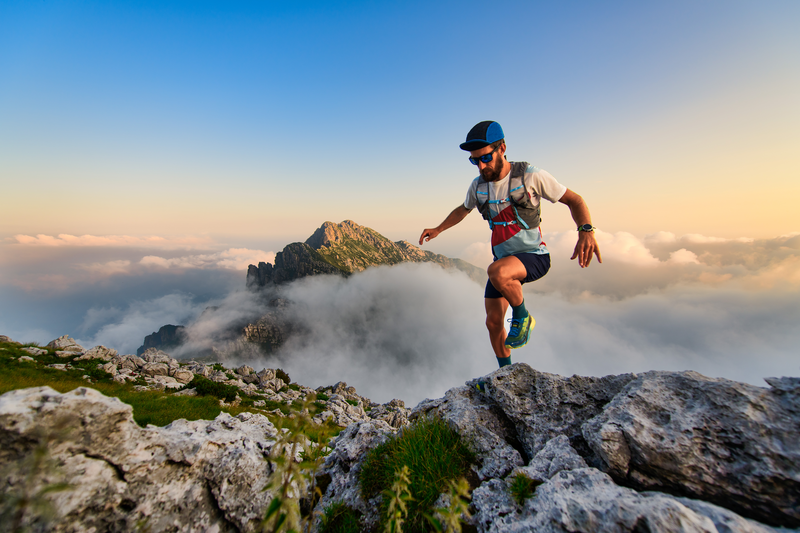
94% of researchers rate our articles as excellent or good
Learn more about the work of our research integrity team to safeguard the quality of each article we publish.
Find out more
ORIGINAL RESEARCH article
Front. Ecol. Evol. , 21 April 2020
Sec. Biogeography and Macroecology
Volume 8 - 2020 | https://doi.org/10.3389/fevo.2020.00066
This article is part of the Research Topic Temporal Patterns and Mechanisms of Biodiversity Across Scales in East Asia View all 12 articles
Extensive grazing activity is threatening the alpine grassland of the Qinghai-Tibetan Plateau. Evidence has shown that grazing exclusion may change the composition, structure, and functions of grassland ecosystems. However, such effects depend on the intensity and duration of exclusion. We explored the effects of short-term (2 and 4 years) and long-term (9 and 11 years) grazing exclusion on plant height, coverage, and diversity and community heterogeneity in the alpine grassland of the Qinghai-Tibetan Plateau. We found no difference in plant diversity between short-term grazing exclusion and control. However, long-term grazing exclusion reduced species richness and increased the Simpson dominance index. This decrease in plant species richness was mainly attributable to the decrease in common species richness (defined as species with a relative coverage of 1∼5%). In addition, community heterogeneity (coefficient of variation, CV) was significantly higher in long-term grazing exclusion than in controlled plots. Structural equation modeling (SEM) demonstrated that long-term grazing exclusion increased the community heterogeneity mainly by reducing species diversity. These results suggest that the effects of grazing exclusion on the composition, structure, and community spatial heterogeneity of the alpine grassland ecosystem are dependent on exclusion duration. Grazing activity may maintain the high biodiversity and community stability of the alpine grassland in the harsh environment of the Qinghai-Tibetan Plateau.
Spatial heterogeneity, or spatial variability (Kolasa and Rollo, 1991), represents the dissimilarity (coefficient of variation) of community properties between multiple subplots within one survey plot (Huston, 1997; Weigelt et al., 2008). Because of the impact of spatial heterogeneity on grassland ecosystem functioning (e.g., productivity) (Huston, 1997; Fukami et al., 2001), uncovering the underlying mechanisms that cause spatial heterogeneity is a necessary step to predicting the change of ecosystem functions under global changes (Naeem et al., 1994; Weigelt et al., 2008; Grman et al., 2010). Many studies have shown that the more diverse communities have a higher probability of maintaining species and being resistant to environmental changes, i.e., more temporally stable (Tilman et al., 2006; Weigelt et al., 2008; Loreau and De Mazancourt, 2013). However, little is known about how spatial variability changes under biodiversity loss. The heterogeneity of the microclimate, such as plant coverage, has been shown to be significantly and positively correlated with biodiversity (Xu et al., 2000). Furthermore, Fukami et al. (2001) showed that biodiversity loss lowers ecosystem reliability (stability) between local communities by increasing the dissimilarity of species compositions, which indicates that biodiversity loss may lead to spatial heterogeneity among communities.
Several mechanisms have been proposed to explain community temporal stability, including compensatory dynamics (Bai et al., 2004; Song and Yu, 2015; Wilcox et al., 2017) and dominant species effects (Polley et al., 2007; Sasaki and Lauenroth, 2011; Wilsey et al., 2014). Given that the hypothesis that biodiversity increases stability can equally be applied to spatial heterogeneity (Fukami et al., 2001; Weigelt et al., 2008), these mechanisms should be applied to explain the causes of community heterogeneity. Compensatory dynamics (e.g., species asynchrony) indicates the ability of species to supplement each other under environmental changes, and higher compensatory effects can contribute to higher ecosystem stability, resulting from decreased variation in productivity (Bai et al., 2004; Song and Yu, 2015; Wilcox et al., 2017). Additionally, the stability of the dominant species is closely connected with the stability of the ecosystem, because the dominant species contributes most of the biomass in the community (Polley et al., 2007; Sasaki and Lauenroth, 2011). The effects of species asynchrony and dominant species are tightly correlated with plant diversity (Bai et al., 2004; Polley et al., 2007). Here, we hypothesize that an increase in biodiversity and asynchrony will lead to a decrease in spatial heterogeneity and that an increase in dominant species heterogeneity will lead to an increase in spatial heterogeneity. Besides biotic factors, the spatial heterogeneity of plant communities can also be influenced by abiotic factors, including pH, nutrients, water content of soils, and so on (Augustine and Frank, 2001; Wijesinghe et al., 2005; Zuo et al., 2009; Ulrich et al., 2014). Ulrich et al. (2014) found that soil variables such as pH can alter the small-scale spatial variability of the plant community structure indirectly by changing species richness. Wu et al. (2014) demonstrated that soil water content can regulate plant community productivity in the semi-arid steppes of China.
The alpine grasslands widespread on the Qinghai-Tibetan Plateau play an important role in ecosystem and water security (Su et al., 2015). The Qinghai-Tibetan Plateau is also one of the most important pastoral areas in China, supporting the production of livestock and the lives of local herdsmen. However, extensive grazing can lead to the decline of grassland productivity (Huang et al., 2007), and the alpine grassland ecosystem on the Qinghai-Tibetan Plateau is facing a series of grassland degradation problems, such as declines in biodiversity and stability (Zhou et al., 2005; Liu et al., 2018; Zhao et al., 2019). Grazing exclusion was considered as an effective measure to alleviate the degradation of the alpine grassland on the Tibetan Plateau (Yan and Lu, 2015). Given the effect of grazing exclusion on plant species diversity and soil properties (Liu et al., 2015; Xiong et al., 2016), we hypothesized that the spatial heterogeneity of plant communities under grazing exclusion would be influenced by the change in biotic and abiotic factors. However, the impact that grazing exclusion activities have on spatial heterogeneity remains controversial.
Here, we investigated the grassland abundance (coverage), height, diversity (species richness and Simpson’s dominance index), and soil properties in short-term (2 and 4 years), long-term (9 and 11 years) grazing exclusion and in paired non-exclusion plots to explore the effects of grazing exclusion on species diversity and community spatial heterogeneity and their relationships in the alpine grassland of the Qinghai-Tibetan Plateau. Such knowledge will be important for policy-makers to better formulate policies to manage natural grasslands in this region.
This study was conducted in an alpine meadow near the Haibei National Field Research Station in the Alpine Grassland Ecosystem (37° 36′–37° 37′ N, 101° 18′–101° 19′ E, 3220 m a.s.l.), located in the northeast of the Qinghai-Tibetan Plateau, China (Table 1). This alpine grassland has a continental monsoon climate (Wang et al., 2014; Ma et al., 2017), with a mean annual air temperature of −1.08°C and annual precipitation of 416.8 mm during the past 5 years (Wang et al., 2018). The soil type was identified as Mollisols (Liu et al., 2018). The pH of the surface soil (0–10 cm) was 7.8, and the bulk density was 0.8 g cm–3. The alpine grassland of this area is a winter pasture, and grazing activity occurs approximately from September to May. The grazing type is free-grazing, with a grazing intensity of 5–6 sheep ha–1 yr–1 (Table 1). The dominant species in the investigated sites include Stipa aliena (grasses), Tibetia himalaica (legumes), Saussurea pulchra, and S. nigrescens (non-legume forbs) (Supplementary Table S1).
Table 1. Information on the short-term and long-term grazing plots. Numbers in parentheses indicate standard deviation.
Fenced (no grazing event occurred since being fenced) and paired non-fenced grassland with stands were investigated as grazing exclusion and control treatments, respectively. Four grazing exclusion treatments (approximately 400 m2, 20 m × 20 m plot for each treatment) with different fenced durations (2, 4, 9, and 11 years) were selected and investigated. Among them, 2-year and 11-year grazing exclusion treatments and their controls (Site A, 37° 36′ 46″ N, 101° 18′ 14″ E, 3193 m a.s.l.) were within one block, and 4-year and 9-year grazing exclusion treatment and their controls (Site B, 37° 37′ 2″ N, 101° 19′ 44″ E, 3221 m a.s.l.) were within another block about 3 km away. The grassland areas of these two sites were 11 ha and 17 ha, respectively.
To compare the variation in vegetation characteristics under the short-term and long-term durations of grazing exclusion, we further divided the four grazing exclusion treatments into short-term (2 and 4 years) and long-term (9 and 11 years) exclusion. The control stands were located outside the fenced grasslands (Table 1 and Supplementary Table S2) and had similar community compositions and structures (Supplementary Figure S3). To avoid an edge influence of the fence on the growth of the plants, we randomly investigated five grassland sub-plots (1 m × 1 m) inside and outside the fence (5–10 m from the fence), respectively. We divided the 1-m2 sub-plots into 16 grid cells (25 cm × 25 cm), and four of them were randomly selected for the community investigation.
We investigated species, grass height, and coverage (1 cm × 1 cm grid method, absolute coverage based on the square area) in August 2018. The total coverage of all the species was calculated as the sum of the absolute coverage of each species, and the absolute coverage of each species was divided by total coverage as the relative coverage of the species. During August 2019, we conducted a comprehensive investigation of the communities at site A and site B. We investigated species, grass height, and coverage at 75 plots (50 cm × 50 cm) in each site. The soil samples (0–10 cm) were collected after the community survey (using a 5-cm-diameter soil auger). In each 1-m2 sub-plot, five soil samples were collected and were pooled together. In the laboratory, we measured soil water content (SWC), pH, soil organic content (SOC), and total nitrogen content (TN). Soil water content was measured by the oven-drying method. Air-dried samples were used to measure soil pH (pH meter, PHS-3C, INESA, China) and concentrations of C and N (elemental analyzer, FLASHEA 112 Series, Thermo Electron, United States).
The small size of the observed sub-plots (25 cm × 25 cm) might lead to imprecise estimates. To reduce this uncertainty, we performed analysis of species-area relationships. The results showed that the observed numbers of the smallest sub-plots (25 cm × 25 cm) could account for 41% (24/59) and 44% (25/57) for the whole area of site A and site B, respectively (Supplementary Figure S10). In addition, we compared the relationships of species richness and plot area between the two sites but found no statistical difference between these two sites.
According to Ma et al. (2017) and Liu et al. (2018), the plants in alpine grassland are divided into four functional groups, namely grasses, sedges, legumes, and non-legume forbs (Supplementary Table S1). The plants of the grassland community were divided into three categories according to relative dominance, including dominant species (greater than 5% of the relative coverage), common species (between 1 and 5%), and rare species (less than 1%) (Mariotte et al., 2013). As a result, four dominant species, 17 common species, and 25 rare species were identified in this study (Supplementary Table S1).
Species richness is the number of species in four cells. The Simpson dominance index (D) is calculated according to Eq. (1):
where ps is the relative coverage of each species (s) (Smith and Wilson, 1996).
The spatial heterogeneity of the community (and of differently dominant species) was calculated as the standard deviation of the total (and differently dominant species) coverage of four cells (σ) divided by the mean value (μ) (coefficient of variation (CV); Weigelt et al., 2008). Asynchrony of species (all species and dominant species in the community) were calculated according to Eq. (2):
where σi is the standard deviation of the relative coverage of species (i), σ is the s.d. of community coverage, and φx is species synchrony (Loreau and De Mazancourt, 2008).
One-way analysis of covariance (ANOVA) was used to test the differences in plant community characteristics (coverage, height, species richness, and Simpson’s dominance index), soil properties (pH, SWC, SOC, TN), and community properties (community spatial heterogeneity, dominant species spatial heterogeneity, and species asynchrony) among the different treatments (short-term and long-term grazing exclusion and their control stands). Pearson correlation analysis was used to test the correlation between factors (biotic and abiotic) (Supplementary Figure S1). Non-metric multidimensional scaling (NMDS) was used to analyze the structural differences among the communities (Supplementary Figure S3). First, the height data of each species were dimension-reduced to obtain the community structure under different treatments, and then an analysis of similarity (ANOSIM) was performed using the “vegan” package of R software to examine the differences in community structure between each of the two treatments. If R = 0 (R is the statistical value measuring the differences between and within groups, ranging from −1 to + 1), then the community structure between treatments was similar. If R > 0 and P < 0.05, then the difference in community structure between treatments was significant. Analysis of covariance (ANCOVA) was used to test the existence of the species dominance effect by determining whether the slope and intercept of the regression models were significantly different among the treatments.
To explore the mechanism of grazing exclusion and its duration on plant biodiversity and community spatial heterogeneity, we first used a simple linear regression to analyze the relationship between species diversity, species asynchrony, dominant species spatial heterogeneity, and community spatial heterogeneity. We further fitted a full structural equation model (SEM) (containing all possible pathways) using the “SEM” package of R software to infer the relative importance of species richness, Simpson’s dominance index, species asynchrony, and dominant species spatial heterogeneity on community spatial heterogeneity (Supplementary Table S4). We then obtained the final model by sequentially deleting the non-significant pathways (Supplementary Figure S7). The evaluation of the model was based on the criteria presented in Schermelleh-Engel et al. (2003).
The results of the ANOVA showed that short-term grazing exclusion had no significant effect on the total coverage (F = 0.1, P = 0.75) but that long-term grazing exclusion significantly reduced the total coverage (F = 23.2, P < 0.001). The total coverage decreased significantly with the increase in grazing exclusion duration (Figure 1A, F = 9.9, P = 0.01). Short-term grazing exclusion had no significant effect on the coverage of dominant species (F = 0.3, P = 0.58), whereas long-term grazing exclusion substantially reduced the coverage of dominant species (F = 17.8, P = 0.001). However, the divergence between them was not significant (Figure 1B, F = 1.2, P = 0.29). Similarly, short-term grazing exclusion had no significant effect on the coverage of common species (F = 1.9, P = 0.18), but long-term grazing exclusion significantly reduced the coverage of common species from 50.8 to 34.7% (F = 6.4, P = 0.02); the coverage of common species decreased significantly (Figure 1C, F = 7.2, P = 0.02) with the increase in grazing exclusion time. For the coverage of rare species, both short-term and long-term grazing exclusion showed a significant increase (F = 14.5, P = 0.001; F = 6.8, P = 0.02), but the difference between them was not significant (Figure 1D, F = 0.1, P = 0.77).
Figure 1. Grassland coverage of the community (A) and dominant (B), common (C), and rare (D) species under short-term and long-term grazing exclusion and in control stands. Boxes in box plots extend from the first (25%) to third (75%) quartiles, with solid and dashed lines at the mean and the median value, respectively. Whiskers extend from the 2.5th to the 97.5th percentile (n = 10). Uppercase letters indicate the differences between control and grazing exclusion under the same duration, while lowercase letters represent the differences between the short-term and long-term application of the same treatment (control or grazing exclusion).
For the different functional groups, short-term grazing exclusion reduced but long-term grazing exclusion increased the relative coverage of grasses (Supplementary Figure S2). Short-term grazing exclusion resulted in a significant decrease in the relative coverage of sedge by 2.8% (F = 8.3, P = 0.01), while the relative coverage of legumes in the long-term grazing exclusion was significantly reduced by 21.9% (F = 104.7, P < 0.001). In addition, both short-term and long-term grazing exclusion increased the relative coverage of non-legume forbs.
There were no significant differences in either species richness or the Simpson’s dominance index between short-term grazing exclusion and the control stands (Figure 2, F = 0.2, P = 0.68; F = 0.1, P = 0.95). However, long-term grazing exclusion significantly reduced species richness (Figure 2A, F = 30.1, P < 0.001) and increased Simpson’s dominance index (Figure 2B, F = 30.1, P < 0.001). The duration of grazing exclusion also exerted a negative effect on species richness, with a decrease from 26 species in the short-term grazing exclusion stand to 17 in the long-term stand (Figure 2A, F = 46.6, P < 0.001). For the different degrees of dominance, short-term grazing exclusion has no significant effect on the diversity of the dominant and the rare species but significantly reduced the diversity of the common species (Supplementary Figure S6, F = 7.9, P = 0.01). Long-term grazing exclusion had significantly negative effects on dominant, common, and rare species richness (P < 0.05). Common species richness decreased the most (from 13 species to 8 species, Supplementary Figure S6).
Figure 2. Plant species diversity (A) and Simpson’s dominance index (B) under short-term and long-term grazing exclusion and in control stands. Uppercase and lowercase letters indicate the differences between control (Grazing) and crazing exclusion and differences under different durations of grazing exclusion, respectively.
The ANOSIM results indicated that the greatest differences in plant community composition occurred among the durations of grazing exclusion (Figure 3 and Supplementary Table S3, R = 0.542, P < 0.001). The community structure changed considerably after long-term grazing exclusion (R = 0.461, P < 0.001). There was also a significant difference in plant community between the short- and long-term grazing exclusion stands (R = 0.542, P < 0.001). Short-term grazing exclusion had a slight influence on the plant community (R = 0.235, P = 0.008). There was no statistically significant difference in plant community composition between the short-term and long-term control stands (R = 0.060, P = 0.137).
Figure 3. Non-metric multidimensional scaling (NMDS) ordination of vegetation in plots based on the height data of different species. Ordinations were based on Bray–Curtis dissimilarities.
The effect of grazing exclusion on community structure was also shown by the change in plant height (Supplementary Figures S4, S5). For the different functional groups, the effects of grazing exclusion on plant height were different between the short-term and long-term exclusion stands. Short-term grazing exclusion significantly increased the height of the grasses, legumes, and non-legume forbs (P < 0.05). However, long-term grazing exclusion significantly decreased the height of the grasses, legumes, and non-legume forbs (P < 0.05).
The effects of grazing exclusion on community spatial heterogeneity were also different between the short-term and long-term exclusion stands (Figure 4). Long-term grazing exclusion exerted a significant influence on the community heterogeneity (F = 11.8, P = 0.003), but the short-term effect was not significant (Figure 4A). Species asynchrony decreased significantly from 0.97 in the short-term grazing exclusion stands to 0.90 in the long-term grazing exclusion stands (Figure 4C, F = 8.7, P = 0.009). For dominant species, short-term grazing exclusion had no significant effect on their heterogeneity and asynchrony (P > 0.05), while long-term grazing exclusion significantly increased the heterogeneity of the dominant species (Figures 4B,D, F = 12.9, P = 0.002).
Figure 4. Community heterogeneity (A,B) and species asynchrony (C,D) for all species (A,C) and dominant species (B,D) under short-term and long-term grazing exclusion and in control stands. Uppercase letters indicate the differences between grazing exclusion and control stands, while lowercase letters represent the differences between short-term and long-term stands.
The simple linear regression model showed that community heterogeneity was significantly and negatively correlated with species richness and species asynchrony (P < 0.001) but positively correlated with the Simpson’s dominance index and dominant species spatial heterogeneity (Figure 5, P = 0.002; P = 0.001). Species asynchrony was significantly and positively correlated with species richness but negatively correlated with Simpson’s dominance index (Supplementary Figures S7A,B). Heterogeneity of dominant species was significantly and negatively correlated with species richness but was not correlated with Simpson’s dominance index (Supplementary Figures S7C,D). The relationship between community heterogeneity and the heterogeneity of common and rare species was non-significant (Supplementary Figure S8, P = 0.768, P = 0.479).
Figure 5. The relationships of species richness (A), Simpson’s dominance index (B), species asynchrony (C), and dominant species heterogeneity (D) with community heterogeneity. Red solid lines represent regression lines, and gray areas are 95% confidence intervals.
The results of the full SEM showed that the combined effect of grazing exclusion and its duration led to an increase in community heterogeneity and that this positive effect was achieved mainly by reducing species richness (Figure 6 and Supplementary Table S4, Chi-square = 6.95, P = 0.07, AIC = 56.95, GFI = 0.98). Grazing exclusion (standardized coefficient (β) = −0.38, P < 0.001) and its duration (β = −0.57, P < 0.001) explained 47% of the variation in species diversity (species richness). Species diversity (β = −0.21, P = 0.01), dominant species heterogeneity (β = 0.26, P < 0.001), and species asynchrony (β = −0.70, P < 0.001) explained 89% of the variation in community heterogeneity. Although Simpson’s dominance index was significantly negatively correlated with species asynchrony (Supplementary Figure S7B) and positively correlated with community heterogeneity (Figure 5B) in linear regression, it was not a significant predictor of community heterogeneity in SEM (Figure 6 and Supplementary Table S4 and Supplementary Figure S9).
Figure 6. A full structural equation model (SEM) of the effects of grazing exclusion and its duration of application on community spatial heterogeneity. Grazing exclusion and its duration can influence community spatial heterogeneity through species richness, Simpson’s dominance index, species asynchrony, and dominant species stability. Red and blue solid arrows indicate significant positive and negative pathways, respectively. Gray dashed arrows represent the pathways that are not significant. Arrow width indicates the strength of the relationship between variables. The standard path coefficients are marked above the arrow. The magnitude of R2 is proportional to the variance explained by each dependent variable in the SEM. AIC, Akaike information criterion; BIC, Bayesian information criterion; NFI, normed fit index; RMSEA, root mean square error of approximation; CFI, comparative fit index. All criteria are within acceptable scope.
Loss of plant diversity will lead to a reduction in ecosystem productivity and stability (Hooper et al., 2005; Oliver et al., 2015; Ren et al., 2016). In this study, we demonstrated that long-term grazing exclusion decreased plant diversity (species richness) and productivity (coverage) (Figures 1, 2), which is consistent with similar experiments in the Kobresia-dominated meadow of the Qinghai-Tibetan Plateau and the lowland grassy ecosystems of southeast Australia (Schultz et al., 2011; Wu et al., 2009). However, the negative effect of grazing exclusion on grassland diversity and productivity was not found in the degraded Stipa tenacissima steppe of southern Tunisia or in the natural grassland ecosystem of western Saudi Arabia (Al-Rowaily et al., 2015; Jeddi and Chaieb, 2010). A meta-analysis in grasslands of China showed that short-term (≤5 years) grazing exclusion significantly increased species richness in alpine steppe and temperate steppe but that this phenomenon was not found in alpine meadow (Xiong et al., 2016). Our research in the alpine meadow of the Qinghai-Tibetan Plateau found that a decrease in species richness in the long-term grazing exclusion stand was mainly a result of decreases in common and rare species richness. On the one hand, in comparison to grazing grassland, grazing exclusion activity led to relatively higher grass and litter biomass, which changed the distribution of light resources in the community and then limited the existence of the common and rare species (Letts et al., 2015). On the other hand, grazing exclusion limited soil trampling, which might improve the soil properties, leading to an improvement of the conditions for vegetation (Liu et al., 2015). As a result, different durations of grazing exclusion should exert different effects on community production and plant diversity. In this study, we demonstrated that short-term grazing exclusion activities increased the height of species with different levels of dominance but did not exert any statistical effects on dominant and rare species richness (Supplementary Figures S5, S6). However, long-term grazing exclusion decreased the species richness of species with different levels of dominance and did not influence the height of species. The result of Pearson correlation analysis showed that soil pH, soil organic carbon, and total nitrogen content were related to Simpson’s dominance index and were not related to species richness (Supplementary Figure S1).
Community structure in a grassland ecosystem changes with composition, and this easy-to-understand causal link has a very complex mechanism (Svenning et al., 2004; El-Keblawy, 2016). Reynolds et al. (2003) stated that changes in plant community structure were the result of plant-microbial-soil interactions. We found that long-term grazing exclusion decreased plant diversity and changed the community structure (Figures 2, 3). The changed community structure can be attributed to long-term regulation among plants and between plants and the environment (Milchunas and Lauenroth, 1995). First, herbivores use a process to select food in their grazing activities, and long-term grazing selection helps to form a copromoted evolutionary model between herbivores and plants (Becerra, 2007). In contrast, long-term grazing exclusion almost stops this copromoted evolution. The main interaction patterns between plant communities and the external biological environment gradually shift from aboveground (herbivores) to the surface (between plants) or below-ground (e.g., soil resources, microorganisms, and small soil-dwelling animals) (Hartnett and Wilson, 1999; Wijesinghe et al., 2005). Hartnett and Wilson (1999) demonstrated that mycorrhizal fungi drove the variation in plant community structure. Community structure characteristics may also be directly affected by nutrient availability in the soil (Huenneke et al., 1990). In addition, long-term grazing exclusion significantly reduced the coverage of dominant species but increased the coverage of rare species (Figure 1). This change in community composition caused by competition among species, especially competition for light resources (Brauer et al., 2012), may further change community structure.
The community heterogeneity (CV) increased (a decrease of community stability in space, ) significantly due to long-term grazing exclusion in this study, which is consistent with the results of a livestock exclusion experiment conducted in Colorado shortgrass steppe (Adler and Lauenroth, 2000). A simple linear model indicated that the increase of community heterogeneity could be attributed to decreases in species diversity, species asynchrony, and dominant species spatial heterogeneity (Figure 5), which is consistent with our hypothesis. We found that the change of community heterogeneity and biotic factors (species diversity, species asynchrony, and dominant species spatial heterogeneity) were not correlated with abiotic factors (pH, soil water content, soil organic carbon, and nitrogen content) (Supplementary Figure S1). So, we only consider biotic factors to discuss the mechanisms that lead to the increase of community heterogeneity under long-term grazing exclusion (based on the mechanisms that maintain community stability). The stability of community biomass is affected by the characteristics of dominant species and plant diversity (Polley et al., 2007). However, when comparing stability among communities, the change in the functional characteristics of dominant species has a greater impact on stability than the change in species diversity (Sasaki and Lauenroth, 2011). In addition, Pan et al. (2016) noted that when the compensation effect of a community is not obvious, the stability of the ecosystem is more sensitive to the loss of diversity.
Species diversity can affect not only plant productivity but also temporal stability and spatial heterogeneity (Fukami et al., 2001; Eisenhauer et al., 2011; Loreau and De Mazancourt, 2013). Experimental evidence indicates that changes in environmental factors alter plant diversity and then increase or decrease the community stability of grasslands (Tilman et al., 2006; Weigelt et al., 2008). Yan and Lu (2015) revealed that a 6–8-year grazing exclusion improved the plant diversity and community stability of degraded grasslands on the Qinghai-Tibetan Plateau. In this study, long-term grazing exclusion caused the loss of species, especially the loss of common species, which led to a decline in community spatial heterogeneity (Figure 5A and Supplementary Figure S6). The full structural equation model indicated that the reduction in species richness was the most important factor affecting community spatial heterogeneity, but Simpson’s dominance index was not maintained in this model as a significant factor of community spatial heterogeneity (Figure 6). Liu et al. (2015) demonstrated that <6 years of grazing exclusion increased plant diversity and contributed to community stability but that >11 years of grazing exclusion decreased community production and plant diversity in the sandy grassland of Ningxia. The evidence presented here suggests that long-term grazing exclusion has not improved the degradation of Qinghai-Tibetan Plateau grasslands but has decreased grassland plant diversity and increase community heterogeneity. Deléglise et al. (2011) also demonstrated that grazing exclusion may lead to an increase in the spatial variability of plant leaf dry matter content in mountain pastures in France.
Previous studies have shown that changes in the dominant species stability contributed most to changes in community stability (Polley et al., 2007; Yang et al., 2017). We found that long-term grazing exclusion significantly increased the heterogeneity of dominant species (Figure 4B) and that community heterogeneity increased with dominant species heterogeneity (Figure 5D). The full SEM demonstrated that dominant species heterogeneity was maintained in this model as a significant factor of community spatial heterogeneity and that species richness could cause the increase of community heterogeneity directly or through influencing dominant species heterogeneity (Figure 6 and Supplementary Figure S7). However, after sequentially deleting the non-significant pathways from the full SEM, we found that the duration of treatment began to show an effect on dominant species heterogeneity (Supplementary Figure S9). One possible explanation for this phenomenon is the change in community heterogeneity with duration of grazing exclusion (Figure 4A).
Species asynchrony represents the interaction of species in the community. Factors that drive asynchrony among species besides environmental stress include species diversity and random effects of species (Loreau and De Mazancourt, 2008). Del Río et al. (2017) suggested that the possible mechanism for maintaining community stability is the compensatory effect between species. Zhang et al. (2016) demonstrated that the reduction of compensatory effects could explain the reduction of stability in a temperate grassland. Our experiments reinforce the idea of a compensatory effect. Interestingly, neither grazing exclusion nor its duration affected species asynchrony (all species) in the SEM, but species asynchrony exhibited significant negative relationships with community spatial heterogeneity (Figures 5C, 6). In our study, short-term and long-term grazing exclusion had no significant effects on dominant species asynchrony (Figure 4D). The asynchrony of the community and of dominant species was insensitive to grazing exclusion and duration, probably because of changes in community composition, such as compensation for coverage among species with different degrees of dominance. Overall, the significant negative correlation between species asynchrony and community spatial heterogeneity emphasized the importance of species compensatory dynamics for grassland ecosystem functioning. Compared with the full SEM, we found that species richness could influence community heterogeneity indirectly through species asynchrony in the final SEM, which is consistent with the result of simple linear regression between species richness and species asynchrony (Supplementary Figure S7A).
In our study, two caveats limited its further application and may cause uncertainties in these results. First, there were limited repetitions in short- (two repetitions, 2 and 4 years) and long-term (two repetitions, 9 and 11 years) grazing exclusion treatments. Second, there was a lack of information about soil biotic and micro-climatic factors, which could result in biases regarding the indirect effect of grazing exclusion on community composition and structure. Despite these caveats, the results of the study still help to increase our understanding of the composition, structure, and function of plant communities under grazing exclusion in the alpine meadow of the Qinghai-Tibetan Plateau and contribute to predicting community spatial heterogeneity in other grasslands through species diversity.
We investigated the responses of community structure, such as abundance (coverage), height, diversity (species richness and Simpson’s dominance index), and soil properties to short-term (2, 4 years) and long-term (9 and 11 years) grazing exclusion in the alpine meadow of the Qinghai-Tibetan Plateau. The results showed that long-term grazing exclusion could reduce species diversity. To compare the results of species richness from the alpine grassland ecosystem with the results from other grassland ecosystems, we summarized the effect of the exclusion on the species richness from related grazing exclusion experiments in different regions (Table 2). We found that the effects of grazing exclusion on grassland plant species richness were dependent on grassland type and exclusion duration. For example, long-term grazing exclusion has caused a loss of species richness in alpine grassland in China and lowland grassy ecosystems of southeast Australia (Wu et al., 2009; Schultz et al., 2011). However, the long-term implementation of grazing exclusion could still contribute to the maintenance of species richness in subalpine grasslands in France and degraded Stipa tenacissima steppe in southern Tunisia (Jeddi and Chaieb, 2010; Deléglise et al., 2011). Several abiotic and biotic factors, including climate and grassland type, could contribute to the divergent effects of grazing exclusion (Xiong et al., 2016). Temperature and precipitation can influence plant species richness after grazing exclusion (Wu et al., 2012; Xiong et al., 2016). For example, the investigations of an alpine grassland transect along the northern Tibetan Plateau indicated that growing season precipitation increased plant species richness by promoting seed germination in the local species pool (Wu et al., 2012). Long-term grazing exclusion could restore the degraded Stipa tenacissima steppe in southern Tunisia by promoting the growth of palatable species (Jeddi and Chaieb, 2010). However, long-term grazing exclusion decreased plant species richness, as in the non-degraded alpine grassland of the Qinghai-Tibetan Plateau (Table 2 and Figure 2). The possible reason for this is the effects of the competitiveness (such as for light or nutrient resources) of dominant grasses on common and rare species under the condition of grazing exclusion (Wu et al., 2009).
In conclusion, our results indicated that grazing exclusion, especially long-term exclusion, failed to improve grassland productivity (grassland coverage) and even reduced the community coverage and plant diversity and altered the community structure of the alpine grassland. Furthermore, our work identified decreased plant diversity as the main reason for the increased spatial heterogeneity of the grazing exclusion in the alpine grassland ecosystem. These results suggest that grazing can maintain relatively higher plant species diversity and community stability in the alpine grassland that covers much of the Qinghai-Tibetan Plateau. However, the effect is not suitable for severely degraded grassland types. Focusing on the impact of grazing exclusion on community composition and structure in different regions is necessary for the sustainable development of each grassland ecosystem.
The datasets generated for this study are available on request to the corresponding author.
JXZ, TZ, and SS designed the research. SS and FZ performed the field survey and data analysis. SS wrote the first draft, with inputs from all authors.
This work was partly funded by the National Key Research and Development Program of China (2019YFC0507704 and 2017YF0503906), National Natural Science Foundation of China (31700374), and Fundamental Research Funds for the Central Universities (lzujbky-2018-ct02 and lzujbky-2019-76).
The authors declare that the research was conducted in the absence of any commercial or financial relationships that could be construed as a potential conflict of interest.
We are grateful to the Haibei National Field Research Station in the Alpine Grassland Ecosystem for maintaining the experimental facility.
The Supplementary Material for this article can be found online at: https://www.frontiersin.org/articles/10.3389/fevo.2020.00066/full#supplementary-material
Adler, P. B., and Lauenroth, W. K. (2000). Livestock exclusion increases the spatial heterogeneity of vegetation in Colorado shortgrass steppe. Appl. Veg. Sci. 3, 213–222. doi: 10.2307/1479000
Al-Rowaily, S. L., El-Bana, M. I., Al-Bakre, D. A., Assaeed, A. M., Hegazy, A. K., and Ali, M. B. (2015). Effects of open grazing and livestock exclusion on floristic composition and diversity in natural ecosystem of Western Saudi Arabia. Saudi J. Biol. Sci. 22, 430–437. doi: 10.1016/j.sjbs.2015.04.012
Augustine, D. J., and Frank, D. A. (2001). Effects of migratory grazers on spatial heterogeneity of soil nitrogen properties in a grassland ecosystem. Ecology 82, 3149–3162. doi: 10.2307/2679841
Bai, Y., Han, X., Wu, J., Chen, Z., and Li, L. (2004). Ecosystem stability and compensatory effects in the Inner Mongolia grassland. Nature 431, 181–184. doi: 10.1038/nature02850
Becerra, J. X. (2007). The impact of herbivore-plant coevolution on plant community structure. Proc. Natl. Acad. Sci. U.S.A. 104, 7483–7488. doi: 10.1073/pnas.0608253104
Brauer, V. S., Stomp, M., and Huisman, J. (2012). The nutrient-load hypothesis: patterns of resource limitation and community structure driven by competition for nutrients and light. Am. Nat. 179, 721–740. doi: 10.1086/665650
Del Río, M., Pretzsch, H., Ruíz-Peinado, R., Ampoorter, E., Annighöfer, P., Barbeito, I., et al. (2017). Species interactions increase the temporal stability of community productivity in Pinus sylvestris-Fagus sylvatica mixtures across Europe. J. Ecol. 105, 1032–1043. doi: 10.1111/1365-2745.12727
Deléglise, C., Loucougaray, G., and Alard, D. (2011). Effects of grazing exclusion on the spatial variability of subalpine plant communities: a multiscale approach. Basic Appl. Ecol. 12, 609–619. doi: 10.1016/j.baae.2011.08.006
Eisenhauer, N., Milcu, A., Allan, E., Nitschke, N., Scherber, C., Temperton, V., et al. (2011). Impact of above- and below-ground invertebrates on temporal and spatial stability of grassland of different diversity. J. Ecol. 99, 572–582. doi: 10.1111/j.1365-2745.2010.01783.x
El-Keblawy, A. (2016). Impact of fencing and irrigation on species composition and diversity of desert plant communities in the united Arab emirates. Land Degrad. Dev. 28, 1354–1362. doi: 10.1002/ldr.2599
Fukami, T., Naeem, S., and Wardle, D. A. (2001). On similarity among local communities in biodiversity experiments. Oikos 95, 340–348. doi: 10.2307/3547380
Grman, E., Lau, J. A., Schoolmaster, D. R., and Gross, K. L. (2010). Mechanisms contributing to stability in ecosystem function depend on the environmental context. Ecol. Lett. 13, 1400–1410. doi: 10.1111/j.1461-0248.2010.01533.x
Hartnett, D. C., and Wilson, G. W. (1999). Mycorrhizae influence plant community structure and diversity in tallgrass prairie. Ecology 80, 1187–1195. doi: 10.2307/177066
Hooper, D. U., Chapin, F. S., Ewel, J. J., Hector, A., Lnchausti, P., Lavorel, S., et al. (2005). Effects of biodiversity on ecosystem functioning: a consensus of current knowledge. Ecol. Monogr. 75, 3–35. doi: 10.1890/04-0922
Huang, D., Wang, K., and Wu, W. L. (2007). Dynamics of soil physical and chemical properties and vegetation succession characteristics during grassland desertification under sheep grazing in an agro-pastoral transition zone in Northern China. J. Arid Environ. 70, 120–136. doi: 10.1016/j.jaridenv.2006.12.009
Huenneke, L. F., Hamburg, S. P., Koide, R., Mooney, H. A., and Vitousek, P. M. (1990). Effects of soil resources on plant invasion and community structure in Californian serpentine grassland. Ecology 71, 478–491. doi: 10.2307/1940302
Huston, M. A. (1997). Hidden treatments in ecological experiments: re-evaluating the ecosystem function of biodiversity. Oecologia 110, 449–460. doi: 10.1007/s004420050180
Jeddi, K., and Chaieb, M. (2010). Changes in soil properties and vegetation following livestock grazing exclusion in degraded arid environments of South Tunisia. Flora 205, 184–189. doi: 10.1016/j.flora.2009.03.002
Kolasa, J., and Rollo, C. D. (1991). “Introduction: the heterogeneity of heterogeneity: a glossary,” in Ecological Heterogeneity, eds J. Kolasa, and S. T. A. Pickett, (New York, NY: Springer), 1–23. doi: 10.1007/978-1-4612-3062-5_1
Letts, B., Lamb, E. G., Mischkolz, J. M., and Romo, J. T. (2015). Litter accumulation drives grassland plant community composition and functional diversity via leaf traits. Plant Ecol. 216, 357–370. doi: 10.1007/s11258-014-0436-6
Liu, H., Mi, Z., Lin, L., Wang, Y., Zhang, Z., Zhang, F., et al. (2018). Shifting plant species composition in response to climate change stabilizes grassland primary production. Proc. Natl. Acad. Sci. U.S.A. 115, 4051–4056. doi: 10.1073/pnas.1700299114
Liu, X., Zhang, K., and Ahmad, B. (2015). Influence of fencing time on vegetation community structure and species diversity in sandy grassland of Ningxia in China. Nat. Environ. Pollut. Technol. 14, 703–708.
Loreau, M., and De Mazancourt, C. (2008). Species synchrony and its drivers: neutral and nonneutral community dynamics in fluctuating environments. Am. Nat. 172, E48–E66. doi: 10.1086/589746
Loreau, M., and De Mazancourt, C. (2013). Biodiversity and ecosystem stability: a synthesis of underlying mechanisms. Ecol. Lett. 16, 106–115. doi: 10.1111/ele.12073
Ma, Z., Liu, H., Mi, Z., Zhang, Z., Wang, Y., Xu, W., et al. (2017). Climate warming reduces the temporal stability of plant community biomass production. Nat. Commun. 8:15378. doi: 10.1038/ncomms15378
Mariotte, P., Vandenberghe, C., Kardol, P., Hagedorn, F., Buttler, A., and Schwinning, S. (2013). Subordinate plant species enhance community resistance against drought in semi-natural grasslands. J. Ecol. 101, 763–773. doi: 10.1111/1365-2745.12064
Milchunas, D. T., and Lauenroth, W. K. (1995). Inertia in plant community structure: state changes after cessation of nutrient-enrichment stress. Ecol. Appl. 5, 452–458. doi: 10.2307/1942035
Naeem, S., Thompson, L. J., Lawler, S. P., Lawton, J. H., and Woodfin, R. M. (1994). Declining biodiversity can alter the performance of ecosystems. Nature 368, 734–737. doi: 10.1038/368734a0
Oliver, T. H., Isaac, N. J. B., August, T. A., Woodcock, B. A., Roy, D. B., Bullock, J. M., et al. (2015). Declining resilience of ecosystem functions under biodiversity loss. Nature 6:10122. doi: 10.1038/ncomms10122
Pan, Q., Tian, D., Naeem, S., Auerswald, K., Elser, J. J., Bai, Y., et al. (2016). Effects of functional diversity loss on ecosystem functions are influenced by compensation. Ecology 97, 2293–2302. doi: 10.1002/ecy.1460
Polley, H. W., Wilsey, B. J., and Derner, J. D. (2007). Dominant species constrain effects of species diversity on temporal variability in biomass production of tallgrass prairie. Oikos 116, 2044–2052. doi: 10.1111/j.2007.0030-1299.16080.x
Ren, Y., Lü, Y., and Fu, B. (2016). Quantifying the impacts of grassland restoration on biodiversity and ecosystem services in China: a meta-analysis. Ecol. Eng. 95, 542–550. doi: 10.1016/j.ecoleng.2016.06.082
Reynolds, H. L., Packer, A., Bever, J. D., and Clay, K. (2003). Grassroots ecology: plant–microbe–soil interactions as drivers of plant community structure and dynamics. Ecology 84, 2281–2291. doi: 10.2307/3450134
Sasaki, T., and Lauenroth, W. K. (2011). Dominant species, rather than diversity, regulates temporal stability of plant communities. Oecologia 166, 761–768. doi: 10.1007/s00442-011-1916-1
Schermelleh-Engel, K., Moosbrugger, H., and Hans, M. (2003). Evaluating the fit of structural equation models: tests of significance and descriptive goodness-of-fit measures. Methods Psychol. Res. 8, 23–74.
Schultz, N. L., Morgan, J. W., and Lunt, I. D. (2011). Effects of grazing exclusion on plant species richness and phytomass accumulation vary across a regional productivity gradient. J. Veg. Sci. 22, 130–142. doi: 10.1111/j.1654-1103.2010.01235.x
Smith, B., and Wilson, J. B. (1996). A consumer’s guide to evenness indices. Oikos 76, 70–82. doi: 10.2307/3545749
Song, M. H., and Yu, F. H. (2015). Reduced compensatory effects explain the nitrogen-mediated reduction in stability of an alpine meadow on the Tibetan Plateau. New Phytol. 207, 70–77. doi: 10.1111/nph.13329
Su, X. K., Wu, Y., Dong, S. K., Wen, L., Li, Y. Y., and Wang, X. X. (2015). Effects of grassland degradation and re-vegetation on carbon and nitrogen storage in the soils of the headwater area nature reserve on the Qinghai-Tibetan Plateau, China. J. Mt. Sci. 12, 582–591. doi: 10.1007/s11629-014-3043-z
Svenning, J. C., Kinner, D. A., Stallard, R. F., Engelbrecht, B. M. J., and Wright, S. J. (2004). Ecological determinism in plant community structure across a tropical forest landscape. Ecology 85, 2526–2538. doi: 10.1890/03-0396
Tilman, D., Reich, P. B., and Knops, J. M. H. (2006). Biodiversity and ecosystem stability in a decade-long grassland experiment. Nature 441, 629–632. doi: 10.1038/nature04742
Ulrich, W., Piwczyński, M., Zaplata, M. K., Winter, S., Schaaf, W., and Fischer, A. (2014). Small-scale spatial variability in phylogenetic community structure during early plant succession depends on soil properties. Oecologia 175, 985–995. doi: 10.1007/s00442-014-2954-2
Wang, Y., Liu, H., Chung, H., Yu, L., Mi, Z., Geng, Y., et al. (2014). Non-growing-season soil respiration is controlled by freezing and thawing processes in the summer monsoon-dominated Tibetan alpine grassland. Glob. Biogeochem. Cycles 28, 1081–1095. doi: 10.1002/2013GB004760
Wang, Y., Song, C., Yu, L., Mi, Z., Wang, S., Zeng, H., et al. (2018). Convergence in temperature sensitivity of soil respiration: evidence from the tibetan alpine grasslands. Soil Biol. Biochem. 122, 50–59. doi: 10.1016/j.soilbio.2018.04.005
Weigelt, A., Schumacher, J., Roscher, C., and Schmid, B. (2008). Does biodiversity increase spatial stability in plant community biomass? Ecol. Lett. 11, 338–347. doi: 10.1111/j.1461-0248.2007.01145.x
Wijesinghe, D. K., John, E. A., and Hutchings, M. J. (2005). Does pattern of soil resource heterogeneity determine plant community structure? An experimental investigation. J. Ecol. 93, 99–112. doi: 10.1111/j.0022-0477.2004.00934.x
Wilcox, K. R., Tredennick, A. T., Koerner, S. E., Grman, E., Hallett, L. M., Avolio, M. L., et al. (2017). Asynchrony among local communities stabilises ecosystem function of metacommunities. Ecol. Lett. 20, 1534–1545. doi: 10.1111/ele.12861
Wilsey, B. J., Daneshgar, P. P., Hofmockel, K., and Polley, H. W. (2014). Invaded grassland communities have altered stability-maintenance mechanisms but equal stability compared to native communities. Ecol. Lett. 17, 92–100. doi: 10.1111/ele.12213
Wu, G. L., Du, G. Z., Liu, Z. H., and Thirgood, S. (2009). Effect of fencing and grazing on a Kobresia-dominated meadow in the Qinghai-Tibetan plateau. Plant Soil 319, 115–126. doi: 10.1016/S0145-305X(00)00063-X
Wu, G. L., Zhang, Z. N., Wang, D., Shi, Z. H., and Zhu, Y. J. (2014). Interactions of soil water content heterogeneity and species diversity patterns in semi-arid steppes on the Loess Plateau of China. J. Hydrol. 519, 1362–1367. doi: 10.1016/j.jhydrol.2014.09.012
Wu, J. S., Zhang, X. Z., Shen, Z. X., Shi, P. L., Yu, C. Q., Song, M. H., et al. (2012). Species richness and diversity of alpine grasslands on the northern Tibetan Plateau: effects of grazing exclusion and growing season precipitation. J. Resour. Ecol. 3, 236–242. doi: 10.1016/j.jenvman.2018.10.097
Xiong, D., Shi, P., Zhang, X., and Zou, C. B. (2016). Effects of grazing exclusion on carbon sequestration and plant diversity in grasslands of China—A meta-analysis. Ecol. Eng. 94, 647–655. doi: 10.1016/j.ecoleng.2016.06.124
Xu, M., Qi, Y., Chen, J., and Yin, W. (2000). Effects of spatial heterogeneity of microenvironment on plant biodiversity in the Southeastern Missouri Ozarks. Geogr. Inform. Sci. 6, 38–47. doi: 10.1080/10824000009480532
Yan, Y., and Lu, X. (2015). Is grazing exclusion effective in restoring vegetation in degraded alpine grasslands in Tibet, China? PeerJ 3:e1020. doi: 10.7717/peerj.1020
Yang, Z., Zhang, Q., Su, F., Zhang, C., Pu, Z., Xia, J., et al. (2017). Daytime warming lowers community temporal stability by reducing the abundance of dominant, stable species. Glob. Change Biol. 23, 154–163. doi: 10.1111/gcb.13391
Zhang, Y., Loreau, M., Lü, X., He, N., Zhang, G., and Han, X. (2016). Nitrogen enrichment weakens ecosystem stability through decreased species asynchrony and population stability in a temperate grassland. Glob. Change Biol. 22, 1445–1455. doi: 10.1111/gcb.13140
Zhao, K., Wang, Z. Y., Li, Y. Q., Zheng, T. L., and Hu, J. B. (2019). Effect of two restorations on the grassland coverage after remediation of take-abandon soil field on the Qinghai-Tibet Plateau. Pratacultural Sci. 36, 2500–2507. doi: 10.11829/j.issn.1001-0629.2019-0066
Zhou, H., Zhao, X., Tang, Y., Gu, S., and Zhou, L. (2005). Alpine grassland degradation and its control in the source region of the Yangtze and Yellow Rivers, China. Grassl. Sci. 51, 191–203. doi: 10.1111/j.1744-697X.2005.00028.x
Keywords: community composition, community structure, grazing exclusion, Qinghai-Tibetan Plateau, spatial heterogeneity
Citation: Song S, Zhu J, Zheng T, Tang Z, Zhang F, Ji C, Shen Z and Zhu J (2020) Long-Term Grazing Exclusion Reduces Species Diversity but Increases Community Heterogeneity in an Alpine Grassland. Front. Ecol. Evol. 8:66. doi: 10.3389/fevo.2020.00066
Received: 21 October 2019; Accepted: 02 March 2020;
Published: 21 April 2020.
Edited by:
Marco A. Molina-Montenegro, University of Talca, ChileReviewed by:
Jianshuang Wu, Institute of Environment and Sustainable Development in Agriculture, Chinese Academy of Agricultural Sciences, ChinaCopyright © 2020 Song, Zhu, Zheng, Tang, Zhang, Ji, Shen and Zhu. This is an open-access article distributed under the terms of the Creative Commons Attribution License (CC BY). The use, distribution or reproduction in other forums is permitted, provided the original author(s) and the copyright owner(s) are credited and that the original publication in this journal is cited, in accordance with accepted academic practice. No use, distribution or reproduction is permitted which does not comply with these terms.
*Correspondence: Jianxiao Zhu, anh6aHVAbHp1LmVkdS5jbg==
Disclaimer: All claims expressed in this article are solely those of the authors and do not necessarily represent those of their affiliated organizations, or those of the publisher, the editors and the reviewers. Any product that may be evaluated in this article or claim that may be made by its manufacturer is not guaranteed or endorsed by the publisher.
Research integrity at Frontiers
Learn more about the work of our research integrity team to safeguard the quality of each article we publish.