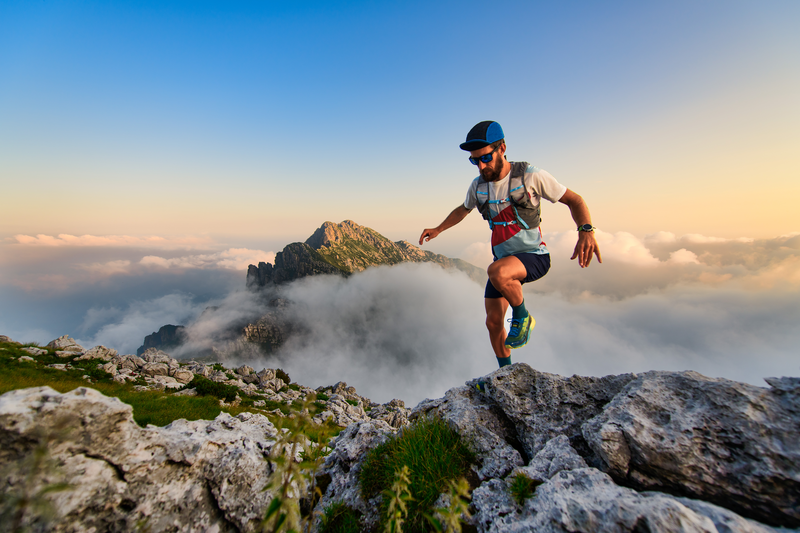
95% of researchers rate our articles as excellent or good
Learn more about the work of our research integrity team to safeguard the quality of each article we publish.
Find out more
ORIGINAL RESEARCH article
Front. Ecol. Evol. , 20 December 2019
Sec. Paleoecology
Volume 7 - 2019 | https://doi.org/10.3389/fevo.2019.00490
This article is part of the Research Topic A Golden Age for Strontium Isotope Research? Current Advances in Paleoecological and Archaeological Research View all 15 articles
Among mammals, including anthropoid primates, the primary factors that affect mobility are body size (larger-bodied species move more than smaller ones), diet (frugivores and trophic omnivores are more mobile than folivores), and habit (terrestrial taxa have larger home ranges than arboreal ones). If similar factors hold for Lemuriformes, we would expect large-bodied (particularly frugivorous) extinct lemurs to have been more mobile than smaller-bodied (particularly folivorous) extant species. Yet multiple lines of evidence (e.g., low Retzius Periodicities, small semicircular canal size, small relative brain size) suggest that extinct lemurs were relatively inactive. If so, they may have had relatively small home ranges, perhaps on par with smaller-bodied extant lemurs. We used strontium isotopes (87Sr/86Sr), which vary spatially primarily as a function of geology, to compare mobility for eight lemur genera: Eulemur, Lemur, Lepilemur, and Propithecus (extant), and Archaeolemur, Megaladapis, Pachylemur, and Palaeopropithecus (extinct). Subfossils came from two sites: Ankilitelo/Mikoboka, a series of sinkholes in a limestone plateau, and Ampasambazimba, a wetland underlain by a variety of igneous and metamorphic rocks. Within either site, we expected more mobile taxa to exhibit more variable 87Sr/86Sr, reflecting larger movement across a diversity of geologies. We found no differences in median 87Sr/86Sr or variance between extinct and extant lemurs at either site (Wilcoxon and Bartlett p > 0.05 for all comparisons). There were apparent but insignificant differences among genera (Kruskal-Wallis and Bartlett p > 0.05). Isotopic variability was greater at Ampasambazimba than at Ankilitelo/Mikoboka, reflecting differences in the underlying geology. One Palaeopropithecus from Ankilitelo/Mikoboka and one Eulemur from Ampasambazimba had unusually elevated 87Sr/86Sr. Both of these individuals could have been deposited at their respective sites by a predatory bird. These results demonstrate the value of 87Sr/86Sr for testing hypotheses related to the behavior of now-extinct species. Strontium isotopes support low mobility for extinct lemurs, and suggest that Lemuriformes as a whole differ from anthropoids in having relatively depressed basal metabolic rates and reduced activity levels. These traits reduce energetic expenditure, and likely developed in response to Madagascar's harsh environments. However, small home ranges also make lemurs more vulnerable to extinction.
Madagascar is well-known for its diversity of plants and animals, including its endemic primates, the lemurs. Over 100 living species of extant lemur are now recognized, and an additional 17 species went extinct in the Late Holocene (Burney et al., 2004; Crowley, 2010). Thanks to decades of multi-disciplinary research, a wealth of information is now available for Madagascar's extinct lemurs. All were larger-bodied than their extant relatives (ca. 12 to >100 kg vs. <100 g to <8 kg; Smith and Jungers, 1997; Jungers et al., 2008; Mittermeier et al., 2008), and we have a reasonably good understanding of what they ate and the kinds of environments that they inhabited (e.g., Jungers et al., 2002; Schwartz et al., 2002; Godfrey et al., 2006, 2012, 2016a; Crowley et al., 2012). However, we do not yet have a clear idea of how much they may have moved among habitats or across landscapes. Gaining further insight into movement patterns for these species would contribute to our understanding of ecological roles (e.g., their importance as seed-dispersers), which in turn could help inform conservation and biodiversity management decisions on Madagascar.
Among mammals, including anthropoid primates, there are a number of factors that affect home ranges. The primary control appears to be body size; smaller-bodied species tend to have smaller home ranges than larger-bodied species (Milton and May, 1976; Harvey and Clutton-Brock, 1981; Lindstedt et al., 1986; Ofstad et al., 2016; Crowley et al., 2017). Beyond this, terrestrial taxa tend to have larger home ranges than arboreal ones, frugivores tend to have larger home ranges than folivores, omnivores and faunivores have larger home ranges than herbivores, and animals living in open habitats tend to have larger home ranges than those living in denser habitats (Milton and May, 1976). These variables are correlated; for example, body size and diet are both tied to metabolism, and range size is related to the distribution and abundance of preferred resources, in addition to body size (Milton and May, 1976). On the basis of this evidence, we might expect the large-bodied (particularly frugivorous and terrestrial) extinct lemurs to have been more mobile than smaller-bodied (particularly folivorous and arboreal) extant species. This would be especially true for Archaeolemur, which has been reconstructed as one of the most terrestrial and frugivorous of the extinct lemurs (e.g., Tattersall, 1973; Jungers et al., 2002; Godfrey et al., 2005).
Yet multiple lines of evidence suggest that the extinct lemurs were relatively inactive. First, none of the giant lemurs have postcranial characteristics suggestive of high agility. Most were arboreal with adaptations for deliberate climbing, and some had adaptations for below-branch suspension (converging on sloths); none were saltatory and none were cursorial (Walker, 1974; Jouffroy and Lessertisseur, 1978; Godfrey et al., 1997; Jungers et al., 2002; Shapiro et al., 2005). Second, small semicircular canals, which are balance organs within the inner ear, also suggest low agility for all of the extinct taxa (Walker et al., 2008). Third, periodicity in the striae of Retzius is low in all measured extinct lemurs (Hogg et al., 2015; Schwartz and Rahantaharivao, unpublished data on Pachylemur). These striae are lamellar growth bands in tooth enamel related to biological rhythms, such as the Havers-Halberg Oscillation (HHO), which modulate life-history related traits like brain and body size, age at first reproduction, and activity levels (Bromage et al., 2012). Because Retzius Periodicity (RP) intervals correlate strongly with body size in most mammals, including anthropoid primates, we would expect the larger-bodied extinct taxa to have longer intervals than smaller-bodied extant lemurs. However, this is not the case; all lemuriforms, including the giant lemurs, have relatively low values for RP (Hogg et al., 2015). Archaeolemur has a slightly higher RP value than other taxa (4 vs. 2 or 3), but this value is still quite low in comparison to anthropoids of similar body size, such as Theropithecus, the gelada (RP = 7), with which Archaeolemur has been compared. Hogg et al. (2015) hypothesized that low RP relates to constraints on energy expenditure in lemurs (i.e., selection for risk-averse life histories), which could impact mobility and thus home-range size. Lastly, extinct lemurs had relatively small brains (Catlett et al., 2010), which further suggests low basal metabolic rate, low energy expenditure, and in turn low activity levels. On the basis of this collective body of evidence, we might expect that extinct lemurs had relatively small home-ranges, perhaps as small as those of much smaller-bodied extant lemurs.
Strontium isotope ratios (87Sr/86Sr) in tooth enamel and bone may be able to detect mobility differences among co-occurring extinct and extant taxa. Strontium ions leached from rocks are taken up by plants, and subsequently into animal tissues with negligible fractionation (Capo et al., 1998; Bentley, 2006; Lewis et al., 2017). Because biologically available (bioavailable) 87Sr/86Sr is closely tied to geology, it varies spatially. Madagascar is well-suited for this type of geochemical approach. Its rocks preserve nearly three billion years of our planet's history (reviewed in Crowley and Sparks, 2018), and consequently, the island's geology is extraordinarily diverse (Roig et al., 2012). Within any given site, more mobile taxa should exhibit more variable 87Sr/86Sr, reflecting larger movement across a diversity of geologies.
We used strontium isotopes to compare mobility for extant and extinct lemur genera that are reasonably well-represented in subfossil deposits and fill much of the spectrum of variation in diet and locomotor habits (Table 1): Eulemur, Lemur, Lepilemur and Propithecus (extant), and Archaeolemur, Megaladapis, Pachylemur, and Palaeopropithecus (extinct). Eulemur is one of the more frugivorous of extant lemurids and an important seed disperser, Lemur is more folivorous but still an important seed disperser, Propithecus is yet more folivorous and more of a seed predator than a seed disperser, and Lepilemur is a specialized folivore. These taxa vary in their preferred locomotor habits; Eulemur, Lemur, and Propithecus spend time both on the ground and in the trees while Lepilemur is primarily arboreal. The extinct genera that we sampled also run the gamut from the frugivorous and seed dispersing Pachylemur to the specialized folivore Megaladapis. Archaeolemur and Palaeopropithecus had more intermediate diets and were likely seed predators (especially Archaeolemur). Pachylemur, Megaladapis and Palaeopropithecus have anatomical adaptations suggesting they were primarily arboreal while Archaeolemur was likely semi-terrestrial.
Because bioavailable 87Sr/86Sr is spatially variable, differences in mobility among taxa can only be examined within single sites or regions (i.e., among co-occurring taxa exposed to the same baseline conditions). We sampled Holocene subfossil material from two localities that have relatively abundant and robust preservation: Ankilitelo/Mikoboka and Ampasambazimba (Crowley, 2010; Muldoon, 2010; Crowley et al., 2012, 2017; Goodman et al., 2013; Godfrey et al., 2016a; Figure 1). Ankilitelo/Mikoboka is a series of sinkholes in a plateau in southwestern Madagascar composed of Eocene limestone, oolitic limestone, and sandstone (Roig et al., 2012). Ampasambazimba is a wetland in Central Madagascar underlain by a variety of igneous and metamorphic rocks dating to the Neoarchean through the Tertiary (Roig et al., 2012).
Figure 1. Maps showing the location of our two study sites in Madagascar, as well as the geology at Ankilitelo/Mikoboka (A), and Ampasambazimba (B). Geologic maps were modified from Roig et al. (2012).
We acquired bones and teeth from previously collected, curated material housed at a variety of museum and university collections, including the University of Antananarivo, the University of Massachusetts Amherst, and the Division of Fossil Primates at the Duke Lemur Center (Supplementary Table 1). In total, we were able to include 25 specimens from Ampasambazimba and 30 specimens from Ankilitelo/Mikoboka.
Specimen surfaces were cleaned using a tooth brush or a rotary Dremel tool equipped with a dental drill bit. We then removed 10–20 mg of powder from each sample using the Dremel or by pulverizing fragments with an agate mortar and pestle. Bone and enamel carbonate were isolated chemically (following Crowley and Wheatley, 2014; Baumann and Crowley, 2015; Crowley et al., 2018). Organics were removed by soaking samples in 30% H2O2 at room temperature. Enamel samples were soaked for 24 h; bone samples were allowed to react for 72 h, and liquid was replaced between 24 and 48 h. Samples were rinsed 5x with ultrapure water and then reacted for 24 h in 1 M calcium-buffered acetic acid at 4°C. They were again rinsed 5x with ultrapure water, and freeze dried. During both chemical pretreatment steps, samples were agitated regularly to help ensure consistent reaction.
Pretreated samples were sent to the Multicollector Inductively Coupled Plasma Mass Spectrometry (MC-ICPMS) Laboratory in the Geology Department at the University of Illinois Urbana-Champaign for 87Sr/86Sr analysis. Three to five mg of each sample were dissolved in 3N HNO3 and filtered through 0.2 mL of Eichrom Sr spec resin (100–150 mm) packed into Teflon ion-exchange columns. Samples were then eluted with a combination of ultrapure water and 0.05 N HNO3 into 4-mL Teflon autosampler vials for analysis. Samples were analyzed on a Nu plasma High Resolution MC-ICPMS (Nu Instruments Ltd, Wrexham, Wales, UK). Data were normalized using SRM-987 (accepted 87Sr/86Sr = 0.710255), and the quality of resulting corrected data was checked using two independent internal standards—South China Sea Coral (87Sr/86Sr = 0.70918) and “E&A” (87Sr/86Sr = 0.70804). Reported precision for the lab is ± 0.00005.
At each site, we compared strontium isotope ratios among genera and between extinct and extant lemurs (combining genera). Because we have small and uneven sample sizes, we used non-parametric Wilcoxon and Kruskal-Wallis analyses. We assessed homoscedasticity using Bartlett tests. For all analyses, we used JMP Pro 14.0 with significance set at α = 0.05.
One Palaeopropithecus from Ankilitelo/Mikoboka and one Eulemur from Ampasambazimba had unusually elevated 87Sr/86Sr compared to other individuals at the same sites (Figures 2, 3). The Palaeopropithecus was not a statistical outlier for extinct lemurs but was a statistical outlier for this genus at Ankilitelo/Mikoboka. Conversely, the Eulemur was a statistical outlier for extant lemurs but was not an outlier for this genus at Ampasambazimba. There was nothing unusual about either of these samples (either in terms of preservation or analysis), which suggests that these data are real. We ran all statistical analyses both including and excluding these two individuals.
Figure 2. Box plots comparing extinct and extant lemurs (A) and all genera (B) at Ampasambazimba. Statistical results do not change if the outlier Eulemur is excluded (χ2 = 0.51, df = 1, p = 0.47, and χ2 = 9.75, df = 6, p = 0.14, respectively).
Figure 3. Box plots comparing extinct and extant lemurs (A) and all genera (B) at Ankilitelo/Mikoboka. Statistical results do not change if the outlier Palaeopropithecus is excluded (χ2 = 1.14, df = 1, p = 0.29, and χ2 = 2.95, df = 5, p = 0.71, respectively). Asterisks (*) indicate material from non-Ankilitelo sinkholes on the Mikoboka plateau.
There were no differences in median 87Sr/86Sr or isotopic variance between extinct and extant lemurs at either Ankilitelo/Mikoboka or Ampasambazimba (Figures 2, 3). These results were consistent whether or not we included the outliers at each site (Wilcoxon and Bartlett p > 0.05 for all comparisons). There were small apparent differences in median 87Sr/86Sr among individual genera at both sites, but these were not significant (Kruskal-Wallis p > 0.05; Figures 2, 3). Likewise, differences in variance among genera at either site were insignificant (Bartlett p > 0.05; Figure 4). Excluding the outlier individuals, variance was reasonably consistent among taxa at both sites, although Palaeopropithecus had considerably smaller variability in 87Sr/86Sr than other taxa at both Ankilitelo and Ampasambazimba (Figure 4). Including the outliers did not affect the significance of these results, although there were some apparent changes. Specifically, Palaeopropithecus had apparently larger variance than all other taxa, including Eulemur, at Ankilitelo/Mikiboka, while variance for Eulemur was roughly two times larger than Archaeolemur, and four times larger than Palaeopropithecus at Ampasambazimba (Figure 4).
Figure 4. Standard deviations of 87Sr/86Sr for each genus at Ampasambazimba (A) and (C) and Ankilitelo/Mikoboka (B) and (D). The dashed blue lines in each panel indicate the average for all taxa. Outliers are included in (A) and (C) and excluded in (B) and (D).
We set out to compare mobility of sympatric extinct and extant lemurs using strontium isotopes. Variability in 87Sr/86Sr exhibited by both extant and extinct lemurs was greater at Ampasambazimba than at Ankilitelo/Mikoboka (Figures 2–4). This likely reflects differences in the underlying geology of the two regions (Figure 1; Crowley et al., 2015). While Ampasambazimba is situated in Quaternary sediments, it is surrounded by Neogene volcanics. There are also outcrops of Ediacaran to Cambrian granites, and very old metamorphic complexes in close proximity to the site, including both Cryogenian paragneiss and schist and Neoarchaean orthogneiss (Roig et al., 2012). Typically, geologic heterogeneity is beneficial for detecting differences in mobility among individuals; however, this degree of geologic complexity may, in fact, hamper our ability to identify differences in mobility among taxa at Ampasambazimba (Figures 2, 4). Even the Eulemur with an elevated Sr isotope ratio could easily have spent much of its life foraging on one or more of these older geologies before meeting its demise at Ampasambazimba. It may have moved to the site on its own, or have been deposited by a predator, such as the crowned eagle (Stephanoaetus mahery), which has previously been implicated in the accumulation of lemur remains at the site (Goodman, 1994).
The story at Ankilitelo/Mikoboka is rather different. Here, the geology is much more homogenous, and overall variability in 87Sr/86Sr is quite low (Figures 3, 4). Curiously, the distribution of strontium isotope ratios at Ankilitelo/Mikoboka is apparently bimodal. This is most obvious for extant lemurs, but it also holds for Megaladapis and Palaeopropithecus. Small apparent isotopic differences may reflect resource partitioning. Extant sympatric lemurs do this by feeding at different times or heights within the forest canopy, or targeting different species of plants (e.g., Ganzhorn, 1988; Wright et al., 2011). Today the habitat surrounding both Ankilitelo and Ampasambazimba is degraded and ruderal vegetation dominates. However, this is likely a relatively recent phenomenon. On the basis of faunal comparisons, Muldoon (2010) argued that vegetation at Ankilitelo during the Late Holocene was similar to succulent woodland or spiny thicket (both of which are native biomes in the region today; Burgess et al., 2004). This type of vegetation would have made it challenging for larger-bodied arboreal folivores, like Megaladapis and Palaeopropithecus, to feed at different canopy heights. However, it seems quite likely that they would have targeted different food species, as extant folivores do today (Ganzhorn, 1988; Warren, 1997; Thalmann, 2006). They may also have spatially segregated where they foraged. Either of these scenarios could result in isotopic differences between lemur taxa. First, co-occurring species of trees may have slightly different 87Sr/86Sr due to differences in rooting depth and nutrient cycling (Poszwa et al., 2004). Second, although the geology of the Mikoboka Plateau is relatively homogenous, there is still some lithologic variability. For example, the primary geology is Eocene limestone (“e4”; Figure 1), but sandstone is also present within this stratigraphic unit (Roig et al., 2012). Moreover, there is a mapped outcrop of Quaternary hardground (Qcs) not far from Ankilitelo (Figure 1), and it is highly likely that additional unmapped smaller outcrops are present in the vicinity of the sinkholes. Both sandstone and Qcs would be expected to have higher 87Sr/86Sr than Eocene limestone given that they are comprised of sediments derived from a variety of sources (Crowley et al., 2015). Strontium isotope ratios <0.708 would be consistent with foraging on Eocene limestone (Mcarthur et al., 2001), while ratios larger than this likely reflect input from either sandstone or Quaternary deposits.
Given that multiple lines of evidence suggest sloth lemurs had small ranges, it seems unlikely that the individual Palaeopropithecus with an elevated 87Sr/86Sr moved a great distance before meeting its demise at Ankilitelo. There are at least two explanations that would not necessarily require long distance ranging for this individual. First, the ratio for this individual (0.71085) could be consistent with foraging on local Quaternary sediments (e.g., Qcs). Yet if this were the case, we might expect at least some other individuals to also have similarly elevated 87Sr/86Sr. Second, like the Eulemur from Ampasambazimba, it is possible that this Palaeopropithecus was transported to Ankilitelo by a predatory bird. Raptors are among the most common taxa represented in the subfossil material from Ankilitelo, and it has been suggested that the sinkhole was used as a roosting and nesting location (Goodman et al., 2013). Most of the remains recovered belong to smaller-bodied hawks, kites, and owls that would not be able to carry a quarry as large as Palaeopropithecus. However, remains of at least one extinct crowned eagle (Stephanoaetus mahery) have also been recovered from Ankilitelo (Goodman and Muldoon, 2016). This species was considerably larger than still-extant raptors, and likely larger than its living congener, the African crowned eagle, which regularly predates primates up to ca. 12 kg (Goodman, 1994; Mitani et al., 2001; Mcgraw, 2006). Although S. mahery likely preferred taxa <25 kg, taphonomic evidence indicates that it was capable of eating extinct lemurs as large as Megaladapis edwardsi (the second largest extinct lemur; Meador et al., 2019).
In summary, 87Sr/86Sr data support comparable mobility for extinct and extant lemurs. This finding contradicts expectations based on body size, but is compatible with other lines of evidence that suggest these extinct taxa were relatively anchored (e.g., Walker et al., 2008; Hogg et al., 2015). Compared to like-sized anthropoids, the extinct lemurs have considerably smaller semi-circular canals, endocranial volume, and lower Retzius Periodicity (Walker et al., 2008; Catlett et al., 2010; Hogg et al., 2015).
Palaeopropithecus, in particular, is thought to have been very slow and sloth like (reviewed in Walker et al., 2008). Elongated forelimbs and curved phalanges demonstrate that it was skilled at below-the-branch quadrupedal suspension (Jungers et al., 2002; Godfrey et al., 2016b) and its semicircular canal radius is comparable to Lepilemur (an animal that weighs ~1 kg). If we exclude the single Palaeopropithecus at Ankilitelo/Mikoboka with an elevated 87Sr/86Sr, we note that this genus has apparently smaller variance than co-occurring taxa at both sites, perhaps on par with Lepilemur (Figures 2, 3).
Both living and extinct Lemuriformes appear to differ from anthropoid primates in their relatively depressed basal metabolism, small brains, low Retzius Periodicity, and reduced activity levels. Collectively, this suite of traits serves to reduce energetic expenditure, and likely developed so lemurs can cope with living in the harsh environments that characterize Madagascar (Wright, 1999; Godfrey et al., 2006; Hogg et al., 2015).
Unfortunately, these same traits may make lemurs more vulnerable to extinction. Home range size is often used as a parameter in evaluating extinction risk for a species. Specifically, species with limited mobility are more threatened by habitat fragmentation than species with greater mobility, and large-bodied species are more threatened by habitat fragmentation than small-bodied ones (Haskell et al., 2002). However, diet, resource density and resource distribution also play a role. Frugivores are more vulnerable than folivores because of differences in the distribution of preferred food resources; indeed, folivore population density may even increase following low- or medium-level disturbances such as cyclones, because young foliage tends to increase as pioneer species fill light gaps caused by the destruction of older fruit-producing trees (Johns and Skorupa, 1987).
Given this, it is, perhaps, unsurprising that larger-bodied and frugivorous lemurs are the ones to have disappeared first. Unfortunately, their extinction has reverberating consequences. Globally, the loss of large-seed dispersal services is one of the most challenging problems facing conservationists in the wake of recent megafaunal extinctions (Corlett, 2013), and this is particularly challenging in places like Madagascar, where these services may have been marginal prior to the loss of the largest-bodied species. The size of the primate frugivore guild on Madagascar is small (Fleagle and Reed, 1996; Goodman and Ganzhorn, 1997). Climatic unpredictability and hypervariability have been posited as contributing to this (Wright, 1999; Wright et al., 2005; Dewar and Richard, 2007). Madagascar also has extended dry or lean seasons which can result in erratic fruiting, and a high frequency of cyclones, which can be extremely destructive to older fruiting trees. Furthermore, at any single point in time, fruit is generally less abundant in Madagascar than it is in other places that are home to many primate species (Federman et al., 2017). Additionally or alternatively, it appears that low levels of fruit nitrogen are responsible for the poor representation of frugivores in Madagascar's primate community (Ganzhorn et al., 2009; Donati et al., 2017; Federman et al., 2017). This argument is grounded in the observations, not merely that the fruits of Madagascar have low nitrogen content, but also that such fruits cannot meet the protein requirements of primates during critical times of the year such as the reproductive season.
What is less clear is the extent to which large, fruit-bearing trees and their seed dispersers were better represented in the recent past, the degree to which they face disproportionate extinction risk in the future, and the degree to which that risk depends on the survival of primates. Although Madagascar today has an unusually low number of plant species that disperse their seeds via endozoochory, most of those that do rely on endozoochory have adaptations to attract primates rather than birds (Albert-Daviaud et al., 2018). This is particularly the case for trees with large seeds (Razafindratsima et al., 2018). Thus, the primate frugivore guild plays a very important role in seed dispersal. Threats to plant communities in Madagascar are compounded by the facts that: (1) the primate frugivore guild on Madagascar is increasingly dominated by species too small to disperse large seeds (Richard and Dewar, 1991; Federman et al., 2016); and (2) still-extant seed-dispersing lemurs (i.e., those more likely to pass seeds whole and undamaged through the gut; Dew and Wright, 1998; Razafindratsima and Martinez, 2012) have small seed-dispersal distances in comparison to like-sized frugivores on other continents (Razafindratsima et al., 2014). When seed dispersers have limited home ranges, the plant community as a whole may be more vulnerable to habitat disturbance, and therefore at greater risk of entering into an extinction vortex. The problem is not merely that the loss of key seed dispersers may result in an increase in the number of “orphaned” plants (those lacking seed dispersers; Bollen et al., 2004; Godfrey et al., 2008; Crowley et al., 2011; Buerki et al., 2015; Albert-Daviaud et al., 2018), but also that the plant species most likely to become orphaned are the trees with the highest above-ground biomass, and therefore the greatest capacity to store carbon. They are precisely the trees that contribute the most to climate stability and the health of the entire ecosystem (Razafindratsima et al., 2018). With this in mind, it is imperative that remaining forest cover be protected and that connectivity among fragments be improved.
The dataset analyzed for this study can be found in Supplementary Table 1.
BC and LG conceived of the project. BC prepared samples and conducted the data analysis. Both authors wrote the manuscript.
Funding was provided by NSF BCS-1749676 to BC and NSF BCS-1750598 to LG.
The authors declare that the research was conducted in the absence of any commercial or financial relationships that could be construed as a potential conflict of interest.
We thank Gideon Bartov, Gregg Gunnell, Ian Macadam, Tom Johnson, Catherine Riddle, and Jani Sparks for technical and logistical assistance. This is Duke Lemur Center publication #1448.
The Supplementary Material for this article can be found online at: https://www.frontiersin.org/articles/10.3389/fevo.2019.00490/full#supplementary-material
Albert-Daviaud, A., Perillo, S., and Stuppy, W. (2018). Seed dispersal syndromes in the Madagascan flora: the unusual importance of primates. Oryx 52, 418–426. doi: 10.1017/S0030605317001600
Baumann, E., and Crowley, B. E. (2015). Stable isotopes reveal ecological differences amongst now-extinct proboscideans from the Cincinnati region, USA. Boreas 44, 240–254. doi: 10.1111/bor.12091
Bentley, R. A. (2006). Strontium isotopes from the Earth to the archaeological skeleton: a review. J. Archaeol. Method Th. 13, 135–187. doi: 10.1007/s10816-006-9009-x
Bollen, A., Elsacker, L. V., and Ganzhorn, J. U. (2004). Relations between fruits and disperser assemblages in a Malagasy littoral forest: a community-level approach. J. Trop. Ecol. 20, 599–612. doi: 10.1017/S0266467404001853
Bromage, T. G., Hogg, R. T., Lacruz, R. S., and Hou, C. (2012). Primate enamel evinces long period biological timing and regulation of life history. J. Theor. Biol. 305, 131–144. doi: 10.1016/j.jtbi.2012.04.007
Buerki, S., Callmander, M. W., Bachman, S., Moat, J., Labat, J.-N., and Forest, F. (2015). Incorporating evolutionary history into conservation planning in biodiversity hotspots. Philos. Trans. Royal Soc. B 370:20140014. doi: 10.1098/rstb.2014.0014
Burgess, N. D., D'amico Hales, J., Underwood, E. C., Dinerstein, E., Olson, D., Itoua, I., et al. (2004). Terrestrial Ecoregions of Africa and Madagascar: A Conservation Assessment. Washington, DC: Island Press.
Burney, D. A., Burney, L. P., Godfrey, L. R., Jungers, W. L., Goodman, S. M., Wright, H. T., et al. (2004). A chronology for late prehistoric Madagascar. J. Hum. Evol. 47, 25–63. doi: 10.1016/j.jhevol.2004.05.005
Capo, R. C., Stewart, B. W., and Chadwick, O. A. (1998). Strontium isotopes as tracers of ecosystem processes: theory and methods. Geoderma 82, 197–225. doi: 10.1016/S0016-7061(97)00102-X
Catlett, K. K., Schwartz, G. T., Godfrey, L. R., and Jungers, W. L. (2010). “Life History Space”: a multivariate analysis of life history variation in extant and extinct Malagasy lemurs. Am. J. Phys. Anthropol. 142, 391–404. doi: 10.1002/ajpa.21236
Corlett, R. T. (2013). The shifted baseline: prehistoric defaunation in the tropics and its consequences for biodiversity conservation. Biol. Conserv. 163, 13–21. doi: 10.1016/j.biocon.2012.11.012
Crowley, B. E. (2010). A refined chronology of prehistoric Madagascar and the demise of the megafauna. Quat. Sci. Rev. 29, 2591–2603. doi: 10.1016/j.quascirev.2010.06.030
Crowley, B. E., Castro, I., Soarimalala, V., and Goodman, S. M. (2018). Isotopic evidence for niche partitioning and the influence of anthropogenic disturbance on endemic and introduced rodents in central Madagascar. Sci. Nat. 105:44. doi: 10.1007/s00114-018-1564-y
Crowley, B. E., Godfrey, L. R., Bankoff, R. J., Perry, G. H., Culleton, B. J., Kennett, D. J., et al. (2017). Island-wide aridity did not trigger recent megafaunal extinctions in Madagascar. Ecography 40, 901–912. doi: 10.1111/ecog.02376
Crowley, B. E., Godfrey, L. R., Guilderson, T. P., Zermeño, P., Koch, P. L., and Dominy, N. J. (2012). Extinction and ecological retreat in a community of primates. Proc. Royal Soc. Lond. B. 279, 3597–3605. doi: 10.1098/rspb.2012.0727
Crowley, B. E., Godfrey, L. R., and Irwin, M. T. (2011). A glance to the past: subfossils, stable isotopes, seed dispersal, and lemur species loss in southern Madagascar. Am. J. Primatol. 73, 25–37. doi: 10.1002/ajp.20817
Crowley, B. E., Slater, P. A., Muldoon, K. M., and Godfrey, L. R. (2015). Reconstructing the mobility of Madagascar's fauna using strontium isotopes: results and implications for management and conservation. Am. J. Phys. Anthropol. 156:252.
Crowley, B. E., and Sparks, J. A. (2018). “Geology,” in Les Aires Protégées Terrestres de Madagascar: Leur Histoire, Description et Biote / The Terrestrial Protected Areas of Madagascar: Their History, Description, and Biota, eds S. M. Goodman, M. J. Raherilalao, and S. Wolhauser (Antananarivo: Association Vahatra), 169–180.
Crowley, B. E., and Wheatley, P. V. (2014). To bleach or not to bleach? Comparing treatment methods for isolating biogenic carbonate. Chem. Geol. 381, 234–242. doi: 10.1016/j.chemgeo.2014.05.006
Dew, J. L., and Wright, P. C. (1998). Frugivory and seed dispersal by four species of primates in Madagascar's eastern rain forest. Biotropica 30, 425–437. doi: 10.1111/j.1744-7429.1998.tb00076.x
Dewar, R. E., and Richard, A. F. (2007). Evolution in the hypervariable environment of Madagascar. Proc. Natl. Acad. Sci. U.S.A. 103, 13723–13727. doi: 10.1073/pnas.0704346104
Donati, G., Santini, L., Eppley, T. M., Arrigo-Nelson, S. J., Balestri, M., Boinski, S., et al. (2017). Low levels of fruit nitrogen as drivers for the evolution of Madagascar's primate communities. Sci. Rep. 7:14406. doi: 10.1038/s41598-017-13906-y
Federman, S., Dornburg, A., Daly, D. C., Downie, A., Perry, G. H., Yoder, A. D., et al. (2016). Implications of lemuriform extinctions for the Malagasy flora. Proc. Natl. Acad. Sci. U.S.A. 113, 5041–5046. doi: 10.1073/pnas.1523825113
Federman, S., Sinnott-Armstrong, M., Baden, A. L., Chapman, C. A., Daly, D. C., Richard, A. R., et al. (2017). The paucity of frugivores in Madagascar may not be due to unpredictable temperatures or fruit resources. PLoS ONE 12:e0168943. doi: 10.1371/journal.pone.0168943
Fleagle, J. G., and Reed, K. E. (1996). Comparing primate communities: a multivariate approach. J. Hum. Evol. 30, 489–510. doi: 10.1006/jhev.1996.0039
Ganzhorn, J. U. (1988). Food partitioning among Malagasy primates. Oecologia 75, 436–450. doi: 10.1007/BF00376949
Ganzhorn, J. U., Arrigo-Nelson, S. J., Boinski, S., Bollen, A., Carrai, V., Derby, A., et al. (2009). Possible fruit protein effects on primate communities in Madagascar and the Neotropics. PLoS ONE 4:e8253. doi: 10.1371/journal.pone.0008253
Godfrey, L. R., Crowley, B. E., Muldoon, K. M., Kelley, E. A., King, S. J., Best, A. W., et al. (2016a). What did Hadropithecus eat, and why should paleoanthropologists care? Am. J. Primatol. 78, 1098–1112. doi: 10.1002/ajp.22506
Godfrey, L. R., Granatosky, M. C., and Jungers, W. L. (2016b). “The hands of subfossil lemurs,” in The Evolution of the Primate Hand: Anatomical, Developmental, Functional, and Paleontological Evidence, eds T. L. Kivell, P. Lemelin, B. G. Richmond, and D. Schmitt (New York, NY: Springer Science), 421–453. doi: 10.1007/978-1-4939-3646-5_15
Godfrey, L. R., Jungers, W. L., and Schwartz, G. T. (2006). “Ecology and extinction of Madagascar's subfossil lemurs,” in Lemurs: Ecology and Adapaptation, eds L. Gould and M. L. Sauther (New York, NY: Springer), 41–64. doi: 10.1007/978-0-387-34586-4_3
Godfrey, L. R., Jungers, W. L., Schwartz, G. T., and Irwin, M. T. (2008). “Ghosts and orphans: Madagascar's vanishing ecosystems,” in Elwyn Simons: A Search for Origins, eds J. G. Fleagle and C. C. Gilbert (New York, NY: Springer), 361–395. doi: 10.1007/978-0-387-73896-3_24
Godfrey, L. R., Samonds, K. E., Jungers, W. L., Sutherland, M. R., and Irwin, M. T. (2004a). Ontogenetic correlates of diet in Malagasy lemurs. Am. J. Phys. Anthropol. 123, 250–276. doi: 10.1002/ajpa.10315
Godfrey, L. R., Semprebon, G., Schwartz, G. T., Burney, D. A., Jungers, W. L., Flanagan, E. K., et al. (2005). New insights into old lemurs: the trophic adaptations of the Archaeolemuridae. Int. J. Primatol. 26, 825–854. doi: 10.1007/s10764-005-5325-3
Godfrey, L. R., Semprebon, G. M., Jungers, W. L., Sutherland, M. R., Simons, E. L., and Solounias, N. (2004b). Dental use wear in extinct lemurs: evidence of diet and niche differentiation. J. Hum. Evol. 47, 145–169. doi: 10.1016/j.jhevol.2004.06.003
Godfrey, L. R., Winchester, J. M., King, S. J., Boyer, D. M., and Jernvall, J. (2012). Dental topography indicates ecological contraction of lemur communities. Am. J. Phys. Anthropol. 148, 215–227. doi: 10.1002/ajpa.21615
Godfrey, L. R., Wunderlich, R. E., and Richmond, B. C. (1997). Reappraisal of the postrcranium of Hadropithecus (Primates, Indroidea). Am. J. Phys. Anthropol. 103, 529–56. doi: 10.1002/(SICI)1096-8644(199708)103:4<529::AID-AJPA9>3.0.CO;2-H
Goodman, S. M. (1994). Description of a new species of subfossil eagle from Madagascar, Stephanoaetus (Aves, Falconiformes) from the deposits of Ampasambazimba. Proc. Biol. Soc. Washington 107, 421–428.
Goodman, S. M., and Ganzhorn, J. U. (1997). Rarity of figs (Ficus) on Madagascar and its relationship to a depauperate frugivore community. Rev. Ecol. Terr. Vie 52, 321–329.
Goodman, S. M., and Muldoon, K. M. (2016). A new subfossil locality for the extinct large Malagasy eagle Stephanoaetus mahery (Aves: Falconiformes): implications for time of extinction and ecological specificity. Holocene 26, 985–989. doi: 10.1177/0959683615622554
Goodman, S. M., Raherilalao, M. J., and Muldoon, K. M. (2013). Bird fossils from Ankilitelo Cave: inference about Holocene environmental changes in Southwestern Madagascar. Zootaxa 5, 534–548. doi: 10.11646/zootaxa.3750.5.6
Gordon, A. D. (2006). Scaling of size and dimorphism in primates II: macroevolution. Int. J. Primatol. 27, 63–105. doi: 10.1007/s10764-005-9004-1
Gould, L. (2006). “Lemur catta ecology: what we know and what we need to know,” in Lemurs: Ecology and Adaptation, eds L. Gould and M. L. Sauther (Boston, MA: Springer), 255–274. doi: 10.1007/978-0-387-34586-4_12
Harvey, P. H., and Clutton-Brock, T. H. (1981). Primate home-range size and metabolic needs. Behav. Ecol. Sociobiol. 8, 151–155. doi: 10.1007/BF00300828
Haskell, J. P., Ritchie, M. E., and Olff, H. (2002). Fractal geometry predicts varying body size scaling relationships for mammal and bird home ranges. Nature 418, 527–530. doi: 10.1038/nature00840
Hogg, R. T., Godfrey, L. R., Schwartz, G. T., Dirks, W., and Bromage, T. G. (2015). Lemur biorhythms and life history evolution. PLoS ONE 10:e0134210. doi: 10.1371/journal.pone.0134210
Isler, K., Kirk, E. C., Miller, J. M., Albrecht, G. A., Gelvin, B. R., and Martin, R. D. (2008). Endocranial volumes of primate species: scaling analyses using a comprehensive and reliable data set. J. Hum. Evol. 55, 967–978. doi: 10.1016/j.jhevol.2008.08.004
Johns, A. D., and Skorupa, J. P. (1987). Responses of rain-forest primates to habitat disturbance: a review Int. J. Primatol. 8, 157–191. doi: 10.1007/BF02735162
Jouffroy, F. K., and Lessertisseur, J. (1978). Etude ecomorphologique des proportions des members des primates et specialement des prosimiens. Annal. Sci. Nat. Zool. 20, 99–128.
Jungers, W. L., Demes, B., and Godfrey, L. R. (2008). “How big were the “giant extinct lemurs of Madagascar?,” in Elwyn Simons: A Search for Origins, eds J. G. Fleagle and C. C. Gilbert (New York, NY: Springer), 343–360. doi: 10.1007/978-0-387-73896-3_23
Jungers, W. L., Godfrey, L. R., Simons, E. L., Wunderlich, R. E., Richmond, B. G., and Chatrath, P. S. (2002). “Ecomorphology and behavior of giant extinct lemurs from Madagascar,” in Reconstructing Behavior in the Primate Fossil Record, eds J. M. Plavcan, R. F. Kay, W. L. Jungers, and C. P. Van Schaik (New York, NY: Kluwer Academic/Plenum), 371–411. doi: 10.1007/978-1-4615-1343-8_10
Lewis, J., Pike, A. W. G., Coath, C. D., and Evershed, R. P. (2017). Strontium concentration, radiogenic (87Sr/86Sr) and stable (δ88Sr) strontium isotope systematics in a controlled feeding study. STAR 3, 45–57. doi: 10.1080/20548923.2017.1303124
Lindstedt, S. L., Miller, B. J., and Buskirk, S. W. (1986). Home range, time, and body size in mammals. Ecology 67, 413–418. doi: 10.2307/1938584
Mcarthur, J. M., Howarth, R. J., and Bailey, T. R. (2001). Strontium isotope stratigraphy: LOWESS Version 3: best fit to the marine Sr-isotope curve for 0–509 Ma and accompanying look-up table for deriving numerical age. Geology 109, 155–170. doi: 10.1086/319243
Mcgraw, W. S. (2006). Primate remains from African crowned eagle (Stephanoaetus coronatus) nests in Ivory Coast's Tai Forest: implications for primate predation and early hominid taphonomy in South Africa. Am. J. Phys. Anthropol. 131, 151–165. doi: 10.1002/ajpa.20420
Meador, L. R., Godfrey, L. R., Rakotondramavo, J. C., Ranivoharimanana, L., Zamora, A., Sutherland, M. R., et al. (2019). Cryptoprocta spelea (Carnivora: Eupleridae): what did it eat and how do we know? J. Mamm. Evol. 26, 237–251. doi: 10.1007/s10914-017-9391-z
Milton, K., and May, M. L. (1976). Body weight, diet and home range area in primates. Nature 259, 459–462. doi: 10.1038/259459a0
Mitani, J. C., Sanders, W. J., Lwanga, J. S., and Windfelder, T. L. (2001). Predatory behavior of crowned hawkeagles (Stephanoaetus coronatus) in Kibale National Park, Uganda. Behav. Ecol. Sociobiol. 49, 187–195. doi: 10.1007/s002650000283
Mittermeier, R. A., Ganzhorn, J. U., Konstant, W. R., Glander, K., Tattersall, I., Groves, C. P., et al. (2008). Lemur diversity in Madagascar. Int. J. Primatol. 29, 1607–1656. doi: 10.1007/s10764-008-9317-y
Muchlinski, M. N., Godfrey, L. R., Muldoon, K. M., and Tongasoa, L. (2011). Evidence for dietary niche separation based on infraorbital foramen size variation among subfossil lemurs. Folia Primatol. 81, 330–345. doi: 10.1159/000323277
Muldoon, K. M. (2010). Paleoenvironment of Ankilitelo Cave (late Holocene, southwestern Madagascar): implications for the extinction of the giant lemurs J. Hum. Evol. 58, 338–352. doi: 10.1016/j.jhevol.2010.01.005
Ofstad, E. G., Herfindal, I., Solberg, E. J., and Sæther, B. E. (2016). Home ranges, habitat and body mass: simple correlates of home range size in ungulates. Proc. Royal Soc. Lond. B 283:20161234. doi: 10.1098/rspb.2016.1234
Poszwa, A., Ferry, B., Dambrine, E., Pollier, B., Wickman, T., Loubet, M., et al. (2004). Variations of bioavailable Sr concentration and 87Sr/86Sr ratio in boreal forest ecosystems. Role of biocycling, mineral weathering and depth of root uptake. Biogeochemistry 67, 1–20. doi: 10.1023/B:BIOG.0000015162.12857.3e
Razafindratsima, O. H., Brown, K. A., Carvalho, F., Johnson, S. E., Wright, P. C., and Dunham, A. E. (2018). Edge effects on components of diversity and above-ground biomass in a tropical rainforest. J. Appl. Ecol. 55, 977–985. doi: 10.1111/1365-2664.12985
Razafindratsima, O. H., Jones, T. A., and Dunham, A. E. (2014). Patterns of movement and seed dispersal by three lemur species. Am. J. Primatol. 76, 84–96. doi: 10.1002/ajp.22199
Razafindratsima, O. H., and Martinez, B. T. (2012). Seed dispersal by red-ruffed lemurs: seed size, viability, and beneficial effect on seedling growth. Ecotropica 18, 15–26.
Richard, A. F., and Dewar, R. E. (1991). Lemur ecology. Annu. Rev. Ecol. Syst. 22, 145–175. doi: 10.1146/annurev.es.22.110191.001045
Roig, J. Y., Tucker, R. D., Delor, C., Peters, S. G., and Théveniaut, H. (2012). Carte Géologique de la République de Madagascar à 1/1,000,000. Antananarivo, République de Madagascar: Ministère des Mines, Programme de Gouvernance des Ressources Minérales.
Schwartz, G. T., Samonds, K. E., Godfrey, L. R., Jungers, W. L., and Simons, E. L. (2002). Dental microstructure and life history in subfossil Malagasy lemurs. Proc. Natl. Acad. Sci. U.S.A. 99, 6124–6129. doi: 10.1073/pnas.092685099
Scott, J. R., Godfrey, L. R., Jungers, W. L., Scott, R. S., Simons, E. L., Teaford, M. F., et al. (2009). Dental microwear texture analysis of two families of subfossil lemurs from Madagascar. J. Hum. Evol. 54, 405–416. doi: 10.1016/j.jhevol.2008.11.003
Shapiro, L. J., Seiffert, C. V., Godfrey, L. R., Jungers, W. L., Simons, E. L., and Randria, G. F. (2005). Morphometric analysis of lumbar vertebrae in extinct Malagasy strepsirrhines. Am. J. Phys. Anthropol. 128, 823–839. doi: 10.1002/ajpa.20122
Smith, R. J., and Jungers, W. L. (1997). Body mass in comparative primatology. J. Hum. Evol. 32, 523–559. doi: 10.1006/jhev.1996.0122
Spoor, F., Garland, T., Krovitz, G., Ryan, T. M., Silcox, M. T., and Walker, A. (2007). The primate semicircular canal system and locomotion. Proc. Natl. Acad. Sci. U.S.A. 104, 10808–10812. doi: 10.1073/pnas.0704250104
Tattersall, I. (1973). Cranial anatomy of the Archaeolemurinae (Lemuroidea, Primates). Anthropol. Papers Am. Mus. Nat. Hist. 52, 1–110.
Thalmann, U. (2006). “Behavioral and ecological adaptations in two small folivorous lemurs with different social organization: Avahi and Lepilemur,” in Lemurs: Ecology and Adaptation, eds L. Gould and M. L. Sauther (Boston, MA: Springer), 327–352. doi: 10.1007/978-0-387-34586-4_15
Walker, A., Ryan, T. M., Silcox, M. T., Simons, E. L., and Spoor, F. (2008). The semicircular canal system and locomotion: the case of extinct lemuroids and lorisoids. Evol. Anthropol. 17, 135–145. doi: 10.1002/evan.20165
Walker, A. C. (1974). “Locomotor adaptations in past and present prosimian primates,” in Primate Locomotion, ed F.A. Jenkins Jr. (New York, NY; London: Academic Press), 349–381. doi: 10.1016/B978-0-12-384050-9.50016-7
Warren, R. D. (1997). Habitat use and support preference of two free-ranging saltatory lemurs (Lepilemur edwardsi and Avahi occidentalis). J. Zool. 241, 325–341. doi: 10.1111/j.1469-7998.1997.tb01963.x
Wright, P. C. (1999). Lemur traits and Madagascar ecology: coping with an island environment. Yearb. Phys. Anthropol. 42, 31–72.
Wright, P. C., Razafindratsita, V. R., Pochron, S. T., and Jernvall, J. (2005). “The key to Madagascar frugivores,” in Tropical Fruits and Frugivores, eds J. L. Dew and J. P. Boubli (New York, NY: Springer).
Keywords: 87Sr/86Sr, mobility, energy conservation, seed dispersal, Madagascar
Citation: Crowley BE and Godfrey LR (2019) Strontium Isotopes Support Small Home Ranges for Extinct Lemurs. Front. Ecol. Evol. 7:490. doi: 10.3389/fevo.2019.00490
Received: 19 September 2019; Accepted: 03 December 2019;
Published: 20 December 2019.
Edited by:
Pasquale Raia, University of Naples Federico II, ItalyReviewed by:
Marina Melchionna, University of Naples Federico II, ItalyCopyright © 2019 Crowley and Godfrey. This is an open-access article distributed under the terms of the Creative Commons Attribution License (CC BY). The use, distribution or reproduction in other forums is permitted, provided the original author(s) and the copyright owner(s) are credited and that the original publication in this journal is cited, in accordance with accepted academic practice. No use, distribution or reproduction is permitted which does not comply with these terms.
*Correspondence: Brooke Erin Crowley, YnJvb2tlLmNyb3dsZXlAdWMuZWR1
Disclaimer: All claims expressed in this article are solely those of the authors and do not necessarily represent those of their affiliated organizations, or those of the publisher, the editors and the reviewers. Any product that may be evaluated in this article or claim that may be made by its manufacturer is not guaranteed or endorsed by the publisher.
Research integrity at Frontiers
Learn more about the work of our research integrity team to safeguard the quality of each article we publish.