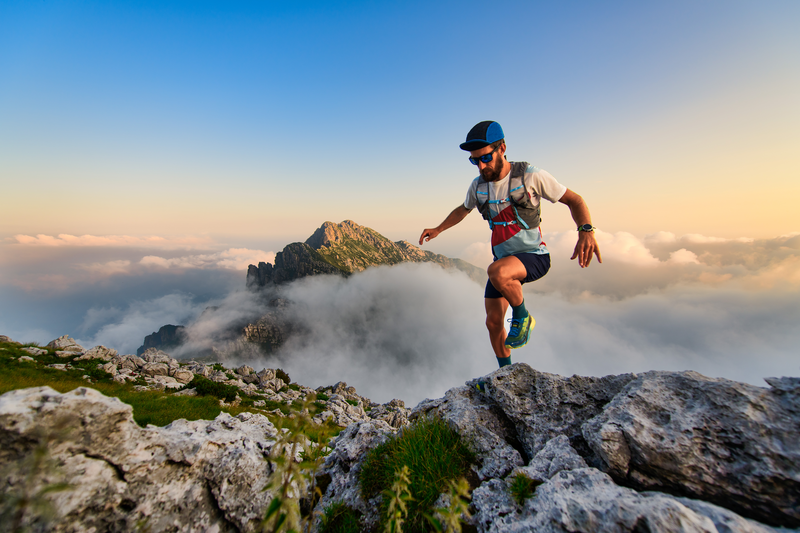
94% of researchers rate our articles as excellent or good
Learn more about the work of our research integrity team to safeguard the quality of each article we publish.
Find out more
REVIEW article
Front. Ecol. Evol. , 10 January 2020
Sec. Behavioral and Evolutionary Ecology
Volume 7 - 2019 | https://doi.org/10.3389/fevo.2019.00472
This article is part of the Research Topic What’s Love Got to Do with it: The Evolution of Monogamy View all 17 articles
Behavioral ecologists, evolutionary biologists, and anthropologists have been long fascinated by the existence of “monogamy” in the animal kingdom. Multiple studies have explored the factors underlying its evolution and maintenance, sometimes with contradicting and contentious conclusions. These studies have been plagued by a persistent use of fuzzy terminology that often leads to researchers comparing “apples with oranges” (e.g., comparing a grouping pattern or social organization with a sexual or genetic mating system). In this review, we provide an overview of research on “monogamy” in mammals generally and primates in particular, and we discuss a number of problems that complicate comparative attempts to understand this issue. We first highlight why the muddled terminology has hindered our understanding of both a rare social organization and a rare mating system. Then, following a short overview of the main hypotheses explaining the evolution of pair-living and sexual monogamy, we critically discuss various claims about the principal drivers of “monogamy” that have been made in several recent comparative studies. We stress the importance of using only high quality and comparable data. We then propose that a productive way to frame and dissect the different components of pair-living and sexual or genetic monogamy is by considering the behavioral and evolutionary implications of those components from the perspectives of all participants in a species' social system. In particular, we highlight the importance of integrating the perspective of “floater” individuals and considering their impacts on local operational sex ratios, competition, and variance in reproductive success across a population. We stress that pair-living need not imply a reduced importance of intra-sexual mate competition, a situation that may have implications for the sexual selection potential that have not yet been fully explored. Finally, we note that there is no reason to assume that different taxa and lineages, even within the same radiation, should follow the same pathway to or share a unifying evolutionary explanation for “monogamy”. The study of the evolution of pair-living, sexual monogamy, and genetic monogamy remains a challenging and exciting area of research.
For decades, behavioral ecologists, evolutionary biologists, and anthropologists have been fascinated by the existence of “monogamy” in the animal kingdom. Thus, a large literature exists exploring the factors underlying its evolution and maintenance (Kleiman, 1977; Trumbo and Eggert, 1994; Kappeler and van Schaik, 2002; Brotherton and Komers, 2003; Møller, 2003; Reichard, 2003a; Kokko and Morrell, 2005; Lukas and Clutton-Brock, 2013; Opie et al., 2013a; Díaz-Muñoz and Bales, 2016; Tecot et al., 2016; French et al., 2018; Klug, 2018; Macdonald et al., 2019). In many animal taxa, the amount of time and energy invested in offspring is conspicuously sex-biased, with females often investing more in each reproductive opportunity than males. This is especially true among mammals, where internal fertilization, gestation, and lactation, almost de facto, demand greater investment by females. Under these circumstances, it is often assumed that males might improve their fitness by increasing their number of mates, whereas female fitness is presumed to be tied less directly to the number of partners they mate with and more directly to access to the resources needed to sustain pregnancy and early infant development (Bateman, 1948; Trivers, 1972; Clutton-Brock, 2007; Clutton-Brock and Huchard, 2013). As a result, “monogamy” (or more specifically, “monogyny,” where an individual male's mating or breeding is restricted, over some time, to a single female) is seen as a paradox in need of explanation (Klug, 2018). Thus, the question is often reduced to when and why males should “settle” for defending access to and mating with only one female when, all else being equal, their reproductive output could be improved by pursuing polygynous mating. Less often discussed, though equally important, is to consider the female perspective; under what conditions is it in a female's reproductive interest to pursue “monandry” instead of “polyandry?” These observations have motivated a number of comparative analyses (Komers and Brotherton, 1997; Brotherton and Komers, 2003; van Schaik and Kappeler, 2003; Cohas and Allainé, 2009; Shultz et al., 2011; Lukas and Clutton-Brock, 2013; Opie et al., 2013a; Huck et al., 2014); the results have generated no small amount of controversy (e.g., de Waal and Gavrilets, 2013; Dixson, 2013; Lukas and Clutton-Brock, 2014).
In this review, we provide an overview of research on the expression and evolution of “monogamy” in mammals in general and primates in particular, and we highlight a number of problems that complicate comparative attempts to understand this issue. We focus on non-human primates, the set of mammals we know best, but refer to other taxa where relevant. We start with a brief, but necessary, discourse about the muddled terminology used and the problems that arise from not paying enough attention to defining terms precisely (section 2.1) (Reichard, 2003a, 2017; Tecot et al., 2016), and we suggest a set of preferred terms which we then use throughout the rest of the manuscript (section 2.2). We follow with a short review of the major hypotheses that have been put forward (section 3) (see also Klug, 2018; Macdonald et al., 2019). Together, sections 2 and 3 provide the needed backdrop for section 4.
In section 4, we critically evaluate several recent comparative studies that have focused on investigating some of the proposed explanations for “monogamy”. We first summarize the general conclusions of these studies, concentrating on two that have arguably received the most attention (section 4.1). We then consider how well the data we have collected over the course of our own 25+ years of collective fieldwork on four different “monogamous” primates support (or not!) the assumptions made and the conclusions reached (section 4.2). Because of the multiple concerns we raise with these studies, we close the section with a set of guiding principles that, we argue, should be adhered to in comparative studies of animal social systems (section 4.3). The last of those principles calls for considering the behavioral and evolutionary implications of particular components of a taxon's social system from the perspectives of all involved participants, not just certain classes of individuals. We devote section 5 to discussing these individual perspectives in detail. We highlight the need to consider how the interests of non-reproductive group members and “floater” animals (i.e., individuals who range unassociated with other reproductively mature individuals) change the range of theoretical evolutionary scenarios. We conclude with a few suggestions for future research directions (section 6) formulated in light of the critiques of recent comparative studies we raise and the questions that arise from explicitly considering the perspectives of all classes of individuals.
Nearly all reviews of the evolution of “monogamy” in mammals state, in one way or another, that while ~90% of bird species are classified as “monogamous” (usually citing Lack, 1968), less than 10% of mammalian species are (usually citing Kleiman, 1977). These reviews almost uniformly point out that “monogamy” is relatively more common among primates than among other mammals (e.g., 30 vs. 5% of species: Lukas and Clutton-Brock, 2013; Opie et al., 2013a). We contend that statements like these—offered at the beginning of review papers as foundational ideas upon which subsequent analyses are developed—are fundamentally problematic because comparative analyses are in danger of “comparing apples with oranges” if they do not pay careful attention to terminology (Dixson, 2013). Precise definitions matter because researchers frequently use similar terms to refer to different social situations (Table 1). This is partly related to different usage conventions for researchers working with different groups of organisms. For example, because the majority of avian taxa are pair-living, birds are often classified in broad-scale comparative analyses as “socially monogamous,” even though it has long been recognized that true “genetic monogamy” occurs in only 14% of passerine birds (Griffith et al., 2002). By contrast, many of the mammalian taxa that are classified as “socially monogamous” in reviews and comparative analyses (e.g., Lukas and Clutton-Brock, 2013; Opie et al., 2013a; Lambert et al., 2018; Macdonald et al., 2019) are decidedly not pair-living; in fact, they often live in groups containing more than one reproductive age individual of one or both sexes (e.g., African wild dogs, Lycaon pictus; meerkats Suricata suricatta; marmosets, genera Callithrix and Mico; tamarins, genera Saguinus and Leontocebus). At the same time, given that they show relatively high rates of reproductive monopolization, their mating system is nonetheless called “monogamous” (Lukas and Clutton-Brock, 2013). Classifying both of these situations as “social monogamy” in a comparative study is problematic because the terms are being used differently for birds and mammals—apples and oranges! Additionally, in some other taxonomic groups (e.g., certain non-social insects), the term “monogamy” is used as a shorthand that applies to only the female's mating perspective to mean sexual monandry, without specifying whether males also mate with a single female (sexual monogyny) or several ones (sexual polygyny) (Bybee et al., 2005; Ivy and Sakaluk, 2005).
Table 1. Some definitions of “monogamy” and “social monogamy” used in prior studies and reviews of primates and other animals.
As we have surely telegraphed by bracketing the word with quotation marks, it is our opinion that much of the confusion and frustration surrounding comparative analyses of “monogamy” is engendered by loose phrasing, a lack of precision and consistency across studies about the phenomena being described or explained (Carter and Perkeybile, 2018), and folk conceptions about what the term implies. Definitions must be made explicit, and primary data should be reviewed, clarified, and cast into consistent terms before they are used (e.g., Borries et al., 2014; Valomy et al., 2015; see also Schradin, 2017). Most obviously, and as others have also noted, uncritical compilations of comparative data risk conflating different ideas about the term “monogamy”. For birds (and often primates), the term is typically used to describe a particular social living situation where there is only one adult of each sex in a group, while mammalogists more commonly use the term to refer to either a mating pattern where a single male and female each have one another as their sole sexual partner, or a breeding pattern, where a single female and male exclusively produce offspring with each other (Tecot et al., 2016; Klug, 2018; Lambert et al., 2018).
The implication of mating or breeding “exclusivity” comes with its own fuzziness. What does it mean for a mating relationship to be exclusive? If an adult mates 90% of the time with one particular opposite-sex individual, is that relationship exclusive? What about 80%? What if a female mates 100% of the time with one male when she is in her periovulatory period, but mates with others outside of that period? What about the converse, where she mates 100% of the time with one male outside of the time when she is most likely to conceive, but has a different partner (or more than one) during the narrow window around ovulation? It is likewise unclear what time frame researchers consider important for operationalizing mating “exclusivity”. Is it over one reproductive event, or several? If litter or clutch size is large, or if the taxon under consideration reproduces only once or a small number of times in a lifetime, then researchers might be willing to classify such a taxon as “monogamous” if females are not seen to mate with more than one male during such single conceptive period or if all offspring are sired by the same male. However, that may be an unsatisfying definition for researchers studying taxa like primates that are long-lived, typically give birth to singleton offspring, and have male-female associations that persist over multiple reproductive events.
Folk conceptions of “monogamy” also often presume that a suite of other behavioral traits tends to co-occur with an exclusive mating relationship, including co-ranging and coordination of activity by a male-female pair, some form of bonding between pair-mates, joint range defense, same-sex intolerance, and cooperative offspring care. While some researchers have conscientiously (and appropriately!) acknowledged that these traits are not necessarily all seen in all taxa that are considered “monogamous” (Wickler and Seibt, 1983; Fuentes, 1998, 2002; Tecot et al., 2016; French et al., 2018), the folk conception persists, as exemplified in the Wikipedia entry on “Monogamy in Animals”, which states that, “Monogamous pairing in animals refers to the natural history of mating systems in which species pair bond to raise offspring. This is associated, usually implicitly, with sexual monogamy” (Wikipedia, 2019).
Finally, even when we recognize that certain comparative studies (e.g., Lukas and Clutton-Brock, 2013) may be concerned primarily with the sexual or genetic mating system and others (e.g., Opie et al., 2013a) with the particular grouping pattern of pair-living, the confusing terminology is problematic, and even circular, for other analyses. For example, some studies aim to compare the degree of extra-pair paternity (EPP) in group-living “monogamous” and “non-monogamous” species (Cohas and Allainé, 2009; Lambert et al., 2018). But if “monogamy” is defined by the degree of paternity monopolization, then EPPs are, by definition, low and should therefore not be used in an analysis that tries to establish whether monogamous or non-monogamous taxa have higher rates of EPPs! One study that explicitly looked at rates of extra-group paternity in pair-living taxa found that genetic studies on pair-living mammals are still astonishingly rare, and only five of the 15 species for which data were available at the time of the study indeed showed no evidence of EPPs (Huck et al., 2014). Moreover, for most of these taxa, data on rates of extra-pair mating (which requires observation, not simply analysis of parentage) are scant or unavailable, so the extent to which, in any of these taxa, mating is restricted to within a pair is unknown.
To summarize, most researchers are likely aware that there are various definitions of “monogamy” and that—depending on the taxa being studied—a veritable menu of different behavioral traits may be considered as part of the definition. The problem is that the terms “pair-living”, “pair-bonded”, “social monogamy”, and “monogamy” are nonetheless seldom distinguished properly, even in the scientific literature, and are often used interchangeably. In no way are we ourselves exempt from being guilty of using this terminological shorthand in less than a consistent manner (e.g., Di Fiore et al., 2007; Fernandez-Duque et al., 2008; Huck et al., 2014; Fernandez-Duque, 2016).
There have been several productive attempts to clearly define relevant terms (Wickler and Seibt, 1983; Tecot et al., 2016; Reichard, 2017; French et al., 2018; Table 1). Below, we follow the lead of Kappeler and van Schaik (2002) who provide some standard terminology that has influenced discussions about primate social systems. Under their framework, the social organization (i.e., who lives with whom) of pair-living refers to situations where a single adult male and a single adult female form a two-adult group without the additional presence of other adults (Table 2). To avoid confusion, we concur that this social organization should be referred to as “pair-living” and should not be called “social monogamy”, as is often done in bird and some mammal studies. That is, the word monogamy should only be used in reference to animals' mating and reproductive behavior, not simply their grouping pattern. Kappeler and van Schaik (2002) also make a distinction between the “social mating system” (who mates with whom), which relates to individual behavior and behavioral choices, and the “genetic mating system” (who has offspring with whom), which relates to realized reproduction. Thus, if one male and one female have an exclusive mating relationship (irrespective of the grouping pattern, or social organization), then the social mating system is monogamous. Some researchers (e.g., Reichard, 2003a, 2017; French et al., 2018) have referred to this situation as sexual monogamy, and we encourage the use of this accurate and useful term for describing such a social mating system (Table 2). As alluded to above, what is considered “exclusive”, as well as the critical time frame over which to consider “exclusivity” still needs to be defined and may depend on the taxon of interest. If a single male and a single female have offspring exclusively with each other (irrespective of whether one or both mate with other individuals, whether they associate in the same social group, whether they belong to different social groups, or whether one or both range solitarily), then the genetic mating system can be considered monogamous and the term genetic monogamy is appropriate. If a species is sexually monogamous it will, necessarily, also be genetically monogamous (because if a female mates with only one male, and the male mates with only one female, there can be no extra-pair offspring), although the reverse is not necessarily true. As for sexual monogamy, the time frame, over which genetic monogamy is determined needs to be considered carefully, depending on the mean litter size and the lifetime number of reproductive events per individual for each species.
An additional layer of complexity relates to the degree of spatiotemporal association between male and female pair partners, an aspect of their social organization. Most pair-living mammals share a territory, spend a large proportion of their time together and have an affiliative relationship that, when properly characterized by behavioral, emotional, and endocrinological characteristics (Hinde et al., 2016; Maninger et al., 2017; Carter and Perkeybile, 2018), can be defined as a pair-bond. In contrast, in some species pair-mates do not manifest such close connections, and these dyads are sometimes referred to as “dispersed pairs” in contrast to “associated pairs” (e.g., fork-marked lemurs, Phaner furcifer: Schülke and Kappeler, 2003).
Species like fork-marked lemurs are perceived as odd, because, as mentioned above, folk conceptions about “monogamy”—and much of the usage of the term in the scientific literature—additionally incorporate ideas and concepts about the nature of the social relationship between pair-mates (e.g., the presence of “pair bonds”) and about the level of offspring care provided by each of the pair-partners (e.g., “biparental care”) or by older siblings or additional reproductive age adults (e.g., “cooperative breeding”). But, clearly, there can be pair-living animals that are not “pair-bonded” (e.g., fork-marked lemurs: Schülke and Kappeler, 2003), males and females can be sexually monogamous without both sexes contributing to offspring care (e.g., Kirk's dik-dik, Madoqua kirki: Brotherton et al., 1997), and males can contribute substantially to offspring care even when the mating system is sexually polygynous or polyandrous (e.g., mustached tamarins, Saguinus mystax: Huck et al., 2005), to name just a few situations that would require careful consideration of definitions. Thus, for comparative studies of pair-living, sexual, or genetic monogamy, it is critical for researchers to also characterize the social relationship between pair-mates, which is an element of a species' social structure (Kappeler and van Schaik, 2002), and to document the level of care or investment provided by each pair-mate and by other non-reproductive adults, which are elements of the species' care system (Kappeler et al., 2019). In the remaining sections of this review, we try to translate, as needed, the terminology used in the various studies we discuss so that it conforms to the definitions provided in Table 2. However, in some cases we deliberately continue using, in quotation marks, the fuzzy terms “monogamy” and “social monogamy” to highlight both that these were terms the authors originally used and the associated ambiguity about their precise meaning.
Our renewed call for clear terminology notwithstanding, we should remain aware that characterizing any of these components for a given taxon often involves assigning one of a set of discrete categories to traits that fall on a continuous dimension. For example, there were no EPPs detected in 35 young of Azara's owl monkeys (Aotus azarae: Huck et al., 2014), extra-pair copulations (EPCs) resulted in 4% of the young of Malagasy giant jumping rats (Hypogeomes antimena: Sommer, 2003), and 7 and 23% of meerkat pups were born by a subordinate female and subordinate male, respectively (Griffin et al., 2003). While it may be straightforward to decide, on the basis of these results, to classify owl monkeys as genetically monogamous, decisions for the other species will be more arbitrary. Should jumping rats also be classified as genetically monogamous, despite the occasional offspring from EPCs? What about meerkats, where <80% of offspring are progeny of the main breeding pair? Ideally, if paternity data were available for more species, the proportion of EPPs would be used as a continuous variable in analyses. Still, given the paucity of data (Huck et al., 2014; Lambert et al., 2018), it may not be feasible to assign continuous values to this trait, and some authors have even argued that it might be preferable to use distinct categories for social systems (Rubenstein et al., 2016), although we disagree with that position for reasons akin to those discussed in the section 4.2. In any case, authors must make explicit the definitions and criteria they use for assigning a taxon to one category or another, with explicit reference to the primary literature where the original data were published (Borries et al., 2016). In species where there is a clear modal pattern, it might be acceptable to use the modal social organization or mating system, but for some taxa the range of variation may be so large that it warrants clearly classifying them as “variable” or polymorphic.
Among primates, the marmosets and tamarins (subfamily Callitrichinae) offer an excellent example to illustrate the problems that follow from inadequate consideration of terminology. These small Neotropical primates, particularly the tamarins (Saguinus, Leontocebus) and lion tamarins (Leontopithecus), have variable social organizations and mating systems. Most of the species in these genera are not pair-living, but rather live in groups that can contain up to four adults of each sex. The variation includes the remarkably uncommon mating pattern among mammals of polyandry, where a single female (even when there are other females in the group) mates with all adult males in the group who are not related to her (Heymann, 2000; Garber et al., 2016). It sometimes happens that one of the males gets the major share of paternity, even over consecutive years (Huck et al., 2005). This means that, while the social mating system is clearly sexually polyandrous, there might still be a tendency, in some groups, toward high male reproductive skew that approaches genetic monogamy. Researchers working with wild populations of marmosets and tamarins have repeatedly stated that they should not be considered “monogamous” (Savage et al., 1996; Heymann, 2000; Díaz-Muñoz, 2011; Garber et al., 2016), and yet in recent comparative analyses, they are either expressly classified or casually referred to as such (Lukas and Clutton-Brock, 2013; Opie et al., 2013a; Lambert et al., 2018).
In a nutshell, we have argued and illustrated with a few examples that using unclear terminology can lead to ambiguity in the data upon which comparative analyses rest, which in turn can make us question the results or validity of these analyses. Furthermore, specific proposed evolutionary drivers that might be relevant for the evolution of certain aspects of social organization may not be relevant for understanding the evolution of the mating system; predictions or conclusions might differ when these components of the social system are considered separately. Tecot et al. (2016) developed specific, separate predictions for the evolution of pair-living, sexual monogamy, and pair-bonding derived from the major hypotheses for “monogamy” reviewed below; the authors pointed out that not all of these hypotheses are relevant for explaining each of these aspects of the social system. These concerns are particularly true for comparative studies that use large datasets for phylogenetic or meta-analyses, where data from many different studies by many different authors are integrated.
There are seven drivers historically and regularly considered in discussions about the evolution of either pair-living or sexual monogamy (see also Tecot et al., 2016; Klug, 2018; Fernandez-Duque et al., in review) (1) The ‘infant care’ hypothesis states that the need for biparental, or communal, care of costly offspring is presumed to force males to stay with a single mate and her offspring (Kleiman, 1977; Huck and Fernandez-Duque, 2013; Rogers et al., 2018; Schacht et al., 2018; Macdonald et al., 2019). This hypothesis assumes that male care is required for the successful raising of offspring. Selective pressures encourage solitary individuals to associate with the mating partner for the period of infant raising. Once bi-parental care is established, the hypothesis also proposes pressures for sexual monogamy, if the costs of parenting are high (Tecot et al., 2016). (2) The ‘female dispersion’ hypothesis states that female scarcity, where females also do not aggregate with one another, leads to an inability of males to monopolize spatial access to more than one female (Emlen and Oring, 1977; Shuster and Wade, 2003; Schacht and Bell, 2016). Under this hypothesis, it is assumed that resources that females rely on are scarce, low quality, or highly dispersed, such that a male would not be able to defend the range of more than one female. Note that this hypothesis relates to the social organization rather than the sexual mating system or pair-bonding. (3) The ‘mate guarding’ hypothesis states that the benefits to males of guarding one female to obtain exclusive mating access outweigh the benefits of seeking additional matings with other females (Emlen and Oring, 1977; Komers and Brotherton, 1997; Fernandez-Duque, 2016). While this hypothesis appears similar to the ‘female dispersion’ hypothesis, it predicts that males will not attempt to mate with more females, even if they are able to cover more than one home-range. It also predicts that, within a population, females will show some reproductive synchronicity, or seasonality, that limit males' opportunities for extra-pair matings, and that both males and females will show sex-specific aggression toward same-sex intruders and that such aggression will be more pronounced in the breeding season. In contrast to the ‘female dispersion’ hypothesis, the ‘mate guarding’ hypothesis relates to both the social organization and the sexual mating system (see “Mate defense” hypothesis of Tecot et al., 2016). (4) The ‘infanticide prevention’ hypothesis (van Schaik and Kappeler, 2003) states that the pressure for females to associate with a male who can protect her, and her offspring, from aggressive and potentially infanticidal conspecifics, leads to the formation of groups consisting of male-female pairs and to sexual monogamy. The hypothesis assumes that females losing their offspring become receptive sooner than if they continue nursing current offspring. It also assumes that a female's partner is the likely sire of her offspring and that the presence of a male increases both his and the female's reproductive success by reducing the success rate of potentially infanticidal intruders. While this hypothesis proposes a selective pressure acting on the social organization, the degree of pair-bonding and the sexual mating system (Tecot et al., 2016), it more strongly relates to pair-living than to sexual monogamy in otherwise group-living species, since one of the assumed counter-strategies of females against infanticide is to mate with multiple males (e.g., Chakrabarti and Jhala, 2019). (5) The ‘predation prevention’ hypothesis states that the pressure of predation leads to a male associating with a single female if their joint permanent presence helps to protect their offspring from predators. The hypothesis assumes that males (or male-female pairs) can deter predators more effectively than females can on their own. This hypothesis is related to the social organization; it does not explain pressures on the sexual mating system. (6) The ‘resource defense’ hypothesis states that pair-living has a selective advantage due to the combined defense of resources year-round. If resources are of low quality, or sparsely distributed, this will generate intra-specific competition that leads to a situation where an area cannot support larger groups. This hypothesis states that resource defense is directed by both males and females against intruders of both sexes, unless males, and females differ greatly in their requirements of specific resources. Under this scenario, pair-bonding is favored because it enhances coordinated territory defense (Rutberg, 1983; Tecot et al., 2016). This hypothesis does not address the social mating system (i.e., the issue of sexual monogamy). (7) Lastly, according to the ‘optimal group size’ hypothesis, pair-living is favored if the costs and benefits of group living in general [not just for defending resources, as in Hypothesis (6)] lead to an optimal group size of only two adults (plus a few offspring). The hypothesis does not presume a link between pair-living and sexual monogamy, because it only refers to the social organization, nor does it presume that two adult group members will be pair-bonded (Tecot et al., 2016). The hypothesis does suggest that changes in predation pressure or resource availability may shift the social organization toward, or away from, an optimal adult group size of two animals. In certain taxa, or under certain conditions, some of these hypotheses (e.g., the ‘mate guarding’ hypothesis) might be sex-reversed. For example, if males are a scarce resource for parental care, females may benefit by guarding a single male from other females.
Over the past few decades, a number of influential studies have investigated one or more of the hypotheses outlined above using broad, comparative data, either for mammals or for particular mammalian groups. For example, two early comparative studies of ungulate mating systems concluded that female dispersion, per se, does not provide a general explanation for the evolution of “monogamy” in mammals (Komers and Brotherton, 1997; Brotherton and Komers, 2003). Rather, the authors concluded that it is the necessity and feasibility of guarding independently ranging females (whose home ranges might nonetheless overlap) that promote its evolution. They also proposed that paternal care has more likely evolved as a consequence of a “monogamous” mating system, and not as its cause.
Another early comparative study of the evolution of “monogamy” focused on primates and used parsimony-based phylogenetic comparative methods to reconstruct the ancestral states from which the social organization of pair-living may have arisen (van Schaik and Kappeler, 2003; see also Kappeler, 2014). The authors suggested that it is important to distinguish two different types of pair-living in primates: “associated” pairs (where pair partners show coordinated activity and spend substantial amounts of time in spatial proximity to one another) and “dispersed pairs” (where pair partners share a home-range, but show little coordination of their activity and do not regularly rest together). Based on presumed phylogenetic relationships and the distribution of behavioral patterns across extant taxa, the study concluded that these two types of pair-living arose from different ancestral social organizations, with associated pairs evolving from ancestors where females lived in mixed-sex groups, and dispersed pairs (which are seen only among strepsirrhine primates) arising from ancestors where females maintained solitary ranges. Thus, for example, pair-living in (almost) genetically monogamous indris (Indri indri: Bonadonna et al., 2019) is proposed to have evolved from a social organization such as that exhibited by ring-tailed lemurs (Lemur catta) or Verreaux's sifakas (Propithecus verreauxi), while co-sleeping in fat-tailed dwarf-lemurs (Cheirogaleus medius) would have evolved from a solitary social organization, such as that exhibited by mouse lemur (Microcebus: Kappeler, 2014). In a more recent study, Shultz et al. (2011) compared four competing models for the evolution of social organization across all primates and concluded that pair-living in all primates—including in those strepsirrhines living in dispersed pairs—evolved from ancestors living in multimale-multifemale social groups rather than from ancestral taxa where females were solitary.
More recently, two ambitious analyses have explored the evolutionary history of “monogamy” among mammals in general (Lukas and Clutton-Brock, 2013) and among primates in particular (Opie et al., 2013a), using comparative phylogenetic methods (Lukas and Clutton-Brock, 2013; Opie et al., 2013a). In the following, we focus mainly on these two studies because they are highly cited, reached somewhat contrasting conclusions, and refer explicitly, for some aspects of the analyses, to primates, the group of mammals on which our own work is based. Both studies analyzed the correlated evolution between “social monogamy” (as both research groups referred to it) and some of its candidate drivers (e.g., the need for male care, female grouping and ranging patterns, and the risk of infanticide). The data used for each of these studies were extracted, primarily, from previously published reviews or encyclopedias, supplemented with additional information compiled by the authors from primary sources, as explained in the supplementary materials. Lukas and Clutton-Brock (2013) classified ~2,545 mammalian species “for which information was available” as either solitary, “socially monogamous”, or group-living, and as showing male care or not. Importantly, their category of “socially monogamous” included many taxa that are pair-living, as well as several taxa that are group-living, but where breeding is largely or exclusively restricted to a single male-female pair. Thus, their study was concerned with the evolution of sexual monogamy rather than the social organization. They likewise compiled data on several ecological and life history variables (e.g., gestation and lactation length, home-range size and overlap) for each taxon. Opie et al. (2013a) compiled a similar dataset on ~230 primate species, scoring each species' mating system as “polygynous”, “polyandrous”, “monogamous”, or some combination thereof. However, despite using these terms that describe mating patterns in their supplementary table, the definition of “monogamy” that Opie et al. (2013a) used in their text (Table 1) suggests that they are referring to the social organization of pair-living rather than to the mating system of sexual monogamy. They also characterized, for each species, whether male care is present or absent and whether female ranges are discrete or overlapping, and they calculated an index of theoretical “infanticide risk” based on how quickly a female who loses an infant may be expected to return to breeding. Both studies then used phylogenetically explicit comparative methods to reconstruct inferred trait conditions at ancestral nodes in the phylogeny and examined whether and how transitions to “social monogamy” (i.e., pair-living in the case of Opie et al., 2013a, and sexual monogamy in Lukas and Clutton-Brock, 2013) were associated with particular female grouping patterns, with patterns of male care, and with changes in infanticide risk.
These two analyses show some consensus with respect to the timing of the evolution of the character state of “social monogamy” in relation to the character state of male care. Transitions to pair-living (in the study by Opie et al., 2013a) and sexual monogamy (in the study by Lukas and Clutton-Brock, 2013), typically preceded or co-occurred with, rather than followed, an increase in male involvement in infant care. Once intense male care evolved, the likelihood of lower rates of extra-pair paternity increased, potentially leading to more strict genetic monogamy. This evolutionary sequence may then contribute to the persistence of sexual monogamy once it arises (Lukas and Clutton-Brock, 2013). The two studies, however, reached rather different conclusions with regard to the ultimate causes of the evolution of “monogamy”. Lukas and Clutton-Brock (2013) concluded that “social monogamy” (i.e., sexual monogamy) evolves most commonly when females are solitary and when males are unable to defend reproductive access to more than one female. They additionally concluded that the evolution of sexual monogamy does not seem to be associated with a high risk of infanticide by males. Opie et al. (2013a), by contrast, claim that “social monogamy” (i.e., pair-living), at least among primates, evolved in response to increased infanticide risk; indeed, they emphasize that position in the title of their article: “Male infanticide leads to social monogamy in primates.”
While the studies reviewed above have indeed contributed to a broader discussion of mating system variation in mammals and have been both influential and widely cited, many of them suffer from some of the concerns that we are raising here, including the problem of terminology discussed in section 2, as well as others we highlight below. For example, the definition of “monogamy” used by Komers and Brotherton (1997) and Brotherton and Komers (2003) is not clear; the illustrations that accompany the analyses seem to point to pair-living, but the species included in their analyses are often group-living, raising the possibility that the authors actually refer to sexual monogamy instead (Table 1). Likewise, the studies by Lukas and Clutton-Brock (2013) and Opie et al. (2013a) utilized rather different definitions for “social monogamy” (Table 1), which complicates direct comparison of their results. Additionally, all studies have relied heavily on data compiled from the secondary literature and from review articles, which raises concerns about both data quality and comparability. Not surprisingly, there has been controversy and critique by other researchers who have questioned some of the conclusions and methods of analysis used in these studies (e.g., de Waal and Gavrilets, 2013; Dixson, 2013; Opie et al., 2013b, 2014; Lukas and Clutton-Brock, 2014).
We would like to stress that we are fervent supporters of using comparative phylogenetic analyses for examining social system evolution, one of us having published one of the first such studies on primates (Di Fiore and Rendall, 1994). But we are skeptical about the value of some published conclusions about the evolution and maintenance of “monogamy”, given our concerns about the process by which the data have been compiled, analyzed, and findings interpreted. We are not alone in trying to highlight the fact that comparative analyses are only as good as the data and measurements they are based upon (Gittleman, 1989; Smith and Jungers, 1997; Houle et al., 2011; Patterson et al., 2014; Sandel et al., 2016).
As field biologists who work with a suite of taxa that are characterized as “monogamous” in many of these analyses, we are of course intimately interested in the questions being considered. Thus, below we illustrate some of the problems with data used in comparative studies specifically for the four taxa of platyrrhine primates (monkeys from South and Central America) on which we have worked and published extensively (e.g., Huck et al., 2004a,b, 2007; Carrillo-Bilbao et al., 2005; Di Fiore et al., 2007; Fernandez-Duque et al., 2008, 2013; Fernandez-Duque, 2016; Spence-Aizenberg et al., 2016; Van Belle et al., 2016, 2018). The four taxa we refer to are owl monkeys (genus Aotus), western and southwestern Amazonian titi monkeys (genus Plecturocebus), tamarins (genera Leontocebus and Saguinus), and saki monkeys (genus Pithecia). All are usually classified as “monogamous” and three of them (owl monkeys, titi monkeys, and tamarins) as showing male care. We focus here on the studies by Opie et al. (2013a) and Lukas and Clutton-Brock (2013), not because we think the problems are exclusive to them, but because the central questions being examined are the ones on which we have worked for decades and for which we know data the best. Similar concerns have been raised about a host of other broad-scale comparative analyses, whether they focus on behavioral, ecological, or morphological traits (e.g., Patterson et al., 2014; Rubenstein et al., 2016; Sandel et al., 2016; Powell et al., 2017; Schradin, 2017; Tanaka et al., 2018), with some interesting follow ups as well (Lukas and Clutton-Brock, 2017; Dey et al., 2019). A more complete analysis of our data in view of recent evaluations of the factors driving the evolution of pair-living, sexual monogamy and biparental care is provided elsewhere (Fernandez-Duque et al., in review).
In addition to issues of terminology, comparative studies have been hampered by an over-reliance on secondary (and sometimes out-of-date) sources, as well as by serious questions about data quality and comparability. We tackle each of these inter-related concerns in turn below.
Judging from the references provided, the raw data used in a number of influential comparative analyses are based heavily on review articles (some of which are 20–40 years old), on encyclopedic summaries, and, partly, on studies done in captivity (e.g., Jolly, 1966; Kleiman and Malcolm, 1981; Kinzey, 1997; Komers and Brotherton, 1997; Nowak, 1999; Shultz et al., 2011; Lukas and Clutton-Brock, 2013; Opie et al., 2013a). Based on our own review of the primary literature and of the references cited in these studies, we think the choice of many of these sources is questionable. We document the extent of the problem in more detail elsewhere (Fernandez-Duque et al., in review). There we present the results of a thorough review of cited references, where we find that more than two-thirds of the references used by Opie et al. (2013a) for classifying parental care for the four taxa of owl monkeys, titi monkeys, sakis, and tamarins—and all but two of the references used by Lukas and Clutton-Brock (2013)—do not support the category assigned for the trait. It is frequent, and unfortunate, that researchers trying to compile data for hundreds or thousands of species seem to rely on previous compilations instead of the primary literature that reports the original data. It is equally problematic if editors request that authors of order-wide analyses reduce the associated reference list, as Lukas and Clutton-Brock (2017) assert happened with their submission.
We acknowledge that checking the original data is time consuming; but, when time is limited, the solution should be to consider fewer taxa or take longer to publish, not to compromise the quality of the analyses (Borries et al., 2016; Schradin, 2017). Whether the inclusion of data of unknown quality is a problem that can influence the main conclusions will depend on the amount of dubious data, and how central those data are to the analysis (Symonds, 2002). When researchers have access to a comparative dataset where all of the data have been carefully vetted, it is then possible to explore the consequences of including or not certain data through simulation and permutation. Such sensitivity analyses should be standard for any comparative analysis.
Further problems associated with data quality and the lack of clarity in how candidate traits are classified are also apparent when we look at the examination of alternative hypotheses for the evolution of pair-living or sexual monogamy. The infanticide prevention hypothesis is among the most contentious ones (Brotherton and Komers, 2003; van Schaik and Kappeler, 2003; Lukas and Clutton-Brock, 2013; Opie et al., 2013a) and offers an illustrative example to unpack. The challenges begin with identifying a measure of acceptable internal validity (Smith, 2019), given the difficulty of operationalizing the “risk of infanticide”, followed by the limited data available on infanticide across the primate order outside of a handful of well-studied species (Dixson, 2013). Because infanticide itself is difficult to directly observe and quantify (van Schaik and Janson, 2000), several broad-scale comparative studies focused instead on quantifying “infanticide risk” and then explored how changes in mating systems may have coevolved with changes in that risk across primate phylogeny (van Schaik, 2000; Lukas and Clutton-Brock, 2013; Opie et al., 2013b). These studies use as a proxy for infanticide risk the ratio between the duration of lactational amenorrhea (i.e., the period from birth to the resumption of ovulatory cycles) and the combined duration of gestation and amenorrhea. The rationale for such a proxy is that when this ratio is high (i.e., lactation is long compared to the total period of maternal investment), infanticide offers higher potential benefits to males since they can substantially reduce females' time to resumption of cycling. Since there are hardly ever adequate data to determine lactational amenorrhea, the length of the lactation period (or, rather, the age at weaning: McNeilly, 2006) is used instead. Opie et al. (2013a) operationalize infanticide risk as L/(L+G) (where L = duration of lactation and G = gestation length). While “gestation length” is a trait that can be reliably defined and accurately measured (given that it is relatively inflexible), the “duration of lactation” is much more difficult to measure, as it is challenging to define the end of weaning (Borries et al., 2014). Therefore, it is not surprising that reported “weaning” ages vary widely between different studies. For example, Goeldi's monkeys (Callimico goeldii) are reported to finish weaning at 112 days of age (Carroll, 1982), to begin weaning at 14–21 days of age but continue to suckle frequently up to 92 days (Jurke and Pryce, 1994), and to have a nursing period that lasts ca. 60–70 days (Lorenz and Heinemann, 1967). When considering this species, Opie et al. (2013a) use a number that is 2 weeks shorter than the longest reported weaning age. For cotton-top tamarins (Saguinus oedipus), while Opie et al. (2013a) use a gestation period of 168 days, a different study suggests that gestation lasts 182–197 days (Ziegler et al., 1987).
Apart from these problems with accurately characterizing the lactation period, the validity of the L/(L+G) proxy for infanticide risk is only informative for species that do not have a circumscribed birth season, have interbirth intervals (IBIs) longer than 1 year, or do not show rapid postpartum ovulation. Species with a circumscribed birth season, that give birth annually, or that can conceive again shortly after parturition, are unlikely to experience substantial risks of sexually selected infanticide, given the impossibility of speeding up female receptivity. Moreover, even if adequate data on gestation and lactation length were available for some taxa within the radiation of interest, it is unjustified to presume that data that are available for one species can be assigned to different species of the same genus or to different genera, however closely related. For example, gestation length for saddle-back tamarins, Leontocebus fuscicollis (mean female body mass = 358 g: Smith and Jungers, 1997) is 150 d (Heistermann and Hodges, 1995), while for cotton-top tamarins, S. oedipus (mean female body mass = 404 g: Smith and Jungers, 1997) it is much longer at 183 d (Oerke et al., 2003). Furthermore, it challenges extensive knowledge on the biology of reproduction to use data on weaning age from captive primates, who have consistent, predictable access to adequate food, for developing evolutionary scenarios in an ecological context. For example, weaning was completed at 19–21 months of age in wild white-headed langurs (Trachypithecus leucocephalus), while in captivity they are reported to be weaned when they are only 6 months of age (Zhao et al., 2008). We have presented elsewhere more detailed analyses of the problems associated with the misuse of gestation length and weaning age data on owl monkeys, titi monkeys and sakis for examining the infanticide prevention hypothesis (Fernandez-Duque et al., in review).
The data on seasonality used in tests of the ‘infanticide prevention’ hypothesis are likewise problematic. For example, Opie et al. (2013a) classify some owl monkey species (A. lemurinus, A. nancymaae, A. trivirgatus) as aseasonal breeders based on reports from studies of captive populations, when field data, even if very limited, suggest that, in most owl monkey species, the majority of birth occur over only a limited number of months (Fernandez-Duque, 2011). When taxa are misclassified for a test of a crucial aspect of a hypothesis, conclusions ought to be considered tentative, even if the analysis itself may be statistically sophisticated and appropriate. We suggest that researchers should start abandoning the dichotomous classification of traits (e.g., seasonal vs. aseasonal) in favor of quantifications, such as the number of weeks or months when births are observed. Even when the quantification of traits might raise new considerations (e.g., how to treat outliers), we think it will improve the quality of the comparative analyses. This change will obviously require that fine-grained natural history data be available for more taxa.
The quality and comparability of the data used in comparative studies to test the ‘female dispersion’ and ‘mate guarding’ hypotheses are likewise concerning. Examining these hypotheses requires knowledge about the spatio-temporal distribution of females, including estimates of home range size and overlap. Compiling appropriate data on these traits is challenging; methods used to determine home ranges vary widely, and, by extension, estimates of the degree of home range overlap between groups and population densities vary as well. Additionally, often no distinction is made between territories (i.e., defended areas) and home ranges (i.e., the area used by an animal for its “normal” activities such as foraging, resting, mating or caring for young: Burt, 1943), but for consideration of some of the hypotheses discussed above, this distinction is quite relevant. The choice of home range estimator, or of overlap metrics, can also profoundly influence estimated sizes and degree of overlap (Fieberg and Kochanny, 2005; Getz et al., 2007; Huck et al., 2008). The problems surrounding appropriate and comparable estimation of home range sizes are not trivial, given that socioecological theory traditionally assumes that a primary driver determining the spatial distribution of females is the distribution of food resources needed to support survival and reproduction, while the main driver of males' spatial distribution is the distribution of females (Emlen and Oring, 1977; van Schaik, 1989; Altmann, 1990; Mitani et al., 1996; Sterck et al., 1997; Schülke and Ostner, 2012).
The data on home-range sizes used in Lukas and Clutton-Brock's (2013) comparative analysis come from PanTHERIA (http://esapubs.org/archive/ecol/E090/184/default.htm), a publicly-accessible species-level database of life history, ecological, and geographical traits of mammals (Jones et al., 2009). When we compared the data on the four taxa we work with, as extracted from PanTHERIA, with our own rewiew of the primare literature, our estimated median home range sizes were approximately twice, three, and seven times larger for tamarins, owl monkeys, and titis and sakis, respectively (Fernandez-Duque et al., in review). Such a discrepancy is both striking and concerning.
Given our apprehensions about the appropriateness and quality of the data that are being used in comparative analyses, we also examined the data on home range size reported in a recent study re-evaluating the link between brain size and behavioral ecology in primates (Powell et al., 2017). The authors obtained home range (HR) size data from “dataset 1”, which they describe as a previously unpublished dataset compiled from the literature. The dataset, available online as Supplementary Information, consists of 289 rows of data, one row for each primate taxon considered. We focused on the 19 rows providing data on “HR size average” and “HR range” for different species of owl monkeys, titis, and sakis. For only nine species were data reported on average home range size, and for only eight species were data reported on the range of home range sizes.
To consider the quality of these data, we compared the values used in Powell et al.'s (2017) analyses with values we extracted ourselves from the references cited by the them (Table 3). At time of submission we were not able to check Sussman (2000), a review reference on platyrrhine primates. Of the 15 comparisons we were able to make between data reported in the comparative study and data in the cited sources, we found exact agreement (i.e., the data fully match) on only four. Two additional concerns arose about how the data included in the table were decided upon. First, we found information on four additional taxa in some of the sources cited that, for unspecified reasons, were not included in Powell et al.'s (2017) dataset. Second, even when the authors report average home range sizes, only one of the original sources cited provided an average home range size, and we could not find information on how the averages were then derived by the study's authors nor what average they computed (i.e., mean or median). To illustrate the possible problems associated with reducing variation in a presumably evolved trait, we focus on the data used from Soini (1986). This source reports home range size estimates from three different groups as 9.7, 24, and 40–42 ha, and Powell et al.'s (2017) dataset then reports an average of 24.9 ha, presumably calculated as (9.7 + 24 + 41)/3. Is there much information of biological value in such an average?
Table 3. Information on average home range size and range of home range sizes for all listed species of the genera Aotus, Callicebus, and Pithecia from Powell et al. (2017) and from our own search of the references cited therein.
With all these issues in mind, we are concerned that many contemporary considerations of hypotheses for the evolution of “monogamy” assume—maybe too readily—that these inferential analyses are solid and robust.
To summarize this section thus far, we have reviewed a number of comparative studies that have tried to test, in mammals and primates, several of the hypotheses outlined in section 3. Then, with a particular focus on a few recent, large-scale analyses, we have highlighted a number of concerns we have with respect to issues of data classification and compilation and have further examined the issue of data quality by comparing the data used in those studies with our own compilation of information from the primary literature for the four primate genera that we know best. This exercise leads us to suggest a few guiding principles around data quality for comparative analyses (see also Borries et al., 2013).
First, we cannot stress enough the importance of researchers more carefully assessing the quality and sources of the data used in their comparative analyses, including explicitly addressing the possible implications of changes in taxonomy. Second, we all need to be more careful to ensure that definitions are used consistently and are comparable between studies, or, at minimum, that we “translate” the terms applied in different studies into a common vocabulary. Third, we must be willing to rigorously exclude taxa for which no relevant field data exist, or to show that data from captive populations are representative of conditions in the wild. Similarly, data for potentially unusual and “atypical” populations should be clearly highlighted as such (e.g., island or provisioned populations). Fourth, we should strive to use only primary peer-reviewed sources for each trait and, as much as possible, not rely on just one reference per species or genus of interest. Fifth, as much as possible, for continuous data we should provide not just the mean or median value for a trait but also some measure of variation, range, number of studies included, and sample size. Moreover, if data from several studies are combined, it should be clearly stated how the “average” value was obtained. Sixth, it is essential that editors and reviewers agree to adhere to, and begin enforcing these minimal standards, to help ensure the quality of the data being used in comparative studies. We suggest that societies and journals consider requesting that authors certify that they have followed adopted standards; this could be done in the same manner that has become common practice for certifying adherence to animal ethics and data sharing guidelines. Finally, with respect to the particular topics of pair-living and sexual monogamy that we are concerned with here, we argue that researchers ought to explore their evolution considering perspectives of all of the different kinds of individuals (e.g., males, females, residents, floaters, helpers, etc.) that comprise those systems (e.g., Jennions and Petrie, 2000; Gowaty, 2004). It is to that topic that we shift our attention below.
Sexual monogamy and pair-living obviously cannot be fully explained by focusing solely on why members of one, or the other sex, might benefit from having only one opposite-sex mating partner or group mate. That is, pair-living and sexual monogamy can only be understood as consequences of the interaction of male and female mating strategies and of the compromises that interaction engenders (Gowaty, 1996; Shuster and Wade, 2003; Hosken et al., 2009). Indeed, mathematical models continue to incorporate factors influencing the variance of reproductive success in both males and females (Shuster and Wade, 2003; Kokko and Morrell, 2005; Port and Johnstone, 2013). Modeling approaches have indicated, for example, that the question of whether mate-guarding by males can be an evolutionary stable strategy (ESS) is also likely to depend, not only on the spatial distribution of females, but on the propensity of females to seek extra-pair copulations (Kokko and Morrell, 2005). In this context, the relevance of the extent of female synchrony of oestrous, which can strongly affect whether males are able to monopolize females, has not been considered in the same way as spatial dispersion in recent comparative phylogenetic analyses (but see Lambert et al., 2018). It is also important to consider the interaction between the spatial distribution of females, their relative oestrous lengths, and the daily travel distances of both males and females. For example, a breeding season of 1 month may be ample time for males, or females, to mate with multiple partners if home ranges are fairly small and close together; on the other hand, in a species with large home ranges, where females are spaced far apart, a month may not be sufficient time for individuals to mate with more than one partner.
Despite these considerations, there is still sometimes a tendency to consider quite different aspects for males and for females—namely distribution of females for males, but variation in clutch size or number of broods for females (Shuster and Wade, 2003). This may often be adequate, but not necessarily always. Additionally, while it is common to point out that not all males will reproduce, this possibility is seldom considered for females. This omission is particularly problematic in systems where reproducing individuals mate with only one partner, since in these systems (e.g., sexually monogamous species) it is often assumed that there will be minimal variance in mate numbers for either males or females (e.g., Table 6.1 in Shuster and Wade, 2003). However, in territorial species with limited breeding spaces, such as many territorial birds or mammals, variance in reproductive success can be high when comparing territory holders and floaters (e.g., Fernandez-Duque and Huck, 2013). Thus, in the following sections, we consider the behavioral, adaptive and evolutionary implications of pair-living and sexual monogamy from the perspectives of the breeding female and the breeding male, as well as from the perspectives of both reproductive and non-reproductive adults. We recognize, of course, that the social system outcome reflects an integration of all of these different perspectives (Gowaty, 1996; Shuster and Wade, 2003; Hosken et al., 2009).
We consider three questions in exploring why a female lives in a pair or is sexually monogamous. First, why are there no other females sharing a home range with a given female? Second, why does only one of them breed when several females share a home range? And third, why does a breeding female not mate with several males, either from her own social group or from other groups?
Asking why females do not share home ranges relates fundamentally to the social organization of a species—in other words, who groups with whom and why? When females range and breed independently, it can result in either a solitary or a pair-living social organization (or, much more rarely, in a one-female, multi-male group). All these systems are characterized by relatively small group sizes. The fact that females do not share home-ranges with other females is most likely related to the distribution of resources needed to support female survival and reproduction (Emlen and Oring, 1977; Sterck et al., 1997; Lukas and Clutton-Brock, 2013); if resource distribution were not part of the explanation, one would expect to at least sometimes see large groups comprising a single female ranging with multiple males. Still, among mammals, we see numerous examples of the reverse: very large groups consisting of a single male associating with multiple females (e.g., Hanuman langurs, Semnopithecus entellus, with harem sizes of up to nine females: Newton, 1994; Southern elephant seals, Mirounga leonina, with harem sizes of up to 90 females: Fabiani et al., 2004). However, among primates, there are no taxa in which a single female lives with many males in rather large groups, nor are we aware of cases of strongly male-skewed social groups in other mammalian taxa. Among the callitrichine primates, and among some hylobatids (gibbons and siamang), there are cases of single females living with several males, but in these cases the number of adult males is invariably small (≤4), and total group size is usually smaller than 10 individuals, including offspring (Heymann, 2000; Reichard and Barelli, 2008; Digby et al., 2010; Garber et al., 2016). Sometimes these groups are referred to as “polyandrous”, although we caution that that word—just like “monogamy”—carries with it connotations about patterns of mating or reproduction and should be eschewed unless it is being used, explicitly, to refer to either the social or genetic mating system.
Explicitly considering the point of view of the breeding male (see section 5.2) might be important for explaining apparent limits to the number of males present in these one-female, multi-male groups. Females may not object to the presence of more than one adult male; still, the selection pressure on males to reject other males may be higher than for the females to encourage them. It is worth mentioning that in several primates with either a modal pair-living social organization or a modal sexually monogamous mating system, sometimes other adults of either sex occasionally reproduce (Digby, 1995; Reichard and Barelli, 2008; Thompson, 2016; Porter et al., 2017).
If females live together with other females but only one of them reproduces, the question arises of why other females do not breed. In most group-living mammals all adult females regularly reproduce (e.g., cercopithecine primates, carnivores, Perissodactyla, and Artiodactyla, bats, elephants, and rodents: Ebensperger et al., 2012; but see Rubenstein et al., 2016). However, in some primates, and in some other group-living mammals, reproduction is highly skewed toward a dominant male-female pair. That is, even when there is more than one reproductively mature female, most females do not normally reproduce. This pattern occurs in several group-living carnivores (e.g., coyotes, Canis latrans: Hennessy et al., 2012; wolves, Canis lupus: vonHoldt et al., 2008; meerkats: Griffin et al., 2003; Young et al., 2007; see also Macdonald et al., 2019) and rodents (e.g., common mole-rats, Cryptomys hottentotus: Bishop et al., 2004; hoary marmots, Marmota caligata: Barash, 1981; Kyle et al., 2007). It is also seen in marmoset and tamarin primates, where reproduction by more than one female is very uncommon (e.g., golden lion tamarins, Leontopithecus rosalia: Dietz and Baker, 1993; saddleback tamarins, Saguinus weddelli, and several other members of the genus Saguinus: Garber et al., 2016).
In some cases, when subordinates are closely related to the opposite-sex adult in the group, inbreeding avoidance seems a likely explanation, and has been demonstrated in female Damaraland mole-rats (Cryptomys damarensis: Cooney and Bennett, 2000). However, in groups were females are not related to the dominant male, other explanations are necessary. Breeding being restricted to, or heavily skewed toward, a single dominant female suggests that some limited resources other than food (e.g., allomaternal infant care) constrain female reproductive output. In primates, group-living in association with true sexual or genetic monogamy is actually quite rare or, arguably, absent. Callitrichines are a special case. They show such high intra-specific variability in their social organization and mating patterns that researchers are reluctant to classify any species as either definitely pair-living or sexually monogamous (Garber et al., 2016). Some taxa tend to be pair-living (e.g., pygmy marmosets, Cebuella pygmaea: Soini, 1982; de la Torre et al., 2009), some may have several females breeding simultaneously (e.g., common marmosets, Callithrix jacchus: Garber et al., 2016), and yet others show monopolization of parentage by one pair despite polyandrous or polygynandrous mating (e.g., mustached tamarins: Huck et al., 2005). Still, what all callitrichine taxa have in common is a high degree of alloparental care of the infants (Garber, 1997; Erb and Porter, 2017). It has been hypothesized that competition for infant care explains the monopolization of reproduction by one female (e.g., Digby, 2000; Yamamoto et al., 2010).
In contrast, it is less clear why subordinate females do not reproduce. The topic remains rather understudied, and “proof” for hypotheses is hard to come by. At a proximate level, there are some hypotheses based on physiological and behavioral mechanisms. For callitrichines, studies of captive individuals suggest that the reproductive cycles of subordinate females are hormonally suppressed by the dominant female (French et al., 1984; Ziegler et al., 1987; Snowdon et al., 1993; Puffer et al., 2004). However, the few studies of wild callitrichines that have examined physiological suppression have failed to find similar evidence (Löttker et al., 2004; Sousa et al., 2005). There are alternative mechanisms, besides physiological ones, that may be regulating the suppression of subordinate females or otherwise reducing their reproductive output. Subordinate females may be prevented behaviorally from copulating, or their offspring may be killed by dominant females (e.g., Abbott, 1984, 1987; Digby, 1995; Digby and Saltzman, 2009). Ultimate explanations for the lack of reproduction in subordinates are not as well understood. It is possible they are “making the best of a bad job”, with the costs of foregoing reproduction being ameliorated by kin-selection benefits or by direct benefits from group living, as has been suggested for male tamarins (Huck et al., 2004a).
When females are mating with only one male, this can simply be related to a scarce distribution of males. In otherwise famously promiscuous domestic cats (Felis silvestris catus), a population on Kerguelen Island showed a monogamous genetic mating system when cat density was so low that it was not beneficial for males to attempt finding more than one female (Say et al., 2002). Despite the potential genetic benefits of mating with several males (Jennions and Petrie, 2000), and despite possible advantages accrued through confusing paternity (e.g., securing additional male investment or reducing the risk of infanticide: Hrdy, 1979; van Noordwijk and van Schaik, 2000; van Schaik et al., 2000; Soltis, 2002), it is unlikely that the benefits of finding several partners are generally as high for females as they are for males, while the costs could be higher. These higher costs can occur through harassment (e.g., normally monandrous parasitoid wasps, Nasonia vitripennis, had reduced longevity if exposed to more males during oviposition: Boulton and Shuker, 2015) or through acquiring sexually transmitted diseases that might have potentially higher costs in females (Thrall et al., 2000). An intriguing exception could be lekking species. There is some indirect evidence that female blue-crowned manakins (Lepidothrix coronata) visit multiple leks before mating, seeking out leks that are beyond their usual home-range and further away than the nearest lek (Durães et al., 2009). While considering lekking strategies of females in more detail is beyond the scope of this review, the possibility warrants further exploration.
Still remaining is the question of why, if several unrelated adult males live in a group, a breeding female mates with only one of them. Influenced, possibly, to an unjustified extent by Bateman's (1948) study of intra-sexual selection and reproductive output in Drosophila, it has long been presumed that females cannot increase their fitness by mating with multiple males. However, even if that were true (but see Jennions and Petrie, 2000), and important challenges to Bateman's paradigm notwithstanding (Tang-Martinez et al., 2000; Drea, 2005; Tang-Martinez and Ryder, 2005; Gowaty et al., 2012), the argument against mating polyandrously is still weak, unless there are actual costs associated that would select against mating with several partners (e.g., Wigby and Chapman, 2005). It is also possible that it is not a female's choice to mate with only one male, but rather that a given male might prevent other ones from mating with her (see section 5.2). Alternatively, females may indeed actively choose to mate with only one male to give him more reason to be monogynous due to increased paternity certainty. This can in turn increase the benefits of male care, since multiple matings by females and the resulting decrease in paternity certainty select against male care (Kokko and Jennions, 2008).
By analogy to the female's perspective, we consider here three parallel questions relevant to understanding pair-living and monogyny from the point of view of the breeding male: (1) why does a male live in a group or occupy a home range that contains no other males; (2) when there are several males in a group or several males with largely overlapping ranges, why might only one of them mate or breed; and (3) why doesn't a male mate with several females, either from his own social group or from other groups?
As for females, this first question refers to the social organization of the species. When other males are breeding, but are not residing in the same group, this results in a solitary, pair-living, or one-male, multi-female (“harem”) organization. All these possible social arrangements may result from resource distribution and availability and from female dispersion (see section 5.1). Behaviorally, males may actively prevent other males from entering the group. In Azara's owl monkeys (Aotus azarae), males, and indeed females as well, fiercely try to prevent solitary intruding floater males from entering a group (Fernandez-Duque and Huck, 2013). In itself, the ability to prevent other males from entering a group might depend partly on aspects of the species' ecology (Krüger et al., 2014).
In groups with more than one male, however, an almost genetically monogamous mating system may result even when the sexual mating system is polyandry, as shown for mustached tamarins (Huck et al., 2005). In these cases, the question arises why some males do not reproduce. Incest avoidance may be one explanation, as it may be a mechanism preventing males from mating with their mother or a sister. Intriguingly, in an intensely studied (>3,000 h of observation) group of mustached tamarins, the son of the breeding females was the only adult male that was never observed to mate with her (Huck et al., 2005). For males unrelated to the breeding female other explanations are necessary. If the breeding male prevents, or the female refrains from, copulations between the female and other males, at least during the female's fertile period, this results in a polygynous or a genetically monogamous mating system. Another reason could be that in pair-living mammals, females tend to be the same size as males, or slightly larger (ungulates: Loison et al., 1999; canids: Macdonald et al., 2019; primates: Smith and Jungers, 1997). Males, therefore, find it more difficult to actively prevent females from mating with other males than males in taxa with substantial body size sexual dimorphism. In the latter case, males may employ more subtle strategies, such as mate guarding. One of the phylogenetic comparative analyses discussed above suggested that a lack of sexual size dimorphism is actually not a consequence of, but rather a prerequisite for, sexual monogamy (Lukas and Clutton-Brock, 2013). Mathematical modeling also suggests that the propensity of females to seek extra-pair copulations strongly affects how worthwhile a mate-guarding strategy may be to males (Kokko and Morrell, 2005). The mate guarding strategy of the male can consist of preventing other males from approaching the female, more than preventing the female from mating with other males. When the female's reproductive status is assessed through olfactory mechanisms, males may try to prevent other males from getting close enough to a female to assess her reproductive status, as has been suggested for Kirk's dik-dik (Madoqua kirkii: Brotherton et al., 1997), Azara's owl monkeys (Aotus azarae: Spence-Aizenberg et al., 2018), and mustached tamarins (Saguinus mystax: Huck et al., 2004a).
Given the profound differences in the reproductive biology of male and female mammals, understanding why males might not attempt to mate with several females remains a challenging question. We consider separately the situation of males in solitary, pair-living, and group-living species.
If females are widely dispersed and solitary, there might be conditions where it becomes difficult for males to assume a roving strategy that has higher fitness benefits than staying with one female and guarding her (e.g., cats on Kerguelen Island; Say et al., 2002; prairie voles, Microtus ochrogaster: Rice et al., 2018). Lukas and Clutton-Brock's (2013) study suggested that the ancestral state for extant mammals with a “monogamous” system, whether pair-living or group living, were solitary females. However, a study focusing only on primates, and distinguishing between “dispersed” and “associated” pairs, concluded that the ancestral state for primates living in “associated” pairs (like all haplorhine pair-living primates) was group living, while species with “dispersed pairs” (e.g., pair-living strepsirrhines) usually had solitary ancestors (van Schaik and Kappeler, 2003). Given that there are no extant solitary haplorhine primates, and that pair-living occurs in a variety of independent haplorhine branches, the most parsimonious proposition for the ancestral state of haplorhines is indeed group-living species.
Living in pairs does not necessarily preclude males, or females, from seeking extra-pair copulations. Among fat-tailed dwarf lemurs (Cheirogaleus medius), who usually live in dispersed pairs, nearly half of the offspring were conceived through EPCs (Fietz, 1999; Fietz et al., 2000). This result raises the question of what prevents males in other pair-living species, such as Azara's owl monkeys (Huck et al., 2014), from mating with females in other groups. Emlen and Oring's (1977) ecological model for the evolution of mating systems suggests that the spatial distribution of females might make it impossible for males to seek EPCs. Yet, the home-ranges of females in pair-living species tend to be smaller, rather than larger, than those of solitary females with a potentially promiscuous mating system (Komers and Brotherton, 1997; Lukas and Clutton-Brock, 2013). In Azara's owl monkeys, home ranges average 6.2 ha (Wartmann et al., 2014), groups regularly travel several hundred meters (300–500 m) in a few hours (Fernandez-Duque, unpublished data) and the ranges of floaters are larger than those of established groups (Huck and Fernandez-Duque, 2017). Thus, male owl monkey can easily transverse several females' ranges in a short time. Similarly, titi monkeys and sakis (Van Belle et al., 2018), as well as gibbons (Reichard, 2003b), all have home-range sizes that are sufficiently small relative to day range length, such that a male should be able to cross several female ranges per day, potentially gaining reproductive access to more than one of them. That extra-pair copulations and paternities have been reported in several species of hylobatids (Reichard, 1995; Kenyon et al., 2011; Barelli et al., 2013) suggests that pair-living males, and females, sometimes do pursue successfully mating opportunities outside the pair.
When multiple females live in a group, it is even more difficult to understand why males do not attempt to mate with several of them. Callitrichines are the only primate clade where males often live with several unrelated adult females and do not normally mate with more than one of them. During more than 3,000 h of observations of a group of mustached tamarins, none of the adult males attempted to copulate with either of the two non-breeding females, while all males unrelated to the breeding females did mate: with her (Huck et al., 2005). However, in the same population, one of us observed a copulation by a subordinate female with a male from a neighboring group (Huck et al., 2005). Furthermore, very occasionally, more than one female may attempt to breed in the same group (Culot et al., 2011). This indicates that tamarin males might not say “no” if the opportunity arises. In no other group-living primate taxon are males known to be sexually monogynous.
Similar strategies as discussed for females (active defense and mate-guarding) may prevent other males from achieving copulations with a guarded female. Another reason why males do not mate polygynously may be that females are preventing it through physical or hormonal mechanisms. That this is more than just a theoretical possibility has been shown for burying beetles (Nicrophorus defodiens), where females try to coerce males into mating monogamously by preventing them from advertising through pheromones to other females (Eggert and Sakaluk, 1995). Although traditionally we have not considered female mammals as having a high potential for coercing sexual monogamy in males, the previously discussed lack of dimorphism that characterizes most pair-living primate taxa can reasonably allow females to have influence over their partner's mating strategies. Additionally, dominant females may also be able to exert control over a male's interest in mating with other females indirectly, by suppressing the ovulation of subordinates.
More generally, it remains necessary to explain how the benefits to a male from staying with a particular female may be higher than the alternative strategy of trying to find and mate with additional females. The relative payoffs will depend on both the costs of mate search, the probability of encountering potential mates (Fromhage et al., 2005) and the accrued benefits from increasing the female's fitness. For example, by reducing the costs of raising offspring for a female, a male might increase the likelihood of future reproduction with the same partner, and male care could likewise directly increase infant survival. Taking at face value the evidence from recent comparative phylogenetic analyses that pair-living typically evolves before extensive parental care (Lukas and Clutton-Brock, 2013), this explanation obviously must be a secondary step that drives extra-pair paternity rates down once an association has been established, rather than being the primary cause. Mathematical modeling investigating situations where male mate-guarding and sexual monogamy are likely to evolve, indicate that important factors are the adult sex ratio (ASR), where an increasingly male-biased sex-ratio would increase a male's mate-guarding time, and the female's likelihood of seeking EPCs, where either very high or very low rates of female EPCs reduce a male's guarding effort (Kokko and Morrell, 2005; Harts and Kokko, 2013). These findings lead to further questions as to why, in some taxa, the ASR can be, or could have been in the past, more male-biased than is typically seen in mammalian systems.
While it is hard enough to understand why males or females refrain from mating with others than their partners, it is even more difficult to understand why some individuals do not reproduce at all, a phenomenon that occurs in group-living species, as well as in pair-living ones (Figure 1). Unless otherwise stated, we consider the arguments for male and female non-breeders and floaters to be similar in pair-living species and in species with sexual monogamy. In both cases, unless there is a strong difference in the adult sex ratio, non-breeders and floaters can be of either sex. This should be the case in pair-living species, while in group-living species that are usually sexually monogamous the sexes might differ more strongly, because one sex might find it easier to “sneak in” breeding possibilities. For example, in callitrichines, it might be easier for males to mate with the dominant female while the dominant male is not watching than for a subordinate female to rear offspring in the presence of a dominant one.
Figure 1. Potential relationships between social organization and the variance in male and female reproductive success. Circles represent social groups. Filled symbols for males and females represent established breeders, while unfilled symbols represent other adult individuals. In all three scenarios there are six adult males and six adult females representing the population. Gray lightning bolts represent the potential for sexual competition (additional competition between individuals from different groups is additionally possible). An equal adult sex ratio is assumed. (A) In pair-living species, the majority of adults will live in established pairs. Competition is mainly between breeders established in groups and floaters. Variance of reproductive success is the same for males and females. How much variance in reproductive success exists depends on the relative number of floaters to established pairs, which in turn depends on the relative tenure length, reproductive rates of pairs, and mortality rate of floaters. (B) In group-living species with an alpha-breeding pair that monopolizes reproduction (possibly with monogamous mating system), the variance in reproductive success is again the same for both sexes, but with mature individuals in the same group that are (usually) not reproducing, the total variance is relatively high, with a high potential for same-sex competition within groups. Competition with potential intruders will depend on who the dispersing sex is, which in group-living species tends to be only one sex, with the exception of the callitrichines. (C) In more typical harem-type social organizations, female reproductive success within groups does not very much, while the variance amongst males is much higher.
Non-breeders would do better holding a breeding position themselves. In the case of cooperative breeders, or when subadults show natal delayed dispersal, the costs of non-breeding might be partly offset by direct or indirect fitness benefits. Quite commonly, non-breeders seem to be making the best of a bad job. For example, non-breeding turkey (Meleagris gallopavo) males participate in the displays of breeders to attract females in a manner that fits expectations from Hamilton's rule. While dominant males clearly have higher fitness, the benefits through indirect fitness for the related non-reproductive males appear to be higher than their probability of successful breeding (Krakauer, 2005). If indirect benefits to subordinate males are suboptimal, then it follows that they should be a constant threat to breeders.
Additionally, floaters, or non-breeders, of both sexes will affect the operational sex ratio, which can fluctuate locally and temporally (Fernandez-Duque and Huck, 2013). A large population of floaters will lead to intense intra-sexual competition. The role of floaters in the social organization and mating system of species needs urgent addressing; this is particularly true in pair-living species, where they are not so conspicuous as bachelor groups or subordinate males are in group-living species (Fernandez-Duque and Huck, 2013). The role of floaters in the formation of communal breeding in group-living species has been previously modeled (Port et al., 2011, 2017; Port and Johnstone, 2013), but not their impact on population dynamics when breeders are usually pair-living. Furthermore, but beyond the scope of this review, it would be interesting to evaluate how various models of reproductive skew (such as queuing or tolerance models: Clutton-Brock, 1998; Reeve et al., 1998; Johnstone, 2000), originally proposed for hymenopteran insects (e.g., Reeve and Ratnieks, 1993) but also applied to studies on group-living primates (Port and Kappeler, 2010; Snyder-Mackler et al., 2012; Port et al., 2018), could be developed to help understand the dynamics of interactions between floaters of both sexes and breeding pairs, e.g., by examining the extent of deviations from pair-living or sexual monogamy in relation to different levels of alloparental care behavior.
Floaters can potentially have a strong impact on population dynamics and on the operation of sexual selection. Given an equal sex ratio, the mean number of offspring must be the same for males and females (Houston and McNamara, 2002; Wade and Shuster, 2002; Kokko and Jennions, 2003). Thus, all else being equal, the variance in reproductive success should be similar for males and females under sexual monogamy, but higher for males than females in a polygynous system (Trivers, 1972; Clutton-Brock, 1988). But what has not been pointed out before is that the magnitude of the variance in reproductive output might differ between pair-living species with sexual monogamy, and group-living species with the same mating system (Figure 1). This would most definitely be the case if the population includes floaters, since floaters also contribute to the variance in reproductive success in a population.
In owl monkeys, the majority of adults live in stable pairs, such that a majority of adult males and a majority of adult females hold reproductive positions. However, there is a substantial number of young adults who after emigrating from their natal groups remain in the population, ranging over the home ranges of several pair-living residents (Figure 1A). These floaters either challenge the same-sex individuals of established pairs to try to gain a breeding position or take advantage of vacancies in resident positions that arise through natural deaths (Fernandez-Duque and Huck, 2013; Huck and Fernandez-Duque, 2017). It has proven difficult to quantify precisely the frequency of those challenges. Over the years, we have quantified the frequency of successful takeovers by floaters (27 female and 23 male replacements in a total of 149 group-years: Fernandez-Duque and Huck, 2013), but the frequency of their failed attempts is likely to go unnoticed by researchers. Equally, while there should be the possibility of sneaky copulations with individuals from neighboring groups, the opportunity of EPCs should be relatively low in closely associated pairs, such as owl monkeys. Male and female owl monkey partners are constantly in very close proximity to each other; they should therefore be able to ward off attempts to copulate with other individuals. Thus, we expect variance in fitness to be similar for males and females, and similarly low amongst breeding individuals of both sexes. However, differences between breeding male and female variance in reproductive success will also depend on the relative numbers of male and female floaters in the population, and variance in reproductive success amongst all adults, i.e., including floaters that never obtain a breeding position, will be much higher than often assumed (e.g., in Table 6.1 in Shuster and Wade, 2003).
By contrast, in group-living species with several potentially reproductively active males and females, and where one alpha male manages to monopolize reproduction, there will be several mature adults of both sexes present on a daily basis that could compete for reproductive opportunities. A large proportion of the population will live in groups, but only a small number will hold a main reproductive position. The total variance of reproductive success should, on a population level, be therefore higher, and opportunities for competition should be more frequent (Figure 1B). Naturally, these arguments focus on a snapshot in time. Conditions might differ if dominance is, for example, age-dependent, so that if skew is measured over a multi-year window, the variance will be actually lower and approach that of the “less competitive” sex. In groups, there will likely not only be competition between group members, but additional interactions with dispersing individuals or neighboring groups could also take place, as in the case of pair-living species. In one-male multi-female groups, essentially all females should reproduce, so there should be very little variance among females (and less than among pair-living females, where floating females might never gain a reproductive position), but very high variance among males (Figure 1C). We find it important to reiterate that pair-living need not imply lack of competition; indeed, competition can be frequent and/or intense, depending on the characteristics of the floater population (Fernandez-Duque and Huck, 2013). Still, competition should occur more sporadically than in group-living sexually monogamous species, and a larger proportion of the population should be able to reproduce at the same time. This might have implications for the sexual selection potential that have not been fully explored.
Our understanding of the evolution of pair-living and sexual monogamy in primates and other mammalian taxa has moved forward some important steps since Kleiman (1977) attempted the first comprehensive review, but work remains to be done. Apart from our hope that some of the issues about data quality and comparability in comparative studies that we addressed above will be dealt with more consistently in the future (see section 4.3) and that some of the hypotheses outlined in section 3 will be revisited using better datasets with clearer definitions, we suggest below several related topics that warrant and will benefit from further attention and research.
First, the likelihood of an individual encountering potential sexual partners depends on various factors, and comparative analyses looking at operational and adult sex ratios, home-range size, female dispersion, degree of home-range overlap, and species-specific life-history traits do try to take a number of those factors into account. We suggest, however, that comparative studies should strive to consider additional factors that are slowly beginning to be investigated. In particular, for pair-living species, the role of floaters in considerations of adult sex ratios has been too long neglected, and we hope that more researchers will attempt to study this elusive component of any species' population biology. We cannot emphasize enough that it took the first 6 years of our research on secretive owl monkeys in Argentina to realize the importance of a floater population; new technologies are already contributing to carefully assessing the existence of floaters in other secretive taxa (Sabol et al., 2018).
Second, if the adult sex ratio plays such a strong role for mating systems as theory suggest (Harts and Kokko, 2013), the factors affecting the adult sex ratio require further exploration as well. For example, does a biased sex ratio start at birth or is it related to differential investment in sons and daughters that affects their survival probability? What is the influence of differential mortality among adult males and females, both residents and floaters? There have been suggestions that in polygynous primates the reduced life expectancy of males relative to females contributes to greater variance in reproductive success in males (Clutton-Brock and Isvaran, 2007; Bronikowski et al., 2011). Among pair-living sexually monogamous primates, it has been hypothesized that natural selection will favor longevity in the sex most responsible for rearing offspring (Allman et al., 1998). Owl monkeys—pair-living, sexually and genetically monogamous, and showing biparental care of offspring—provide some evidence in support of this hypothesis. Male and female owl monkeys show similar levels of intra-sexual competition and have similar life expectancies (Larson et al., 2016).
Third, more research is needed on the communication between pair-mates and between groups. van Schaik and Kappeler (2003; see also Kappeler, 2014) suggested a different ancestral state for extant dispersed vs. associated pairs, and it is clear that more work is needed to understand the various behavioral mechanisms coordinating the relationship between pair-mates. For example, in our work with three taxa (owl monkeys, titis, and sakis) that are consistently classified as pair-living and sexually monogamous, we have, over the years, documented qualitatively different patterns of male-female interaction among them (Spence-Aizenberg et al., 2016; Porter et al., 2017). Loud-calls and duetting are suggested as one important set of behaviors involved in regulating pair-bonding, group cohesion, and inter-group communication. A number of studies have explored the possible functions of loud-calls and duetting in regulating territoriality and use of space by groups of pair-living owl monkeys, titi monkeys, and gibbons (Mitani, 1985a,b; Caselli et al., 2014, 2015; Ham et al., 2016, 2017; Adret et al., 2018; García de la Chica et al., 2019), though similar studies have not yet been done on sakis.
Fourth, the need to better understand male-female relationships is also related to questions about mate-guarding and extra-pair copulations by males, and potentially also by females, as research on burying beetles has shown (Eggert and Sakaluk, 1995). In turn, more detailed knowledge on male-female interactions will allow progress in assessing the presence or not of a bond between breeding male and female (pair-bonding). This would be particularly useful, given the suggestion that these types of pairs might have evolved through different routes (van Schaik and Kappeler, 2003; Kappeler, 2014). Studies of captive individuals have provided the best data on pair-bonds in rodents and primates, including hormonal regulation, neurogenetics, neurobiology and behavioral indicators of emotional distress (Smith et al., 2010; Gobrogge and Wang, 2015; Maninger et al., 2017; Carter and Perkeybile, 2018). Unfortunately, far less is known about degree of bonding in other species and very little physiological data on these aspects exist for wild primates, and in particular not in dispersed pairs. As humans show, a pair-bond can exist without constant proximity (Gavrilets, 2012), but pair-bonding is probably not required for keeping a pair-living social organization or even a monogamous mating system, given the occurrence of “dispersed pairs” in species like fork-marked lemurs (Schülke and Kappeler, 2003). Even if floaters were the most important source of intra-sexual competition, pair-living groups also interact with other groups, leading to opportunities for competition.
Fifth, while the relationship between a presumed father's care behavior, pair-living, and sexual and genetic monogamy have received substantial attention (Huck et al., 2014; Klug, 2018; Rogers et al., 2018), among primates (with the exception of the callitrichines) alloparental care seems to be a little explored dimension associated with sexual monogamy and pair-living. Sibling participation in infant care is virtually absent in owl monkeys, titi monkeys and siamangs (Lappan, 2008; Fernandez-Duque et al., 2009), where it is the male and the female who share parental duties. In the less strictly pair-living sakis (genus Pithecia), we have seen some carrying of offspring by older siblings and very rarely by an adult male (Di Fiore et al., unpublished data), and a study on an island population without dispersal possibilities found some carrying by other adult and sub-adult females in P. pithecia (Homburg, 1997). The variable participation of adult and sub-adult non-breeders in offspring care tentatively suggests that concession models of reproductive skew or tolerance models (see section 5.3) may sometimes be important for understanding the evolution of social and genetic mating systems in group-living primates.
Finally, we have tentatively suggested in the section on proposed terminology that, where possible, researchers should seek to abandon categorizations of potentially continuous variables. In many cases, however, it needs to be explored in more detail how this could be best approached, because unthinking use of measurable values might create new problems, and it has been suggested (though there were problems with that study as well similar to the ones discussed in the section 4.2) that using continuous data can sometimes lead to a false perception of continuity (Rubenstein et al., 2016).
Both in our work and when writing the sections above, our premise has been that evolutionary biologists attempting to understand the evolution of any aspect of an animal social system are primarily interested in two main topics. First, under the assumption that the behavioral traits that shape the social system have a genetically inheritable component, researchers are interested in understanding whether and how individual variation in those traits is associated with differences in fitness. Such consequences on fitness can be operationalized through proxies like number of copulations or genetically assigned paternity, but, unfortunately, we often lack empirical data on the fitness consequences of variation between individuals in behaviors associated with both pair-living, sexual and genetic monogamy, such as levels of mate guarding, intolerance of same sex adults, or parental investment in offspring (but see Shuster et al., 2019 for an exciting example of what is needed). Second, as evidenced by the continued growth in the number of comparative studies, researchers are interested in understanding the evolutionary and phylogenetic history of animal social systems as emergent phenomena. These analyses aim to shed light on how, when, and why particular aspects of social systems have arisen and on inferring the social systems of extinct taxa. With respect to this second topic, we believe that at the moment no robust conclusions can yet be drawn because of the problems associated with comparative studies that we have outlined in detail above. Similar analyses based on sounder datasets with more clearly defined and comparable terms are required. Our calls for caution notwithstanding, recent analyses of the evolution of “monogamy” have provided tantalizing possible explanations and opened up further questions. If Lukas and Clutton-Brock (2013), for example, are right about a likely solitary ancestral route to a monogamous mating system in most mammalian taxa, then primatologists can look forward to decades of research trying to understand why primates seem to deviate from this pattern (van Schaik and Kappeler, 2003). There is no a priori reason to expect a single explanation for the evolution of pair-living, sexual or genetic monogamy either across mammals or across primates, but understanding how and why lineages differ in their evolutionary routes to these traits will help shed additional light on the ecological, social, and phylogenetic factors that influence social evolution. In this context, it is also important to incorporate all of the various elements that tend to be conflated in folk conceptions of “monogamy” (i.e., pair-living, pair-bonding, sexual monogamy, genetic monogamy, and infant care patterns) into the same comparative analyses, because, while these are partly separate phenomena, they can still clearly interact with and influence one another.
We admit to a bit of frustration at realizing that our closing recommendations for how to make progress in the comparative study of both pair-living and sexual monogamy are neither new nor theoretically ground-breaking, but rather a plea for returning to the bedrock of scientific inquiry: for sound comparative analysis, we need more high-quality natural history data on a wider range of animal taxa. Our recommendations can be summed up as follows: we must stop the deluge of lots of data of doubtful quality; we must strive for high quality natural history data; we must incorporate perspectives of all participants in a social system, not only breeding males and females, but also non-reproductive adult group members as well as floaters living outside of established groups; and we must, when reaching conclusions, distinguish between the actual data and the statistical and evolutionary inferences (with their associated uncertainties) made from them (Schradin, 2017). Comparative studies have laudable goals, but their potential can only be realized if suitable, comparable data are available to analyze. As others have stated previously, comparative studies can be no more reliable than the data on which they are based (Smith and Jungers, 1997).
MH wrote the first draft of the manuscript. AD, EF-D, and MH wrote different sections of the manuscript. AD and EF-D implemented and funded the studies that resulted in the data reported. All authors contributed to manuscript revision, read and approved the submitted version.
The authors declare that the research was conducted in the absence of any commercial or financial relationships that could be construed as a potential conflict of interest.
We are most grateful to Alex Ophir and Nancy Solomon for inviting us to contribute to this special issue of Frontiers in Ecology and Evolution. The ideas presented benefited from discussions with and comments on early drafts by A. García de la Chica, B. Finkel, R. Lewis, M. Snodderly, A. Sandel, C. Valeggia, S. Van Belle, S. Van Kuijk, and members of the Biological Anthropology Behavior, Ecology, and Informatics (BABEI) lab group at the University of Texas at Austin. We also appreciate the helpful and constructive feedback provided by two reviewers.
Fieldwork related to the data discussed and presented here was supported through grants awarded to AD, EF-D, and their students by the Wenner-Gren Foundation, the L.S.B. Leakey Foundation, the J. William Fulbright Scholar Program, Primate Conservation, Inc., Idea Wild, the National Geographic Society, as well by the New York Consortium in Evolutionary Primatology, New York University, the Zoological Society of San Diego, the University of Pennsylvania, the University of Texas at Austin, and Yale University, as well as through grants awarded to Eckhard W. Heyman and MH by the Deutsche Forschungsgesellschaft (HE 1870/10-1,2,3, HU1746-2/1). The Owl Monkey Project of Argentina was supported through the following grants to EFD: NSF-BCS-0621020, 1232349, 1503753 and 1848954; NSF-REU 0837921, 0924352 and 1026991; NSF-RAPID-1219368; NIA- P30 AG012836-19, and NICHD R24 HD-044964-11.
The research described here was done in full agreement with all Ecuadorian and Argentinean legislation and was approved by the IACUC committees of New York University, the University of Texas at Austin, and Yale University.
Abbott, D. H. (1984). Behavioral and physiological suppression of fertility in subordinate marmoset monkeys. Am. J. Primatol. 6, 169–186. doi: 10.1002/ajp.1350060305
Abbott, D. H. (1987). Behaviourally mediated suppression of reproduction in female primates. J. Zool. Lond. 213, 455–470. doi: 10.1111/j.1469-7998.1987.tb03720.x
Adret, P., Dingess, K., Caselli, C., Vermeer, J., Martínez, J., Luna Amancio, J., et al. (2018). Duetting patterns of titi monkeys (Primates, Pitheciidae: Callicebinae) and relationships with phylogeny. Animals 8:178. doi: 10.3390/ani8100178
Allman, J., Rosin, A., Kumar, R., and Hasenstaub, A. (1998). Parenting and survival in anthropoid primates: caretakers live longer. Proc. Natl. Acad. Sci. U.S.A. 95, 6866–6869. doi: 10.1073/pnas.95.12.6866
Altmann, J. (1990). Primate males go where the females are. Anim. Behav. 39, 193–195. doi: 10.1016/S0003-3472(05)80740-7
Barash, D. P. (1981). Mate guarding and gallivanting by male hoary marmots (Marmota caligata). Behav. Ecol. Sociobiol. 9, 187–193. doi: 10.1007/BF00302936
Barelli, C., Matsudaira, K., Wolf, T., Roos, C., Heistermann, M., Hodges, K., et al. (2013). Extra-pair paternity confirmed in wild white-handed gibbons. Am. J. Primatol. 75, 1185–1195. doi: 10.1002/ajp.22180
Bateman, A. (1948). Intra-sexual selection in Drosophila. Heredity 2, 349–368. doi: 10.1038/hdy.1948.21
Bishop, J. M., Jarvis, J. U. M., Spinks, A. C., Bennett, N. C., and O'Ryan, C. (2004). Molecular insight into patterns of colony composition and paternity in the common mole-rat Cryptomys hottentotus hottentotus. Mol. Ecol. 13, 1217–1229. doi: 10.1111/j.1365-294X.2004.02131.x
Bonadonna, G., Torti, V., De Gregorio, C., Valente, D., Randrianarison, R. M., Pozzi, L., et al. (2019). Evidence of genetic monogamy in the lemur Indri (Indri indri). Am. J. Primatol. 81:e22993. doi: 10.1002/ajp.22993
Borries, C., Gordon, A. D., and Koenig, A. (2013). Beware of primate life history data: a plea for data standards and a repository. PLoS ONE 8:e67200. doi: 10.1371/journal.pone.0067200
Borries, C., Lu, A., Ossi-Lupo, K., Larney, E., and Koenig, A. (2014). The meaning of weaning in wild Phayre's leaf monkeys: last nipple contact, survival, and independence. Am. J. Phys. Anthropol. 154, 291–301. doi: 10.1002/ajpa.22511
Borries, C., Sandel, A. A., Koenig, A., Fernandez-Duque, E., Kamilar, J. M., Amoroso, C. R., et al. (2016). Transparency, usability, and reproducibility: guiding principles for improving comparative databases using primates as examples. Evol. Anthropol. 25, 232–238. doi: 10.1002/evan.21502
Boulton, R. A., and Shuker, D. M. (2015). The costs and benefits of multiple mating in a mostly monandrous wasp. Evolution 69, 939–949. doi: 10.1111/evo.12636
Bronikowski, A. M., Altmann, J., Brockman, D. K., Cords, M., Fedigan, L. M., Pusey, A., et al. (2011). Aging in the natural world: comparative data reveal similar mortality patterns across primates. Science 331, 1325–1328. doi: 10.1126/science.1201571
Brotherton, P. N. M., and Komers, P. E. (2003). “Mate guarding and the evolution of social monogamy in mammals,” in Monogamy, eds U. H. Reichard and C. Boesch (Cambridge: Cambridge University Press), 42–58. doi: 10.1017/CBO9781139087247.003
Brotherton, P. N. M., Pemberton, J. M., Komers, P. E., and Malarky, G. (1997). Genetic and behavioural evidence of monogamy in a mammal, Kirk's dik–dik (Madoqua kirkii). Proc. R. Soc. Lond. Series B 264, 675–681. doi: 10.1098/rspb.1997.0096
Bull, C. M. (2000). Monogamy in lizards. Behav. Processes., 51, 7–20. doi: 10.1016/S0376-6357(00)00115-7
Burt, W. H. (1943). Territoriality and home range concepts as applied to mammals. J. Mammal. 24, 346–352. doi: 10.2307/1374834
Bybee, L. F., Millar, J. G., Paine, T. D., and Hanlon, C. C. (2005). Effects of single versus multiple mates: monogamy results in increased fecundity for the beetle Phoracantha semipunctata. J. Insect Behav. 18, 513–527. doi: 10.1007/s10905-005-5609-7
Carrillo-Bilbao, G., Di Fiore, A., and Fernández-Duque, E. (2005). Dieta, forrajeo y presupuesto de tiempo en cotoncillos (Callicebus discolor) del Parque Nacional Yasuní en la Amazonia Ecuatoriana. Neotropical Primates 13, 7–11. doi: 10.1896/1413-4705.13.2.7
Carroll, J. B. (1982). Maintenance of the Goeldi's monkey Callimico goeldii at Jersey wildlife preservation trust. Int. Zoo Yearbook 22, 101–105. doi: 10.1111/j.1748-1090.1982.tb02015.x
Carter, C. S., and Perkeybile, A. M. (2018). The monogamy paradox: what do love and sex have to do with it? Front. Ecol. Evol. 6:202. doi: 10.3389/fevo.2018.00202
Caselli, C. B., Mennill, D. J., Bicca-Marques, J. C., and Setz, E. Z. F. (2014). Vocal behavior of black-fronted titi monkeys (Callicebus nigrifrons): acoustic properties and behavioral contexts of loud calls. Am. J. Primatol. 76, 788–800. doi: 10.1002/ajp.22270
Caselli, C. B., Mennill, D. J., Gestich, C. C., Setz, E. Z. F., and Bicca-Marques, J. C. (2015). Playback responses of socially monogamous black-fronted titi monkeys to simulated solitary and paired intruders. Am. J. Primatol. 77, 1135–1142. doi: 10.1002/ajp.22447
Chakrabarti, S., and Jhala, Y. V. (2019). Battle of the sexes: a multi-male mating strategy helps lionesses win the gender war of fitness. Behav. Ecol. 30,1050–1061. doi: 10.1093/beheco/arz048
Clutton-Brock, T. (1998). Reproductive skew, concessions and limited control. Trends Ecol. Evol. 13, 288–292. doi: 10.1016/S0169-5347(98)01402-5
Clutton-Brock, T. (2007). Sexual selection in males and females. Science 318, 1882–1885. doi: 10.1126/science.1133311
Clutton-Brock, T. H., (ed.). (1988). Reproductive Success: Studies of Individual Variation in Contrasting Breeding Systems. Chicago, IL: University of Chicago Press
Clutton-Brock, T. H. (1989). “Mammalian mating systems,” in Proceedings of the Royal Society of London, Series B, 236, 339–372.
Clutton-Brock, T. H., and Huchard, E. (2013). Social competition and selection in males and females. Philos. Trans. R. Soc. B 368:20130074. doi: 10.1098/rstb.2013.0074
Clutton-Brock, T. H., and Isvaran, K. (2007). Sex differences in ageing in natural populations of vertebrates. Proc. R. Soc. B 274, 3097–3104. doi: 10.1098/rspb.2007.1138
Cohas, A., and Allainé, D. (2009). Social structure influences extra-pair paternity in socially monogamous mammals. Biol. Lett. 5, 313–316. doi: 10.1098/rsbl.2008.0760
Cooney, R., and Bennett, N. C. (2000). Inbreeding avoidance and reproductive skew in a cooperative mammal. Proc. R. Soc. Lond. B 267, 801–806. doi: 10.1098/rspb.2000.1074
Culot, L., Lledo-Ferrer, Y., Hoelscher, O., Muñoz Lazo, F. J. J., Huynen, M.-C., and Heymann, E. W. (2011). Reproductive failure, possible maternal infanticide, and cannibalism in wild moustached tamarins, Saguinus mystax. Primates 52, 179–186. doi: 10.1007/s10329-011-0238-6
de la Torre, S., Yépez, P., and Snowdon, C. T. (2009). “Conservation status of pygmy marmosets (Cebuella pygmaea) in Ecuador,” in The Smallest Anthropoids, eds S. M. Ford, L. M. Porter, and L. C. Davis (Boston, MA: Springer), 451–464. doi: 10.1007/978-1-4419-0293-1_22
de Waal, F. B. M., and Gavrilets, S. (2013). Monogamy with a purpose. Proc. Natl. Acad. Sci. U.S.A. 110, 15167–15168. doi: 10.1073/pnas.1315839110
Dey, C. J., O'Connor, C. M., Wilkinson, H., Shultz, S., Balshine, S., and Fitzpatrick, J. L. (2019). Confounding social and mating systems predictably lead to biased results when examining the evolution of cooperative breeding in cichlids: a response to Tanaka et al. Ethology 125, 409–414. doi: 10.1111/eth.12883
Di Fiore, A., Fernandez-Duque, E., and Hurst, D. (2007). Adult male replacement in socially monogamous equatorial saki monkeys (Pithecia aequatorialis). Folia Primatol. 78, 88–98. doi: 10.1159/000097059
Di Fiore, A., and Rendall, D. (1994). Evolution of social organization: a reappraisal for primates by using phylogenetic methods. Proc. Natl. Acad. Sci. U.S.A. 91, 9941–9945. doi: 10.1073/pnas.91.21.9941
Díaz-Muñoz, S. L. (2011). Paternity and relatedness in a polyandrous nonhuman primate: testing adaptive hypotheses of male reproductive cooperation. Anim. Behav. 82, 563–571. doi: 10.1016/j.anbehav.2011.06.013
Díaz-Muñoz, S. L., and Bales, K. L. (2016). “Monogamy” in primates: variability, trends, and synthesis. Am. J. Primatol. 78, 283–287. doi: 10.1002/ajp.22463
Dietz, J., and Baker, A. (1993). Polygyny and female reproductive success in golden lion tamarins, Leontopithecus rosalia. Anim. Behav. 46, 1067–1078. doi: 10.1006/anbe.1993.1297
Digby, L. (1995). Infant care, infanticide, and female reproductive strategies in polygynous groups of common marmosets (Callithrix jacchus). Behav. Sociobiol. 37, 51–61. doi: 10.1007/BF00173899
Digby, L. (2000). “Infanticide by female mammals: implications for the evolution of social systems,” in Infanticide by Males and its Implications, eds C. P. van Schaik and C. H. Janson (Cambridge: Cambridge University Press), 423–446. doi: 10.1017/CBO9780511542312.019
Digby, L., and Saltzman, W. (2009). “Balancing cooperation and competition in callitrichid primates: examining the relative risk of infanticide across species,” in The Smallest Anthropoids, eds S. M. Ford, L. M. Porter, and L. C. Davis (Boston, MA: Springer US), 135–153. doi: 10.1007/978-1-4419-0293-1_7
Digby, L. J., Saltzman, W., and Ferrari, S. F. (2010). “Callitrichines,” in Primates in Perspective, eds C. J. Campbell, A. Fuentes, K. C. MacKinnon, S. K. Bearder, and R. M. Stumpf (Oxford: Oxford University Press), 91–107.
Dixson, A. F. (2013). Male infanticide and primate monogamy. Proc. Natl. Acad. Sci. U.S.A. 110, E4937. doi: 10.1073/pnas.1318645110
Drea, C. M. C. (2005). Bateman revisited: the reproductive tactics of female primates. Am. Zool. 45, 915–923. doi: 10.1093/icb/45.5.915
Durães, R., Loiselle, B. A., Parker, P. G., and Blake, J. G. (2009). Female mate choice across spatial scales: influence of lek and male attributes on mating success of blue-crowned manakins. Proc. R. Soc. B 276, 1875–1881. doi: 10.1098/rspb.2008.1752
Ebensperger, L. A., Rivera, D. S., and Hayes, L. D. (2012). Direct fitness of group living mammals varies with breeding strategy, climate and fitness estimates. J. Anim. Ecol. 81, 1013–1023. doi: 10.1111/j.1365-2656.2012.01973.x
Eggert, A.-K., and Sakaluk, S. K. (1995). Female-coerced monogamy in burying beetles. Behav. Ecol. Sociobiol. 37, 147–153. doi: 10.1007/BF00176711
Emlen, S., and Oring, L. (1977). Ecology, sexual selection, and the evolution of mating systems. Science 197, 215–223. doi: 10.1126/science.327542
Erb, W. M., and Porter, L. M. (2017). Mother's little helpers: what we know (and don't know) about cooperative infant care in callitrichines. Evol. Anthropol. 26, 25–37. doi: 10.1002/evan.21516
Fabiani, A., Galimberti, F., Sanvito, S., and Hoelzel, A. R. (2004). Extreme polygyny among southern elephant seals on Sea Lion Island, Falkland Islands. Behav. Ecol. 15, 961–969. doi: 10.1093/beheco/arh112
Fernandez-Duque, E. (2007). “Aotinae: social monogamy in the only nocturnal haplorhines,” in Primates in Perspective, 1st ed., C. J. Campbell, A. Fuentes, K. C. Mackinnon, M. Panger, and S. K. Bearder (Oxford: Oxford University Press)
Fernandez-Duque, E. (2011). “The Aotinae: social monogamy in the only nocturnal anthropoid,” in Primates in Perspective, 2nd Edn, eds C. J. Campbell, A. Fuentes, K. C. MacKinnon, S. K. Bearder, and R. M. Stumpf (Oxford: Oxford University Press), 140–154.
Fernandez-Duque, E. (2016). Social monogamy in wild owl monkeys (Aotus azarae) of Argentina: the potential influences of resource distribution and ranging patterns. Am. J. Primatol. 78, 355–371. doi: 10.1002/ajp.22397
Fernandez-Duque, E., Di Fiore, A., and Huck, M. (2013). “The behavior, ecology, and social evolution of New World monkeys,” in The Evolution of Primate Societies, eds J. C. Mitani, J. Call, P. M. Kappeler, R. A. Palombit, and J. B. Silk (Chicago, IL: University of Chicago Press), 43–64.
Fernandez-Duque, E., and Huck, M. (2013). Till death (or an intruder) do us part: intrasexual-competition in a monogamous primate. PLoS ONE 8:e53724. doi: 10.1371/journal.pone.0053724
Fernandez-Duque, E., Juárez, C. P., and Di Fiore, A. (2008). Adult male replacement and subsequent infant care by male and siblings in socially monogamous owl monkeys (Aotus azarai). Primates 49, 81–84. doi: 10.1007/s10329-007-0056-z
Fernandez-Duque, E., Valeggia, C. R., and Mendoza, S. P. (2009). The biology of paternal care in human and nonhuman primates. Annu. Rev. Anthropol. 38, 115–130. doi: 10.1146/annurev-anthro-091908-164334
Fieberg, J., and Kochanny, C. O. (2005). Quantifying home-range overlap: the importance of the utilization distribution. J. Wildl. Manage. 69, 1346–1359. doi: 10.2193/0022-541X(2005)69[1346:QHOTIO]2.0.CO;2
Fietz, J. (1999). Monogamy as a rule rather than exception in nocturnal lemurs: the case of the fat-tailed dwarf lemur, Cheirogaleus medius. Ethology 105, 255–272. doi: 10.1046/j.1439-0310.1999.00380.x
Fietz, J., Zischler, H., Schwiegk, C., Tomiuk, J., Dausmann, K., and Ganzhorn, J. (2000). High rates of extra-pair young in the pair-living fat-tailed dwarf lemur, Cheirogaleus medius. Behav. Ecol. Sociobiol. 49, 8–17. doi: 10.1007/s002650000269
French, J., Abbott, D. H., and Snowdon, C. (1984). The effect of social environment on estrogen excretion, scent marking, and sociosexual behavior in tamarins (Saguinus oedipus). Am. J. Primatol. 6, 155–167. doi: 10.1002/ajp.1350060304
French, J. A., Cavanaugh, J., Mustoe, A. C., Carp, S. B., and Womack, S. L. (2018). Social monogamy in nonhuman primates: phylogeny, phenotype, and physiology. J. Sex Res. 55, 410–434. doi: 10.1080/00224499.2017.1339774.
Fromhage, L., Elgar, M. A., and Schneider, J. M. (2005). Faithful without care: the evolution of monogyny. Evolution 59, 1400–1405. doi: 10.1111/j.0014-3820.2005.tb01790.x
Fuentes, A. (1998). Re-evaluating primate monogamy. Am. Anthropol. 100, 890–907. doi: 10.1525/aa.1998.100.4.890
Fuentes, A. (2002). Patterns and trends in primate pair bonds. Int. J. Primatol. 23, 953–978. doi: 10.1023/A:1019647514080
Garber, P. A. (1997). One for all and breeding for one: cooperation and competition as a tamarin reproductive strategy. Evol. Anthropol. 5, 187–199.
Garber, P. A., Porter, L. M., Spross, J., and Di Fiore, A. (2016). Tamarins: insights into monogamous and non-monogamous single female social and breeding systems. Am. J. Primatol. 78, 298–314. doi: 10.1002/ajp.22370
García de la Chica, A., Rotundo, M., Depeine, M., Huck, M., and Fernandez-Duque, E. E. (2019). Sexual dimorphism and the evaluation of sexual selection in loud calls of azara's owl monkeys (Aotus azarae) of Argentina. Primates. doi: 10.1007/s10329-019-00773-6. [Epub ahead of print].
Gavrilets, S. (2012). Human origins and the transition from promiscuity to pair-bonding. Proc. Natl. Acad. Sci. U.S.A. 109, 9923–9928. doi: 10.1073/pnas.1200717109. [Epub ahead of print].
Getz, W. M., Fortmann-Roe, S., Cross, P. C., Lyons, A. J., Ryan, S. J., and Wilmers, C. C. (2007). LoCoH: nonparametric kernel methods for constructing home ranges and utilization distributions. PLoS ONE 2:e207. doi: 10.1371/journal.pone.0000207
Gittleman, J. L. (1989). “The comparative approach in ethology: aims and limitations,” in Perspectives in Ethology, eds P. P. G. Bateson and P. H. Klopfer (New York: Plenum Publishing), 55–83.
Gobrogge, K., and Wang, Z. (2015). Neuropeptidergic regulation of pair-bonding and stress buffering: lessons from voles. Horm. Behav. 76, 91–105. doi: 10.1016/j.yhbeh.2015.08.010
Gowaty, P. A. (1996). “Battles of the sexes and the origins of monogamy,” in Partnerships in Birds: The Study of Monogamy, ed J. Black (Oxford: Oxford University Press), 21–52.
Gowaty, P. A. (2004). “Sex roles, contests for the control of reproduction and sexual selection,” in Sexual Selection in Primates: New and Comparative Perspectives, eds P. M. Kappeler and C. P. van Schaik (Cambridge: Cambridge University Press), 37–54. doi: 10.1017/CBO9780511542459.005
Gowaty, P. A., Kim, Y.-K., and Anderson, W. W. (2012). No evidence of sexual selection in a repetition of Bateman's classic study of Drosophila melanogaster. Proc. Natl. Acad. Sci. U.S.A. 109, 11740–11745. doi: 10.1073/pnas.1207851109
Griffin, A., Pemberton, J., Brotherton, P., McIlrath, G., Gaynor, D., Kansky, R., et al. (2003). A genetic analysis of breeding success in the cooperative meerkat (Suricata suricatta). Behav. Ecol. 14, 472–480. doi: 10.1093/beheco/arg040
Griffith, S. C., Owens, I. P. F., and Thuman, K. A. (2002). Extra pair paternity in birds: a review of interspecific variation and adaptive function. Mol. Ecol. 11, 2195–2212. doi: 10.1046/j.1365-294X.2002.01613.x
Ham, S., Hedwig, D., Lappan, S., and Choe, J. C. (2016). Song functions in nonduetting gibbons: evidence from playback experiments on Javan gibbons (Hylobates moloch). Int. J. Primatol. 37, 225–240. doi: 10.1007/s10764-016-9897-x
Ham, S., Lappan, S., Hedwig, D., and Choe, J. C. (2017). Female songs of the nonduetting Javan gibbons (Hylobates moloch) function for territorial defense. Int. J. Primatol. 38, 533–552. doi: 10.1007/s10764-017-9964-y
Harts, A. M. F., and Kokko, H. (2013). Understanding promiscuity: when is seeking additional mates better than guarding an already found one? Evolution 67, 2838–2848. doi: 10.1111/evo.12163
Heistermann, M., and Hodges, J. K. (1995). Endocrine monitoring of the ovarian cycle and pregnancy in the saddle-back tamarin (Saguinus fuscicollis) by measurement of steroid conjugates in urine. Am. J. Primatol. 35, 117–127. doi: 10.1002/ajp.1350350204
Hennessy, C. A., Dubach, J., and Gehrt, S. D. (2012). Long-term pair bonding and genetic evidence for monogamy among urban coyotes (Canis latrans). J. Mammal. 93, 732–742. doi: 10.1644/11-MAMM-A-184.1
Heymann, E. W. (2000). “The number of adult males in callitrichine groups and its implications for callitrichine social evolution,” in Primate Males: Causes and Consequences of Variation in Group Composition, ed P. Kappeler (Cambridge: Cambridge University Press), 64–71.
Hilgartner, R., Fichtel, C., Kappeler, P. M., and Zinner, D. (2012). Determinants of pair-living in red-tailed sportive lemurs (Lepilemur ruficaudatus). Ethology, 466–479. doi: 10.1111/j.1439-0310.2012.02033.x
Hinde, K., Muth, C., Maninger, N., Ragen, B. J., Larke, R. H., Jarcho, M. R., et al. (2016). Challenges to the pair bond: neural and hormonal effects of separation and reunion in a monogamous primate. Front. Behav. Neurosci. 10:221. doi: 10.3389/fnbeh.2016.00221
Homburg, I. (1997). Ökologie und Sozialverhalten vonWeißgesichts-Sakis (Pithecia pithecia pithecia Linneaus 1766) (PhD thesis). Cuvillier Verlag, Göttingen, Germany.
Hosken, D. J., Stockley, P., Treganza, T., and Wedell, N. (2009). Monogamy and the battle of the sexes. Annu. Rev. Entomol. 54, 361–378. doi: 10.1146/annurev.ento.54.110807.090608
Houle, D., Pélabon, C., Wagner, G. P., and Hansen, T. F. (2011). Measurement and meaning in biology. Q. Rev. Biol. 86, 3–34. doi: 10.1086/658408
Houston, A. I., and McNamara, J. M. (2002). A self–consistent approach to paternity and parental effort. Philos. Trans. R. Soc. Lond. Series B. 357, 351–362. doi: 10.1098/rstb.2001.0925
Hrdy, S. (1979). Infanticide among animals: a review, classification and examination of the implications for the reproductive strategies of females. Ethol. Sociobiol. 1, 1–13. doi: 10.1016/0162-3095(79)90004-9
Huck, M., Davison, J., and Roper, T. J. (2008). Comparison of two sampling protocols and four home-range estimators using radio-tracking data from urban badgers Meles meles. Wildlife Biol. 14, 467–477. doi: 10.2981/0909-6396-14.4.467
Huck, M., and Fernandez-Duque, E. (2013). “When dads help: male behavioral care during primate infant development,” in Building Babies: Primate Development in Proximate and Ultimate Perspective, eds K. B. H. Clancy, K. Hinde, and J. N. Rutherford (New York, NY: Springer), 361–385. doi: 10.1007/978-1-4614-4060-4_16
Huck, M., and Fernandez-Duque, E. (2017). The floater's dilemma: use of space by wild solitary Azara's owl monkeys, Aotus azarae, in relation to group ranges. Anim. Behav. 127, 33–41. doi: 10.1016/j.anbehav.2017.02.025
Huck, M., Fernandez-Duque, E., Babb, P., and Schurr, T. (2014). Correlates of genetic monogamy in socially monogamous mammals: insights from Azara's owl monkeys. Proc. R. Soc. Series B. 281:20140195. doi: 10.1098/rspb.2014.0195
Huck, M., Löttker, P., Böhle, U.-R., and Heymann, E. (2005). Paternity and kinship patterns in polyandrous moustached tamarins (Saguinus mystax). Am. J. Phys. Anthropol. 127, 449–464. doi: 10.1002/ajpa.20136
Huck, M., Löttker, P., and Heymann, E. W. (2004a). Proximate mechanisms of reproductive monopolization in male moustached tamarins (Saguinus mystax). Am. J. Primatol. 64, 39–56. doi: 10.1002/ajp.20060
Huck, M., Löttker, P., and Heymann, E. W. (2004b). The many faces of helping: possible costs and benefits of infant carrying and food transfer in wild moustached tamarins (Saguinus mystax). Behaviour 141, 915–934. doi: 10.1163/1568539042265635
Huck, M., Roos, C., and Heymann, E. W. (2007). Spatio-genetic population structure in mustached tamarins, Saguinus mystax. Am. J. Phys. Anthropol. 132, 576–583. doi: 10.1002/ajpa.20559
Ivy, T., and Sakaluk, S. (2005). Polyandry promotes enhanced offspring survival in decorated crickets. Evolution 59, 152–159. doi: 10.1111/j.0014-3820.2005.tb00902.x
Jennions, M. D., and Petrie, M. (2000). Why do females mate multiply? A review of the genetic benefits. Biol. Rev. 75, 21–64. doi: 10.1017/S0006323199005423
Johnstone, R. A. (2000). Models of reproductive skew: a review and synthesis. Ethology 106, 5–26. doi: 10.1046/j.1439-0310.2000.00529.x
Jolly, A. (1966). Lemur Behavior: A Madagascar Field Study. Chicago: The University of Chicago Press.
Jones, K. E., Bielby, J., Cardillo, M., Fritz, S. A., O'Dell, J., Orme, C. D. L., et al. (2009). PanTHERIA: a species-level database of life history, ecology, and geography of extant and recently extinct mammals. Ecology 90, 2648–2648. doi: 10.1890/08-1494.1
Jurke, M. H., and Pryce, C. R. (1994). Parental and infant behaviour during early periods of infant care in Goeldi's monkey, Callimico goeldii. Anim. Behav. 48, 1095–1112. doi: 10.1006/anbe.1994.1343
Kappeler, P., and van Schaik, C. P. (2002). Evolution of primate social systems. Int. J. Primatol. 23, 707–740. doi: 10.1023/A:1015520830318
Kappeler, P. M. (2014). Lemur behaviour informs the evolution of social monogamy. Trends Ecol. Evol. 29, 591–593. doi: 10.1016/j.tree.2014.09.005
Kappeler, P. M., Clutton-Brock, T., Shultz, S., and Lukas, D. (2019). Social complexity: patterns, processes, and evolution. Behav. Ecol. Sociobiol. 73:5. doi: 10.1007/s00265-018-2613-4
Kenyon, M., Roos, C., Binh, V. T., and Chivers, D. (2011). Extrapair paternity in golden-cheeked gibbons (Nomascus gabriellae) in the secondary lowland forest of Cat Tien National Park, Vietnam. Folia Primatol. 82, 154–164. doi: 10.1159/000333143
Kinzey, W. G. (1997). “Synopsis of the new world primates: callicebus,” in New World Primates: Ecology, Evolution, and Behavior, ed W. G. Kinzey (New York, NY: Aldine de Gruyter), 213–221.
Kleiman, D. G., and Malcolm, J. R. (1981). “The evolution of male parental investment in mammals,” in Parental Care in Mammals, eds D. Gubernick and P. Klopfer (New York, NY: Plenum Press), 347–387. doi: 10.1007/978-1-4613-3150-6_9
Klug, H. (2018). Why monogamy? A review of potential ultimate drivers. Front. Ecol. Evol. 6:30. doi: 10.3389/fevo.2018.00030
Kokko, H., and Jennions, M. (2003). It takes two to tango. Trends Ecol. Evol. 18, 103–104. doi: 10.1016/S0169-5347(03)00009-0
Kokko, H., and Jennions, M. D. (2008). Parental investment, sexual selection and sex ratios. J. Evol. Biol. 21, 919–948. doi: 10.1111/j.1420-9101.2008.01540.x
Kokko, H., and Morrell, L. J. (2005). Mate guarding, male attractiveness, and paternity under social monogamy. Behav. Ecol. 16, 724–731. doi: 10.1093/beheco/ari050
Komers, P., and Brotherton, P. (1997). Female space use is the best predictor of monogamy in mammals. Proc. R. Soc. Series B 264, 1261–1270. doi: 10.1098/rspb.1997.0174
Krakauer, A. H. (2005). Kin selection and cooperative courtship in wild turkeys. Nature 434, 69–72. doi: 10.1038/nature03325
Krüger, O., Wolf, J. B. W., Jonker, R. M., Hoffman, J. I., and Trillmich, F. (2014). Disentangling the contribution of sexual selection and ecology to the evolution of size dimorphism in pinnipeds. Evolution 68, 1485–1496. doi: 10.1111/evo.12370
Kyle, C. J., Karels, T. J., Davis, C. S., Mebs, S., Clark, B., Strobeck, C., et al. (2007). Social structure and facultative mating systems of hoary marmots (Marmota caligata). Mol. Ecol. 16, 1245–1255. doi: 10.1111/j.1365-294X.2006.03211.x
Lambert, C. T., Sabol, A. C., and Solomon, N. G. (2018). Genetic monogamy in socially monogamous mammals is primarily predicted by multiple life history factors: a meta-analysis. Front. Ecol. Evol. 6:139. doi: 10.3389/fevo.2018.00139
Lappan, S. (2008). Male care of infants in a siamang (Symphalangus syndactylus) population including socially monogamous and polyandrous groups. Behav. Ecol. Sociobiol. 62, 1307–1317. doi: 10.1007/s00265-008-0559-7
Larson, S. M., Colchero, F., Jones, O. R., Williams, L., and Fernandez-Duque, E. (2016). Age and sex-specific mortality of wild and captive populations of a monogamous pair-bonded primate (Aotus azarae). Am. J. Primatol. 78, 315–325. doi: 10.1002/ajp.22408
Loison, A., Gaillard, J.-M., Pélabon, C., and Yoccoz, N. G. (1999). What factors shape sexual size dimorphism in ungulates? Evol. Ecol. Res. 1, 611–633.
Lorenz, V. R., and Heinemann, H. (1967). Beitrag zur Morphologie und körperlichen Jugendentwicklung des Springtamarin Callimico goeldii (Thomas, 1904). Folia Primatol. 6, 1–27. doi: 10.1159/000155064
Löttker, P., Huck, M., Heymann, E. W., and Heistermann, M. (2004). Endocrine correlates of reproductive status in breeding and nonbreeding wild female moustached tamarins. Int. J. Primatol. 25, 919–937. doi: 10.1023/B:IJOP.0000029129.52481.e4
Lukas, D., and Clutton-Brock, T. (2014). Evolution of social monogamy in primates is not consistently associated with male infanticide. Proc. Natl. Acad. Sci. U.S.A. 111:e1674. doi: 10.1073/pnas.1401012111
Lukas, D., and Clutton-Brock, T. (2017). Invited reply: comparative studies need to rely both on sound natural history data and on excellent statistical analysis. R. Soc. Open Sci. 4:171211. doi: 10.1098/rsos.171211
Lukas, D., and Clutton-Brock, T. H. (2013). The evolution of social monogamy in mammals. Science 341, 526–530. doi: 10.1126/science.1238677
Macdonald, D. W., Campbell, L. A. D., Kamler, J. F., Marino, J., Werhahn, G., and Sillero-Zubiri, C. (2019). Monogamy: cause, consequence, or corollary of success in wild canids? Front. Ecol. Evol., 7:341. doi: 10.3389/fevo.2019.00341
Maninger, N., Mendoza, S. P., Williams, D. R., Mason, W. A., Cherry, S. R., Rowland, D. J., et al. (2017). Imaging, behavior and endocrine analysis of “jealousy” in a monogamous primate. Front. Ecol. Evol. 5:119. doi: 10.3389/fevo.2017.00119
McNeilly, A. S. (2006). “Suckling and the control of gonadotropin secretion,” in Knobil and Neill's Physiology of Reproduction, ed J. D. Neill (London: Elsevier Academic Press), 2511–2551. doi: 10.1016/B978-012515400-0/50051-8
Mitani, J. (1985a). Gibbon song duets and intergroup spacing. Behaviour 92, 59–96. doi: 10.1163/156853985X00389
Mitani, J. (1985b). Responses of gibbons (Hylobates muelleri) to self, neighbor, and stranger song duets. Int. J. Primatol. 6, 193–200. doi: 10.1007/BF02693653
Mitani, J. C., Gros-Louis, J., and Manson, J. H. (1996). Number of males in primate groups: comparative tests of competing hypotheses. Am. J. Primatol. 38, 315–332.
Møller, A. P. (2003). “The evolution of monogamy: mating relationships, parental care and sexual selection,” in Monogamy, eds U. H. Reichard and C. Boesch (Cambridge: Cambridge University Press), 29–41. doi: 10.1017/CBO9781139087247.002
Müller, A. E., and Thalmann, U. (2000). Origin and evolution of primate social organisation: a reconstruction. Biol. Rev. Camb. Philos. Soc. 75, 405–435. doi: 10.1017/S0006323100005533
Newton, P. (1994). Social stability and change among forest Hanuman langurs (Presbytis entellus). Primates 39, 489–498. doi: 10.1007/BF02381957
Norconk, M. A. (2007). “Sakis, uakaris, and titi monkeys: Behavioral diversity in a radiation of seed predators” in Primates in Perspective. eds C. J. Campbell, A. Fuentes, K. C. Mackinnon, M. Panger, and S. K. Bearder (Oxford: Oxford University Press).
Oerke, A.-K., Heistermann, M., Küderling, I., Martin, R. D., and Hodges, J. K. (2003). Monitoring reproduction in Callitrichidae by means of ultrasonography. Evol. Anthropol. 11, 183–185. doi: 10.1002/evan.10087
Opie, C., Atkinson, Q. D., Dunbar, R. I., and Shultz, S. (2014). Reply to Lukas and Clutton-Brock: infanticide still drives primate monogamy. Proc. Natl. Acad. Sci. U.S.A. 111:E1675. doi: 10.1073/pnas.1403165111
Opie, C., Atkinson, Q. D., Dunbar, R. I. M., and Shultz, S. (2013a). Male infanticide leads to social monogamy in primates. Proc. Natl. Acad. Sci. U.S.A. 110, 13328–13332. doi: 10.1073/pnas.1307903110
Opie, C., Atkinson, Q. D., Dunbar, R. I. M., and Shultz, S. (2013b). Reply to Dixson: infanticide triggers primate monogamy. Proc. Natl. Acad. Sci. U.S.A. 110:E4938. doi: 10.1073/pnas.1319662110
Patterson, S. K., Sandel, A. A., Miller, J. A., and Mitani, J. C. (2014). Data quality and the comparative method: the case of primate group size. Int. J. Primatol. 35, 990–1003. doi: 10.1007/s10764-014-9777-1
Port, M., and Johnstone, R. A. (2013). Facing the crowd: intruder pressure, within-group competition, and the resolution of conflicts over group-membership. Ecol. Evol. 3, 1209–1218. doi: 10.1002/ece3.533
Port, M., and Kappeler, P. M. (2010). The utility of reproductive skew models in the study of male primates, a critical evaluation. Evol. Anthropol. 19, 46–56. doi: 10.1002/evan.20243
Port, M., Kappeler, P. M., Johnstone, R. A., Sherratt, A. E. T. N., and Bronstein, E. J. L. (2011). Communal defense of territories and the evolution of sociality. Am. Nat. 178, 787–800. doi: 10.1086/662672
Port, M., Schülke, O., and Ostner, J. (2017). From individual to group territoriality: competitive environments promote the evolution of sociality. Am. Nat. 189, E46–E57. doi: 10.1086/690218
Port, M., Schülke, O., and Ostner, J. (2018). Reproductive tolerance in male primates: old paradigms and new evidence. Evol. Anthropol. 27, 107–120. doi: 10.1002/evan.21586
Porter, A. M., Grote, M. N., Isbell, L. A., Fernandez-Duque, E., and Di Fiore, A. (2017). Delayed dispersal and immigration in equatorial sakis (Pithecia aequatorialis): factors in the transition from pair- to group-living. Folia Primatol. 88, 11–27. doi: 10.1159/000464147
Powell, L. E., Isler, K., and Barton, R. A. (2017). Re-evaluating the link between brain size and behavioural ecology in primates. Proc. R. Soc. B 284:20171765. doi: 10.1098/rspb.2017.1765
Puffer, A. M., Fite, J. E., French, J. A., Rukstalis, M., Hopkins, E. C., and Patera, K. J. (2004). Influence of the mother's reproductive state on the hormonal status of daughters in marmosets (Callithrix kuhlii). Am. J. Primatol. 64, 29–37. doi: 10.1002/ajp.20059
Reeve, H. K., Emlen, S. T., and Keller, L. (1998). Reproductive sharing in animal societies: reproductive incentives or incomplete control by dominant breeders? Behav. Ecol. 9, 267–278. doi: 10.1093/beheco/9.3.267
Reeve, H. K., and Ratnieks, F. L. W. (1993). “Queen–queen conflicts in polygynous societies: mutual tolerance and reproductive skew,” in Queen Number and Sociality in Insects, ed L. Keller (Oxford: Oxford University Press), 45–85.
Reichard, U. (1995). Extra-pair copulations in a monogamous gibbon (Hylobates lar). Ethology 100, 99–112. doi: 10.1111/j.1439-0310.1995.tb00319.x
Reichard, U., and Barelli, C. (2008). Life history and reproductive strategies of Khao Yai Hylobates lar: implications for social evolution in apes. Int. J. Primatol. 29, 823–844. doi: 10.1007/s10764-008-9285-2
Reichard, U. H. (2003a). “Monogamy: past and present,” in Monogamy, eds U. H. Reichard and C. Boesch (Cambridge: Cambridge University Press), 3–26. doi: 10.1017/CBO9781139087247.001
Reichard, U. H. (2003b). “Social monogamy in gibbons: The male perspective,” in Monogamy, eds U. H. Reichard and C. Boesch (Cambridge: Cambridge University Press), 190–213. doi: 10.1017/CBO9781139087247.013
Reichard, U. H. (2017). “Monogamy,” in The International Encyclopedia of Primatology, eds M. Bezanson, K. C. MacKinnon, E. Riley, C. J. Campbell, K. A. I. A. Nekaris, A. Estrada, et al. (Hoboken, NJ, USA: John Wiley & Sons, Inc.), 831–835.
Rice, M. A., Restrepo, L. F., and Ophir, A. G. (2018). When to cheat: modeling dynamics of paternity and promiscuity in socially monogamous prairie voles (Microtus ochrogaster). Front. Ecol. Evol. 6:141. doi: 10.3389/fevo.2018.00141
Rogers, F. D., Rhemtulla, M., Ferrer, E., and Bales, K. L. (2018). Longitudinal trajectories and inter-parental dynamics of prairie vole biparental care. Front. Ecol. Evol. 6:73. doi: 10.3389/fevo.2018.00073
Rowe, N., and Myers, M. (2011). All The World's Primates [online]. Available online at: http://alltheworldsprimates.org
Rowe, N., and Myers, M. (2012). All The World's Primates [online]. Available online at: http://alltheworldsprimates.org
Rubenstein, D. R., Botero, C. A., and Lacey, E. A. (2016). Discrete but variable structure of animal societies leads to the false perception of a social continuum. R. Soc. Open Sci. 3:160147. doi: 10.1098/rsos.160147
Rutberg, A. (1983). The evolution of monogamy in primates. J. Theor. Biol. 104, 93–112. doi: 10.1016/0022-5193(83)90403-4
Sabol, A. C., Solomon, N. G., and Dantzer, B. (2018). How to study socially monogamous behavior in secretive animals? Using social network analyses and automated tracking systems to study the social behavior of prairie voles. Front. Ecol. Evol. 6:178. doi: 10.3389/fevo.2018.00178
Sandel, A. A., Miller, J. A., Mitani, J. C., Nunn, C. L., Patterson, S. K., and Garamszegi, L. Z. (2016). Assessing sources of error in comparative analyses of primate behavior: intraspecific variation in group size and the social brain hypothesis. J. Hum. Evol. 94, 126–133. doi: 10.1016/j.jhevol.2016.03.007
Savage, A., Giraldo, L., Soto, L., and Snowdon, C. (1996). Demography, group composition, and dispersal in wild cotton-top tamarin (Saguinus oedipus) groups. Am. J. Primatol. 38, 85–100.
Say, L., Devillard, S., Natoli, E., and Pontier, D. (2002). The mating system of feral cats (Felis catus L.) in a sub-Antarctic environment. Polar Biol. 25, 838–842. doi: 10.1007/s00300-002-0427-2
Schacht, R., and Bell, A. V. (2016). The evolution of monogamy in response to partner scarcity. Sci. Rep. 6:32472. doi: 10.1038/srep32472
Schacht, R., Davis, H. E., and Kramer, K. L. (2018). Patterning of paternal investment in response to socioecological change. Front. Ecol. Evol. 6:142. doi: 10.3389/fevo.2018.00142
Schradin, C. (2017). Comparative studies need to rely both on sound natural history data and on excellent statistical analysis. R. Soc. Open Sci. 4:170346. doi: 10.1098/rsos.170346
Schülke, O., and Kappeler, P. M. (2003). So near and yet so far: territorial pairs but low cohesion between pair partners in a nocturnal lemur, Phaner furcifer. Anim. Behav. 65, 331–343. doi: 10.1006/anbe.2003.2018
Schülke, O., and Ostner, J. (2012). “Ecological and social influences on sociality,” in The Evolution of Primate Societies, eds J. C. Mitani, J. Call, P. M. Kappeler, R. A. Palombit, and J. B. Silk (Chicago, IL: University of Chicago Press), 269–292.
Shanee, S., and Shanee, N. (2011). Observations of terrestrial behavior in the Peruvian night monkey (Aotus miconax) in an anthropogenic landscape, La Esperanza, Peru. Neotrop. Primates. 18, 55–58. doi: 10.1896/044.018.0205
Shultz, S., Opie, C., and Atkinson, Q. D. (2011). Stepwise evolution of stable sociality in primates. Nature 479, 219–222. doi: 10.1038/nature10601
Shuster, S. M., and Wade, M. J. (2003). Mating Systems and Strategies. Princeton, NJ: Princeton University Press.
Shuster, S. M., Willen, R. M., Keane, B., and Solomon, N. G. (2019). Alternative mating tactics in socially monogamous prairie voles, Microtus ochrogaster. Front. Ecol. Evol. 7:7. doi: 10.3389/fevo.2019.00007
Smith, A. S., Ågmo, A., Birnie, A. K., and French, J. A. (2010). Manipulation of the oxytocin system alters social behavior and attraction in pair-bonding primates, Callithrix penicillata. Horm. Behav. 57, 255–262. doi: 10.1016/j.yhbeh.2009.12.004
Smith, R., and Jungers, W. (1997). Body mass in comparative primatology. J. Hum. Evol. 32, 523–559. doi: 10.1006/jhev.1996.0122
Smith, R. J. (2019). Living with observational data in biological anthropology. Am. J. Phys. Anthropol. 169, 591–598. doi: 10.1002/ajpa.23862
Snowdon, C. T., Ziegler, T. E., and Widowski, T. M. (1993). Further hormonal suppression of eldest daughter cotton-top tamarins following birth of infants. Am. J. Primatol. 31, 11–21. doi: 10.1002/ajp.1350310103
Snyder-Mackler, N., Alberts, S. C., and Bergman, T. J. (2012). Concessions of an alpha male? Cooperative defence and shared reproduction in multi-male primate groups. Proc. R. Soc. 279, 3788–3795. doi: 10.1098/rspb.2012.0842
Soini, P. (1982). Ecology and population dynamics of the pygmy marmoset, Cebuella pygmaea. Folia Primatol. 39, 1–21. doi: 10.1159/000156066
Soini, P. (1986). A synecological study of a primate community in the Pacaya-Samiria National Reserve, Peru. Primate Conserv. 7, 63–71.
Soltis, J. (2002). Do primate females gain nonprocreative benefits by mating with multiple males? Theoretical and empirical considerations. Evol. Anthropol. 11, 187–197. doi: 10.1002/evan.10025
Sommer, S. (2003). “Social and reproductive monogamy in rodents: the case of the Malagasy giant jumping rat (Hypogeomys antimena),” in Monogamy: Mating Strategies and Partnerships in Birds, Humans and Other Mammals, eds U. H. Reichard and C. Boesch (Cambridge: Cambridge University Press), 109–124. doi: 10.1017/CBO9781139087247.007
Sousa, M. B., Albuquerque, A. C., Albuquerque Fda, S., Araujo, A., Yamamoto, M. E., and Arruda, M. de F. (2005). Behavioral strategies and hormonal profiles of dominant and subordinate common marmoset (Callithrix jacchus) females in wild monogamous groups. Am. J. Primatol. 67, 37–50. doi: 10.1002/ajp.20168
Spence-Aizenberg, A., Di Fiore, A., and Fernandez-Duque, E. (2016). Social monogamy, male–female relationships, and biparental care in wild titi monkeys (Callicebus discolor). Primates 57, 103–112. doi: 10.1007/s10329-015-0489-8
Spence-Aizenberg, A., Kimball, B. A., Williams, L. E., and Fernandez-Duque, E. (2018). Chemical composition of glandular secretions from a pair-living monogamous primate: sex, age, and gland differences in captive and wild owl monkeys (Aotus spp.). Am. J. Primatol. 80:e22730. doi: 10.1002/ajp.22730
Sterck, E., Watts, D., and van Schaik, C. P. (1997). The evolution of female social relationships in nonhuman primates. Behav. Ecol. Sociobiol. 41, 291–309. doi: 10.1007/s002650050390
Sussman, R. W. (2000). Primate Ecology and Social Structure, Volume 2: New World Monkeys. Needham Heights, MA: Pearson Custom Publishing.
Symonds, M. R. E. (2002). The effects of topological inaccuracy in evolutionary trees on the phylogenetic comparative method of independent contrasts. Syst. Biol. 51, 541–553. doi: 10.1080/10635150290069977
Tanaka, H., Frommen, J. G., Koblmüller, S., Sefc, K. M., McGee, M., Kohda, M., et al. (2018). Evolutionary transitions to cooperative societies in fishes revisited. Ethology 124, 777–789. doi: 10.1111/eth.12813
Tang-Martinez, Z., Fedigan, L. M., and Strum, S. C. (2000). “Paradigms and primates: Bateman's Principle, passive females, and perspectives from other taxa,” in Primate Encounters: Models of Science, Gender, and Society (Chicago, IL: University of Chicago Press), 261–274.
Tang-Martinez, Z., and Ryder, T. B. (2005). The problem with paradigms: Bateman's worldview as a case study. Integr. Comp. Biol. 45, 821–830. doi: 10.1093/icb/45.5.821
Tecot, S. R., Singletary, B., and Eadie, E. (2016). Why “monogamy” isn't good enough: pair-living, pair-bonding, and monogamy. Am. J. Primatol. 78, 340–354. doi: 10.1002/ajp.22412
Thompson, C. L. (2016). To pair or not to pair: sources of social variability with white-faced saki monkeys (Pithecia pithecia) as a case study. Am. J. Primatol. 78, 561–572. doi: 10.1002/ajp.22360
Thrall, P. H., Antonovics, J., and Dobson, A. P. (2000). Sexually transmitted diseases in polygynous mating systems: prevalence and impact on reproductive success. Proc. R. Soc. Series B 267, 1555–1563. doi: 10.1098/rspb.2000.1178
Trivers, R. L. (1972). “Parental investment and sexual selection,” in Sexual Selection and the Descent Of Man, ed. B. Campbell (Chicago, IL: Aldine).
Trumbo, S. T., and Eggert, A.-K. (1994). Beyond monogamy: territory quality influences sexual advertisement in male burying beetles. Anim. Behav. 48, 1043–1047. doi: 10.1006/anbe.1994.1337
Valomy, M., Hayes, L. D., and Schradin, C. (2015). Social organization in Eulipotyphla: evidence for a social shrew. Biol. Lett. 11:20150825. doi: 10.1098/rsbl.2015.0825
Van Belle, S., Fernandez-Duque, E., and Di Fiore, A. (2016). Demography and life history of wild red titi monkeys (Callicebus discolor) and equatorial sakis (Pithecia aequatorialis) in Amazonian Ecuador: a 12-year study. Am. J. Primatol. 78, 204–215. doi: 10.1002/ajp.22493
Van Belle, S., Porter, A., Fernandez-Duque, E., and Di Fiore, A. (2018). Ranging behavior and potential for territoriality in equatorial sakis (Pithecia aequatorialis) in Amazonian Ecuador. Am. J. Phys. Anthropol. 167, 701–712. doi: 10.1002/ajpa.23645
van Noordwijk, M. A., and van Schaik, C. P. (2000). “Reproductive patterns in eutherian mammals: Adaptations against infanticide?” in Infanticide by Males and its Implications, eds C. P. van Schaik and C. H. Janson (Cambridge: Cambridge University Press), 361–387.
van Schaik, C. P. (1989). “The ecology of social relationships amongst female primates,” in Comparative Socioecology: The Behavioural Ecology of Humans and Other Mammals, eds V. Standen and R. A. Foley (Oxford: Blackwell Scientific Publications), 195–218.
van Schaik, C. P. (2000). “Vulnerability to infanticide by males: patterns among mammals,” in Infanticide by Males and its Implications, eds C. P. Van Schaik and C. Janson (Cambridge, UK: Cambridge University Press), 61–71. doi: 10.1017/CBO9780511542312
van Schaik, C. P., and Dunbar, R. I. M. (1990). The evolution of monogamy in large primates: a new hypothesis and some crucial tests. Behaviour, 115, 30–61. doi: 10.1163/156853990X00284
van Schaik, C. P., Hodges, J. K., and Nunn, C. L. (2000). “Paternity confusion and the ovarian cycles of female primates,” in Infanticide by Males and its Implications, eds C. P. van Schaik and C. H. Janson (Cambridge: Cambridge University Press), 361–387. doi: 10.1017/CBO9780511542312.017
van Schaik, C. P., and Janson, C. H. (2000). “Infanticide by males: prospectus,” in Infanticide by Males and its Implications, eds C. P. Van Schaik and C. H. Janson (Cambridge: Cambridge University Press), 1–6. doi: 10.1017/CBO9780511542312.002
van Schaik, C. P., and Kappeler, P. M. (2003). “The evolution of social monogamy in primates,” in Monogamy, eds U. H. Reichard and C. Boesch (Cambridge: Cambridge University Press), 59–80. doi: 10.1017/CBO9781139087247.004
Vié, J.-C., Richard-Hansen, C., and Fournier-Chambrillon, C. (2001). Abundance, use of space, and activity patterns of white-faced sakis (Pithecia pithecia) in French Guiana. Am. J. Primatol. 55, 203–221. doi: 10.1002/ajp.1055.
vonHoldt, B. M., Stahler, D. R., Smith, D. W., Earl, D. A., Pollinger, J. P., and Wayne, R. K. (2008). The genealogy and genetic viability of reintroduced Yellowstone grey wolves. Mol. Ecol. 17, 252–274. doi: 10.1111/j.1365-294X.2007.03468.x
Wade, M. J., and Shuster, S. M. (2002). The evolution of parental care in the context of sexual selection: a critical reassessment of parental investment theory. Am. Nat. 160, 285–292. doi: 10.1086/341520
Wartmann, F. M., Juárez, C. P., and Fernandez-Duque, E. (2014). Size, site fidelity, and overlap of home ranges and core areas in the socially monogamous owl monkey (Aotus azarae) of northern Argentina. Int. J. Primatol. 35, 919–939. doi: 10.1007/s10764-014-9771-7
Wickler, W., and Seibt, U. (1983). “Chapter 2: Monogamy: an ambiguous concept,” in Mate Choice, ed. P. Bateson (Cambridge: Cambridge University Press), 33–50.
Wigby, S., and Chapman, T. (2005). Sex peptide causes mating costs in female Drosophila melanogaster. Curr. Biol. 15, 316–321. doi: 10.1016/j.cub.2005.01.051
Wikipedia (2019). Monogamy in Animals. Available online at: https://en.wikipedia.org/wiki/Monogamy_in_animals (accessed June 1, 2019).
Wittenberger, J. F., and Tilson, R. L. (1980). The evolution of monogamy: hypotheses and evidence. Annu. Rev. Ecol. Syst. 11, 197–232. doi: 10.1146/annurev.es.11.110180.001213
Wright, P. C. (1996). “The Neotropical primate adaptation to nocturnality,” in Adaptive Radiations of Neotropical Primates, eds M. A. Norconk, A. L. Rosenberger, and P. A. Garber (Boston, MA: Springer US).
Yamamoto, M. E., Araújo, A., de Sousa, M. B. C., Arruda, M., and de, F. (2010). Social organization in Callithrix jacchus: cooperation and competition. Adv. Study Behav. 42, 259–273. doi: 10.1016/S0065-3454(10)42008-2
Young, A. J., Spong, G., and Clutton-Brock, T. (2007). Subordinate male meerkats prospect for extra-group paternity: alternative reproductive tactics in a cooperative mammal. Proc. R. Soc. 274, 1603–1609. doi: 10.1098/rspb.2007.0316
Zhao, Q., Tan, C. L., and Pan, W. (2008). Weaning age, infant care, and behavioral development in Trachypithecus leucocephalus. Int. J. Primatol. 29, 583–591. doi: 10.1007/s10764-008-9255-8
Keywords: monogamy, paternal care, aotus, callicebus, pithecia, primates, pair bonding, pair-living
Citation: Huck M, Di Fiore A and Fernandez-Duque E (2020) Of Apples and Oranges? The Evolution of “Monogamy” in Non-human Primates. Front. Ecol. Evol. 7:472. doi: 10.3389/fevo.2019.00472
Received: 07 June 2019; Accepted: 21 November 2019;
Published: 10 January 2020.
Edited by:
Alexander G. Ophir, Cornell University, United StatesReviewed by:
Dieter Lukas, Max Planck Institute for Evolutionary Anthropology, GermanyCopyright © 2020 Huck, Di Fiore and Fernandez-Duque. This is an open-access article distributed under the terms of the Creative Commons Attribution License (CC BY). The use, distribution or reproduction in other forums is permitted, provided the original author(s) and the copyright owner(s) are credited and that the original publication in this journal is cited, in accordance with accepted academic practice. No use, distribution or reproduction is permitted which does not comply with these terms.
*Correspondence: Maren Huck, bS5odWNrQGRlcmJ5LmFjLnVr
Disclaimer: All claims expressed in this article are solely those of the authors and do not necessarily represent those of their affiliated organizations, or those of the publisher, the editors and the reviewers. Any product that may be evaluated in this article or claim that may be made by its manufacturer is not guaranteed or endorsed by the publisher.
Research integrity at Frontiers
Learn more about the work of our research integrity team to safeguard the quality of each article we publish.