- 1Department of Terrestrial Ecology, Netherlands Institute of Ecology (NIOO-KNAW), Wageningen, Netherlands
- 2Section Plant Ecology and Phytochemistry, Institute of Biology, Leiden University, Leiden, Netherlands
Soil legacies are typically examined for individual plant species, and we poorly understand how soil legacy effects created by entire plant communities influence plant growth. We used soils collected from a biodiversity field experiment to examine how the soil legacy effects of plant diversity influence the growth of a focal plant species. In the field, we experimentally assembled and maintained grassland communities (0, 1, 2, 4, or 9 species) for two years. We collected soil from all plots and examined the growth of Jacobaea vulgaris in these soils under controlled conditions, and compared this to the performance of individuals that were planted directly in the plots. J. vulgaris was not part of the species pool used in the biodiversity experiment, but commonly occurs in the area. To disentangle different components of the legacy effects (soil nutrients vs. soil biota), in the pot experiment we tested the effects of plant growth in pure field soil and in sterilized background soil inoculated with live or with sterilized field soil. We found a weak positive legacy effect of plant diversity on J. vulgaris root biomass, but only in pure field soil and not in the inoculated treatments. Interestingly, for individuals planted in the field plots, plant biomass was negatively related to the diversity of the surrounding plant community but this was mainly due to high biomass in bare plots. In the pot experiment, plant biomass also varied among soils collected from different monocultures. Soil fungal community composition was not affected by the diversity of the plant community, but the biomass of the plants grown in pots with pure field soil correlated with fungal composition. The biomass of plants grown in pure field soil was also positively correlated with nitrogen availability in the soil, and negatively with the cover of three plants species in the communities. In conclusion, our study does not provide strong evidence for an important role of plant diversity on soil legacy effects on J. vulgaris, and shows that for this plant species, performance is related to both the biotic and abiotic characteristics of the soil in which it grows.
Introduction
Plants can alter the biotic and abiotic conditions in the soil, and this can affect other plants that grow later in this soil. Most studies that examine these plant-soil interactions have examined the impacts of an individual species, via its effect on the soil, on the performance of another plant in pot experiments (Kulmatiski et al., 2008). In natural communities such as grasslands, plants do not grow in isolation but interact with other plants. An important caveat in our understanding of the role of plant-soil interactions in natural plant communities is that we poorly understand how entire plant communities influence the soil, and, in turn, how these soil legacies influence plants that grow later in this soil (Bever, 2003; Petermann et al., 2008; Bever et al., 2010; van der Putten et al., 2013; Bennett et al., 2019).
Plant species vary greatly in how they influence the soil. In mixed plant communities the impact of a plant species on the soil will depend on how strongly this species influences the soil, but also on the abundance of this plant species in the community, on the identity of the other species that also influence the soil, as well as on the characteristics of the soil. Hence, it is very difficult to predict how soil legacy effects created by different plant communities will influence the growth of other plants in the soil. It is well known though, that different plant communities harbor unique soil communities (e.g., Bezemer et al., 2010; Heinen et al., 2018), and that these soil communities can differentially affect plants that grow later in the soil (e.g., Kardol et al., 2006). Whether this also depends on the number of species that make up the plant community is less well-understood. Most studies that examine the relationship between plant diversity and soil, so far, focus on the role of soil biota in driving diversity-ecosystem function patterns (e.g., Kulmatiski et al., 2012; but see Wurst et al., 2015). Various plant biodiversity experiments have shown that the number of species in a plant community is related to the diversity, composition and functioning of organisms in the soil (e.g., Wardle et al., 2003; Eisenhauer et al., 2010; Lange et al., 2015). Several studies have also shown that soil pathogens build up in soil of monospecific plant communities resulting in conspecific legacy effects (e.g., Maron et al., 2011; Schnitzer et al., 2011). Likewise, accumulation of pathogens that are less species-specific could give rise to heterospecific soil legacy effects. These changes in soil biota can result in positive relationships between the richness of the plant community and the performance of a plant when it grows in the soil of that community (Wurst et al., 2015; Luo et al., 2016). The opposite may be true for beneficial soil organisms such as mycorrhizal fungi.
While soil biota are a common mechanism of soil mediated effects between plants (e.g., Wardle et al., 2004; van der Putten et al., 2013), changes in nutrient availabilities, allelochemicals or other soil abiotic properties can also be a mechanism (Reynolds et al., 2003; Ehrenfeld et al., 2005; Perkins and Nowak, 2013; Bennett and Klironomos, 2018). Such negative effects could be strongest in monocultures and be diluted in mixed plant communities and hence also changes in abiotic soil conditions could result in a positive relationship between the plant diversity of a plot and the performance of plants that grow in the soil collected from these plant diversity plots.
Jacobaea vulgaris is a native plant species in the Asteracaea family. It occurs in varying densities in natural grasslands in Western Europe and is an important nectar source for numerous insects (Harper and Wood, 1957). For plants grown in pots we have previously shown that this species is highly sensitive to soil legacies created by other plant species (van de Voorde et al., 2011). Typically growth of J. vulgaris is reduced in soils conditioned by other species, compared to growth in sterile soil (Jing et al., 2015). Interestingly, these negative species-specific legacy effects are reduced when the soil is mixed with soil from other plant species, probably due to diluting the effects of e.g., soil pathogens, or allelopathy caused by specific plant species (van de Voorde et al., 2011). Hence, we may expect a positive effect of plant diversity on the overall negative soil legacy effects that influence this species (sensu Luo et al., 2016).
In this study we examine legacy effects of soils collected from grassland plots in which the diversity of the plant community was manipulated and maintained at levels of 0, 1, 2, 4, or 9 species per plot. The legacy effects were assessed using the phytometer plant J. vulgaris. This species was not present in any of the plots in which we maintained plant diversity and hence we tested how soil legacy effects of plant diversity influence the performance of a new species that colonizes the community. To evaluate to what extent the potential legacy effects were caused by soil biota we examined J. vulgaris performance in a pot experiment with pots filled with soil collected from the field plots and in pots filled with a standard sterilized background soil and inoculated with 20% live or sterilized soil from the field plots. After establishment of the plant communities in the field, in each plot we also planted J. vulgaris seedlings, and measured their performance in the field. This enabled us to compare the soil-mediated diversity effect on J. vulgaris observed in pots and the performance of this species in the field inside the diversity plots. Finally, to gain further insight on soil biota in comparison with soil abiotic properties as drivers of legacy effects, we measured the fungal community and we determined abiotic characteristics such as nutrient availability, organic matter and pH in the soil from each plot.
Specifically we examine:
(i) the soil legacy effect of plant diversity on the growth of J. vulgaris
(ii) the relationship between the abundance of plant species in the community and the soil legacy effect on J. vulgaris
(iii) whether the diversity-soil legacy relationship depends on the sterilization or inoculation treatments and how this is related to soil biotic and abiotic characteristics
(iv) how plant growth of J. vulgaris in pots filled with field soil or in pots inoculated with field soil is related to plant growth in the soils in the field.
Materials and Methods
Plant Species
Jacobaea vulgaris Gaertn. is a biannual or short-lived perennial plant that commonly occurs in natural and semi-natural areas throughout Europe and Asia. As is characteristic of Jacobaea (Senecio) species J. vulgaris contains pyrrolizidine alkaloids known to deter generalist insect herbivores (Cheng et al., 2011). Pot experiments carried out in our lab have shown that J. vulgaris exhibits a strong negative conspecific plant–soil feedback but that plant growth also responds to soil legacy effects created by other plant species and that these effects range from positive to strongly negative (van de Voorde et al., 2011). Experimental J. vulgaris plants for this study were grown from seeds collected from a single natural plant population from the area surrounding the grassland site in which the biodiversity experiment was carried out (Mossel, Ede, The Netherlands). J. vulgaris naturally occurs in this area.
Biodiversity Experiment
To study how the diversity of the plant community influences soil legacy effects we used an established biodiversity field experiment. For a detailed description of the experiment see Kostenko et al. (2012). In brief, in the summer 2008, seventy plots (3 × 3 m) separated by 1-m-wide paths were established in a fenced area within a former arable field that was restored to natural grassland (Mossel, Ede, The Netherlands). In September 2008, the plots were sown with a single plant species (monocultures) or with mixtures of 2, 4, or 9 species randomly chosen from a pool of 12 local grassland species that naturally co-occur in the studied area (Supplementary Table S1). Jacobaea vulgaris was not sown. Plots with the same species composition were replicated twice using a complete randomized design. There were 12 monocultures (one for each plant species), nine combinations of two species, 11 combinations of four species, and three combinations of nine species. One legume (Trifolium arvense), one forb (Tripleurospermum maritimum), and two grass species (Agrostis capillaris and Anthoxanthum odoratum) established poorly in monocultures, although these species were present in the mixed communities. The monocultures of these four species were therefore excluded from the analyses. Four plots were kept free of all plants. In the sown plots, the sowing density was 4,000 seeds per m2. The sown species composition was maintained by hand weeding from the beginning of the growing season (late April) until the end of the growing season (late August) and paths between plots were regularly mown during each growing season. The experimental site was fenced to exclude large vertebrate herbivores.
At the end of August 2009, one year after sowing and after the different plant diversity treatments had established for one entire season, 25, 8-week-old J. vulgaris rosettes (5 cm diameter) were planted in a regular grid of 0.3 × 0.3 m in the central 1.2 × 1.2 m square of each plot. The rosettes were grown from surface-sterilized seeds (1 min in 2% sodium hypochlorite solution and rinsed with water) germinated on glass beads and transplanted into seedling trays filled with sterilized potting compost in a greenhouse (21/16°C day/night, 16 h photoperiod). The resident plant community around the rosettes of the J. vulgaris plants was not removed in order to test the effects of the surrounding community on the establishment of J. vulgaris seedlings. In bare plots, no other plants than the 25 J. vulgaris plants were present.
Soil Collection
In each plot, 25 soil cores (15 cm deep, 5 cm diameter) were collected in a regular rectangular grid 2 years after the establishment of the experiment. The soil cores were not taken underneath or in direct vicinity of the J. vulgaris plants. The soil collected from each plot was stored individually in a plastic bag and transported to the laboratory 1 h after collection. In the laboratory, the soil samples were pooled per plot and sieved through a 0.5 cm mesh. Then, each soil sample was split in three subsamples: (1) A subsample of 1.0 g homogenized soil. This subsample was stored at −20°C for molecular analysis. (2) A subsample of 100 g soil. This subsample was oven-dried at 40°C and sieved (4 mm mesh size) to be used for chemical analysis. (3) The remaining soil was kept in a 4°C climate chamber for several days before using it for the soil legacy bioassay.
Soil Legacy Bioassay
Seeds of J. vulgaris plants were surface sterilized (1 min in 2% sodium hypochlorite solution and rinsed with water) and germinated on glass beads. Pots (800 ml) were filled with: (1) 800 mg of field-conditioned soil (“Field soil” treatment); (2) 160 g of field-conditioned soil mixed with 640 g (1:4 inoculation ratio) sterilized background field soil (“Live inoculum” treatment); (3) 160 g of sterilized field-conditioned soil mixed with 640 g sterilized background field soil (“Sterilized inoculum” treatment). Additionally, 10 pots were filled with 800 mg of sterilized background field soil (“Sterilized background soil” treatment; Figure 1). Background soil was collected from the grassland area surrounding the experiment (Mossel, Ede, The Netherlands). Sterilization of soils was achieved using gamma irradiation (>25 KGray, Isotron, Ede, The Netherlands). The difference between the live inoculum treatment and the sterilized inoculum treatment is an indication for the biotic soil legacy effect. The field soil treatment shows the overall legacy effects of plant diversity, while the comparison of the live and sterilized inoculum treatment with the 100% background soil treatment shows the effects of inoculation (Figure 1). The soil collected from each plot was kept separate and was used to fill two pots for each of the three treatments, resulting in 420 pots (70 plots × 3 treatments × 2 replicates per plot). One J. vulgaris seedling was transplanted into each pot. Seedlings that died during the first week of the experiment were replaced. Pots were randomly located within a greenhouse (21/16°C day/night, relative humidity 50–60%, 16 h photoperiod). Natural daylight was supplemented by 400 W metal halide lamps (225 μmol m–2 s–1 PAR). Plants were watered three times per week and randomly rearranged within the greenhouse once a week. After 8 weeks of growth, shoots were clipped; roots were carefully removed from the soil and rinsed. Shoot and root biomass of each pot was then oven-dried (70°C for 3 days) and weighed. Two root samples from the “live inoculum” treatment were lost during this process. These samples were not included in the statistical analyses.
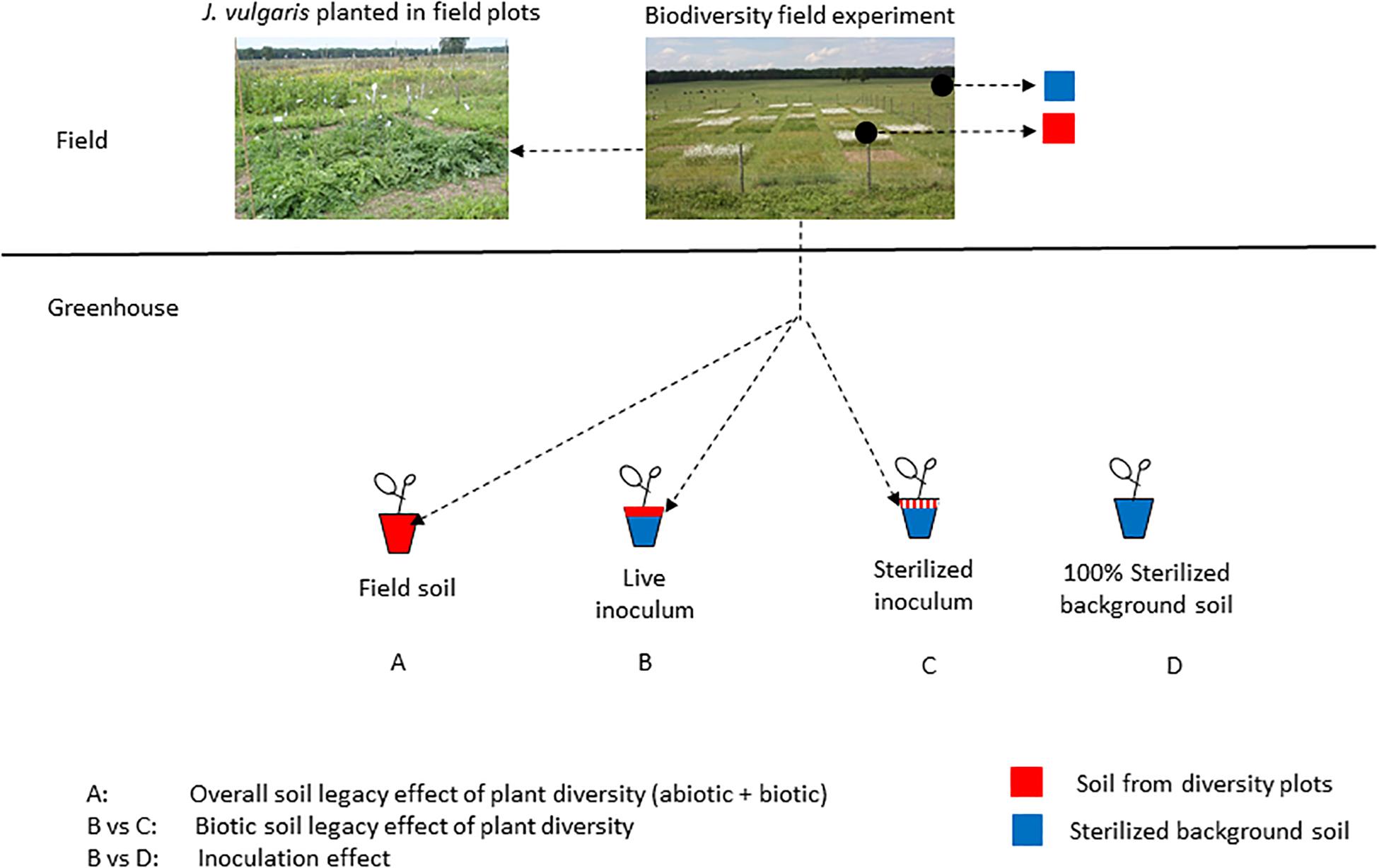
Figure 1. Schematic overview of the experimental setup. Soil was collected from field plots that differed in plant diversity (0, 1, 2, 4, 9 species) and the legacy effect of these soils on J. vulgaris was determined in a pot experiment (pure field-conditioned soil; sterilized background soil mixed with live inoculum; sterilized background soil mixed with sterilized inoculum). The effects were compared to the performance of J. vulgaris planted in the plots (left photo shows J. vulgaris in a bare plot).
Plant Measurements in the Field
In August 2010, all J. vulgaris plants were at the rosette stage. To estimate the size of the J. vulgaris plants in the field, we collected two random plants per plot and measured their shoot and root biomass and the number of rosette leaves and the length of the longest leaf. For all remaining J. vulgaris plants we recorded the number of rosette leaves and the length of the longest leaf. Then, based on the number of rosette leaves, the length of the longest leaf and the biomass of the collected plants, we constructed a model to predict the aboveground plant biomass (g) of all field-grown plants (for details see Kostenko et al., 2012). Furthermore, in August 2010 we determined the cover of all sown species in three squares (1 × 1 m) along a diagonal transect in each experimental plot.
Molecular Analysis of the Soil Fungal Community
The composition of the fungal community in the soil collected from each experimental plot was determined by T-RFLP (Terminal restriction fragment length polymorphism) analysis. Total DNA was extracted from 0.5 g frozen soil (−20°C) with a Power Soil DNA isolation kit (MOBIO laboratories, Inc.) using a bead beating system. DNA quantity was checked using 1.5% agarose gel electrophoresis. The ITS region of the fungal rDNA was amplified by PCR using the primers ITS1F (White et al., 1990) and ITS4 (Gardes and Bruns, 1993), which were labeled with FAM and NED respectively. The PCR reaction contained 13.8 μl Milli-Q, 2.5 μl 10× Fast Start High Fidelity Reaction Buffer (Roche Diagnostics), 2.5 μl DNTP Mix (2mM each), 2.5 μl ITS1F-6FAM primer (10 μM), 2.5 μl ITS4-NED primer (0.2 μM), 0.2 μl Fast Start High Fidelity Enzym Blend (5 U/μl) (Roche Diagnostics) and 1 μl template DNA. PCR program conditions were 5 min at 95°C, 34 cycles of 30 s at 95°C, 40 s at 55°C and 1 min at 72°C, followed by 10 min at 72°C before cooling. PCR product presence and quality were verified on 1.5% agarose gels prior to restriction digestion. Two restriction enzymes, HhaI and TaqαI (New England Biolabs, Ipswich, MA, United States), were used to digest dual end-labeled DNA amplicons. A mixture containing 3.5 μl ddH2O, 1 μl buffer, 0.1 μl Bovine Serume Albumin, 5 μl PCR product and 0.4 μl restriction enzyme was incubated at 37°C (HhaI) or at 65°C (TaqαI) for 3 h, and inactivated at 80°C for 20 min. Restriction products were purified using ethanol precipitation. Fragment length polymorphism analysis was performed on an automated 3130 Genetic Analyzer sequencer (Applied Biosystems) with GeneScan-500 LIZ, Applied Biosystems as a size standard. Samples which were over- (highest peak > 80,000 rfu) or under-loaded (highest peak < 1,000 rfu) were re-run with an adjusted concentration. Peaks were aligned to TRFs among the samples by applying a clustering threshold of 0.5 bp. Only peaks higher than 0.2% of the sum of all peaks in a sample were included.
Abiotic Soil Characteristics
Concentrations of plant available mineral nitrogen (NH4+ and NO3–), potassium (K) and magnesium (Mg) in the soil samples were determined colorimetrically in 1:10 (w/v) 0.01 M CaCl2 using a Traacs 800 autoanalyser (TechniCon Systems Inc., Oakland, CA, United States). The C:N ratio in soil samples was measured on a FlashEA 1112 Series NC soil analyzer (Thermo Fisher Scientific, Waltham, MA, United States). pH was measured in 2:5 dry soil:water suspensions. The percentage organic carbon (C) was determined according to Nelson and Sommers (1982). Soil organic matter was determined by the loss-on-ignition method. Approximately 5 g of soil was oven dried at 105°C for 16 h and weighed. The sample was then burned at 550°C for 5 h and weighed again. Soil organic matter was calculated as the percentage weight loss between the oven-dried and burned samples. Total plant available phosphorous (P) was determined according to Olsen et al. (1954) and absorbance was measured at 720 nm (Supplementary Table S2).
Data Analyses
To examine the soil legacy effects of plant diversity on the growth of J. vulgaris plants we used mixed-effects models with plant community diversity (0–9 species) and soil treatment (field soil, live inoculum, sterilized inoculum) as fixed effects. Plant diversity was transformed as log2(species richness + 1). We included plot identity as a random effect to incorporate that multiple pots were filled with soil collected from the same plot. Individual comparisons within each soil treatment were based on a Tukey HSD test. We repeated this analysis by excluding the bare plots. The effect of plant diversity on soil nutrients was analyzed using a general linear model with plant diversity as a log-linear factor [log2(species richness + 1)] and bare plots included or excluded from the model for all plots. Monoculture plots were compared using a general linear model with plant identity as a fixed variable. Univariate analyses were performed in R statistical language, ver. 3.4.3 (R Core Team, 2017).
The presence/absence matrix of T-RFLP fingerprints was analyzed using correspondence analysis and canonical correspondence analysis (CA and CCA) and the combined abiotic soil characteristics with principle component and redundancy analysis (PCA and RDA, centered and standardized data) in CANOCO version 5.03 (Šmilauer and Lepš, 2014). Significances in multivariate analyses were inferred by Monte Carlo permutation tests (999 permutations).
The association between the performance of the plants grown in the field-conditioned soil and plants grown in the field plots, as well as the relationship between plant growth and the cover of different species in the plant communities was analyzed using a Spearman rank-based correlation test. The association between the performance of the plants grown in the field-conditioned soil and fungal community composition of the soil was analyzed using CCA with forward model selection procedure. Finally, the association between the performance of the plants grown in the field-conditioned soil and soil nutrient composition was analyzed using multiple linear regression analysis with a stepwise model selection algorithm by AIC. In the latter two tests plant species richness was included as continuous covariate.
Structural Equation Modeling
Structural equation modeling (SEM, Shipley, 2002) was used to examine the strength of pathways linking soil legacies of plant diversity with the performance of J. vulgaris plants in the greenhouse bioassay. The conceptual model, presented in Supplementary Figure S1, considered direct effects of plant species richness on the performance of J. vulgaris and indirect effects via changes in soil nutrients or changes in fungal community composition. Plant species richness was included as a fixed continuous factor to incorporate the continuity of plant species richness in the analysis. We examined in separate models the “field soil” treatment, the “live inoculums” treatment, and the “sterilized inoculums” treatment and did this separately for root and shoot biomass. For multivariate variables (soil nutrient composition, soil fungal TRF composition) the first axis of a Principle Component Analysis (PCA) or Correspondence Analysis (CA) respectively was used in the SEM analysis. Plant biomass was log transformed prior to the SEM analysis. All variables used in the SEM were observed variables. Structural equation modeling was carried out using the lavaan package in R. All final models provided good fit to the data (Supplementary Table S4). Additional information about the SEM procedure is presented in the Supporting information (Supplementary Table S4).
Results
Soil Legacy Bioassay
There was a significant effect of the three soil treatments on shoot (Table 1 and Figure 2) and root biomass of J. vulgaris (Table 1 and Figure 2). Plants produced least shoot biomass when grown in field soil and the highest shoot biomass in sterilized background soil inoculated with sterilized inoculum independent of the plant diversity treatment (Table 1 and Figure 2). Root biomass was also lowest in pure field soil (Table 1 and Figure 2). When bare plots were excluded from the analyses, root biomass was higher in the soil of high diverse plant communities but this was only true for the pure field soil treatment (significant interaction; Table 1 and Figure 2). Shoot biomass also tended to increase in soils originating from plots with higher plant diversity but this effect was not significant (p = 0.077; Table 1 and Figure 2). Biomass of J. vulgaris did not differ significantly between soils from different monocultures (Supplementary Figure S2 and Supplementary Table S2). Root and shoot biomass of the potted plants was larger in legume soils than in grass or forb soils but only in pots with pure field soil (Supplementary Figure S3).
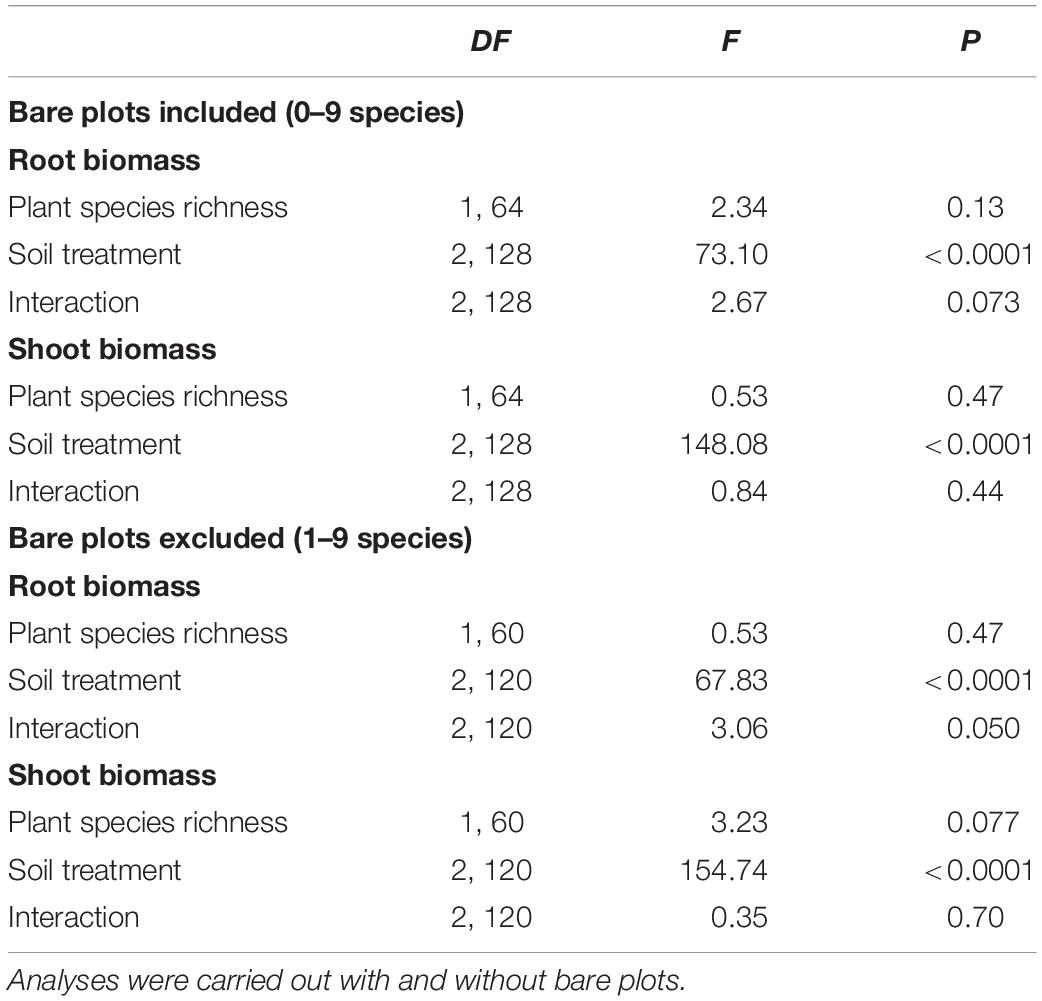
Table 1. Effects of plant species richness, soil conditioning treatment, and their interaction on root and shoot biomass of J. vulgaris plants in the pot experiment.
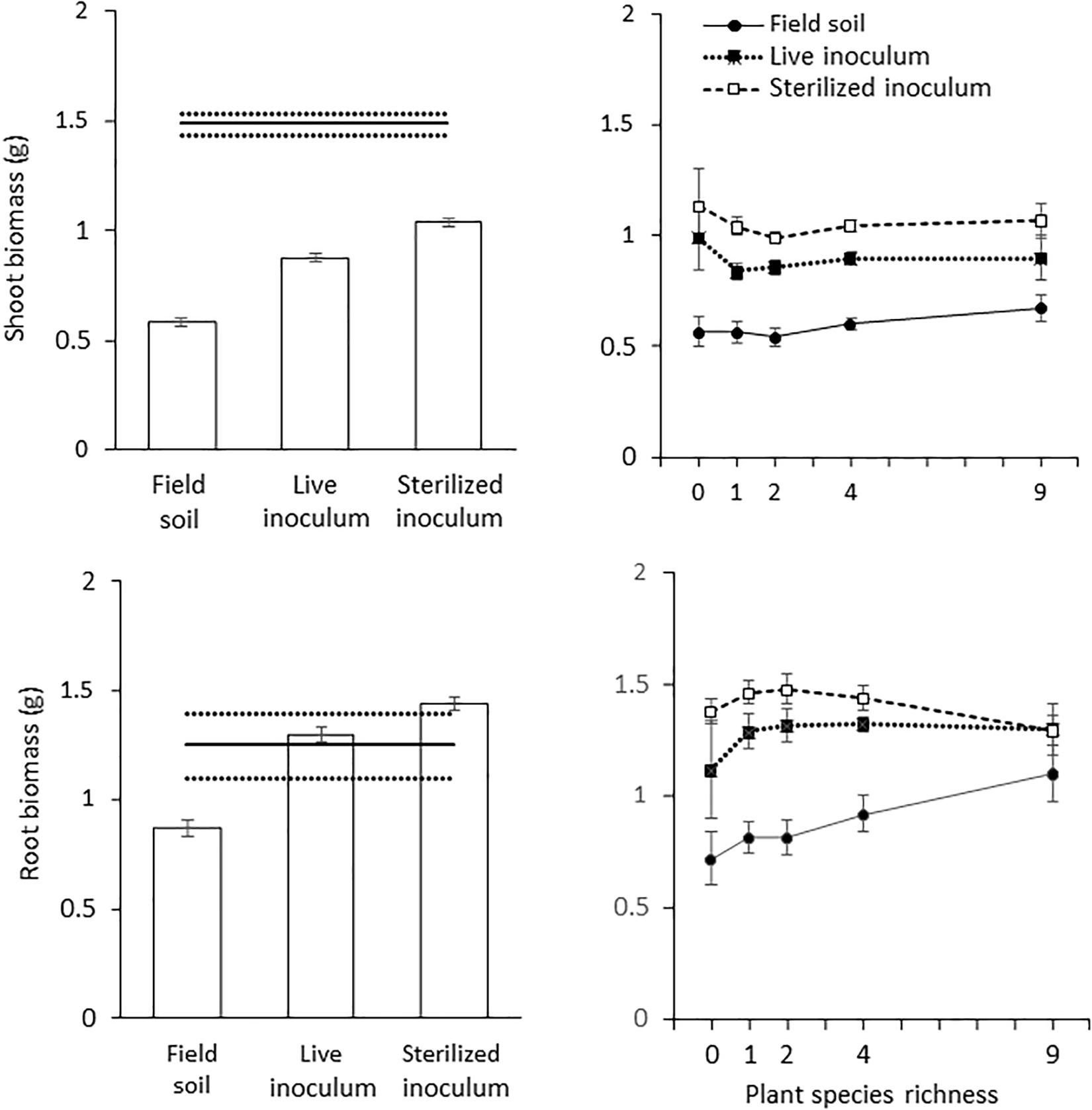
Figure 2. Response of J. vulgaris to plant diversity. Shown are means (± SE) of all diversity levels (left panels) of shoot biomass (top panels) and root biomass (bottom panels) of J. vulgaris plants growing in pure field soil, in sterilized background soil mixed with live or sterilized inoculum; and for each diversity level (right panels). The solid black line with dashed lines represents the biomass (mean ± SE) of plants grown in sterilized background soil.
Soil Characteristics
The composition of the fungal community was not affected by the diversity of the plant community growing in the soil (pseudo-F = 1.1, P = 0.141; Figure 3). However, there was a clear discrimination of the fungal community composition of the bare plots, the fungal community composition of the low diversity plots (with one and two plant species), and the fungal communities of the highest diversity plots (with four and nine plant species; Figure 3). The fungal composition varied between monocultures (pseudo-F = 1.1; P = 0.004; Supplementary Figure S4). Individual soil abiotic characteristics did not vary significantly between the diversity treatments or among monocultures (Figure 3 and Supplementary Table S3). Multivariate analyses (RDA), however, showed that the composition of the soil abiotic characteristics varied significantly between monocultures (F = 1.80; P = 0.019), but not between diversity levels (Supplementary Figures S5, S6).
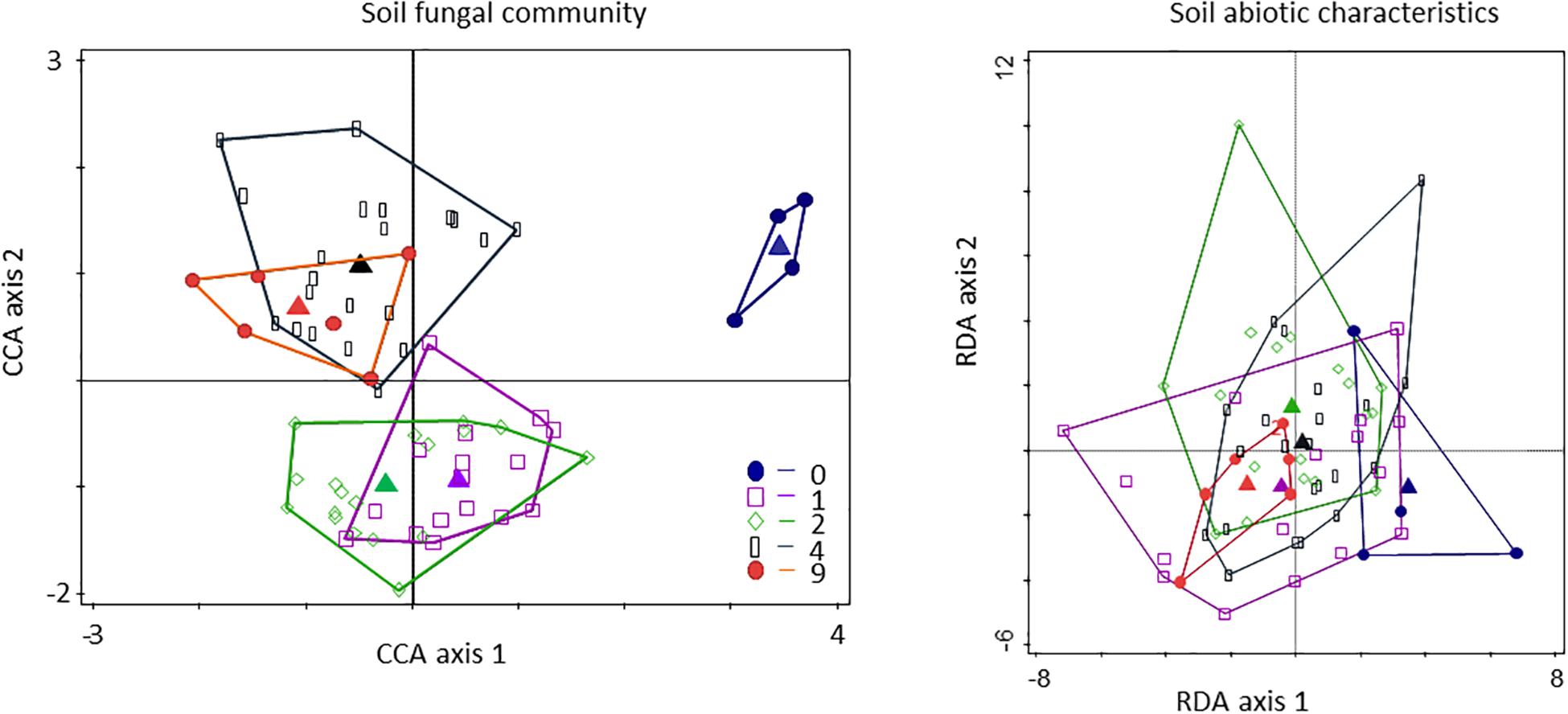
Figure 3. Classified plot diagram (CCA) of soil fungi and soil abiotic characteristics (RDA) depicturing the separation of plots defined by the plant diversity treatment. For fungi score scaling is focused on T-RFLP scores. Symbols represent the individual plots (blue circles – plots without sown vegetation; purple squares – one species plots; green diamonds – two species plots; black rectangles – four species plots; red circle – nine species plots). Triangles represent the average relative composition of plots belonging to individual levels of a plant diversity (0, 1, 2, 4, 9 plant species). The distance between the triangles approximates the average dissimilarity of the plots with a certain diversity level as measured by their chi-square distance.
Relationship Between J. vulgaris Performance in Field Plots and in Pots
Shoot and root biomass of field-grown plants generally declined with increasing plant diversity but this was mainly due to the large size of plants in the bare plots where there was no competition with other plants (Supplementary Figure S7). The shoot biomass of J. vulgaris plants grown in field soil in the greenhouse positively correlated with the shoot biomass of the plants grown in the field (Table 2 and Figure 4), but this correlation was not significant for plots with the highest level of diversity (Figure 4). There was no relationship between the biomass of field-grown plants and plant growth in pots with inoculated field soil (Table 2).
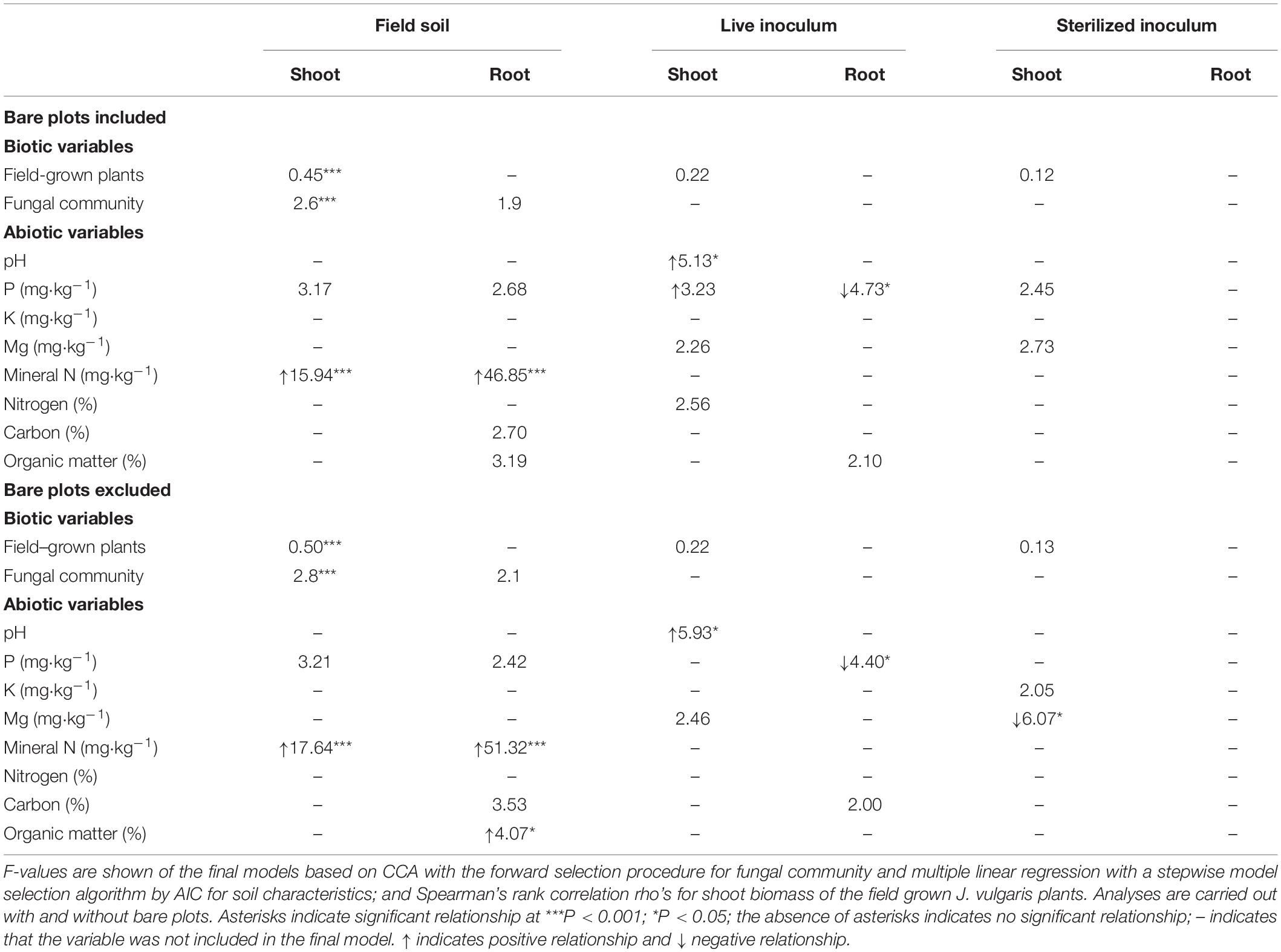
Table 2. Relationship between the performance of plants (root and shoot biomass) from the three soil treatments (field soil; live inoculum; sterilized inoculum), and performance of plants grown in the field experiment, fungal community composition, and soil nutrient composition.
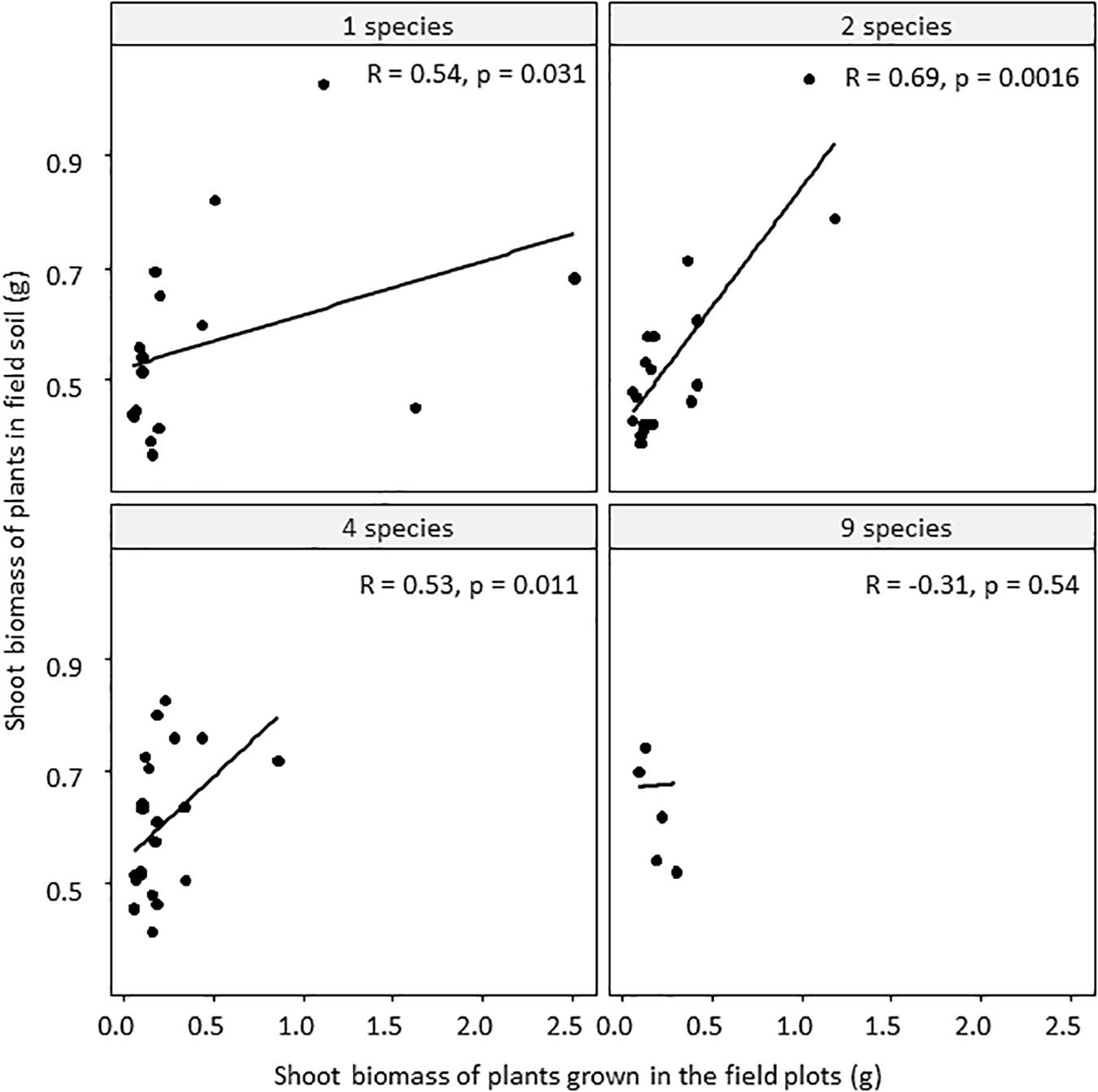
Figure 4. Relationships between shoot biomass of plants grown in the field soil treatment and plants grown in the field in the biodiversity plots, for the different levels of diversity (1, 2, 4, or 9 plant species). R is the Spearman rank correlation coefficient, p-values are based on a Spearman rank correlation test.
Direct and Indirect Effects of Biotic and Abiotic Soil Characteristics on Plant Growth
The shoot biomass of potted plants grown in pure field-conditioned soils significantly correlated with the fungal community composition and with soil nutrients (Table 2). The SEM analysis revealed that soil abiotic and biotic characteristics were related. In the field soil treatment, shoot biomass of potted plants was related to plant diversity and fungal community composition (Figure 5). Root biomass of potted plants grown in field soil, instead, was only influenced by soil abiotic characteristics (Figure 5). Plant biomass in the inoculated soils was not influenced by abiotic or biotic soil characteristics nor by plant diversity, except for an unexpected relationship between soil fungal composition and shoot biomass in the sterilized inoculum treatment (Supplementary Figure S8).
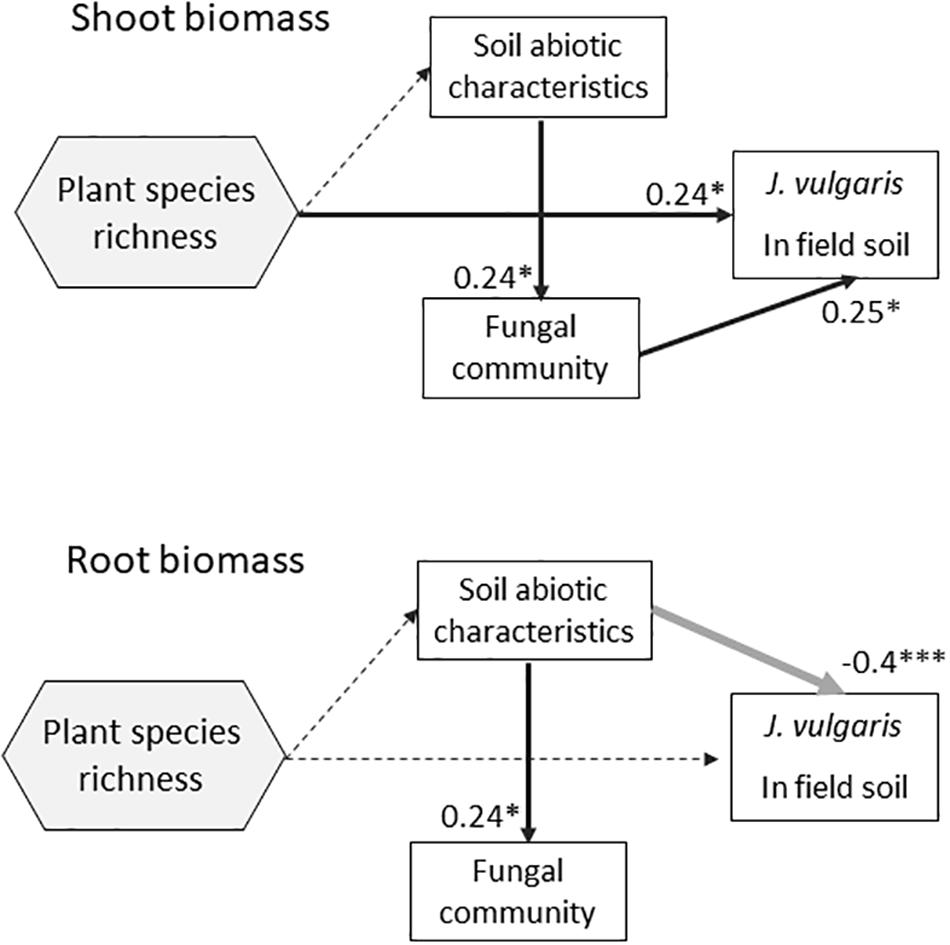
Figure 5. Structural equation model testing the direct and indirect effects (mediated by the changes in soil abiotic characteristics or soil fungal community composition) of plant diversity on J. vulgaris root and shoot biomass in pots with pure field soil. Soil was collected from the field experiment with plots with plant communities that differed in diversity (0, 1, 2, 4, 9 species). Plant species richness was included as fixed continuous factor. For soil abiotic and fungal communities we included the first axis of a multivariate analysis of all soil abiotic characteristics or fungal TRF peaks respectively. The arrows show significant relationships (black = positive, gray = negative), dashed arrows indicate non-significant relationships. The explained variance is also presented and the significance (∗P < 0.05; ∗∗∗P < 0.001).
Relationship Between J. vulgaris Performance in Pots and Cover of Sown Species in the Field
The biomass of potted J. vulgaris plants grown in field soil negatively correlated with the cover of several plant species in the plots from which the soil was collected (particularly, Achillea millefolium, Hypochaeris radicata, and Leucanthemum vulgare), but this relationship was not significant for plants grown in inoculated soils (Supplementary Figures S9, S10).
Discussion
In this study, we examined the soil legacy effects of plant diversity on the focal plant J. vulgaris. With our design, we tested the biotic and the overall soil-mediated effects of plant diversity on plant growth of J. vulgaris, and compared this to the performance of J. vulgaris planted in communities with different diversity levels. We observed that in the pot experiment there was a trend for a positive legacy effect of the diversity of the plant community on J. vulgaris root biomass, but this effect was only present in the field soil treatment. Similar results were observed in a study where genetic diversity of a single species was manipulated (Luo et al., 2016). That study focused on effects of inoculated soil and the authors concluded that dilution of pathogens was the likely mechanism (Luo et al., 2016). Other studies have shown that high species diversity reduces the inhibitory effects of soil biota in plant communities (Hendriks et al., 2013; Yang et al., 2015; Guerrero-Ramírez et al., 2019).
One of the questions that we set out to examine is how the diversity-soil legacy relationship depends on the sterilization or inoculation treatments. The results show that the diversity effect was only significant in the pure field soil treatment, and not in the inoculated treatments. Hence, our study provides no support for the role of soil biota in the legacy effects of plant diversity. It is interesting to note that sterilization of soil from high diverse plant communities (nine plant species) did not affect the root biomass of J. vulgaris while sterilization of soil from the communities with lower plant diversity (1, 2, or 4 plant species) enhanced J. vulgaris root biomass (Supplementary Table S5), similar to the results reported by Luo et al. (2016). This indicates that suppressive (biotic) effects may have been present in the low diversity soils but further research is needed to confirm this.
In our study, plant biomass was higher in pots with soil from plots in which only legumes had grown but this was only true in pots filled with pure field soil and not in the inoculated soil. Legumes live in symbiosis with nitrogen fixing rhizobacteria and we hypothesize that the observed effects were due to nitrogen availability, even though we did not detect differences in nitrogen availability in the soil chemical analysis among monocultures.
Soil legacies can be due to a myriad of changes in the soil that are caused by the first plant or plant community and then influence a second plant (Kulmatiski et al., 2012; Wurst et al., 2015). In this study, we examined the plant-diversity mediated effects on soil fungal composition and soil chemical properties. We expected that the build-up of species-specific fungal pathogens would be high in monocultures and be diluted with increasing plant diversity and that these pathogenic fungi would also negatively affect J. vulgaris at high densities in the soil. Hence, we expected a positive relationship between biomass of J. vulgaris and plant diversity. Earlier studies have shown that soil legacy effects on J. vulgaris can be explained by soil fungal composition (Bezemer et al., 2006; Wang et al., 2019). In our study, soil fungal community composition was not strongly affected by the diversity of the plant community but it was significantly related to the biomass of J. vulgaris when the plants were grown in the pure field soil. However, plant biomass was also positively related to nitrogen availability in the soil, highlighting that it is unlikely that soil legacy effects on plant growth can be explained by a single factor, and that most likely it is a combination of many components that change in the soil (e.g., changes in soil fungi and changes in nitrogen availability). It is important to note that the abundance of specific fungal species or groups of fungi in the soil will be much more important for plant growth than the composition of the entire fungal community. Future studies, therefore, should examine the effects of different fungi on plant growth and the absolute abundance of these fungi in the soil.
The diversity of the plant community can greatly affect plant performance (Tilman, 1997; Scherber et al., 2003; Agrawal, 2004) and these effects can be driven by changes in the soil (Maron et al., 2011; Kulmatiski et al., 2012). Interestingly, and in contrast to the potted plants, in the field, the growth of J. vulgaris plants tended to be negatively related to the diversity of the surrounding plant community, however, this was mainly due to high biomass in the bare plots. Previous work on J. vulgaris has shown that this ruderal species is strongly negatively affected by interspecific plant competition (McEvoy et al., 1993). Clearly, the J. vulgaris plants in the field were influenced by the effects of neighboring plants both above- and belowground on light and space availability, as well as by the changes in soil characteristics such as nutrient availability or e.g., soil pathogens caused by these neighbors. Our results indicate that plant diversity may negatively affect the performance of new colonizing plant species, i.e., invaders, that establish in these communities, as shown by other studies (e.g., Hooper et al., 2005; Maron and Marler, 2008). However, for J. vulgaris this is mainly due to increased competition with other plants in more diverse communities, probably due to an increase in overall plant density in more diverse communities as a result of more complete use of resources due to niche differences between species (Scherber et al., 2010; Sun et al., 2014). Evidence from our study suggests that this diversity effect is not due to soil pathogens. It is important to note that we measured the performance of J. vulgaris plants at the rosette stage both in the field and in the greenhouse experiment and it is possible that the soil legacy effects of plant diversity will be greater when plants reach the flowering stage (Dudenhöffer et al., 2018).
An important question is whether soil mediated effects measured in potted plants can be extrapolated to plant growth in the same soil under field conditions (Heinze et al., 2016). In this study, we detected several relationships between growth of potted and plants that grew in the field plots. While this suggests that soil factors can explain the growth of J. vulgaris and that results from potted experiments can be extrapolated to plant performance in the field, we note that there are many differences between plants grown in pots and plants grown in field plots and that comparisons should be made with great caution.
We did not detect differences between the effects of the monoculture soils on plant performance. Several other studies carried out with soil collected from the same area have shown that the performance of J. vulgaris depends greatly on the identity of the species that grew previously in the soil. Some of these studies tested this with soil collected from potted plants (e.g., van de Voorde et al., 2011), but others have shown such effects with field collected soil from monocultures (e.g., Kos et al., 2015). It is possible that the period of 2 years of conditioning prior to collecting the soil for the current experiment was too short, so that the plants had not altered the soil sufficiently. The experimental plots were established in an area with a relatively homogeneous plant community consisting of roughly 12 species per m2. The legacy effects of this previous plant community may still have been present in the soil and this may explain why we did not observe strong soil legacy effects. However, the analyses of soil abiotic characteristics and the soil fungi in the monocultural plots shows that there are clear differences among the soils from the different plots. Although we did not find strong differences in performance of J. vulgaris in the different monoculture soils, we did observe a negative relationship between the abundance of three of the sown forb species in the field and the performance of J. vulgaris in the soil legacy bioassay. However, as this effect was not detected in the monoculture soils, these results should be interpreted with caution.
In conclusion, our study provides only weak evidence that plant diversity-mediated soil legacies influence the performance of J. vulgaris. Hence, the importance of soil legacies in influencing plants in the field is still debatable.
Data Availability Statement
The datasets generated for this study are available on request to the corresponding author.
Author Contributions
OK and MB conceived the original idea, conducted experiments, analyzed the data, and wrote the manuscript.
Funding
This study was funded by the Netherlands Organization for Scientific Research (NWO, VIDI Grant No 864.07.009 and VICI Grant No 865.14.006). This is publication no. 6947 of the Netherlands Institute of Ecology (NIOO-KNAW).
Conflict of Interest
The authors declare that the research was conducted in the absence of any commercial or financial relationships that could be construed as a potential conflict of interest.
Acknowledgments
We thank Saskia Grootemaat for assistance with the field and greenhouse work, and Henk Martens for assistance with the molecular work.
Supplementary Material
The Supplementary Material for this article can be found online at: https://www.frontiersin.org/articles/10.3389/fevo.2020.00087/full#supplementary-material
References
Agrawal, A. A. (2004). Resistance and susceptibility of milkweed: competition, root herbivory, and plant genetic variation. Ecology 85, 2118–2133. doi: 10.1890/03-4084
Bennett, J. A., and Klironomos, J. (2018). Mechanisms of plant-soil feedback: interactions among biotic and abiotic drivers. New Phytol. 222, 91–96. doi: 10.1111/nph.15603
Bennett, J. A., Koch, A. M., Forsythe, J., Johnson, N. C., Tilman, D., and Klironomos, J. (2019). Resistance of soil biota and plant growth to disturbance increases with plant diversity. Ecology Lett. 23, 119–128. doi: 10.1111/ele.13408
Bever, J. D. (2003). Soil community feedback and the coexistence of competitors: conceptual frameworks and empirical tests. New Phytol. 157, 465–473. doi: 10.1046/j.1469-8137.2003.00714.x
Bever, J. D., Dickie, I. A., Facelli, E., Facelli, J. M., Klironomos, J., Moora, M., et al. (2010). Rooting theories of plant community ecology in microbial interactions. Trends Ecol. Evol. 25, 468–478. doi: 10.1016/j.tree.2010.05.004
Bezemer, T. M., Fountain, M., Barea, J., Christensen, S., Dekker, S., Duyts, H., et al. (2010). Divergent composition but similar function of soil food webs of individual plants: plant species and community effects. Ecology 91, 3027–3036. doi: 10.1890/09-2198.1
Bezemer, T. M., Harvey, J. A., Kowalchuk, G. A., Korpershoek, H., and van der Putten, W. H. (2006). Interplay between Senecio jacobaea and plant, soil, and aboveground insect community composition. Ecology 87, 2002–2013. doi: 10.1890/0012-9658(2006)87[2002:ibsjap]2.0.co;2
Cheng, D., Kirk, H., Mulder, P. P. J., Vrieling, K., and Klinkhamer, P. G. L. (2011). Pyrrozidine alkaloid variation in shoots and roots of segregating hybrids between Jacobaea vulgaris and Jacobaea aquatica. New Phytol. 192, 1010–1023. doi: 10.1111/j.1469-8137.2011.03841.x
Dudenhöffer, J.-H., Ebeling, A., Klein, A.-M., and Wagg, C. (2018). Beyond biomass: soil feedbacks are transient over plant life stages and alter fitness. J. Ecol. 106, 230–241. doi: 10.1111/1365-2745.12870
Ehrenfeld, J. G., Ravit, B., and Elgersma, K. (2005). Fedback in the plant-soil system. Ann. Rev. Environ. Resour. 30, 75–115. doi: 10.1146/annurev.energy.30.050504.144212
Eisenhauer, N., Bessler, H., Engels, C., Gleixner, G., Habekost, M., Milcu, A., et al. (2010). Plant diversity effects on soil microorganisms support the singular hypothesis. Ecology 91, 485–496. doi: 10.1890/08-2338.1
Gardes, M., and Bruns, T. D. (1993). ITS primers with enhanced specificity for basidiomycetes - application to the identification of mycorrhizae and rusts. Mol. Ecol. 2, 113–118. doi: 10.1111/j.1365-294X.1993.tb00005.x
Guerrero-Ramírez, N. R., Reich, P. B., Wagg, C., Ciobanu, M., and Eisenhauer, N. (2019). Diversity-dependent plant–soil feedbacks underlie long-term plant diversity effects on primary productivity. Ecosphere 10:e02704. doi: 10.1002/ecs2.2704
Harper, J. L., and Wood, W. A. (1957). Biological flora of the british isles: Senecio jacobaea L. J. Ecol. 45, 617–637. doi: 10.2307/2256946
Heinen, R., Van der Sluis, M., Biere, A., Harvey, J. A., and Bezemer, T. M. (2018). Plant community composition but not plant traits determine the outcome of soil legacy effects on plants and insects. J. Ecol. 106, 1217–1228. doi: 10.1111/1365-2745.12907
Heinze, J., Sitte, M., Schindhelm, A., Wright, J., and Joshi, J. (2016). Plant-soil feedbacks: a comparative study on the relative importance of soil feedbacks in the greenhouse versus the field. Oecologia 181, 559–569. doi: 10.1007/s00442-016-3591-8
Hendriks, M., Mommer, L., de Caluwe, H., Smit-Tiekstra, A. E., Van der Putten, W. H., and De Kroon, H. (2013). Independent variations of plant and soil mixtures reveal soil feedback effects on plant community overyielding. J. Ecol. 101, 287–297. doi: 10.1111/1365-2745.12032
Hooper, D. U., Chapin, F. S., Ewel, J. J., Hector, A., Inchausti, P., Lavorel, S., et al. (2005). Effects of biodiversity on ecosystem functioning: a consensus of current knowledge. Ecol. Monogr. 75, 3–35. doi: 10.1890/04-0922
Jing, J., Bezemer, T. M., and Van der Putten, W. H. (2015). Interspecific competition of early successional plant species in ex-arable fields as influenced by plant-soil feedback. Basic Appl. Ecol. 16, 112–119. doi: 10.1016/j.baae.2015.01.001
Kardol, P., Bezemer, T. M., and Van der Putten, W. H. (2006). Temporal variation in plant-soil feedback controls succession. Ecol. Lett. 9, 1080–1088. doi: 10.1111/j.1461-0248.2006.00953.x
Kos, M., Tuijl, M. A. B., de Roo, J., Mulder, P. P. J., and Bezemer, T. M. (2015). Species-specific plant-soil feedback effects on above-ground plant-insect interactions. J. Ecol. 103, 904–914. doi: 10.1111/1365-2745.12402
Kostenko, O., Grootemaat, S., Van der Putten, W. H., and Bezemer, T. M. (2012). Effects of diversity and identity of the neighbouring plant community on the abundance of arthropods on individual ragwort (Jacobaea vulgaris) plants. Entomol. Exp. Appl. 144, 27–36. doi: 10.1111/j.1570-7458.2012.01251.x
Kulmatiski, A., Beard, K. H., and Heavilin, J. (2012). Plant–soil feedbacks provide an additional explanation for diversity–productivity relationships. Proc. R. Soc. B Biol. Sci. 279, 3020–3026. doi: 10.1098/rspb.2012.0285
Kulmatiski, A., Beard, K. H., Stevens, J. R., and Cobbold, S. M. (2008). Plant-soil feedbacks: a meta-analytical review. Ecol. Lett. 11, 980–992. doi: 10.1111/j.1461-0248.2008.01209.x
Lange, M., Eisenhauer, N., Sierra, C. A., Bessler, H., Engels, C., Griffiths, R. I., et al. (2015). Plant diversity increases soil microbial activity and soil carbon storage. Nat. Commun. 6:6707. doi: 10.1038/ncomms7707
Luo, W., Callaway, R. M., and Atwater, D. Z. (2016). Intraspecific diversity buffers the inhibitory effects of soil biota. Ecology 97, 1913–1918. doi: 10.1002/ecy.1469
Maron, J. L., and Marler, M. (2008). Effects of Native species diversity and resource additions on invader impact. Am. Nat. 172, S18–S33. doi: 10.1086/588303
Maron, J. L., Marler, M., Klironomos, J. N., and Cleveland, C. C. (2011). Soil fungal pathogens and the relationship between plant diversity and productivity. Ecol. Lett. 14, 36–41. doi: 10.1111/j.1461-0248.2010.01547.x
McEvoy, P. B., Rudd, N. T., Cox, C. S., and Huso, M. (1993). Disturbance, competition, and herbivory effects on ragwort Senecio jacobaea populations. Ecol. Monogr. 63, 55–75. doi: 10.2307/2937123
Nelson, D. E., and Sommers, L. E. (1982). “Total carbon, organic carbon, and organic matter,” in Methods of Soil Analysis, ed. A. L. Page (Madison: American Society of Agronomy), 539–580.
Olsen, S., Cole, C., Watanabe, F., and Dean, L. (1954). Estimation of Available Phosphorus In Soils By Extraction With Sodium Bicarbonate. Washington, D.C: United States Government Publishing Office.
Perkins, L. B., and Nowak, R. S. (2013). Native and non-native grasses generate common types of plant-soil feedbacks by altering soil nutrients and microbial communities. Oikos 122, 199–208. doi: 10.1111/j.1600-0706.2012.20592.x
Petermann, J. S., Fergus, A. J. F., Turnbull, L. A., and Schmid, B. (2008). Janzen-Connell effects are widespread and strong enough to maintain diversity in grasslands. Ecology 89, 2399–2406. doi: 10.1890/07-2056.1
R Development Core Team (2017). R: A Language and Environment for Statistical Computing. Vienna: R Foundation for Statistical Computing.
Reynolds, H. L., Packer, A., Bever, J. D., and Clay, K. (2003). Grassroots ecology: plant-microbe-soil interactions as drivers of plant community structure and dynamics. Ecology 84, 2281–2291. doi: 10.1890/02-0298
Scherber, C., Crawley, M. J., and Porembski, S. (2003). The effects of herbivory and competition on the invasive alien plant Senecio inaequidens (Asteraceae). Divers. Distrib. 9, 415–426. doi: 10.1046/j.1472-4642.2003.00049.x
Scherber, C., Eisenhauer, N., Weisser, W. W., Schmid, B., Voigt, W., Fischer, M., et al. (2010). Bottom-up effects of plant diversity on multitrophic interactions in a biodiversity experiment. Nature 468, 553–556. doi: 10.1038/nature09492
Schnitzer, S. A., Klironomos, J. N., HilleRisLambers, J., Kinkel, L. L., Reich, P. B., Xiao, K., et al. (2011). Soil microbes drive the classic plant diversity–productivity pattern. Ecology 92, 296–303. doi: 10.1890/10-0773.1
Shipley, B. (2002). Cause and Correlation in Biology: A User’s Guide to Path Analysis, Structural Equations and Causal Inference. Cambridge: Cambridge University Press.
Šmilauer, P., and Lepš, J. (2014). Multivariate Analysis of Ecological Data Using CANOCO 5. Cambridge: Cambridge University Press.
Sun, Y., Müller-Schärer, H., and Schaffner, U. (2014). Plant neighbours rather than soil biota determine impact of an alien plant invader. Funct. Ecol. 28, 1545–1555. doi: 10.1111/1365-2435.12295
Tilman, D. (1997). Community invasibility, recruitment limitation, and grassland biodiversity. Ecology 78, 81–92. doi: 10.1890/0012-9658(1997)078[0081:cirlag]2.0.co;2
van de Voorde, T. F. J., Van der Putten, W. H., and Bezemer, T. M. (2011). Intra- and interspecific plant–soil interactions, soil legacies and priority effects during old-field succession. J. Ecol. 99, 945–953. doi: 10.1111/j.1365-2745.2011.01815.x
van der Putten, W. H., Bardgett, R. D., Bever, J. D., Bezemer, T. M., Casper, B. B., Fukami, T., et al. (2013). Plant-soil feedbacks: the past, the present and future challenges. J. Ecol. 101, 265–276. doi: 10.1111/1365-2745.12054
Wang, M., Ruan, W., Kostenko, O., Carvalho, S., Hannula, S. E., Mulder, P. P. J., et al. (2019). Removal of soil biota alters soil feedback effects on plant growth and defense chemistry. New Phytol. 221, 1478–1491. doi: 10.1111/nph.15485
Wardle, D. A., Bardgett, R. D., Klironomos, J. N., Setala, H., van der Putten, W. H., and Wall, D. H. (2004). Ecological linkages between aboveground and belowground biota. Science 304, 1629–1633. doi: 10.1126/science.1094875
Wardle, D. A., Yeates, G. W., Williamson, W., and Bonner, K. I. (2003). The response of a three trophic level soil food web to the identity and diversity of plant species and functional groups. Oikos 102, 45–56. doi: 10.1034/j.1600-0706.2003.12481.x
White, T. J., Bruns, T. D., Lee, S. B., and Taylor, J. W. (1990). “Analysis of phylogenetic relationships by amplification and direct sequencing of ribosomal DNA genes,” in PCR Protocols: A Guide to Methods and Applications, ed. M. A. E. A. Innis (San Diego: Academic Press), 315–322. doi: 10.1016/b978-0-12-372180-8.50042-1
Wurst, S., Kaiser, N., Nitzsche, S., Haase, J., Auge, H., and Rillig, M. C. (2015). Tree diversity modifies distance-dependent effects on seedling emergence but not plant–soil feedbacks of temperate trees. Ecology 96, 1529–1539. doi: 10.1890/14-1166.1
Keywords: biodiversity, Jacobaea vulgaris, plant-soil feedback, soil biota, plant species richness, soil fungi, T-RFLP
Citation: Kostenko O and Bezemer TM (2020) Abiotic and Biotic Soil Legacy Effects of Plant Diversity on Plant Performance. Front. Ecol. Evol. 8:87. doi: 10.3389/fevo.2020.00087
Received: 14 June 2019; Accepted: 17 March 2020;
Published: 03 April 2020.
Edited by:
Justin Wright, Duke University, United StatesReviewed by:
Lora B. Perkins, South Dakota State University, United StatesJana S. Petermann, University of Salzburg, Austria
Copyright © 2020 Kostenko and Bezemer. This is an open-access article distributed under the terms of the Creative Commons Attribution License (CC BY). The use, distribution or reproduction in other forums is permitted, provided the original author(s) and the copyright owner(s) are credited and that the original publication in this journal is cited, in accordance with accepted academic practice. No use, distribution or reproduction is permitted which does not comply with these terms.
*Correspondence: T. Martijn Bezemer, dC5tLmJlemVtZXJAYmlvbG9neS5sZWlkZW51bml2Lm5s