- 1Division of Sustainable Development, Hamad bin Khalifa University, Doha, Qatar
- 2Office of Research and Development, United States Environmental Protection Agency, Washington, DC, United States
Trees in urban areas have a significant impact on air quality and other environmental issues. Trees can affect the concentration of air pollutants that we breathe in by directly removing pollutants or avoiding emissions and secondary pollutant formation in the atmosphere. In addition, trees have other benefits including increasing property value, intercepting storm water runoff, and saving energy needed for cooling of buildings in hot seasons. In this work, we estimate economic and environmental benefits of three tree species typical for desert regions such as Acacia tortilis, Ziziphus spina-christi, and Phoenix dactylifera. The benefits varied by species with Acacia tortilis having the highest overall benefits, mostly because of its large leaf surface area and canopy shape. Tree benefits from carbon reduction reached up to US $14 billion annually. Mature trees tended to be more beneficial than smaller trees for improving environmental conditions. The location of trees had minimal impact on the overall economic value. This assessment provides urban planners, foresters, and developers in desert regions with the information needed to make informed decisions on the economic and environmental benefits of urban tree planting.
Introduction
Air pollution in urban areas has drawn significant attention from researchers, urban planners and policy makers due to the rapid increase in industrial, transportation and construction activities leading to degraded air quality. This deterioration in air quality has been shown to adversely affect human health and the environment in urban cities (Eisenmana et al., 2019). Several mitigation measures have been proposed to improve urban air quality including green vegetation. Recently, planting trees has been explored as an abatement method for air pollution (Deng et al., 2013; Setälä et al., 2016). Vegetation in urban areas has been reported to have significant positive impact on raising property values, reducing flooding severity and improving air quality (Gallagher et al., 2015; Janhäll, 2015). Trees can improve air quality by removing particles and gases from the atmosphere (Janhäll, 2015) and converting carbon dioxide into oxygen through the process of photosynthesis (Deng et al., 2013). When located near air pollution sources, trees and bushes can also increase air dispersion, improving local air quality, although some tree characteristics can also inhibit air flow and result in air pollution increases (Abhijith et al., 2017; Baldauf, 2017). Moreover, trees provide various ecosystem services in urban environments such as the regulation of temperature by providing shading, thermal comfort and the removal of other gaseous pollutants (Logan, 1989; Amorim et al., 2013; Selmi et al., 2016). In addition, trees can directly impact particulate matter (PM) concentrations in the air by removing, emitting, or particles being re-suspended from leaf or branch surfaces (Nowak, 2000; Mell et al., 2010). Mitigation of PM in urban desert areas with high levels of dust can be of special importance due to the effects of man-made and natural PM on human health (Teather et al., 2013), visibility (United States Environmental Protection Agency, 2010) and solar energy production applications (Aissa et al., 2016; Isaifan et al., 2017; Al-Thani et al., 2018).
Greening of urban cities can support human health, provide socio-economic and environmental benefits, and bring color to an otherwise gray urban landscape. Hence, green infrastructure and human health are inter-related. In a unique review article that was published recently, Kumar et al. (2019) shed light on the nexus between green infrastructure, air quality and human health. The authors provided a holistic understanding of the links between the three aspects to facilitate decision making for policy and urban planners. The authors concluded that urban vegetation can facilitate broad health benefits, but there is little empirical evidence linking these benefits to air pollution reduction by urban vegetation. Hence, considerable efforts are needed to establish the underlying policies, design and engineering guidelines governing its deployment. Moreover, they stressed that the need for developing urban greening design guidelines is vital to promote and optimize greening benefits, and measuring green infrastructure's socio-economic and health benefits.
Understanding and quantifying the structure of the street tree resources within an urban environment is critical to evaluate their environmental benefits and for basic street tree management (Nowak et al., 2015). The most appropriate procedure to aid in street tree management is a comprehensive inventory (or census) of the street tree population which includes location, tree types and characteristics. In cases where street tree inventories are not available or cannot be conducted, Nowak et al. (2015) suggested a method for street tree sampling to provide a relatively inexpensive and straight forward procedure to obtain an overall street tree composition. The method provided tree counts with a standard error of around 10% of the total population estimate. On the other hand, Baldauf (2017) has reported on the characteristics of roadside vegetation that can result in improved local air quality. The design conditions included height, thickness, coverage, porosity/density, and species characteristics that promote improved air quality. These design specifications are of critical importance for urban planners and developers to understand how best to preserve existing roadside vegetation or plant vegetative barriers in order to reduce air pollution impacts near transportation facilities. For instance, the spacing between trees affects airflow for a longer distance. In general, studies have reported on decreased near-road pollutant concentrations with vegetation that were of minimum 5 m thickness, with most approaching 10 m or more (Neft et al., 2016).
The environmental-economic value of trees has been reported in several studies. Nowak (2000) estimated a $9.5 million economic benefit in 1994 for New York City due to the removal of an estimated 1,821 metric tons of air pollutants by trees. The study involved other cities in the United States as well, and the different rate of pollution removal by trees from one city to another was dependent on the level of air pollution, duration of in-leaf season, precipitation and other meteorological variables. This study found that large, healthy trees with trunk diameters >30 inch remove ~70 times more air pollution annually than small, healthy trees with trunk size <10 inch in diameter (Nowak, 2000).
In order to estimate the air quality, environmental benefits of green infrastructure within urban areas, the US Department of Agriculture's Forest Service (USFS) developed the i-Tree software package which provides urban and rural forestry analysis and benefits assessment tools (USDA Forest Service, 2006). Several studies have reported on the use of i-Tree to estimate tree benefits in the United States (Nowak, 1994; RIDEM Division of Forest Environment, 2014b). However, very few studies have applied this model in other regions around the world. Selmi et al. (2016) reported on the first study to apply the i-Tree Eco model to estimate air pollution removal by urban trees in Strasbourg, France. The model showed that the trees managed by the city could remove 88 ton of pollutants annually. These included the removal of 12 tons of particulate matter <10 micorns (PM10) (the coarse fraction), 5 tons of PM <2.5 microns (PM2.5), 14 tons of nitrogen dioxide (NO2), 56 tons of ozone (O3), 1 ton of carbon monoxide (CO) and 1 ton of sulfur dioxide (SO2). The study also compared actual local emissions and the simulated removal rates. The results showed that the public trees of Strasbourg removed about 7% of the emitted PM10 in the city while the impact of trees to remove other pollutants was relatively much negligible. It is worth noting that trees impact on ozone levels depends on the specific type of each species (Churkina et al., 2017). In an interesting study that compared different types of trees, Bonn et al. (2018) reported that individual tree types alter the ozone production rate and concentration as well as the secondary organic aerosol mass in different ways. The results showed that trees such as black locust, European oak and poplar intensively emitting isoprene result in higher ozone concentrations. On the other hand, tree species emitting primarily monoterpenes such as beech, magnolia and wayfaring trees yield less. Hence, the decision of planting certain tree species is essential for future urban planning including urban greening, consideration of the beneficial, and harmful aspects of tree species need to ensure that citizens benefit from and are not being negatively affected by climate adaptation strategies.
Very few studies have considered the efficiency of different urban tree species to reduce air pollution in desert cities experiencing high levels of PM concentrations due to anthropogenic and natural sources (Isaifan et al., 2018). In general, the ability of trees to improve air quality varies because of different plant characteristics. The effect of leaf surface structure, for instance, has been shown to have a major influence on PM2.5 capture (Pugh et al., 2012; Chen et al., 2017).
Research on the suitability of different tree species to be implemented to reduce air pollution in desert regions of the world, such as the Middle East, has been limited. This region has different plant species and characteristics that require investigation to understand air quality and economic benefits of planting.
Among countries in the Arabian Peninsula, the State of Qatar can experience severe air pollution episodes in major cities (Lanouar and Al-malk, 2016). Qatar also has arid conditions and is characterized by poor soil quality and stony ground that makes up 88% of the land. Nevertheless, Qatar has a plant variety often considered rather rich in quantity and diversity (Abdel Bari, 2005). As a result, planting trees in Qatar cities can provide economic and environmental benefits for air quality improvement and other ecosystem services.
Due to the nature of the harsh climatic conditions, with high temperatures and scarce rains, the dominant plant species consist of annuals, ephemerals, and short-lived perennials, as commonly found in arid zones. These plants make up over 60% of the total flora whereas perennials represented by trees, lianes, shrubs, under shrubs, and perennial herbs are about 40% of the total number of taxa recorded (Abdel Bari, 2005). More specifically, there are seven tree species in the State of Qatar (Abdel Bari, 2005), which includes: the mangroves (a) Avicennia marina, (b) Acacia ehrenbergiana, (c) Acacia tortilis, (d) Ziziphus nummularia, (e) Ziziphus spina-christi; the lianes (f) Cocculus pendulus, and (g) Ephedra foliata (a gymnosperm) (Al Amin, 1983; Abdel Bari, 2005). In addition to the above seven naturalized species, Phoenix dactylifera grows in the Arabian Peninsula.
In this paper, the economic and air quality environmental benefits were evaluated of the three most abundant urban tree species in Qatar: Acacia tortilis (Wattles), Ziziphus spina-christi (Sidra) and Phoenix dactylifera (Palm Date) (Isaifan et al., 2018). Other trees which grow in Qatar were not evaluated in this study since these species are not currently available in the i-Tree software package. The economic value of each tree was estimated based on its capability to intercept urban storm water runoff, adding property value, reducing electricity by enhanced cooling, minimizing air pollution by removing pollutants or avoiding the formation of secondary pollutants, and carbon dioxide capture and sequestration.
Methodology
To evaluate the environmental-economic benefits of the three abundant urban trees, the National Tree Benefit Calculator (NTBC; Casey Tree and Davey Tree Expert Co.), derived from the i-Tree software suite, was used in a case study for Doha, Qatar. The NTBC is a free tool for public use which estimates the economic benefits of trees due to environmental improvements. The NTBC allows the user to calculate a first-order approximation of the benefits of individual trees. This tool uses the i-Tree street-tree assessment tool, STREETS, for these calculations (RIDEM Division of Forest Environment, 2014a). Although this software can be of great benefit for a general overview on the environmental and non-environmental benefits of trees, it has some limitations. First, the model evaluates the direct removal of PM and gaseous pollutants through dry deposition, leaf stomata uptake of gaseous pollutant and leaf interception of PM. Nevertheless, diffusion of very small particles is not accounted for in this model but still occurs and could be of a very big benefit since many health studies suggest it is those ultrafine particles causing the adverse health impacts. Another limitation is that this model suggests that location of planting trees has minimal impact on the overall economic value. Hence, it does not consider specific location in its assessment since it only evaluates regional effects. Many studies suggest that PM removal is higher when the plants are located near the polluting source. In addition, some other effects may be more localized, so location may matter when considering a specific city, but the model cannot account for that either.
The inputs required for the assessment include: the location of the area, the tree species name, a tree diameter value that ranges between 0 and 45 inches, and the type of land use where the tree is or will be planted. The tool provides an output of an annual estimate of the environmental and economic value provided by that tree. Thus, the tool provides an initial summary of the tree's value in a chosen urban or rural area. For the current version of NTBC, a specific location in the United States must be selected to represent the area of interest since this tool was developed primarily for use in this country. The Southern California desert was chosen since this region has the same climate zone as Qatar (Figure 1) based on the six main groups of world climates: Arid, Polar, Temperate, Tropical, Mediterranean and Mountains (Roy et al., 2012).
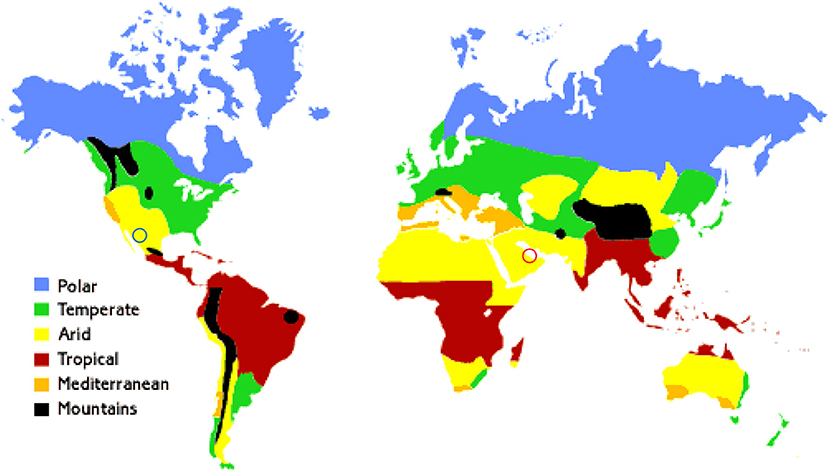
Figure 1. World Climate Zones showing the (red circle) State of Qatar having the same climate zone as the (blue circle) desert in Southern California (Internet GeographyWorld Climate Zone, 2015).
After selecting the location, the tree species being evaluated are input into the tool. Table 1 shows the eight tree species and their growth locations within Qatar. For this study, the three tree species that grow most abundantly in the urban environment of Doha were investigated: Acacia tortilis (Wattles), Ziziphus spina-christi (Sidra) and Phoenix dactylifera (Palm Date) (Norton et al., 2009).
Since no urban tree inventories are available for Doha, assumptions were made on the sizes of the trees. For this study, a trunk of 10 inch-diameter (~25 cm) represented the average of all young tree species, while 45 in. trunk diameter (~114 cm) represented the average of all mature grown trees. For the type of land use, the NTBC tool has the following categories: single family residential, multi-family residential, small commercial business, industrial or large commercial business, and park or other vacant land. For this analysis, the environmental benefits were calculated for three different land use types based on typical development in Doha: (1) single family residential, (2) industrial or large commercial business, and (3) park or other vacant land.
Results and Discussion
The economic-environmental benefits of the trees in Doha, Qatar were compared in two categories: air quality-environmental and non-air quality-environmental benefits. Air quality-environmental benefits involve trees ability to reduce concentrations of primary pollutants in the air and capture CO2. Other environmental benefits include the ability of trees to add value to property, saving energy due to shading and reduced urban heat island effects, and the interception of storm-water runoff. In each category, the trees were compared by the species type and age (young vs. mature tree) and the three location types where trees are planted (residential, industrial, and park or open space).
Air-Quality Environmental Benefits
Primary Air Pollution Reduction
Trees can reduce the concentrations of air pollutants by avoiding emissions from energy sources and limiting secondary pollutant formation due to cooling effects, as well as directly removing pollutants from the air on to leaf and branch surfaces.
Avoided air pollution
Figure 2 shows the environmental economic value of the avoided portion of air pollution by the presence of the three urban trees in a desert area. The term “avoided” refers to the trees ability to reduce the need for energy production that will emit air pollution. Energy production can be reduced when trees provide shading and thermal comfort that limits the need for air conditioning. For example, temperatures were estimated to be reduced over an area of about 120 acres near a park of ~500 acres in a city near Tokyo, which led to savings in air conditioning electricity. The monetary value of this energy saving was estimated to be US$650 per hour between 1 and 2 p.m. (Ca et al., 1998). Thus, trees can enhance energy conservation in surrounding locations which cause reductions in greenhouse gas and primary pollutant emissions from fossil fuel burning power plants, which includes PM, SO2, NO2, and CO2. The latest available data on air emissions in Qatar were reported by the World Bank (Economics Trading Qatar-CO2 Emissions, 2019). The CO2 emissions in Qatar were 107,854 kt in 2014 stemming from the burning of fossil fuels and the manufacture of cement. They also include CO2 produced during consumption of solid, liquid, and gas fuels and gas flaring, according to the World Bank collection of development indicators, compiled from officially recognized sources (Economics Trading Qatar-CO2 Emissions, 2019).
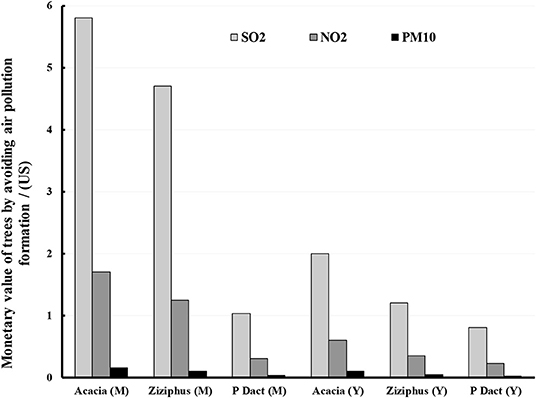
Figure 2. The monetary value of trees by avoiding air pollution formation. “M” indicates mature trees with trunk diameter of 45 in. or greater, “Y” indicates young tress with trunk size of <10 in, “P Dact” indicates Phoenix Dactylifera.
Trees can also reduce air pollution by changing the microclimate and avoiding the formation of secondary pollutants. The extent of the reduction in air pollution formation depends on the amount of vegetation and meteorological conditions in a specific region. Nowak et al. reported that air quality improvement by trees in cities during daytime of the in-leaf season averages around 0.51% for PM, 0.44% for SO2, and 0.33% for NO2 (Nowak and Heisler, 2010). In addition, Ottosen and Kumar (2019) have reported on the first long time-series measurement of multiple pollutants to assess the mitigation of traffic pollution when trees are planted along road side. This time-series took into consideration the influence of the annual vegetation cycle, wind direction and high vs. low concentrations of both gaseous and particulate matter pollutants across a hedge. The results showed a concentration difference of −52% for PM1, −44% for PM2.5, and −35% for PM10 at the beginning of the maturity phase.
Trees can also increase emissions of certain chemicals, notably volatile organic compounds (VOC) which can contribute to the formation of O3 and secondary organic particles. As shown in Figure 2, the trees evaluated in this study do not contribute to the air quality environmental value related to VOC. The actual value is around 0.001 US $ which is negligible as shown in the figure. In addition to VOC emission rates varying by species, emissions also depend on air temperature (Owen et al., 2003). However, since trees generally lower air temperature through shading, increased tree cover can lower the overall VOC emissions from surrounding trees, reducing O3 and secondary particle formation in urban areas.
The results show that mature trees are more efficient than young trees for air quality-environmental benefits by avoiding air pollution emissions and secondary formations. The increase in tree age provides a larger canopy and bigger Leaf Surface Area (LSA). Nowak (1994) reported that large, healthy trees (diameter >30 in) have ~60–70 times more ability to reduce air pollution emissions annually than small healthy trees (diameter <10 in) (Nowak, 1994; USDA Forest Service, 2006; RIDEM Division of Forest Environment, 2014b; Nowak et al., 2015). This also applies to trees ability to avoid secondary pollution formation since larger tree canopies result in lower air temperatures due to increased shading. In addition to shading, trees have a cooling effect on the surrounding environment since leaves transpire large amounts of moisture (Jensen and Kozlowski, 1975; United States Department of Agriculture, 1977; Al Amin, 1983; Owen et al., 2003).
Direct removal of air pollution
Air pollutants can be directly removed from the air as contaminants deposit or absorb onto tree surfaces. Leaves usually act as the main receptors of pollutants, trapping PM on the spines or hairs of the leaf surfaces. In other instances, particles adhere to the waxy surface or onto a moisture film. The size and number of pores or stomata on tree surfaces can be another important factor in pollutant removal. In addition, the electrical charge on the leaf may also attract particles with the opposite charge (United States Department of Agriculture, 1977).
Figure 3 shows that mature trees are more efficient at reducing air pollution by deposition and absorption compared to young trees. Dry deposition occurs when the vegetation removes atmospheric pollutants during non-precipitation periods (Cavanagh, 2005). This process depends on several parameters such as the type of pollutant (particulate or gaseous) and volatility. For PM, the density, morphology and size are important parameters. Deposition also depends on the properties and characteristics of the plant surfaces such as the chemical composition, roughness and size as discussed previously. Deposition of pollutants can be affected by the overall pattern of leaves which includes the arrangement, number, density, and proximity to each other. For example, thick canopies, like those found in forests, are more efficient at reducing air pollution than individual or largely-spaced trees provided sufficient air flow occurs. In addition to leaves, particles and gases in the air can be deposited on tree trunks and branches. Trees with several branches forming a large, complex frame would be more effective at removing air pollution than trees with few branches. Likewise, a large trunk with rough-surfaced bark can trap more PM than a small or smooth bark trunk (United States Department of Agriculture, 1977). Dry deposition can also be affected by weather variables including temperature, solar radiation, wind speed, and direction (Styszko et al., 2018).
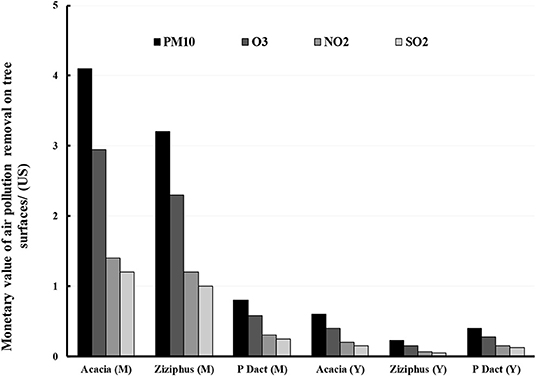
Figure 3. The monetary value of air pollution removal on tree surfaces. “M” indicates mature trees with trunk diameter of 45 in. or greater, “Y” indicates young tress with trunk size of <10 in, “P Dact” indicates Phoenix Dactylifera.
Pollutants may be removed and accumulate onto leaf surfaces in two ways: through leaf stomata uptake of gaseous pollutants and leaf interception of PM (Nowak et al., 2006). The first process involves gaseous pollutants and small, ultrafine particles of diameters <100 nm that diffuse into the stomata. This action leads to the capture and adsorption of these pollutants into the inner part of leaves, penetrating the leaf boundary layer. Gaseous pollutants can also be removed via dry deposition by reacting with chemicals on the plant surfaces. The removal of larger diameter PM via dry deposition can occur when leaf surfaces intercept these particles, especially enhanced by the presence of fine hairs, surface roughness, increased leaf area, or stickiness. Larger particles can penetrate the leaf boundary layer by impaction, when airflow bends around the leaf but the particles continue in a straight line to impact the surface, or sedimentation, where the particles settle onto the leaf surface by gravity. Sedimentation depends on the particle diameter and density, occurring when the particles are typically larger than 5 μm in diameter. The removal of PM through sedimentation may be offset by the re-suspension of the settled particles from the leaf surfaces during high wind speeds.
For effective environmental benefits in urban areas, tree species must be tolerant of the high air pollution levels often found at these locations. Acacia tortilis has been reported to have a higher air pollution tolerance index (APTI) than Ziziphus spina-christi and Phoenix dactylifera. Trees vary in their tolerance and reaction to air pollution by the APTI as mentioned earlier. In addition, the impact of trees on air pollution reduction depends on various species characteristics such as size when mature, estimated water use or evaporation and leaf surface characteristics. In experiments with big tooth aspen, plants of the same size were grown under identical conditions and fumigated regularly with SO2 (Jensen and Kozlowski, 1975). Some of the plants in the experiment grew vigorously which indicates that these plants can thrive when exposed to this contaminant. Others became stunted and sickly although they belonged to the same species. This difference in interaction with the environment and air pollution levels depends on the genetic makeup of the individual tree which consequently accounts for the difference in growth (Tchounwou et al., 2012). The presence or absence of certain chromosomes makes some trees more tolerant to air pollution. Finding trees with this genetic tolerance is basic to the breeding and selection of pollution-tolerant trees since they function for a longer time (United States Department of Agriculture, 1977; Tchounwou et al., 2012). The APTI was estimated to be 30, 13.2 and 11.5 for Acacia tortilis, Ziziphus spina-christi, and Phoenix dactylifera, respectively (Das and Prasad, 2010; Salih et al., 2017). The higher the APTI, the more tolerant the tree species is to air pollution. Acacia tortilis is also more tolerant to high alkalinity, drought, high temperatures and sandy and stony soil (Bui et al., 2014). While deciduous trees may appear to provide greater air quality benefits than conifers due to the larger leaves, most conifers keep their greenery all year and actually may have more leaf surface area and longer life (United States Department of Agriculture, 1977).
Carbon Sequestration
Trees sequester CO2 in their roots, trunks, and leaves while growing, and in wood products after being harvested. In addition, trees can lower CO2 concentrations (by avoiding the need to emit CO2) by reducing heating and air conditioning demands, reducing emissions associated with power production as discussed previously. The latter factor is critical in cities due to the urban heat island effect where metropolitan areas have higher air temperatures than surrounding areas due to excessive human activities and heat-absorbing surfaces. On the other hand, trees can affect the levels of CO2 by directly emitting it through decomposition.
The results shown in Figure 4 indicate that mature trees can reduce more CO2 than young ones. The figure displays two types of CO2. The dark legend corresponds to the avoidable part which is due to the cooling effect of trees which indirectly reduces the emissions from power plants needed for air conditioning. The light legend part corresponds to CO2 sequestered/up taken and stored in trees. Large trees (stem diameter >30 in) store ~800–900 times more carbon than small trees (<10 in), In addition, large, healthy trees remove about 50 times more carbon annually than small, healthy trees. Carbon storage by urban forests is estimated at about 919 million tons annually, which equal about $119 billion in economic benefits in the United States. The annual gross carbon sequestration by urban forests is estimated at 36.7 million tons with an estimated value of US $4.8 billion (Nowak and Greenfield, 2018). Similarly, based on the amount of CO2 emitted due to fossil fuel burning in Qatar for 2014 (107,854 kt) (Economics Trading Qatar-CO2 Emissions, 2019), trees can sequester carbon with the value of US $14.1 billion. In terms of tree species, Acacia tortilis is more efficient for the reduction in CO2 than Ziziphus spina-christi and Phoenix dactylifera which is related to its ability to abate air pollution and its higher APTI as mentioned previously.
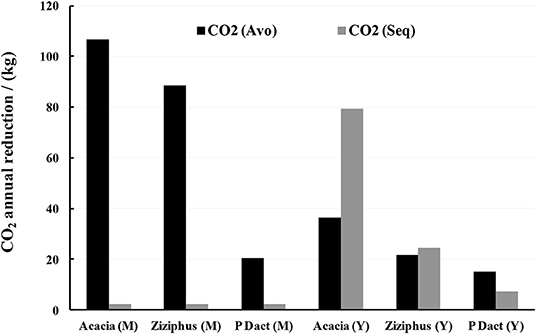
Figure 4. Annual CO2 reduction by trees in urban desert areas. “M” indicates mature trees with trunk diameter of 45 in. or greater, “Y” indicates young tress with trunk size of <10 in, “P Dact” indicates Phoenix Dactylifera.
Although trees ability to capture CO2 from the atmosphere is unquestionable, Tang and Chen (2016) investigated to what extent anthropogenic CO2 emissions generated from cities can be offset through conserving or increasing carbon (C) stored within urban areas in Beijing, China. They found out that although carbon sequestration of street trees was 3.1 ± 1.8 Gg C yr−1 in 2014, it was only equivalent to about 0.2% of its annual CO2-equivalent emissions from total energy consumption, indicating a rather limited role in offsetting overall anthropogenic CO2 emissions as per the study.
Non-air Quality Environmental Benefits
Although this paper primarily focuses on the air quality benefits of urban trees, consideration of other environmental benefits must be examined as part of a holistic evaluation of the utility of urban trees. The following sections provide a brief overview of these other, non-air quality benefits.
Property Values
As discussed, trees can have a positive impact on property values. Table 2 shows a comparison of the non-air quality environmental benefits related to property value of young vs. mature Acacia tortilis, Ziziphus spina-christi, and Phoenix dactylifera trees when planted in residential, industrial and park areas.
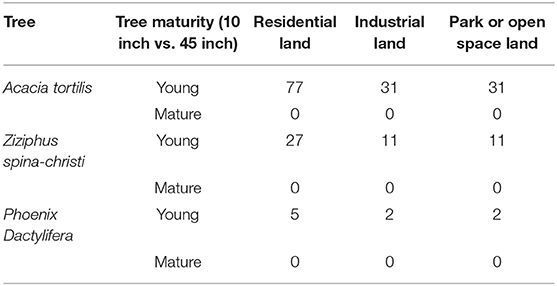
Table 2. The annual increase in property value (US$) of young vs. mature urban trees when planted in different locations.
The results show that the value of tree species depends on whether the tree planting occurs in residential areas or in industrial and park locations. Trees planted in the vicinity of single family homes have a greater property value benefit than those planted within multi-family homes, parks or commercial property locations (Threlfall et al., 2016). Real estate agents state that trees tend to increase property sale prices due to their appearance or “curb appeal,” with home buyers typically paying more for properties with ample vs. few or no trees (Anderson and Cordell, 1988; Wolf, 2007; Isaifan et al., 2018; Casey Trees and Davey Tree Expert Co, 2019). In addition, industrial and commercial land use might experience some additional property value especially where air pollution removal credits can be obtained to provide ownership or “rental” rights to the removed pollutants and a company may sell, buy or apply those credits to offset their own emissions (Casey Trees and Davey Tree Expert Co, 2019). While these values have been determined based on land use in the United States, the benefits of trees planted in similar locations is generally consistent in Qatar and other parts of the world.
The results show as well that mature trees have no additional property value since they have reached the limit of their growth life, while the young trees add an incremental annual value as their impact increase as they grow. To estimate this value, the NTBC model uses the tree's LSA to determine the increases in property values. The tool estimates that a property with more trees (and more LSA) tends to have a higher value than one with fewer trees (and lower LSA). The results shown in Table 2 are annual and accumulate incrementally over time for smaller trees because these trees typically add more LSA each growing season. The 10-inch Acacia tortilis, for instance, will add 163 square feet (around 15 m2) of LSA the year after planting. In subsequent years, this tree will add more LSA, and the property value will increase accordingly. Alternatively, the mature 45-inch Acacia tortilis selected will add 0 square feet of LSA the year after planting, as well as in subsequent years, since it has reached its life growth limit. In terms of tree species, Acacia tortilis has a significantly higher annual increase in property value compared with Ziziphus spina-christi and Phoenix dactylifera since the shape of the Acacia tortilis canopy provides higher LSA than Ziziphus spina-christi and Phoenix dactylifera.
Storm Water Runoff
Reductions of storm water runoff are another benefit provided by urban trees. Table 3 shows the annual volume of runoff water intercepted by the tree species evaluated in this study.
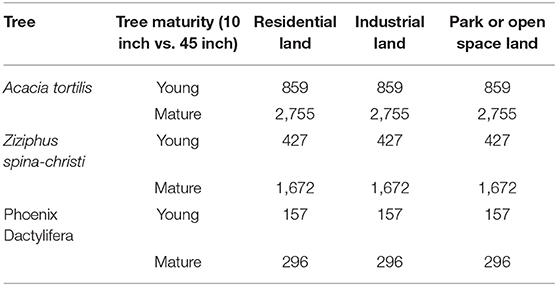
Table 3. Gallons of storm water runoff intercepted annually by young vs. mature urban trees when planted in different locations.
Urban storm water runoff generally washes litter and chemicals from roadways and parking lots into streams, rivers and oceans. The more impermeable the surfaces, such as asphalt, concrete, and building rooftops, the faster pollutants can be washed into the community surface waterways. Polluted water runoff can negatively affect aquatic life and drinking water which consequently impact the health of the entire ecosystem. Therefore, planting and growing more trees in urban areas can help control runoff at the source as trees act as mini-reservoirs (Penn State Extension, 2002; Casey Trees and Davey Tree Expert Co, 2019). More specifically, trees hold and intercept rain on their leaves, bark, and branches. In addition, they increase infiltration and storage of rainwater through their root system and by reducing soil erosion since they tend to slow rainfall before it strikes the soil (Zuazo and Rodriguez, 2008; Casey Trees and Davey Tree Expert Co, 2019).
The results in Table 3 suggest no impact of the type of land use on the ability of each tree species to intercept storm water runoff. Acacia tortilis showed a higher capacity to intercept storm water compared with Ziziphus spina-christi and Phoenix dactylifera. The mature trees have much higher benefits than young trees as the size of the mature tree provides more surface area for rain storage and a large root system for uptake.
Urban Heat Island Cooling
The last role of trees in the non-air quality environmental benefits evaluated was the tree's indirect effect on reducing energy consumption through cooling and reducing the urban heat island (UHI) effect, shown in Table 4.
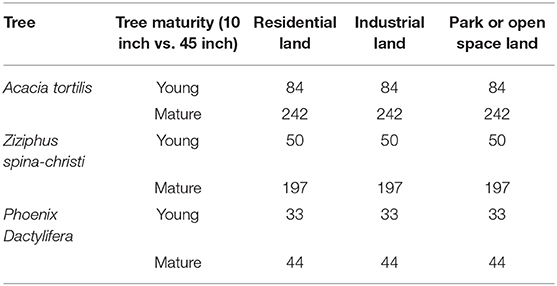
Table 4. Annual energy saving kWh of cooling and reduced consumption of oil and natural gas by young vs. mature urban trees when planted in different locations.
The results show two main trends in terms of age and species type. First, mature trees have higher benefit and value than smaller trees when considering energy consumption. In addition, Acacia tortilis has greater benefits in terms of annual energy savings than Ziziphus spina-christi and Phoenix dactylifera. The thermal comfort and cooling effect is largely dependent on the size of the tree and its canopy. Acacia tortilis has significantly larger canopy surface than Ziziphus spina-christi and Phoenix dactylifera and hence it induces higher energy savings, with less need for air conditioning use. The impact of type of land use is insignificant for this benefit. For simplicity, the software assumes that location differences are negligible and the energy saved is the same in all locations per species type and age.
UHI impact in urban cities can be divided into two categories: (i) the effects on people and (ii) the effects on the microclimate (US Environmental Protection Agency, 2008). An example of the impact of UHI on people is observed by the damage to the thermoregulatory system manifested in the form of cardiovascular problems and induced by heat stress. This in-turn causes the deterioration of physical well-being within a city (Hassid et al., 2000; Hayhoe et al., 2010). Hence, in order to counter balance these negative impacts, dwellers tend to seek comfort indoor by the use of air conditioning which exacerbate the outdoor microclimatic conditions (Smith and Levermore, 2008; Shahmohamadi et al., 2011). This excessive dependence on cooling needs not only increase particulate emissions from fuel burning, but also elevates the formation of ozone particles at the ground level, leading to further alteration of the local microclimate and macroclimate. Examples of these alterations are wind pattern modifications, changes in humidity, the increase in precipitation and flooding, changes in local ecosystems and finally, the increase in global warming due to the increasing demand for cooling energy (Behringer, 2009).
Nevertheless, the nighttime effect of UHIs can be critically harmful especially during a heat wave (HW), since it deprives residents in urban areas of the cool relief found in rural areas during the night. A recent study showed that there are synergistic reactions between UHIs and HWs which have greater and stronger impact in cities with more pronounced effect during nighttime (Founda and Santamouris, 2017). This has been attributed to lower pressure gradients during nighttime which resulted in the deceleration of synoptic winds and prevalence of low winds, particularly during HW periods. It was also reported that low wind speeds imply that advection mechanisms are not contributory to UHI intensity during nighttime and radiative processes mainly control the magnitude of the nocturnal UHI.
Conclusion
The environmental-economic values of three tree species (Acacia tortilis, Ziziphus spina-christi and Phoenix dactylifera) in desert areas were estimated using the NTBC. The environmental-economic value of each tree was estimated based on its capability to avoid air pollution by reducing secondary pollution formation, minimizing air pollution by deposition, CO2 capture and sequestration, adding property value, intercepting urban storm water runoff, and reducing electricity use by promoting urban cooling.
Mature trees with diameters of 45 in. were compared with young trees with diameters of 10 in. Mature tree species (1) are more efficient at reducing air pollution, (2) have higher capacity to intercept storm water runoff, and (3) can reduce energy consumption compared to young trees. Acacia tortilis was found to be the most efficient tree species for the above three environmental benefits as well as reducing air pollution levels through dry deposition, avoiding further pollution formation and CO2 removal. The primary benefit of younger trees is increased property values over time. Since younger trees tend to have lower initial costs and will grow into mature trees over time, the benefits of younger trees can still be substantial, particular if the needs for the environmental benefits are long term and not immediate.
Several limitations of using this method has been outlined which are mainly related to the assumption of location due to the absence of any data or tools developed for the local region. In addition, the NTBC model assumes that the environmental benefits are attained through the direct removal of PM and gaseous pollutants via dry deposition, leaf stomata uptake of gaseous pollutant and leaf interception of PM. Nevertheless, diffusion of very small particles is not accounted for in this model but still occurs. Moreover, this model suggests that location of planting trees has minimal impact on the overall economic value. Hence, it does not consider specific location in its assessment since it only evaluates regional effects, contrary to previous research suggesting that PM removal is higher when the plants are located near the polluting source.
Data Availability Statement
The datasets generated for this study are available on request to the corresponding author.
Author Contributions
All authors listed have made a substantial, direct and intellectual contribution to the work, and approved it for publication.
Funding
The publication of this article was funded by the Qatar National Library.
Disclaimer
This document has been reviewed in accordance with the US Environmental Protection Agency policy and approved for publication. Mention of trade names or commercial products does not constitute endorsement or recommendation for use. The views expressed in this journal article are those of the authors and do not necessarily reflect the views or policies or the US Environmental Protection Agency.
Conflict of Interest
The authors declare that the research was conducted in the absence of any commercial or financial relationships that could be construed as a potential conflict of interest.
The reviewer PK declared a past co-authorship with one of the authors, RB to the handling editor.
References
Abdel Bari, E. (2005). The flora of the state of Qatar: its history and present-day status. Qatar. Univ. Sci. J. 25, 113–118. doi: 10.5339/qmj.2005.2.25
Abdel Bary, E. (2013). Plant Life in Qatar. Doha: Environmental Studies Center, Qatar University, 1–44.
Aissa, B., Isaifan, R. J., Abdulla, A., and Madhavan, V. (2016). Structural and physical properties of the desert-dust particles and their influence on the PV panels performance in Qatar. Sci. Rep. 6:31467. doi: 10.1038/srep31467
Al Amin, H. (1983). Wild Plants of Qatar Fo Arab Organization for Agricultural Development. Richmond: Kingprint Limited.
Al-Thani, H., Koc, M., and Isaifan, R. J. (2018). Investigations on deposited dust fallout in urban doha: characterization, source apportionment and mitigation. Environ. Ecol. Res. 5, 493–506. doi: 10.13189/eer.2018.060510
Amorim, J. H., Valente, J., Cascão, P., Rodrigues, V., Pimentel, C., Miranda, A. I., et al. (2013). Pedestrian exposure to air pollution in cities: modeling the effect of roadside trees. Adv. Meteorol. 2013:e964904. doi: 10.1155/2013/964904
Anderson, L. M., and Cordell, H. K. (1988). Influence of trees on residential property values in athens, Georgia (USA): a survey based on actual sales prices. Landscape 15, 153–164. doi: 10.1016/0169-2046(88)90023-0
Baldauf, R. (2017). Roadside vegetation design characteristics that can improve local, near-road air quality. Transp. Res. Part D Transp. Environ. 52, 354–361. doi: 10.1016/j.trd.2017.03.013
Bonn, B., von Schneidemesser, E., Butler, T., Churkina, G., Ehlers, C., Grote, R., et al. (2018). Impact of vegetative emissions on urban ozone and biogenic secondary organic aerosol: box model study for Berlin, Germany. J. Clean. Prod. 176, 827–841. doi: 10.1016/j.jclepro.2017.12.164
Bui, E., Thornhill, A., and Miller, J. (2014). Salt and alkaline tolerance are lin ked in Acacia. Biol. Lett. 10, 1–4. doi: 10.1098/rsbl.2014.0278
Ca, V., Asaeda, T., and Abu, E. (1998). Reductions in air conditioning energy caused by nearby park. Energy Build. 29, 83–92. doi: 10.1016/S0378-7788(98)00032-2
Casey Trees Davey Tree Expert Co (2019). National Tree Benefit Calculator-Property Value. Available online at: http://www.treebenefits.com/calculator/
Cavanagh, J. (2005). Potential of Vegetation to Mitigate Road-Generated Air Pollution. Lincoln: Landcare Research.
Chen, L., Liu, C., Zhang, L., Zou, R., and Zhang, Z. (2017). Variation in tree species ability to capture and retain airborne fine particulate matter (PM2.5). Sci. Rep. 7:13206. doi: 10.1038/s41598-017-03360-1
Churkina, G., Kuik, F., Bonn, B., Lauer, A., diger Grote, R., and Tomiak, K. (2017). Effect of VOC emissions from vegetation on air quality in Berlin during a heatwave. Environ. Sci. Technol. 51, 6120–6130. doi: 10.1021/acs.est.6b06514
Das, S., and Prasad, P. (2010). Seasonal variation in air pollution tolerance indices and selection of plant species for industrial areas of rourkela. Ind. J. Environ. Protect. 30, 978–988.
Deng, X. Z., Zhao, C. H., and Yan, H. M. (2013). Systematic modeling of impacts of land use and land cover changes on regional climate: a review. Adv. Meteorol. 317678, 1–12. doi: 10.1155/2013/317678
Economics Trading Qatar-CO2 Emissions (2019). Economics Trading Qatar-CO2 Emissions. Available online at: https://tradingeconomics.com/qatar/co2-emissions-kt-wb-data.html (accessed October 01, 2019).
Eisenmana, T. S., Churkina, G., Jariwala, S. P., Kumar, P., Lovasi, G. S., Pataki, D. E., et al. (2019). Urban trees, air quality and asthma: an interdisciplaniry review. Landsc. Urban Plan. 187, 47–59. doi: 10.1016/j.landurbplan.2019.02.010
Founda, D., and Santamouris, M. (2017). Synergies between urban heat island and heat waves in Athens (Greece), during an extremely hot summer (2012). Sci. Rep. 7:10973. doi: 10.1038/s41598-017-11407-6
Gallagher, J., Baldauf, R., Fuller, C. H., Kumar, P., Gill, L. W., and Mcnabola, A. (2015). Passive methods for improving air quality in the built environment: a review of porous and solid barriers. Atmos. Environ. 120, 61–70. doi: 10.1016/j.atmosenv.2015.08.075
Hassid, S., Santamouris, M., Papanikolaou, N., Linardi, A., Klitsikas, N., Georgakis, C., et al. (2000). The effect of the Athens heat island on air conditioning load. Energy Build. 32, 131–141. doi: 10.1016/S0378-7788(99)00045-6
Hayhoe, K., Sheridan, S., Kalkstein, L., and Greene, S. (2010). Climate change, heat waves, and mortality projections for Chicago. J. Gt. Lakes Resour. 36, 65–73. doi: 10.1016/j.jglr.2009.12.009
Internet GeographyWorld Climate Zone (2015). Internet GeographyWorld Climate Zone. Available online at: http://www.geography.learnontheinternet.co.uk/topics/climatezones.html (accessed October 01, 2019).
Isaifan, R. J., Al-Thani, H., Ayoub, M., Aissa, B., and Koc, M. (2018). The economic value of common urban trees in the State of Qatar from an air quality control perspective. J. Environ. Sci. Pollut. Res. 4, 285–288. doi: 10.30799/jespr.137.18040301
Isaifan, R. J., Samara, A., Suwaileh, W., Johnson, D., Yiming, W., Abdallah, A. A., et al. (2017). Improved self-cleaning properties of an efficient and easy to scale up TiO2 thin films prepared by adsorptive self-assembly. Sci. Rep. 7:19466. doi: 10.1038/s41598-017-07826-0
Janhäll, S. (2015). Review on urban vegetation and particle air pollution - deposition and dispersion. Atmos. Environ. 105, 130–137. doi: 10.1016/j.atmosenv.2015.01.052
Jensen, K., and Kozlowski, T. (1975). Absorption and translocation of sulfure dioxide by seedlings of four forest tree species. J. Environ. Air Qual. 4, 379–382. doi: 10.2134/jeq1975.00472425000400030021x
Kumar, L. M. P., Druckman, A., Gallagher, J., Gatersleben, B., Allison, S., Eisenman, T., et al. (2019). The nexus between air pollution, green infrastructure and human health. Environ. Int. 133:105181. doi: 10.1016/j.envint.2019.105181
Lanouar, C., and Al-malk, A. Y. (2016). Air Pollution in Qatar: Causes and Challenges Air Pollution in Qatar: Causes and Challenges. Doha: WP/CBE.
Logan, J. (1989). Ozone in rural areas of the United States. J. Geophys. Res. 94, 8511–8532. doi: 10.1029/JD094iD06p08511
Mell, W., Manzello, S., Maranghides, A., Buty, D., and Rehm, R. (2010). The wildland urban interface fire problem- current approaches and research needs. Int. J. Urban Reg. Res. 19, 238–251. doi: 10.1071/WF07131
Neft, S. I., Scungio, M., Culver, N., and Singh, S. (2016). Simulations of aerosol filtration by vegetation: validation of existing models with available lab, Aerosol. Sci. Technol. 50, 937–946. doi: 10.1080/02786826.2016.1206653
Norton, J., Abdul Majid, S., Allan, D., Al Safran, M., Böer, B., and Richer, R. (2009). An Illustrated Checklist of the Flora of Qatar. Doha: UNESCO Office in Doha.
Nowak, D., and Greenfield, E. (2018). U.S. urban forest statistics, values and projections. J. For. 116, 164–177. doi: 10.1093/jofore/fvx004
Nowak, D. J. (1994). “Atmospheric carbon dioxide reduction by Chicago's urban forest,” in Chicago's Urban Forest Ecosystem: Results of the Chicago Urban Forest Climate Project, General Technical Report NE-186, eds E. G. McPherson, D. J. Nowak, and R. A. Rowntree (Chicago, IL: US Department of Agriculture, Forest Service), 83–94.
Nowak, D. J. (2000). The Effects of Urban Trees on Air Quality. Syracuse, NY: USDA Forest Service, 1–4.
Nowak, D. J., Crane, D. E., and Stevens, J. C. (2006). Air pollution removal by urban trees and shrubs in the United States. Urban For. Urban Green. 4, 115–123. doi: 10.1016/j.ufug.2006.01.007
Nowak, D. J., and Heisler, G. M. (2010). Improving Air Quality with Trees and Parks. Ashburn, VA; Saint Paul, MN: National Recreation and Parks Association, Research Series Monograph 44.
Nowak, J. B. D., Walton, J., and Baldwin, J. (2015). Simple street tree sampling. Arboric. Urban For. 41, 346–354. Available online at: https://www.nrs.fs.fed.us/pubs/52905
Ottosen, T., and Kumar, P. (2019). The influence of the vegetation cycle on the mitigation of air pollution by a deciduous roadside hedge. Sustain. Cities Soc. 53:101919. doi: 10.1016/j.scs.2019.101919
Owen, S., Mackenzie, A., Stewart, H., Donovan, R., and Hewitt, C. (2003). Biogenic volatile organic compound (VOC) emission estimates from an urban tree caopy. Ecol. Appl. 13, 927–938. doi: 10.1890/01-5177
Penn State Extension (2002). The Role of Trees & Forests in Healthy Watersheds. North Central Philadelphia, PA: Penn State Extension.
Pugh, M., Mackenzie, A. R., Whyatt, J. D., and Hewitt, C. N. (2012). Effectiveness of green infrastructure for improvement of air quality in urban street canyons. Environ. Sci. Technol. Lett. 46, 7692–7699. doi: 10.1021/es300826w
Qatar Natural History Group Flora of Qatar (2010). Qatar Natural History Group Flora of Qatar. Available online at: http://www.floraofqatar.com/ (accessed October 01, 2019).
RIDEM Division of Forest Environment (2014a). Cover Assessment and Tree Benefit Analysis Report. Providence, RI: RIDEM Division of Forest Environment.
RIDEM Division of Forest Environment (2014b). State of Rhode Island i-Tree canopy cover Assessment and Tree Benefits Analysis Report. Providence, RI: RIDEM Division of Forest Environment.
Roy, S., Byrne, J., and Pickering, C. (2012). A systematic quantitative review of urban tree benefits, costs, and assessment methods across cities in different climatic zones. Urban For. Urban Green. 11, 351–363. doi: 10.1016/j.ufug.2012.06.006
Salih, A. A., Mohamed, A. A., Abahussain, A. A., and Tashtoosh, F. (2017). Use of some trees to mitigate air and soil pollution around oil refinery, Kingdom of Bahrain. J. Environ. Sci. Pollut. Res. 2, 167–170. Available online at: https://pdfs.semanticscholar.org/8a45/60d1a7e4bb77044bedc4b9425be453899a07.pdf
Selmi, W., Weber, C., Rivière, E., Blond, N., Mehdi, L., and Nowak, D. (2016). Air pollution removal by trees in public green spaces in Strasbourg city, France. Urban For. Urban Green. 2, 192–201. doi: 10.1016/j.ufug.2016.04.010
Setälä, H. M., Francini, G., Allen, J. A., Hui, N., Jumpponen, A., and Kotze, D. J. (2016). Vegetation type and age drive changes in soil properties, nitrogen, and carbon sequestration in urban parks under cold climate. Front. Ecol. Evol. 4, 1–14. doi: 10.3389/fevo.2016.00093
Shahmohamadi, P., Che-Ani, A., Maulud, K., Tawil, N., and Abdullah, N. (2011). The impact of anthropogenic heat on formation of urban heat island and energy consumption balance. Urban Stud. Res. 2011:497524. doi: 10.1155/2011/497524
Smith, G., and Levermore, C. (2008). Designing urban spaces and buildings to improve sustainability and quality of life in a warmer world, Energy Policy 36, 4558–4562. doi: 10.1016/j.enpol.2008.09.011
Styszko, K., Jaszczur, M., Teneta, J., Hassan, Q., Burzynska, P., Marcinek, E., et al. (2018). An analysis of the dust deposition on solar photovoltaic modules. Environ. Sci. Pollut. Res. 25, 1–9. doi: 10.1007/s11356-018-1847-z
Tang, S., and Chen, A. (2016). Carbon storage and sequestration of urban trees in Beijing, China. Front. Ecol. Evol. 4, 1–8. doi: 10.3389/fevo.2016.00053
Tchounwou, P., Yedjou, C., Patlolla, A., and Sutton, D. (2012). Heavy metals toxicity and the environment. Mol. Clin. Environ. Toxicol. 101, 133–164. doi: 10.1007/978-3-7643-8340-4_6
Teather, K., Hogan, N., Critchley, K., Gibson, M., Craig, S., and Hill, J. (2013). Examining the links between air quality, climate change and respiratory health in Qatar. Avicenna 9, 1–11. doi: 10.5339/avi.2013.9
Threlfall, S., Ossola, A., Hahs, A., Williams, N., and Wilson, L. (2016). Variation in vegetation structure and composition across urban green space types. Front. Ecol. Evol. 4, 1–12. doi: 10.3389/fevo.2016.00066
United States Department of Agriculture (1977). How Trees Help Clean the Air. Washington, DC: United States Department of Agriculture.
United States Environmental Protection Agency (2010). Particulate Matter Urban-Focused Visibility Assessment Final Document. Piedmont, NC: United States Environmental Protection Agency.
US Environmental Protection Agency (2008). Reducing Urban Heat Islands: Compendium of Strategies, Urban Heat Island Basics. Washington, DC: US Environmental Protection Agency.
USDA Forest Service (2006). i-Tree. Available online at: www.itreetools.org
Keywords: air quality, urban trees, economic assessment, urban planning, gaseous pollutants, particulate matter
Citation: Isaifan RJ and Baldauf RW (2020) Estimating Economic and Environmental Benefits of Urban Trees in Desert Regions. Front. Ecol. Evol. 8:16. doi: 10.3389/fevo.2020.00016
Received: 04 October 2019; Accepted: 21 January 2020;
Published: 13 February 2020.
Edited by:
Galina Churkina, Potsdam-Institut für Klimafolgenforschung (PIK), GermanyReviewed by:
Anthony James Brazel, Arizona State University, United StatesPrashant Kumar, University of Surrey, United Kingdom
Copyright © 2020 Isaifan and Baldauf. This is an open-access article distributed under the terms of the Creative Commons Attribution License (CC BY). The use, distribution or reproduction in other forums is permitted, provided the original author(s) and the copyright owner(s) are credited and that the original publication in this journal is cited, in accordance with accepted academic practice. No use, distribution or reproduction is permitted which does not comply with these terms.
*Correspondence: Rima J. Isaifan, risaifan@hbku.edu.qa
†These authors have contributed equally to this work