- 1Department of Earth Sciences, Carleton University, Ottawa, ON, Canada
- 2Palaeobiology, Canadian Museum of Nature, Ottawa, ON, Canada
- 3Department of Geology and Paleontology, National Museum of Nature and Science, Tsukuba, Japan
- 4Graduate School of Life and Environmental Sciences, University of Tsukuba, Tsukuba, Japan
In the present study, a series of phylogenetic analyses of morphological, molecular, and combined morphological-molecular datasets were conducted to investigate the relationships of 23 extant and 44 fossil caniforme genera, in order to test the phylogenetic position of putative stem pinniped Puijila within a comprehensive evolutionary framework. With Canis as an outgroup, a Bayesian Inference analysis employing tip-dating of a combined molecular-morphological (i.e., Total Evidence) dataset recovered a topology in which musteloids are the sister group to a monophyletic pinniped clade, to the exclusion of ursids, and recovered Puijila and Potamotherium along the stem of Pinnipedia. A similar topology was recovered in a parsimony analysis of the same dataset. These results suggest the pinniped stem may be expanded to include additional fossil arctoid taxa, including Puijila, Potamotherium, and Kolponomos. The tip-dating analysis suggested a divergence time between pinnipeds and musteloids of ~45.16 million years ago (Ma), though a basal split between otarioids and phocids is not estimated to occur until ~26.52 Ma. These results provide further support for prolonged freshwater and nearshore phases in the evolution of pinnipeds, prior to the evolution of the extreme level of aquatic adaptation displayed by extant taxa. Ancestral character state reconstruction was used to investigate character evolution, to determine the frequency of reversals and parallelisms characterizing the three extant clades within Pinnipedia. Although the phylogenetic analyses did not directly provide any evidence of parallel evolution within the pinniped extant families, it is apparent from the inspection of previously-proposed pinniped synapomorphies, within the context of a molecular-based phylogenetic framework, that many traits shared between extant pinnipeds have arisen independently in the three clades. Notably, those traits relating to homodonty and limb-bone specialization for aquatic locomotion appear to have multiple origins within the crown group, as suggested by the retention of the plesiomorphic conditions in early-diverging fossil members of the three extant families. Thus, while the present analysis identifies a new suite of morphological synapomorphies for Pinnipedia, the frequency of reversals and other homoplasies within the clade limit their diagnostic value.
Introduction
From the 1960's until the late 1980's, pinnipeds (seals, sea lions, and walruses) were widely believed to be diphyletic, based partly on the divergent locomotory styles and morphologies observed in extant taxa (Mivart, 1885; McLaren, 1960). A diphyletic view aligns phocids with musteloids (weasels, raccoons, and allies), and grouped otarioids (otariids: fur seals and sea lions; and odobenids: walrus and kin) with ursoids (bears) (Figure 1A; Ray, 1976; Tedford, 1976; Repenning and Tedford, 1977; de Muizon, 1982a,b; Wozencraft, 1989; Nojima, 1990). The paradigm shifted when Wyss (1987) provided evidence from inner ear morphology strongly suggesting a monophyletic relationship of phocids, otariids, and odobenids (Figure 1B). Wyss' careful observation of this anatomy and subsequent recognition of pinniped homologies corroborated earlier morphological (Weber, 1904; Gregory, 1910; Davies, 1958), cytogenetic (Fay et al., 1967), and biomolecular (Sarich, 1969; Arnason, 1974; Romero-Herrera et al., 1978; de Jong and Goodman, 1982; Miyamoto and Goodman, 1986; Sato et al., 2009) work identifying pinnipeds as monophyletic. From the publication of Wyss (1987) to the present, the vast majority of phylogenetic studies have confirmed Wyss' assertion, regardless if the nature of the evidence was molecular (Dragoo and Honeycutt, 1997; Flynn and Nedbal, 1998; Flynn et al., 2005; Fulton and Strobeck, 2006; Sato et al., 2006; Yonezawa et al., 2009), morphological (Berta and Wyss, 1994; Deméré and Berta, 2002; Kohno, 2006; Furbish, 2015), or a combination of the two (Flynn et al., 2000). However, some morphological (Koretsky et al., 2016), biomechanical (Kuhn and Frey, 2012) and biogeographical (Koretsky and Barnes, 2006) studies continue to uncover evidence questioning the monophyly of pinnipeds, though these studies disregard the wealth of molecular evidence in support of monophyly.
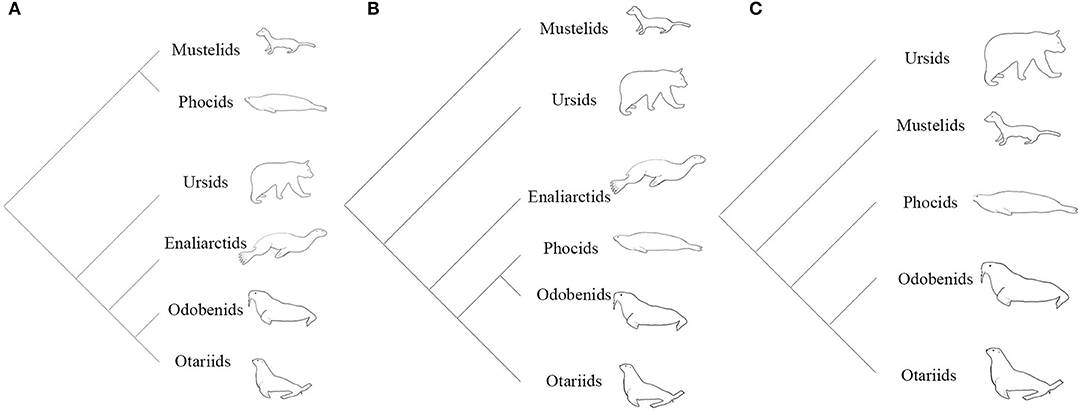
Figure 1. (A) Hypothesis of the evolutionary relationships of arctoids under a diphyletic view. (B) Hypothesis of the evolutionary relationships of arctoids under a monophyletic view based on morphological evidence (Wyss, 1987; Berta and Wyss, 1994). (C) Hypothesis of the evolutionary relationships of arctoids under a monophyletic view using molecular data (Flynn et al., 2005; Fulton and Strobeck, 2007; Sato et al., 2009).
Though pinniped monophyly has been well-supported by both molecular and morphological data, it is not apparent how phocids and otariids developed such disparate morphologies and locomotory modes. A diphyletic origin of pinnipeds serves as a compelling argument to explain the contrasting swimming styles and morphological disparity observed between otariids and phocids (Kuhn and Frey, 2012). Otariids are more ursid-like in general appearance and retain the capability of inverting their hindlimbs on land, allowing them to perform some semblance of quadrupedal ambulatory locomotion (Berta, 2012). When submerged, otariids engage in pectoral oscillations, using their enlarged foreflippers to figuratively fly through the water (English, 1976). The more fusiform phocids are unable to revert their hindlimbs forward on land, and swim using pelvic oscillations, producing thrust underwater via lateral undulations of their pelvic region and alternate protraction and retraction of their hindflippers (Fish et al., 1988; Pierce et al., 2011). The highly autapomorphic Odobenus rosmarus, the lone extant member of the once speciose Odobenidae, is not easily accommodated into either group. Odobenus is more otariid-like in general appearance, but shares a peculiar suite of features with phocids, not found in otariids (Wyss, 1987; Berta and Wyss, 1994). Odobenus displays an intermediary swimming style, using pectoral oscillations at slow speeds and pelvic undulations at high speeds (Gordon, 1981). Interpretation of fossil evidence led diphyly proponents to identify Odobenus as a highly-derived otarioid, a notion supported by molecular studies, though not within the framework of a diphyletic Pinnipedia (Flynn et al., 2000; Fulton and Strobeck, 2007).
The evolutionary relationships advocated by proponents of pinniped diphyly were initially not subject to the rigor of appropriate cladistic methods (McLaren, 1960). Later studies invoking cladistic methods continued to recover these relationships, though these analyses typically excluded characters that may have arisen in parallel due to the functional constraints associated with aquatic specialization (Tedford, 1976; de Muizon, 1982a). Pinniped diphyly is supported by a small number of morphological features shared between otarioids and ursids on the one hand, and phocids and mustelids on the other hand (McLaren, 1960; Tedford, 1976; de Muizon, 1982a). The features shared between otarioids and phocids, largely in the postcranial skeleton and auditory region, were disregarded due to their perceived susceptibility to homoplasy (Tedford, 1976), a notion later shown to be untenable within an appropriate phylogenetic framework (Wyss, 1987, 1988). Cladistic analyses of large morphological datasets have unilaterally supported pinniped monophyly (Berta and Wyss, 1994). However, such analyses have had difficulty establishing the relationship of pinnipeds to other arctoids, as their results are typically incongruent with the relationships identified by molecular analyses (e.g., Berta and Wyss, 1994 vs. Bininda-Emonds et al., 1999; Fulton and Strobeck, 2007). This problem is exacerbated by the paucity of fossil remains representing early-diverging pinnipeds that could fill the gap between them and any putative arctoid clade (Deméré et al., 2003).
Since the establishment of pinniped monophyly (Wyss, 1987, 1988; Berta et al., 1989), the most contentious issue in pinniped systematics has been the placement of pinnipeds within Arctoidea. Phylogenetic analyses of morphological data are in disagreement over the sister group of pinnipeds, with musteloids and ursids both presenting compelling cases. Identification of Ursidae as the sister group to pinnipeds was a minor component of early hypotheses of pinniped monophyly (Wyss, 1987; Berta and Wyss, 1994), as the most recent common ancestor of pinnipeds was envisioned as ursid-like. Enaliarctos, the earliest-diverging pinnipedimorph known at the time, possesses many features which were thought to characterize ursids ancestrally, including a shelf-like P4 protocone, a labiolingually-restricted M1, and a deep lateral basioccipital embayment for the inferior petrosal sinus (Mitchell and Tedford, 1973; Flynn et al., 1988; Hunt and Barnes, 1994). However, Wolsan (1993) and Kohno (1993, 1994, 1996a) recovered musteloids as the sister group to pinnipeds, a pairing strongly supported by molecular data (Figure 1C; Flynn et al., 2005; Fulton and Strobeck, 2007; Sato et al., 2009, 2012; Doronina et al., 2015).
Puijila darwini, uncovered from lacustrine deposits in the High Arctic, was originally proposed to be a transitional pinniped (Rybczynski et al., 2009), filling in the gap between the fully-flippered Enaliarctos and terrestrial carnivorans. This hypothesis was based on a relatively small parsimony analysis, employing exclusively morphological characters, investigating the relationships of Puijila to terrestrial and semi-aquatic arctoids. However, this interpretation requires a more comprehensive phylogenetic analysis (Diedrich, 2011; Berta, 2012; Boessenecker and Churchill, 2013; Koretsky and Domning, 2014; Koretsky et al., 2016). In the initial analysis (Rybczynski et al., 2009), Puijila was recovered in a clade with Enaliarctos, Potamotherium, and Amphicticeps, thereby supporting the notion Puijila is either a pinniped, a pinnipedimorph, or an earlier-diverging member of this clade. However, an extensive sampling of crown-group pinnipeds was not included.
Many comparative analyses of carnivorans, including phylogenetic investigations, have excluded pinnipeds (Bininda-Emonds and Gittleman, 2000), with researchers concerned the extreme aquatic adaptations of pinnipeds may obscure functional patterns across terrestrial carnivorans. Moreover, analyses investigating the interrelationships of pinnipeds and other arctoids have been limited to extant/crown taxa. Morphological analyses have included only broadly-defined “Mustelidae,” “Ursidae,” and/or “Amphicyonidae” as outgroups (Kohno, 1993, 1994; Berta and Wyss, 1994). Assimilating in-group taxa into a hypothetical common ancestor is indeed more prudent than using exemplary taxa (Bininda-Emonds et al., 1998), but still requires many assumptions (e.g., monophyly), which can be easily violated by incompletely preserved extinct taxa.
Excepting Finarelli (2008), which included only a single pinniped species, phylogenetic analyses of fossil arctoids have omitted extant taxa (Wolsan, 1993; Wang et al., 2005). Such analyses have provided valuable insights into the nuanced differences between the stem-ward taxa of each clade. However, without molecular data constraining the topology, it is difficult to retrieve robustly-supported topologies and reconstruct ancestral nodes.
The present work synthesizes these detached lines of inquiry, which are made less incongruous by the inclusion of numerous extinct taxa from the respective arctoid families and subfamilies. A total-evidence tip-dating approach is employed to test the proposed allocation of Puijila darwini to the stem of Pinnipedia, and to investigate the evolutionary relationships of and patterns of character evolution associated with early-diverging pinnipeds. The addition of extinct arctoids, and a broad sampling of extant taxa, including both morphological and molecular data, allows for the reconstruction of ancestral nodes to be made from the phylogenetic method itself.
Institutional Abbreviations
AMNH, American Museum of Natural History, New York, USA; BSP, Bayerische Staatssammlung für Paleontologie und Historische Geologie, Munich, Germany; CMN, Canadian Museum of Nature, Ottawa, Canada; FMNH, Field Museum of Natural History, Chicago USA; FSL, Departement des Sciences de la Terre, Universit. Claude Bernard, Lyon, France; JODA, John Day Fossil Beds, Kimberly, USA; LACM, Natural History Museum of Los Angeles County, Los Angeles, USA; MGL, Musée Geologique Cantonal, Lausanne, Switzerland; MNHN, Institut de Paleontologie, Museum National d'Histoire Naturelle, Paris, France; NMB, Naturhistorisches Museum Basel, Basel, Switzerland; NMNS, National Museum of Nature and Science, Tsukuba, Japan; NUFV, Nunavut Fossil Vertebrate Collection (housed in CMN); ROM, Royal Ontario Museum, Ottawa, Canada; UOMNH, University of Oregon Museum of Natural History, Eugene, USA; USNM, Smithsonian Institution National Museum of Natural History; UWBM, University of Washington Burke Museum, Seattle, USA; YPM, Yale Peabody Museum, New Haven, USA.
Materials and Methods
Selection of Taxa
Appendix 1 in Supplementary Material lists the taxa coded for use in the analyses, and identifies which taxa are included in each analysis. Completeness was considered when selecting extinct taxa. For example, Eotaria, the earliest-known otariid (Boessenecker and Churchill, 2015; Velez-Juarbe, 2017), was not included, as it is known only from fragmentary mandibular and dental elements (10.29% scored). Semantor, a semi-aquatic arctoid with disputed phylogenetic affinities (Orlov, 1933), is similarly known from only post-cranial elements (14.70% scored overall), and was also excluded from the present analyses. A primary goal in the selection of extinct taxa was to sample early-diverging members of each clade (as identified in Wolsan, 1993; Berta and Wyss, 1994; Wang et al., 2005; Finarelli, 2008), following the methodological reasoning of Wang et al. (2005). A representative taxon of every known family within Arctoidea, extinct or extant, is included. See the Supplementary Information file for detailed explanations for each included taxon.
Canidae was identified as an ideal outgroup for this analysis, reflecting the sister group relationship between canids and arctoids (Tomiya and Tseng, 2016), and the availability of molecular data for the group. The earliest diverging canids are Prohesperocyon and Hesperocyon. The latter is well-represented in the fossil record, and does not display the specializations observed in later-diverging canids, so it is included in the present study. Canis lupus is included as the lone extant canid.
Total Evidence Dating
To date the phylogeny and coestimate the times and evolutionary rates, a “total-evidence dating” approach, was employed (Pyron, 2011; Ronquist et al., 2012b, 2016; Gavryushkina et al., 2016; Lee, 2016; Zhang et al., 2016; Wang et al., 2017). Also known as fossil-tip calibration, this approach allows for the simultaneous analysis of extant and extinct taxa, integrating known age ranges of extinct taxa (directly associated with fossil tips) to infer phylogeny, divergence times, and macroevolutionary parameters (Ronquist et al., 2012a). Furthermore, employing a fossilized birth-death model into a tip-dating analysis allows for the incorporation of information concerning speciation, extinction, and sampling processes (Zhang et al., 2016).
Molecular Data
Following Sato et al. (2009), nucleotide sequences of five nuclear coding genes were obtained—APOB (Apolipoprotein B), BRCA1 (Breast cancer 1, early onset), RAG1 (Recombination activating gene 1), IRBP (Interstitial retinol binding protein 3), VWF (Von Willebrand factor)—for a selection of extant arctoids (see Appendix 2 in Supplementary Material). The sequence data were downloaded from GenBank (NCBI, and accession numbers are listed in Table S3). Sequences were aligned using the MUSCLE alignment tool in Mesquite (Maddison and Maddison, 2015). To create identical lengths of each individual sequence for each taxon, sequences were manually trimmed in regions that were poorly aligned and/or displayed >50% gaps for a specific column. This ensures that when all five genes are analyzed together, homologous sites for each gene are aligned. Each aligned gene was then allocated to a discrete partition within the dataset. Data was partitioned into six units—Morphological, APOB, BRCA1, RAG1, IRBP, VWF. The Mk model was applied to the Morphological partition. To determine the evolutionary models of best fit for each data partition of molecular data, marginal likelihoods for each available model were calculated and compared separately for each partition using the stepping stone method (Xie et al., 2010), a path sampling method which obviates the controversial use of the harmonic mean method to estimate likelihood (Fan et al., 2010). Table S2 lists the models and variants that were considered, and their marginal likelihood scores when subject to a Markov Chain Monte Carlo analysis of 5,500,000 generations and 50 steps. The models with the highest average marginal likelihood score (averaged between the two runs), were selected. The GTR + gamma model was selected for VWF, APOB, and BRCA1, and the GTR + invgamma model was selected for RAG1 and IRBP. The models-of-best-fit were later applied to their relevant data partitions in the Bayesian analyses.
Molecular data was included for at least one taxon from each extant caniform family—Canidae (Canis lupus), Ursidae (Ursus arctos), Mephitidae (Mephitis mephitis), Ailuridae (Ailurus fulgens), Procyonidae (Procyon lotor), Mustelidae (Gulo gulo, Lontra canadiensis, Enhydra lutris, Neovison vison, Taxidea taxus), Odobenidae (Odobenus rosmarus), Phocidae (Cystophora cristata, Erignathus barbatus, Halichoerus grypus, Mirounga angustirostris, Monachus monachus, Phoca vitulina), and Otariidae (Arctocephalus sp., Callorhinus ursinus, Eumetopias jubatus, Otaria byronia, Zalophus californianus). The inclusion of taxa was based on the availability of their selected genes in GenBank. Gaps and missing characters were treated as missing data. Canis lupus was specified as the outgroup.
Morphological Data
Eighty-two craniomandibular characters, fifty dental characters, and seventy-two postcranial characters were coded when possible for all taxa in the analysis. While some characters are novel, the majority of characters were derived from previous phylogenetic analyses of Carnivora, including those focusing on pinnipeds (Berta and Wyss, 1994; Kohno, 1996a,b, 2006; Deméré and Berta, 2001, 2002, 2005; Boessenecker and Churchill, 2013, 2015, 2018; Amson and de Muizon, 2014; Churchill et al., 2014; Furbish, 2015), musteloids (Bryant et al., 1993; Wolsan, 1993; Ahrens, 2014; Valenciano et al., 2016), arctoids (Tedford et al., 1994; Wang et al., 2005; Finarelli, 2008), and carnivorans more broadly (Wyss and Flynn, 1993; Wesley-Hunt and Flynn, 2005; Spaulding and Flynn, 2012; Tomiya and Tseng, 2016) (see Appendix 1 in Supplementary Material for full character list). Many of these characters were then modified, either by editing existing character states or by adding character states, to better reflect the diversity of these characters across arctoids. All changes are noted in the Supplementary Information File. All taxa were specifically coded for this study (Appendix 2 in Supplementary Material).
Some phylogenetic analyses of pinnipeds (Deméré and Berta, 2002) have attempted to quantify and discretize characters that gauge nuanced differences in size and/or shape of morphological features, an approach followed in the present analysis. Further work on the morphological characters used in the study of extinct carnivorans should use statistical techniques to test the discretization or “binning” of different character states (Prieto-Marquez, 2010; Zou and Zhang, 2016).
A representative from every known arctoid family was coded, including members of the extinct Amphicyonidae, Oligobuninae and Desmatophocidae (Supplementary Table 1 in Supplementary Material). When possible, adult male specimens were selected for character coding, due to the high sexual dimorphism displayed in many modern (Gittleman and Valkenburgh, 1997) and extinct arctoids (Hunt and Skolnick, 1996; Cullen et al., 2014).
To code their character states, specimens representing the extinct taxa were observed personally. Data for certain characters were also gathered from the literature if not discernible in the specimens available to us. Those concerning the internal cranial anatomy were inferred from video files of CT scans spanning the different axes of the cranium (from www.digimorph.org) and the literature (Ahrens, 2014; Geraads and Spassov, 2016; Grohé et al., 2016).
Analysis
The total-evidence tip-dating analysis was initially run in MrBayes v3.2.6 (Huelsenbeck and Ronquist, 2001; Ronquist and Huelsenbeck, 2003) for 10,000,000 generations, with a sampling frequency of 1,000 and a diagnostic frequency of 1,000. To ensure convergence upon similar results, two independent runs of MCMC (Markov-chain Monte Carlo) were specified for each analysis, with four chains (three hot and one cold) per run. Stationarity and convergence of the posterior probabilities were gauged using Tracer v1.6 (Rambaut, 2007) for OSX. Convergence of parameters was identified when the plot of the log likelihoods (“the Trace line”) varied about a constant value. After accepting convergence, runs were considered sufficiently long when the effective sample sizes (ESS's) for each trace rose above 200. AWTY (Wilgenbusch, 2004) was offline as of this analysis, so no test of convergence of tree topologies was used, though analyses were only terminated when the average standard deviation of split frequencies was <0.01. Inspection of the trace plots also allowed us to identify the burn-in of each analysis, which was subsequently discarded. Following termination of the runs and discarding of the burn-in, the consensus tree showing all compatible clades was requested (contype = allcompat).
A relaxed clock model was implemented to account for evolutionary rate variation across branches and over time. The clock rate was associated with a lognormal prior with a mean of −7.0 and a standard deviation of 0.6 on the natural logarithm scale. The analysis specified an independent gamma rates model, and the variance increase parameter had an exponential prior with rate 10. The fossilized birth-death model was employed (Heath et al., 2014), with the following priors: speciationpr = exp(10), extinctionpr = beta(1,1), fossilizationpr = beta(1,1). The extant sample proportion was estimated to be 0.085. Treeagepr was set at 45, following Matzke and Wright (2016). The full script for this analysis is available in Appendix 3 in Supplementary Material.
Fossil stratigraphic ages, used to introduce time intervals for fossil samples, are listed in Appendix 4 in Supplementary Material. Fossil ages include ranges of uncertainty.
Ancestral character state reconstruction (ACSR) was performed for selected characters (those identified as possible pinniped or pinnipedimorph synapomorphies and homoplastic characters in this and other analyses) under the maximum likelihood approach using Mesquite v. 3.04. The Trace Character History function was applied to the phylogenetic tree recovered from total-evidence tip-dating, with the morphological characters used as the source of stored characters. The mK1 model was selected as the model of character state evolution, with equal probability for any character change. ACSR was used to identy the likely plesiomorphic condition for each character at the base of Pinnipedia, and to identify any possible reversals or instances of parallel trait development within the clade.
Parsimony Analysis of Combined Morphological-Molecular Dataset
Parsimony analysis was performed on the same dataset with the same outgroup using TNT 1.1 (Goloboff et al., 2008). One thousand replicates of random addition sequences were requested, followed by 500 iterations of tree bisection reconnection (TBR) and parsimony ratchet. Bootstrap support values (BS) were also obtained using TNT, using symmetric-resampling frequencies and 1,000 replicates.
Results and Discussion
Total-Evidence Dating
Musteloids are identified as the sister group to pinnipeds (pp = 0.31), and Puijila and Potamotherium are identified as the sister clade to Pinnipedimorpha (pp = 0.89). Otariids and Odobenids are recovered as sister taxa (pp = 0.69), and desmatophocids are identified as the sister group to the otariid-odobenid coupling (pp = 0.75), to the exclusion of phocids. The present analysis indicates a divergence date between pinnipeds and musteloids in the Eocene, ranging from the Ypresian to the early Bartonian (95% highest posterior density interval 41.17–50.3 Ma) (Figure 2). Divergence time estimates for selected nodes are listed in Table S3.
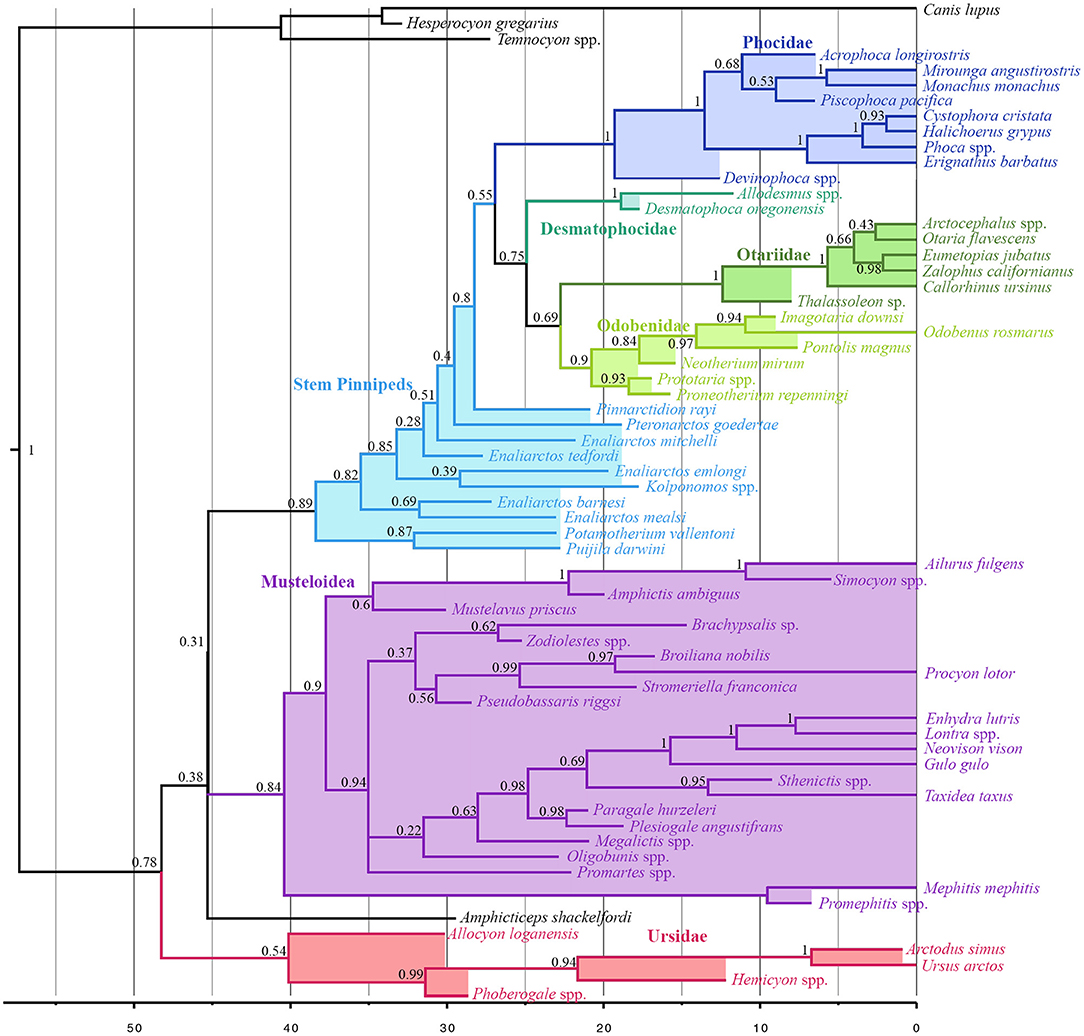
Figure 2. All compatible clades consensus tree of Total-Evidence Tip-Dating analysis using Bayesian inference. Numbers at nodes indicate posterior probability values for that node.
Divergence time estimates within crown group Pinnipedia produced by the present analysis do not stray wildly from those posited by other analyses, and in fact recover a divergence between Phocidae and Otarioidea (~26.94 Ma) roughly midway between those of Arnason et al. (2006) on the one hand, and Higdon et al. (2007) and Fulton and Strobeck (2010) on the other. The present analysis identifies an early Miocene (~22.76) split between Otariidae and Odobenidae, in agreement with that of several other analyses (Higdon et al., 2007; Yonezawa et al., 2009; Nyakatura and Bininda-Emonds, 2012), while other analyses using node calibration have recovered a later divergence date inconsistent with the ages of the earliest odobenid, Proneotherium (Fulton and Strobeck, 2010). Beyond the crown pinniped radiation, the present investigation identifies older divergence times than many other analyses employing node- or tip-dating (excepting Arnason et al., 2006).
On the other side of the pinniped-musteloid split, the oldest incontestable pinniped fossils appear in the earliest Chattian (Berta, 1991; Prothero et al., 2001), far later than both the estimated early-to-mid Eocene split from musteloids, and the estimated ~38.41 Ma divergence time between Puijila/Potamotherium and all later-diverging pinnipedimorphs (Berta, 1991; Berta and Wyss, 1994). This long gap would allow plenty of time for the establishment of morphological characteristics reflecting the pronounced level of aquatic adaptation exhibited by Enaliarctos (Berta and Ray, 1990), ostensibly following a long initial phase of evolution in a non-marine setting represented by Puijila and Potamotherium, from lacustrine and fluvial deposits, respectively.
Pinnipedimorph Taxonomy
The Pinnipedimorpha was erected by Berta (1991) and includes Enaliarctos and all of its descendants, including a monophyletic crown-group Pinnipedia, which is strongly supported in all phylogenetic analyses performed in the present study. This study finds Puijila and Potamotherium to be members of this lineage that diverged prior to Enaliarctos, suggesting that a more inclusive taxonomic definition is warranted. All phylogenetic analyses in the current study recover a monophyletic group that includes crown group pinnipeds and the stem pinnipeds recognized here (Puijila, Potamotherium, Kolponomos, Enaliarctos, Pteronarctos, Pinnarctidion). A monophyletic Pinnipedia is restricted to crown group pinnipeds in both analyses. The reasonably strong support for a monophyletic Puijila + Potamotherium + Pinnipedimorpha in both phylogenetic analyses suggests Puijila and Potamotherium may confidently be identified as stem pinnipeds. Amphicticeps shackelfordi and Allocyon loganensis, a pair of taxa previously identified as possible early-diverging members of the pinniped divergence (Tedford et al., 1994; Rybczynski et al., 2009), are also recovered along the pinniped stem in the parsimony analysis (Figure 3), though this placement is very poorly supported (BS = 2).
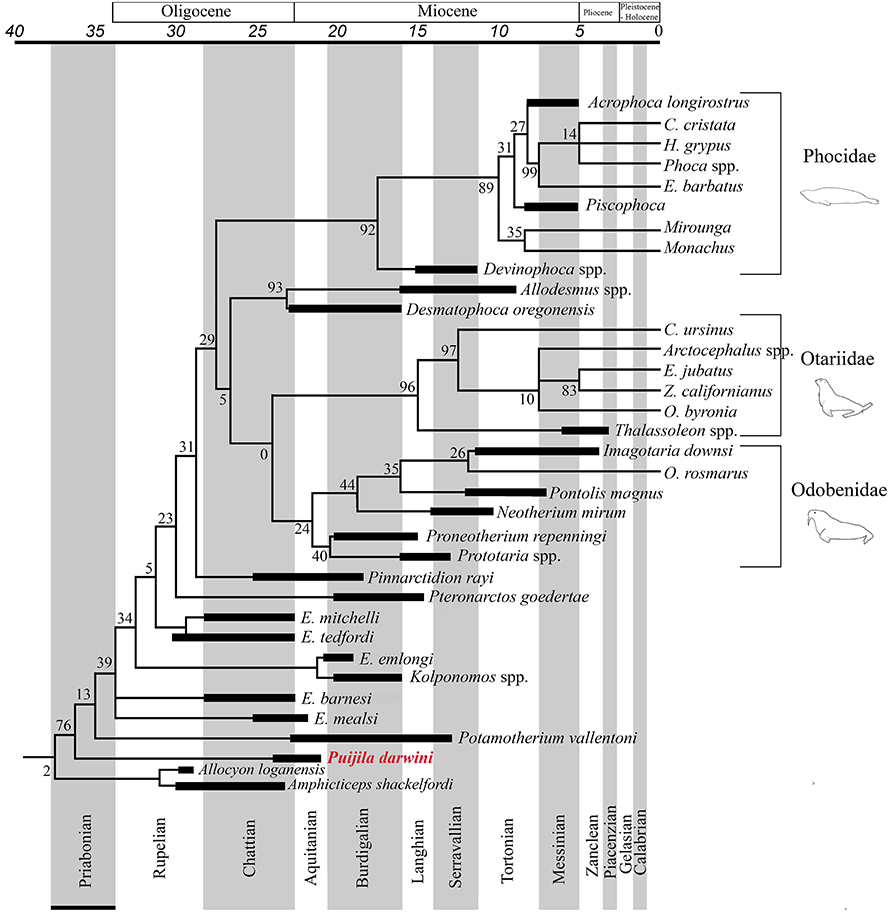
Figure 3. Pinniped branch of the strict consensus tree from the parsimony analysis of the combined molecular and morphological dataset, with a geological time-scale. Black bars indicate age ranges (including range of uncertainty) of extinct taxa.
Puijila and Potamotherium do not possess many of the conventional pinniped synapomorphies, but as potential stem pinnipedimorphs, they should not be expected to possess these features. The present Bayesian analysis suggest the features of modern pinnipeds arose sequentially, as observed in many other secondarily aquatic tetrapods (Hall, 1999), rather than as an integrated package.
Ten synapomorphies were identified for a clade of Potamotherium + Puijila + pinnipedimorphs (Table 1), eight of which are identified (>50% likelihood) at the base of the clade in the ACSR analysis. Unsurprisingly, it is the basicranial region that supports the phylogenetic affinities of Puijila and Potamotherium, as half of these possible synapomophies relate to the basicranial region. Unfortunately, data from the basicranial region is incompletely known from many fossil specimens, as the majority of basicranial characters are not preserved or are difficult to access. As these regions are of significant phylogenetic utility, conclusions on the precise phylogenetic relationships of early-diverging fossil pinnipeds may be reinterpreted once such data becomes available. Preservational biases have occasionally drastically misled identifications of fossil specimens (Donoghue and Purnell, 2009; Pattinson et al., 2014), and they can cause directional shifts in phylogenetic analyses (Sansom and Wills, 2013). Fossil specimens may appear to lack later derivations or specializations associated with more crown-ward clades, and thus, may erroneously be shifted toward the stem (Sansom, 2014).
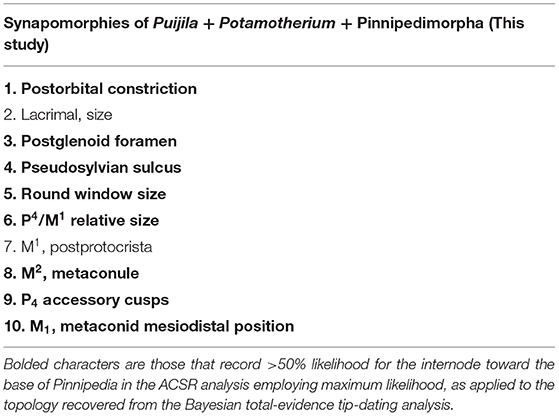
Table 1. Synapomorphies of the clade Puijila + Potamotherium + Pinnipedimorpha, as identified in the parsimony analysis of morphological data.
Many previous phylogenetic analyses identifying Potamotherium as a mustelid have been limited in their observations to just a handful of specimens (Wyss, 1987; Bininda-Emonds and Russell, 1996; Wang et al., 2005; Finarelli, 2008) or relied on Savage (1957) thorough description of the taxon (Furbish, 2015). The pinnipedimorph affinities of Potamotherium become increasingly apparent upon inspection of additional undescribed material of Potamotherium from the collections at NMB, MNHN, and FSL. Potamotherium displays several polymorphic characters, with the derived states commonly representing pinnipedimorph synapomorphies. The variable appearance of these pinnipedimorph synapomorphies suggests a transitional morphology between terrestrial arctoids and pinnipedimorphs. These features include: presence of large antorbital process, mandible deepest anteriorly, reduction of the fossa for the tensor tympani, and presence of a palatal midline ridge.
Although the group Pinnipedimorpha is well-supported, the details of the evolution of the stem lineages are less well-resolved. For example, there is considerable cranial and dental diversity between different species of Enaliarctos (Cullen et al., 2014; the present study), and significant taxonomic revision of the genus, with comparison to other stem pinnipeds overlapping Enaliarctos in age, may be warranted. Despite superficially appearing less aquatically-specialized than Potamotherium, Puijila shares many traits with Enaliarctos to the exclusion of Potamotherium, though the similarities are not sufficient to recover a closer relationship between the two in our phylogenetic analyses. Many of these traits are also observed in otarioids in general, but not phocids, including: a thin, projecting tympanic crest, an inflated and somewhat rounded caudal entotympanic, and presence of an alisphenoid canal. Specifically, the tympanic crest and tympanic bulla of Puijila strongly resemble those of Enaliarctos emlongi and Enaliarctos mealsi. We also identified the shared presence of an intrabullar septum in Puijila, Enaliarctos emlongi, and Enaliarctos mealsi. Additional traits found in Puijila, Enaliarctos, and later-diverging pinnipedimorphs, to the exclusion of Potamotherium, include: absence of I1, a reduced M1 metaconid, presence of sharp ventral keel on the axis, and presence of a cylindrical lesser tuberosity of the humerus.
The presence/absence of the alisphenoid canal has been considered as a character of significant phylogenetic utility (Tedford, 1976; Koretsky et al., 2016). The alisphenoid canal carries multiple structures, including a branch of the external carotid artery, likely protecting it from occlusion during contraction of the pterygoideus muscle (Ewer, 1973). Accordingly, the alisphenoid canal may have been lost in taxa whose carotid canals lie closer to the orbit, so that the external carotid artery is largely unexposed to the adjacent musculature. This is borne out by mustelids and mephitids, who display a reduced post-canine dentition, and in phocids, who possess large orbits. While it appears unlikely an organism could have lost the alisphenoid canal and subsequently regained it, we cannot, a priori, rule such a scenario out. Thus, the character was not specified as irreversible in our analysis, though we do admit a reversal to the presence of the canal seems unlikely. Within the topology produced by the TEA, we can assume the loss of the alisphenoid canal represents an autapomophy for Potamotherium, rather than a synapomorphy of a hypothetical Potamotherium + Phocidae clade (de Muizon, 1982a; Koretsky and Rahmat, 2015). It is likely the alisphenoid canal was lost multiple times in arctoids, including at least once in phocids, mephitids, the clade containing mustelids + procyonids, and Ailuropoda, respectively.
Reappraisal of Pinniped Synapomorphies
Pinnipedia (Illiger, 1811) encompasses the last common ancestor of otarioids and phocids, and all of its descendants (Berta and Wyss, 1994). Wyss (1987) and Berta and Wyss (1994), suggested reversals were likely more common than convergence throughout the early evolutionary history of pinnipeds. This view was based on tree topologies in which Phocidae was recovered as the sister group to Odobenidae, and Ursidae was identified as the sister group to pinnipeds. Such a view has become less tenable as molecular analyses have revised our interpretation of these relationships. Many of these putative reversals may now be reinterpreted, in light of the present analysis. Reversals now appear less common than convergences within pinnipeds, as the ACSR analysis identifies over twice as many likely parallelisms (n = 30; interpreted as a trait that arises at least once in multiple crown group clades) as likely reversals (n = 14), and a few of these reversals are in fact identified as arising in parallel in multiple crown group clades (Table S4). This notion is also supported by the morphology of the early-diverging fossil representatives of the three extant pinniped families—Prototaria (or Proneotherium), Eotaria, and Devinophoca. In spite of their possession of odobenid, otariid, and phocid synapomorphies, respectively, these taxa also possess a suite of primitive characters, lacking some of the aquatic specializations previously used to define Pinnipedia.
In light of the recent discovery of fossils attributable to Prototaria, Proneotherium, Eotaria, and Devinophoca, it appears that previously cited pinniped synapomorphies are more variable in distribution among crown group pinnipeds than previously believed, and may not define the base of Pinnipedia. Table 2 lists all previously proposed synapomorphies of crown pinnipeds (Pinnipedia), and notes if the early-diverging fossil crown members of each pinniped family display the ancestral or derived character state, and the likelihood the synapomorphy is present at the identified at the base of pinnipedia based on the ACSR of the tree derived from the total-evidence tip-dating analysis. The ancestral state is observed at least once in a crown member for 11 of the 16 characters, and seven of 16 of these features are identified as unlikely to characterize the base of Pinnipedia using ACSR. The five characters that do not display retention of an ancestral state are postcranial characters that are poorly sampled for these extinct taxa, many of which are only known from skulls, mandibles, and/or a few postcranial elements. The appearance of an ancestral state in any of these fossil forms could theoretically represent an anomalous derivation or reversal. However, if early-diverging representatives from more than one family retain the ancestral condition, then it is equally parsimonious to assume the derived condition did not characterize the base of Pinnipedia. As Table 2 demonstrates, this latter scenario describes six of the 16 characters. It must be assumed these numbers would increase if there were less missing data (currently only 44/80 total possible codings).
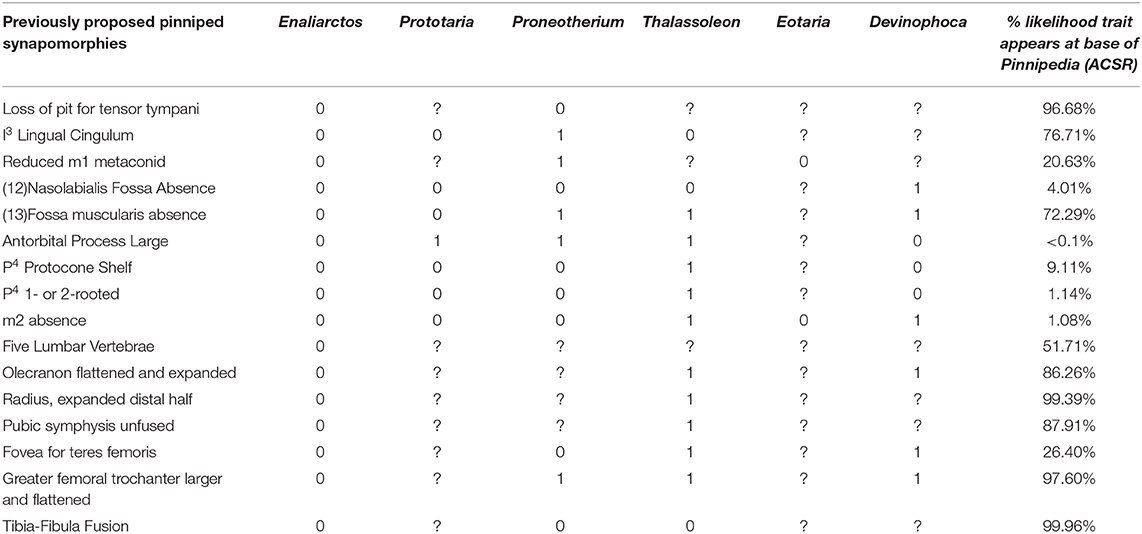
Table 2. List of previously proposed synapomorphies of crown group Pinnipedia (Berta and Wyss, 1994), and their occurrence in select fossil pinnipedimorphs.
The following features have been used to delineate a monophyletic Pinnipedia to the exclusion of the stem “pinnipedimorphs” Enaliarctos, Pteronarctos, and Pacificotaria: pit for tensor tympani absent, I3 lingual cingulum absent, M1−2 trigonid suppressed, nasolabialis fossa absent, antorbital process large, P4 protocone shelf absent, P4 one- or two-rooted, and M2 absent (Berta and Wyss, 1994).
The absence of the pit for the tensor tympani does appear to be a synapomorphy of crown pinnipeds (Wyss, 1987; Berta and Wyss, 1994), as the tensor tympani appears to insert on the Eustachian canal in all extant pinnipeds sampled. However, Wesley-Hunt and Flynn (2005) identified a transitional state in Pteronarctos, in which the fossa for the tensor tympani is present, but only shallowly excavated into the promontory. Likewise, the fossa for the tensor tympani is greatly reduced in Puijila, several specimens of Potamotherium (Mitchell and Tedford, 1973; Pers. Obs.), and multiple species of Enaliarctos (Berta, 1991). CT data of the inner ear of early-diverging otariids, phocids, and odobenids should clarify the timing of this transition to determine if the fossa for the tensor tympani was lost multiple times.
Lingual cingulum of the I3 is retained in Prototaria (Kohno, 1994; Kohno et al., 1995) and Thalassoleon (Deméré and Berta, 2005). An unreduced M1 metaconid or trigonid is retained in Eotaria (Boessenecker and Churchill, 2013). The nasolabialis fossa appears to have been lost multiple times in pinnipeds, as it is present in a stem otariid (Thalassoleon), multiple fossil odobenids (Proneotherium and Imagotaria), and a possible desmatophocid (Pinnarctidion). Polarity for a large antorbital process must now be reversed, and the character can be conceived of as a possible synapomorphy for otarioids, with further derivation in both otariids and odobenids. A protocone shelf of the P4 is retained in Devinophoca and Proneotherium (Churchill and Clementz, 2016). Reduction of the number of roots of P4 (<3) appears to be a synapomorphy of pinnipeds, however, Devinophoca may display a transitional stage in which the posterior root is bilobed, though the roots are nevertheless well-merged (Koretsky and Holec, 2002). A similar P4 root morphology is observed in Proneotherium and Neotherium. The M2 is retained in Pontolis (Deméré, 1994), Eotaria (Boessenecker and Churchill, 2013), and even atavistically reappears in extant otariids (Drehmer et al., 2004).
Of these proposed synapomorphies, only absence of the fossa for the tensor tympani and reduction of P4 roots appear to definitively define the node at the base of Pinnipedia. Berta and Wyss (1994) also listed a set of ambiguous postcranial synapomorphies of Pinnipedia, though their presence or absence in Pteronarctos and several species of Enaliarctos cannot be asserted without postcranial remains. These are: five lumbar vertebrae present, flattened and posteriorly expanded olecranon process, expanded distal end of radius, pubic symphysis unfused, loss of fovea teres femoris, greater trochanter of femur lost. These synapomorphies have not been discovered in pinnipedimorphs, nor have the plesiomorphic conditions been identified in any fossil pinnipeds. However, some of these are approached by Potamotherium and Enaliarctos, who display somewhat expanded distal radii, somewhat expanded greater trochanters, and a reduced fovea teres femoris. Unsurprisingly, many of these features are thought to be related to aquatic adaptation—general flattening of the long-bones, posterior expansion of the olecranon process, loss of the fovea teres femoris—as they are observed homoplastically in other clades of aquatic mammals (Gingerich et al., 1990, 1994).
Another more inclusive clade than the Pinnipedimorpha is also recognized—Pinnipediformes (Berta, 1994). This group encompasses Pteronarctos and Pinnipedia, to the exclusion of Enaliarctos (Berta, 1994). Four additional unambiguous synapomorphies were identified for Pinnipediformes: absence of lacrimal-jugal contact, maxilla contributes heavily to orbit, embrasure pit of P4-M1 shallow or absent, mastoid process in close proximity to paroccipital process and connected by a ridge.
The extent of the lacrimal is difficult to ascertain in fossil pinnipeds and their ancestors, as the lacrimal becomes well-fused to surrounding bones in ontogeny. It appears likely this is a pinniped synapomorphy. The discovery of juvenile specimens displaying the presence or absence of a lacrimal in Puijila or Enaliarctos could confirm or undermine this contention. The maxillary contribution to the orbit is also likely a pinniped or pinnipediforme synapomorphy, but fusion of these elements in Enaliarctos and Puijila precludes certain determination. While the embrasure pit of Proneotherium is reduced (Boessenecker and Churchill, 2013), it is nevertheless present, while all extant pinnipeds observed lack an embrasure pit outright. In phocids, the mastoid and paroccipital processes are widely separated, and the polarity of this character should be reversed, so it is considered a synapomorphy of otarioids.
Another proposed synapomorphy of Pinnipedia is the presence of prominent orbital vacuities (Wyss, 1987; Berta, 1991), which are unossified spaces on the medial orbital wall (Wyss, 1987). The presence of orbital vacuities appears to be unique to crown pinnipeds. The orbital vacuities are similar in phocids and otariids, but are placed far posteriorly in Odobenus (Kohno, 2006), though fossil walruses do possess a pinniped-like orbital vacuity (Deméré and Berta, 2001). Though similarly placed, the otariid orbital vacuity is of a different configuration than the phocid orbital vacuity (Wozencraft, 1989; Bininda-Emonds and Russell, 1996), and for that reason, Berta and Wyss (1994) speculate on an independent origin of orbital vacuities in the three pinniped families, a hypothesis supported by the present phylogenetic analysis. Orbital vacuities are absent in Pinnarctidion, present in Allodesmus (Berta and Wyss, 1994) and possibly incipient in Desmatophoca (Deméré and Berta, 2002).
Additional ambiguous synapomorphies of crown pinnipeds of Berta and Wyss (1994) include: enlarged tuberosities of the humerus, and a flattened and enlarged greater trochanter. A somewhat enlarged, though unflattened greater trochanter of the femur is observed in Thalassoleon. A somewhat enlarged lesser tubercle of the humerus that continues down the shaft as a cylindrical ridge, is observed in Puijila, Semantor, and the desmatophocids.
The earliest-diverging otariid (Thalassoleon) and phocid (Devinophoca) included in the analysis both display decidedly derived morphologies (Deméré and Berta, 2005; Koretsky et al., 2016). Yet, several character states previously believed to have been lost at the base of crown Pinnipedia are retained even in these taxa (Table 2). The earliest-diverging odobenid (Kohno, 1994; Boessenecker and Churchill, 2013) displays only a single purported pinniped synapomorphy of Berta and Wyss (1994)—presence of the antorbital process—a character which is now identified as an otarioid synapomorphy, and the specific configuration of this character in Prototaria is more appropriately considered an odobenid synapomorphy (Berta and Wyss, 1994). Deméré and Berta (2002) commented on the lack of the fovea for the teres femoris in Proneotherium, suggesting it would be expected given its transitional morphology. However, as this was offered as a pinniped synapomorphy, it should theoretically be present in Proneotherium. It would not be unexpected for a transitional odobenid to lack odobenid synapomorphies, but it would be unconceivable to observe a transitional odobenid lacking several pinniped synapomorphies, considering how few synapomorphies are identified (Berta and Wyss, 1994).
From the results of the present study, we reject many of the synapomorphies previously used to diagnose Pinnipedia, as many were founded on hypotheses of sister group relationships of Pinnipedia-Ursidae and Phocidae-Odobenidae. The present analysis recognizes a new suite of possible synapomorphies that support a monophyletic crown Pinnipedia, to the exclusion of Puijila, Potamotherium, Enaliarctos, and Pteronarctos. Admittedly, many of these possible synapomorphies cannot, at the present time, be identified in early-diverging members of the crown families due to inadequate preservation, and may turn out to represent independent derivations. Also, several of these synapomorphies require reversals to the ancestral condition in Prototaria, Proneotherium, Thalassoleon, and/or Devinophoca (synapomorphies 1, 3, 4, 6, 8, 10, 11, 12, 14 in Table 3), lessening the confidence with which we can assign them to the base of Pinnipedia. Furthermore, as with those identified by Berta and Wyss (1994), many of the synapomorphies identified here are uniquely approached by stem pinnipedimorphs, among arctoids. The stem pinnipedimorphs identified in the analysis appear to display a transitional or incipient state for many of these characters (synapomorphies 7, 8, 11, and 13 in Table 3), suggesting selective pressures favoring those traits were already present in stem pinnipedimorphs.
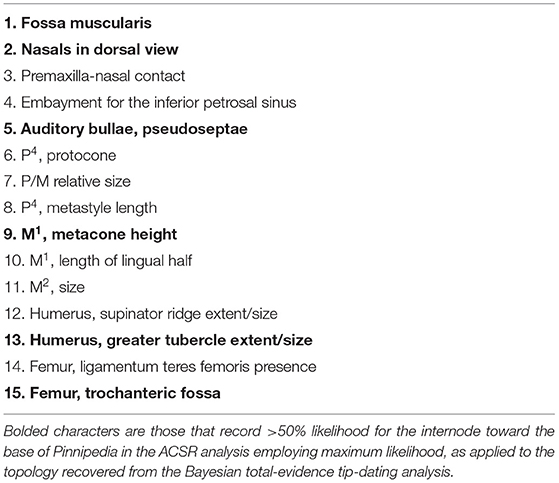
Table 3. Synapomorphies of the clade Pinnipedia, as identified in the parsimony analysis of morphological data using TNT.
Thus, while the present analysis identifies a suite of potential pinniped synapomorphies, an investigation of their distribution among extinct taxa indicates that several of these features appear to be incipiently present in stem pinnipeds and others may have arisen in parallel among the three extant pinniped families. In the absence of incontestable transitional fossils, a more thorough investigation into the alpha and beta taxonomy of these known early-diverging taxa may provide additional clues regarding the divergence of otariids and phocids.
Review of Parallel Evolution and Swimming Specialization in Arctoids
Swimming has evolved multiple times in arctoids (Berta et al., 2005). While no other known arctoids have developed the remarkable swimming specializations observed in pinnipedimorphs, many other taxa are considered to be semi-aquatic, including otters, the American mink, the European mink, the polar bear, the robust otter-like fossil musteloid Mionictis (Baskin, 1998), and, if its affinities lie outside the pinniped divergence, Kolponomos. Otters, minks and Mionictis all share with Puijila and Potamotherium a similar ectomorph bodyplan and several other features related to swimming and aquatic feeding, including webbed digits (Savage, 1957; Berta et al., 2005; Rybczynski et al., 2009). These features are so numerous that they have even previously been used to assert the lutrine affinities of Mionictis (known from the middle Miocene) (Baskin, 1998; Tseng et al., 2009) and Potamotherium, though neither of these appear possible in the light of divergence time estimate studies consistently identifying a late Miocene divergence of Lutrinae (Sato et al., 2009). Considering these arctoid taxa converged upon similar specializations related to swimming, perhaps similar shifts could have begot flippers independently in otariids, phocids, and odobenids.
Adaptive radiations were thought to characterize the stem of pinnipedimorphs (Simpson, 1944), but recent evidence, based on tests of cranial disparity (Jones et al., 2015) and molecular markers (Higdon et al., 2007), suggest rapid radiations did not occur until the otarioid-phocoid split. Subsequent adaptive radiations appear to have taken place separately as otariids and odobenids diverged. This scenario is supported by the primitive morphologies of the early-diverging members of each extant pinniped family. Most notably, the early diverging odobenids Prototaria and Proneotherium do not depart significantly, in terms of morphology, from Enaliarctos and Pteronarctos. However, the results of the total-evidence tip-dating analysis indicate relatively rapid divergence events occurred along the stem, prior to a lull in identified speciations as the clades in crown Pinnipedia began to diverge. This high level of alpha and beta diversity is also reflected in the wide geographic distribution of stem pinnipeds.
Other molecular work has found evidence of genes that appear correlated with aquatic adaptation more broadly across marine mammals (Wang et al., 2008; Zhou et al., 2015), and of parallel shifts accelerating evolutionary rates of genes in multiple marine mammal clades (Chikina et al., 2016). However, convergent evolution of genes has been difficult to detect across marine mammal clades. Parallel substitutions of functionally-enriched genes do not appear to occur more frequently between marine mammals than they do between marine and terrestrial mammals (Irwin and Árnason, 1994; Foote et al., 2015; Zhou et al., 2015). While convergent molecular evolution and convergent phenotypic evolution are both common phenomena, adaptive molecular convergence associated with phenotypic convergence is uncommon (Foote et al., 2015).
Though our phylogenetic analyses identify a number of possible synapomorphies of crown pinnipeds, it is not possible to ascertain whether or not these shared traits arose via common ancestry or due to similar selective pressures on closely-related groups of organisms. Modern cladistics methods are not infallible. While more reliable than phenetic interpretations, phylogenetic inference of morphological data remains imprecise, and thus, susceptible to systematic error. This limits the confidence we can have in topological placements from such studies, including the present study.
The Mk model has monopolized Bayesian Inference of discrete morphological data. While it has been shown to be effective in simple data sets, it may not be able to accurately model morphological evolution. The Mk model employs a continuous-time Markov process running over finite state spaces (Klopfstein et al., 2015). A Markov process is conditioned only on the active state of the process. The past and future of the process are independent of the current state. Furthermore, the Markov process is also assumed to be at stationarity and time-reversible. A process attains stationarity when the current state ceases being dependent on the starting state. A process is time-reversible when its stochasticity does not become inconsistent or ill-defined if time is reversed.
The stationarity and time-reversibility of this Markov process prevents the Mk model from accounting for directional evolution (Klopfstein et al., 2015). Directional evolution, one of the three modes of selection originally proposed by Darwin (1859), involves an extreme phenotype being favored. Such selection is believed to be strong when an organism must adapt to a drastic and sudden shift in ecological pressures. Conceptually, directional selection would be a powerful propeller for an organism beginning to adapt to an aquatic existence. Conversely, in organisms that are secondarily aquatic, or highly specialized in some other manner, it should be unlikely, though not impossible, for organisms to revert to a less specialized form.
Since the Mk model does not allow for the input of assumptions of directional selection, it is unlikely that parallel evolution would reveal itself in a topology using the Mk model. A priori assumptions of directional selection are indeed difficult to integrate into an evolutionary model, but successful work has been carried out on characterizing directional selection in molecular datasets (Merritt and Quattro, 2001; Creevey and McInerney, 2004; Nielsen, 2005; Kosiol et al., 2008; McClellan, 2013; Enard et al., 2014; Bloom, 2017), allowing directional selection to be integrated into models of molecular evolution (Huelsenbeck et al., 2006; Ronquist et al., 2012b). Unfortunately, the non-objective nature of morphological characters and datasets, as outlined earlier in this chapter, makes it difficult to characterize directional selection as it relates to adaptive phenotypes. The extent of parallel evolution in pinnipeds may thus be greater than that suggested by the present study and others. It is possible that the extreme aquatic specializations characterizing extant pinnipeds, including flippers, developed in parallel in otariids, odobenids, and phocids, but our current phylogenetic methods are not capable of detecting such an event.
Conclusion
Pinnipedimorpha, as currently defined, includes the most recent common ancestor of Enaliarctos and all of its descendents. The phylogenetic analyses of the present study provide support for the placement of additional fossil arctoid taxa at the base of this group. These “proto” pinnipeds come from disparate locations, deposits, and ages, and display a wide range of aquatic adaptations, ranging from the large-bodied molluscivore Kolponomos (Stirton, 1960), to the freshwater otter-like forms Puijila and Potamotherium, to the aquatically-specialized Semantor. More detailed study of additional fossil arctoid taxa (including Amphicticeps), particularly with regards to their internal cranial architecture, may further explain the complexities associated with the transition of pinnipeds from a terrestrial to an aquatic environment.
At present, evidence overwhelmingly favors a monophyletic origin of pinnipeds. However, otariids, odobenids, and phocids display a startling amount of parallel evolution, as many of their shared features are absent in the early-diverging fossil ancestors of each family. Within a monophyletic framework, we postulate that parallel evolution may be the mechanism explaining their specialization within the aquatic realm, particularly with regards to raptorial feeding and hydrodynamic locomotion. A definitive answer awaits fossil evidence and the advancement of morphological phylogenetic methods. In the mean-time, statistical tests of convergence (Muschick et al., 2012; Ingram and Mahler, 2013; Arbuckle et al., 2014) could be applied to cladistic data sets of arctoids, to further examine the likelihood of parallel evolution within pinnipeds.
Data Availability Statement
The datasets generated for this study can be found in the Appendix 3.
Author Contributions
NR and NK conceived of the study. RP and NK collected data for the phylogenetic analysis. RP and HM ran the analyses. RP wrote the manuscript in consultation with NR, HM, and NK.
Funding
This research was supported by a Burke-VPCSG, a Paleontological Society student research grant, and an NSERC CGS-M to RP, and a NSERC discovery grant to HM JSPS-ESA11740286 to NK.
Conflict of Interest
The authors declare that the research was conducted in the absence of any commercial or financial relationships that could be construed as a potential conflict of interest.
Acknowledgments
We are grateful to the staff of the Canadian Museum of Nature and the Government of Nunavut for allowing us to study NUFV-405. For assistance and access to fossil collections, we thank: W. Simpson (FMNH), J. Galkin (AMNH), C. Sidor, R. Eng, M. Riven, J. Bradley (Burke), F. Mayer (BNHM), D. Bohaska (NMNH), N. Famoso, G Retallack, G. Davis (UOMNH), J. Samuels (JODA), A. Pritchard, J. Gauthier (YPM), K. Seymour, D. C. Evans, B. Lim, J. Miller (ROM), C. de Muizon, G. Billet (MNHN), R. Marchant (MGL), G. Roessner (BSP), and E. Robert (FSL). For useful discussion on phylogenetics and taxonomy, and their encouragement to tackle this research question, we thank Annalisa Berta, Thomas Cullen, Susumu Tomiya, Takahiro Yonezawa, Alberto Valenciano, and John Flynn. For providing morphological information of Amphicticeps and other basal musteloids and amphicynodontids (including useful casts) since the initial stage of this study, we thank Xiaoming Wang (LACM). We would also like to thank Morgan Churchill and Olaf Bininda-Emonds for comments that greatly improved the focus and scope of the manuscript.
Supplementary Material
The Supplementary Material for this article can be found online at: https://www.frontiersin.org/articles/10.3389/fevo.2019.00457/full#supplementary-material
References
Ahrens, H. E. (2014). Morphometric study of phylogenetic and ecologic signals in procyonid (Mammalia: Carnivora) endocasts. Anat. Rec. 297, 2318–2330. doi: 10.1002/ar.22996
Amson, E., and de Muizon, C. (2014). A new durophagous phocid (Mammalia: Carnivora) from the late Neogene of Peru and considerations on monachine seals phylogeny. J. Syst. Palaeontol. 12, 523–548. doi: 10.1080/14772019.2013.799610
Arbuckle, K., Bennett, C. M., and Speed, M. P. (2014). A simple measure of the strength of convergent evolution. Methods Ecol. Evol. 5, 685–693. doi: 10.1111/2041-210X.12195
Arnason, U. (1974). Comparative chromosome studies in Pinnipedia. Heredita 76, 179–225. doi: 10.1111/j.1601-5223.1974.tb01340.x
Arnason, Ú., Gullberg, A., Janke, A., Kullberg, M., Lehman, N., Petrov, E. A., et al. (2006). Pinniped phylogeny and a new hypothesis for their origin and dispersal. Mol. Phylogen. Evol. 41, 345–354. doi: 10.1016/j.ympev.2006.05.022
Baskin, J. (1998). “Mustelidae,” in Evolution of Tertiary Mammals of North America, Vol. 1, eds C. M. Janis, K. M. Scott, and L.L. Jacobs (Cambridge: Cambridge University Press, 152–173.
Berta, A. (1991). New Enaliarctos (Pinnipedimorpha) From the Oligocene and Miocene of Oregon and role of “Enaliarctids” in Pinniped phylogeny. Smithsonian Contributions to Paleontology. Washington: Smithsonian Institution Press.
Berta, A. (1994). New specimens of the Pinnipediform Pteronarctos from the Miocene of Oregon. Smithson. Contrib. Paleobiol. 78, 1–30.
Berta, A. (2012). Return to the Sea: The Life and Evolutionary Times of Marine Mammals. Berkeley: Univ of California Press.
Berta, A., and Ray, C. E. (1990). Skeletal morphology and locomotor capabilities of the archaic pinniped Enaliarctos mealsi. J. Vertebr. Paleontol. 10, 141–157. doi: 10.1080/02724634.1990.10011803
Berta, A., Ray, C. E., and Wyss, A. R. (1989). Skeleton of the oldest known pinniped, Enaliarctos mealsi. Science 244, 60–62. doi: 10.1126/science.244.4900.60
Berta, A., and Wyss, A. R. (1994). “Pinniped phylogeny,” Proceedings of the San Diego Society of Natural History, Vol. 29:33–56.
Bininda-Emonds, O. R., and Gittleman, J. L. (2000). Are pinnipeds functionally different from fissiped carnivores? The importance of phylogenetic comparative analyses. Evolution 54, 1011–1023. doi: 10.1111/j.0014-3820.2000.tb00100.x
Bininda-Emonds, O. R. P., Bryant, H. N., and Russell, A. P. (1998). Supraspecific taxa as terminals in cladistic analysis: implicit assumptions of monophyly and a comparison of methods. Biol. J. Linn. Soc. 64, 101–133. doi: 10.1111/j.1095-8312.1998.tb01536.x
Bininda-Emonds, O. R. P., Gittleman, J. L., and Purvis, A. (1999). Building large trees by combining phylogenetic information: a complete phylogeny of the extant Carnivora (Mammalia). Biol. Rev. 74, 143–175. doi: 10.1017/S0006323199005307
Bininda-Emonds, O. R. P., and Russell, A. P. (1996). Morphological Perspective on the Phylogenetic Relationships of the Extand Phocid Seals (Mammalia: Carnivora: Phocidae). Bonn: Zoologisches Forschungsinstitut.
Bloom, J. D. (2017). Identification of positive selection in genes is greatly improved by using experimentally informed site-specific models. Biol. Dir. 12:1. doi: 10.1186/s13062-016-0172-z
Boessenecker, R. W., and Churchill, M. (2013). A reevaluation of the morphology, paleoecology, and phylogenetic relationships of the enigmatic walrus Pelagiarctos. PloS ONE 8:e54311. doi: 10.1371/journal.pone.0054311
Boessenecker, R. W., and Churchill, M. (2015). The oldest known fur seal. Biol. Lett. 11:20140835. doi: 10.1098/rsbl.2014.0835
Boessenecker, R. W., and Churchill, M. (2018). The last of the desmatophocid seals: a new species of Allodesmus from the upper Miocene of Washington, USA, and a revision of the taxonomy of Desmatophocidae. Zool. J. Linn. Soc. 184, 211–235. doi: 10.1093/zoolinnean/zlx098
Bryant, H. N., Russell, A. P., and Fitch, W. D. (1993). Phylogenetic relationships within the extant Mustelidae (Carnivora): appraisal of the cladistic status of the Simpsonian subfamilies. Zool. J. Linn. Soc. 108, 301–334. doi: 10.1111/j.1096-3642.1993.tb00301.x
Chikina, M., Robinson, J. D., and Clark, N. L. (2016). Hundreds of genes experienced convergent shifts in selective pressure in marine mammals. Mol. Biol. Evol. 33, 2182–2192. doi: 10.1093/molbev/msw112
Churchill, M., Boessenecker, R., and Clementz, M. T. (2014). Colonization of the southern hemisphere by fur seals and sea lions (Carnivora: Otariidae) revealed by combined evidence phylogenetic and Bayesian biogeographical analysis. Zool. J. Linn. Soc. 172, 200–225. doi: 10.1111/zoj.12163
Churchill, M., and Clementz, M. T. (2016). The evolution of aquatic feeding in seals: insights from Enaliarctos (Carnivora: Pinnipedimorpha), the oldest known seal. J. Evol. Biol. 29, 319–334. doi: 10.1111/jeb.12783
Creevey, C. J., and McInerney, J. O. (2004). Clann: investigating phylogenetic information through supertree analyses. Bioinformatics 21, 390–392. doi: 10.1093/bioinformatics/bti020
Cullen, T. M., Fraser, D., Rybczynski, N., and Schröder-Adams, C. (2014). Early evolution of sexual dimorphism and polygyny in Pinnipedia. Evolution 68, 1469–1484. doi: 10.1111/evo.12360
Darwin, C. (1859). On the Origin of Species by Means of Natural Selection Or the Preservation of Favoured Races in the Struggle for Life, ed H. Milford. Oxford: Oxford University Press.
Davies, J. L. (1958). The Pinnipedia: an essay in zoogeography. Geograph. Rev. 48, 474–493. doi: 10.2307/211670
de Jong, W. W., and Goodman, M. (1982). Mammalian phylogeny studied by sequence-analysis of the eye lens protein alpha-crystallin. Z Saugetierkd Int. J. Mammal. Biol. 47, 257–276.
de Muizon, C. (1982a). Les relations phylogénétiques des Lutrinae (Mustelidae, Mammalia). Geobios 15, 259–277. doi: 10.1016/S0016-6995(82)80118-6
Deméré, T. A. (1994). “Two new species of fossil walruses (Pinnipedia: Odobenidae) from the upper Pliocene San Diego Formation, California,” in Proceedings of the San Diego Society of Natural History, Vol. 29. 77–98.
Deméré, T. A., and Berta, A. (2001). A reevaluation of Proneotherium repenningi from the Miocene Astoria Formation of Oregon and its position as a basal odobenid. J. Vertebr. Paleontol. 21, 279–310. doi: 10.1671/0272-4634(2001)021[0279:AROPRF]2.0.CO;2
Deméré, T. A., and Berta, A. (2002). “The Miocene pinniped Desmatophoca oregonensis Condon, 1906 (Mammalia: Carnivora) from the Astoria Formation, Oregon. Later Cenozoic Mammals of Land and Sea: tributes to the Career of Clayton E. Ray. Smithson. Contrib. Paleobiol. 93, 113–147.
Deméré, T. A., and Berta, A. (2005). New skeletal material of Thalassoleon (Otariidae: Pinnipedia) from the Late Miocene-Early Pliocene (Hemiphillian) of California. Bull. Florida Mus. Nat. Hist. 45, 379–411.
Deméré, T. A., Berta, A., and Adam, P. J. (2003). Pinnipedimorph evolutionary biogeography. Bull. Am. Mus. Nat. Hist.279, 33–76. doi: 10.1206/0003-0090(2003)279<0032:C>2.0.CO;2
Diedrich, C. (2011). The world's oldest fossil seal record. Nat. Sci. 3, 914–920. doi: 10.4236/ns.2011.311117
Donoghue, P. C., and Purnell, M. A. (2009). Distinguishing heat from light in debate over controversial fossils. BioEssays 31, 178–189. doi: 10.1002/bies.200800128
Doronina, L., Churakov, G., Shi, J., Brosius, J., Baertsch, R., Clawson, H., et al. (2015). Exploring massive incomplete lineage sorting in arctoids (Laurasiatheria, Carnivora). Mol. Biol. Evol. 32, 3194–3204. doi: 10.1093/molbev/msv188
Dragoo, J. W., and Honeycutt, R. L. (1997). Systematics of mustelid-like carnivores. J. Mammal. 78, 426–443. doi: 10.2307/1382896
Drehmer, C. J., Fabián, M. E., and Menegheti, J. O. (2004). Dental anomalies in the Atlantic population of South American sea lion, Otaria byronia (Pinnipedia, Otariidae): evolutionary implications and ecological approach. Latin Am. J. Aquat. Mammals 3, 7–18. doi: 10.5597/lajam00044
Enard, D., Messer, P. W., and Petrov, D. A. (2014). Genome-wide signals of positive selection in human evolution. Genome Res. 24, 885–895. doi: 10.1101/gr.164822.113
English, A. W. (1976). Limb movements and locomotor function in the California sea lion (Zalophus californianus). J. Zool. 178, 341–364. doi: 10.1111/j.1469-7998.1976.tb02274.x
Fan, Y., Wu, R., Chen, M. H., Kuo, L., and Lewis, P. O. (2010). Choosing among partition models in Bayesian phylogenetics. Mol. Biol. Evol. 28, 523–532. doi: 10.1093/molbev/msq224
Fay, F. H., Rausch, V. R., and Felitz, E. T. (1967). Cytogeneic comparison of some pinnipeds (Mammalia: Eutheria). Can. J. Zool. 45, 773–778. doi: 10.1139/z67-088
Finarelli, J. A. (2008). A total evidence phylogeny of the Arctoidea (Carnivora: Mammalia): relationships among basal taxa. J. Mammal. Evol. 15, 231–259. doi: 10.1007/s10914-008-9074-x
Fish, F. E., Innes, S., and Ronald, K. (1988). Kinematics and estimated thrust production of swimming harp and ringed seals. J. Exp. Biol. 137, 157–173.
Flynn, J. J., Finarelli, J. A., Zehr, S., Hsu, J., and Nebdal, M. A. (2005). Molecular phylogeny of the Carnivora (Mammalia): assessing the impact of increased sampling on resolving enigmatic relationships. Syst. Biol. 54, 317–337. doi: 10.1080/10635150590923326
Flynn, J. J., Nebdal, M. A., Dragoo, J. W., and Honeycutt, R. L. (2000). Whence the red panda? Mol. Phylogen. Evol. 17, 190–199. doi: 10.1006/mpev.2000.0819
Flynn, J. J., and Nedbal, M. A. (1998). Phylogeny of the Carnivora (Mammalia): congruence vs incompatibility among multiple data sets. Mol. Phylogen. Evol. 9, 414–426. doi: 10.1006/mpev.1998.0504
Flynn, J. J., Neff, N. A., and Tedford, R. H. (1988). Phylogeny of the Carnivora. Phylogeny Classif. Tetrapods 2, 73–116.
Foote, A. D., Liu, Y., Thomas, G. W., Vina,r, T., Alföldi, J., Deng, J., et al. (2015). Convergent evolution of the genomes of marine mammals. Nat. Genet. 47:272. doi: 10.1038/ng.3198
Fulton, T. L., and Strobeck, C. (2006). Molecular phylogeny of the Arctoidea (Carnivora): effect of missing data on supertree and supermatrix analyses of multiple gene data sets. Mol. Phylogen. Evol. 41, 165–181. doi: 10.1016/j.ympev.2006.05.025
Fulton, T. L., and Strobeck, C. (2007). Novel phylogeny of the raccoon family (Procyonidae: Carnivora) based on nuclear and mitochondrial DNA evidence. Mol. Phylogen. Evol. 43, 1171–1177. doi: 10.1016/j.ympev.2006.10.019
Fulton, T. L., and Strobeck, C. (2010). Multiple fossil calibrations, nuclear loci and mitochondrial genomes provide new insight into biogeography and divergence timing for true seals (Phocidae, Pinnipedia). J. Biogeograp. 37, 814–829. doi: 10.1111/j.1365-2699.2010.02271.x
Furbish, R. (2015). Something Old, Something New, Something Swimming in the Blue: An Analysis of the Pinniped Family Desmatophocidae, its Phylogenetic Position and Swimming Mode. Diss. San Diego, CA: San Diego State University.
Gavryushkina, A., Heath, T. A., Ksepka, D. T., Stadler, T., Welch, D., and Drummond, A. J. (2016). Bayesian total-evidence dating reveals the recent crown radiation of penguins. Syst. Biol. 66, 57–73. doi: 10.1093/sysbio/syw060
Geraads, D., and Spassov, N. (2016). Musteloid carnivores from the upper Miocene of South-Western Bulgaria, and the phylogeny of the Mephitidae. Geodiversitas 38, 543–559. doi: 10.5252/g2016n4a5
Gingerich, P. D., Raza, S. M., Arif, M., Anwar, M., and Zhou, X. (1994). New whale from the Eocene of Pakistan and the origin of cetacean swimming. Nature 368:844. doi: 10.1038/368844a0
Gingerich, P. D., Smith, B. H., and Simons, E. L. (1990). Hind limbs of Eocene Basilosaurus: evidence of feet in whales. Science 249, 154–157. doi: 10.1126/science.249.4965.154
Gittleman, J. L., and Valkenburgh, B. V. (1997). Sexual dimorphism in the canines and skulls of carnivores: effects of size, phylogency, and behavioural ecology. J. Zool. 242, 97–117. doi: 10.1111/j.1469-7998.1997.tb02932.x
Goloboff, P. A., Farris, J. S., and Nixon, K. C. (2008). TNT, a free program for phylogenetic analysis. Cladistics 24, 774–786. doi: 10.1111/j.1096-0031.2008.00217.x
Gordon, K. R. (1981). Locomotor behaviour of the walrus (Odobenus). J. Zool. 195, 349–367. doi: 10.1111/j.1469-7998.1981.tb03470.x
Gregory, W. K. (1910). The Orders of Mammals, Vol. 27. New York, NY: Order of the Trustees [American Museum of Natural History].
Grohé, C., Tseng, Z. J., Lebrun, R., Boistel, R., and Flynn, J. J. (2016). Bony labyrinth shape variation in extant Carnivora: a case study of Musteloidea. J. Anat. 228, 366–383. doi: 10.1111/joa.12421
Hall, B. K. (1999). The Neural Crest in Development and Evolution. New York, NY: Springer Science & Business Media.
Heath, T. A., Huelsenbeck, J. P., and Stadler, T. (2014). The fossilized birth–death process for coherent calibration of divergence-time estimates. Proc. Natl. Acad. Sci. 111, 2957–E966. doi: 10.1073/pnas.1319091111
Higdon, J. W., Bininda-Emonds, O. R., Beck, R. M., and Ferguson, S. H. (2007). Phylogeny and divergence of the pinnipeds (Carnivora, Mammalia) assessed using a multigene dataset. BMC Evol. Biol. 7:216. doi: 10.1186/1471-2148-7-216
Huelsenbeck, J. P., Jain, S., Frost, S. W., and Pond, S. L. K. (2006). A Dirichlet process model for detecting positive selection in protein-coding DNA sequences. Proc. Natl. Acad. Sci. 103, 6263–6268. doi: 10.1073/pnas.0508279103
Huelsenbeck, J. P., and Ronquist, F. (2001). MRBAYES: Bayesian inference of phylogenetic trees. Bioinformatics 17, 754–755. doi: 10.1093/bioinformatics/17.8.754
Hunt, R. M. Jr., and Barnes, L. G. (1994). Basicranial evidence for ursid affinity of the oldest pinnipeds. Proceedings of San Diego Society of Natural History, Vol. 29, 57–67.
Hunt, R. M. Jr., and Skolnick, R. (1996). The giant mustelid Megalictis from the early Miocene carnivore dens at Agate Fossil Beds National Monument, Nebraska; earliest evidence of dimorphism in New World Mustelidae (Carnivora, Mammalia). Rocky Mt. Geol. 31, 35–48.
Illiger, J. K. W. (1811). Prodomus Systematis Mammalium et Avium Additis Terminis Zoographicii Utriusque Classis Eorumque Versione Germanica. Berlin: sumptibus C. Salfeld.
Ingram, T., and Mahler, D. L. (2013). SURFACE: detecting convergent evolution from comparative data by fitting Ornstein-Uhlenbeck models with stepwise Akaike Information Criterion. Methods Ecol. Evol. 4, 416–425. doi: 10.1111/2041-210X.12034
Irwin, D. M., and Árnason, Ú. (1994). Cytochromeb gene of marine mammals: phylogeny and evolution. J. Mammal. Evol. 2, 37–55. doi: 10.1007/BF01464349
Jones, K. E., Smaers, J. B., and Goswami, A. (2015). Impact of the terrestrial-aquatic transition on disparity and rates of evolution in the carnivoran skull. BMC Evol. Biol. 15:1. doi: 10.1186/s12862-015-0285-5
Klopfstein, S., Vilhemlsen, L., and Ronquist, F. (2015). A nonstationary Markov model detects directional evolution in hymenopteran morphology. Syst. Biol. 64, 1089–1103. doi: 10.1093/sysbio/syv052
Kohno, N. (1993). “Have the pinnipeds been derived from the ursids,” in Abstracts of the Annual Meeting of the Palaeontological Society of Japan (Tsukuba). 79.
Kohno, N. (1994). A new Miocene pinniped in the genus Prototaria (Carnivora: Odobenidae) from the Moniwa Formation, Miyagi, Japan. J. Vertebr. Paleontol. 14, 414–426. doi: 10.1080/02724634.1994.10011568
Kohno, N. (1996a). “The Oligo-Miocene aquatic arctoid carnivore Potamotherium, and its bearing on the relationships of pinnipeds,” in Resumes Readaptations Milieu Aquatique, Vol. 1 (Poitiers), 22–23.
Kohno, N. (1996b). Miocene pinniped Allodesmus with special reference to the “Mito seal” from Ibaraki Prefecture, Central Japan. Transact. Proc. Palaeontol. Soc. Jpn. 181, 388–404.
Kohno, N. (2006). A new Miocene odobenid (Mammalia: Carnivora) from Hokkaido, Japan, and its implications for odobenid phylogeny. J. Vertebr. Paleontol. 26, 411–421. doi: 10.1671/0272-4634(2006)26[411:ANMOMC]2.0.CO;2
Kohno, N., Barnes, L. G., and Hirota, K. (1995). Miocene fossil pinnipeds of the genera Prototaria and Neotherium (Carnivora; Otariidae; Imagotariinae) in the north Pacific Ocean: evolution, relationships, and distribution. Island Arc. 3, 285–308. doi: 10.1111/j.1440-1738.1994.tb00117.x
Koretsky, I. A., and Barnes, L. G. (2006). “Pinniped evolutionary history and paleobiogeography,” in Mesozoic and Cenozoic Vertebrates and Paleoenvironments: Tributes to the Career of Professor Dan Grigorescu (Bucharest: Ars Docendi), 143–153.
Koretsky, I. A., Barnes, L. G., and Rahmat, S. J. (2016). Re-Evaluation of Morphological Characters Questions Current Views of Pinniped Origins. Vestnik zoologii 50, 327–354. doi: 10.1515/vzoo-2016-0040
Koretsky, I. A., and Domning, D. P. (2014). One of the oldest seals (Carnivora, Phocidae) from the Old World. J. Vertebr. Paleontol. 34, 224–229. doi: 10.1080/02724634.2013.787428
Koretsky, I. A., and Holec, P. (2002). A primitive seal (Mammalia: Phocidae) from the early middle Miocene of Central Paratethys. Smithson. Contrib. Paleobiol. 93, 163–178.
Koretsky, I. A., and Rahmat, S. J. (2015). A new species of the subfamily Devinophocinae (Carnivora, Phocidae) from the Central Paratethys. Res. Paleontol. Stratigrap. 121, 31–47. doi: 10.13130/2039-4942/6399
Kosiol, C., Vinar, T., da Fonseca, R. R., Hubisz, M. J., Bustamante, C. D., Nielsen, R., et al. (2008). Patterns of positive selection in six mammalian genomes. PloS Genet. 4:e1000144. doi: 10.1371/journal.pgen.1000144
Kuhn, C., and Frey, E. (2012). Walking like caterpillars, flying like bats—pinniped locomotion. Palaeobiodivers. Palaeoenviron. 92, 197–210. doi: 10.1007/s12549-012-0077-5
Lee, M. S. (2016). Multiple morphological clocks and total evidence tip-dating in mammals. Biol. Lett. 12:20160033. doi: 10.1098/rsbl.2016.0033
Maddison, W. P., and Maddison, D. R. (2015). Mesquite: A Modular System for Evolutionary Analysis. Version 2.75. 2011.
Matzke, N. J., and Wright, A. (2016). Inferring node dates from tip dates in fossil canidae: the importance of tree priors. Bio. Lett. 12:20160328. doi: 10.1098/rsbl.2016.0328
McClellan, D. A. (2013). Directional darwinian selection in proteins. BMC Bioinform. 14 (Suppl. 13):S6. doi: 10.1186/1471-2105-14-S13-S6
McLaren, I. A. (1960). Are the pinnipedia biphyletic? System. Zoolog. 9, 18–28. doi: 10.2307/2411537
Merritt, T. J. S., and Quattro, J. M. (2001). Evidence for a period of directional selection following gene duplication in a neurally expressed locus of triosephosphate isomerase. Genetics 159:689697.
Mitchell, E., and Tedford, R. H. (1973). The enaliarctinae: a new group of extinct aquatic carnivora and a consideration of the origin of the otariidae. Bull. AMNH 151, 201–284.
Mivart, S. G. (1885). On the anatomy, classification, and distribution of the arctoidea. Proc. Zoolog. Soc. Lond. 53, 340–404. doi: 10.1111/j.1096-3642.1885.tb02915.x
Miyamoto, M. M., and Goodman, M. (1986). Biomolecular systematics of eutherian mammals: phylogenetic patterns and classification. Syste.Bio. 35, 230–240. doi: 10.1093/sysbio/35.2.230
Muschick, M., Indermaur, A., and Salzburger, W. (2012). Convergent evolution within an adaptive radiation of cichlid fishes. Curr.Bio. 22, 2362–2368. doi: 10.1016/j.cub.2012.10.048
Nielsen, R. (2005). Molecular signatures of natural selection. Annu. Rev. Genet. 39, 197–218. doi: 10.1146/annurev.genet.39.073003.112420
Nojima, T. (1990). A morphological consideration of the relationships of pinnipeds to other carnivorans based on the bony tentorium and bony falx. Mar. Mammal Sci. 6, 54–74. doi: 10.1111/j.1748-7692.1990.tb00226.x
Nyakatura, K., and Bininda-Emonds, O. R. (2012). Updating the evolutionary history of Carnivora (Mammalia): a new species-level supertree complete with divergence time estimates. BMC biol. 10:12. doi: 10.1186/PREACCEPT-5398900576110216
Orlov, J. A. (1933). Semantor macrurus (ordo Pinnipedia, fam. semantoridae fam. nova) aus den neogen-ablagerungen westsibiriens. Trav.l'Instit. Paléozool. Acad. Sci. URSS 2, 165–268.
Pattinson, D. J., Thompson, R. S., Piotrowski, A. K., and Asher, R. J. (2014). Phylogeny, paleontology, and primates: do incomplete fossils bias the tree of life? Syst.Biol. 64, 169–186. doi: 10.1093/sysbio/syu077
Pierce, S. E., Clack, J. A., and Hutchinson, J. R. (2011). Comparative axial morphology in pinnipeds and its correlation with aquatic locomotory behaviour. J. Anato. 219, 502–514. doi: 10.1111/j.1469-7580.2011.01406.x
Prieto-Marquez, A. (2010). Global phylogeny of hadrosauridae (Dinosauria: Ornithopoda) using parsimony and bayesian methods. Zool. J. Linn. Soc. 159, 435–502. doi: 10.1111/j.1096-3642.2009.00617.x
Prothero, D. R., Bitboul, C. Z., Moore, G. W., and Niem, A. R. (2001). Magnetic Stratigraphy and Tectonic Rotation of the Oligocene Alsea, Yaquina, and Nye Formations. Lincoln County.
Pyron, R. A. (2011). Divergence time estimation using fossils as terminal taxa and the origins of lissamphibia. Syst. Biol. 60, 466–481. doi: 10.1093/sysbio/syr047
Repenning, C. A., and Tedford, R. H. (1977). Otarioid Seals of the Neogene. No. 992. Washington: US Govt. Print. Off.
Romero-Herrera, A. E., Lehmann, H., Joyseyet, K. A., and Friday, A. E. (1978). On the evolution of myoglobin. Philos. Trans. Royal Soc. Lond. B 283, 61–163. doi: 10.1098/rstb.1978.0018
Ronquist, F., and Huelsenbeck, J. P. (2003). MrBayes 3: bayesian phylogenetic inference under mixed models. Bioinformatics 19, 1572–1574. doi: 10.1093/bioinformatics/btg180
Ronquist, F., Klopfstein, S., Vilhelmsen, L., Schulmeister, S., Murray, D. L., and Rasnitsyn, A. P. (2012a). A total-evidence approach to dating with fossils, applied to the early radiation of the hymenoptera. Syst. Biol. 61, 973–999. doi: 10.1093/sysbio/sys058
Ronquist, F., Lartillot, N., and Phillips, M. J. (2016). Closing the gap between rocks and clocks using total evidence dating. Philos. Trans. Royal Soc. B 371:20150136. doi: 10.1098/rstb.2015.0136
Ronquist, F., Teslenko, M., van der Mark, P., Ayres, D. L., Darling, A., Höhna, S., et al. (2012b). MrBayes 3.2: efficient bayesian phylogenetic inference and model choice across a large model space. Syst. Biol. 61, 539–542. doi: 10.1093/sysbio/sys029
Rybczynski, N., Dawson, M. R., and Tedford, R. H. (2009). A semi-aquatic arctic mammalian carnivore from the miocene epoch and origin of pinnipedia. Nature 458, 1021–1024. doi: 10.1038/nature07985
Sansom, R. S. (2014). Bias and sensitivity in the placement of fossil taxa resulting from interpretations of missing data. Syst. Biol. 64, 256–266. doi: 10.1093/sysbio/syu093
Sansom, R. S., and Wills, M. A. (2013). Fossilization causes organisms to appear erroneously primitive by distorting evolutionary trees. Sci. Rep. 3:2545. doi: 10.1038/srep02545
Sarich, V. M. (1969). Pinniped evolution and the rate of evolution of carnivore albumins. U. S. Geol. Surv. Profes. Pap. 992, 1–93.
Sato, J. J., Wolsan, M., Minami, S., Hosoda, T., Sinaga, M. H., Hiyama, K., et al. (2009). Deciphering and dating the red panda's ancestry and early adaptive radiation of musteloidea. Mol.Phylogen. Evolu. 53, 907–922. doi: 10.1016/j.ympev.2009.08.019
Sato, J. J., Wolsan, M., Prevosti, F. J., D'Elía, G., Begg, C., Begg, K., et al. (2012). Evolutionary and biogeographic history of weasel-like carnivorans (Musteloidea). Mol. Phylogen. Evol. 63, 745–757. doi: 10.1016/j.ympev.2012.02.025
Sato, J. J., Wolsan, M., Suzuki, H., Hosoda, T., Yamaguchi, Y., Hiyama, K., et al. (2006). Evidence from nuclear DNA sequences sheds light on the phylogenetic relationships of pinnipedia: single origin with affinity to musteloidea. Zool. Sci. 23, 125–146. doi: 10.2108/zsj.23.125
Savage, R. J. G. (1957). “October. The anatomy of Potamotherium an Oligocene lutrine,” in Proceedings of the Zoological Society of London, Vol. 129 (Oxford: Blackwell Publishing Ltd, 151–244.
Spaulding, M., and Flynn, J. J. (2012). Phylogeny of the carnivoramorpha: the impact of postcranial characters. J. Syst. Palaeontol. 10, 653–677. doi: 10.1080/14772019.2011.630681
Stirton, R. A. (1960). A Marine Carnivore From the Clallam Miocene Formation, Washington. A Review of the Sirenia and Desmostylia. 345.
Tedford, R. H. (1976). Relationship of pinnipeds to other carnivores (Mammalia). Syst. Biol. 25, 363–374. doi: 10.2307/2412511
Tedford, R. H., Barnes, L. G., and Ray, C. E. (1994). “The early Miocene littoral ursoid carnivoran Kolponomos: systematics and mode of life,” in Proceedings of the San Diego Society of Natural History, Vol. 29, 11–32.
Tomiya, S., and Tseng, Z. J. (2016). Whence the beardogs? Reappraisal of the Middle to Late Eocene ‘Miacis' from Texas, USA, and the origin of Amphicyonidae (Mammalia, Carnivora). Royal Soc. open sci. 3:160518. doi: 10.1098/rsos.160518
Tseng, Z. J., Wang, X., and Stewart, J. D. (2009). A new immigrant mustelid (Carnivora, Mammalia) from the middle Miocene Temblor Formation of central California. PaleoBios. 29, 13–23.
Valenciano, A., Baskin, J. A., Abella, J., Pérez-Ramos, A., Álvarez-Sierra, M. Á., Morales, J., et al. (2016). Megalictis, the Bone-Crushing Giant Mustelid (Carnivora, Mustelidae, Oligobuninae) from the Early Miocene of North America. PLoS ONE 11:e0152430. doi: 10.1371/journal.pone.0152430
Velez-Juarbe, J. (2017). Eotaria citrica, sp. nov., a new stem otariid from the “Topanga” formation of Southern California. PeerJ 5:e3022. doi: 10.7717/peerj.3022
Wang, X., Grohé, C., Su, D. F., White, S. C., Ji, X., Kelley, J., et al. (2017). A new otter of giant size, Siamogale melilutra sp. nov. (Lutrinae: Mustelidae: Carnivora) from the latest miocene shuitangba site in north-eastern Yunnan, south-western China, and a total evidence analysis of lutrines. J. Syst. Palaeontol. 16, 39–65. doi: 10.1080/14772019.2016.1267666
Wang, X., McKenna, M. C., and Dashzeveg, D. (2005). Amphicticeps and Amphicynodon (Arctoidea, Carnivora) from Hsanda Gol Formation, central Mongolia and phylogeny of basal arctoids with comments on zoogeography. Am. Mus. Novitates 3483, 1−60. doi: 10.1206/0003-0082(2005)483[0001:AAAACF]2.0.CO;2
Wang, Z., Yuan, L., Rossiter, S. J., Zuo, X., Ru, B., Zhong, H., et al. (2008). Adaptive evolution of 5′ HoxD genes in the origin and diversification of the cetacean flipper. Mol. Biol. Evol. 26, 613–622. doi: 10.1093/molbev/msn282
Weber, M. W. C. (1904). Die Säugetiere: Einführung in die Anatomie und Systematik der recenten und fossilen Mammalia, ed J. G. Fischer.
Wesley-Hunt, G. D., and Flynn, J. J. (2005). Phylogeny of the Carnivora: basal relationships among the carnivoramorphans, and assessment of the position of ‘Miacoidea'relative to Carnivora. J. Syst. Palaeontol. 3, 1–28. doi: 10.1017/S1477201904001518
Wilgenbusch, J. C. (2004). AWTY: A System for Graphical Exploration of MCMC Convergence in Bayesian Phylogenetic Inference. Available online at: http://ceb.csit.fsu.edu/awty
Wolsan, M. (1993). Phylogeny and classification of early European Mustelida (Mammalia: Carnivora). Acta Theriol. 38, 345–384. doi: 10.4098/AT.arch.93-29
Wozencraft, W. C. (1989). “The phylogeny of the recent Carnivora,” in Carnivore Behavior, Ecology, and Evolution (New York City, NY: Springer US), 495–535.
Wyss, A. R. (1987). The walrus auditory region and the monophyly of pinnipeds. Amer. Mus. Novitates 2871, 1–31.
Wyss, A. R. (1988). On “retrogression” in the evolution of the Phocinae and phylogenetic affinities of the monk seals. Am. Museum Nat. Hist. 2924, 1–38.
Wyss, A. R., and Flynn, J. J. (1993). “A phylogenetic analysis and definition of the Carnivora,” in Mammal Phylogeny: Placentals, eds F. S. Szalay, M. J. Novacek, and M. C. McKenna. New York, NY: Springer, 32–52.
Xie, W., Lewis, P. O., Fan, Y., Kuo, L., and Chen, M. H. (2010). Improving marginal likelihood estimation for Bayesian phylogenetic model selection. Syst. Biol. 60, 150–160. doi: 10.1093/sysbio/syq085
Yonezawa, T., Kohno, N., and Hasegawa, M. (2009). The monophyletic origin of sea lions and fur seals (Carnivora; Otariidae) in the Southern Hemisphere. Gene 441, 89–99. doi: 10.1016/j.gene.2009.01.022
Zhang, C., Stadler, T., Klopfstein, S., Heath, T. A., and Ronquist, F. (2016). Total-evidence dating under the fossilized birth-death process. Syst. Biol. 65, 228–249. doi: 10.1093/sysbio/syv080
Zhou, X., Seim, I., and Gladyshev, V. N. (2015). Convergent evolution of marine mammals is associated with distinct substitutions in common genes. Sci. Rep. 5:16550. doi: 10.1038/srep16550
Keywords: total evidence dating, pinniped, phylogenetic analysis, aquatic adaptation, parallel evolution
Citation: Paterson RS, Rybczynski N, Kohno N and Maddin HC (2020) A Total Evidence Phylogenetic Analysis of Pinniped Phylogeny and the Possibility of Parallel Evolution Within a Monophyletic Framework. Front. Ecol. Evol. 7:457. doi: 10.3389/fevo.2019.00457
Received: 13 May 2019; Accepted: 13 November 2019;
Published: 17 January 2020.
Edited by:
Michel Laurin, UMR7207 Centre de Recherche sur la Paléobiodiversité et les Paléoenvironnements (CR2P), FranceReviewed by:
Olaf R. P. Bininda-Emonds, University of Oldenburg, GermanyMorgan Churchill, University of Wisconsin–Oshkosh, United States
Copyright © 2020 Paterson, Rybczynski, Kohno and Maddin. This is an open-access article distributed under the terms of the Creative Commons Attribution License (CC BY). The use, distribution or reproduction in other forums is permitted, provided the original author(s) and the copyright owner(s) are credited and that the original publication in this journal is cited, in accordance with accepted academic practice. No use, distribution or reproduction is permitted which does not comply with these terms.
*Correspondence: Ryan S. Paterson, cnlhbnBhdGVyc29uJiN4MDAwNDA7Y21haWwuY2FybGV0b24uY2E=