadian clock in ungulates. PLoS One 9:e106997. doi: 10.1371/journal.pone.0106997
Ericsson, G., Dettki, H., Neumann, W., Arnemo, J. M., and Singh, N. J. (2015). Offset between GPS collar-recorded temperature in moose and ambient weather station data. Eur. J. Wildl. Res. 61, 919–922. doi: 10.1007/s10344-015-0968-7
Evans, A. L., Fahlman, A., Ericsson, G., Haga, H. A., and Arnemo, J. M. (2012). Physiological evaluation of free-ranging moose (Alces alces) immobilized with etorphine-xylazine-acepromazine in Northern Sweden. Acta Vet. Scand. 54:77. doi: 10.1186/1751-0147-54-77
Evans, A. L., Singh, N. J., Friebe, A., Arnemo, J. M., Laske, T. G., Fröbert, O., et al. (2016). Drivers of hibernation in the brown bear. Front. Zool. 13:7. doi: 10.1186/s12983-016-0140-6
Fiorello, C. V., Harms, C. A., Chinnadurai, S. K., and Strahl-Heldreth, D. (2016). Best-practice guidelines for field-based surgery and anesthesia on free-ranging wildlife. II. Surgery. J. Wildl. Dis. 52, S28–S39. doi: 10.7589/52.2S.S28
Friebe, A., Evans, A. L., Arnemo, J. M., Blanc, S., Brunberg, S., Fleissner, G., et al. (2014). Factors affecting date of implantation, parturition, and den entry estimated from activity and body temperature in free-ranging brown bears. PLoS One 9:e101410. doi: 10.1371/journal.pone.0101410
Fuchs, B., Sørheim, K. M., Chincarini, M., Brunberg, E., Stubsjøen, S. M., Bratbergsengen, K., et al. (2019a). Heart rate sensor validation and seasonal and diurnal variation of body temperature and heart rate in domestic sheep. Vet. Anim. Sci. 8:100075. doi: 10.1016/j.vas.2019.100075
Fuchs, B., Yamazaki, K., Evans, A. L., Tsubota, T., Koike, S., Naganuma, T., et al. (2019b). Heart rate during hyperphagia differs between two bear species. Biol. Lett. 15:20180681. doi: 10.1098/rsbl.2018.0681
Geiser, F. (2004). Metabolic rate and body temperature reduction during hibernation and daily torpor. Ann. Rev. Physiol. 66, 239–274. doi: 10.1146/annurev.physiol.66.032102.115105
Gervasi, V., Brunberg, S., and Swenson, J. E. (2006). An individual-based method to measure animal activity levels: a test on brown bears. Wildl. Soc. Bull. 34, 1314–1319. doi: 10.2193/0091-7648(2006)34[1314:aimtma]2.0.co;2
Gillingham, M. P., and Klein, D. R. (1992). Late-winter activity patterns of moose (Alces alces gigas) in western Alaska. Can. J. Zool. 70, 293–299. doi: 10.1139/z92-044
Green, J. A. (2011). The heart rate method for estimating metabolic rate: review and recommendations. Compar. Biochem. Physiol. Part A 158, 287–304. doi: 10.1016/j.cbpa.2010.09.011
Heldmaier, G., Ortmann, S., and Elvert, R. (2004). Natural hypometabolism during hibernation and daily torpor in mammals. Respir. Physiol. Neurobiol. 141, 317–329. doi: 10.1016/j.resp.2004.03.014
Herberg, A. M., St-Louis, V., Carstensen, M., Fieberg, J., Thompson, D. P., Crouse, J. A., et al. (2018). Calibration of a rumen bolus to measure continuous internal body temperature in moose. Wildl. Soc. Bull. 42, 328–337. doi: 10.1002/wsb.894
Klassen, N. A., and Rea, R. V. (2008). What do we know about nocturnal activity of moose? Alces 44, 101–109.
Kreeger, T. J., and Arnemo, J. M. (2018). Handbook of Wildlife Chemical Immobilization, 5th Edn. Published by authors.
Langvatn, R. (1992). Basissignal for hjerteaktivitet hos elg ved ulike typer fysisk aktivitet. NINA Oppdragsmelding 178, 1–15.
Lenarz, M. S., Nelson, M. E., Schrage, M. W., and Edwards, A. J. (2009). Temperature mediated moose survival in northeastern Minnesota. J. Wildl. Manag. 73, 503–510. doi: 10.2193/2008-265
Lian, M., Evans, A. L., Bertelsen, M. F., Fahlman, A., Haga, H. A., Ericsson, G., et al. (2014). Improvement of arterial oxygenation in free-ranging moose (Alces alces) immobilized with etorphine-acepromazine-xylazine. Acta Vet. Scand. 56:51. doi: 10.1186/s13028-014-0051-5
Lovegrove, B. G. (2005). Seasonal thermoregulatory responses in mammals. J. Comp. Physiol. B 175, 231–247. doi: 10.1007/s00360-005-0477-1
Lowe, S. J., Patterson, B. R., and Schaefer, J. A. (2010). Lack of behavioral responses of moose (Alces alces) to high ambient temperatures near the southern periphery of their range. Can. J. Zool. 88, 1032–1041. doi: 10.1139/Z10-071
Malmsten, J., Söderquist, L., Thulin, C.-G., Widén, D. G., Yon, L., Hutchings, M. R., et al. (2014). Reproductive characteristics in female Swedish moose (Alces alces), with emphasis on puberty, timing of oestrus, and mating. Acta Vet. Scand. 56:23. doi: 10.1186/1751-0147-56-23
Mauget, C., Mauget, R., and Sempéré, A. (1997). Metabolic rate in female European roe deer (Capreolus capreolus): incidence of reproduction. Can. J. Zool. 75, 731–739. doi: 10.1139/z97-094
McCann, N., Moen, R., and Harris, T. (2013). Warm-season heat stress in moose (Alces alces). Can. J. Zool. 91, 893–898. doi: 10.1139/cjz-2013-0175
Mesteig, K., Tyler, N., and Blix, A. (2000). Seasonal changes in heart rate and food intake in reindeer (Rangifer tarandus tarandus). Acta Physiol. 170, 145–151. doi: 10.1046/j.1365-201x.2000.00767.x
Minicucci, L., Carstensen, M., Crouse, J., Arnemo, J. M., and Evans, A. (2018). A technique for deployment of rumen bolus transmitters in free-ranging moose (Alces alces). J. Zoo Wildl. Med. 49, 227–230. doi: 10.1638/2017-0027R.1
Monteith, K. L., Klaver, R. W., Hersey, K. R., Holland, A. A., Thomas, T. P., and Kauffman, M. J. (2015). Effects of climate and plant phenology on recruitment of moose at the southern extent of their range. Oecologia 178, 1137–1148. doi: 10.1007/s00442-015-3296-4
Ostrowski, S., Mésochina, P., and Williams, J. B. (2006). Physiological adjustments of sand gazelles (Gazella subgutturosa) to a boom-or-bust economy: standard fasting metabolic rate, total evaporative water loss, and changes in the sizes of organs during food and water restriction. Physiol. Biochem. Zool. 79, 810–819. doi: 10.1086/504614
Pagon, N., Grignolio, S., Pipia, A., Bongi, P., Bertolucci, C., and Apollonio, M. (2013). Seasonal variation of activity patterns in roe deer in a temperate forested area. Chronobiol. Int. 30, 772–785. doi: 10.3109/07420528.2013.765887
Pinheiro, J., and Bates, D. (2000). Mixed-Effects Models in S and S-PLUS. Berlin: Springer Science & Business Media.
Regelin, W. L., Schwartz, C. C., and Franzmann, A. W. (1985). Seasonal energy metabolism of adult moose. J. Wildl. Manag. 42, 388–393. doi: 10.2307/3801539
Renecker, L. A., and Hudson, R. J. (1985). Telemetered heart rate as an index of energy expenditure in moose (Alces alces). Compar. Biochem. Physiol. Part A 82, 161–165. doi: 10.1016/0300-9629(85)90721-2
Renecker, L. A., and Hudson, R. J. (1986). Seasonal energy expenditures and thermoregulatory responses of moose. Can. J. Zool. 64, 322–327. doi: 10.1139/z86-052
Renecker, L. A., and Hudson, R. J. (1989). Ecological metabolism of moose in aspen-dominated boreal forests, central Alberta. Can. J. Zool. 67, 1923–1928. doi: 10.1139/z89-275
Riek, A., Stölzl, A., Bernedo, R. M., Ruf, T., Arnold, W., Hambly, C., et al. (2019). Energy expenditure and body temperature variations in llamas living in the High Andes of Peru. Sci. Rep. 9:4037. doi: 10.1038/s41598-019-40576-9
Roshchevsky, M., Chermnykh, N., and Azarov, J. (1999). Cardiac reactions in the behaviour of young moose. Alces 35, 143–151.
Ruf, T. (1999). The Lomb-Scargle periodogram in biological rhythm research: analysis of incomplete and unequally spaced time-series. Biol. Rhythm Res. 30, 178–201. doi: 10.1076/brhm.30.2.178.1422
Ruf, T., and Geiser, F. (2015). Daily torpor and hibernation in birds and mammals. Biol. Rev. 90, 891–926. doi: 10.1111/brv.12137
Rutz, C., and Hays, G. C. (2009). New frontiers in biologging science. Biol. Lett. 5, 289–292. doi: 10.1098/rsbl.2009.0089
Scheibe, K., Berger, A., Langbein, J., Streich, W., and Eichhorn, K. (1999). Comparative analysis of ultradian and circadian behavioural rhythms for diagnosis of biorhythmic state of animals. Biol. Rhythm Res. 30, 216–233. doi: 10.1076/brhm.30.2.216.1420
Schmidt, N. M., Grøndahl, C., Evans, A. L., Desforges, J.-P., Blake, J., Hansen, L. H., et al. (2020). On the interplay between hypothermia and reproduction in a high arctic ungulate. Sci. Rep. 10, 1–7. doi: 10.1038/s41598-020-58298-8
Schwartz, C. C., Regelin, W. L., and Franzmann, A. (1984). Seasonal dynamics of food intake in moose. Alces 20, 223–244.
Signer, C., Ruf, T., and Arnold, W. (2011). Hypometabolism and basking: the strategies of Alpine ibex to endure harsh over-wintering conditions. Funct. Ecol. 25, 537–547. doi: 10.1111/j.1365-2435.2010.01806.x
Simpson, G. L. (2018). gratia: ggplot-based Graphics and Other Useful Functions for GAMs Fitted Using mgcv. R package version 0.1–4.
Singh, N. J., Börger, L., Dettki, H., Bunnefeld, N., and Ericsson, G. (2012). From migration to nomadism: movement variability in a northern ungulate across its latitudinal range. Ecol. Appl. 22, 2007–2020. doi: 10.1890/12-0245.1
Solberg, E. J., Heim, M., Arnemo, J. M., Sæther, B.-E., and Os, Ø (2003). Does rectal palpation of pregnant moose cows affect pre-and neo-natal mortality of their calves? Alces 39, 65–77.
Theil, P. K., Coutant, A. E., and Olesen, C. R. (2004). Seasonal changes and activity-dependent variation in heart rate of roe deer. J. Mammal. 85, 245–253. doi: 10.1644/1545-15422004085<0245:SCAAVI<2.0.CO;2
Thiel, A., Evans, A. L., Fuchs, B., Arnemo, J. M., Aronsson, M., and Persson, J. (2019). Effects of reproduction and environmental factors on body temperature and activity patterns of wolverines. Front. Zool. 16:21. doi: 10.1186/s12983-019-0319-8
Thompson, D. P., Barboza, P. S., Crouse, J. A., Mcdonough, T. J., Badajos, O. H., and Herberg, A. M. (2019). Body temperature patterns vary with day, season, and body condition of moose (Alces alces). J. Mammal. 100, 1466–1478. doi: 10.1093/jmammal/gyz119
Trethowan, P. D., Hart, T., Loveridge, A. J., Haw, A., Fuller, A., and Macdonald, D. W. (2016). Improved homeothermy and hypothermia in African lions during gestation. Biol. Lett. 12:20160645. doi: 10.1098/rsbl.2016.0645
Turbill, C., Ruf, T., Mang, T., and Arnold, W. (2011). Regulation of heart rate and rumen temperature in red deer: effects of season and food intake. J. Exp. Biol. 214, 963–970. doi: 10.1242/jeb.052282
Van Ballenberghe, V., and Miquelle, D. G. (1990). Activity of Moose during Spring and Summer in Interior Alaska. J. Wildl. Manag. 54, 391–396. doi: 10.2307/3809645
van Beest, F. M., and Milner, J. M. (2013). Behavioural responses to thermal conditions affect seasonal mass change in a heat-sensitive northern ungulate. PLoS One 8:e65972. doi: 10.1371/journal.pone.0065972
van Beest, F. M., Van Moorter, B., and Milner, J. M. (2012). Temperature-mediated habitat use and selection by a heat-sensitive northern ungulate. Anim. Behav. 84, 723–735. doi: 10.1016/j.anbehav.2012.06.032
Van Oort, B. E., Tyler, N. J., Gerkema, M. P., Folkow, L., Blix, A. S., and Stokkan, K.-A. (2005). Circadian organization in reindeer. Nature 438, 1095–1096. doi: 10.1038/4381095a
van Oort, B. E., Tyler, N. J., Gerkema, M. P., Folkow, L., and Stokkan, K.-A. (2007). Where clocks are redundant: weak circadian mechanisms in reindeer living under polar photic conditions. Naturwissenschaften 94, 183–194. doi: 10.1007/s00114-006-0174-2
Keywords: activity, Alces alces, body temperature, global positioning system, heart rate, metabolic rate, moose, seasonal variation
Citation: Græsli AR, Thiel A, Fuchs B, Singh NJ, Stenbacka F, Ericsson G, Neumann W, Arnemo JM and Evans AL (2020) Seasonal Hypometabolism in Female Moose. Front. Ecol. Evol. 8:107. doi: 10.3389/fevo.2020.00107
Received: 26 October 2019; Accepted: 01 April 2020;
Published: 05 May 2020.
Edited by:
R. Terry Bowyer, University of Alaska Fairbanks, United StatesReviewed by:
Ron Moen, University of Minnesota Duluth, United StatesCopyright © 2020 Græsli, Thiel, Fuchs, Singh, Stenbacka, Ericsson, Neumann, Arnemo and Evans. This is an open-access article distributed under the terms of the Creative Commons Attribution License (CC BY). The use, distribution or reproduction in other forums is permitted, provided the original author(s) and the copyright owner(s) are credited and that the original publication in this journal is cited, in accordance with accepted academic practice. No use, distribution or reproduction is permitted which does not comply with these terms.
*Correspondence: Anne Randi Græsli, YW5uZS5ncmFzbGlAaW5uLm5v
†These authors have contributed equally to this work
Disclaimer: All claims expressed in this article are solely those of the authors and do not necessarily represent those of their affiliated organizations, or those of the publisher, the editors and the reviewers. Any product that may be evaluated in this article or claim that may be made by its manufacturer is not guaranteed or endorsed by the publisher.
Research integrity at Frontiers
Learn more about the work of our research integrity team to safeguard the quality of each article we publish.