- 1Kalcounis-Rueppell Lab, The University of North Carolina at Greensboro, Biology Department, Greensboro, NC, United States
- 2Gillam Lab, North Dakota State University, Biological Sciences Department, Fargo, ND, United States
- 3Kalcounis-Rueppell Lab, The University of Alberta, Biological Sciences Department, Edmonton, AB, Canada
Bats could be a useful study system for studying the evolution of social communication, as they exhibit a high diversity of social group size and complexity. However, the study of bat social calls has been limited, as they are nocturnal, volant animals that produce predominately ultrasonic vocalizations. Passive acoustic monitoring studies occasionally capture bat in-flight social calls. The information from surrounding echolocation calls can provide information on species identity, activity level, and foraging behavior. We used passive acoustic monitoring in Greensboro, North Carolina, to identify seven types of in-flight social calls from Eptesicus fuscus, Lasiurus borealis, Lasiurus cinereus, Nycticeius humeralis, Perimyotis subflavus, and Tadarida brasiliensis. Eptesicus fuscus, N. humeralis, and T. brasiliensis differed in total social call production, and the proportional use of call types. Shared called types exhibited species-specific signatures, indicating the potential for bats to discern signaler identity. Social call production was positively correlated with bat activity. Social calls were often temporally clustered into independent social calling bouts. The complex and upsweep bouts of E. fuscus were associated with foraging, and the likelihood of complex bouts was negatively correlated with heterospecific activity. The production of N. humeralis downsweep, downsweep-upsweep, and upsweep bouts varied by season and site, but not according to bat activity, foraging, or time of night. Species differed in which call types were most commonly emitted, and these calls are associated with different contexts, suggesting that bats exhibit species-specific differences in in-flight social behavior.
Introduction
Species can differ in social communication behavior due to different call types used (Blumstein and Armitage, 1997; McComb and Semple, 2005), different rates of call production (Kalcounis-Rueppell et al., 2018), species specific differences in call characteristics (Insley, 1992; Rendell et al., 1999; Musolf et al., 2015), and differences in information encoded (Medvin et al., 1993; Pollard, 2011). The social complexity hypothesis posits that species-specific differences in communication systems can arise due to differences in social systems (Freeberg et al., 2012). Across species, repertoire size has been positively correlated with social group complexity (Blumstein and Armitage, 1997; McComb and Semple, 2005). Group size is often correlated with call complexity, as more complex calls can encode more information about individual identity (Medvin et al., 1993; Wilkinson, 2003; Freeberg, 2006; Pollard, 2011).
Bats exhibit an extensive range in social group size (Davis et al., 1962; Constantine, 1966), social group organization (Wilkinson, 1984; Barclay et al., 1988; Willis and Brigham, 2004; Garroway and Broders, 2007; Patriquin et al., 2010), and mating systems (Bradbury, 1977; Barclay et al., 1979; Thomas et al., 1979; Vaughan and Vaughan, 1986; Heckel and von Helversen, 2002; Keeley and Keeley, 2004). Vocal learning has been observed in some species of bats (Knörnschild et al., 2012; Vernes and Wilkinson, in press). However, knowledge of bat social calls has been limited, as bats are nocturnal, volant, and produce predominantly ultrasonic vocalizations. Most of what is known about bat social calls pertains to those emitted in the roost. Social calls in the roost have been observed to mediate short-term agonistic encounters (Barclay et al., 1979), long-term territoriality (Bradbury, 1977; Behr and von Helversen, 2004; Davidson and Wilkinson, 2004; Bohn et al., 2008), mate attraction (Bradbury, 1977; Voigt and von Helversen, 1999; Davidson and Wilkinson, 2002, 2004; Behr and von Helversen, 2004; Bohn et al., 2008; Knörnschild and Tschapka, 2012), and parental care (Barclay et al., 1979; Matsumura, 1979; Scherrer and Wilkinson, 1993; Pfalzer and Kusch, 2003; Wilkinson, 2003; Bohn et al., 2008; Knörnschild and Von Helversen, 2008; Knörnschild et al., 2012).
While the roost is likely where the majority of a bat's social interactions occur, on account of higher densities, there are behavioral contexts that occur predominantly, if not solely in flight, that may be associated with social calls. Social calls associated with foraging competition have been observed in free living T. brasiliensis and Pipistrellus spp. as well as Eptesicus fuscus in the laboratory (Barlow and Jones, 1997; Corcoran and Conner, 2014; Wright et al., 2014). Cooperative foraging has been observed in Noctilio albiventris, Phyllostomus hastatus, and Nycticeius humeralis (Wilkinson, 1992; Wilkinson and Boughman, 1998; Dechmann et al., 2009). While foraging, Noctilio leporinus produce social calls to avoid collision (Suthers, 1965). In some species, when young are learning to fly, mother-pup pairs fly together and produce social calls to maintain cohesion (Pfalzer and Kusch, 2003). Thyroptera tricolor in flight emit inquiry calls to locate roosting group mates (Gillam and Chaverri, 2012). Bats in flight are exposed to predation risk, and their distress calls induce predator mobbing behaviors in conspecifics and heterospecifics (Russ et al., 2004; Knörnschild and Tschapka, 2012). While socially hibernating species may mate in the hibernaculum (Barclay et al., 1979; Thomas et al., 1979), the use of in-flight social calls to attract mates has been observed in Pipistrellus pipistrellus (Lundberg and Gerell, 1986).
Challenges in comparing the communication behaviors of different bat species arise from there having been few studies to develop a system for classifying bat social calls, and disagreement between the existing classification schemes. The classification scheme of Pfalzer and Kusch (2003) used observed contexts to classify calls, and they found correlation between context and spectrogram shape. Type A calls were noisy and used in agonistic contexts, type B were repeating trills used in distress, type C were single pulses used for group cohesion, and type D were composed of different pulse types used for mate attraction and territoriality (Pfalzer and Kusch, 2003). Studies classifying social calls by spectrogram shape have often further broken single pulse calls into different groups, though have still disagreed on the number of call types (Melendez et al., 2006; Wright et al., 2013; Middleton et al., 2014). Lack of correspondence between different classification systems highlight the diversity of bat repertoires as well as the utility of developing more standardized cross-species classification systems for researchers to better study and communicate about the social calls of different species.
The use of passive acoustic monitoring to study the ecology and conservation of bats has increased in recent years due to threats, such as white nose syndrome and wind turbines, that require long term monitoring (Ford et al., 2011; Loeb et al., 2015). Based on species specific differences in echolocation call characteristics, researchers can use recordings of echolocation calls to measure bat species presence and abundance (Britzke et al., 2013; Li and Kalcounis-Rueppell, 2018; Schimpp et al., 2018). The recordings are associated with time of night, time of year, and location, allowing for the analyses of spatial and temporal patterns (Li and Kalcounis-Rueppell, 2018; Schimpp et al., 2018). As bats produce foraging buzzes with distinct spectral and temporal characteristics, passive acoustic monitoring also provides a measure of foraging behavior (Kalcounis-Rueppell et al., 2013; Grider et al., 2016). Bat social calls are sometimes present in the recordings generated during passive acoustic monitoring (Bohn and Gillam, 2018). It may be possible to use information from the surrounding echolocation pulses and the spatial and temporal data about the recording to study bat in-flight social calls (Bohn and Gillam, 2018).
Most information on species-specific calling behavior in bats pertains to bat echolocation calls (reviewed by Jones and Siemers, 2011). By using the species-specific characteristics of echolocation pulses surrounding social calls, it is possible to assign social calls to species. Number of social calls per species can be related to bat activity to test if species differ in how often they produce social calls. After classifying calls to type, species repertoires can be compared. Social call spectral and temporal characteristics can be measured to test for species-specific signatures. Species-specific differences in the spectral and temporal characteristics of social calls have been observed (Pfalzer and Kusch, 2003; Russ et al., 2004; Luo et al., 2017). Analysis of the screech calls from 31 species from Emballonuridae, Rhinolophidae, Hipposideridae, Miniopteridae, and Vespertilionidae showed that call characteristics varied according to phylogeny, morphology, and social group size (Luo et al., 2017).
Several variables that can be measured using passive acoustic monitoring could be relevant to the production of in-flight social calls. The production of agonistic social calls by P. pipistrellus and cohesive calls by P. hastatus are correlated with increased bat abundance (Wilkinson and Boughman, 1998; Bartonička et al., 2007; Budenz et al., 2009). The number of species present may affect which types of social calls are produced. Some social calls, such as those used by Pipistrellus spp. for foraging competition appear to be only used for conspecific communication (Barlow and Jones, 1997; Barratt et al., 1997). Other call types, such as the distress calls of Pipistrellus spp. are used to communicate with conspecifics and heterospecifics (Russ et al., 2004). Laboratory studies of E. fuscus show that calls used for competing over prey items are frequently followed by a terminal buzz by the emitter, while other call types are not (Wright et al., 2013, 2014). The sonar jamming calls used by T. brasiliensis occur simultaneously to the foraging buzz of the intended receiver (Corcoran and Conner, 2014). Therefore, it would be expected that social calls used for interactions while foraging would be more associated with foraging buzzes than call types with other functions. Social calls associated with maintaining group cohesion when exiting the roost would be expected to occur mostly at the start of the night. Social calls associated with locating the roost would be expected to occur mostly at the end of the night.
Social call production may vary throughout the year, as the reproduction and social organization of bats, particularly in temperate climates, exhibit seasonality. The social organization of spring and summer maternity and bachelor colonies differs from that of winter hibernacula (Bradshaw, 1962; Senior et al., 2005; Perry and Thill, 2008; Hein et al., 2009). Tandem flights of mothers and young of the year occur in late summer (Pfalzer and Kusch, 2003). Bats mate predominately in the fall, and in many species, this is associated with swarming to hibernacula (Bradshaw, 1962; Lundberg and Gerell, 1986; Senior et al., 2005; Burns and Broders, 2015). Middleton (2006) observed seasonality in the complex social calls of common pipistrelles, used for territoriality and mate attraction, finding that call production peaked in April-May and again in September.
The objective of this study was to use passive acoustic monitoring to test the hypothesis that bats use dedicated social calls to mediate different types of social interactions while flying. We predicted that if bats use social calls for multiple functions in-flight, bats would produce different types of social calls with distinct spectral and temporal parameters, consistent with types produced by bats in other regions. As bat species differ in social group organization, we predicted species will differ in how often they produce social calls, and proportional call type usage. We predicted that shared called types exhibit greater between species variation than within species variation in spectral and temporal characteristics, which could allow for species recognition. We predicted that the production of social calls is related to behaviorally relevant factors such as bat activity, whether multiple species are present, foraging activity, time of night, and time of year.
Materials and Methods
Recording
The area in which we sampled included Peabody Park and recreation areas that are part of the University of North Carolina at Greensboro (Parker et al., 2019). Two sites were in a mowed, recreational field area and the other two were in a forested area. One site in each area contained a constructed wetland (described full in Parker et al., 2019). Calls were recorded using Song Meter SMBAT4 FS detectors (Wildlife Acoustics Inc. Massachusetts, United States). The detector had a sampling rate of 256 kHz. Triggers were signals with a minimum frequency of 16 kHz, minimum amplitude of 12 dB, and a minimum duration of 1.5 ms. Detectors were able to record continuously, avoiding a loss of data due to lag during periods of high bat activity. We sampled from March 15th, 2017 to June 30th, 2018. From each site we used 6 randomly selected nights for March of 2017, and 11 randomly selected nights for each of the following months. Due to detector failures, not all of the 684 possible detector nights were sampled. We used a final sample size of 679 detector nights for our analyses. To avoid having replication for some seasons but not others, for analyses of the context of call production, we only used detector nights from April 1, 2017 to March 31st, 2018.
Social Call Identification
From the 679 detector nights of recordings, we examined all recorded calls, and we identified social calls, by viewing the spectrogram for each recorded file in Kaleidoscope 4.3 (Wildlife Acoustics Inc. Massachusetts, United States) in Bat Analysis Mode. Signal of interest parameters were set between 8 and 120 kHz, 2 and 500 ms, with a maximum intersyllable gap of 500 ms, and a minimum of 2 pulses. For viewing, the fast Fourier transformation window (FFT) was set to 256, with a window size of 128, and a maximum cache size of 256 MB. We considered social calls as non-echolocation tonal sounds produced during bat passes that did not have another known source. We considered a social call to be a complex social call rather than multiple social calls if the calls were separated by silence without echolocation calls between the pulses, as is consistent with previous studies (Pfalzer and Kusch, 2003; Wright et al., 2013).
While noisy bat social calls have been reported (Barclay et al., 1979; Pfalzer and Kusch, 2003), these were in instances where researchers were able to visually confirm the vocalization as coming from a bat. Given how little is known about the social calls of bats, particularly North American species in flight, without visual confirmation there is not sufficient evidence to conclude that a noise pulse surrounded by echolocation calls was a bat vocalization.
Bat Species Identification
Social calls were assigned to bat species based on manually comparing the spectral and temporal characteristics of the surrounding echolocation pulses to those reported in the Sonobat reference library (Sonobat, DND Designs, Arcata, California) and to a library of known species recordings generated from multiple bioacoustics studies (Buchler, 1980; O'Farrell and Gannon, 1999; O'Farrell et al., 1999; Kurta et al., 2007; Kunz and Parsons, 2009; Li and Wilkins, 2014). We used manual identification to species for bat passes with social calls because the presence of non-echolocation calls, such as social calls, in a recording, can reduce the accuracy of automatic identification software. Social calls were assigned to a species only when there was a single species present in the recording based on at least three clear and complete echolocation calls with call characteristics typical for that species and none with the typical call characteristics of another species. Social calls in files where characteristic echolocation calls for multiple species were present are reported herein as “Multiple Species” as it was not possible to determine which of the species present produced the social call. Social calls in files with consistent echolocation call characteristics across all calls, which suggested presence of a single species, yet insufficient recording quality to allow for species identification were labeled herein as “No ID.”
We used the automatic identification feature in Kaleidoscope 4.3 to identify bat passes that did not contain social calls. We used the Bats of North America 4.3 library with the possible species set as Eptesicus fuscus, Lasiurus borealis, L. cinereus, Lasionycteris noctivagans, Myotis lucifugus, M. septentrionalis, Nycticieus humeralis, Perimyotis subflavus, and Tadarida brasiliensis (as in Kalcounis-Rueppell et al., 2007; Grider et al., 2016). Recordings needed at least 3 complete echolocation calls for identification. Identification accuracy was set as neutral. We used a conservative approach to species specific identification and only used the automatic identification for bat passes with a match ratio of at least 0.6 (60% of the calls in the recording were identified as belonging to that species). Recordings with a match ratio lower than 0.6 were considered as No ID. A 0.6 match ratio is an appropriate threshold for the species of this region, as previous studies have found that manual and automatic identification generally agree for bat passes at and above this threshold (Schimpp et al., 2018; Parker et al., 2019).
Social Call Classification
We manually classified social calls to type based on the shape of the spectrogram. There is no single agreed upon system with which to classify bat social call types. The classification systems proposed by Melendez et al. (2006), Middleton et al. (2014), and Wright et al. (2013) each classify social calls based on how frequency changes over time. The classification system proposed by Pfalzer and Kusch (2003) groups calls by behavioral context, and across species, similar spectrogram shapes served similar functions. The systems disagree on how to lump or split certain call shapes. Despite the differences in how different spectrogram shapes are grouped together or separately, there are common patterns of frequency change over time seen in the different classification systems. Therefore, we used a hybrid of the systems proposed by Melendez et al. (2006), Middleton et al. (2014), Pfalzer and Kusch (2003), and Wright et al. (2013) so that different patterns of frequency change were classified as different call types, while calls with the same pattern of frequency change would be the same call type. We categorized calls to one of seven types depending on the direction(s) of frequency change over time as follows.
Downsweeps are single pulse calls with a bandwidth of at least 5 kHz where the only frequency changes are decreases (Pfalzer and Kusch, 2003; Melendez et al., 2006; Wright et al., 2013; Middleton et al., 2014). Downsweep social calls can be distinguished from echolocation calls based on differences in concavity, frequency range, and duration. Downsweeps needed to have a duration of at least 14 ms to be considered social calls, as this exceeds the typical duration of search phase echolocation calls for most species in our region. Upsweeps are single pulse calls with a bandwidth of at least 5 kHz where the only frequency changes are increases (Pfalzer and Kusch, 2003; Wright et al., 2013; Middleton et al., 2014). Quasi-Constant Frequency (QCF) single pulse calls have a bandwidth of <5 kHz (Pfalzer and Kusch, 2003; Melendez et al., 2006; Wright et al., 2013; Middleton et al., 2014). U-Shaped single pulse calls have a bandwidth of at least 5 kHz with a single frequency decrease followed by a single increase (Pfalzer and Kusch, 2003; Wright et al., 2013; Middleton et al., 2014). Inverted-U-Shaped single pulse calls have a bandwidth of at least 5 kHz with a single frequency increase followed by a single decrease (Pfalzer and Kusch, 2003; Melendez et al., 2006; Wright et al., 2013; Middleton et al., 2014). Oscillating single pulse calls have a bandwidth of at least 5 kHz and multiple changes in frequency direction (Pfalzer and Kusch, 2003; Melendez et al., 2006; Wright et al., 2013; Middleton et al., 2014). Complex calls are those with multiple pulses within 50 ms of one another, without separation by echolocation pulses (Pfalzer and Kusch, 2003; Melendez et al., 2006; Wright et al., 2013; Middleton et al., 2014).
Call Measurements
We considered a call to be suitable for measurement if it had a high signal to noise ratio, did not appear to be incomplete due to attenuation of part of the call, and was not interrupted by other sounds. We used SASLab Pro (Avisoft Bioacoustics, Berlin, Germany) to isolate social calls and measure call parameters for all single species social calls of suitable quality from the three species which produced at least 100 measurable social calls. We used an FFT of 512, with a bandwidth of 1,880 Hz, a resolution of 500 Hz, a temporal resolution overlap of 87.5, a frame size of 100%, and a flat top window. We measured call parameters using the automatic measuring tool with a single threshold and adjusted the threshold for each call to the highest threshold that measures the entire duration of the signal. We removed any background noise that may have interfered with the software's ability to measure the signal of interest. We manually validated the values the automatic measuring tool produced. From each social call we measured duration, number of pulses, start frequency (fstart), center frequency (fcenter) end frequency (fend), peak frequency (fpeak), minimum frequency (fmin), and maximum frequency (fmax). Using the measured call characteristics, we calculated the total bandwidth (bandwidth; fmax-fmin), average slope of the first half (s1; fcenter-fstart / 0.5 * duration), and average slope of the second half (s2; fend-fcenter/0.5 * duration) for each call. Additionally, we isolated 40 echolocation calls from randomly selected recordings with social calls from each species, and measured them in the same manner, to test for differences in the call characteristics of echolocation and social calls. For all calls measured, measurements were solely taken from the fundamental frequency. Data from harmonics were not collected, as harmonics attenuate more rapidly, and are less likely to be detected in field recordings.
Frequency was measured in kHz, and temporal characteristics were measured in milliseconds (ms). Slopes were calculated and reported as kHz/ms, however for statistical analyses slopes were transformed by multiplying the slope by 10, to ensure that the slope characteristics are of the same order of magnitude as the other characteristics. Doing so did not change the predictive capabilities of the discriminant function analysis (DFA) or the results of the MANOVA.
Statistical Analysis
Species-Specific Differences in Social Call Production and Characteristics
Species-specific differences in social call production
To test for species-specific differences in call production between E. fuscus, N humeralis, and T. brasiliensis, we performed a Chi-Squared test to test the null hypothesis that whether a bat pass contained at least one social call was independent of species. To test for species-specific differences in call type usage between E. fuscus, N humeralis, and T. brasiliensis, we used a Chi-squared test to test the null hypothesis that whether a social call was a complex call was independent of species.
Analysis of spectral and temporal characteristics
We performed discriminant function analysis (DFA) within species across call types to determine whether the spectral and temporal characteristics of social calls differed from echolocation calls and among different social call types. DFA procedures have a high risk of Type I errors if the dataset has psuedoreplicates (Mundry and Sommer, 2007). Social calls in the same bat pass are likely to be from the same individual. For bat passes where there were multiple measured calls of the same type, we calculated the average parameter values for all calls of that type in that recording and treated the average values as a single call. We only conducted statistical analyses on call types where there were more than 15 measured calls from different recordings. Within E. fuscus there were sufficient sample sizes for echolocation, complex, downsweep, inverted-u, oscillating, u-shaped, and upsweep calls. Within N. humeralis there were sufficient sample sizes for echolocation, downsweep, oscillating, QCF, and upsweep social calls. Within T. brasiliensis there were sufficient sample sizes for echolocation and complex calls. To reduce the number of variables, we ran all analyses of call characteristics with only duration, fpeak, bandwidth, s1, and s2. For each species, a random two thirds of the calls were used to train the model. The remaining third of the calls were used to test the model, with the manually assigned type compared to the model prediction.
To test for species specific signatures, we performed DFA tests across species within shared call types. We tested call types where there were two or more species with more than 15 measured calls of that type from different recordings. There were sufficient sample sizes to test for species specific differences in complex calls between E. fuscus and T. brasiliensis, in downsweeps between E. fuscus and N, humeralis, in oscillating calls between E. fuscus and N. humeralis, and in upsweeps between E. fuscus and N. humeralis. For comparisons of complex calls, we included number of pulses. Whether a call was in the training or test set for across species comparisons was independent of whether it was used in the training or test set for within species comparisons. For across species comparisons we conducted MANOVA tests to obtain a measure of significance.
Context of Social Calls
Temporal clustering of social calls
For analyses pertaining to the context of social call production, we only used detector nights between April 1st, 2017 to March 31st, 2018. As season was a variable tested, it was important to not have data from two springs, but only one summer, fall, and winter. There were often multiple social calls in a single bat pass and social calls within seconds of another in successive bat passes. To avoid treating non-independent calls as independent, we performed bout analysis on the intervals between successive social calls. Bout analysis models the length of the interval between behaviors as a function of a fast process, which determines the length of time between events in the same cluster, and a slow process which determines the length of time between clusters (Sibly et al., 1990). The parameters of the two-process model give the probability densities of fast and slow process events, and the ratio of fast to slow process events, which are used to determine the maximum interval for behaviors in the same cluster (Sibly et al., 1990; Langton et al., 1995). We used maximum likelihood bout analysis (Langton et al., 1995), as this method is not affected by subjective choices of histogram bin width (Luque and Guinet, 2007). Intervals were not recorded for the first social call of the detector night. Intervals between social calls of one species and social calls of another were not included. Intervals longer than 3 h were not included in the model, as the model produces divide by zero errors if excessively large and rare intervals are not excluded.
Using the maximum interval for events within the same bout criterion determined by bout analysis, we grouped together social calls that were not temporally independent of one another. Successive social calls with an interval shorter than the criterion were considered to be within the same bout, while successive social calls separated by an interval longer than the criterion were temporally independent. It is not possible to determine whether social calls are all from the same individual, or whether a call and response interaction includes multiple individuals. Temporal clusters of social calls and temporally isolated social calls were considered independent social call bouts.
Context of social call production
For all analyses of social call context, time of night was analyzed as hour after sunset, based on the time of sunset reported for Greensboro, North Carolina by the National Oceanic and Atmospheric Administration Solar Calculator (National Oceanic and Atmospheric Administration). We calculated species-specific bat activity per hour after sunset as the number of bat passes within that hour, for that species. Within E. fuscus and N. humeralis, we ran binary logistic regression on the presence-absence of at least one social call for that species independent of type, for each hour after sunset, as a function of hourly bat activity for that species and hour after sunset.
Within E. fuscus and N. humeralis, we used multivariate logistic regression to test the contexts of different social call types. We used randomly selected bat passes for each species without social calls to compare the contexts of when bats were producing social calls to when they were not. We modeled bout type as a function of hourly conspecific activity, hourly heterospecifics activity, the presence of foraging buzzes, season, hour after sunset, and site. Bouts were classified based on the type(s) of social calls present. Total number of calls, number of calls per type, and ordering of calls were not used to classify bouts. For each bout we manually determined whether it was in a bat pass with a foraging buzz. Winter was defined as December 21st to March 20th, spring as March 21st to June 20th, summer as June 21st to September 20th, and fall as September 21st to December 20th. We used the calendar definitions for seasons as we do not have information on the specific timing of seasonal events for bats in the North Carolina Piedmont.
We had small sample sizes for some bout types. For E. fuscus, we ran our analysis using bouts of only complex calls and bouts of only upsweep calls. For N. humeralis, we ran our analysis using bouts of only downsweep calls, bouts of only upsweep calls, and bouts containing downsweep and upsweep calls. For foraging activity, the absence of foraging buzzes was used as the reference category. For season, winter was used as the reference category. For site, the recreational field control site was used as the reference category. All statistical analyses were conducted in R (R Core Team, 2018). We used the packages MASS for DFA tests (Venables and Ripley, 2002), diveMove for bout analysis (Luque, 2007), nnet for multinomial logistic regression (Venables and Ripley, 2002), and ggplot2 for data visualization (Wickham, 2016).
Results
Species-Specific Differences in Social Call Production and Characteristics
Calls Recorded
We examined 123,007 recordings from 679 detector nights, 97,543 of which were recordings of bats and 25,464 of which were noise. Of the bat recordings, 2,883 recordings contained one or more bat social call (3.0%). Within the 2,883 recordings, 6,614 individual social calls were identified (Table 1). In 1,558 of the recordings with social calls, a single, identifiable species was present (54.0%), allowing the 3,772 social calls they contained to be assigned to as either E. fuscus, L. borealis, L. cinereus, N. humeralis, P. subflavus, or T. brasiliensis. In 1,147 recordings, two or more species were present (40.0%), and therefore the 2,475 social calls from these recordings could not be identified to a single species. The remaining 178 recordings appeared to contain only one species, but the echolocation calls were insufficient to determine which species (6.0%), and these contained 367 social calls.
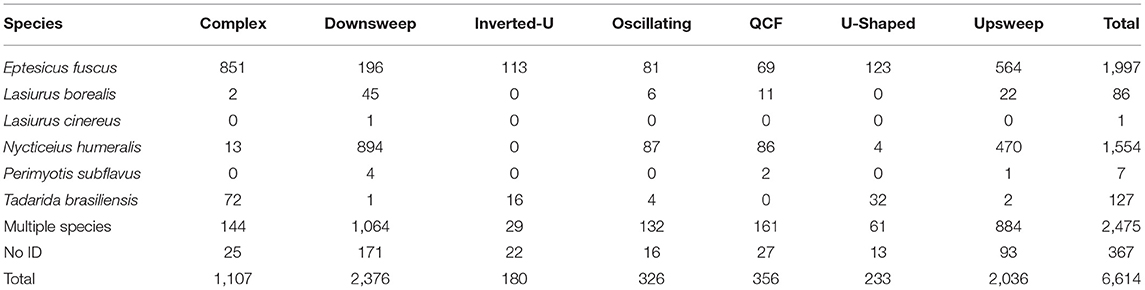
Table 1. Number of in-flight social calls, by species and call type, recorded at the UNCG Wetlands, Greensboro, NC, from March 15th 2017 to June 30th, 2018.
For single species bat passes with social calls, we compared manual classification to the automatic identification results. Manual and automatic classification agreed for 81.3% of the 1,171 passes assigned to a specific species by both methods (Supplemental Table 1). When looking specifically at bat passes at or above the match ratio threshold of 0.6, there was agreement for 90.6% of bat passes assigned to a specific species. Additionally, there were 421 bat passes that could be identified manually but not with automatic identification. Only three species produced sufficient sample sizes for statistical analyses: E. fuscus, N. humeralis, and T. brasiliensis. We observed all seven proposed social call types, though not all species produced all call types (Figure 1).
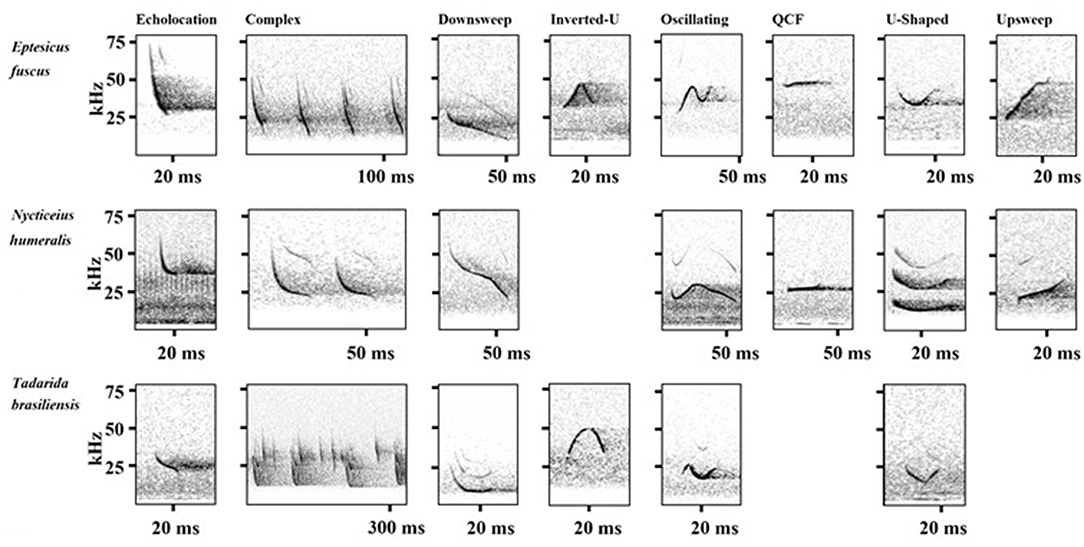
Figure 1. Representative spectrograms of echolocation and in-flight social calls of Eptesicus fuscus, Nycticeius humeralis, and Tadarida brasiliensis collected at the UNCG Wetlands, Greensboro, NC, from March 15th, 2017 to June 30th, 2018. Species-specific differences in spectral and temporal characteristics were tested for and detected in the complex calls of E. fuscus and T. brasiliensis [Wilks' λ: 0.290; c. F(6,216) = 88.0; p < 0.0001], the downsweep calls of E. fuscus and N. humeralis [Wilks' λ: 0.592; c. F(5,370) = 51.0; p < 0.0001], the oscillating calls of E. fuscus and N. humeralis [Wilks' λ: 0.317; c. F(5,42) = 18.1; p < 0.0001], and the upsweep calls of E. fuscus and N. humeralis [Wilks' λ: 0.280; c. F(5,383) = 196.6; p < 0.0001].
Species-Specific Differences in Call Production
We observed 28,598 bat passes that could be confidently identified as E. fuscus, 3,868 that could be confidently identified as N. humeralis, and 1,678 that could be confidently identified as T. brasiliensis. For bat passes classified using automatic identification, 74.4% of those labeled E. fuscus, 42.6% of those labeled N. humeralis, and 60.1% of those labeled T. brasiliensis were at or above the match ratio threshold of 0.6. Of E. fuscus bat passes, 682 (2.4%) contained at least one social call. Of N. humeralis bat passes, 771 (19.9%) contained at least one social call. Of T. brasiliensis bat passes, 63 (3.8%) contained at least one social call. The proportions of bat passes with social calls differed among species (X2 = 2474.7, d.f. = 2, p < 0.0001).
There were differences in the proportion of complex social calls among E. fuscus, N. humeralis, and T. brasiliensis (X2 = 875.4, d.f. = 2, p < 0.0001). Complex calls made up 42.6 % of E. fuscus social calls, 0.8% of N. humeralis social calls, and 56.7% of T. brasiliensis social calls (calculated from the values given in Table 1). For E. fuscus and T. brasiliensis, complex calls were the most common social call type produced. For N. humeralis, downsweep calls were the most common type produced (57.5% of N. humeralis social calls, calculated from the values given in Table 1). All seven proposed call types were observed to be produced by E. fuscus. There were no observations of N. humeralis producing inverted-u calls, or of T. brasiliensis producing QCF calls.
Call Classification
Spectral and temporal characteristics for E. fuscus, N. humeralis, and T. brasiliensis echolocation and social calls are reported in Supplemental Table 2. The E. fuscus DFA training set consisted of 357 calls and the test set consisted of 175 calls. The E. fuscus DFA produced five canonical dimensions explaining variation between call types. Duration, s1, and s2, were the most important variables for discriminating E. fuscus call types (Table 2). The model agreed with manual classification for 86.9% of the E. fuscus calls in the test set (Supplemental Table 3). For all call types except for oscillating, of which there were only four calls in the test set, the DFA agreed with manual classification more often than not for E. fuscus. Three of the eighteen echolocation calls were confused for social calls in E. fuscus, but no social calls were confused for echolocation calls. The N. humeralis DFA training set consisted of 460 calls, and the test set consisted of 237 calls. The N. humeralis DFA produced four canonical dimensions explaining variation between call types. Duration, s1, and s2 were most useful for discriminating call types of N. humeralis (Table 3). The model agreed with manual classification for 96.6% of N. humeralis calls (Supplemental Table 4). There were no instances of echolocation calls confused for social calls or social calls confused for echolocation calls in N. humeralis. The T. brasiliensis training set consisted of 38 calls and the test set consisted of 27 calls. With two groups, only a single canonical dimension was produced, which was primarily explained by variation in fpeak, bandwidth, and s1 (loading scores: duration < −0.001, fpeak: 0.114, bandwidth: −0.159, s1: −0.129, s2: 0.004). The DFA agreed with manual classification for all calls for T. brasiliensis.
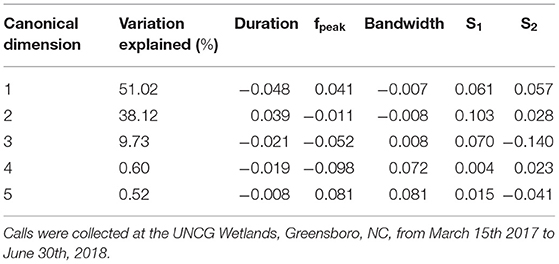
Table 2. Loading scores of call duration, peak frequency (fpeak), bandwidth, average slope of the first half of the call (S1) and average slope of the second half of the call (S2) contributing to the canonical dimensions used in the discriminant function analysis to discriminate call types within Eptesicus fuscus and percentage of variation explained by each dimension.
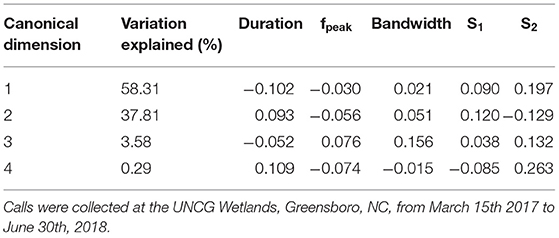
Table 3. Loading scores of call duration, peak frequency (fpeak), bandwidth, average slope of the first half of the call (S1) and average slope of the second half of the call (S2) contributing to the canonical dimensions used to discriminate call types within Nycticeius humeralis and percentage of variation explained by each dimension.
Species-Specific Differences Within Call Types
There were significant differences in the spectral and temporal characteristics of E. fuscus and T. brasiliensis complex social calls [Wilks' λ: 0.290; c. F(6, 216) = 88.0; p < 0.0001]. The training set for the DFA consisted of 147 calls, and the test set consisted of 76 calls. The most useful variables for discriminating between E. fuscus and T. brasiliensis complex social calls were fpeak, s1, and s2 (loading scores: duration: 0.006, pulses: −0.014, fpeak: −0.101, bandwidth: 0.008, s1: −0.119, s2: 0.226). The complex social calls of E. fuscus had a higher peak frequency and steeper slope than those of T. brasiliensis (Supplemental Table 2, Figure 1). Eptesicus fuscus complex calls consisted of multiple similar downsweeps, while the individual pulses of T. brasiliensis complex calls were downsweeps, u-shaped, or oscillating pulses. The DFA agreed with manual classification for 100% of calls.
There were significant differences in the spectral and temporal characteristics of E. fuscus and N. humeralis downsweep social calls [Wilks' λ: 0.592; c. F(5, 370) = 51.0; p < 0.0001]. The training set for the DFA consisted of 241 calls, and the test set consisted of 135 calls. The most useful variables for discriminating between the two species were duration and bandwidth (loading scores: duration: 0.136, fpeak: −0.003, bandwidth: 0.044, s1: −0.017, s2: 0.023). The downsweep social calls of N. humeralis were longer in duration and encompassed a wider bandwidth than those of E. fuscus (Supplemental Table 2, Figure 1). The DFA agreed with manual classification for 89.6% of calls.
There were significant differences in the spectral and temporal characteristics of E. fuscus and N. humeralis oscillating social calls [Wilks' λ: 0.317; c. F(5, 42) = 18.1; p < 0.0001]. The training set for the DFA consisted of 34 calls and the test set consisted of 14 calls. The most useful variables for discriminating between E. fuscus and N. humeralis oscillating social calls were fpeak, s1, and s2 (loading scores: duration: 0.040, fpeak: −0.118, bandwidth: −0.089, s1: 0.128, s2: −0.093). The oscillating social calls of N. humeralis had steeper average slopes for both the first and second halves of the call (Supplemental Table 2, Figure 1). The oscillating social calls of E. fuscus had a higher peak frequency (Supplemental Table 2, Figure 1). The DFA agreed with manual classification for 92.9% of calls.
There were significant differences in the spectral and temporal characteristics of E. fuscus and N. humeralis upsweep social calls [Wilks' λ: 0.280; c. F(5, 383) = 196.6; p < 0.0001]. The training set for the DFA consisted of 253 calls, and the test set consisted of 136 calls. The most important variables for discriminating between E. fuscus and N. humeralis upsweep social calls were duration, fpeak, and bandwidth (loading scores: duration: 0.106, fpeak: −0.145, bandwidth: −0.109, s1: −0.002, s2: −0.036). The upsweep social calls of N. humeralis were longer in duration than those of E. fuscus (Supplemental Table 2, Figure 1). The upsweep social calls of E. fuscus were of a higher frequency and encompassed a broader bandwidth than those of N. humeralis (Supplemental Table 2, Figure 1). The DFA agreed with manual classification for 93.4% of calls.
Context of Social Calls
Temporal Clustering of Social Calls
For analysis of call context, we used only detector nights from April 1st, 2017 to March 31st, 2018 to avoid having replicates for Spring but not for the other seasons. From 520 detector nights during this period, we examined 89,579 recordings, 69,410 were recordings of bats and 20,169 were noise. A total of 4,105 social calls were identified from 1,672 of these recordings (Table 4). Fitting the distribution of inter-call intervals to a two-process model suggested a maximum interval of 4.435 s for social calls within the same cluster (proportion of fast process events to slow process events: 0.568, probability density of fast process events: 1.573, probability density of slow process events: 0.002). Clustering together social calls within 4.435 s of another led to 1,727 bouts.
Social Call Production
Eptesicus fuscus was recorded during 1,484 detector hours, and E. fuscus social calls were recorded during 204 of those detector hours. Hourly E. fuscus activity was positively correlated with the probability of recording at least one E. fuscus social call (β: 0.042; SE: 0.003: p < 0.001). The probability of E. fuscus social call production was not correlated with time after sunset (β: −0.041; SE: 0.029; p: 0.157).
Nycticeius humeralis was recorded during 752 detector hours, and N. humeralis social calls were recorded during 181 of those detector hours. Hourly N. humeralis activity was positively correlated with the probability of recording at least one N. humeralis social call (β: 0.123; SE: 0.026; p < 0.001). The probability of N. humeralis social call production was not correlated with time after sunset (β: −0.031; SE: 0.027; p: 0.281).
Classification of Bout Types
We observed 67 types of bouts, based on the types on social calls contained in each cluster (Figure 2). Fifty-two types had fewer than ten observations, and twenty-three of those were only observed once. There were four bout types with sufficient sample sizes for analyses; complex bouts, downsweep bouts, downsweep-upsweep bouts, and upsweep bouts. Complex bouts, downsweep bouts, downsweep-upsweep bouts, and upsweep bouts made up 1,227 of the 1,727 bouts observed (71.1%). Production of complex bouts, downsweep bouts, downsweep-upsweep bouts, and upsweep bouts by species are given in Table 5.
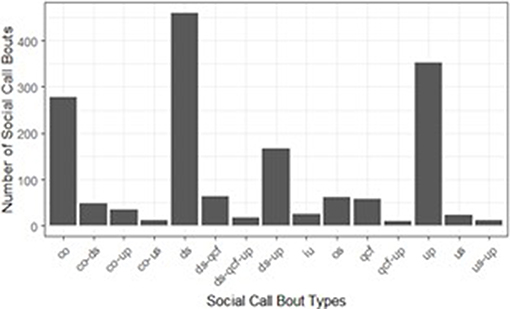
Figure 2. Common types of social call bouts, based on the types of individual social calls contained. Call type abbreviations: co, complex; ds, downsweep; iu, inverted-u; os, oscillating; qcf, quasi-constant frequency; up, upsweep; us, u-shaped. Calls were collected at the UNCG Wetlands, Greensboro, NC, from April 1st, 2017 to March 31st, 2018.
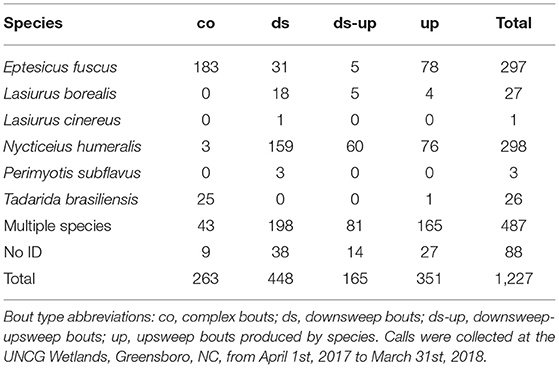
Table 5. Number of bouts, by species and type, for those types used in multivariate logistic regression.
Context of Bout Types
We analyzed the context of E. fuscus social call bouts in comparison to the context of 878 randomly selected E. fuscus bat passes without social calls. Within E. fuscus, type of social call bout was significantly correlated with hourly heterospecific activity, the presence of foraging buzzes, season, and site (Table 6). There was no difference in context between bat passes without social calls and social call bouts based on hourly conspecific activity or time of night (Table 6). The probability of a complex call bout relative to the probability of a bat pass without social calls was negatively correlated with hourly heterospecific activity (Figure 3A). Both bout types were more likely to be found with foraging buzzes than bat passes without social calls. Only 13.2% of bat passes without social calls contained foraging buzzes, while 41.2% of complex call bouts and 37.2% of upsweep bouts were found in association with a foraging buzz (Figure 3B). The probability of complex call bouts relative to bat passes without social calls was highest during the winter (Figure 3C). The probability of upsweep call bouts relative to bat passes without social calls was higher in spring than it was during the winter (Figure 3C). Complex call bout production varied less across season than upsweep bout production or bat passes without social calls (Figure 3C) The probabilities of either bout types were lower at the recreational field wetland (Figure 3D).
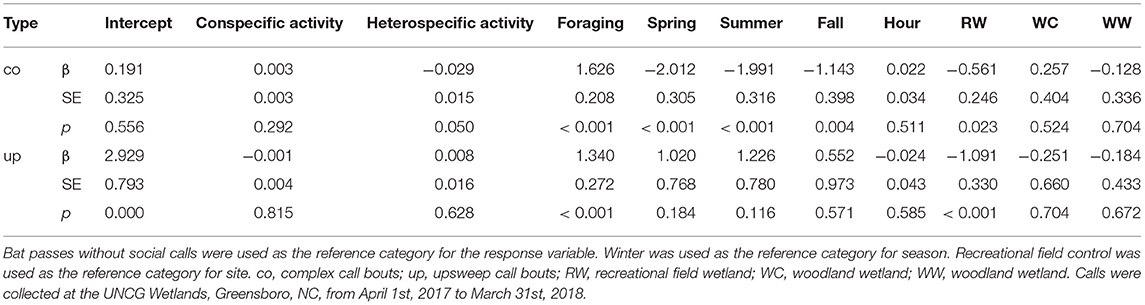
Table 6. Multinomial logistic regression results for the effect of hourly conspecific activity, hourly heterospecifics activity, foraging, season, hour after sunset and site on social calling bout type for Eptesicus fuscus.
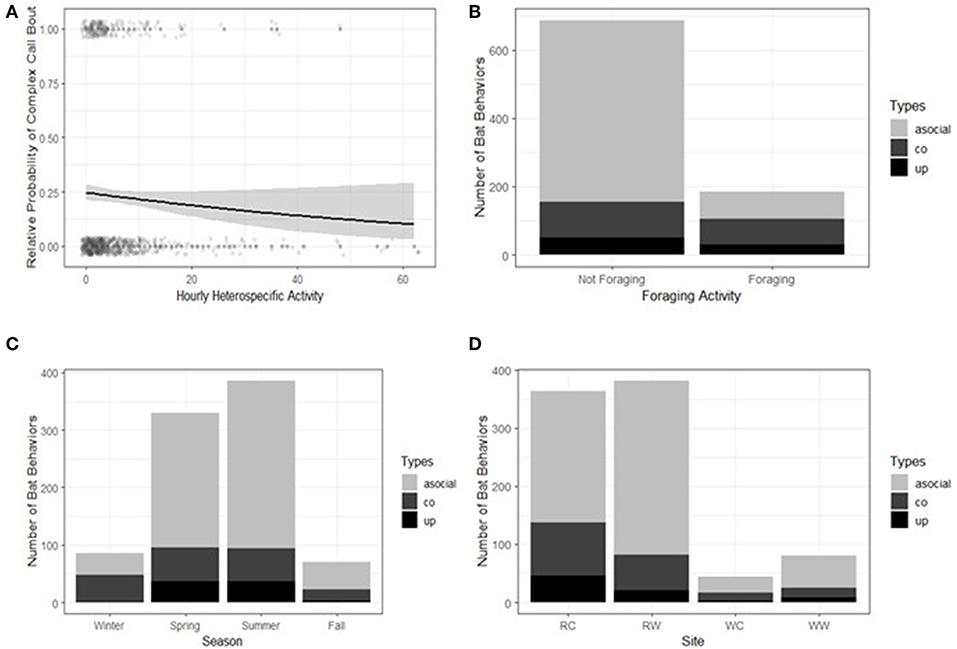
Figure 3. Context of Eptesicus fuscus social call bouts compared to the context of bat passes without social calls. (A) Probability of complex call bouts relative to passes without social calls was negatively correlated with heterospecific activity, (B) social call bout production varied with presence of foraging buzzes, (C) social call bout production varied with season, and (D) social call bout production varied with site. Bout type abbreviations: co, complex bouts; up, upsweep bouts. Site abbreviations: RC, recreational field control; RW, recreational field wetland; WC, woodland control; WC, woodland wetland. Calls were collected at the UNCG Wetlands, Greensboro, NC, from April 1st, 2017 to March 31st, 2018.
We analyzed the context of N. humeralis social call bouts in comparison to the context of 43 randomly selected N. humeralis bat passes without social calls. Within N. humeralis, the contexts of social call bouts differed from bat passes without social calls by season and site (Table 7). There was no significant relationship between hourly conspecific activity, hourly heterospecific activity, foraging buzzes, or time of night, and whether a social call bout was produced (Table 7). The probability of downsweep call bouts relative to bat passes without social calls was lower in the summer than it was during the winter (Figure 4A). The probability of downsweep-upsweep call bouts relative to bat passes without social calls was lower in spring, summer, and autumn than it was during the winter (Figure 4A). The probabilities of all three bout types were lower at the recreational wetland site (Figure 4B). The probabilities of downsweep-upsweep call bouts and upsweep bouts were lower at the woodlands control site (Figure 4B). The probability of downsweep-upsweep bouts was lower at the woodland wetland site (Figure 4B).
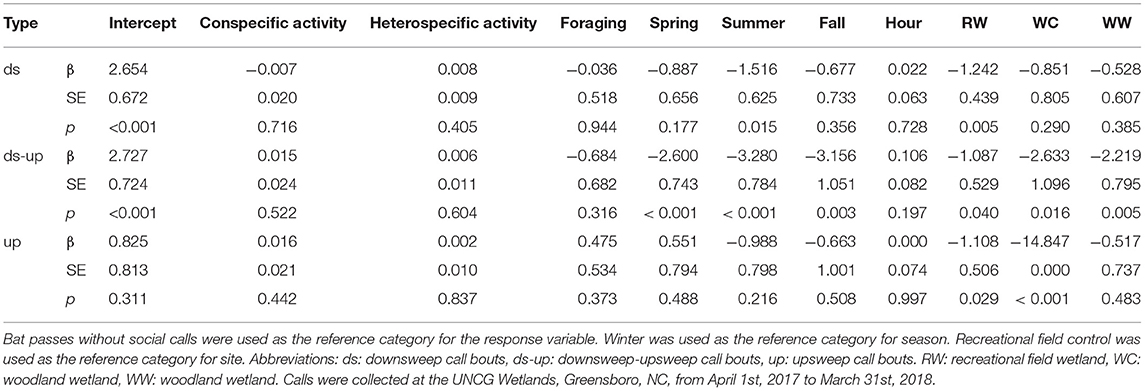
Table 7. Multinomial logistic regression results for the effect of hourly conspecific activity, hourly heterospecifics activity, foraging, season, hour after sunset and site on social calling bout type for Nycticeius humeralis.
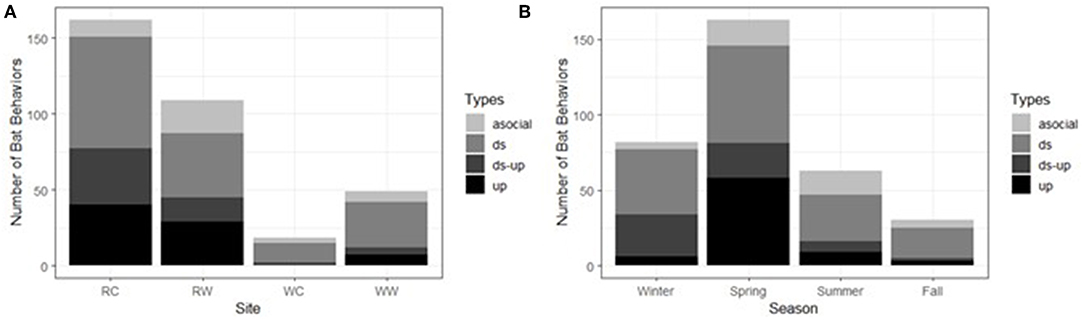
Figure 4. Context of Nycticeius humeralis social call bouts compared to the context of bat passes without social calls. Production of social calls varied with (A) season and (B) site Bout type abbreviations: ds, downsweep bouts; ds-up, downsweep-upsweep bouts; up, upsweep bouts. Site abbreviations: RC, recreational field control; RW, recreational field wetland; WC, woodland control; WC, woodland wetland. Calls were collected at the UNCG Wetlands, Greensboro, NC, from April 1st, 2017 to March 31st, 2018.
Discussion
The social call types detected using passive acoustic monitoring are broadly consistent with those from laboratory studies and studies of European species (Pfalzer and Kusch, 2003; Melendez et al., 2006; Wright et al., 2013; Middleton et al., 2014). Most of the call types we recorded for E. fuscus are consistent with the laboratory studies of Wright et al. (2013), however they did not observe the oscillating call observed herein, and we did not observe the short frequency modulated call they reported. To our knowledge this is the first time these social calls have been observed for E. fuscus in the field, as well as for most of the N. humeralis social calls in any context. The one N. humeralis call type which appears to have been described before was the oscillating call, which functions as a pup-isolation call in the roost (Scherrer and Wilkinson, 1993). The oscillating call of N. humeralis greatly resembles mother-pup calls used by T. brasiliensis in the roost (Bohn et al., 2008). The oscillating calls produced by T. brasiliensis in-flight did not resemble the N. humeralis oscillating call, but rather the sonar jamming oscillating calls reported by Corcoran and Conner (2014) or individual pulses from the complex calls. The song-like, complex calls of T. brasiliensis have mostly been described from studies in the roost or laboratory, however they have also been observed in flight (Bohn et al., 2008; Bohn and Gillam, 2018).
Social calls were differentiated from echolocation calls through visual examination of spectrogram shape, and this difference was supported by DFA. While downsweep social calls and echolocation calls both have frequency solely decreasing through the call, downsweep social calls are not likely to be abnormal echolocation calls. Within species, mean duration of downsweeps exceeded twice the duration of echolocation calls and frequency characteristics for downsweeps were consistently lower than corresponding frequency characteristics for echolocation calls. While T. brasiliensis echolocation calls do increase in duration when flying at high altitudes, the bandwidth decreases to near constant frequency, making it unlikely that the broadband downsweep calls are actually isolated calls from distant and high-altitude T. brasiliensis (Gillam et al., 2009). The echolocation calls of high-altitude T. brasiliensis still have a peak frequency of ~25 kHz, therefore it is unlikely that the higher frequency QCF calls were high altitude T. brasiliensis echolocation calls mistaken for social calls (Gillam et al., 2009).
We found species specific differences in the temporal and spectral characteristics of all call types tested. Analysis of the screech calls of 31 species of bats from 5 families in China showed species specific differences in social call characteristics correlated with phylogeny, morphology, and social group size (Luo et al., 2017). While three species are insufficient for a phylogenetic analysis of the causes of variation, it is interesting to note that the direction of species-specific differences between two species were not consistent across call types. Eptesicus fuscus upsweeps were higher bandwidth than N. humeralis upsweeps, while E. fuscus downsweeps were lower bandwidth than N. humeralis downsweeps, suggesting that there may not be a consistent phylogenetic signal across multiple call types.
The presence of species-specific signatures in social calls suggests the possibility that a bat listening to the call would be able to discern emitter species. However, playback experiments would be needed to determine if bats actually attend to these differences in social call characteristics. Playback studies have yielded mixed results as to whether bats attend to species-specific signatures (Russ et al., 2004; Schöner et al., 2010). Whether species discrimination occurs may depend on call function. Entering a roost of heterospecifics would likely be disadvantageous, and therefore species discrimination would be expected, while deterring a predator may be advantageous regardless of the species of the predator's immediate prey, and therefore species discrimination would not occur (Russ et al., 2004; Schöner et al., 2010). We found that social calls were often produced in multiple species bat passes, possibly suggesting some types may function for communicating with heterospecifics.
The most common call type for E. fuscus was the complex call, and the majority of complex calls were produced by E. fuscus. In a laboratory setting, E. fuscus complex calls were emitted when two bats were approaching the same insect (Wright et al., 2014). Complex calls functioned for food item defense, where the bat closer to the insect emitted the call and the second bat would alter its flight trajectory to avoid the emitter and the insect (Wright et al., 2014). We found that complex bouts were negatively correlated to heterospecific activity, suggesting this food item defense interaction occurs predominantly between conspecifics. While all species used in this study are insectivorous, they exhibit preferences for different insect taxa (Safi and Kerth, 2007). It is not surprising that a call associated with food item defense would be predominately used to communicate with conspecifics, as conspecifics present more competition for food than do heterospecifics. The use of complex calls to compete for food solely with conspecifics has also been observed in P. pipistrellus and P. pygmaeus, each of which only respond to conspecific complex calls (Barlow and Jones, 1997; Barratt et al., 1997). The social call production of E. fuscus suggests the most common in-flight social interaction for this species is to compete with conspecifics for food. Direct video observations and playbacks of complex calls to free flying E. fuscus could be used to test this hypothesis.
The complex and upsweep calls of E. fuscus exhibit seasonal patterns. While the number of complex call bouts remained fairly constant across seasons, they became proportionally more common in the winter due to the decrease in overall bat activity. As these calls appear to mediate foraging competition, it makes sense that they are relatively more common when resources are scarce. Relative production of upsweeps was highest during the spring. In a laboratory setting, upsweep calls were mostly produced by juvenile E. fuscus (Wright et al., 2013). However, use by juveniles cannot explain the prevalence of upsweep calls in the spring, as the young of the year have not yet been born (Bradshaw, 1962; Rydell, 1989).
Upsweep calls were commonly produced by E. fuscus and N. humeralis. However, upsweep calls are associated with different contexts in the two species. The upsweeps of E. fuscus exhibited an association with foraging buzzes that was not seen with the upsweeps of N. humeralis, suggesting the calls serves different functions. This is unusual, as previous across species studies on bat social calls have shown that the same call types serve the same functions across species, even when species specific signatures are present (Pfalzer and Kusch, 2003; Russ et al., 2004; Carter et al., 2012; Luo et al., 2017). Further studies on the contexts of upsweep calls in other vesper bats coupled with phylogenetic analysis could be used to test if the upsweeps of E. fuscus and N. humeralis are due to convergent call evolution, or if the function of the call changed over evolutionary history.
In other bat species, downsweep calls have been observed to function in maintaining group cohesion (Pfalzer and Kusch, 2003; Carter et al., 2012; Gillam and Chaverri, 2012). Production of downsweep, downsweep-upsweep, and upsweep bouts was found similar contexts, suggesting some redundancy in downsweep and upsweep call function. Calls to maintain group cohesion would be advantageous when commuting as a group to foraging patches, seen in Phyllostomus hastatus (Wilkinson and Boughman, 1998). Female N. humeralis cooperatively forage (Wilkinson, 1992). We found that social call production relative to bat activity for N. humeralis far exceeded that of other species, and the most common call type produced by N. humeralis was the downsweep. Maintaining group cohesion while commuting could explain why N. humeralis produces social calls, particularly downsweeps, more often than other species. However, the infrequent association between either downsweep or upsweep calls with foraging buzzes suggests that, even if used to maintain group cohesion when commuting, N. humeralis does not use them to advertise foraging patches. Bats respond to the foraging buzzes of conspecifics (Dechmann et al., 2009). It is possible that cooperatively foraging N. humeralis use social calls to maintain cohesion when commuting, and then attend to foraging buzzes as a cue when prey have been located.
Downsweep, downsweep-upsweep, and upsweep bouts were often recorded in bat passes where multiple species were present. Maintaining group cohesion in-flight would only occur with conspecifics from the same social groups. Cambell's monkeys, Cercopithecus campbelli, use contact call types that encode more individual information when in the presence of heterospecifics, likely due to the more complex acoustic environment (Coye et al., 2018). If a similar phenomenon occurs in bats, that may explain the use of contact calls when heterospecifics are present. While bats can attend to echolocation calls to maintain group cohesion (Dechmann et al., 2009; Egert-Berg et al., 2018), dedicated social calls may still be useful for group cohesion as social calls can encode more information (Gillam and Fenton, 2016) and are often louder and lower frequency, allowing for transmission over longer distances (Middleton et al., 2014).
Alternatively, downsweep and upsweep calls may serve multiple functions depending on context. Phyllostomus hastatus screech calls function for anti-predator mobbing (Knörnschild and Tschapka, 2012) and cohesion when commuting (Wilkinson and Boughman, 1998). Pippistrellus spp. complex calls function in mate attraction (Lundberg and Gerell, 1986) and foraging competition (Barlow and Jones, 1997). The exchange of contact calls by group members during agonistic interactions with non-group members has also been observed in birds (Nowicki, 1983; Hopp et al., 2001). Heterospecifics would be inherently non-group members. The majority of downsweep, downsweep-upsweep, and upsweep bouts were produced in the spring, when bats are returning from hibernacula and winter-feeding grounds (Scales and Wilkins, 2007; Valdez and Cryan, 2009). Therefore, agonistic encounters with unfamiliar individuals would be highest when bats are first starting to be active on the landscape again. Male N. humeralis are solitary living in the spring and summer (Perry and Thill, 2008; Hein et al., 2009), therefore any bat a male N. humeralis encounters would be a non-group member. An increased rate of agonistic signal production when presented with unfamiliar individuals has been observed in birds and frogs (Lesbarrères and Lodé, 2002; Briefer et al., 2008). Playbacks in spring versus other seasons could also be used to test this hypothesis.
A possible function for the apparent signal redundancy across species for downsweep and upsweep calls may be that call type usage varies by signaler identity. Wright et al. (2013) found that the likelihood of E. fuscus upsweep calls were positively correlated with the number of juveniles and males in a dyad. If age or sex specific social call use exists for other species, then social calls may be useful for assessing population demographics in ecological and conservation passive acoustic monitoring studies. Sexual segregation of foraging habitats has been observed in some bat species (Senior et al., 2005; Safi et al., 2007). The use of Bayesian statistics to infer sex from echolocation call characteristics has been suggested as a method for discerning sex ratios from passive acoustic monitoring (Lehnen et al., 2018), however some bat species, including E. fuscus, do not exhibit sex-specific differences in echolocation call characteristics (Heller et al., 1989; Masters et al., 1995).
Eptesicus fuscus, N. humeralis, and T. brasiliensis differ in how often they produce social calls associated with different contexts. Nycticeius humeralis produces social calls the most often. Downsweeps and upsweeps are the main call types used by N. humeralis, and appear to be broad functioning contact calls. The higher use of contact calls may be related to females cooperatively foraging (Wilkinson, 1992), and the lower sociality of males (Perry and Thill, 2008; Hein et al., 2009), leading to increased contact with unfamiliar individuals. Eptesicus fuscus most commonly produced a call type used for foraging competition (Wright et al., 2014), used specifically to compete with conspecifics. E. fuscus also commonly produced upsweeps, which in laboratory settings is mostly produced by juvenile and male bats (Wright et al., 2013), however E. fuscus upsweep calls are associated with foraging buzzes, unlike N. humeralis upsweep calls. Tadarida brasiliensis social calls were occasionally recorded, and were primarily complex calls. Eptesicus fuscus, N. humeralis, and T. brasiliensis have different social behaviors in the roosts (Davis et al., 1962; Wilkinson, 1992; Willis and Brigham, 2004; Bohn et al., 2008; Perry and Thill, 2008; Hein et al., 2009). Differential usage of social calls suggests these species also exhibit different social behaviors while in flight.
Data Availability Statement
The data used for this study have been made available at https://doi.org/10.6084/m9.figshare.8145926.v2.
Ethics Statement
The recording of bats in this study was approved by the UNCG Institutional Animal Care and Use Committee and by the North Carolina Wildlife Resources Commission.
Author Contributions
MK-R and BS conceived of the study. BS identified bat passes with social calls, identified bat passes to species, analyzed call spectral and temporal characteristics, performed all statistical analyses, and wrote the initial draft of the manuscript. HL verified the species identification for many of the bat passes, contributed to the selection of statistical analyses, and contributed to variable selection for the context of social call production. MK-R contributed to the selection of statistical analyses and to the writing of the manuscript.
Funding
The recordings used in this study were generated as a part of the UNCG Wetlands project, which was funded by a Duke Energy Water Resource Fund Grant (Grant 20161820), the UNCG Green Fund, and the UNCG Provost Office.
Conflict of Interest
The authors declare that the research was conducted in the absence of any commercial or financial relationships that could be construed as a potential conflict of interest.
Acknowledgments
We would like to thank Olav Rueppell, Malcolm Schug, Aaron Corcoran, Bryan McLean, Angela Larsen, Rada Petric, Kevin Parker, and Becky Malin for their valuable input toward the development of this project and the writing of this manuscript. We would like to thank Greg Brosek, Tronjay Davis, Ashley Rose, Sean Costner, and Carlos Castellon, who helped identify recordings containing social calls. The UNCG Biology Department provided support for this research. Thanks to the UNCG Wetlands committee for allowing us to use this dataset, and to their graduate assistant Kristina Morales who maintained the recording equipment. The Wetlands Project was made possible through: The City of Greensboro, Greensboro Science Center, UNCG Biology Department, UNCG Chemistry Department, UNCG Geography Department, UNCG Office of Provost, Peabody Park Preservation Committee, UNCG Office of Sustainability, The UNCG Undergraduate Research, Scholarship, and Creativity Office, UNCG Facilities Design and Construction, UNCG Facilities Grounds and Garage, and the community of volunteers aiding with the installation of the Wetlands. The Wetlands Project was funded by grants from the Duke Energy Water Resources Fund, the UNCG Green Fund, and the UNCG Provost Office. We would like to thank the two reviewers whose feedback has improved this paper.
Supplementary Material
The Supplementary Material for this article can be found online at: https://www.frontiersin.org/articles/10.3389/fevo.2019.00441/full#supplementary-material
References
Barclay, R. M., Faure, P. A., and Farr, D. R. (1988). Roosting behavior and roost selection by migrating silver-haired bats (Lasionycteris noctivagans). J. Mammal. 69, 821–825. doi: 10.2307/1381639
Barclay, R. M., Fenton, M. B., and Thomas, D. W. (1979). Social behavior of the little brown bat, Myotis lucifugus. Behav. Ecol. Sociobiol. 6, 137–146. doi: 10.1007/BF00292560
Barlow, K. E., and Jones, G. (1997). Function of pipistrelle social calls: field data and a playback experiment. Anim. Behav. 53, 991–999. doi: 10.1006/anbe.1996.0398
Barratt, E. M., Deaville, R., Burland, T. M., Bruford, M. W., Jones, G., Racey, P. A., et al. (1997). DNA answers the call of pipistrelle bat species. Nature 387:138. doi: 10.1038/387138b0
Bartonička, T., Rehák, Z., and Gaisler, J. (2007). Can pipistrelles, Pipistrellus pipistrellus (Schreber, 1774) and Pipistrellus pygmaeus (Leach, 1825), foraging in a group, change parameters of their signals? J. Zool. 272, 194–201. doi: 10.1111/j.1469-7998.2006.00255.x
Behr, O., and von Helversen, O. (2004). Bat serenades—complex courtship songs of the sac-winged bat (Saccopteryx bilineata). Behav. Ecol. Sociobiol. 56, 106–115. doi: 10.1007/s00265-004-0768-7
Blumstein, D. T., and Armitage, K. B. (1997). Does sociality drive the evolution of communicative complexity? A comparative test with ground-dwelling sciurid alarm calls. Am. Nat. 150, 179–200. doi: 10.1086/286062
Bohn, K. M., and Gillam, E. H. (2018). In-flight social calls: a primer for biologists and managers studying echolocation. Can. J. Zool. 96, 787–800. doi: 10.1139/cjz-2017-0188
Bohn, K. M., Schmidt-French, B., Ma, S. T., and Pollak, G. D. (2008). Syllable acoustics, temporal patterns, and call composition vary with behavioral context in Mexican free-tailed bats. J. Acoust. Soc. Am. 124, 1838–1848. doi: 10.1121/1.2953314
Bradbury, J. W. (1977). Lek mating behavior in the hammer-headed bat. Ethology 45, 225–255. doi: 10.1111/j.1439-0310.1977.tb02120.x
Bradshaw, G. V. (1962). Reproductive cycle of the California leaf-nosed bat, Macrotus californicus. Science 136, 645–646. doi: 10.1126/science.136.3516.645
Briefer, E., Rybak, F., and Aubin, T. (2008). When to be a dear enemy: flexible acoustic relationships of neighbouring skylarks, Alauda arvensis. Anim. Behav. 76, 1319–1325. doi: 10.1016/j.anbehav.2008.06.017
Britzke, E. R., Gillam, E. H., and Murray, K. L. (2013). Current state of understanding of ultrasonic detectors for the study of bat ecology. Acta Theriol. 58, 109–117. doi: 10.1007/s13364-013-0131-3
Buchler, E. R. (1980). The development of flight, foraging, and echolocation in the little brown bat (Myotis lucifugus). Behav. Ecol. Sociobiol. 6, 211–218. doi: 10.1007/BF00569202
Budenz, T., Heib, S., and Kusch, J. (2009). Functions of bat social calls: the influence of local abundance, interspecific interactions and season on the production of pipistrelle (Pipistrellus pipistrellus) type D social calls. Acta Chiropterol. 11, 173–182. doi: 10.3161/150811009X465794
Burns, L. E., and Broders, H. G. (2015). Maximizing mating opportunities: higher autumn swarming activity in male versus female Myotis bats. J. Mammal. 96, 1326–1336. doi: 10.1093/jmammal/gyv141
Carter, G. G., Logsdon, R., Arnold, B. D., Menchaca, A., and Medellin, R. A. (2012). Adult vampire bats produce contact calls when isolated: acoustic variation by species, population, colony, and individual. PLoS ONE 7:e38791. doi: 10.1371/journal.pone.0038791
Constantine, D. G. (1966). Ecological observations on lasiurine bats in Iowa. J. Mammal. 47, 34–41. doi: 10.2307/1378064
Corcoran, A. J., and Conner, W. E. (2014). Bats jamming bats: food competition through sonar interference. Science 346, 745–747. doi: 10.1126/science.1259512
Coye, C., Ouattara, K., Arlet, M. E., Lemasson, A., and Zuberbühler, K. (2018). Flexible use of simple and combined calls in female Campbell's monkeys. Anim. Behav. 141, 171–181. doi: 10.1016/j.anbehav.2018.05.014
Davidson, S. M., and Wilkinson, G. S. (2002). Geographic and individual variation in vocalizations by male Saccopteryx bilineata (Chiroptera: Emballonuridae). J. Mammal. 83, 526–535.2.0. doi: 10.1644/1545-1542(2002)083andlt;0526:GAIVIVandgt;2.0.CO;2
Davidson, S. M., and Wilkinson, G. S. (2004). Function of male song in the greater white-lined bat, Saccopteryx bilineata. Anim. Behav. 67, 883–891. doi: 10.1016/j.anbehav.2003.06.016
Davis, R. B., Herreid, C. F., and Short, H. L. (1962). Mexican free-tailed bats in Texas. Ecol. Monogr. 32, 311–346. doi: 10.2307/1942378
Dechmann, D. K., Heucke, S. L., Giuggioli, L., Safi, K., Voigt, C. C., and Wikelski, M. (2009). Experimental evidence for group hunting via eavesdropping in echolocating bats. Proc. R. Soc. Lond. B Biol. Sci. (2009) 276, 2721–2728. doi: 10.1098/rspb.2009.0473
Egert-Berg, K., Hurme, E. R., Greif, S., Goldstein, A., Harten, L., Flores-Martínez, J. J., et al. (2018). Resource ephemerality drives social foraging in bats. Curr. Biol., 28, 3667–3673. doi: 10.1016/j.cub.2018.09.064
Ford, W. M., Britzke, E. R., Dobony, C. A., Rodrigue, J. L., and Johnson, J. B. (2011). Patterns of acoustical activity of bats prior to and following white-nose syndrome occurrence. J. Fish Wildl. Manag. 2, 125–134. doi: 10.3996/042011-JFWM-027
Freeberg, T. M. (2006). Social complexity can drive vocal complexity: group size influences vocal information in Carolina chickadees. Psychol. Sci. 17, 557–561. doi: 10.1111/j.1467-9280.2006.01743.x
Freeberg, T. M., Dunbar, R. I., and Ord, T. J. (2012). Social complexity as a proximate and ultimate factor in communicative complexity. Philos. Trans. R. Soc. B Biol. Sci. 367, 1785–1801. doi: 10.1098/rstb.2011.0213
Garroway, C. J., and Broders, H. G. (2007). Nonrandom association patterns at northern long-eared bat maternity roosts. Can. J. Zool. 85, 956–964. doi: 10.1139/Z07-079
Gillam, E., and Fenton, M. B. (2016). “Roles of acoustic social communication in the lives of bats,” in Bat Bioacoustics, eds M. B. Fenton, A. D. Grinnell, A. N. Popper, and R. R. Fay (New York, NY: Springer), 117–139.
Gillam, E. H., and Chaverri, G. (2012). Strong individual signatures and weaker group signatures in contact calls of Spix's disc-winged bat, Thyroptera tricolor. Anim. Behav. 83, 269–276. doi: 10.1016/j.anbehav.2011.11.002
Gillam, E. H., McCracken, G. F., Westbrook, J. K., Lee, Y.-F., Jensen, M. L., and Balsley, B. B. (2009). Bats aloft: variability in echolocation call structure at high altitudes. Behav. Ecol. Sociobiol. 64, 69–79. doi: 10.1007/s00265-009-0819-1
Grider, J. F., Larsen, A. L., Homyack, J. A., and Kalcounis-Rueppell, M. C. (2016). Winter activity of coastal plain populations of bat species affected by white-nose syndrome and wind energy facilities. PLoS ONE 11:e0166512. doi: 10.1371/journal.pone.0166512
Heckel, G., and von Helversen, O. (2002). Male tactics and reproductive success in the harem polygynous bat Saccopteryx bilineata. Behav. Ecol. 13, 750–756. doi: 10.1093/beheco/13.6.750
Hein, C. D., Miller, K. V., and Castleberry, S. B. (2009). Evening bat summer roost-site selection on a managed pine landscape. J. Wildl. Manag. 73, 511–517. doi: 10.2193/2008-211
Heller, K.-G., and Helversen, O. v (1989). Resource partitioning of sonar frequency bands in rhinolophoid bats. Oecologia 80, 178–186. doi: 10.1007/BF00380148
Hopp, S. L., Jablonski, P., and Brown, J. L. (2001). Recognition of group membership by voice in Mexican jays, Aphelocoma ultramarina. Anim. Behav. 62, 297–303. doi: 10.1006/anbe.2001.1745
Insley, S. J. (1992). Mother-offspring separation and acoustic stereotypy: a comparison of call morphology in two species of pinnipeds. Behaviour 120, 103–122. doi: 10.1163/156853992X00237
Jones, G., and Siemers, B. M. (2011). The communicative potential of bat echolocation pulses. J. Comp. Physiol. A 197, 447–457. doi: 10.1007/s00359-010-0565-x
Kalcounis-Rueppell, M. C., Briones, K. M., Homyack, J. A., Petric, R., Marshall, M. M., and Miller, D. A. (2013). Hard forest edges act as conduits, not filters, for bats. Wildl. Soc. Bull. 37, 571–576. doi: 10.1002/wsb.289
Kalcounis-Rueppell, M. C., Payne, V. H., Huff, S. R., and Boyko, A. L. (2007). Effects of wastewater treatment plant effluent on bat foraging ecology in an urban stream system. Biol. Conserv. 138, 120–130. doi: 10.1016/j.biocon.2007.04.009
Kalcounis-Rueppell, M. C., Petric, R., and Marler, C. (2018). The bold, silent type: predictors of ultrasonic vocalizations in the genus Peromyscus. Front. Ecol. Evol. 6:198. doi: 10.3389/fevo.2018.00198
Keeley, A. T., and Keeley, B. W. (2004). The mating system of Tadarida brasiliensis (Chiroptera: Molossidae) in a large highway bridge colony. J. Mammal. 85, 113–119. doi: 10.1644/BME-004
Knörnschild, M., Nagy, M., Metz, M., Mayer, F., and von Helversen, O. (2012). Learned vocal group signatures in the polygynous bat Saccopteryx bilineata. Anim. Behav. 84, 761–769. doi: 10.1016/j.anbehav.2012.06.029
Knörnschild, M., and Tschapka, M. (2012). Predator mobbing behaviour in the greater spear-nosed bat, Phyllostomus hastatus. Chiropt. Neotropical 18, 1132–1135.
Knörnschild, M., and Von Helversen, O. (2008). Nonmutual vocal mother–pup recognition in the greater sac-winged bat. Anim. Behav. 76, 1001–1009. doi: 10.1016/j.anbehav.2008.05.018
Kunz, T. H., and Parsons, S. (2009). Ecological and Behavioral Methods for the Study of Bats. Baltimore, MD: Johns Hopkins University Press.
Kurta, A., Winhold, L., Whitaker, J. O. Jr., and Foster, R. (2007). Range expansion and changing abundance of the eastern pipistrelle (Chiroptera: Vespertilionidae) in the central Great Lakes region. Am. Midl. Nat. 157, 404–411. doi: 10.1674/0003-0031(2007)157[404:REACAO]2.0.CO;2
Langton, S. D., Collett, D., and Sibly, R. M. (1995). Splitting behaviour into bouts; a maximum likelihood approach. Behaviour 781–799. doi: 10.1163/156853995X00144
Lehnen, L., Schorcht, W., Karst, I., Biedermann, M., Kerth, G., and Puechmaille, S. J. (2018). Using Approximate Bayesian Computation to infer sex ratios from acoustic data. PLoS ONE 13:e0199428. doi: 10.1371/journal.pone.0199428
Lesbarrères, D., and Lodé, T. (2002). Variations in male calls and responses to an unfamiliar advertisement call in a territorial breeding anuran, Rana dalmatina: evidence for a “dear enemy” effect. Ethol. Ecol. Evol. 14, 287–295. doi: 10.1080/08927014.2002.9522731
Li, H., and Kalcounis-Rueppell, M. (2018). Separating the effects of water quality and urbanization on temperate insectivorous bats at the landscape scale. Ecol. Evol. 8, 667–678. doi: 10.1002/ece3.3693
Li, H., and Wilkins, K. T. (2014). Patch or mosaic: bat activity responds to fine-scale urban heterogeneity in a medium-sized city in the United States. Urban Ecosyst. 17, 1013–1031. doi: 10.1007/s11252-014-0369-9
Loeb, S. C., Rodhouse, J. T, Ellison, E. L, Lausen, L., et al. (2015). A Plan for the North American Bat Monitoring Program (NABat). Gen Tech Rep SRS-208 US Dep. Agric. For. Serv. South. Res. Stn, Asheville, NC. 208, 1–100.
Lundberg, K., and Gerell, R. (1986). Territorial advertisement and mate attraction in the bat Pipistrellus pipistrellus. Ethology 71, 115–124. doi: 10.1111/j.1439-0310.1986.tb00577.x
Luo, B., Huang, X., Li, Y., Lu, G., Zhao, J., Zhang, K., et al. (2017). Social call divergence in bats: a comparative analysis. Behav. Ecol. 28, 533–540. doi: 10.1093/beheco/arw184
Luque, S., and Guinet, C. (2007). A maximum likelihood approach for identifying dive bouts improves accuracy, precision and objectivity. Behaviour 144, 1315–1332. doi: 10.1163/156853907782418213
Masters, W. M., Raver, K. A., and Kazial, K. A. (1995). Sonar signals of big brown bats, Eptesicus fuscus, contain information about individual identity, age and family affiliation. Anim. Behav. 50, 1243–1260. doi: 10.1016/0003-3472(95)80041-7
Matsumura, S. (1979). Mother-infant communication in a horseshoe bat (Rhinolophus ferrumequinum nippon): development of vocalization. J. Mammal. 60, 76–84. doi: 10.2307/1379760
McComb, K., and Semple, S. (2005). Coevolution of vocal communication and sociality in primates. Biol. Lett. 1, 381–385. doi: 10.1098/rsbl.2005.0366
Medvin, M. B., Stoddard, P. K., and Beecher, M. D. (1993). Signals for parent-offspring recognition: a comparative analysis of the begging calls of cliff swallows and barn swallows. Anim. Behav. 45, 841–850. doi: 10.1006/anbe.1993.1105
Melendez, K. V., Jones, D. L., and Feng, A. S. (2006). Classification of communication signals of the little brown bat. J. Acoust. Soc. Am. 120:2. doi: 10.1121/1.2211488
Middleton, N., Froud, A., and French, K. (2014). Social calls of the bats of Britain and Ireland. Exeter, UK: Pelagic Publishing Ltd.
Middleton, N. E. (2006). A study of the emission of social calls by Pipistrellus spp. within central Scotland; including a description of their typical social call structure. BaTML Publ. 3, 23–28.
Mundry, R., and Sommer, C. (2007). Discriminant function analysis with nonindependent data: consequences and an alternative. Anim. Behav. 74, 965–976. doi: 10.1016/j.anbehav.2006.12.028
Musolf, K., Meindl, S., Larsen, A. L., Kalcounis-Rueppell, M. C., and Penn, D. J. (2015). Ultrasonic vocalizations of male mice differ among species and females show assortative preferences for male calls. PLoS ONE 10:e0134123. doi: 10.1371/journal.pone.0134123
Nowicki, S. (1983). Flock-specific recognition of chickadee calls. Behav. Ecol. Sociobiol. 12, 317–320. doi: 10.1007/BF00302899
O'Farrell, M. J., and Gannon, W. L. (1999). A comparison of acoustic versus capture techniques for the inventory of bats. J. Mammal. 80, 24–30. doi: 10.2307/1383204
O'Farrell, M. J., Miller, B. W., and Gannon, W. L. (1999). Qualitative identification of free-flying bats using the Anabat detector. J. Mammal. 80, 11–23. doi: 10.2307/1383203
Parker, K. A., Springall, B. T., Garshong, R. A., Malachi, A. N., Dorn, L. E., Costa-Terryll, A., et al. (2019). Rapid Increases in Bat Activity and Diversity after Wetland Construction in an Urban Ecosystem. Wetlands 39:717. doi: 10.1007/s13157-018-1115-5
Patriquin, K. J., Leonard, M. L., Broders, H. G., and Garroway, C. J. (2010). Do social networks of female northern long-eared bats vary with reproductive period and age? Behav. Ecol. Sociobiol. 64, 899–913. doi: 10.1007/s00265-010-0905-4
Perry, R. W., and Thill, R. E. (2008). Diurnal roosts of male evening bats (Nycticeius humeralis) in diversely managed pine-hardwood forests. Am. Midl. Nat. 160, 374–386. doi: 10.1674/0003-0031(2008)160[374:DROMEB]2.0.CO;2
Pfalzer, G., and Kusch, J. (2003). Structure and variability of bat social calls: implications for specificity and individual recognition. J. Zool. 261, 21–33. doi: 10.1017/S0952836903003935
Pollard, K. A. (2011). Making the most of alarm signals: the adaptive value of individual discrimination in an alarm context. Behav. Ecol. 22, 93–100. doi: 10.1093/beheco/arq.179
R Core Team (2018). R: A Language and Environment for Statistical Computing. R Foundation for Statistical Computing, Vienna. Available online at: https://www.R-project.org/
Rendell, L. E., Matthews, J. N., Gill, A., Gordon, J. C. D., and Macdonald, D. W. (1999). Quantitative analysis of tonal calls from five odontocete species, examining interspecific and intraspecific variation. J. Zool. 249, 403–410. doi: 10.1111/j.1469-7998.1999.tb01209.x
Russ, J. M., Jones, G., Mackie, I. J., and Racey, P. A. (2004). Interspecific responses to distress calls in bats (Chiroptera: Vespertilionidae): a function for convergence in call design? Anim. Behav. 67, 1005–1014. doi: 10.1016/j.anbehav.2003.09.003
Rydell, J. (1989). Feeding activity of the northern bat Eptesicus nilssoni during pregnancy and lactation. Oecologia 80, 562–565. doi: 10.1007/BF00380082
Safi, K., and Kerth, G. (2007). Comparative analyses suggest that information transfer promoted sociality in male bats in the temperate zone. Am. Nat. 170, 465–472. doi: 10.1086/520116
Safi, K., König, B., and Kerth, G. (2007). Sex differences in population genetics, home range size and habitat use of the parti-colored bat (Vespertilio murinus, Linnaeus 1758) in Switzerland and their consequences for conservation. Biol. Conserv. 137, 28–36. doi: 10.1016/j.biocon.2007.01.011
Scales, J. A., and Wilkins, K. T. (2007). Seasonality and fidelity in roost use of the Mexican free-tailed bat, Tadarida brasiliensis, in an urban setting. West. North Am. Nat. 67, 402–408. doi: 10.3398/1527-0904(2007)67[402:SAFIRU]2.0.CO;2
Scherrer, J. A., and Wilkinson, G. S. (1993). Evening bat isolation calls provide evidence for heritable signatures. Anim. Behav. 46, 847–860. doi: 10.1006/anbe.1993.1270
Schimpp, S. A., Li, H., and Kalcounis-Rueppell, M. C. (2018). Determining species specific nightly bat activity in sites with varying urban intensity. Urban Ecosyst. 21:541. doi: 10.1007/s11252-018-0737-y
Schöner, C. R., Schöner, M. G., and Kerth, G. (2010). Similar is not the same: social calls of conspecifics are more effective in attracting wild bats to day roosts than those of other bat species. Behav. Ecol. Sociobiol. 64, 2053–2063. doi: 10.1007/s00265-010-1019-8
Senior, P., Butlin, R. K., and Altringham, J. D. (2005). Sex and segregation in temperate bats. Proc. R. Soc. Lond. B Biol. Sci. 272, 2467–2473. doi: 10.1098/rspb.2005.3237
Sibly, R. M., Nott, H. M. R., and Fletcher, D. J. (1990). Splitting behaviour into bouts. Anim. Behav. 39, 63–69. doi: 10.1016/S0003-3472(05)80726-2
Suthers, R. A. (1965). Acoustic orientation by fish-catching bats. J. Exp. Zool. Part Ecol. Genet. Physiol. 158, 319–347. doi: 10.1002/jez.1401580307
Thomas, D. W., Brock Fenton, M., and Barclay, R. M. (1979). Social behavior of the little brown bat, Myotis lucifugus. Behav. Ecol. Sociobiol. 6, 129–136. doi: 10.1007/BF00292559
Valdez, E. W., and Cryan, P. M. (2009). Food habits of the hoary bat (Lasiurus cinereus) during spring migration through New Mexico. Southwest. Nat. 54, 195–200. doi: 10.1894/PS-45.1
Vaughan, T. A., and Vaughan, R. P. (1986). Seasonality and the behavior of the African yellow-winged bat. J. Mammal. 67, 91–102. doi: 10.2307/1381005
Venables, W. N., and Ripley, B. D. (2002). Modern Applied Statistics With S, 4th Edn. New York, NY: Springer. doi: 10.1007/978-0-387-21706-2
Vernes, S., and Wilkinson, G. (in press). Behaviour, biology, evolution of vocal learning in bats. bioRxiv 646703. doi: 10.1101/646703
Voigt, C. C., and von Helversen, O. (1999). Storage and display of odour by male Saccopteryx bilineata (Chiroptera, Emballonuridae). Behav. Ecol. Sociobiol. 47, 29–40. doi: 10.1007/s002650050646
Wickham, H. (2016). ggplot2: Elegant Graphics for Data Analysis. New York, NY: Springer-Verlag. doi: 10.1007/978-3-319-24277-4_9
Wilkinson, G. S. (1984). Reciprocal food sharing in the vampire bat. Nature 308:181. doi: 10.1038/308181a0
Wilkinson, G. S. (1992). Information transfer at evening bat colonies. Anim. Behav. 44, 501–518. doi: 10.1016/0003-3472(92)90059-I
Wilkinson, G. S. (2003). “Social and vocal complexity in bats,” in Animal Social Complexity: Intelligence, Culture, and Individualized Societies (Cambridge, MA:Harvard University Press), 322–341. doi: 10.4159/harvard.9780674419131.c24
Wilkinson, G. S., and Boughman, J. W. (1998). Social calls coordinate foraging in greater spear-nosed bats. Anim. Behav. 55, 337–350. doi: 10.1006/anbe.1997.0557
Willis, C. K., and Brigham, R. M. (2004). Roost switching, roost sharing and social cohesion: forest-dwelling big brown bats, Eptesicus fuscus, conform to the fission–fusion model. Anim. Behav. 68, 495–505. doi: 10.1016/j.anbehav.2003.08.028
Wright, G. S., Chiu, C., Xian, W., Moss, C. F., and Wilkinson, G. S. (2013). Social calls of flying big brown bats (Eptesicus fuscus). Front. Physiol. 4:214. doi: 10.3389/fphys.2013.00214
Keywords: chiroptera, communication, bioacoustics, vespertilionidae, molossidae, social calls
Citation: Springall BT, Li H and Kalcounis-Rueppell MC (2019) The In-Flight Social Calls of Insectivorous Bats: Species Specific Behaviors and Contexts of Social Call Production. Front. Ecol. Evol. 7:441. doi: 10.3389/fevo.2019.00441
Received: 25 June 2019; Accepted: 30 October 2019;
Published: 19 November 2019.
Edited by:
Ann Valerie Hedrick, University of California, Davis, United StatesReviewed by:
Gerald S. Wilkinson, University of Maryland, College Park, United StatesJiang Feng, Northeast Normal University, China
Copyright © 2019 Springall, Li and Kalcounis-Rueppell. This is an open-access article distributed under the terms of the Creative Commons Attribution License (CC BY). The use, distribution or reproduction in other forums is permitted, provided the original author(s) and the copyright owner(s) are credited and that the original publication in this journal is cited, in accordance with accepted academic practice. No use, distribution or reproduction is permitted which does not comply with these terms.
*Correspondence: Brian T. Springall, brian.t.springall@gmail.com