- 1College of Forestry, Oregon State University, Corvallis, OR, United States
- 2School of Forest Resources and Environmental Science, Michigan Technological University, Houghton, MI, United States
- 3Waterton Lakes National Park, Waterton, AB, Canada
- 4Environmental Law Institute, Washington, DC, United States
- 5Kilpatrick Townsend and Stockton, LLP, Washington, DC, United States
- 6Native American Studies, University of Lethbridge, Lethbridge, AB, Canada
Until Euro-American colonization, Indigenous people used fire to modify eco-cultural systems, developing robust Traditional Ecological Knowledge (TEK). Since 1980, wildfire activity has increased due to fire suppression and climate change. In 2017, in Waterton Lakes National Park, AB, the Kenow wildfire burned 19,303 ha, exhibiting extreme fire behavior. It affected forests and the Eskerine Complex, a native-grass prairie treated with prescribed burns since 2006 to reduce aspen (Populus tremuloides) encroachment linked to fire suppression and bison (Bison bison bison) extirpation. One year post-fire, the Kenow wildfire caused vigorous aspen sprouting, altered stand structure to an early-seral state and changed dominant land cover from grass to mineral soil. It did not change aspen-cover extent or cause non-native grass eruption, but it reduced native-grass diversity and produced more pronounced shifts in ecosystem structure and biodiversity than the prescribed burn. The 2017 Kenow wildfire and prescribed burns differed in phenological timing, scale, and severity. Prescribed burns occurred in late spring, with little fuel available, while the Kenow wildfire occurred in late summer, with abundant fuel—amplifying the difference in severity. As in other climate-limited fire regimes, prescribed burns treatments did not mitigate the severity of the Kenow wildfire. To more effectively reduce the extent of aspen cover, future prescribed burns in this system could be applied in the late season. Incorporating TEK in adaptive co-management can help create ecosystems more resilient to fire and pervasive stressors such as invasive plants, provided one contextualizes current conditions and how they differ from historical conditions.
Introduction
Environmental sciences increasingly recognize the interconnectedness among humans and ecosystems as fundamental to understanding the development and sustainability of terrestrial and aquatic systems (Ostrom, 2009). Humans have interacted with North American ecosystems for 24,000 year BP (Bourgeon et al., 2017), with human presence in Western North America for at least 14,000 year BP (Gilbert et al., 2008). Indigenous peoples relied upon ecosystems for survival and increased ecosystem productivity by modifying environmental conditions. Because floral and faunal adaptive capacity maintains resilience to change until a threshold is reached (Holling, 1973; Millar and Stephenson, 2015), species assemblages and food webs shifted in response to these anthropogenic modifications, yet maintained relative similarity and persistence over time (Falk, 2017).
Fire, a primary means by which Indigenous peoples modified eco-cultural systems (Boyd, 1999), frequently occurred in North America prior to Euro-American colonization, promoting ecosystem structure and composition reliant on fire to maintain those conditions (White et al., 2011; Pyne, 2019, p. 40–46). Indigenous fire management had measureable effects (e.g., keeping prairies open by burning woody vegetation; Pollard, 1910; Roos et al., 2018). As Indigenous peoples' populations declined due to disease and colonization, their fire-management impacts on plant communities changed (Liebmann et al., 2016).
Since 1980, North American wildfire activity has increased, linked to fire suppression, climatic variations, and persistent drought (Stephens et al., 2009; Parisien et al., 2011). Recent wildfires have exhibited record size, more extreme fire behavior, and higher severity (Dennison et al., 2014). Today, large wildfires threaten ecosystem function and conservation because of interaction among pervasive stressors such as climate change, invasive species, and land-use, as well as management changes, resulting in no-analog ecosystems and the erosion of ecological memory (Williams and Jackson, 2007; Stephens et al., 2009; Johnstone et al., 2016). This has generated keen interest in restoring natural processes to unravel the negative consequences of contemporary changes on underlying historical eco-cultural relationships and in addressing long-term anthropogenic factors (e.g., climate change and agriculture) across the North American prairies (Van Auken, 2009).
Historically frequent in North American prairies, fire created a spatial mosaic. Native grasslands have been declining in North America since the 1880s, attributable in part to agricultural expansion, fire suppression, bison (Bison bison bison) extirpation, and aspen (Populus tremuloides) encroachment (Campbell et al., 1994; Samson and Knopf, 1994; Simonson and Johnson, 2005). Less than 5% of pre-Euro-American colonization prairie remains (Knapp et al., 1999). Key uncertainties persist regarding the consequences of large contemporary fires, particularly in grasslands where fire and bison herbivory have been eliminated for decades to centuries.
Physical evidence of fire is important to quantify interactions among fire frequency and plant communities. However, while the contribution of natural and human ignition to fire-return intervals may be indistinguishable (Barrett, 1996), the historical effects of wildfire and anthropogenic fire differ, with severe wildfire creating greater changes in plant communities (e.g., reducing woody cover in grasslands) than fires set by Indigenous peoples (Pyne, 2017, p. 39–40). While all fires are complex, the complex effects of Indigenous-set fires on landscapes are poorly understood (Keeley, 2002; Liebmann et al., 2016). For example, indigenous and non-indigenous managers and scientists lack full insight into how or why Indigenous peoples employed fire management throughout North America, such as in California coastal forests and the Southwestern US, because some Traditional Ecological Knowledge (TEK), which is based on oral histories, has been lost due to colonialism, forced relocation, and death of knowledge keepers. In this paper we examine how reinstating historical, traditional land-use processes via ecological restoration can create ecosystems more resilient to pervasive stressors.
TEK provides a bridge to historical land management and its objectives and inherent values. TEK is knowledge and practices passed orally across generations informed by cultural memories, sensitivity to change, and reciprocity (Kimmerer, 2000). TEK land-care processes include modifying vegetation with fire to improve ecosystem productivity and manipulate large-herbivore herds (Zedeño et al., 2014; Roos et al., 2018). TEK practices increase biodiversity and ecological resiliency by creating patchy landscape mosaics (Turner et al., 2000) and offer a web of knowledge that includes values that can help restore ecosystems (Lightfoot et al., 2013). Reinstatement of anthropogenic prescribed burning in habitat types, such as grasslands, where pre-Euro-American colonization Indigenous peoples applied prescribed burning to improve wildlife habitat and food resources for humans, is a form of using TEK as an adaptive management tool to restore ecosystems (Turner et al., 2000; Keeley, 2002).
Since 2008, we have been studying fire and multi-trophic relationships in Waterton Lakes National Park (“Waterton”), Alberta (AB), within the Crown of the Continent Ecosystem, which contains all large mammal species present in 1880 except for wild, free-ranging plains bison. Specifically, we have been examining the effects of prescribed burns on grassland and aspen community structure, elk (Cervus elaphus) browsing, and trophic interactions involving wolves (Canis lupus), the primary elk predator (Eisenberg et al., 2014). A significant amount of intact fescue prairie persists in Waterton, albeit reduced from its historical extent (Levesque, 2005). Mammal dynamics here may have changed with time, including their densities and migratory patterns. However, plant communities have remained similar per palynological data, albeit with encroachment of woody species on grassland (Hills et al., 1985; Flores, 1991; Campbell et al., 1994).
From 1633 to 1900 in the Waterton fescue prairie, time between fires (Mean Fire Interval—MFI) averaged 7 years (Barrett, 1996). This short MFI was historically related to a strong climate/fire linkage augmented by fires set by Indigenous peoples (Krawchuk and Moritz, 2011; Roos et al., 2018). Waterton sought to re-establish this fire regime with early-season prescribed burns, to reduce aspen land cover and restore native prairie (White et al., 2011). In Waterton, early-season burning was implemented to mitigate and reduce the risk created by prescribed burning, which could more easily escape prescribed areas during the late season, when there is more fuel available. In the Eskerine Complex, a 1,377-ha prairie, Waterton has set periodic prescribed burns since 2006 (Figure 1).
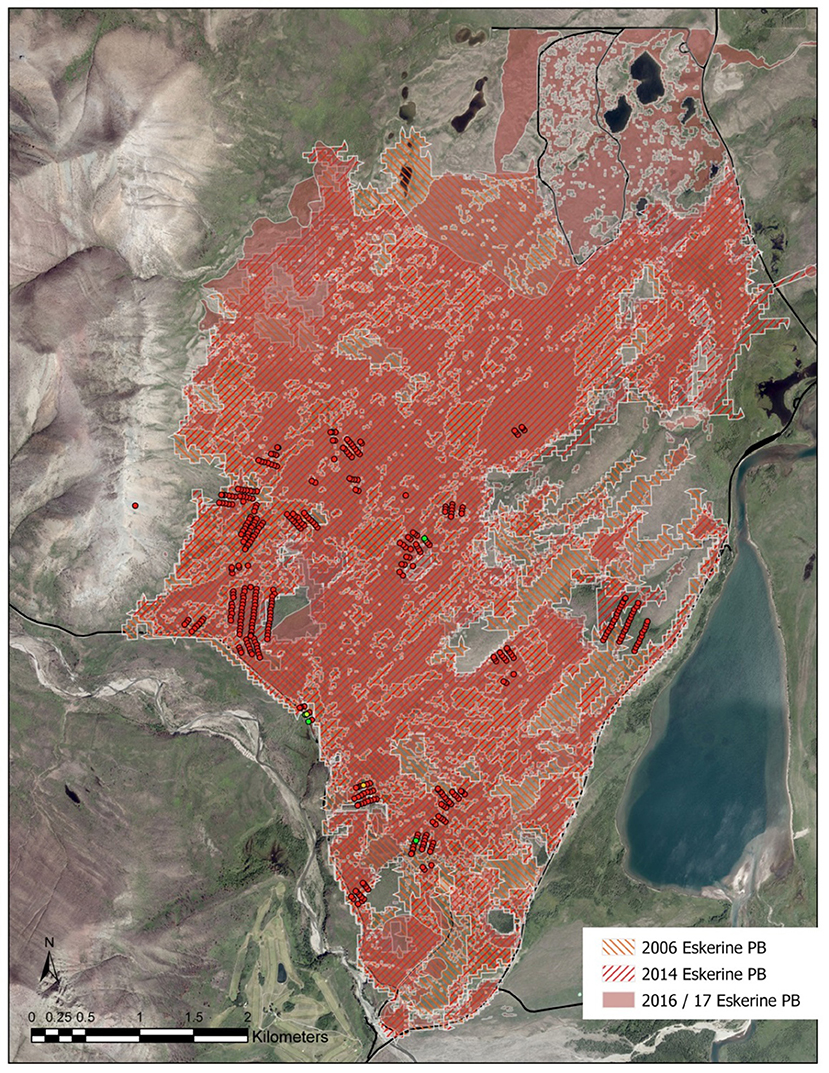
Figure 1. Prescribed fires 2006–2017, Waterton Lakes National Park. Shows the extents of three prescribed burns (PBs) on the Eskerine Complex grassland area of Waterton Lakes National Park, map by Adam Collingwood. Does not include the Kenow wildfire, which covered 100% of the area and is presented in Figure 2.
Reinstating a short MFI has worked to reduce the extent of aspen cover in other systems that are elk winter range, because elk typically browse aspen that sprout after a fire, to the point of killing them. However, wolves (the dominant elk predator in western North America, where present, Eisenberg et al., 2014) were not present in the ecosystem in any of the published studies on this topic (Turner et al., 1994; Romme et al., 1995; Hobbs, 1996; Baker et al., 1997). It is well-documented in ecosystems in Wyoming, Utah, and Colorado that in the absence of a wolf population, elk browse aspen sprouts to death regardless of snow, resulting in little to no recruitment of aspen into the forest canopy (Turner et al., 1994; Romme et al., 1995; Hobbs, 1996; Baker et al., 1997). Moreover, prior studies of aspen treatments do not account for the potential historical impacts of bison in these systems, in which plant communities co-evolved with bison grazing and horning-up of shrubs, or for domesticated horses, which arrived during Euro-American colonization (Flores, 1991; Campbell et al., 1994; Romme et al., 1995; Baker et al., 1997). They further do not account for changes in elk landscape use since 1890, which are not well documented in most places, yet contemporary elk herds use agricultural lands that in some cases, such as Waterton, occur immediately outside of national parks. Additionally, land-use policies in the early 1900s often involved grazing livestock within national parks such as Waterton, with likely impacts on aspen.
Wildfire size and behavior has become a research focal area given the propensity of climate change to increase wildfire threats to human eco-cultural systems. In September 2017, the third-driest summer on record in southwest AB, the lightning-ignited Kenow wildfire burned approximately 35,000 ha, including 19,303 ha within Waterton. Historically, lightning ignitions in the Crown of the Continent Ecosystem created large, stand-replacing fires. These fires naturally have large patches of high-severity fire with 75–100% overstory mortality (Johnson and Wowchuk, 1993). A moist 2017 spring created abundant fuel, and unusually hot, dry, summer conditions and high winds caused a lightning fire to spread rapidly and exhibit extreme fire behavior. On a landscape-scale, North American wildfires, generally patchy and of mixed severity, create heterogeneous plant communities (Amoroso et al., 2011). Regionally, while other fires in Western North America have been larger in size (e.g., Turner et al., 1994), the Kenow wildfire exhibited large, uniform patches of very high severity (Figure 2). What is most astonishing and extreme about this fire is the fact that almost the entire boundary burned with very high severity. That is not common, as wildfires are usually of mixed severity (Turner et al., 1994).
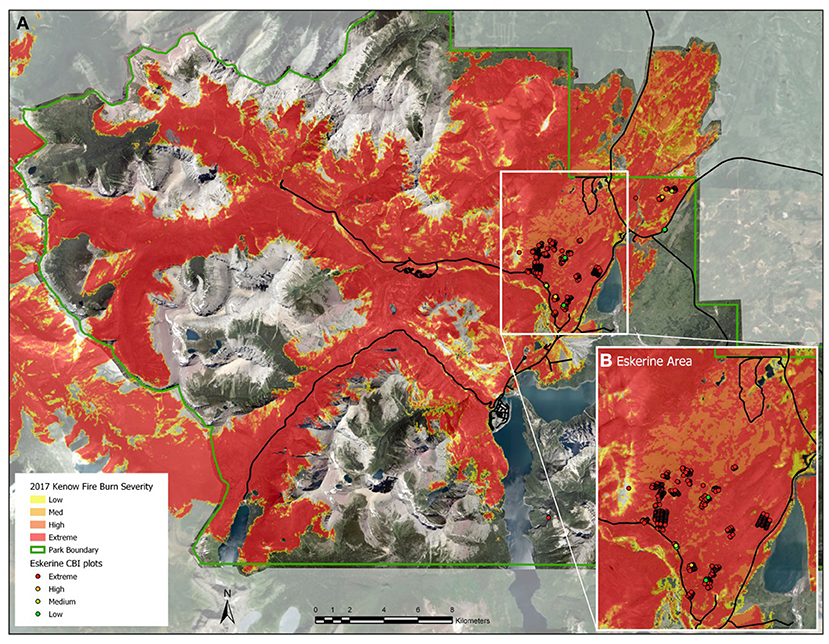
Figure 2. Kenow wildfire fire severity. (A) Fire severity of Waterton Lakes National Park derived from Landsat 8 dNBR values. Threshold dNBR values for Low, Medium, High, and Extreme are 99–270, 271–440, 441–660, and >660, respectively. Inset map shows the Eskerine Complex area, with CBI plot locations; and (B) Fire severity detail for the Eskerine Complex, maps by Adam Collingwood.
The 2017 Kenow wildfire altered our Waterton research landscape (Figure 3A), inviting questions about disturbance processes, plant-community resilience to fire, and integration of TEK into research to better understand fire impacts and adaptively manage ecosystems for resiliency. We wanted to know whether fire is restoring aspen to its historical extent in a system where free-ranging, wild bison are absent, elk are the dominant herbivore, and Indigenous peoples have been removed from some of their traditional lands. We further wanted to know how fire affects fescue prairie graminoids at patch and landscape scales. A key objective of this research was to examine how adaptive management that incorporates TEK can better mitigate stressors such as climate change and invasive species.
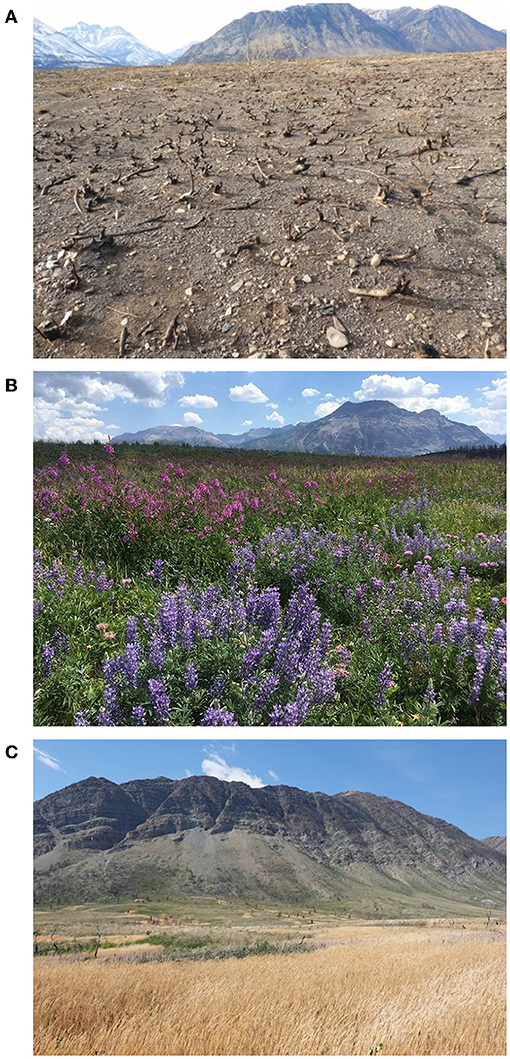
Figure 3. (A–C) Aftermath of the Kenow wildfire, Eskerine Complex Waterton Lakes National Park, Fall 2017/Summer 2018 photographs. (A) Eskerine Complex aspen stand, post Kenow wildfire, fall 2017 (Ryan Peruniak); (B) Eskerine Complex prairie vegetation response, July 2018 (Angela Cook); (C) Calamagrostis response, Eskerine Complex (Cristina Eisenberg).
For reference conditions we used historical photographs of our study area (ca 1890–1910), geospatial data, and TEK shared through oral traditions by the Kainai First Nation, a Blackfoot Confederacy tribe with pre-Euro-American colonization history here (Levesque, 2005; Gann et al., 2019). We did not do a geospatial analysis of historical photographs because that has not been the focus of our aspen and grassland community ecology research. Geospatial analyses of Waterton historical photographs, including those taken of our study site, are currently underway by the Mountain Legacy Project.
Tribal land abuts Waterton, encouraging collaboration and adaptive co-management. Our objectives in this article are to present patterns of plant responses to fire, using insights from 12 years of cumulative research in Waterton, and to examine how adaptive management can mitigate stressors such as invasive species and climate change.
Study Area
Our study site, the Eskerine Complex, lies in the Waterton foothills-parkland ecoregion (fescue prairie that provides elk winter range). This low-elevation (1,200–1,500 m) fescue prairie in good-to-excellent range health contains aspen and shrubs (e.g., Symphoricarpos spp.; Achuff et al., 2005). Pervasive stressors include climate change, drought, elk herbivory, and invasive plant species. In 2017, prior to the Kenow wildfire, dominant native grasses were Idaho fescue (Festuca idahoensis), Parry's oatgrass (Danthonia parryi), rough fescue (F. campestris), and Columbia needlegrass (Achnatherum nelsonii). Non-native, invasive grasses were Kentucky bluegrass (Poa pratensis), Timothy grass (Phleum pratense), smooth brome (Bromus inermis), and sheep fescue (F. ovina).
Elk have a density of up to 24 per km−2 in Waterton, numbering 1,000 individuals in 2017. Elk primarily eat grass, although they can shift their winter diet to woody species (Cook, 2002). They can have destructive browse impacts on aspen, but this has not occurred in Waterton. Bison, a migratory, primarily grass-eating species, historically used the foothills-parkland ecoregion, altering the biophysical environment by damaging woody species with their horns, keeping the prairie open. Their nitrogen-rich urine and feces increased grass resiliency (Knapp et al., 1999; Gates et al., 2010). Waterton maintained a captive bison herd from 1952 to 2017: 7–30 animals in the 124-ha Summer Bison Paddock (SBP) and the 56-ha Winter Bison Paddock (WBP) (Johnston, 2008). Captive bison densities were very different from historic bison densities. While no reliable report of historic bison densities exists, oral histories and early explorer accounts state bison migrated through this area at high densities for short periods. For example, on July 27, 1806, Lewis and Clark reported when they encountered the Blackfoot on Two Medicine Creek near Waterton that they “continued to pass immence herds of buffa loe (sic) from the late afternoon through all of the following night” (Moulton, 1987, p. 136). Between 2008 and 2018, the single wolf pack here ranged from 2 to 12 individuals and was the primary elk predator (Eisenberg et al., 2014, Johnston, personal communication).
In a microhistological study we conducted in 2017, pre-Kenow wildfire, we found elk and bison diet similarities. Elk diet during January 2017–April 2017 consisted of 3.9–9.3% woody material. Elk only ate aspen in February 2017 (1.4%) and April 2017 (0.5%). Bison ate 0% aspen, and 0% woody species. Elk mainly ate grass (81.8–87.7%), as did bison (85.3–93.5%). Rough fescue was the top grass eaten by elk (21.8–28.6%) and bison (21.5–34.2%) (Eisenberg et al., unpublished data).
Archeological data provide evidence of Indigenous presence in southwest AB for more than 10,000 years BP. These Indigenous peoples were ancestors of today's Blackfoot, Cree, and Kootenai peoples. Per archeological evidence, the Indigenous peoples who inhabited this region prior to Euro-American colonization subsisted on bison as one of their primary food sources and traveled from the prairies to the foothills to hunt bison and forage for food (Malainey and Sherriff, 1996; Langemann and Perry, 2007; Reeves, 2007; Roos et al., 2018).
Tree-ring studies, pollen-core data, and charcoal layers furnish evidence of fire in the Crown of the Continent Ecosystem across millennia (Hills et al., 1985; Reeves, 2007), revealing successional shifts in plant communities caused by climate change, fire, and human activity. Pollen cores (ca 18,400 BP) extracted from a corridor ice-free during the Wisconsinan glaciation near Calgary, AB, show a grassland similar to what covers Waterton today (Mott and Jackson, 1982), containing Graminea, herbs, including Chenopodium spp., and shrubs. In the Eskerine Complex, the previous wildfire occurred in 1910 (Barrett, 1996). A culture of fire exclusion followed until Waterton set prescribed burns in spring 2006, 2014, and 2017, informed by historical fire regimes, to restore the Eskerine Complex (Figure 1).
Materials and Methods
In 2008, we began sampling Eskerine Complex aspen stands (n = 30). Through each stand we randomly positioned 4-m-wide, variable-length transects (marked with 1-m rebar stakes with 0.20 m exposed above the soil surface) that ran from the edge of the aspen stand (indicated by the first aspen sprout) to the last aspen sprout along the transect line. In each transect we placed 2-m-radius aspen plots every 20 m running from the transect start, with a plot forced to the end of each transect (n = 405). In some of our analyses (e.g., aspen sprout density), we used as a baseline the 2008 aspen transect and plot data for aspen stands that were not burned in the 2006 prescribed burn, given that these data reflect lack of prescribed burning and a 98-year interval since the previous wildfire. Because the 2006 prescribed burn occurred 3 growing seasons before our study began, we lacked relevant detail of this prescribed burn post-fire. There were few aspen stands within the bison paddocks. We chose to not sample them due to the small sample size they presented and the confounding treatment factor of bison trampling, inhibiting a robust comparison to aspen stands outside the bison paddock.
In the prairie, we randomly sampled 171 2-m-radius prairie plots dominated by grass cover ≥20 m from aspen. We stratified the Eskerine Complex into three zones (Table 1), the unfenced Eskerine Complex (Eskerine prairie) and two fenced areas: the Summer Bison Paddock (SBP prairie) and Winter Bison Paddock (WBP prairie). We refer to grass-dominated plots in the three zones collectively as “Eskerine prairie grass plots.” In these plots, we measured proportion of cover by grass (basal), shrubs (basal), and bare mineral soil. We also identified grass species and categorized them as native and non-native species. We measured post-fire ecological response in prairie plots in the late growing season.
The field data we collected in both aspen stand plots and prairie grass plots were used to assess fire impacts 1-year post-Kenow wildfire on Eskerine Complex composition and aspen stand extent and structure in late spring 2018, before plant phenology could create detection bias of mineral soil. Because of the patchiness and low severity overall of the 2006 prescribed burn, we used 2006 data from unburned aspen stands only, focusing most of our analysis on 2014 and 2017 prescribed burns. In 2017 we added the Kenow wildfire to our study, to examine how wildfire effects compare to prescribed burns. We did not do a comparison of wildfire vs. prescribed fire because aspen ecological response to fire (e.g., sprout basal area, stem mortality) is best measured 2 years post-fire (Romme et al., 1995), and this paper presents our findings from 1-year post-Kenow wildfire.
Fire severity is the degree to which a site has been altered or disrupted by fire. It is a product of fire intensity (energy output from fire) and residence time, combined with site-specific biotic and abiotic conditions (Lentile et al., 2006; Keeley, 2009). Fire severity includes aboveground and belowground organic-matter consumption by fire, although in temperate ecosystems, fire sometimes does not lead to significant effects on soil organic matter (Twidwell et al., 2012; Jian et al., 2018). We assessed Kenow wildfire severity with satellite-based and field-based metrics. For the satellite-based metric, we used the Differenced Normalized Burn Ratio (dNBR), which detects change between pre-and post-fire remotely sensed data (shortwave-infrared and near-infrared portions of the spectrum). Initial dNBR threshold values of 99–270, 271–440, 441–660, and >600 were used to delineate low, moderate, high, and extreme severity, respectively, based on the Composite Burn Index (CBI) (Key and Benson, 2006; Morgan et al., 2014). The CBI is a field-based visual assessment of fuel consumed, soil charring, and vegetation rejuvenation, applied in 30 × 30 m plots, working with five strata (soil, understory vegetation, mid-canopy, overstory, and dominant overstory vegetation), evaluated individually and then combined for an overall plot-level severity value.
For our field-based metric, we derived a fire severity index (FSI) from the CBI, eliminating ecological response covariates (Table 2) to avoid conflating fire effects and ecological responses (Lentile et al., 2006; Keeley, 2009; Morgan et al., 2014). We used a 4-category system because the 3-category system did not capture nuances at the high end of the severity spectrum that could have significant ecological effects later, and applied it to aspen stands only. We define extreme fire severity as a condition resulting in >75% of all of the following indicators: tree crown scorch, crown torch, mortality, non-tree vegetation mortality, litter consumption, exposed mineral soil (Table 2). The initial dNBR severity thresholds (defined above) correctly classified all Eskerine Complex plots into their respective FSI categories, so no further changes were made to the dNBR classes.
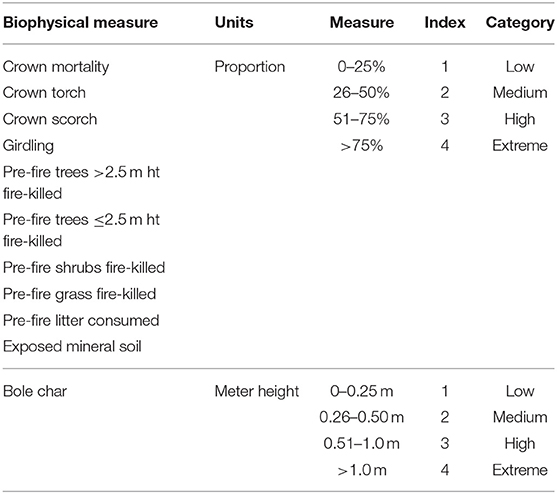
Table 2. Fire Severity Index (FSI) metrics, based on the Composite Burn Index (CBI), with an added category of “extreme” to enable us to capture nuances at the high end of the severity spectrum that could have significant ecological effects.
We mapped Eskerine Complex aspen stands with mapping-grade Trimble GPS receivers. We collected stratified vegetation boundaries in 2016 and 2018 and calculated the area in ArcMap (10.5). Data included cover in ha of total aspen (mature aspen stems ≥2.5 m-ht + all aspen sprout stems <2.5 m-ht); canopy (mature aspen stems ≥2.5 m-ht); regeneration (aspen sprout stems <2.5 m-ht); and shrub avulsion (shrubs that extend continuously outward from an aspen stand), to detect area-over-time vegetation changes in response to prescribed burns. This enabled high-resolution examination of aspen-community composition (Anderson, 2019). Analyses focused on assessing aspen and prairie-plot changes 1-year post-fire, because aspen ecological response to fire takes years to develop (Romme et al., 2011). We tested for deviation from assumptions of normality by observing residual plots.
Results
In all of Waterton, per our dNBR analysis, in forested areas, the Kenow wildfire burned with 75.4% extreme, 12.6% high, 6.4% moderate, and 5.6% low fire severities (Figures 2A,B). Within the Eskerine Complex only, we observed an average field-based FSI (on a scale of 0–4) of 3.91 ± 0.03, which falls into our extreme category. 97% of Eskerine aspen plots burned with extreme severity. The extreme FSI Eskerine plot burns matched the severity of fire in 75% of the Kenow fire. The proportion of different severity classes (field-data based and satellite data based) matched. Our rebar transect markers indicate that the Kenow wildfire and post-fire wind removed up to 0.70 m of topsoil from some rebar stakes through combustion or wind dispersal, although these visual observations indicated that soil removal patterns were very patchy. Post-Kenow wildfire soil removal revealed many bison bones and some artifacts such as arrowheads.
In Eskerine Complex prairie grass plots, proportion of grass cover dropped significantly from 2016 (t = −35.42, 338 df, p ≤ 0.00001)—a shift in dominant cover type (Figure 4). Post-2014 and 2017 prescribed burns, we detected no mineral soil in prairie grass plots. In 2018, proportion of mineral soil in prairie grass plots was 50.84 ± 1.42%, and became the dominant cover type (Figure 4). Between 2016 and 2018, proportion of shrub cover was unchanged, due to re-sprouting.
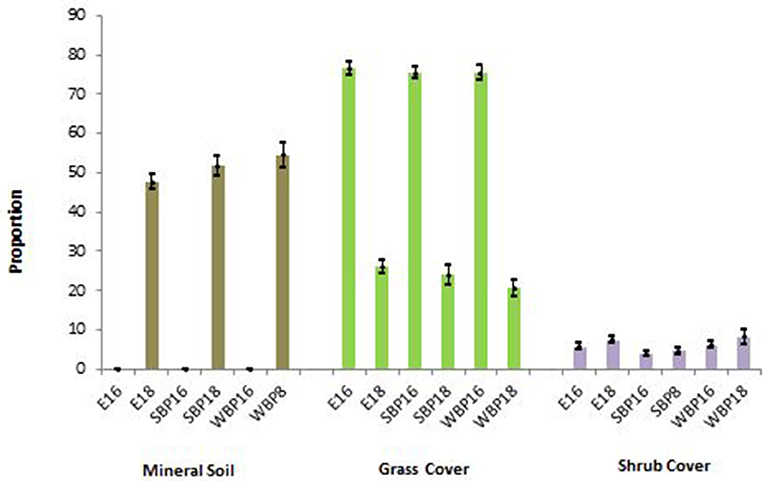
Figure 4. Change in cover proportion by type and site on the Eskerine Complex prairie in Waterton Lakes National Park. E, Eskerine prairie plots; SBP, Summer Bison Paddock prairie plots; WBP, Winter Bison Paddock prairie plots; 18, the year 2018; 16, the year 2016. These sites are all located within the Eskerine Complex prairie.
Dominant native and non-native grasses in 2016 and 2018 were the same (see Study Area section), except for sheep fescue. Frequency of native-species detection (presence in plot) by year was similar, except for Columbia needlegrass (increased from 30 to 149 detections) and Parry's oatgrass (decrease from 164 to 111 detections). Three of four non-native species decreased: Timothy grass from 25 to 18 detections; smooth brome from 15 to 4 detections; and sheep fescue from 9 to 0 detections. Reedgrass (Calamagrostis spp.) and lamb's quarters (Chenopodium album), previously seldom detected here, became common in aspen stands, seed-sprouting from mineral soil (Figure 3C).
Proportion of native grass cover in our plots was equivalent between 2016 and 2018. In 2016, Eskerine prairie plots had a higher proportion of native grass cover, 95.56 ± 1.12%, than SBP prairie plots, 83.76 ± 3.59% (t = 3.63, 118 df, p = 0.0004), and WBP prairie plots, 85.92 ± 3.70% (t = 2.87, 119 df, p = 0.005), attributable to 1960s−1970s inadvertent introduction of non-native agronomic species. In 2018, Eskerine prairie plots had a higher proportion of native grass, 90.08 ± 2.58%, than SBP prairie plots, 79.44 ± 4.51% (t = 2.19, 119 df, p = 0.03).
Between 2016 and 2018, mean number of native-grass species decreased (Appendix Table 1) significantly as follows: Eskerine prairie t = −3.77, 140 df, p = 0.002; SBP prairie t = −2.38, 98 df, p = 0.02; WBP prairie t = −4.92, 98 df, p ≤ 0.00001. In 2016, the mean number of native grass species was lower in the Eskerine prairie than the SBP prairie (t = −2.64, 119 df, p = 0.009) and WBP prairie (t = −3.57, 119 df, p = 0.0005). In 2018, the Eskerine prairie had fewer native-grass species than the SBP prairie (t = −2.71, 119 df, p = 0.01), but no difference existed between Eskerine and WBP prairie plots.
When we evaluated the influence of the number of prescribed burns (0–3 prescribed burns, Figure 1) on grass-plot ecological response to the Kenow wildfire (Appendix Table 2), the proportion of native grass was higher in plots that had 2 prescribed burns, vs. 0 prescribed burns (t = −2.19, 70 df, p = 0.03; Appendix Table 2).
In mapped aspen stands, the only significant change between 2016 and 2018 was the canopy area per stand, which decreased from 1.92 ± 0.88 ha to 0.03 ± 0.02 ha (t = 2.13, 58 df, p = 0.037), bringing these stands to an early-seral state (Figure 5).
Within Eskerine Complex aspen stands, sprout density was 621.26 ± 586.94 ha−1 in 2008; 16,120.07 ± 1057.55 ha−1 in 2016, and 32,324.48 ± 2755.87 ha−1 in 2018. The highest density measured in 2018 was 481,443.88 sprouts ha−1. Difference in density was significant between 2008 and 2015 (t = 5.08, 735 df, p ≤ 0.00001); 2015 and 2018 (t = −3.92, 783 df, p ≤ 0.00001); and 2008 and 2018 (t = 6.26, 734 df, p ≤ 0.00001). For all years, aspen sprout density was patchy, with no evidence of sprouting from seed.
Discussion
Aldo Leopold famously wrote, “The last word in ignorance is the man who says of an animal or plant, ‘What good is it?’ If the land mechanism as a whole is good, then every part is good, whether we understand it or not. If the biota, in the course of eons, has built something we like but do not understand, then who but a fool would discard seemingly useless parts? To keep every cog and wheel is the first precaution of intelligent tinkering” (Leopold, 1986, p. 190). This statement is critical to conserve ecosystems and manage them for restoration and resilience.
Bison were long part of Waterton, and humans hunted them. An entry from Peter Fiddler's journal (December 28, 1792) describes a hunt in Shepard Creek (near what is today Waterton), while a grass fire burned nearby (Haig, 1990). By 1880, bison were mostly extirpated from North America (Flores, 1991). Today the Buffalo Treaty, Iinni (means “bison” in Blackfoot) Initiative, and reintroduction efforts (Banff National Park, 2017, pending Badger-Two Medicine, Montana, and Band Ranch, Kainai Reserve, AB) are advancing bison recovery (Little Bear, 2014).
However, Aldo Leopold's statement did not acknowledge relationships inherent in eco-cultural systems such as the Eskerine Complex. Waterton contains many archeological sites, including buffalo-drivelines and pounds—features spatially related to prairie fire, used for communal hunting, including a buffalo jump on the SW Eskerine Complex edge (Langemann and Perry, 2007; Reeves, 2007). As part of adaptive co-management, managers strive to incorporate ecological and eco-cultural factors in physically restoring landscapes and increasing landscape resilience to pervasive stressors. Ecological prescriptions that focus on Western Science paradigms are well-established in North America. Re-establishing historical eco-cultural dynamics here by relying on TEK primarily transferred via oral histories, is less common in adaptive management plans (Armatas et al., 2016). Restoring TEK may be more challenging than restoring this system's physical components. Yet, relationships among the Kainai, the Eskerine Complex, and the broader Crown of the Continent Ecosystem arguably retain more accessible TEK than other systems with similar post-colonization eco-cultural change, from which TEK has been lost due to colonial removal of Indigenous people.
Our data suggest that while TEK land-use patterns, such as anthropogenic burning, can be replicated by managers with the objective of diminishing woody encroachment on grasslands, the results may not be effective without incorporating some of the other ecological factors present historically, such as bison in Waterton. While plains bison primarily eat grass, they suppress aspen and other woody species with their horns. The bones and artifacts the Kenow wildfire revealed indicate that bison and human hunters were once part of this system (Hills et al., 1985; Roos et al., 2018). These relationships had trophic impacts on human wellbeing and survival then, as now. The important role of people tending ecosystems with frequent fire has been recognized (Reyes-García et al., 2018), but Indigenous-set fire was also used in a system that had free-ranging bison. This seems especially important in the Eskerine, where without anthropogenic burning and bison grazing, aspen might continue to expand in the short term (e.g., <100 year), although this expansion may have edaphic limitations (Campbell et al., 1994).
On a longer temporal scale, the climate in AB is projected to continue to become hotter and drier, which could cause aspen to decline (e.g., ≥ 100 year; Gray et al., 2011). Regardless of which temporal lens one uses to look at natural resources and ecological restoration, the same stewardship principles—and contextual limitations of TEK—might apply to other frequent-fire ecosystems globally. In ecological restoration practices, TEK applications may need to be adapted to account for current conditions (e.g., lack of free-ranging bison; Gann et al., 2019).
The long-term perspectives afforded by this research project, in addition to the unexpected impacts of the Kenow wildfire (e.g., stability of proportion of native grasses in our plots, decrease of detection frequency of non-native grasses, proliferation of Calamgrostis spp.), illustrate an important concept in disturbance ecology. Specifically, fires in the Eskerine Complex are not individual events easily bounded in space and time. Rather, multiple fires overlap, and their effects represent the synergy among fire frequency and magnitude, ecological productivity, and adaptive traits that confer resilience to disturbance. These multiple fires can lead to achieving Waterton's goal of restoring grassland community composition. Conceptualizing disturbance as a synergistic process builds on the classic definition of disturbance as a relatively discrete event (Pickett and White, 1985). Because fire promotes persistence of fire-adapted species at the expense of others, recurring fire derived from human ignitions aims to push the system toward a desired condition that benefits both social and ecological components. Temporally dispersed events, such as 1910 and 2017 wildfires, are too infrequent to induce a process-based response fully indicative of historical dynamics, highlighting the importance of continuing prescribed burns, and perhaps applying them during the late season.
Prescribed burn objectives (diminishing area of aspen cover on the prairie) were not fully realized after 3 prescribed burns over 11 years, likely because low-severity early-season prescribed burns were applied, rather than late-season higher-severity fires. Understanding fire as a “process” in which historical reductions in woody plant cover resulted from extreme late-season wildfires set by Indigenous peoples per TEK practices (to hunt bison in the fall, so that the meat wouldn't spoil, and they would have food for winter), rather than low–severity, early-season human-set fires, requires managing near-term expectations as we bring ecosystems slowly back into alignment with historical relationships that persisted longer than the 100+ years of fire exclusion (Falk et al., 2011). A tangible benefit of prescribed burns here was that they did not increase the proportion of non-native grass on the prairie, which decreased post-Kenow wildfire.
Given its extreme behavior, can the Kenow wildfire be described as “catastrophic?” Not from a plant-community perspective (Falk, 2017). Our data illustrate this plant community's strong resiliency to extreme wildfire (Figures 3A–C). We do not consider these responses a state shift (transition from one ecological state to another; Stringham et al., 2003). With the exception of proportion of bare mineral soil, this landscape's fundamental vegetation cover characteristics remained unchanged, indicating ecological stability (Suding et al., 2004). This suggests that because patches of higher-severity fire were part of the foothills-parkland historical fire regime (Roos et al., 2018), prescribed burns can help maintain native plant communities adapted to the full range of fire effects. It further indicates that prescribed burns combined with wildfire can help maintain native grasses, particularly in landscapes prone to extreme fire, and that high-severity burns are necessary for effective grassland restoration.
Conclusion
The 2017 Kenow wildfire and prescribed burns differed in severity, scale, and phenological timing. The Kenow wildfire significantly shifted ecosystem structure and grass diversity, while prescribed burns removed little aspen canopy and exposed no mineral soil. Prescribed burns occurred in late spring, with little fuel available, while the Kenow wildfire occurred in late summer, with abundant fuel—amplifying this difference (Knapp et al., 2009). As in other climate-limited fire regimes, prescribed burns treatments did not mitigate the severity of the Kenow wildfire (Stephens et al., 2009). Elsewhere, thinning treatments combined with prescribed burn treatments have been more effective than prescribed burning alone at mitigating subsequent wildfire severity (Stephens et al., 2009).
Several themes emerged from the findings we have presented above. First, ecosystems are resilient, and it is unwise to assume that seemingly extreme disturbance is an ecological catastrophe. Second, as large disturbances become more common, restoring historical ecological processes including both gradual and punctuated changes will help stakeholders plan for future change. Third, ecological plant legacies can help restore systems that appear to be degraded. Fourth, collaboration across cultures and disciplines, which includes TEK, can help create more resilient ecosystems. And finally, the full historical context of TEK and traditional practices must be considered, realizing that TEK applications may need to be adapted to account for current conditions.
Data Availability Statement
The datasets generated for this study are available on request to the corresponding author.
Author Contributions
CEi conceived of the idea for the paper, wrote the manuscript, and did the statistical analysis. CA wrote the manuscript, did mapping, and statistical analysis. AC did geospatial analysis, mapping, and writing. CD, GM, DH, RS, and SM contributed to the framing of the concepts presented and to the writing. SK, JS-M, and LL contributed to the TEK concepts and the writing. BJ and CEd contributed to the writing.
Funding
This work was supported by Parks Canada, Earthwatch Institute, the Kainai Board of Education, the AGL Foundation, and the Davin Family.
Conflict of Interest
SK was employed by the Environmental Law Institute. JS-M was employed by the company Kilpatrick Townsend and Stockton, LLP.
The remaining authors declare that the research was conducted in the absence of any commercial or financial relationships that could be construed as a potential conflict of interest.
Acknowledgments
We thank Earthwatch volunteers and Kainai Community Fellows for field assistance. We are grateful to Wayne Padgett, Steve Wirt, Michael McLellan, Rob Watt, Mike Bruised Head, Kansie Fox, Elliot Fox, and the late Narcisse Blood, John Russell, and Paul Doescher for advice and guidance. Thanks to Walt Anderson for a friendly editorial review of this manuscript.
Supplementary Material
The Supplementary Material for this article can be found online at: https://www.frontiersin.org/articles/10.3389/fevo.2019.00436/full#supplementary-material
References
Achuff, P. L., McNeil, R. L., Coleman, M. L., Wallis, C., and Wershler, C. (2005). Ecological Land Classification of Waterton Lakes National Park, AB. Ottawa, ON: Parks Canada.
Amoroso, M. M., Daniels, L. D., Bataineh, M., and Andison, D. W. (2011). Evidence of mixed-severity fires in the foothills of the Rocky Mountains of west-central Alberta, Canada. For. Ecol. Manage. 262, 2240–2249. doi: 10.1016/j.foreco.2011.08.016
Anderson, C. (2019). Examining aspen expansion from before and after prescribed burning in a native fescue grassland through geospatial techniques (a master's thesis). Houghton, MI: Michigan Technological University.
Armatas, C. A., Venn, T. J., McBride, B. B., Watson, A. E., and Carver, S. J. (2016). Opportunities to utilize traditional phenological knowledge to support adaptive management of social-ecological systems vulnerable to changes in climate and fire regimes. Ecol. Soc. 21:16. doi: 10.5751/ES-07905-210116
Baker, W. L., Munroe, J. A., and Hessl, A. E. (1997). The effects of elk on aspen in the winter range in Rocky Mountain National Park. Ecography 20, 155–165. doi: 10.1111/j.1600-0587.1997.tb00358.x
Barrett, S. W. (1996). The Historic Role of Fire in Waterton Lakes National Park, Alberta. Waterton, AB: Parks Canada Technical Report.
Bourgeon, L., Burke, A., and Higham, T. (2017). Earliest human presence in North America dated to the last glacial maximum: new radiocarbon dates from Bluefish Caves, Canada. PLoS ONE 12:e0169486. doi: 10.1371/journal.pone.0169486
Boyd, R. T. (ed.). (1999). Indians, Fire, and the Land in the Pacific Northwest. Corvallis, OR: Oregon State University Press.
Campbell, C., Campbell, I. D., Blyth, C. B., and McAndrews, J. H., et al. (1994). Bison extirpation may have caused aspen expansion in western Canada. Ecography 19, 360–362. doi: 10.1111/j.1600-0587.1994.tb00113.x
Cook, J. G. (2002). “Nutrition and food,” in North American Elk: Ecology and Management, eds D. Toweill and J. W. Thomas (Washington, DC: Smithsonian Institution Press), 344–345.
Dennison, P. E., Brewer, S. C., Arnold, J. D., and Moritz, M. A. (2014). Large wildfire trends in the western United States, 1984–2011. Geophys. Res. Lett. 41, 2928–2933. doi: 10.1002/2014GL059576
Eisenberg, C., Hibbs, D. E., Ripple, W. J., and Salwasser, H. (2014). Context dependence of elk vigilance and wolf predation risk. Can. J. Zool. 92, 727–736. doi: 10.1139/cjz-2014-0049
Falk, D. A. (2017). Restoration ecology, resilience, and the axes of change. Ann. Missouri Bot. Gar. 102, 201–216. doi: 10.3417/2017006
Falk, D. A., Heyerdahl, E. K., Brown, P. M., Farris, C., Ful,é, P. Z., McKenzie, D., et al. (2011). Multi-scale controls of historical forest-fire regimes: new insights from fire-scar networks. Front. Ecol. Environ. 9, 446–454. doi: 10.1890/100052
Flores, D. (1991). Bison ecology and bison diplomacy: the southern plains from 1800 to 1850. J. Amer. Hist. 78, 465–485. doi: 10.2307/2079530
Gann, G., McDonald, T., Walder, B., Aronson, J., Nelson, C. R., Eisenberg, C., et al. (2019). International principles and standards for the practice of ecological restoration. Restor. Ecol. 27, S3–S46. doi: 10.1111/rec.13035
Gates, C. C., Freese, C. H., Gogan, J. P., and Kotzman, M. (2010). American Bison: Status Survey and Conservation Guidelines 2010. Gland: International Union for the Conservation of Nature.
Gilbert, M. T., Jenkins, D. L., Götherstrom, A., Naveran, N., Sanchez, J. J., Hofreiter, M., et al. (2008). DNA from pre-Clovis human coprolites in Oregon, North America. Science 320, 786–789. doi: 10.1126/science.1154116
Gray, L. K., Gylander, T., Mbogga, M. S., Chen, P. Y., and Hamann, A. (2011). Assisted migration to address climate change: recommendations for aspen reforestation in western Can. Ecol. Appl. 21, 1591–1603. doi: 10.1890/10-1054.1
Haig B. (ed.). (1990). Journal of a Journey Over Land from Buckingham House to the Rocky Mountains in 1792: A Look at Peter Fidler's Journal. Lethbridge, AB: Historical Research Centre.
Hills, L. V., Christensen, O. A., Fergusson, A., Driver, J. C., and Reeves, B. O. K. (1985). Postglacial pollen and paleoclimate in southwestern Alberta and southeastern British Columbia. Syllogeus 55, 344–396.
Hobbs, N. T. (1996). Modification of ecosystems by ungulates. J. Wild. Manage. 60, 695–713. doi: 10.2307/3802368
Holling, C. S. (1973). Resilience and stability of ecological systems. Annu. Rev. Ecol. Syst. 4, 1–23. doi: 10.1146/annurev.es.04.110173.000245
Jian, M., Berli, M., and Ghezzehei, T. A. (2018). Soil structural degradation during low-severity burns. Geophys. Res. Lett. 45, 5553–5561. doi: 10.1029/2018GL078053
Johnson, E. A., and Wowchuk, D. R. (1993). Wildfires in the southern Canadian Rocky Mountains and their relationship to mid-tropospheric anomalies. Can. J. For. Res. 23, 1213–1222. doi: 10.1139/x93-153
Johnston, B. (2008). Assessing the Feasibility of Establishing Semi Free-Ranging Bison in Waterton Lakes National Park. Waterton Park, AB: Parks Canada.
Johnstone, J. F., Allen, C. D., Franklin, J. F., Frelich, L. E., Harvey, B. J., Higuera, P. E., et al. (2016). Changing disturbance regimes, ecological memory, and forest resilience. Front. Ecol. Environ. 14, 369–378. doi: 10.1002/fee.1311
Keeley, J. E. (2002). Native American impacts on fire regimes of the California coastal ranges. J. Biogeog. 29, 303–320. doi: 10.1046/j.1365-2699.2002.00676.x
Keeley, J. E. (2009). Fire intensity, fire severity, and burn severity: a brief review and suggested usage. Int. J. Wildland Fire 18, 116–126. doi: 10.1071/WF07049
Key, C. H., and Benson, N. C. (2006). “Landscape assessment: sampling and analysis methods,” in FIREMON: Fire Effects Monitoring and Inventory System, USDA Rocky Mountain Research Station, General Technical Report 164, eds D. C. Lutes, R. E. Keane, J. F. Caratti, C. H. Key, N. C. Benson, S. Sutherland, et al. (Fort Collins, CO: USDA Forest Service), LA1–LA51.
Kimmerer, R. W. (2000). Native knowledge for native ecosystems. J. Forest. 98, 4–9. doi: 10.1093/jof/98.8.4
Knapp, A. K., Blair, J. M., Briggs, D. C., Collins, S. L., Hartnett, D. C., Jonson, L. C., et al. (1999). The keystone role of bison in North American tallgrass prairie. BioScience 49, 39–50. doi: 10.2307/1313492
Knapp, E. E., Estes, B. L., and Skinner, C. N. (2009). Ecological Effects of Prescribed Fire Season: A Literature Review and Synthesis for Managers, PSW-GTR-224. Redding, CA: USDA Forest Service; Pacific Southwest Research Station.
Krawchuk, M. A., and Moritz, M. A. (2011). Constraints on global fire activity vary across a resource gradient. Ecology 92, 121–132. doi: 10.1890/09-1843.1
Langemann, G., and Perry, W. (2007). Waterton Lakes National Park Archaeological Research Programme, 1997-2006. Calgary, AB: Cultural Resource Services; Western and Northern Service Centre Parks Canada.
Lentile, L. B., Holden,. Z. A., Smith, A. M., Falkowski, M. J., Hudak, A. T., Morgan, P., et al. (2006). Remote sensing techniques to assess active fire characteristics and post-fire effects. Int. J. Wildland Fire 15, 319–345. doi: 10.1071/WF05097
Leopold, A. (1986). A Sand County Almanac: And Sketches Here and There. New York, NY: Ballantine Books.
Levesque, L. (2005). Investigating Landscape Change and Ecological Restoration: An Integrated Approach Using Historical Ecology and Geographical Information Systems in Waterton Lakes National Park, Alberta (a master's thesis). Victoria, BC: University of Victoria.
Liebmann, M. J., Farella, J., Roos, C. I., Stack, A., Martini, S., and Swetnam, T. W. (2016). Native American depopulation, reforestation, and fire regimes in the Southwest United States, 1492–1900 CE. Proc. Natl. Acad. Sci. U.S.A. 113, E696–E704. doi: 10.1073/pnas.1521744113
Lightfoot, K. G., Cuthrell, R. Q., Striplen, C. J., and Hylkema, M. G. (2013). Rethinking the study of landscape management practices among hunter-gatherers in North America. Am. Antiquity 78, 285–301. doi: 10.7183/0002-7316.78.2.285
Little Bear, L. (2014). The Buffalo: A Treaty of Co-operation, Renewal, and Restoration. Banff, AB: American Bison Society. Available online at: http://www.ambisonsociety.org/Buffalo-Treaty-2nd-Anniversary.aspx (accessed September 29, 2018).
Malainey, M. E., and Sherriff, B. L. (1996). Adjusting our perceptions: historical and archaeological evidence of winter on the plains of western Canada. Plains Anthropol. 41, 333–357. doi: 10.1080/2052546.1996.11931810
Millar, C. I., and Stephenson, N. L. (2015). Temperate forest health in an era of emergingmegadisturbance. Science 349, 823–826. doi: 10.1126/science.aaa9933
Morgan, P. R., Keane, R. E., Dillon, G. K., Jain, T. B., Hudak, A. T., Karau, E. C., et al. (2014). Challenges of assessing fire and burn severity using field measures, remote sensing, and modeling. Int. J. Wildland Fire 23, 1045–1060. doi: 10.1071/WF13058
Mott, R. J., and Jackson, L. E. Jr. (1982). An 18,000 year palynological record from the southern Alberta segment of the classical Wisconsinan “Ice-free Corridor.” Can. J. Earth Sci. 19, 504–513. doi: 10.1139/e82-041
Moulton, G. E. (1987). The Journals of the Lewis and Clark Expedition, Vol. 8. Lincoln, NE: University of Nebraska Press.
Ostrom, E. (2009). A general framework for analyzing sustainability of social-ecological systems. Science 325, 419–422. doi: 10.1126/science.1172133
Parisien, M. A., Parks, S. A., Krawchuk, M. A., Flannigan, M. D., Bowman, L. M., and Moritz, M. A. (2011). Scale-dependent controls on the area burned in the boreal forest of Canada, 1980 2005. Ecol. Appl. 21,789–805. doi: 10.1890/10-0326.1
Pickett, S. T. A., and White, P. S. (eds.). (1985). The Ecology of Natural Disturbance and Patch Dynamics. Orlando, FL: Academic Press.
Pollard, H. (1910). Starting Prairie Fire. Available online at: https://hermis.alberta.ca/paa/PhotoGalleryDetails.aspx?CollectionID=2&st=P138&ReturnUrl=2fpaa%2fSearch.aspx%3fCollectionID%3d2%26st%3dP138&dv=True&DeptID=1&ObjectID=P138 (accessed October 10, 2018).
Pyne, S. J. (2017). Fire in America: A Cultural History of Wildland and Rural Fire. Seatte, WA: University of Washington Press.
Reeves, B. O. K. (2007). “Native peoples and archaeology of Waterton Glacier Peace Park,” in Sustaining Rocky Mountain Landscapes: Science, Policy, and Management for the Crown of the Continent Ecosystem. Resources for the Future, eds T. Prato and D. Fagre (New York, NY: Routledge), 39–54.
Reyes-García, V., Fernández-Llamazares, Á., McElwee, P., Molnár, Z., Öllerer, K., Wilson, S. J., et al. (2018). The contributions of Indigenous peoples and local communities to ecological restoration. Restor. Ecol. 27, 3–8. doi: 10.1111/rec.12894
Romme, W. H., Boyce, M. S., Gresswell, R., Merrill, E. J., Minshall, G. W., Whitlock, C., et al. (2011). Twenty years after the 1988 Yellowstone fires: lessons about disturbance and ecosystems. Ecosystems 14, 1196–1215. doi: 10.1007/s10021-011-9470-6
Romme, W. H., Turner, M. G., Wallace, L. L., and Walker, J. S. (1995). Aspen, elk, and fire in the Northern Range of Yellowstone National Park. Ecology 76, 2097–2106. doi: 10.2307/1941684
Roos, C. I., Zedeño, M. N., Hollenback, K. L., and Erlick, M. M. (2018). Indigenous impacts on North American Great Plains fire regimes of the past millennium. Proc. Natl. Acad. Sci. U.S.A. 115, 8143–8148. doi: 10.1073/pnas.1805259115
Samson, F., and Knopf, F. (1994). Prairie conservation in North America. BioScience 44, 418–421. doi: 10.2307/1312365
Simonson, J. T., and Johnson, E. A. (2005). Development of the cultural landscape in the forest-grassland transition in southern Alberta controlled by topographic variables. J. Veg. Sci. 16, 523–532. doi: 10.1111/j.1654-1103.2005.tb02392.x
Stephens, S. L., Moghaddas, J. J., Edminster, C., Fiedler, C. E., Haase, S., Harrington, M., et al. (2009). Fire treatment effects on vegetation structure, fuels, and potential fire severity in western US Forests. Ecol. Appl. 19, 305–320. doi: 10.1890/07-1755.1
Stringham, T. K., Krueger, W. C., and Shaver, P. L. (2003). State and transition modeling: an ecological process approach. J. Range Manage. 56, 106–113. doi: 10.2307/4003893
Suding, K. N., Gross, K. L., and Houseman, G. R. (2004). Alternative states and positive feedbacks in restoration ecology. TREE 19, 46–53. doi: 10.1016/j.tree.2003.10.005
Turner, M. G., Hargrove, W. W., Gardner, R. H., and Romme, W. H. (1994). Effects of fire on landscape heterogeneity in Yellowstone National Park, Wyoming. J. Veg. Sci. 5, 731–742. doi: 10.2307/3235886
Turner, N. J., Ignace, M. G., and Ignace, R. (2000). Traditional ecological knowledge and wisdom of aboriginal peoples in British Columbia. Ecol. Appl. 10, 1275–1287. doi: 10.1890/1051-0761(2000)010[1275:TEKAWO]2.0.CO;2
Twidwell, D., Rogers, W. E., McMahon, E. A., Thomas, B. R., Kreuter, U. P., and Blankenship, T. L. (2012). Prescribed extreme fire effects on richness and invasion in coastal prairie. Inv. Plant Sci. Manage. 5, 330–340. doi: 10.1614/IPSM-D-12-00017.1
Van Auken, O. W. (2009). Causes and consequences of woody plant encroachment into western North American grasslands. J. Env. Man. 90, 2931–2942. doi: 10.1016/j.jenvman.2009.04.023
White, C. A., Perrakis, D. D., Kafka, V. G., and Ennis, T. (2011). Burning at the edge: integrating biophysical and eco-cultural fire processes in Canada's parks and protected areas. Fire Ecol. 7, 74–106. doi: 10.4996/fireecology.0701074
Williams, J. W., and Jackson, S. T. (2007). Novel climates, no-analog communities, and ecological surprises. Front. Ecol. Environ. 5, 475–482. doi: 10.1890/070037
Keywords: fire, traditional ecological knowledge, prairie, bison, colonization, resiliency, adaptive management
Citation: Eisenberg C, Anderson CL, Collingwood A, Sissons R, Dunn CJ, Meigs GW, Hibbs DE, Murphy S, Kuiper SD, SpearChief-Morris J, Little Bear L, Johnston B and Edson CB (2019) Out of the Ashes: Ecological Resilience to Extreme Wildfire, Prescribed Burns, and Indigenous Burning in Ecosystems. Front. Ecol. Evol. 7:436. doi: 10.3389/fevo.2019.00436
Received: 09 December 2018; Accepted: 25 October 2019;
Published: 26 November 2019.
Edited by:
David Jack Coates, Conservation and Attractions (DBCA), AustraliaReviewed by:
Urs Kreuter, Texas A&M University, United StatesJoshua Simon Halofsky, Washington Department of Natural Resources, United States
Copyright © 2019 Eisenberg, Anderson, Collingwood, Sissons, Dunn, Meigs, Hibbs, Murphy, Kuiper, SpearChief-Morris, Little Bear, Johnston and Edson. This is an open-access article distributed under the terms of the Creative Commons Attribution License (CC BY). The use, distribution or reproduction in other forums is permitted, provided the original author(s) and the copyright owner(s) are credited and that the original publication in this journal is cited, in accordance with accepted academic practice. No use, distribution or reproduction is permitted which does not comply with these terms.
*Correspondence: Cristina Eisenberg, cristina.eisenberg@oregonstate.edu