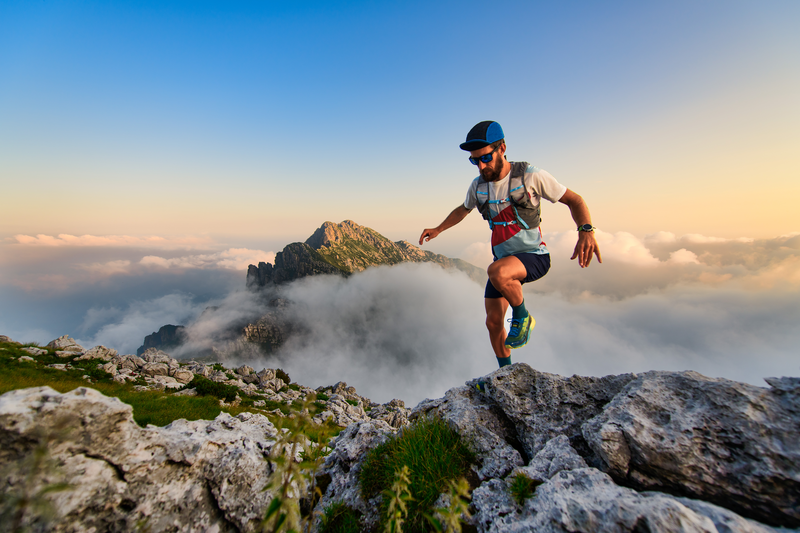
94% of researchers rate our articles as excellent or good
Learn more about the work of our research integrity team to safeguard the quality of each article we publish.
Find out more
PERSPECTIVE article
Front. Ecol. Evol. , 13 March 2020
Sec. Coevolution
Volume 8 - 2020 | https://doi.org/10.3389/fevo.2020.00061
This article is part of the Research Topic Symbiosis in a Changing Environment View all 11 articles
Microbes have driven eco-evolutionary adaptations organizing biodiversity from the origin of life. They are ubiquitous and abundant, facilitating the biochemical processes that make Earth habitable and shape ecosystem structures, functions, and services. Recent studies reveal that commensalistic and beneficial microbes associated with wild and domesticated plants may aid in establishing sustainable agriculture for a changing climate. However, developing microbe-based biotechnologies and ecosystem services requires a thorough understanding of the diversity and complexity of microbial interactions with each other and with higher organisms. We discuss the hot and blind spots in contemporary research on plant microbiomes, and how the latest molecular biological techniques and empirical eco-evolutionary approaches could elevate our perception of microbe–plant interactions through multidisciplinary studies.
Recent advances in molecular microbiology have revolutionized the ability of the scientific community to understand and study the diversity and functions of microbes associated with animals and plants, leading to a plethora of related literature. A Web of Science search (January 23, 2020) using “microbiome” or “microbiome and plant” as topic keywords produced 28,733 references published, with 49 and 1,211 of the papers ranked as “hot” and “highly cited.” More than 99% of the papers were published after 2010. These studies are largely based on mass sequencing of taxonomic marker genes for bacteria and fungi (community sequencing), and to lesser extent, metagenomes of microbial communities. Currently, studies relying on molecular methodologies dominate plant microbiome literature.
Today we know that microbes are ubiquitous and essential associates of virtually all higher organisms. For example, the microbial cells colonizing the human body appear to be more abundant as our somatic cells and contain overwhelmingly more genes than our human genome (Gilbert et al., 2018). Similarly, microbes have been found in virtually all plants (Partida-Martinez and Heil, 2011), and are known for their immense significance e.g., as plant beneficial mycorrhizae and endophytes. Pathogens such as coffee rust, leaf blight of rubber, Panama disease of banana, chestnut blight, Dutch elm disease, and potato blight have been culprits for great historical convulsions with social impacts (Schumann, 1991). Historically the potato blight, the causal agent of the Irish potato famine causing the death by starvation of one million people and overseas emigration of a further two million people in the mid-1800s (Schumann, 1991), has been particularly influential in the emergence of germ theory and in shaping the conceptual model of disease triangle (Schumann, 1991).
We propose that as microbes associated with their shared host plants comprise multipartite entities, the theories of hologenome evolution (Saikkonen et al., 2006; Zilber-Rosenberg and Rosenberg, 2008; Moran and Sloan, 2015; Vandenkoornhuyse et al., 2015; Theis et al., 2016; Rosenberg and Zilber-Rosenberg, 2018) and the geographic mosaic of coevolution (Thompson, 2005) provide usable frameworks to understand these microbial-plant interactions and their importance for ecological, evolutionary and genetic processes. Similarly to human and animal microbiomes (Gilbert et al., 2018), the majority of plant microbiomes are acquired from the environment or transmitted horizontally between individuals. However, in modular organisms (Chapman, 1981) characterized by somatic embryogenesis, such as most seed plants, many long-lasting microbe-plant interactions involve either vertical or pseudo-vertical transmission from host plant to its sexual and/or vegetative offspring (Wilkinson, 1997; Saikkonen et al., 1998; Cankar et al., 2005). Thus, plant microbiomes provide a particularly fertile ground for ecologists and evolutionary biologists interested in all levels of selection in co-evolutionary processes. The use of metagenomic tools allow us to determine the diversity of microbiomes and the “-omics” approaches (genomics, proteomics and metabolomics) can be used to examine how genomic information is translated into structures and functions in the interactions among plant and its microbial partners (Delmotte et al., 2009; Lundberg et al., 2012; Sessitsch et al., 2012; Hardoim et al., 2015; Agler et al., 2016; Saikkonen et al., 2016; van der Heijden and Hartmann, 2016).
Furthermore, if we take into account that phenotypic selection may treat the plant and its associated microbes individually or in concert as a phenotypic and metagenomic unit, the implications for conceptions of genetics, epigenetics (Jaenisch and Bird, 2003; Zilber-Rosenberg and Rosenberg, 2008; Vannier et al., 2015) and natural selection are profound. The next step toward a better understanding of the diverse roles of microbiomes is to combine metagenomic surveys regarding the composition and function of microbiomes with empirical and theoretical biological approaches including ecology, physiology, genetics and epigenetics, phenotypic evolution, and coevolution of interacting species (Saikkonen et al., 2004, 2016; Prosser et al., 2007; Vandenkoornhuyse et al., 2015; Vannier et al., 2015; Rosenberg and Zilber-Rosenberg, 2016; van Overbeek and Saikkonen, 2016). This requires the acknowledgment and breaking of certain barriers and challenges associated with scientific traditions and management of increasing information overload.
We contend that human perspective often hampers understanding of the complex nature of microbe–plant interactions and their importance to ecosystem functions and services. Conventional wisdom biased by human perceptions might misdirect scientific progress. We outline three constraining human factors, “pitfall of scientific discipline,” “the dilemma of classification,” and “overly optimistic expectations,” which will require multidisciplinary research approaches to be conquered.
Pitfall of scientific discipline, i.e., dogmatic concept formation in scientific discipline, may constrain one's options. Conventional life science disciplines, such as plant physiology, evolutionary ecology or phytopathology, either largely ignore microbes (Holland and Polacco, 1994; Compant et al., 2016; Kauppinen et al., 2016; Saikkonen et al., 2016; van Overbeek and Saikkonen, 2016) or examine microbe–plant interactions from limited, pre-defined perspectives, and largely build on the knowledge and traditions of their own discipline. Such strongly canalized approaches limit our ability to acknowledge the complexity of biological interactions and different levels of regulation and selection (Koskella et al., 2017).
For example, phytopathology examines pathogen–host plant interactions focusing on the ecology and evolution of pathogen–host interactions, and how these interactions might be modulated by microbial community interactions in the pathobiome (Vayssier-Taussat et al., 2014). Although community-level approaches that include other microbial plant associates are increasingly being adopted in plant pathology (Santhanam et al., 2015; Syed Ab Rahman et al., 2018), a significant proportion of phytopathology research is dedicated to epidemiology, disease diagnosis, and management of microbial species of economic or environmental importance alone. This, combined with the long tradition of one microbe–one plant approach in the field, might overemphasize the pathogenic phase of the microbe's lifecycle and divert attention from other relevant aspects of the same microbe–plant interaction, and overlook the impact of whole microbiomes in disease development or repression.
Meanwhile, an increasing number of evolutionary ecology studies reveal that the same microbial species commonly occupy several ecological niches. Thus, the nature of microbe–plant interactions is labile and context-dependent in ecological and evolutionary time rather than always beneficial or disadvantageous to the host (Saikkonen et al., 1998, 2004; Wäli et al., 2013; Rybakova et al., 2016; Selosse et al., 2018). The same microbial species might be labeled a pathogen, parasite, or endophyte, and referred to by different names. For example, a mutation of a single locus may convert a fungal plant pathogen to a non-pathogenic endophytic symbiont (Freeman and Rodriguez, 1993), and the nature of the interaction with the host plant is conditioned on environmental factors (e.g., herbivory or available resources), life-history characters, and genetic combinations of the host and the microbe (Rybakova et al., 2016). Likewise, the bacterial species Clavibacter michiganensis is known as a plant pathogenic species, and C. michiganensis subspecies are regarded as quarantine organisms. However, an endophytic bacterial strain, Clavibacter str Enf12, isolated from subnival alpine plants and classified as C. michiganensis, is shown to increase the chilling tolerance of its host plants, and has not been reported to be pathogenic (Ding et al., 2011; Eichenlaub and Gartemann, 2011). Further, the very same microbial species are sometimes named differently in different contexts. As to the naming of microbes, a common endophyte of birch trees, Fusicladium betulae, was named Venturia ditricha by a forest pathologist. Venturia is a teleomorph (the sexual reproductive stage) and is now its formal name, but the fact is the species is a biotrophic pathogen and is more commonly an asymptomatic anamorphic (an asexual reproductive stage) endophyte than obviously pathogenic (Ahlholm et al., 2002a; Helander et al., 2007). Similarly, some plant mutualistic mycorrhizal fungi are reported as asymptomatic endophytes and organic matter degrading saprotrophs (Weiß et al., 2016; Grelet et al., 2017; Smith et al., 2017; Martino et al., 2018; Schneider-Maunoury et al., 2018). The question is whether we should consider a microbe saprophytic or pathogenic if it inhabits its host plant asymptomatically throughout most of its life cycle—in the case of perennial hosts, perhaps over years—and manifests itself as pathogenic or saprophytic during only a short period of its life.
Similarly to scientific disciplines, the dilemma of classification, i.e., grouping microbes either based on their taxa or functional role, directs and limits researchers' interests to different parts of microbial communities. Consequently, the related scientific theories have largely developed separately (van Overbeek and Saikkonen, 2016). For example, bacteria, fungi, and viruses are usually studied separately, although they coexist and likely often interact with each other (Saikkonen et al., 2004; van Overbeek and Saikkonen, 2016). Furthermore, in ecological and evolutionary literature microbe–plant interactions are generally treated separately from the species–species and multitrophic interactions of higher organisms (e.g., plant–herbivore interactions), although all plant and animal interactions unavoidably involve microbes. We believe that understanding any biological interaction requires taking microbiomes of higher organisms into account, because together the organism and the diverse assemblages of its symbiotic microbes form a holobiont, an extended phenotype and the target of phenotypic selection. This concept of holobiont should include all plant associated microbes whenever experimentally feasible.
Finally, overly optimistic expectations commonly emerge when a scientist assembles information, becomes excited by attractive discoveries, concepts and/or economically profitable application opportunities, and potentially misses fundamental biological background knowledge. Metagenomic and proteomic approaches illuminate the ubiquity, diversity, and importance of microbes, and open our eyes to associated potential opportunities. Evidence shows that the majority of microorganisms detected by metagenomic tools colonize their host plants asymptomatically (Compant et al., 2016; Gopal and Gupta, 2016; Nissinen et al., 2019). These microbial associates of plants are commonly defined as endophytes (Wilson, 1995). Taxonomically, endophytes are diverse; they include archaeal, bacterial, fungal, and protistic microorganisms. Most are considered plant commensals but few, such as the fungal Epichloë species commonly inhabiting cool-season grasses, are regarded as mutualistic, especially in high-nutrient agroecosystems (Kauppinen et al., 2016; Saikkonen et al., 2016). Plant mutualistic bacterial endophytes are less documented, but accumulating genomic and metabolomic information on bacterial genomes and modulations in plant metabolism strongly suggests they have a role in plant growth, development, and stress tolerance (Sessitsch et al., 2012; Hardoim et al., 2015; van Overbeek and Saikkonen, 2016; Esmaeel et al., 2018). Inspired by evidence that endophytic microorganisms are involved in denitrification, nitrogen fixation, and greenhouse gas emissions, and that they can affect plant tolerance to abiotic stress, and virtually all types of plant–plant, plant–herbivore or plant–pathogen interactions, an increasing number of scientists have become interested in the potential of endophytic microorganisms in the bioeconomy. Here we propose that this development might have directed the focus on overly ambitious goals and expectations for the following reasons.
The more we look at the basic biology of microbes, the more obvious it becomes that although we could govern endophytic microbes, we cannot fully control them, especially in the agricultural fields and nature. Microbial interactions are known to be labile and context dependent ranging from antagonistic to mutualistic in both ecological and evolutionary time (Saikkonen et al., 1998, 2004; Lopes et al., 2009; Berg et al., 2016; Brader et al., 2017). Microbes have potential to evolve rapidly as response to changing selection forces, and thereby affect nature of their interaction with the host as well as associated community and ecosystem processes (Freeman and Rodriguez, 1993; Saikkonen et al., 2004; terHorst et al., 2014). Thus, mutualistic, commensalistic and antagonistic microbial taxa are inseparable and present in virtually all microbiomes studied. We should aim to understand the microbiome dynamics, and consider microbes as part of the diverse multi-kingdom community (van Elsas et al., 2012; Koch et al., 2018), particularly when utilizing plant mutualistic endophytes in sustainable agriculture and food production.
Recent plant microbiome research is largely focused on describing structure and functions of microbial communities in different plant associated niches, and linking specific microbial taxa to plant performance. Unarguably, these studies have rapidly given us solid understanding of broad taxonomic trends in plant associated microbiota. Integration of metagenomics to proteomics, metabolomics and other omics-approaches is now enabling associating microbiome structural shifts to plant holobiont functioning. However, the mass of data provided by these techniques can easily overwhelm researchers that currently often lack the tools to organize and process the data, resulting in flow of papers cataloging plant and/or microbial functions or even unannotated genes. Here, integration of ecological theories in these studies (as also researcher training) would provide structuring framework, connecting now largely separated microbiome research to other fields of plant-microbe interactions and ecological and evolutionary biology.
Indeed, plant microbiome research is transitioning from descriptive surveys to comprehensive understanding of the plant holobiome in the eco-evolutionary framework. Identification of keystone microbial species and interconnected microbial hubs, as well as their putative functions (Sessitsch et al., 2012; Agler et al., 2016; Saikkonen et al., 2016; van der Heijden and Hartmann, 2016), are the basic pieces required to solve the eco-evolutionary puzzle, comprised of interacting free-living microbes and holobiome-units in ecosystems.
Easy data acquisition has created the illusion of controllability and the hope of microbiology-based innovations. This has led to the renaissance of microbial research seeking solutions for global issues such as diseases, food security, and sustainable agricultural practices (Duhamel and Vandenkoornhuyse, 2013; Kauppinen et al., 2016; Busby et al., 2017; Finkel et al., 2017). However, the major challenge is that we are still a long way from understanding microbial versatility and how it relates to the ecology and evolution of plant holobionts interacting with each other and their environment. Meeting these challenges requires combining novel molecular and microbiological tools with empirical and theoretical biological approaches including ecology, genetics and epigenetics, phenotypic evolution, and coevolution of interacting species. Otherwise we lack theoretical insights (Prosser et al., 2007), and ignore the complexity and dynamics of microbe–plant interactions in man-made and natural ecosystems.
We propose that to assemble the plant holobiome puzzle, future work should take into account the following premises and presumptions.
First, the majority of plant-microbe studies are either plant or microbe centered. Accordingly, studies are often designed and interpreted with either the plant as the active member which recruits its microbes (Gehring and Whitham, 1994) or the host plant as a background variable (e.g., “plant genotype”) or a habitat. Instead, both the plant and associated microbes should be taken into account as active members of the interaction (Saikkonen et al., 1999; Bulgarelli et al., 2012; Dini-Andreote and Raaijmakers, 2018). This requires developing true dialogue, reciprocal understanding, and mutual collaboration between researchers from different life science fields.
Second, we contend that microbe–plant interactions follow similar evolutionary and ecological processes as host–pathogen or host–parasite interactions, and therefore need not to be treated differently (Saikkonen et al., 2004; Cordovez et al., 2019). The prevailing insight is that like other species interactions, even obligate microbe-plant mutualism is based on mutual exploitation rather than reciprocal altruism and benefits to the partners are only rarely symmetric (Thompson, 1994; Maynard Smith and Szathmary, 1995; Doebeli and Knowlton, 1998; Saikkonen et al., 2004). Symbioses between microbes and their host plants may also involve reciprocal manipulation of phenotypes, including morphology and physiology, and lifecycle of partners (Saikkonen et al., 2016). Thus, conflicting selection forces are likely to destabilize them, and the outcome of interactions can change in time and space projecting the ecological surface of a dynamic fitness landscape with adaptive peaks and valleys (Thompson, 1994, 2005; Saikkonen et al., 2004). For example, mutualistic mycorrhizae and Epichloë endophytes can become parasitic in the presence of herbivores or hemiparasitic plants especially in resource-limited environments (Ahlholm et al., 2002b; Lehtonen et al., 2005; Saikkonen et al., 2010a; Wäli et al., 2013).
Third, co-occurring plant-associated microbes, irrespective of taxa, are likely to interact with each other. Microbes compete, interact chemically, and/or mediate the host quality to each other. Chemical interplay may include signaling and chemical cross-talk among microbes and their host plant cells (Hamilton et al., 2012; Compant et al., 2016; van Overbeek and Saikkonen, 2016) but can also extend to cover other organisms feeding on the shared host plant as well as associated food webs (Lehtonen et al., 2006; Saikkonen et al., 2006; Saari et al., 2010; Li et al., 2014). It is noteworthy that certain pathways regulating plant responses to heterotrophic organisms, such as salicylic acid and jasmonic acid pathways, have been shown to counteract (Ballaré, 2011; Thaler et al., 2012; Pineda et al., 2013). For instance, chemical crosstalk between mutualistic microbes, biotrophic and necrotrophic pathogens (Bastias et al., 2018), and herbivores may constrain the host plant from reaching optimal pathogen- or herbivore-specific resistance (Ahlholm et al., 2002a), but is likely to be beneficial to overall fitness of the holobiont. Similarly, community-level approaches to bacterial and fungal interactions are needed to understand the importance of the structure and functions of microbial community associated with plants on plant performance as well as on ecosystem functions and services (Dini-Andreote and Raaijmakers, 2018). More knowledge about multispecies coevolution is necessary to fully understand any particular bipartite microbe–plant interactions and how they modulate cascading interactions across trophic layers.
Fourth, the performance of plant-associated organisms such as mutualistic, commensalistic, and antagonistic microbes, as well as herbivores, may also be a response to genetically or epigenetically determined plant traits rather than interconnected associations among them. The role of epigenetics is still poorly understood. In contrast, empirical evidence suggests that for example genetic compatibility can determine endophytic microbe-plant combinations that can be mutualistic or commensalistic depending on environmental conditions (Saikkonen et al., 2010b). Furthermore genetically determined plant traits can determine plant quality to heterotrophic organisms such as microbes and/or herbivores (Fritz and Simms, 1992) and genetic correlations between plant resistance to pathogens and herbivores have been suggested to constrain plants from reaching optimal species-specific resistance (Ahlholm et al., 2002a).
Fifth, microbes are highly diverse and versatile, and the ecological role of microbes is often complex and liable to change. Microbes can occupy several ecological niches that may overlap, and the nature of microbe–plant association is context dependent. The same ecological function can be provided by several different microbes, and individual microbes can be—at least partially—substitutable by others. This calls importance for studies on the microbial mediated key functions in different environments.
The spectrum, complexity, and dynamics of microbe–plant interactions demonstrate that plant microbiomes provide a fertile ground for understanding unit of selection, multitrophic interactions, evolution of life histories, and co-evolutionary processes, and how microbiomes should be taken into account when developing microbe-based biotechnologies and ecosystem services. Two unanswered questions remain: (1) How plants and their associated microbes individually and/or in concert as a phenotypic unit respond to prevailing selection pressures? (2) What proportion of ecological outcome is determined by genetics, epigenetics, and phenotypic plasticity in the ecologically relevant traits, and thus in the adaptive radiation of holobionts? Present barriers must be dismantled in order to disseminate current knowledge of plant microbiomes and create a more conceptual framework with empirical and theoretical examples, and predictable hypotheses (van Overbeek and Saikkonen, 2016; Frank et al., 2017).
This knowledge is urgently needed to tackle the foremost global challenges of our times: the biodiversity loss, climate change and the increasing demand of food production to meet world's population growth (Springmann et al., 2018; Tollefson, 2019). For example, in the debate on biodiversity loss and endangered species, particular attention should be paid to the importance of abundant keystone species maintaining crucial ecosystem functions that are vital to thriving ecosystems or species that may threaten other species by disrupting ecosystem functions. Microbes are largely ignored in this context although they are essential for processes that make Earth habitable to primary producers and other organisms subsisting on them. Similarly microbes should be taken into account in risk analyses and solutions to aim mitigating climate change as well as in sustainable food production (Duhamel and Vandenkoornhuyse, 2013; Gundel et al., 2013; Kauppinen et al., 2016). For example, recent evidence suggests that microbes can increase carbon sink in terrestrial ecosystems by enhancing carbon uptake into soils and into plants by promoting plant growth (e.g., mycorrhizae, rhizobia and endophytes) or alter the flux of greenhouse gases from the soil to the atmosphere (Clemmensen et al., 2013; Iqbal et al., 2013; Averill et al., 2014). Beneficial microbial plant symbionts have been suggested to have great potential in sustainable agricultural and horticultural practices, and for environmental improvement as well (Duhamel and Vandenkoornhuyse, 2013; Gundel et al., 2013; Kauppinen et al., 2016). These goals might partly be overly ambitious because microbe-plant interactions are complex, labile and context dependent, and thus, we never can fully control plant associated microbiomes in nature. However, some of these ambitious goals can be attained if we invest resources in multidisciplinary collaboration across various fields of expertise, as understanding the plant-microbiome dynamics and factors impacting holobiont ecology and evolution can enable us to utilize the plant microbiomes for sustainable future.
KS, RN, and MH have contributed equally in initializing, innovating, and writing the paper.
This work was supported by the Academy of Finland, Grant Nos. 295976 and 326226 and Finnish Cultural Foundation.
The authors declare that the research was conducted in the absence of any commercial or financial relationships that could be construed as a potential conflict of interest.
Agler, M. T., Ruhe, J., Kroll, S., Morhenn, C., Kim, S. T., Weigel, D., et al. (2016). Microbial hub taxa link host and abiotic factors to plant microbiome variation. PLoS Biol. 14:e1002352. doi: 10.1371/journal.pbio.1002352
Ahlholm, J. U., Helander, M., Elamo, P., Saloniemi, I., Neuvonen, S., Hanhimäki, S., et al. (2002a). Micro-fungi and invertebrate herbivores on birch trees: fungal mediated plant-herbivore interactions or responses to host quality? Ecol. Lett. 5, 648–655. doi: 10.1046/j.1461-0248.2002.00368.x
Ahlholm, J. U., Helander, M., Lehtimäki, S., Wäli, P., and Saikkonen, K. (2002b). Vertically transmitted fungal endophytes: different responses of host-parasite systems to environmental conditions. Oikos 99, 173–183. doi: 10.1034/j.1600-0706.2002.990118.x
Averill, C., Turner, B. L., and Finzi, A. C. (2014). Mycorrhiza-mediated competition between plants and decomposers drives soil carbon storage. Nature 505, 543–545. doi: 10.1038/nature12901
Ballaré, C. L. (2011). Jasmonate-induced defenses: a tale of intelligence, collaborators and rascals. Trends Plant Sci. 16, 249–257. doi: 10.1016/j.tplants.2010.12.001
Bastias, D. A., Martínez-Ghersa, M. A., Ballaré, C. L., and Gundel, P. E. (2018). Epichloë fungal endophytes and plant defences: not just alkaloids. Trends Plant Sci. 22, 939–948. doi: 10.1016/j.tplants.2017.08.005
Berg, G., Rybakova, D., Grube, M., and Köberl, M. (2016). The plant microbiome explored: implications for experimental botany. J. Exp. Bot. 67, 995–1002. doi: 10.1093/jxb/erv466
Brader, G., Compant, S., Vescio, K., Mitter, B., Trogniz, F., Ma, L.-J., et al. (2017). Ecology and genomic insights into plant-pathogenic and plant-nonpathogenic endophytes. Annu. Rev. Phytopathol. 55, 61–83. doi: 10.1146/annurev-phyto-080516-035641
Bulgarelli, D., Rott, M., Schlaeppi, K., Ver Loren van Themaat, E., Ahmadinejad, N., Assenza, F., et al. (2012). Revealing structure and assembly cues for Arabidopsis root-inhabiting bacterial microbiota. Nature 488, 91–95. doi: 10.1038/nature11336
Busby, P. E., Soman, C., Wagner, M. R., Friesen, M. L., Kremer, J., Bennett, A., et al. (2017). Research priorities for harnessing plant microbiomes in sustainable agriculture. PLoS Biol. 15:e2001793. doi: 10.1371/journal.pbio.2001793
Cankar, K., Kraigher, H., Ravnikar, M., and Rupnik, M. (2005). Bacterial endophytes from seeds of Norway spruce (Picea abies L. Karst). FEMS Microbiol. Lett. 244, 341–345. doi: 10.1016/j.femsle.2005.02.008
Chapman, G. (1981). Individuality and modular organisms. Biol. J. Linn. Soc. 15, 177–183. doi: 10.1111/j.1095-8312.1981.tb00757.x
Clemmensen, K. E., Bahr, A., Ovaskainen, O., Dahlberg, A., Ekblad, A., Wallander, H., et al. (2013). Roots and associated fungi drive long-term carbon sequestration in boreal forest. Science 339, 1615–1618. doi: 10.1126/science.1231923
Compant, S., Saikkonen, K., Mitter, B., Campisano, A., and Mercado-Blanco, J. (2016). Editorial special issue: soil, plants and endophytes. Plant Soil 405, 1–11. doi: 10.1007/s11104-016-2927-9
Cordovez, V., Dini-Andreote, F., Carrión, V. J., and Raaijmakers, J. M. (2019). Ecology and evolution of plant microbiomes. Ann. Rev. Microbiol. 73, 69–88. doi: 10.1146/annurev-micro-090817-062524
Delmotte, N., Knief, C., Chaffron, S., Innerebner, G., Roschitzki, B., Schlapbach, R., et al. (2009). Community proteogenomics reveals insights into the physiology of phyllosphere bacteria. Proc. Natl. Acad. Sci. U.S.A. 106, 16428–16433. doi: 10.1073/pnas.0905240106
Ding, S., Huang, C. L., Sheng, H. M., Song, C. L., Li, Y. B., and An, L. Z. (2011). Effect of inoculation with the endophyte Clavibacter sp. strain Enf12 on chilling tolerance in Chorispora bungeana. Physiol. Plantarum 141, 141–151. doi: 10.1111/j.1399-3054.2010.01428.x
Dini-Andreote, F., and Raaijmakers, J. M. (2018). Embracing community ecology in plant microbe research. Trends Plant Sci. 23, 467–469. doi: 10.1016/j.tplants.2018.03.013
Doebeli, M., and Knowlton, N. (1998). The evolution of interspecific mutualism. Proc. Natl. Acad. Sci. U.S.A. 95, 8676–8680. doi: 10.1073/pnas.95.15.8676
Duhamel, M., and Vandenkoornhuyse, P. (2013). Sustainable agriculture: possible trajectories from mutualistic symbiosis and plant neodomestication. Trends Plant Sci. 18, 597–600. doi: 10.1016/j.tplants.2013.08.010
Eichenlaub, R., and Gartemann, K.-H. (2011). The Clavibacter michiganensis subspecies: molecular investigation of gram-positive bacterial plant pathogens. Annu. Rev. Phytopathol. 49, 445–464. doi: 10.1146/annurev-phyto-072910-095258
Esmaeel, Q., Miotto, L., Rondeau, M., Leclère, V., Clément, C., Jacquard, C., et al. (2018). Paraburkholderia phytofirmans PsJN-Plants interaction: from perception to the induced mechanisms. Front. Microbiol. 9:2093. doi: 10.3389/fmicb.2018.02093
Finkel, O. M., Castrillo, G., Herrera Paredes, S., Salas González, I., and Dangl, J. L. (2017). Understanding and exploiting plant beneficial microbes. Curr. Opin. Plant Biol. 38, 155–163. doi: 10.1016/j.pbi.2017.04.018
Frank, A. C., Saldierna Guzmán, J. P., and Shay, J. E. (2017). Transmission of bacterial endophytes. Microorganisms 5:70. doi: 10.3390/microorganisms5040070
Freeman, S., and Rodriguez, R. J. (1993). Genetic conversion of a fungal plant pathogen to a nonpathogenic, endophytic mutualist. Science 260, 75–78. doi: 10.1126/science.260.5104.75
Fritz, R. D., and Simms, E. L. (1992). Plant Resistance to Herbivores and Pathogens: Ecology, Evolution, and Genetics. Chicago: University of Chicago Press doi: 10.7208/chicago/9780226924854.001.0001
Gehring, C. A., and Whitham, T. C. (1994). Interactions between aboveground herbivores and the mycorrhizal mutualists of plants. Trends Ecol. Evol. 9, 251–255. doi: 10.1016/0169-5347(94)90290-9
Gilbert, J. A., Blaser, M. J., Caporaso, J. G., Jansson, J. K., Lynch, S. V., and Knight, R. (2018). Current understanding of the human microbiome. Nat. Med. 24, 392–400. doi: 10.1038/nm.4517
Gopal, M., and Gupta, A. (2016). Microbiome selection could spur next-generation plant breeding strategies. Front. Microbiol. 7:1971. doi: 10.3389/fmicb.2016.01971
Grelet, G. A., Ba, R., Goeke, D. F., Houliston, G. J., Taylor, A. F. S., and Durall, D. M. (2017). A plant growth-promoting symbiosis between Mycena galopus and Vaccinium corymbosum seedlings. Mycorrhiza 27, 831–839. doi: 10.1007/s00572-017-0797-5
Gundel, P. E., Pérez, L. I., Helander, M., and Saikkonen, K. (2013). Symbiotically modified organisms: nontoxic fungal endophytes in grasses. Trends Plant Sci. 18, 420–427. doi: 10.1016/j.tplants.2013.03.003
Hamilton, C. E., Gundel, P. E., Helander, M., and Saikkonen, K. (2012). Endophytic mediation of reactive oxygen species and antioxidant activity in plants: a review. Fungal Divers. 54, 1–10. doi: 10.1007/s13225-012-0158-9
Hardoim, P. R., van Overbeek, L. S., Berg, G., Pirttilä, A. M., Compant, S., Campisano, A., et al. (2015). The hidden world within plants: ecological and evolutionary considerations for defining functioning of microbial endophytes. Microbiol. Mol. Biol. Rev. 79, 293–320. doi: 10.1128/MMBR.00050-14
Helander, M., Ahlholm, J., Sieber, T. N., Hinneri, S., and Saikkonen, K. (2007). Fragmented environment affects birch leaf endophytes. New Phytol. 175, 547–553. doi: 10.1111/j.1469-8137.2007.02110.x
Holland, M. A., and Polacco, J. C. (1994). PPFMs and other covert contaminants: is there more to plant physiology than just plant?_Annu. Rev. Plant Physiol. Plant Mol. Biol. 45, 197–209. doi: 10.1146/annurev.pp.45.060194.001213
Iqbal, J., Nelson, J. A., and McCulley, R. L. (2013). Fungal endophyte presence and genotype affect plant diversity and soil-to-atmosphere trace gas fluxes. Plant Soil 364, 15–27. doi: 10.1007/s11104-012-1326-0
Jaenisch, R., and Bird, A. (2003). Epigenetic regulation of gene expression: how the genome integrates intrinsic and environmental signals. Nat. Genet. 33, 245–254. doi: 10.1038/ng1089
Kauppinen, M., Saikkonen, K., Helander, M., and Pirttilä, A. M. (2016). Epichloë grass endophytes in sustainable agriculture. Nat. Plants 2:15224. doi: 10.1038/nplants.2015.224
Koch, E., Becker, J. O., Berg, G., Hauschild, R., Jehle, J., Köhl, J., et al. (2018). Biocontrol of plant diseases is not an unsafe technology! J. Plant Dis. Protect. 125, 121–125. doi: 10.1007/s41348-018-0158-4
Koskella, B., Hall, L. J., and Metcalf, C. J. E. (2017). The microbiome beyond the horizon of ecological and evolutionary theory. Nat. Ecol. Evol. 1, 1606–1615. doi: 10.1038/s41559-017-0340-2
Lehtonen, P., Helander, M., and Saikkonen, K. (2005). Are endophyte-mediated effects on herbivores conditional on soil nutrients? Oecologia 142, 38–45. doi: 10.1007/s00442-004-1701-5
Lehtonen, P. T., Helander, M., Siddiqui, S. A., Lehto, K., and Saikkonen, K. (2006). Endophytic fungus decreases plant virus infections in meadow ryegrass (Lolium pratense). Biol. Lett. 2, 620–623. doi: 10.1098/rsbl.2006.0499
Li, T., Blande, J. D., Gundel, P., Helander, M., and Saikkonen, K. (2014). Epichloë endophytes alter inducible indirect defences in host grasses. PLoS ONE 9:e101331. doi: 10.1371/journal.pone.0101331
Lopes, J. R., Daugherty, M. P., and Almeida, R. P. P. (2009). Context-dependent transmission of a generalist plant pathogen: host species and pathogen strain mediate insect vector competence. Entomol. Exp. Appl. 131, 216–224. doi: 10.1111/j.1570-7458.2009.00847.x
Lundberg, D. S., Lebeis, S. L., Paredes, S. H., Yourstone, S., Gehring, J., Malfatti, S., et al. (2012). Defining the core Arabidopsis thaliana root microbiome. Nature 488, 86–90. doi: 10.1038/nature11237
Martino, E., Morin, E., Grelet, G. A., Kuo, A., Kohler, A., Daghino, S., et al. (2018). Comparative genomics and transcriptomics depict ericoid mycorrhizal fungi as versatile saprotrophs and plant mutualists. N. Phytol. 217, 1213–1229. doi: 10.1111/nph.14974
Moran, N. A., and Sloan, D. B. (2015). The hologenome concept: helpful or hollow? PLOS Biol. 13:e1002311. doi: 10.1371/journal.pbio.1002311
Nissinen, R., Helander, M., Kumar, M., and Saikkonen, K. (2019). Heritable Epichloë symbiosis shapes plant fungal but not bacterial communities. Sci. Rep. 9:5253. doi: 10.1038/s41598-019-41603-5
Partida-Martinez, L. P., and Heil, M. (2011). The microbe-free plant: fact or artifact? Front. Plant Sci. 2:100. doi: 10.3389/fpls.2011.00100
Pineda, A., Dicke, M., Pieterse, C. M. J., and Pozo, M. J. (2013). Beneficial microbes in a changing environment: are they always helping plants to deal with insects? Funct. Ecol. 27, 574–586. doi: 10.1111/1365-2435.12050
Prosser, J. I., Bohannan, B. J., Curtis, T. P., Ellis, R. J., Firestone, M. K., Freckleton, R. P., et al. (2007). The role of ecological theory in microbial ecology. Nat. Rev. Microbiol. 5, 384–392. doi: 10.1038/nrmicro1643
Rosenberg, E., and Zilber-Rosenberg, I. (2016). Microbes drive evolution of animals and plants: the hologenome concept. mBio 7:e01395. doi: 10.1128/mBio.01395-15
Rosenberg, E., and Zilber-Rosenberg, I. (2018). The hologenome concept of evolution after 10 years. Microbiome 6:78. doi: 10.1186/s40168-018-0457-9
Rybakova, D., Schmuck, M., Wetzlinger, U., Varo-Suarez, A., Murgu, O., Müller, H., et al. (2016). Kill or cure? The interaction between endophytic Paenibacillus and Serratia strains and the host plant is shaped by plant growth conditions. Plant Soil 405, 65–79. doi: 10.1007/s11104-015-2572-8
Saari, S., Sundell, J., Huitu, O., Helander, M., Ketoja, E., Ylönen, H., et al. (2010). Fungal-mediated multitrophic interactions - do grass endophytes in diet protect voles from predators? PLoS ONE 5:e9845. doi: 10.1371/journal.pone.0009845
Saikkonen, K., Ahonen-Jonnarth, U., Markkola, A., Helander, M., Tuomi, J., Roitto, M., et al. (1999). Defoliation and mycorrhizal symbiosis: a functional balance between carbon sources and below-ground sinks. Ecol. Lett. 2, 19–26. doi: 10.1046/j.1461-0248.1999.21042.x
Saikkonen, K., Faeth, S. H., Helander, M., and Sullivan, T. J. (1998). Fungal endophytes: a continuum of interactions with host plants. Annu. Rev. Ecol. Syst. 29, 319–343. doi: 10.1146/annurev.ecolsys.29.1.319
Saikkonen, K., Lehtonen, P., Helander, M., Koricheva, J., and Faeth, S. H. (2006). Model systems in ecology: dissecting the endophyte-grass literature. Trends Plant Sci. 11, 428–433. doi: 10.1016/j.tplants.2006.07.001
Saikkonen, K., Saari, S., and Helander, M. (2010a). Defensive mutualism between plants and endophytic fungi? Fung. Div. 41, 101–113. doi: 10.1007/s13225-010-0023-7
Saikkonen, K., Wäli, P., Helander, M., and Faeth, S. H. (2004). Evolution of endophyte-plant symbioses. Trends Plant Sci. 9, 275–280. doi: 10.1016/j.tplants.2004.04.005
Saikkonen, K., Wäli, P. R., and Helander, M. (2010b). Genetic compatibility determines endophyte-grass combinations. PLoS ONE 5:e11395. doi: 10.1371/journal.pone.0011395
Saikkonen, K., Young, C. A., Helander, M., and Schardl, C. L. (2016). Endophytic Epichloë species and their grass hosts: from evolution to applications. Plant Molec. Biol. 90, 665–675. doi: 10.1007/s11103-015-0399-6
Santhanam, R., Luu, V. T., Weinhold, A., Goldberg, J., Oh, Y., and Baldwin, I. T. (2015). Native root-associated bacteria rescue a plant from a sudden-wilt disease that emerged during continuous cropping. Proc. Natl. Acad. Sci. U.S.A. 112, e5013–e5020. doi: 10.1073/pnas.1505765112
Schneider-Maunoury, L., Leclercq, S., Clément, C., Covès, H., Lambourdière, J., Sauve, M., et al. (2018). Is Tuber melanosporum colonizing the roots of herbaceous, non-ectomycorrhizal plants? Fungal Ecol. 31, 59–68. doi: 10.1016/j.funeco.2017.10.004
Selosse, M. A., Schneider-Maunoury, L., and Martos, F. (2018). Time to re-think fungal ecology? Fungal ecological niches are often prejudged. N. Phytol. 217, 968–972. doi: 10.1111/nph.14983
Sessitsch, A., Hardoim, P. R., Döring, J., Weilharter, A., Krause, A., Woyke, T., et al. (2012). Functional characteristics of an endophyte community colonizing rice roots as revealed by metagenomic analysis. Mol. Plant-Microbe Interact. 25, 28–36. doi: 10.1094/MPMI-08-11-0204
Smith, G. R., Finlay, R. D., Stenlid, J., Vasaitis, R., and Menkis, A. (2017). Growing evidence for facultative biotrophy in saprotrophic fungi: data from microcosm tests with 201 species of wood-decay basidiomycetes. N. Phytol. 215, 747–755. doi: 10.1111/nph.14551
Springmann, M., Clark, M., Mason-D'Croz, D., Wiebe, K., Bodirsky, B. L., Lassaletta, L., et al. (2018). Options for keeping the food system within environmental limits. Nature 562, 519–524. doi: 10.1038/s41586-018-0594-0
Syed Ab Rahman, S. F., Singh, E., Pieterse, C. M. J., and Schenk, P. M. (2018). Emerging microbial biocontrol strategies for plant pathogens. Plant Sci. 267, 102–111. doi: 10.1016/j.plantsci.2017.11.012
terHorst, C. P., Lennon, J. T., and Lau, J. A. (2014). The relative importance of rapid evolution for plant-microbe interactions depends on ecological context. Proc. R. Soc. B. 281:20140028. doi: 10.1098/rspb.2014.0028
Thaler, J. S., Humphrey, P. T., and Whiteman, N. K. (2012). Evolution of jasmonate and salicylate signal crosstalk. Trends Plant Sci. 17, 260–270. doi: 10.1016/j.tplants.2012.02.010
Theis, K. R., Dheilly, N. M., Klassen, J. L., Brucker, R. M., Baines, J. F., Bosch, T. C., et al. (2016). Getting the hologenome concept right: an eco-evolutionary framework for hosts and their microbiomes. mSystems 1:e00028. doi: 10.1128/mSystems.00028-16
Thompson, J. N. (1994). The Coevolutionary Process. London: University of Chicago Press. doi: 10.7208/chicago/9780226797670.001.0001
Thompson, J. N. (2005). The Geographic Mosaic of Coevolution. Chicago: University of Chicago Press. doi: 10.7208/chicago/9780226118697.001.0001
Tollefson, J. (2019). One million species face extinction. Nature 569:171. doi: 10.1038/d41586-019-01448-4
van der Heijden, M. G. A., and Hartmann, M. (2016). Networking in the plant microbiome. PLoS Biol. 14:e1002378. doi: 10.1371/journal.pbio.1002378
van Elsas, J. D., Chiurazzi, M., Mallon, C. A., Elhottova, D., Kristufek, V., and Salles, J. F. (2012). Microbial diversity determines the invasion of soil by a bacterial pathogen. Proc. Natl. Acad. Sci. U.S.A. 109, 1159–1164. doi: 10.1073/pnas.1109326109
van Overbeek, L. S., and Saikkonen, K. (2016). Impact of bacterial-fungal interactions on the colonization of the endosphere. Trends Plant Sci. 21, 230–242. doi: 10.1016/j.tplants.2016.01.003
Vandenkoornhuyse, P., Quaiser, A., Duhamel, M., Le Van, A., and Dufresne, A. (2015). The importance of the microbiome of the plant holobiont. N. Phytol. 206, 1196–1206. doi: 10.1111/nph.13312
Vannier, N., Mony, C., Bittebière, A. K., and Vandenkoornhuys, P. (2015). Epigenetic mechanisms and microbiota as a toolbox for plant phenotypic adjustment to environment. Front. Plant Sci. 6:1159. doi: 10.3389/fpls.2015.01159
Vayssier-Taussat, M., Albina, E., Citti, C., Cosson, J. F., Jacques, M. A., Lebrun, M. H., et al. (2014). Shifting the paradigm from pathogens to pathobiome: new concepts in the light of meta-omics. Front. Cell Infect. Microbiol. 4:29. doi: 10.3389/fcimb.2014.00029
Wäli, P. P., Wäli, P. R., Saikkonen, K., and Tuomi, J. (2013). Is the pathogenic ergot fungus a conditional defensive mutualist for its host grass? PLoS ONE 8:e69249. doi: 10.1371/journal.pone.0069249
Weiß, M., Waller, F., Zuccaro, A., and Selosse, M. A. (2016). Sebacinales – one thousand and one interactions with land plants. N. Phytol. 211, 20–40. doi: 10.1111/nph.13977
Wilkinson, D. M. (1997). The role of seed dispersal in the evolution of mycorrhizae. Oikos 78, 394–396. doi: 10.2307/3546308
Wilson, D. (1995). Endophyte: the evolution of a term, and clarification of its use and definition. Oikos 73, 274–276. doi: 10.2307/3545919
Keywords: bacteria, fungi, microbiome, endophytes, pathogens, saprobes, ecology, evolution
Citation: Saikkonen K, Nissinen R and Helander M (2020) Toward Comprehensive Plant Microbiome Research. Front. Ecol. Evol. 8:61. doi: 10.3389/fevo.2020.00061
Received: 24 November 2019; Accepted: 26 February 2020;
Published: 13 March 2020.
Edited by:
Anne Duplouy, Lund University, SwedenReviewed by:
Arne Weinhold, Freie Universität Berlin, GermanyCopyright © 2020 Saikkonen, Nissinen and Helander. This is an open-access article distributed under the terms of the Creative Commons Attribution License (CC BY). The use, distribution or reproduction in other forums is permitted, provided the original author(s) and the copyright owner(s) are credited and that the original publication in this journal is cited, in accordance with accepted academic practice. No use, distribution or reproduction is permitted which does not comply with these terms.
*Correspondence: Kari Saikkonen, a2FyaXNhaWtAdXR1LmZp
Disclaimer: All claims expressed in this article are solely those of the authors and do not necessarily represent those of their affiliated organizations, or those of the publisher, the editors and the reviewers. Any product that may be evaluated in this article or claim that may be made by its manufacturer is not guaranteed or endorsed by the publisher.
Research integrity at Frontiers
Learn more about the work of our research integrity team to safeguard the quality of each article we publish.