- 1Marine Spatial Ecology Lab, School of Biological Sciences and Australian Research Council Centre of Excellence for Coral Reef Studies, University of Queensland, Brisbane, QLD, Australia
- 2Oceans and Atmosphere, Commonwealth Scientific and Industrial Research Organisation, St. Lucia, QLD, Australia
The concept of resilience is long established across a wide-range of disciplines, but its evaluation in many ecosystems has been challenging due to the complexities involved in quantifying a somewhat abstract dynamical phenomenon. We develop a framework of resilience-related concepts and describe their methodological approaches. Seven broad approaches were identified under the three principle concepts of (1) ecological resilience (ecological resilience, precariousness and current attractor), (2) engineering resilience (short-term recovery rate and long-term reef performance), and (3) vulnerability (absolute and relative vulnerability) respectively. Using specific examples, we assess the strengths and limitations of each approach and their capacity to answer common management questions. The current synthesis provides new directions for resilience assessments to be incorporated into management decisions and has implications on the research agenda for advances in resilience assessments.
Introduction
From its early use in physical sciences and ecology, the concept of resilience is now ubiquitous across natural and social sciences including psychology (Luthar et al., 2000), urban planning (Eraydin and Tasan-Kok, 2013), disaster management (Manyena, 2006), resource-dependent industries (Marshall, 2010), economics (Common and Perrings, 1992; Brand, 2009), and governance (Lebel et al., 2006). The concept has become increasingly malleable to fit different objectives and exhibits considerable variety in its application (Brand and Jax, 2007; Martin-Breen and Anderies, 2011; Davidson et al., 2016).
While quantification is necessary to operationalize the resilience of ecosystems, it has largely remained a conceptual phenomenon until recently. Feature issues published in the Journal of Applied Ecology (Angeler and Allen, 2016) and Trends in Ecology and Evolution (Hodgson et al., 2015) demonstrate ongoing dialogue to quantify resilience and the relevant key questions. How can resilience be operationalized using existing frameworks? What are the appropriate metrics to measure resilience in different ecosystems? Although recent studies advocate for generic indicators to achieve standardized quantification across systems, such as the measurement of resistance and recovery (Hodgson et al., 2015; Nimmo et al., 2015), general metrics may not be easy to measure across all systems, each with distinct system dynamics exhibiting different behaviors and governed by different processes and mechanisms (Dakos et al., 2015; Yeung and Richardson, 2015; Petraitis and Dudgeon, 2016).
Reviews of resilience have focused on quantification approaches across multiple ecosystems (Scheffer et al., 2015; Allen et al., 2016; Quinlan et al., 2016), drawing heavily on models of ecosystems including lakes (Carpenter et al., 1999), savannahs (Walker et al., 1981), and coral reefs. Although a number of reviews have considered the processes that drive resilience and its application to management (Bellwood et al., 2004; Hughes et al., 2005; Nyström et al., 2008; Mumby et al., 2014a), a critical review of approaches is lacking.
Here, we synthesize progress on the measurement of resilience on coral reefs and identify several novel, additional concepts that might have utility. We begin with a brief historical perspective of reef resilience concepts and provide a framework to categorize approaches. We then assess the strengths and weaknesses of various approaches and identify appropriate management questions. Finally we discuss new directions for resilience assessments, with an emphasis on improving the most common metrics-based approaches.
Historical Perspective of Coral Reef Resilience Science
In the late 1960s, ecologists began to study the ability of ecosystems to exhibit alternative community states, each of which appeared to be stable over time, occurred in the same environment, and was fairly resistant to disturbance (Lewontin, 1969; Holling, 1973). The term given to this phenomenon was “ecological resilience” and ecosystems were said to be attracted to alternative equilibrial states (Holling, 1973). Perhaps the first evidence that coral reefs might exhibit multiple “stable” states was the discovery of a patch of the relatively unpalatable macroalga, Asparogopsis taxiformis, after a ship grounding and pollution event on the outer Great Barrier Reef (GBR, Hatcher, 1984). Hatcher hypothesized that pollutants had provided an opportunity for the algae to bloom and once established, algae persisted by reaching a size escape from herbivory. Thus, different communities could emerge at different environmental levels but the system was likely stable even when nutrients returned to pre-disturbance levels (though this was not verified).
Nearly a decade later, the prospect of major shifts in coral community structure was revisited in a special issue of the journal American Zoologist. Done (1992) coined the term “phase shift” to describe a conspicuous change in community structure, referring to a drastic change from coral to macroalgal dominance. The term phase shift is symptomatic with no explicit connotation of stability (Petraitis and Dudgeon, 2004), and might involve alternative community states that become resistant to change owing to ecological feedbacks, or simply represent a reversible monotonic response of an ecosystem to a changing environment. In the same special issue of the journal, Knowlton (1992) published the first critical examination of mechanisms that might drive the existence of multiple alternative states on coral reefs. She mooted the idea that depleted herbivory could influence the competitive outcomes between corals and macroalgae and stabilize algal-dominated states. McManus and Polsenberg (2004) then proposed a conceptual model of key factors involved in reef phase shifts. To develop and reinforce the existence of multiple alternative states in reefs, Mumby and colleagues (Mumby et al., 2007) used a spatially-explicit and field-validated model of Caribbean coral communities. They proposed that the emergence of alternative states was a recent phenomenon in the Caribbean because reefs are not predicted to shift and lock when fast-growing acroporids or herbivory by sea urchins are returned to the ecosystem (Mumby et al., 2013b) yet both had been devastated from disease in the 1980s.
Although the existence of alternative stable states is a nuanced and by no means a universal phenomenon (Fung et al., 2011; Mumby et al., 2013a), the fact that some reefs have low resilience is beyond question. As more cases of reef decline and recovery were investigated, it became clear that reefs are profoundly dynamic systems that react and recover differently to perturbations, and that resilience cannot be taken for granted (Pearson, 1981; Brown and Suharsono, 1990; Ginsburg, 1993; Hughes and Connell, 1999; Bellwood et al., 2004; Graham et al., 2015). Moreover, Connell (1997) reviewed global patterns of reef recovery and found striking variability across the Pacific and the Caribbean; a pattern that persists today (Roff and Mumby, 2012).
With growing awareness that the health of some reefs was experiencing persistent decline in the 1990s (Hughes, 1994; Steneck, 1994) – perhaps best marked by Bob Ginsburg’s “Colloquium on coral reef hazards and health” (Ginsburg, 1993) – science, management, and conservation agencies became increasingly engaged in understanding the processes determining the fate of reefs. West and Salm (2003) raised the idea of resilience-based management (RBM), albeit without coining the term, and proposed that interventions should be directed toward reefs with less exposure to natural and anthropogenic disturbances and to focus interventions on the processes that confer resilience by either facilitating recovery or help resist stress and disturbance (McClanahan et al., 2012). RBM has now been defined as “using knowledge of current and future drivers influencing ecosystem function (e.g., coral disease outbreaks; changes in land-use, trade, or fishing practices) to prioritize, implement, and adapt management actions that sustain ecosystems and human well-being” (McLeod et al., 2019).
Research into coral reef resilience continues to blossom and appears to follow five trajectories. The largest is the quest to understand individual processes underlying reef resilience, both in isolation and as cumulative stressors. This literature is too large to be summarized here but includes research into connectivity, demographic rates, ecological interactions and stress responses (Andres and Rodenhouse, 1993; Roberts, 1997; Cowen et al., 2006; Nyström et al., 2008; Fabricius et al., 2011; McClanahan et al., 2011; Doropoulos et al., 2016; Ortiz et al., 2018). Second is the identification of empirical resilience metrics that can be used to compare reef sites (West and Salm, 2003; Obura and Grimsditch, 2009; Maynard et al., 2010; McClanahan et al., 2012; Jouffray et al., 2015; Guest et al., 2018). Third is the use of statistical models to predict community trajectories and infer drivers of resilience (Zychaluk et al., 2012; Cooper et al., 2015; Gross and Edmunds, 2015). Fourth is the development of mechanistic ecological models to understand and integrate the processes of resilience and/or predict reef trajectories under multiple stresses (Mumby et al., 2007, 2014b; Anthony et al., 2011; Fung et al., 2011; Blackwood et al., 2012; Bozec and Mumby, 2015). Finally, a broader set of frameworks has become available to link resilience science to decision-making (Game et al., 2008; McLeod et al., 2009, 2012, 2019; Mumby et al., 2011; Anthony et al., 2015; Mumby and Anthony, 2015).
Resilience Concepts on Coral Reefs
There are three major usages of the term resilience in the coral reef and environmental literature. We introduce all three here in brief and then describe the behavior of the most popular in greater detail in a later section. The earliest usage of resilience considered the concepts of engineering and ecological resilience proposed by Holling (1996), which emerged from the modeling of predator-prey interactions revealing the ability of a system to undergo profound changes in community state (Holling, 1961, 1973; Lewontin, 1969; May, 1972). Engineering resilience refers to the time a system takes to return to a single equilibrium after a perturbation (Pimm, 1984; Holling, 1996). Ecological resilience considers the likelihood of the system (or social system, Marshall, 2010) to shift between multiple equilibria, separated by basins of attraction (Figure 1; Walker et al., 2002; Kinzig et al., 2006; Mumby et al., 2014b). A basin of attractor is a space in which the system tends to remain (Walker et al., 2004), see Figure 2 for images illustrating coral-dominated and algal-dominated reefs. Ecosystems can move toward different attractors because of the numerous reinforcing feedbacks that drive their response even without external perturbations such as coral bleaching and storm damage.
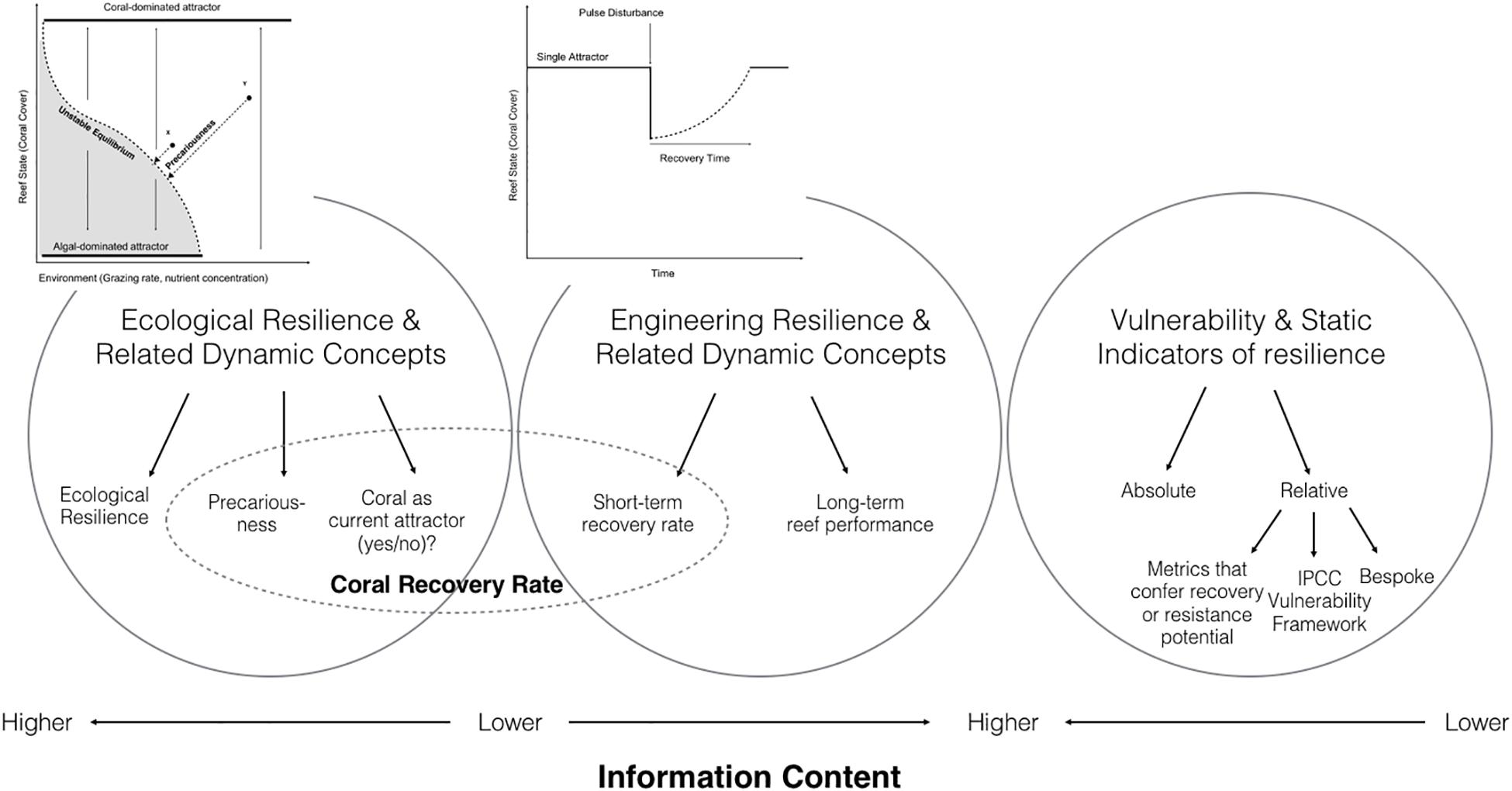
Figure 1. Conceptual diagram of the various methods of measuring ecological resilience, engineering resilience and vulnerability. Precariousness is the distance of a reef to the suspected unstable equilibrium/tipping point. The precariousness of Reef X is greater than Reef Y. Reefs that fall in the shaded gray area has an algal-dominated current attractor, and reefs are in the white area has a coral-dominated current attractor. Dotted line represents cross-cutting methods that relate to coral recovery rate.
The second usage of the word resilience is an umbrella term and considers the behavior of integrated socio-ecological systems, in which ecosystem dynamics might only be a single element amongst other components taken into consideration such as the role of human activity (Nyström et al., 2000; Folke, 2006). Resilience in this context has been described under the umbrellas of “resilience thinking” (Walker and Salt, 2006) or “panarchy” (Gunderson and Holling, 2001), and provides a framework to consider the factors that stabilize and drive a system. The key aspects of resilience thinking include resilience, adaptability (adaptive capacity) and transformability (Walker and Salt, 2006; Folke et al., 2010; Bellwood et al., 2012). The concept of panarchy refers to the hierarchical set of adaptive cycles at different scales and their effects across all scales (Gunderson and Holling, 2001). These frameworks do not explicitly invoke “ecological resilience,” though multiple attractors may exist within the wider system or single components. Resilience thinking and panarchy provide a flexible framework to model complex systems and help identify metrics that confer resilience in social-ecological systems.
The third development of resilience on coral reefs is motivated by the ecological and social applications described earlier and seeks field-based metrics to ascertain the relative “resilience” of sites (Hughes et al., 2005; McClanahan et al., 2012; Jouffray et al., 2015; Maynard et al., 2015). Because such snapshot metrics do not quantify dynamical aspects of the system – which is the preserve of engineering and ecological resilience – they do not measure resilience directly; rather, they are useful in identifying sites that have a desirable set of metrics that indicate greater resilience. A major value of these descriptive approaches is that they do not depend on sophisticated models and are relatively easy to implement in the field. Since this approach does not quantify resilience per se, these approaches could be categorized as “vulnerability” measures (Mumby et al., 2014a). However, since the term resilience is so widely used for these approaches, we continue its use here adding the discriminators “recovery” and “resistance” metrics sensu McClanahan et al. (2012).
Vulnerability as an Alternative to the Resilience Concept
The concept of vulnerability emerged from the field of risk and hazard research (White, 1974), and is the “degree to which a system, subsystem, or system component is likely to experience harm due to exposure to a hazard, either a perturbation or stressor” (Turner et al., 2003). Because vulnerability is an encompassing concept warranting a number of measurement approaches (Alwang et al., 2001; Eakin and Luers, 2006), we discriminate between approaches that attempt to quantify the absolute vulnerability of a system versus those that create a relative measure of vulnerability. Obtaining an absolute vulnerability measure typically requires the specification of a critical threshold followed by an estimate of the probability of its transgression (Adger, 2006). Examples might be the probability that coral cover falls below 10% within the next decade, or the risk of flooding in coastal cities in 2050 (Hallegatte et al., 2013). Estimation of absolute vulnerability requires a system model, whether it be statistical, analytical or simulation-based. Moreover, the absolute vulnerability of a reef is context-dependent in relation to the coral community that dominates a reef (e.g., fast-growing and sensitive Acropora versus slow-growing and robust Porites), driven by a combination of biological and environmental drivers (e.g., Done, 1982; Gouezo et al., 2019). For example, using simulation modeling for coral communities on the Great Barrier Reef and the Caribbean, Ortiz et al. (2014) showed that the vulnerability of reefs to thermal disturbances was dependent on the diversity of coral functional groups.
The second approach to measuring vulnerability, which we term “relative vulnerability,” often combines system metrics to compare vulnerability among sites or points in time. Here, the goal is not to calculate the absolute probability of an event occurring but rather to rank the relative vulnerability of sites and identify opportunities to reduce vulnerability (Eakin and Luers, 2006). A common way to measure relative vulnerability is to use the three components of the vulnerability framework: exposure, sensitivity and adaptive capacity (Adger, 2006; Cinner et al., 2012). Vulnerability is calculated using the equation “exposure + sensitivity – adaptive capacity,” popularized by the Intergovernmental Panel on Climate Change (McCarthy, 2001). Exposure is the nature and degree to which a system is exposed to stressors; sensitivity is the degree to which the stressors affect a system (i.e., and is the opposite of resistance); and adaptive capacity is the ability of the system to adjust to the stressors or recover (Gallopín, 2006). Metrics are chosen to represent exposure, sensitivity and adaptive capacity, and are measured and combined to provide a relative index for comparison of sites (Cinner et al., 2012). Sensitivity and adaptive capacity may be estimated based on existing biological understanding of the systems in question. For example, Cinner et al. (2012) produced a sensitivity based on the proportion of households engaged in fisheries and whether households also engage in non-fisheries components. This method has been used to compare the vulnerability of fishing communities in face of climate change (Allison et al., 2009; Cinner et al., 2013) and to identify priority areas for management (Maynard et al., 2010, 2015). Alternatively, many studies also seek to estimate the relative vulnerability of sites with a bespoke framework using specific components selected to suit the purpose of the study (i.e., resilience metrics).
Conceptual Framework for Measuring Reef Resilience and Vulnerability
Following the major concepts of ecological resilience, engineering resilience, and vulnerability, a framework of reef resilience quantification methods is proposed below and supported by case studies where applicable (Figure 1). Additional potential methods that have not been applied to reefs are also introduced under this framework. Note that we place empirical metrics relating to site resilience under “vulnerability” because they do not capture the dynamical properties of ecosystems but we agree that they can legitimately be described as resilience indices.
Ecological Resilience Related Approaches
Ecological Resilience
An emerging approach to calculate the resilience of coral reefs uses mechanistic ecological models to integrate available science. Mechanistic models can be used to ascertain whether multiple attractors are likely to exist and if so, predict the probability that a reef is pushed across tipping points within a specific period. This probabilistic approach embodies the original concept of ecological resilience (Holling, 1973) and is an alternative to quantifying the “level of disturbance” needed to tip a system, because the idea of measuring the “amount of disturbance” across multiple disparate forms of disturbance (bleaching, cyclones, etc.) is impenetrable (van Woesik, 2013). Rather, it is simpler to predict the probability of a system flipping attractor (see also van Nes and Scheffer (2007) who have developed a probabilistic approach to measuring the resilience of lacustrine (lake) ecosystems).
A number of models have been compared to independent time-series data for the Caribbean (Mumby et al., 2007; Kubicek and Borell, 2011) and Indo-Pacific (Melbourne-Thomas et al., 2011; Gurney et al., 2013; Sebastian and McClanahan, 2013; Ortiz et al., 2014). For instance, the ecological resilience of the Belize Barrier Reef was modeled and mapped under two levels of local management action (business-as-usual versus ban of herbivore fisheries) and two levels of action toward climate change (business-as-usual versus a green economy; Mumby et al., 2014b). Resilience was calculated as the probability that individual reefs would still be exhibiting coral recovery by 2030 (i.e., remaining under the attractor of a coral-dominated state even though coral dominance is never actually attained). The study found that implementation of a herbivore fisheries ban enacted in 2009 might increase the resilience of the reef sixfold, although the benefits were variable among reefs. The concept was further operationalized by identifying thresholds of herbivore harvest and size limits that maintain resilience in face of external disturbances (Bozec et al., 2016).
Precariousness
The prediction of resilience requires information on current system state, the location of underlying tipping points (unstable equilibrium, Figure 1), and predictive models of the disturbance regime. If the latter is unknown, an “instantaneous” measure of resilience can be obtained by estimating the distance of the reef to suspected tipping points. This is termed precariousness (Figure 1; Walker et al., 2004) and can be used as a relative measure of resilience among sites. The closer a reef sits from the unstable equilibrium, the more precarious it is. The measurement of precariousness requires a comparison of the current system state to known tipping points. Although precariousness has been suggested in the wider ecological literature (Walker et al., 2004; Hodgson et al., 2015), it has not been specifically measured for coral reefs. While the locations of unstable equilibria and thresholds have only been estimated for a very limited range of reefs, an interim approach might be to use the distance between a reef’s current state and the “tipping points” identified for some Indian Ocean and Caribbean reefs for average system state against fish biomass (McClanahan et al., 2011; Karr et al., 2015). One advantage is that precariousness does not require the user to undertake modeling themselves. However, its shortcoming is the inability to account for exposure to external disturbances, such that two sites might lie equidistant from tipping points (i.e., same precariousness) with one having a greater risk of being perturbed and hence lower resilience. In summary, the technique has its merits as a simple tool to compare relative resilience of sites that share a similar disturbance regime (Yeung and Richardson, 2015).
Current Attractor
An even simpler approach than precariousness is to know whether the reef is likely to exhibit recovery or decline before the next disturbance. The current attractor identifies whether a reef is attracted toward the coral or alternative state at a point in time (Figure 1). If a snapshot of the reef state is available, then, like precariousness, the identification of the current attractor requires existing knowledge on the unstable equilibrium to identify which basin of attraction the reef currently sits in (i.e., coral-, algal- or sponge-attractors). In the absence of models of the ecosystem, it might be possible to estimate the existence of tipping points from statistical analyses of monitoring data; i.e., community states where reef trajectories are uncertain and variable showing either recovery or decline between disturbances (Zychaluk et al., 2012). Analyses of this type would require extensive data with at least short time series. The direction of each post-disturbance trajectory would allow the attractor to be identified at each initial state. Care must be taken not to confound this analyses with data from different physical environments where different attractors might occur (e.g., whereas a reef at 5 m might show recovery when coral cover is say 10%, a reef at 30 m might show decline when coral cover reaches an equivalent level). Moreover, a suite of early-warning algorithms have been developed to indicate whether a system is approaching a tipping point (Scheffer et al., 2009). The efficacy of such approaches, which often focus on critical slowing down of dynamics near tipping points, remain uncertain for coral reefs which are non-equilibrial ecosystems driven by massive episodic disturbance.
Engineering Resilience Related Approaches
The original definition of engineering resilience is impractical for coral reefs because reefs rarely attain a single coral-dominated equilibrium. However, two aspects of recovery rate can be operationalized. The first is a short-term recovery rate, usually measured directly from time-series. The second, termed reef performance, uses models to hindcast reef trajectories or project into the future, typically over longer periods of time (Mumby and Anthony, 2015).
Short-Term Recovery Rates
Rates of change and trends have commonly been used to examine coral reef recovery and decline by tracking changes in coral cover over time (Emslie et al., 2008; Graham et al., 2011; Johns et al., 2014). Despite being easily applicable, simple averaging is problematic as calculated rates are highly influenced by the initial coral cover and assumes linear growth rates (Côté et al., 2006). A useful way to obtain short-term recovery rate is through statistical models that can incorporate ecological processes and dynamics such as multivariate autoregression models (Zychaluk et al., 2012; Cooper et al., 2015; Gross and Edmunds, 2015; Lam et al., 2018). Recovery is usually quantified using exponential rates to reduce the dependence on initial state, such as the instantaneous growth rate of a logistic growth function (Haddon, 2001). Reefs with faster recovery rates have higher engineering resilience. In a coral reef context, Mumby (2009) demonstrated that recovery rates increase the farther the system sits from a tipping point (though they can slow as they approach a stable equilibrium). Thus, recovery rates can also be used as an instantaneous proxy of ecological resilience irrespective of future disturbance, and are correlated to precariousness. It is important to distinguish the two approaches of short-term recovery rates and current attractor, although both relies on recovery rates, Current Attractor relies on impacts of disturbance and observations of the recovery trend immediately after the impact. Rates of short-term recovery are also related to the diversity of corals in any given system – dependent on the ocean geographical location being studied – as well as other ecological characteristics of the system such as fish diversity, system state following disturbance, and anthropogenic pollution (Bellwood et al., 2004; Fabricius, 2005; Graham et al., 2011, 2015). While short-term recovery rates cannot project reef state far into the future, they provide a useful basis for comparing the recoverability of reef locations (Ortiz et al., 2018). Moreover, while many studies rely on total coral cover as a single metric to examine return rate, it is also necessary to consider the type of community of corals in multivariate space or by functional groups (e.g., Ortiz et al., 2014; Tanner, 2017; Kayal et al., 2018; Gouezo et al., 2019).
Reef Performance
In many cases, including the GBR, the state of the reef might decline despite significant investments in reef management such as improvements in water quality (De’ath et al., 2012). Here, the value of management is best viewed using counterfactual analyses that ask how much worse the system would look had management action been reduced (Mumby et al., 2017). Or alternatively, how much healthier is the reef given our management interventions (Figure 3). Projecting how the reef might look into the future under different management scenarios provides a basis for management strategy evaluation. Mumby and Anthony (2015) proposed a framework that compares the trajectories of reefs under different levels of management and explicitly differentiates the degree to which local manageable stressors versus climate change and ocean acidification would contribute to loss of future reef state (see also Wolff et al., 2018). Such comparisons can also incorporate global differences in fish assemblages that result from different management strategies (Edwards et al., 2014; Harborne et al., 2018).
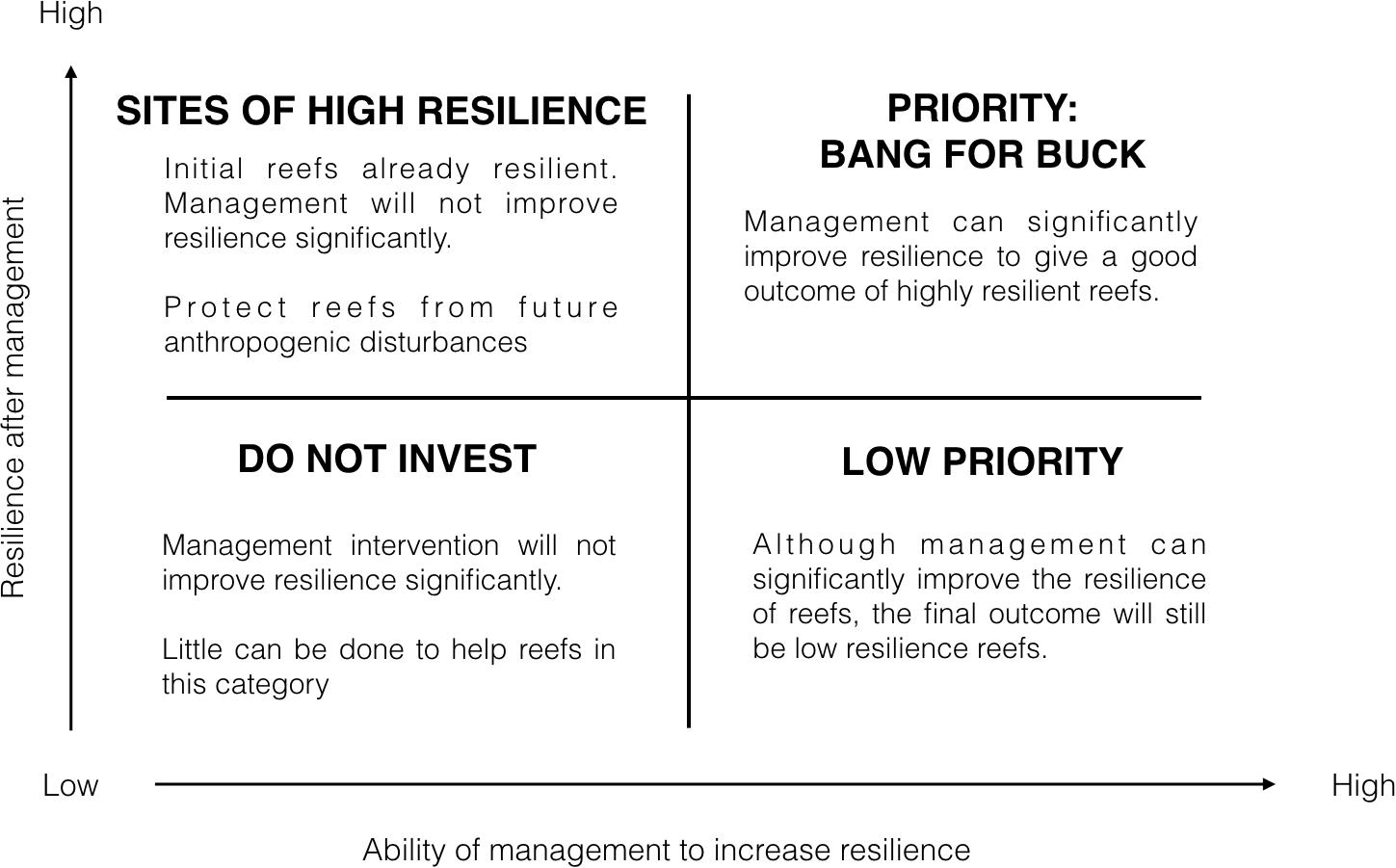
Figure 3. Decision making framework for investment strategy for locations with varying resilience levels and potential management impact, modified from Mumby and Anthony (2015).
Because such methods are based on projections of reefs into the future they rely on models, even if simplistic. The goal is to compare alternative possible trajectories that might include (i) a purely natural system where the stressors could include cyclones but no climate change, (ii) the addition of climate change and ocean acidification, (iii) further addition of unmanageable local stressors (which may include historical accumulation of pollutants), and (iv) the further addition of potentially manageable stressors (e.g., anchor damage, fishing, crown-of-thorns starfish) (Figure 4). Reef performance simply expresses the average state of the reef under a one trajectory as a percentage of the reef’s state under a less stressful trajectory. For example, a performance of 50% over the years 2020–2030 implies that coral cover under business-as-usual (all stressors) will, on average, be half of that in the absence of a pristine ecosystem between the years 2020 and 2030.
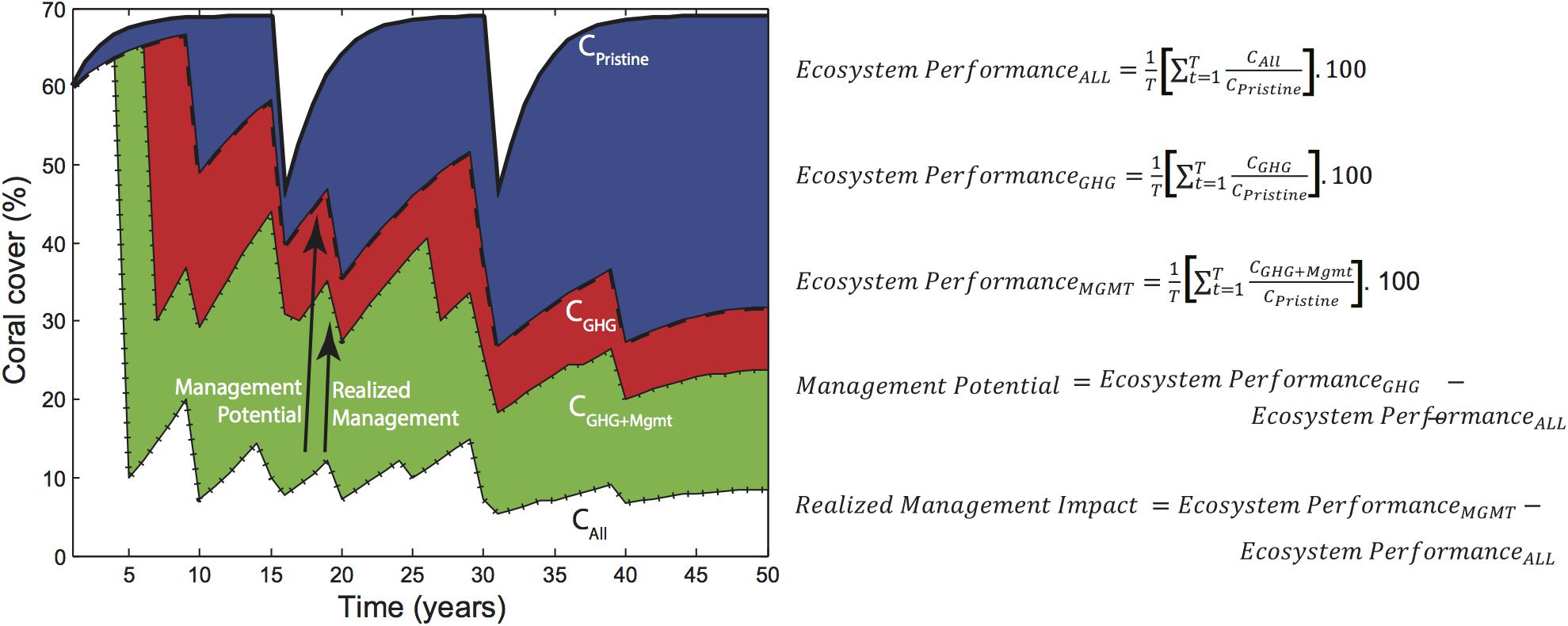
Figure 4. Use of Reef Performance metrics with modeled projections of reef futures with various levels of stressors (adapted from Mumby and Anthony, 2015). Results integrated over T time steps, t.
The approach can be applied irrespective of the existence of alternative attractors as it simply compares potential trajectories. Metrics of relative management potential can then be calculated and compared to inform decisions. This approach has been taken at a coarse scale on the Great Barrier Reef (Wolff et al., 2018), and is mostly limited by the spatial resolution of input data layers (particularly climate projections) and scientific understanding of the changes in reef state that management can elicit.
Vulnerability Related Approaches
Despite its roots in social science, the vulnerability concept is increasingly used in ecology (Beroya-Eitner, 2016) and thus incorporated in the proposed resilience framework (Figure 1). Vulnerability is context-specific (Barnett et al., 2008) and its measurement can be grouped into two general categories. Absolute vulnerability allows comparisons across space and time, whereas relative measures only indicate rankings and are project/case-specific. It is useful to consider the suitability of using absolute or relative measures as data and skill requirements differ greatly (Alwang et al., 2001).
Absolute Vulnerability
A measure of absolute vulnerability can be achieved through simulation or statistical models, providing data are available for the appropriate parameterization. The measurement of absolute vulnerability requires an explicit benchmark (Alwang et al., 2001), such as the vulnerability of corals to an increase of 1°C in seawater temperatures, to physical hurricane impact, or any other specific kind of disturbance. This approach is best implemented using models, be they statistical or otherwise. System models are useful in quantifying vulnerability because the incorporation of explicit biological responses and ecological interactions lend themselves to projecting the response of a system into new environments (Scheffer and Carpenter, 2003; Nyström et al., 2008). One example of an absolute vulnerability measurement in coral reef ecosystems is estimation of the number of years before coral cover fell below 10% in Belize (Mumby et al., 2014b). Statistical approaches offer an alternative to simulation models for understanding system dynamics. For example, Zychaluk et al. (2012) used a Markov process to project the average trajectory of coral reefs toward coral, algal or other endpoints. Alternative model formulations are also being used to incorporate a greater range of covariates (Cooper et al., 2015), explore reef ecosystem stability in response to multiple disturbances (Gross and Edmunds, 2015), and to determine trajectories using Bayesian methods through reef accretion and recovery following disturbance (van Woesik, 2013).
Relative Vulnerability and Resilience Indices
Most published assessments of reef resilience utilized relative vulnerability and identified a series of reef attributes that confer resilience. Attributes are measured in the field, scored and aggregated to provide an overall “resilience metric” at each reef location. Attributes are chosen by literature review (Obura and Grimsditch, 2009), collegial expert opinion (McClanahan et al., 2012), consultation of fishermen to anticipate social-economical responses to change (Marshall and Marshall, 2007), and relevance to geographic location (Maynard et al., 2010). As vulnerability approaches stems from social science, studies under this category most readily incorporate both human stressors in addition to environmental and ecological factors. Individual attributes are often weighted by importance prior to integration (Maynard et al., 2010; McClanahan et al., 2012). For example, Maynard et al. (2010) created an empirical framework where resilience attributes (indicators) were classified, ranked and weighted following scientific evidence. The final resilience score is simply the sum of all weighted scores. The great benefit of this metrics approach (i.e., relative vulnerability) is that it can be measured easily in the field and used to rank the vulnerability of sites (Beroya-Eitner, 2016). Like all the approaches reviewed, it has some limitations and caveats (Table 1), which are well known by implementers of these methods.
Most resilience indices do not measure ecological processes directly; rather they focus on state (e.g., coral cover) or proxies of process (e.g., fish biomass for herbivory). Not surprisingly, it is preferable to use the closest proxy to the process as possible. For example, a study of the association between herbivory and juvenile coral density on Caribbean reefs found that better metrics explained were three times more successful at resolving the relationship (Steneck et al., 2018). Parrotfish biomass only explained 8% of the variance of juvenile coral density whereas a metric that accounted for parrotfish species and size effects on grazing, and the surface area of grazable substrate explained 23% of variance.
Although the use of weightings can emphasize the disproportionate importance of some processes over others (Maynard et al., 2010), an implicit assumption remains that processes integrate linearly (Barnett et al., 2008). In reality, reinforcing feedback mechanisms are important drivers of coral reef dynamics (Mumby and Steneck, 2008) and tend to generate non-linear interactions among processes (Birkeland, 2004). For example, the outcome of having a “high” value for coral larval supply depends strongly on the processes that influence settlement and post-settlement mortality, such as macroalgal cover (Dixon et al., 2015; Doropoulos et al., 2015) and corallivory (Doropoulos et al., 2016). The non-linear and context-dependencies among processes are more easily dealt with using mechanistic models. It is also important to recognize that vulnerability assessments are sensitive to the number of metrics included; the more metrics included, the less any particular attribute can influence the overall score (McClanahan et al., 2012). Yet an extreme value for one attribute (e.g., macroalgal cover) might be sufficient to reduce recovery severely (Mumby et al., 2015).
Metrics are often assumed to be uni-directional, but factors can affect resilience in both directions depending on context and environment (Table 2). For example, ocean currents that contribute to connectivity may also become a threat to resilience by carrying pollutants, nutrients and diseases (Nyström et al., 2000; McClanahan et al., 2002). Finally, while the empirical nature of vulnerability metrics approach is appealing, its dependence on field surveys tends to make it difficult to scale up. This is the converse problem with modeling studies which have large spatial extent but limited resolution at small-scales. Ultimately, the choice of approach (or approaches) will be determined, in part, by the scale or scales at which management is focused. Recent studies have advocated a nested approach that makes this explicit (Maynard et al., 2015).
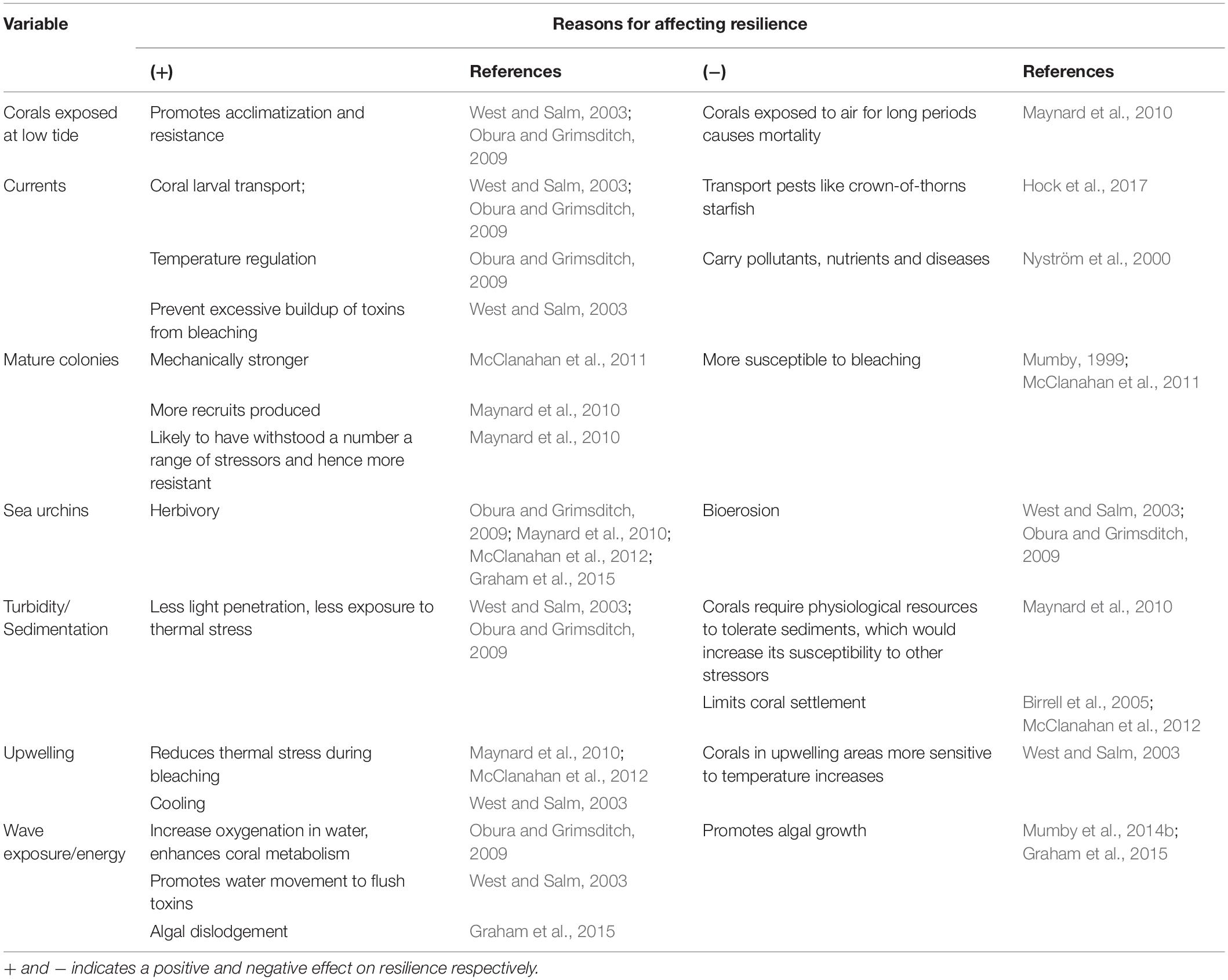
Table 2. Variables used in resilience assessments that can have both positive and negative effects on resilience.
Application of Resilience Assessments to Reef Management
The multiplicity of methods for resilience assessments has outpaced their incorporation into management. Each resilience method can be used to answer a suite of management questions (Table 3). Commonly, the end-point for many resilience assessments is a map of ranked vulnerabilities or “resilience metrics,” yet the pathway for these metrics to be incorporated into management decisions can be unclear. There has been some consideration on how to prioritize depending on the level of threats expected. Game et al. (2008) and Anthony et al. (2015) put forward comprehensive frameworks for the management process. What appears to be under-developed is formalizing the link between the nature of a management intervention and its expected influence on resilience (or vulnerability).
A useful attempt to operationalize RBM utilized a metrics-based approach and identified metrics that were under potential management control and scored the likely ease of implementing such management at the site (Maynard et al., 2010). Another key consideration would be the degree to which management would improve the outlook or resilience score if implemented. Mumby et al. (2014b) specifically simulated the impact of a change in fisheries policy and mapped the expected increase in resilience across the reef. Mumby and Anthony (2015) went further and suggested management prioritizations would benefit from a simple framework that plotted current state or resilience on one axis and the degree to which management could improve that state or resilience on the other (Figure 3). A given management intervention would achieve the greatest “bang for buck” where the scope for increasing resilience is maximized. Similarly, areas of low resilience that cannot be improved by management would receive a low priority for intervention.
A similar strategy to estimate the benefits of management implementation could be developed for metrics-based approaches. In addition to a site’s current vulnerability score, it would be possible to estimate the degree to which an intervention might increase that score, subject to local constraints. For example, a site might only have a standardized herbivory score of 0.5, implying intermediate herbivorous fish biomass. Were a marine reserve to be established, herbivory would be expected to increase to the maximum score possible subject to limitations of the benthos, such as the local habitat complexity and the availability of food (cover of algal turfs). The researcher might turn to relevant analyses of bivariate relationships between variables to estimate these constraints; i.e., the relationships between herbivore biomass, habitat complexity, and algal cover (McClanahan et al., 2011; Karr et al., 2015). The outcome might be that herbivory could increase to a value of 0.8, which when combined with other influences of a reserve on other metrics, leads to a new vulnerability measure, “potential vulnerability if management enacted.” Moreover, alternate attractors are not always between coral and macroalgal dominated states. For example, transitions from coral to other organism assemblages have been documented throughout the globe and include shifts to corallimorphs, soft corals, sponges, urchin barrens, sea anemones, and ascidians (Norström et al., 2009). Management interventions to reduce the probability of these transitions remaining stable require different strategies to managing transitions from corals to macroalgae.
To move toward strategic management planning, predictive approaches that link interventions to probable future reef responses would be useful. Given that the use of empirical metrics is the most widely-used resilience quantification approach, scientists need to facilitate the efficacy and predictability of metrics-based approaches. We believe there has not yet been a study to resolve which metrics has the most predictive power, and a meta-analysis is long overdue. As the key metrics are resolved, studies can take into account the relative influence of metrics on resilience and produce a more nuanced analysis based on the interactions of multiple metrics. There are specific examples finding rugosity and herbivory to be predictors of flipping to from coral-dominated to algal-dominated attractors. Studies from the Caribbean and Pacific have both found algal turf canopy height to be a good predictor of coral recruitment failure, as well as macroalgal cover. Most resilience indicators are snapshot datasets, such as biomass, cover, and structural complexity. In some cases it is hoped that data like herbivore biomass is a reasonable proxy of herbivory (though see Steneck et al., 2018, for its limitations). Yet longer-term data on key processes, such as calcification – derived from coral cores – have rarely (if ever) been used as part of a resilience/vulnerability assessment. It would be instructive to evaluate the predictive power of such metrics as good indicators of, say exposure to stressful environments. One challenge is to create a more sophisticated but accessible method for the integration of attribute values that captures the complex non-linear interactions of physical, biological and ecological processes on reefs (Barbier et al., 2008; Nyström et al., 2008). This could be achieved by coupling ecological models with an interface that allows users to enter their attribute data and receive a prediction of resilience or recovery rate. Some attempts have been made to provide a means of diagnosing and interpreting reef ecological data, but these remain in their infancy (Flower et al., 2017). A more formal quantitative approach would utilize monitoring datasets from specific reefs into a larger statistical framework – such as Bayesian Belief Networks (Wooldridge and Done, 2004; Renken and Mumby, 2009) – capable of making short-term predictions for a given reef’s outlook based on local environmental effects, current state, and disturbance history (Eason et al., 2016). Such statistical models would not only help practitioners identify appropriate resilience attributes for a given context, but they would help utilize the vast amounts of monitoring data available. And importantly, by providing a tool to help understand reef resilience, they would provide an incentive for practitioners and scientists to contribute their data and build a community-wide understanding of the drivers of reef health in different environments.
Author Contributions
PM and VL conceived the original idea. VL conducted the literature review, collected the data, and wrote the manuscript. PM discussed and provided inputs on the interpretation of the results and co-authored the manuscript. CD and Y-MB discussed ideas and edited the manuscript.
Funding
This project is supported with funding from the Australian Government’s National Environmental Science Program and an Australian Research Council Linkage Grant to PM; and an International Postgraduate Research Scholarship to VL.
Conflict of Interest
The authors declare that the research was conducted in the absence of any commercial or financial relationships that could be construed as a potential conflict of interest.
References
Allen, C. R., Angeler, D. G., Cumming, G. S., Folke, C., Twidwell, D., and Uden, D. R. (2016). Quantifying spatial resilience. J. Appl. Ecol. 53, 625–635. doi: 10.1111/1365-2664.12634
Allison, E. H., Perry, A. L., Badjeck, M.-C., Neil Adger, W., Brown, K., Conway, D., et al. (2009). Vulnerability of national economies to the impacts of climate change on fisheries. Fish Fish. 10, 173–196. doi: 10.1111/j.1467-2979.2008.00310.x
Alwang, J., Siegel, P. B., and Jorgensen, S. L. (2001). “Vulnerability : a view from different disciplines (English),” in Social Protection Discussion Paper Series; No. SP 0115 (Washington, DC: The World Bank).
Andres, N. G., and Rodenhouse, N. L. (1993). Resilience of corals to hurricanes: a simulation model. Coral Reefs 12, 167–175. doi: 10.1007/BF00334476
Angeler, D. G., and Allen, C. R. (2016). Quantifying resilience. J. Appl. Ecol. 53, 617–624. doi: 10.1111/1365-2664.12649
Anthony, K. R. N., Marshall, P. A., Abdulla, A., Beeden, R., Bergh, C., Black, R., et al. (2015). Operationalizing resilience for adaptive coral reef management under global environmental change. Glob. Chang. Biol. 21, 48–61. doi: 10.1111/gcb.12700
Anthony, K. R. N., Maynard, J. A., Diaz-Pulido, G., Mumby, P. J., Marshall, P. A., Cao, L., et al. (2011). Ocean acidification and warming will lower coral reef resilience. Glob. Chang. Biol. 17, 1798–1808. doi: 10.1111/j.1365-2486.2010.02364.x
Barbier, E. B., Koch, E. W., Silliman, B. R., Hacker, S. D., Wolanski, E., Primavera, J., et al. (2008). Coastal ecosystem-based management with nonlinear ecological functions and values. Science 319, 321–323. doi: 10.1126/science.1150349
Barnett, J., Lambert, S., and Fry, I. (2008). The hazards of indicators: insights from the environmental vulnerability index. Ann. Assoc. Am. Geogr. 98, 102–119. doi: 10.1080/00045600701734315
Bellwood, D. R., Baird, A. H., Depczynski, M., González-Cabello, A., Hoey, A. S., Lefèvre, C. D., et al. (2012). Coral recovery may not herald the return of fishes on damaged coral reefs. Oecologia 170, 567–573. doi: 10.1007/s00442-012-2306-z
Bellwood, D. R., Hughes, T. P., Folke, C., and Nyström, M. (2004). Confronting the coral reef crisis. Nature 429, 827–833. doi: 10.1038/nature02691
Beroya-Eitner, M. A. (2016). Ecological vulnerability indicators. Ecol. Indic. 60, 329–334. doi: 10.1016/j.ecolind.2015.07.001
Birrell, C. L., McCook, L. J., and Willis, B. L. (2005). Effects of algal turfs and sediment on coral settlement. Mar. Pollut. Bull. 51, 408–414. doi: 10.1016/j.marpolbul.2004.10.022
Blackwood, J. C., Hastings, A., and Mumby, P. J. (2012). The effect of fishing on hysteresis in Caribbean coral reefs. Theor. Ecol. 5, 105–114. doi: 10.1007/s12080-010-0102-0
Bozec, Y.-M., and Mumby, P. J. (2015). Synergistic impacts of global warming on the resilience of coral reefs. Philos. Trans. R. Soc. B Biol. Sci. 370:20130267. doi: 10.1098/rstb.2013.0267
Bozec, Y.-M., O’Farrell, S., Bruggemann, J. H., Luckhurst, B. E., and Mumby, P. J. (2016). Tradeoffs between fisheries harvest and the resilience of coral reefs. Proc. Natl. Acad. Sci.U.S.A. 113, 4536–4541. doi: 10.1073/pnas.1601529113
Brand, F. (2009). Critical natural capital revisited: ecological resilience and sustainable development. Ecol. Econ. 68, 605–612. doi: 10.1016/j.ecolecon.2008.09.013
Brand, F. S., and Jax, K. (2007). Focusing the meaning(s) of resilience: resilience as a descriptive concept and a boundary object. Ecol. Soc. 12:23.
Brown, B. E., Suharsono (1990). Damage and recovery of coral reefs affected by El Niño related seawater warming in the Thousand Islands, Indonesia. Coral Reefs 8, 163–170. doi: 10.1007/BF00265007
Bruckner, A. W. (2012). Static measurements of the resilience of Caribbean coral populations. Rev. Biol. Trop. 60, 39–57. doi: 10.15517/rbt.v60i0.19844
Carpenter, S. R., Ludwig, D., and Brock, W. A. (1999). Management of eutrophication for lakes subject to potentially irreversible change. Ecol. Appl. 9, 751–771. doi: 10.1890/1051-0761(1999)009[0751:moefls]2.0.co;2
Cinner, J. E., Huchery, C., Darling, E. S., Humphries, A. T., Graham, N. A. J., Hicks, C. C., et al. (2013). Evaluating social and ecological vulnerability of coral reef fisheries to climate change. PLoS One 8:e74321. doi: 10.1371/journal.pone.0074321
Cinner, J. E., McClanahan, T., Graham, N., Daw, T., Maina, J., Stead, S., et al. (2012). Vulnerability of coastal communities to key impacts of climate change on coral reef fisheries. Glob. Environ. Chang. 22, 12–20. doi: 10.1016/j.gloenvcha.2011.09.018
Common, M., and Perrings, C. (1992). Towards an ecological economics of sustainability. Ecol. Econ. 6, 7–34. doi: 10.1016/0921-8009(92)90036-r
Connell, J. H. (1997). Disturbance and recovery of coral assemblages. Coral Reefs 16, S101–S113. doi: 10.1007/s003380050246
Cooper, J. K., Spencer, M., and Bruno, J. F. (2015). Stochastic dynamics of a warmer Great Barrier Reef. Ecology 96, 1802–1811. doi: 10.1890/14-0112.1
Côté, I. M., Reynolds, J. D., Gardner, T. A., Gill, J. A., Hutchinson, D. J., and Watkinson, A. R. (2006). “New approaches to estimating recent ecological changes on coral reefs,” in Coral Reef Conservation, eds I. M. Côté and J. D. Reynolds (Cambridge: Cambridge University Press), 293–313. doi: 10.1017/cbo9780511804472.011
Cowen, R. K., Paris, C. B., and Srinivasan, A. (2006). Scaling of connectivity in marine populations. Science 311, 522–527. doi: 10.1126/science.1122039
Dakos, V., Carpenter, S. R., van Nes, E. H., and Scheffer, M. (2015). Resilience indicators: prospects and limitations for early warnings of regime shifts. Philos. Trans. R. Soc. B Biol. Sci. 370:1659. doi: 10.1098/rstb.2013.0263
Davidson, J. L., Jacobson, C., Lyth, A., Dedekorkut-Howes, A., Baldwin, C. L., Ellison, J. C., et al. (2016). Interrogating resilience: toward a typology to improve its operationalization. Ecol. Soc. 21:27.
De’ath, G., Fabricius, K. E., Sweatman, H., and Puotinen, M. (2012). The 27–year decline of coral cover on the Great Barrier Reef and its causes. Proc. Natl. Acad. Sci. U.S.A. 109, 17995–17999. doi: 10.1073/pnas.1208909109
Dixon, G. B., Davies, S. W., Aglyamova, G. V., Meyer, E., Bay, L. K., and Matz, M. V. (2015). Genomic determinants of coral heat tolerance across latitudes. Science 348, 1460–1462. doi: 10.1126/science.1261224
Done, T. J. (1982). Patterns in the distribution of coral communities across the central Great Barrier Reef. Coral Reefs 1, 95–107. doi: 10.1007/bf00301691
Done, T. J. (1992). Phase-shifts in coral-reef communities and their ecological significance. Hydrobiologia 247, 121–132. doi: 10.1007/bf00008211
Doropoulos, C., Roff, G., Bozec, Y.-M., Zupan, M., Werminghausen, J., and Mumby, P. J. (2016). Characterising the ecological trade-offs throughout the early ontogeny of coral recruitment. Ecol. Monogr. 86, 20–44. doi: 10.1890/15-0668.1
Doropoulos, C., Ward, S., Roff, G., Gonzalez-Rivero, M., and Mumby, P. J. (2015). Linking demographic processes of juvenile corals to benthic recovery trajectories in two common reef habitats. PLoS One 10:e0128535. doi: 10.1371/journal.pone.0128535
Eakin, H., and Luers, A. L. (2006). Assessing the vulnerability of social-environmental systems. Annu. Rev. Environ. Resour. 31, 365–394. doi: 10.1146/annurev.energy.30.050504.144352
Eason, T., Garmestani, A. S., Stow, C. A., Rojo, C., Alvarez-Cobelas, M., and Cabezas, H. (2016). Managing for resilience: an information theory-based approach to assessing ecosystems. J. Appl. Ecol. 53, 656–665. doi: 10.1111/1365-2664.12597
Edwards, C. B., Friedlander, A. M., Green, A. G., Hardt, M. J., Sala, E., Sweatman, H. P., et al. (2014). Global assessment of the status of coral reef herbivorous fishes: evidence for fishing effects. Proc. R. Soc. Lond. B Biol. Sci. 281:20131835. doi: 10.1098/rspb.2013.1835
Emslie, M., Cheal, A., Sweatman, H., and Delean, S. (2008). Recovery from disturbance of coral and reef fish communities on the Great Barrier Reef, Australia. Mar. Ecol. Prog. Ser. 371, 177–190. doi: 10.3354/meps07657
Eraydin, A., and Tasan-Kok, T. (eds) (2013). Resilience Thinking In Urban Planning. Dordrecht: Springer.
Fabricius, K. E. (2005). Effects of terrestrial runoff on the ecology of corals and coral reefs: review and synthesis. Mar. Pollut. Bull. 50, 125–146. doi: 10.1016/j.marpolbul.2004.11.028
Fabricius, K. E., Langdon, C., Uthicke, S., Humphrey, C., Noonan, S., De’ath, G., et al. (2011). Losers and winners in coral reefs acclimatized to elevated carbon dioxide concentrations. Nat. Clim. Chang. 1, 165–169. doi: 10.1038/nclimate1122
Flower, J., Ortiz, J. C., Chollett, I., Abdullah, S., Castro-Sanguino, C., Hock, K., et al. (2017). Interpreting coral reef monitoring data: a guide for improved management decisions. Ecol. Indic. 72, 848–869. doi: 10.1016/j.ecolind.2016.09.003
Folke, C. (2006). Resilience: the emergence of a perspective for social–ecological systems analyses. Glob. Environ. Chang. 16, 253–267. doi: 10.1016/j.gloenvcha.2006.04.002
Folke, C., Carpenter, S. R., Walker, B., Scheffer, M., Chapin, T., and Rockström, J. (2010). Resilience thinking: integrating resilience, adaptability and transformability. Ecol. Soc. 15:20.
Fung, T., Seymour, R. M., and Johnson, C. R. (2011). Alternative stable states and phase shifts in coral reefs under anthropogenic stress. Ecology 92, 967–982. doi: 10.1890/10-0378.1
Gallopín, G. C. (2006). Linkages between vulnerability, resilience, and adaptive capacity. Glob. Environ. Chang. 16, 293–303. doi: 10.1016/j.gloenvcha.2006.02.004
Game, E. T., McDonald-Madden, E., Puotinen, M. L., and Possingham, H. P. (2008). Should we protect the strong or the weak? Risk, resilience, and the selection of marine protected areas. Conserv. Biol. 22, 1619–1629. doi: 10.1111/j.1523-1739.2008.01037.x
R. N. Ginsburg (ed.) (1993). Global Aspects Of Coral Reefs: Health, Hazards And History. Miami, FL: Stanford University.
Gouezo, M., Golbuu, Y., Fabricius, K., Olsudong, D., Mereb, G., Nestor, V., et al. (2019). Drivers of recovery and reassembly of coral reef communities. Proc. Biol. Sci. 286:20182908. doi: 10.1098/rspb.2018.2908
Graham, N., Nash, K., and Kool, J. (2011). Coral reef recovery dynamics in a changing world. Coral Reefs 30, 283–294. doi: 10.1007/s00338-010-0717-z
Graham, N. A. J., Jennings, S., MacNeil, M. A., Mouillot, D., and Wilson, S. K. (2015). Predicting climate-driven regime shifts versus rebound potential in coral reefs. Nature 518, 94–97. doi: 10.1038/nature14140
Gross, K., and Edmunds, P. J. (2015). Stability of Caribbean coral communities quantified by long-term monitoring and autoregression models. Ecology 96, 1812–1822. doi: 10.1890/14-0941.1
Guest, J. R., Edmunds, P. J., Gates, R. D., Kuffner, I. B., Andersson, A. J., Barnes, B. B., et al. (2018). A framework for identifying and characterising coral reef “oases” against a backdrop of degradation. J. Appl. Ecol. 55, 2865–2875. doi: 10.1111/1365-2664.13179
Gunderson, L. H., and Holling, C. S. (eds) (2001). Panarchy: Understanding Transformations In Human And Natural Systems. Washington D.C: Island Press.
Gurney, G. G., Melbourne-Thomas, J., Geronimo, R. C., Aliño, P. M., and Johnson, C. R. (2013). Modelling coral reef futures to inform management: can reducing local-scale stressors conserve reefs under climate change? PLoS One 8:e80137. doi: 10.1371/journal.pone.0080137
Hallegatte, S., Green, C., Nicholls, R. J., and Corfee-Morlot, J. (2013). Future flood losses in major coastal cities. Nat. Clim. Chang. 3, 802–806. doi: 10.1038/nclimate1979
Harborne, A. R., Green, A. L., Peterson, N. A., Beger, M., Golbuu, Y., Houk, P., et al. (2018). Modelling and mapping regional-scale patterns of fishing impact and fish stocks to support coral-reef management in Micronesia. Divers. Distribut. 24, 1729–1743. doi: 10.1111/ddi.12814
Hatcher, B. (1984). A maritime accident provides evidence for alternate stable states in benthic communities on coral reefs. Coral Reefs 3, 199–204. doi: 10.1007/bf00288255
Hock, K., Wolff, N. H., Ortiz, J. C., Condie, S. A., Anthony, K. R. N., Blackwell, P. G., et al. (2017). Connectivity and systemic resilience of the Great Barrier Reef. PLoS Biol. 15:e2003355. doi: 10.1371/journal.pbio.2003355
Hodgson, D., McDonald, J. L., and Hosken, D. J. (2015). What do you mean, ‘resilient’? Trends Ecol. Evol. 30, 503–506. doi: 10.1016/j.tree.2015.06.010
Holling, C. S. (1961). Principles of insect predation. Ann. Rev. Entomol. 6, 163–182. doi: 10.1146/annurev.en.06.010161.001115
Holling, C. S. (1973). Resilience and stability of ecological systems. Annu. Rev. Ecol. Syst. 4, 1–23. doi: 10.1146/annurev.es.04.110173.000245
Holling, C. S. (1996). “Engineering resilience versus ecological resilience,” in Engineering Within Ecological Constraints, ed. P. C. Schulze (Washington D.C: National Academy Press), 31–44.
Hughes, T., and Connell, J. (1999). Multiple stressors on coral reefs: a long-term perspective. Limnol. Oceanogr. 44, 932–940. doi: 10.1038/s41467-019-12431-y
Hughes, T. P. (1994). Catastrophes, phase shifts, and large-scale degradation of a Caribbean coral reef. Science 265, 1547–1551. doi: 10.1126/science.265.5178.1547
Hughes, T. P., Bellwood, D. R., Folke, C., Steneck, R. S., and Wilson, J. (2005). New paradigms for supporting the resilience of marine ecosystems. Trends Ecol. Evol. 20, 380–386. doi: 10.1016/j.tree.2005.03.022
Johns, K., Osborne, K., and Logan, M. (2014). Contrasting rates of coral recovery and reassembly in coral communities on the Great Barrier Reef. Coral Reefs 33, 553–563. doi: 10.1007/s00338-014-1148-z
Jouffray, J. B., Nystrom, M., Norstrom, A. V., Williams, I. D., Wedding, L. M., Kittinger, J. N., et al. (2015). Identifying multiple coral reef regimes and their drivers across the Hawaiian archipelago. Philos. Trans. R. Soc. B Biol. Sci. 370:20130268. doi: 10.1098/rstb.2013.0268
Karr, K. A., Fujita, R., Halpern, B. S., Kappel, C. V., Crowder, L., Selkoe, K. A., et al. (2015). Thresholds in Caribbean coral reefs: implications for ecosystem-based fishery management. J. Appl. Ecol. 52, 402–412. doi: 10.1111/1365-2664.12388
Kayal, M., Lenihan, H. S., Brooks, A. J., Holbrook, S. J., Schmitt, R. J., and Kendall, B. E. (2018). Predicting coral community recovery using multi-species population dynamics models. Ecol. Lett. 21, 1790–1799. doi: 10.1111/ele.13153
Kinzig, A. P., Ryan, P., Etienne, M., Allison, H., Elmqvist, T., and Walker, B. H. (2006). Resilience and regime shifts: assessing cascading effects. Ecol. Soc. 11:20.
Knowlton, N. (1992). Thresholds and multiple stable states in coral reef community dynamics. Am. Zool. 32, 674–682. doi: 10.2307/3883648
Kubicek, A., and Borell, E. (2011). “Modelling resilience and phase shifts in coral reefs: application of different modelling approaches,” in Modelling Complex Ecological Dynamics, eds F. Jopp, H. Reuter, and B. Breckling (Berlin: Springer), 241–255. doi: 10.1007/978-3-642-05029-9_17
Lam, V. Y. Y., Chaloupka, M., Thompson, A., Doropoulos, C., and Mumby, P. J. (2018). Acute drivers influence recent inshore Great Barrier Reef dynamics. Proc. Biol. Sci. 285:20182063. doi: 10.1098/rspb.2018.2063
Lebel, L., Anderies, J. M., Campbell, B., Folke, C., Hatfield-Dodds, S., Hughes, T. P., et al. (2006). Governance and the capacity to manage resilience in regional social-ecological systems. Ecol. Soc. 11:19.
Lewontin, R. C. (1969). The meaning of stability. Brookhaven Symposia Biol. Divers. Stabil. Ecol. Syst. 22, 12–24.
Luthar, S. S., Cicchetti, D., and Becker, B. (2000). The construct of resilience: a critical evaluation and guidelines for future work. Child Dev. 71, 543–562. doi: 10.1111/1467-8624.00164
Mamauag, S. S., and Alin̄o, P. M., Martinez, R. J. S., Muallil, R. M., Doctor, M. V. A., Dizon, E. C., et al. (2013). A framework for vulnerability assessment of coastal fisheries ecosystems to climate change—Tool for understanding resilience of fisheries (VA-TURF). Fish. Res. 147, 381–393. doi: 10.1016/j.fishres.2013.07.007
Manyena, S. B. (2006). The concept of resilience revisited. Disasters 30, 434–450. doi: 10.1111/j.0361-3666.2006.00331.x
Marshall, N. A. (2010). Understanding social resilience to climate variability in primary enterprises and industries. Glob. Environ. Chang. 20, 36–43. doi: 10.1016/j.gloenvcha.2009.10.003
Marshall, N. A., and Marshall, P. A. (2007). Conceptualizing and operationalizing social resilience within commercial fisheries in northern Australia. Ecol. Soc. 12, 1–14.
Martin-Breen, P., and Anderies, J. M. (2011). Resilience: A literature Review. New York, NY: The Rockefeller Foundation.
May, R. M. (1972). Will a large complex system be stable? Nature 238, 413–414. doi: 10.1038/238413a0
Maynard, J. A., Marshall, P. A., Johnson, J. E., and Harman, S. (2010). Building resilience into practical conservation: identifying local management responses to global climate change in the southern Great Barrier Reef. Coral Reefs 29, 381–391. doi: 10.1007/s00338-010-0603-8
Maynard, J. A., McKagan, S., Raymundo, L., Johnson, S., Ahmadia, G. N., Johnston, L., et al. (2015). Assessing relative resilience potential of coral reefs to inform management. Biol. Conserv. 192, 109–119. doi: 10.1016/j.biocon.2015.09.001
McCarthy, J. J. (2001). Climate Change 2001: Impacts, Adaptation, and Vulnerability. Contribution of Working Group II to the Third Assessment Report of the Intergovernmental Panel on Climate Change. Cambridge: Cambridge University Press.
McClanahan, T. R., Campbell, S. J., Darling, E. S., Eakin, C. M., Heron, S. F., Jupiter, S. D., et al. (2012). Prioritizing key resilience indicators to support coral reef management in a changing climate. PLoS One 7:e42884. doi: 10.1371/journal.pone.0042884
McClanahan, T. R., Graham, N. A. J., MacNeil, M. A., Muthiga, N. A., Cinner, J. E., Bruggemann, J. H., et al. (2011). Critical thresholds and tangible targets for ecosystem-based management of coral reef fisheries. Proc. Natl. Acad. Sci. U.S.A. 108, 17230–17233. doi: 10.1073/pnas.1106861108
McClanahan, T. R., Polunin, N. V. C., and Done, T. (2002). Ecological states and the resilience of coral reefs. Conserv. Ecol. 6:18.
McLeod, E., Anthony, K. R. N., Mumby, P. J., Maynard, J., Beeden, R., Graham, N. A. J., et al. (2019). The future of resilience-based management in coral reef ecosystems. J. Environ. Manag. 233, 291–301. doi: 10.1016/j.jenvman.2018.11.034
McLeod, E., Green, A., Game, E., Anthony, K., Cinner, J., Heron, S. F., et al. (2012). Integrating climate and ocean change vulnerability into conservation planning. Coast. Manag. 40, 651–672. doi: 10.1080/08920753.2012.728123
McLeod, E., Salm, R., Green, A., and Almany, J. (2009). Designing marine protected area networks to address the impacts of climate change. Front. Ecol. Environ. 7, 362–370. doi: 10.1890/070211
McManus, J. W., and Polsenberg, J. F. (2004). Coral–algal phase shifts on coral reefs: ecological and environmental aspects. Prog. Oceanogr. 60, 263–279. doi: 10.1016/j.pocean.2004.02.014
Melbourne-Thomas, J., Johnson, C. R., Aliño, P. M., Geronimo, R. C., Villanoy, C. L., and Gurney, G. G. (2011). A multi-scale biophysical model to inform regional management of coral reefs in the western Philippines and South China Sea. Environ. Modell. Softw. 26, 66–82. doi: 10.1016/j.envsoft.2010.03.033
Mumby, P. J. (1999). Bleaching and hurricane disturbances to populations of coral recruits in Belize. Mar. Ecol. Prog. Ser. 190, 27–35. doi: 10.3354/meps190027
Mumby, P. J. (2009). Phase shifts and the stability of macroalgal communities on Caribbean coral reefs. Coral Reefs 28, 761–773. doi: 10.1007/s00338-009-0506-508
Mumby, P. J., and Anthony, K. R. N. (2015). Resilience metrics to inform ecosystem management under global change with application to coral reefs. Methods Ecol. Evol. 6, 1088–1096. doi: 10.1111/2041-210x.12380
Mumby, P. J., and Steneck, R. S. (2008). Coral reef management and conservation in light of rapidly evolving ecological paradigms. Trends Ecol. Evol. 23, 555–563. doi: 10.1016/j.tree.2008.06.011
Mumby, P. J., Bejarano, S., Golbuu, Y., Steneck, R. S., Arnold, S. N., van Woesik, R., et al. (2013a). Empirical relationships among resilience indicators on Micronesian reefs. Coral Reefs 32, 213–226. doi: 10.1007/s00338-012-0966-960
Mumby, P. J., Chollett, I., Bozec, Y.-M., and Wolff, N. H. (2014a). Ecological resilience, robustness and vulnerability: how do these concepts benefit ecosystem management? Curr. Opin. Environ. Sustain. 7, 22–27. doi: 10.1016/j.cosust.2013.11.021
Mumby, P. J., Hastings, A., and Edwards, H. J. (2007). Thresholds and the resilience of Caribbean coral reefs. Nature 450, 98–101. doi: 10.1038/nature06252
Mumby, P. J., Iglesias-Prieto, R., Hooten, A. J., Sale, P. F., Hoegh-Guldberg, O., Edwards, A. J., et al. (2011). Revisiting climate thresholds and ecosystem collapse. Front. Ecol. Environ. 9, 94–96. doi: 10.1890/11.WB.002
Mumby, P. J., Sanchirico, J. N., Broad, K., Beck, M. W., Tyedmers, P., Morikawa, M., et al. (2017). Avoiding a crisis of motivation for ocean management under global environmental change. Glob. Chang. Biol. 23, 4483–4496. doi: 10.1111/gcb.13698
Mumby, P. J., Steneck, R. S., Adjeroud, M., and Arnold, S. N. (2015). High resilience masks underlying sensitivity to algal phase shifts of Pacific coral reefs. Oikos 125, 644–655. doi: 10.1111/oik.02673
Mumby, P. J., Steneck, R. S., and Hastings, A. (2013b). Evidence for and against the existence of alternate attractors on coral reefs. Oikos 122, 481–491. doi: 10.1111/j.1600-0706.2012.00262.x
Mumby, P. J., Wolff, N. H., Bozec, Y. M., Chollett, I., and Halloran, P. (2014b). Operationalizing the resilience of coral reefs in an era of climate change. Conserv. Lett. 7, 176–187. doi: 10.1111/conl.12047
Nimmo, D. G., Mac Nally, R., Cunningham, S. C., Haslem, A., and Bennett, A. F. (2015). Vive la résistance: reviving resistance for 21st century conservation. Trends Ecol. Evol. 30, 516–523. doi: 10.1016/j.tree.2015.07.008
Norström, A. V., Nyström, M., Lokrantz, J., and Folke, C. (2009). Alternative states on coral reefs: beyond coral-macroalgal phase shifts. Mar. Ecol. Prog. Ser. 376, 295–306. doi: 10.3354/meps07815
Nyström, M., Folke, C., and Moberg, F. (2000). Coral reef disturbance and resilience in a human-dominated environment. Trends Ecol. Evol. 15, 413–417. doi: 10.1016/S0169-5347(00)01948-1940
Nyström, M., Graham, N. A. J., Lokrantz, J., and Norstrom, A. V. (2008). Capturing the cornerstones of coral reef resilience: linking theory to practice. Coral Reefs 27, 795–809. doi: 10.1007/s00338-008-0426-z
Obura, D., and Grimsditch, G. (2009). Resilience Assessment Of Coral Reefs: Assessment Protocol For Coral Reefs, Focusing On Coral Bleaching And Thermal Stress. Gland: IUCN.
Ortiz, J. C., Bozec, Y.-M., Wolff, N. H., Doropoulos, C., and Mumby, P. J. (2014). Global disparity in the ecological benefits of reducing carbon emissions for coral reefs. Nat. Clim. Chang. 4, 1090–1094. doi: 10.1038/nclimate2439
Ortiz, J. C., Wolff, N. H., Anthony, K. R. N., Devlin, M., Lewis, S., and Mumby, P. J. (2018). Impaired recovery of the Great Barrier Reef under cumulative stress. Sci. Adv. 4:eaar6127. doi: 10.1126/sciadv.aar6127
Pearson, R. G. (1981). Recovery and recolonization of coral reefs. Mar. Ecol. Prog. Ser. 4, 105–122. doi: 10.3354/meps004105
Petraitis, P. S., and Dudgeon, S. R. (2004). Detection of alternative stable states in marine communities. J. Exp. Mar. Biol. Ecol. 300, 343–371. doi: 10.1016/j.jembe.2003.12.026
Petraitis, P. S., and Dudgeon, S. R. (2016). Cusps and butterflies: multiple stable states in marine systems as catastrophes. Mar. Freshw. Res. 67, 37–46. doi: 10.1071/mf14229
Quinlan, A. E., Berbés-Blázquez, M., Haider, L. J., Peterson, G. D., and Allen, C. (2016). Measuring and assessing resilience: broadening understanding through multiple disciplinary perspectives. J. Appl. Ecol. 53, 677–687. doi: 10.1111/1365-2664.12550
Renken, H., and Mumby, P. J. (2009). Modelling the dynamics of coral reef macroalgae using a Bayesian belief network approach. Ecol. Modell. 220, 1305–1314. doi: 10.1016/j.ecolmodel.2009.02.022
Roberts, C. M. (1997). Connectivity and management of Caribbean coral reefs. Science 278, 1454–1457. doi: 10.1126/science.278.5342.1454
Roff, G., and Mumby, P. J. (2012). Global disparity in the resilience of coral reefs. Trends Ecol. Evol. 27, 404–413. doi: 10.1016/j.tree.2012.04.007
Scheffer, M., Bascompte, J., Brock, W. A., Brovkin, V., Carpenter, S. R., Dakos, V., et al. (2009). Early-warning signals for critical transitions. Nature 461, 53–59. doi: 10.1038/nature08227
Scheffer, M., and Carpenter, S. R. (2003). Catastrophic regime shifts in ecosystems: linking theory to observation. Trends Ecol. Evol. 18, 648–656. doi: 10.1016/j.tree.2003.09.002
Scheffer, M., Carpenter, S. R., Dakos, V., and van Nes, E. H. (2015). Generic indicators of ecological resilience: inferring the chance of a critical transition. Ann. Rev. Ecol. Evol. Syst. 46, 145–167. doi: 10.1146/annurev-ecolsys-112414-154242
Sebastian, C. R., and McClanahan, T. R. (2013). Description and validation of production processes in the coral reef ecosystem model CAFFEE (Coral-Algae-Fish-Fisheries Ecosystem Energetics) with a fisheries closure and climatic disturbance. Ecol. Modell. 263, 326–348. doi: 10.1016/j.ecolmodel.2013.05.012
Steneck, R. S. (1994). “Is herbivore loss more damaging to reefs than hurricanes? Case studies from two Caribbean reef systems (1978–1988),” in Proceedings of the Colloquium on Global Aspects of Coral Reefs: Health, Hazards, and History, (Miami: Rosenstiel School of Marine and Atmospheric Science), 220–226.
Steneck, R. S., Mumby, P. J., MacDonald, C., Rasher, D. B., and Stoyle, G. (2018). Attenuating effects of ecosystem management on coral reefs. Sci. Adv. 4:eaao5493. doi: 10.1126/sciadv.aao5493
Tanner, J. E. (2017). Multi-decadal analysis reveals contrasting patterns of resilience and decline in coral assemblages. Coral Reefs 36, 1225–1233. doi: 10.1007/s00338-017-1614-1615
Turner, B. L., Kasperson, R. E., Matson, P. A., McCarthy, J. J., Corell, R. W., Christensen, L., et al. (2003). A framework for vulnerability analysis in sustainability science. Proc. Natl. Acad. Sci. U.S.A. 100, 8074–8079. doi: 10.1073/pnas.1231335100
van Nes, E. H., and Scheffer, M. (2007). Slow recovery from perturbations as a generic indicator of a nearby catastrophic shift. Am. Nat. 169, 738–747. doi: 10.1086/516845
van Woesik, R. (2013). Quantifying uncertainty and resilience on coral reefs using a Bayesian approach. Environ. Res. Lett. 8:044051. doi: 10.1088/1748-9326/8/4/044051
Walker, B., Carpenter, S., Anderies, J., Abel, N., Cumming, G., Janssen, M., et al. (2002). Resilience management in social-ecological systems: a working hypothesis for a participatory approach. Conserv. Ecol. 6:14.
Walker, B., Holling, C. S., Carpenter, S. R., and Kinzig, A. (2004). Resilience, adaptability and transformability in social-ecological systems. Ecol. Soc. 9:5.
Walker, B., and Salt, D. (2006). Resilience Thinking: Sustaining Ecosystems And People In A Changing World. Washington D.C: Island Press.
Walker, B. H., Ludwig, D., Holling, C. S., and Peterman, R. M. (1981). Stability of semi-arid savanna grazing systems. J. Ecol. 69, 473–498. doi: 10.2307/2259679
West, J. M., and Salm, R. V. (2003). Resistance and resilience to coral bleaching: implications for coral reef conservation and management. Conserv. Biol. 17, 956–967. doi: 10.1046/j.1523-1739.2003.02055.x
White, G. F. (1974). “Natural hazards research: concepts, methods, and policy implications,” in Natural Hazards: Local, National, Global, ed. G. F. White (New York: Oxford University Press), 3–16.
Wolff, N. H., Mumby, P. J., Devlin, M., and Anthony, K. R. N. (2018). Vulnerability of the Great Barrier Reef to climate change and local pressures. Glob. Chang. Biol. 24, 1978–1991. doi: 10.1111/gcb.14043
Wooldridge, S., and Done, T. J. (2004). Learning to predict large-scale coral bleaching from past events: a bayesian approach using remotely sensed data, in-situ data, and environmental proxies. Coral Reefs 23, 96–108. doi: 10.1007/s00338-003-0361-y
Yeung, A. C. Y., and Richardson, J. S. (2015). Some conceptual and operational considerations when measuring ‘resilience’: a response to Hodgson et al. Trends Ecol. Evol. 31, 2–3. doi: 10.1016/j.tree.2015.10.005
Keywords: management, recovery, resistance, assessment, framework
Citation: Lam VYY, Doropoulos C, Bozec Y-M and Mumby PJ (2020) Resilience Concepts and Their Application to Coral Reefs. Front. Ecol. Evol. 8:49. doi: 10.3389/fevo.2020.00049
Received: 11 August 2019; Accepted: 18 February 2020;
Published: 18 March 2020.
Edited by:
Jeanne C. Chambers, United States Department of Agriculture (USDA), United StatesReviewed by:
Jean-Luc Solandt, Independent Researcher, Ross-on-Wye, United KingdomPeter Houk, University of Guam Marine Laboratory, United States
Copyright © 2020 Lam, Doropoulos, Bozec and Mumby. This is an open-access article distributed under the terms of the Creative Commons Attribution License (CC BY). The use, distribution or reproduction in other forums is permitted, provided the original author(s) and the copyright owner(s) are credited and that the original publication in this journal is cited, in accordance with accepted academic practice. No use, distribution or reproduction is permitted which does not comply with these terms.
*Correspondence: Vivian Y. Y. Lam, dml2aWFubGFteXlAZ21haWwuY29t