- 1German National Reference Centre for Borrelia, Bavarian Health and Food Safety Authority, Oberschleissheim, Germany
- 2Department of Biology and Biochemistry, University of Bath, Bath, United Kingdom
The Borrelia burgdorferi sensu lato species complex currently consists of more than 20 species that utilize small vertebrate hosts as reservoirs and ticks of the genus Ixodes as vectors. The bacterial parasite species differ in their ecology (reservoir hosts, competent vectors) as well as geographical distribution. Several studies have defined reservoir host association as a driver for diversification, speciation and pattern of spatial occurrence of populations but in this review we focus on vector association and its role in diversification and speciation of Borrelia. Borrelia bavariensis, a member of the species complex, uses small mammals as reservoir hosts and can cause Lyme borreliosis in humans. Phylogeographic analysis employing both genetic and genomic data shows that this species has undergone a recent range expansion, invading Europe from its probable original range in Asia while undergoing a drastic genetic bottleneck, suggesting that the colonization of Europe was a single event. We hypothesize that this invasion-like range expansion coincided with a change in the parasite's tick vector, switching from Ixodes persulcatus in Asia to I. ricinus in Europe. Making extensive use of the ecological concept of niche, we discuss the importance of host and vector associations in defining spatial range and their possible role in speciation in the B. burgdorferi sensu lato species complex.
Introduction—Parasite Invasiveness
Just like their hosts, parasites may on occasion abruptly expand their geographic range in an invasion-like process (Hatcher et al., 2012; Poulin, 2017; Chalkowski et al., 2018). The term “invasion” can be controversial. Some authors (e.g., Hoffmann and Courchamp, 2016) consider the natural expansion of a species' geographic range beyond its previously occurring limits to be essentially the same thing as a biological invasion caused by human introduction, while others (Wilson et al., 2016) insist that the term “invasion” should be reserved for those cases where colonization is due to human agency. We don't intend to enter this debate, but suggest that when a natural colonization by a species of new geographic space occurs rapidly and on a single occasion as the result of the transfer of a small number of individuals, and involves its entry into and adaptation to a new niche, it is bound to share many of the characteristics of a human-assisted invasion. In particular, we note that such abrupt range expansions differ significantly from the gradual changes in geographic range that occur when the boundaries of an entire ecosystem shift with gradual geological or climatic change. For these reasons, in this paper we describe the range expansion of the particular parasite with which we are concerned as “invasion-like.”
Regardless of whether human agency is involved, an invading parasite enters a new geographic region in which it did not previously occur and where it now causes disease (Hatcher et al., 2012). However, one can consider different mechanisms of invasion by parasites, as being due either to range expansion of host/vector populations (e.g., through changing climatic conditions) followed or paralleled by parasite expansion into expanded host/vector populations or due to “spill-over” and adaptation to new hosts/vectors. The invading parasite may cause changes in the population dynamics of the invaded population of the original host either directly through parasitism (Dunn, 2009), or indirectly by affecting the success of the original host's competitors and predators (Hatcher et al., 2006). In addition, it may affect populations of new host species through parasite “spillover” (Iglesias et al., 2015), and also alter the success of other parasites already present in the new domain through competition for existing and new hosts (Dunn, 2009). In turn, the parasite itself may as a result of its introduction undergo evolutionary change in order to adapt to its new circumstances (e.g., Juhasova et al., 2016).
In this paper we consider the invasion-like range expansion into Europe from Asia of one particular vector-borne bacterial parasite, Borrelia bavariensis, a member of the Lyme Borreliosis group of spirochetes, Borrelia burgdorferi sensu lato (s.l.). The concept of geographical invasion by bacteria and other prokaryote microbes is relatively less well-developed than for eukaryotic organisms (Mallon et al., 2015) or viruses (Kilpatrick, 2011); one reason for this is that bacteria have until recently been widely assumed to be globally distributed and rapidly dispersed, with their abundance in any particular place determined only by the suitability of the local environment (O'Malley, 2007).
In the case of the invasive B. bavariensis, additional interest is generated by the fact that this organism is an obligate parasite of vertebrate animals transmitted by an arthropod vector; this raises the question of the extent to which the evolved adaptation of a parasite with a complex life history (Auld and Tinsley, 2015) limits the extent of its geographic range. Consideration of how B. bavariensis extended its geographic range through vector adaptation may also shed light on the phylogeography of other members of the B. burgdorferi s.l. species complex.
Most previous work on parasite invasions has referred to eukaryotic parasites, and much less attention has been devoted to invasion-like range expansions of vector-transmitted pathogens and parasites. A notable exception to this generalization is that outbreaks of vector transmitted viruses in new geographic zones have been intensively investigated (reviewed by Randolph and Rogers, 2010). West Nile Virus (Charrel et al., 2003), Chikungunya virus (Tsetsarkin et al., 2007; Fritz et al., 2019), Dengue virus (Delatte et al., 2011), Bluetongue virus (Mardulyn et al., 2013), Zika virus (Metsky et al., 2017), and Sindbis virus (Ling et al., 2019) have received particular attention. In many of these cases, colonization of new geographic zones has involved human assistance. However, much less is known about invasion like range expansions in vector-borne bacteria.
Understanding how invasions of microbial parasites occur focuses attention on prediction. Identifying those parasites that pose the greatest potential threats to the human and animal health through invasion permits prophylactic strategies to be devised and scarce public health resources to be allocated with maximal effect. To identify factors that make the parasite potentially “invasive,” we need to understand the biotic and abiotic drivers that initially (i.e., before the invasion) acted to limit the geographic range of the parasite in question. These will be many, but it is obviously particularly important to understand the contribution(s) made by the home range(s) of the parasite's existing host(s) and vector(s). In this paper we make particular use of the ecological and evolutionary concept of niche to consider the likely adaptive mechanisms that enable both vector-switching and geographic invasion. Vector-switch is considered here as the adaptation of a parasite to a vector previously non-permissive for transmission, i.e., not vector-competent. Landscape genomics has already proved useful in understanding adaptation of invading free-living species to the physical environment (Li et al., 2017), but we are not aware of previous attempts to analyse the geographic invasiveness of a bacterial parasite in terms of its adaptation to a new parasitic or vector niche.
Niche theory has already been used as a tool to help understand how free-living microbes can succeed in invading natural microbial communities through the evolution of adaptive traits (see for example, Eisenhauer et al., 2013). Apart from selection and adaptation ecological processes including migration and population dynamics are important features of invasion processes. Thus, in their perspective paper on microbial invasions, Kinnunen et al. (2016) are sparing in their use of the term “niche,” instead they emphasize ecological processes such as migration and population dynamics. Such processes are almost certainly important in the invasion of free-living microbes into new environments. We appreciate that these processes may well be important in determining the probability of an invasion occurring in vector-transmitted parasites too (e.g., Ogden et al., 2008, 2013a; Mechai et al., 2018), but in the present paper we have chosen to focus on evolutionary change in the form of horizontal gene transfer and adaptation (Jacquot et al., 2014). Not least, this is because both these processes leave an identifiable signature in the parasite's genome (Longdon et al., 2018).
We have structured our review into two parts, the first part is on niche theory, explores niche concepts and the role of niches in biological invasion processes. The second part surveys the history and geographic distribution of the B. burgdorferi s.l. species complex and identifies B. bavariensis as a case to study the biological invasion of a vector-transmitted bacterial parasite. Emphasis is allotted to the role of tick vectors in driving diversification and speciation in this bacterial species complex, describing main vectors of Borrelia and factors that may influence vector competence (e.g., the genetic make-up of the bacteria, tick physiology, immunity, microbiome). Last but not least we put forward hypotheses on the mechanisms of the invasion-like spread of a bacterial parasite into new geographic areas.
Parasites: Niche and Invasions
The concept of multidimensional niche space helps to understand how the limits of an organism's geographic range are determined (Gaston, 2003) and therefore also casts light on the mechanisms of invasions and invasion-like range expansions and whether particular species are inherently invasive (Peterson et al., 2011). Analogous to the niche occupied by a statue in a public building, an ecological niche is an ecological space occupied by a particular organism that defines its role in the ecosystem. The larger and more complex the boundaries of the niche, the more extensive and many-faceted is the ecological role of the particular organism.
The term “niche” was originally introduced by Grinnell (1917), who defined it in descriptive environmental terms, and was adapted by Elton (1927), who instead chose to use functional terms based on community role. Although “niche” is fundamentally a phenomenological concept that aims to describe an organism's way of life (for example in exploiting other organisms for food, and evading exploitation by organisms that seek to predate or parasitize it), niche is of course also about the precision with which the organism is genetically adapted (for example through its physiology) to the environmental conditions it encounters. Hutchinson (1957) greatly improved the concept by pointing out that an organism's niche has many “dimensions” each of which describes one aspect of the focal organism's way of life and environment (allowing the Grinnell and Elton usages of the term to be combined).
Niche Helps to Understand Invasiveness
An “unoccupied niche” is susceptible to colonization, with or without human assistance, and identifying that niche leads to predictions about which organisms will invade and how they will spread. One prediction, for example, is that a species having “a broader niche” will be more invasive than another with “a narrower niche” (Vazquez, 2006). The hypothesis that a “broader” niche allows the organism to display a more extensive set of adaptations to varying ecological conditions, so that a larger geographic area will be potentially available to it (Slatyer et al., 2013). The idea that invasiveness is correlated with overall “niche breadth” can be tested at a simple level; the hypothesis is not universally validated by evidence but has received strong support in some cases (e.g., Sol, 2016; Granot et al., 2017). Unfortunately, however, it is not self-evident what is meant by overall “niche breadth” nor how it can be measured or compared. The concept of the Hutchinsonian niche with its multiple dimensions helps to visualize the niche's complexity, but since each dimension varies in breadth, possibly but not necessarily independently of all other dimensions, it is often not clear how the various dimensions can be summed to give a single measure of niche breadth. For example, what units should be used to describe a niche's dimensions? It is thus difficult to characterize the overall “breadth” of a niche in a simple way.
A further problem is that niche breadth may be maintained through evolutionary adaptation to more than one niche dimension, in which case, local populations of a species may each be separately adapted to local conditions, so that “niche breadth” is not actually a characteristic of the genome of the species in general at all, but a reflection of its genetic diversity. As a consequence, an invasion into a new geographic region would most likely be achieved by migration from only a suitably pre-adapted subset of the original species and this might be expected to leave the signature of a selective sweep in the genome of the invading population. Alternatively, the original niche breadth of a species may be the result of phenotypic plasticity, a situation that would be likely to favor invasiveness without genetic change. Such phenotypic plasticity is likely to be present in species that originally inhabit rapidly fluctuating environments (Lee and Gelembiuk, 2008).
Finally, it is simplistic to suppose that the existence of a correlation between niche breadth and geographic range size necessarily implies a causal relationship in the direction of the former to the latter. It may also be the case that larger geographic range actually drives adaptation to greater variety of niches (Jahner et al., 2011).
The Parasitic Niche
The above discussion indicates that there are problems in using niche theory to understand invasiveness (i.e., the tendency to invasion-like geographic range expansion when the opportunity for this arises). Parasites, however, offer a way out of these difficulties. Obligate vector-borne parasites live their entire lives within their hosts and vectors and do not occur free in the environment. Niches occupied by such parasites are therefore almost all entirely associated with selective forces exerted by (or at least mediated through) their vectors and hosts. Because related parasites tend to have vectors and hosts that share many characteristics, and can thus be measured in the same way, parasites offer an exceptionally favorable opportunity to test niche-related ideas about invasiveness; we have a much better chance of realistically comparing “niche breadth” between related parasites. We might say that a broader parasitic niche would be one that has a larger number of dimensions (i.e., corresponding to a larger number of hosts/vectors) or dimensions that are spatially more extensive (i.e., the geographic ranges of their hosts/vectors are larger). The precision with which the parasite's niche is described might be increased by considering each of the different physiological or biochemical interactions between the parasite and its host (for example the various immune defenses deployed by the host, and associated countermeasures used by the parasite) to be a separate dimension of the niche. As far as we are aware, however, the hypothesis that the geographical invasiveness of obligate parasites is related to the breadth of host- and/or vector-associated niche space has not been tested (e.g., Vazquez, 2006; Slatyer et al., 2013).
On the other hand, parasites co-evolve with hosts and vectors (Woolhouse et al., 2002; Wade, 2007; Toft and Andersson, 2010), because parasites exert strong selective pressures on host(s) (and vectors) which in turn evolve to reject the parasite, effectively narrowing the niche available to the parasite. Much of the program of parasitological research is concerned with identifying such co-evolutionary adaptations (Penczykowski et al., 2016). Co-evolution is notoriously tricky to study, because it is a system that is subject to positive feedback. It is often found that co-evolution is local, rapid and episodic, affecting several different gene pairs that coevolve episodically and occur unpredictably in time and space (this is Thompson's “geographic mosaic” model; Thompson, 1999).
Another aspect of niche theory that is relevant here is that the fundamental niche of an organism, which extends to the furthest limits of all dimensions that can be tolerated by that species, is almost always greater in extent than the realized niche, which is that part of the niche that is actually occupied. The greater the mismatch between the realized and fundamental niche, the greater is the potential for invasion (Soberon and Arroyo-Pena, 2017). It is obvious that the extent to which the size of the realized niche approaches that of the fundamental niche is subject to limitations due to local physical environmental conditions; for example, if it is too hot, too cold, too wet, or too dry. In the case of a parasite, this kind of environmental limitation may be mediated through biotic interactions (because of their own physical limitations, hosts, and vectors are not present everywhere). It is less intuitively obvious that in the case of parasites, biotic limitations may not be due to unsuitable environmental conditions, but due to phylogeographic contingency: any one of a set of potentially interacting parasites, hosts and vectors may be able to persist in a particular geographic zone, but is nevertheless presently absent simply because the organism in question has not yet expanded its range to occupy all of its fundamental niche. This absence of either the host or the vector may then limit the occurrence of the parasite.
Such a mismatch between the fundamental and realized niche can be corrected by geographic range expansion. Considering this from the point of view of the parasite, such realization of potential niche space can happen in two ways: first the parasite may invade a new environment when an existing host or vector expands its own range, taking the parasite with it. In this case, successful range expansion of the parasite depends completely on the invasiveness of the existing host or vector. Alternatively, a parasite may start utilizing a new species as host. This parasite-host combination might not have been utilized previously simply because that host species did not co-occur with the parasite in its existing range. Removal of a former landscape or climatic barrier to geographic range expansion of that host or vector may allow the parasite to expand its geographic range within what was actually always the fundamental niche simply because it can now exploit a new host or vector in this previously unexploited environment. For obligate parasites, the opportunity to enter the new geographic zone will likely depend on being transported there within an existing host or vector, but once it is there its success may depend on a switch in its host(s) or vector(s). An example of this is the invasion of European fresh-water habitats by the fungal parasite Aphanomyces astaci, a parasite of the introduced North American signal crayfish, Pacifastacus leniusculus. As a result, the parasite has increased the extent of its realized niche to include the European crayfish host Austropotamobius pallipes, which is now revealed as a part of its fundamental niche that was previously only latent (Edgerton et al., 2004; Vrålstad et al., 2011).
Different Ecological Processes Limit Expansion of the Fundamental and Realized Niches
The increase in size of the realized niche by geographic range expansion to occupy a greater fraction of the fundamental niche necessarily involves an increase in population size. This is an ecological process that need not involve evolutionary change at all. The implication is that once an existing barrier to exploitation within one or more existing niche dimensions has been removed, population dynamic feedbacks will allow population expansion to occur; density-dependent limitations will be temporarily reduced and allow an increase in the number of surviving progeny. As the fraction of the fundamental niche that is actually realized increases, these feedbacks will act to slow and eventually stop further population growth. By contrast, an invading species may expand its geographic range (i.e., invade a new adaptive zone) because of some genetically based adaptive change (in the case of a parasite this might be the ability to exploit a new host or vector). In this case we see a change in the extent of the fundamental niche, which may or may not be accompanied by a change in the proportion of the fundamental niche that is actually realized.
In the latter case, it is appropriate to ask what sets limits to the size (geographic extent) of the fundamental niche? Why can parasites not simply adapt to colonize more and more kinds of host or vector? Of course, the answer is that there are trade-offs to the acquisition of new adaptations. In the case of a parasite, the fitness advantage that accrues as a result of the ability to utilize a new host or vector species may be offset by a loss of fitness that results from a decreased ability to exploit existing hosts or vectors.
The Borrelia burgdorferi Sensu Lato (S.L.) Species Complex
Lyme borreliosis (or Lyme disease) is a disease of humans caused by tick-transmitted parasitic spirochetal bacteria belonging to the B. burgdorferi s.l. species complex. The bacteria are maintained in natural transmission cycles between their vertebrate reservoir hosts and vector ticks of the genus Ixodes (Figure 1; Kurtenbach et al., 2006; Radolf et al., 2012). Currently more than 20 genospecies have been described that vary in their host associations, vector associations and geographical distribution (Table 1, Figure 2). Adaptation to different hosts has been considered to be one of the main drivers for diversification of species of the B. burgdorferi s.l. species complex and for determining the spatial range and structure of populations (Piesman, 2002; Kurtenbach et al., 2006; Hoen et al., 2009; Vollmer et al., 2011, 2013; Margos et al., 2012b; Ogden et al., 2013b; Mechai et al., 2018). Although an extensive range of wild vertebrates can act as hosts for the B. burgdorferi s.l. complex as a whole, with lizards, birds, and small mammals being exploited, most single B. burgdorferi s.l. species can utilize only a subset of these hosts to support their life cycle (Gern et al., 1998; Gern and Humair, 2002). There is also a number of Ixodes species that act as competent vectors for B. burgdorferi s.l. As for hosts, many single B. burgdorferi s.l. species are adapted to utilize a subset of these Ixodes species as vector (Masuzawa, 2004; Margos et al., 2011). However, the impact of vector association on B. burgdorferi s.l. diversification and speciation is less well-explored.
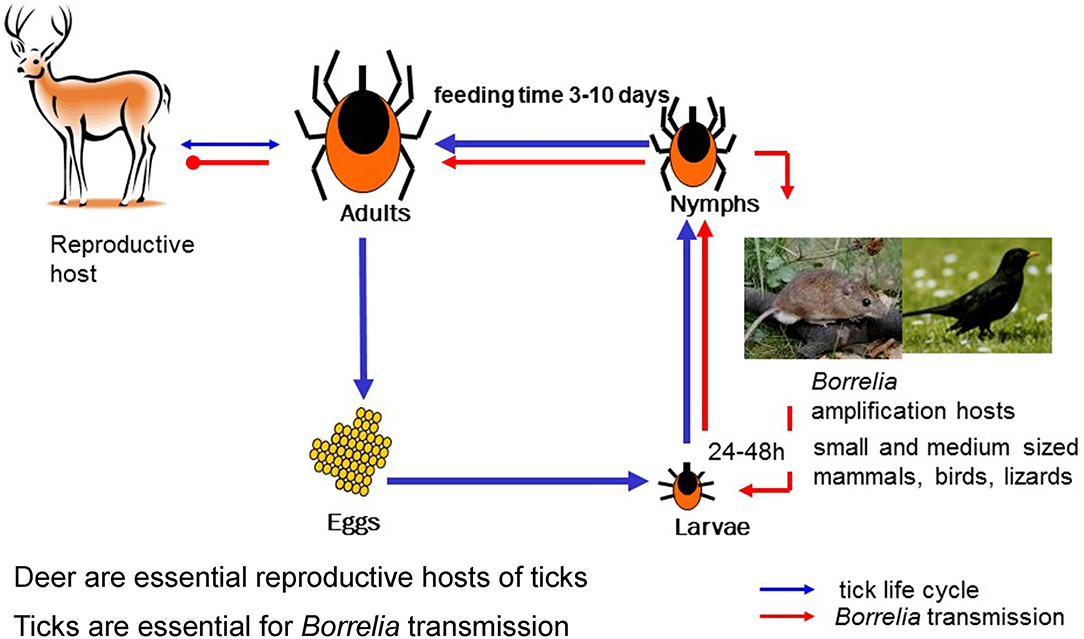
Figure 1. Ixodes and Borrelia life cycle. The developmental time from egg to adult Ixodes tick depends on climate and environmental conditions and may take 2–7 years. Each of the stages, larvae, nymph and adult female take one blood meal that can—depending on the stage—last 3–10 days. Engorged ticks drop off the host, digest the blood meal, and molt into the next stage. Importantly, both, larvae and nymphs, prefer small to medium sized vertebrates as host. This and the questing phenology of these stages ensures transmission of Borrelia from host to vector and to the next host. Adult females prefer larger animals such as deer as host. Deer has been shown to be incompetent as Borrelia reservoir host but they are essential for maintaining tick populations. Humans are considered accidental hosts and are dead-end hosts for Borrelia.
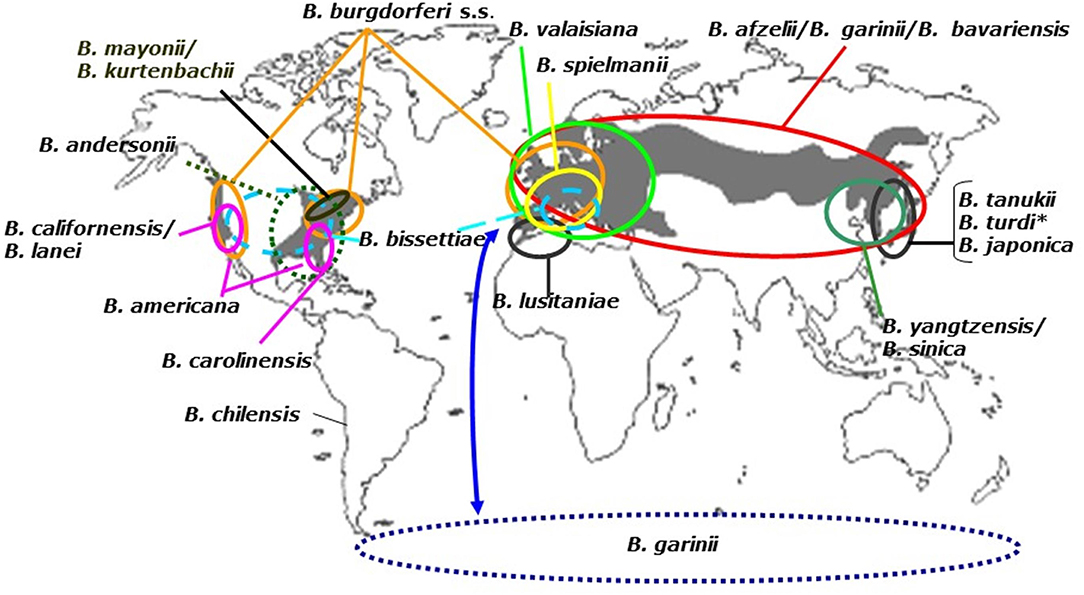
Figure 2. Distribution of Borrelia burgdorferi sensu lato genospecies. To date, 21 Borrelia genospecies have been named and their geographic ranges are shown here. The gray background corresponds to the presence of Ixodes vectors (see above). B. turdi (*), also occurs in Europe. The dotted circle represents the B. garinii transmission cycle that ensues in sea bird colonies in the Southern and Northern hemisphere. The latter is not shown to reasons of clarity.
Geographic Ranges of the Lyme Borreliosis Spirochetes
The dependence of Borrelia on both vector and reservoir hosts determines the geographic distribution of the Lyme borreliosis group of spirochetes. With few exceptions such as B. chilensis (Ivanova et al., 2014), most B. burgdorferi s.l. species are found between 40 and 60° latitude where Lyme borreliosis is reported to occur in humans (Figure 2).
The geographic ranges of the various B. burgdorferi s.l. species (Margos et al., 2011) are in each case limited to those locations in which both reservoir hosts and vector ticks are able to maintain natural transmission cycles (Kurtenbach et al., 2006, 2010) and thus we should be able to define the fundamental niche of each Borrelia species simply by taking account of where its vectors and hosts occur. So long as at least one associated vector and one associated host are present in a particular geographic zone, a Borrelia spirochete utilizing them could theoretically be maintained there. But because most B. burgdorferi s.l. species can utilize multiple vertebrate host species and a number can utilize more than one vector, and because the ecological associations between borreliae, ticks, and reservoir hosts are not all equivalent in strength, the realized niche actually achieved by each B. burgdorferi s.l. species will almost always in practice be considerably less than the simple overlap of the geographic measures (Tsao, 2009). The actual spatial limitation for each spirochete species (i.e., its realized niche) will be roughly equivalent to the sum of all those areas in which both at least one vector species and one host species occur at sufficiently high density to maintain its transmission cycle. In more formal terms, the value of the basic reproduction number R0 for every local population of the parasite, summed over all its hosts and vectors, must exceed unity (Randolph, 1998; Hartemink et al., 2008; Tsao, 2009). The relative contributions to the overall R0 of particular host and vector associations may of course vary between particular locations within its distribution.
Importantly, however, we cannot simply add up values of R0 that have been determined for each vector and each host under laboratory conditions, because the presence of less efficient vectors and hosts will impact negatively on the value of R0 achieved by the “best” vectors and hosts (Randolph and Craine, 1995; Ostfeld et al., 2006). These negative effects may be very important. Some potential mammalian hosts (mostly large animals such as ruminants) can be colonized by B. burgdorferi s.l. spirochetes when they are bitten by an infected tick vector, but are completely non-permissive when it comes to transmission of the bacteria to a new tick. In fact, feeding on a deer may actually clear a Borrelia infection in a tick (Telford et al., 1988; Kurtenbach et al., 1998). The presence of such “dead end hosts” can influence the parasite's success in diametrically opposite ways. On one hand, the presence of deer in a particular geographic zone may permit the population density of the vector ticks that transmit Borrelia to increase; this increases the likelihood of successful transmission of spirochetes from infected reservoir hosts to ticks and thus increases R0. On the other hand, the presence of large numbers of deer may drive ticks to feed with greater probability on deer than on small mammals and this may actually suppress the spirochete infection rate of true reservoir hosts in that location (reviewed by Ogden and Tsao, 2009; Kilpatrick et al., 2017).
Furthermore, the application of multilocus sequence typing to B. burgdorferi sensu stricto in combination with landscape ecology has shown that variation in host availability or landscape connectivity can strongly impact the spatial pattern of population distribution (Hoen et al., 2009; Margos et al., 2012b; Mechai et al., 2018). In the late nineteenth century, human-induced changes to habitats and severe hunting in the northeastern USA almost led to regional extinction of deer, which are important hosts for tick survival (Barbour and Fish, 1993). As a consequence of a likely decline of vector populations, Borrelia populations were reduced to spatially divided refuge populations in Northeast and Midwest. Independent expansion of these populations correlated with reintroduction of hosts for vector and Borrelia as well as environmental ecological changes such as reforestation (Hoen et al., 2009). Other population boundaries correlated with landscape barriers such as the Great Plains and/or the Rocky Mountains (Margos et al., 2012b). In a more recent study attempting to understand spatial spread pattern of B. burgdorferi s.s. into a zone of emerging Lyme borreliosis risk, the importance of landscape connectivity for the spatial distribution of B. burgdorferi s.s. of certain sequence types was underlined (Mechai et al., 2018).
Apart from host associations that impact the distribution of Borrelia species and populations, the non-uniform distribution pattern of Borrelia genospecies observed in field studies suggests that vector associations (based on differential vector competence) do indeed play an important role in limiting their geographic distribution ranges (Figure 3; Margos et al., 2011). Evidence for this is provided by the ability of some Borrelia species to utilize a wide range of vectors (Masuzawa, 2004; Margos et al., 2012a), for example B. garinii can be vectored by I. persulcatus, I.pavlovskyi, I. ricinus, and I. uriae. Accordingly, B. garinii's geographic distribution ranges from Japan to France and to sea bird colonies in both the Northern and Southern Hemispheres (Figure 2). B. garinii has been found in sea bird colonies in Newfoundland (Munro et al., 2017) but has not been discovered in I. scapularis dominated regions or in I. pacificus (Hamer et al., 2007; Hoen et al., 2009; Ogden et al., 2010; Mechai et al., 2013; Fedorova et al., 2014). Similarly, B. burgdorferi s.s. is able to utilize I. scapularis, I. pacificus, I. spinipalpis, and I. affinis as vectors in North America (Burgdorfer et al., 1982; Maupin et al., 1994; Clover and Lane, 1995; Maggi et al., 2010), as well as I. ricinus in Europe (Rauter and Hartung, 2005; Sertour et al., 2018), but has not been found in I. persulcatus (Korenberg et al., 2002; Mukhacheva and Kovalev, 2013). B. valaisiana, a bird adapted Borrelia species, is frequently found in Europe associated with I. ricinus but only a single occurrence in Russia has been recorded (Kurilshikov et al., 2014) suggesting that I. persulcatus is not a competent vector. Consistent with this, in the overlapping zone of I. ricinus and I. persulcatus, the prevalence of B. valaisiana is higher in I. ricinus and drops to much lower values in I. persulcatus (Bormane et al., 2004).
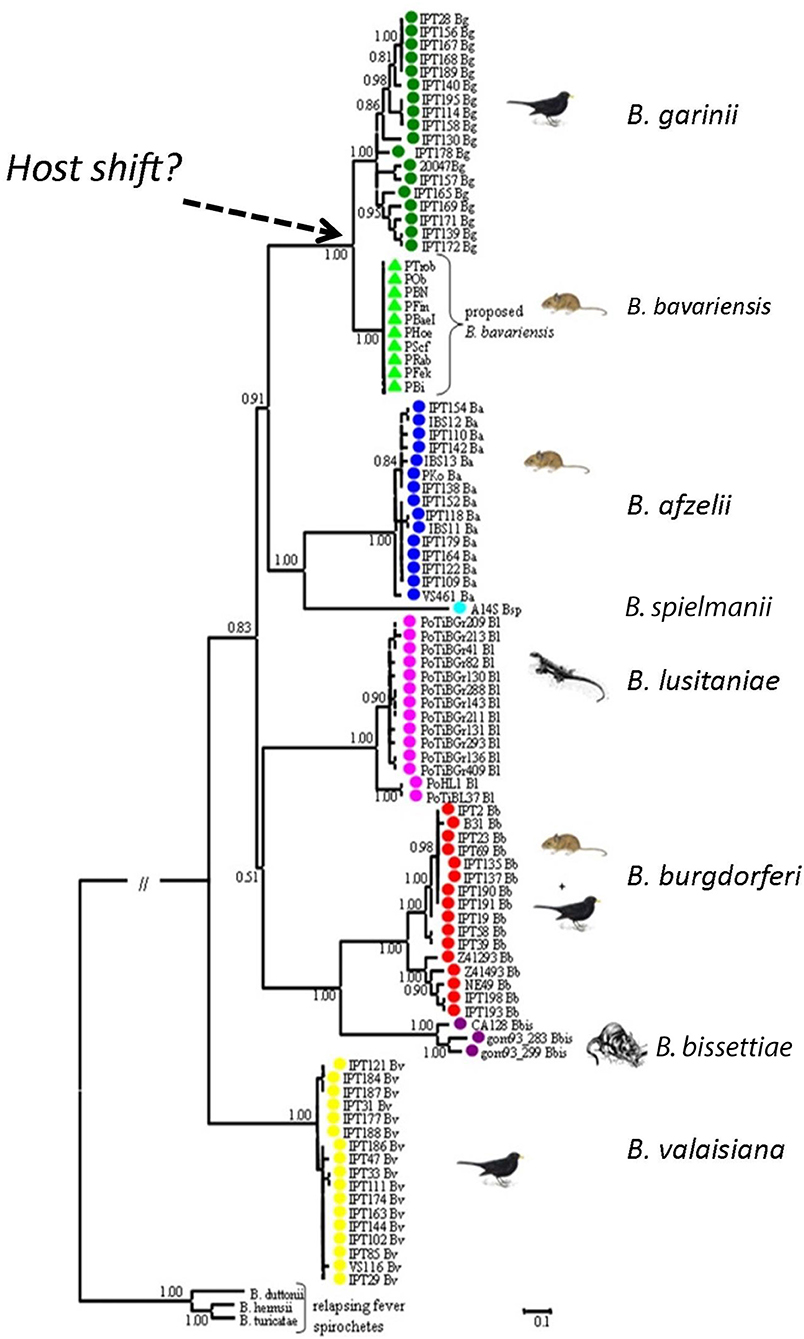
Figure 3. Phylogenetic relationship of Borrelia genospecies. The phylogeny was generated using concatenated sequences of MLST housekeeping genes. Borrelia bavariensis is closest related and forms a sister clade to B. garinii. With permission from Margos et al. (2009), Applied and Environmental Microbiology 75, 5410–5416.
Borrelia bavariensis—A Model for Vector Adaptation, Spatial Spread, and Species Invasion
Borrelia bavariensis is one of the >20 named and proposed genospecies within the B. burgdorferi s.l. species complex (Margos et al., 2016). Borrelia bavariensis was described as a new genospecies of the B. burgdorferi s.l. species complex using MLSA (Figure 3; Margos et al., 2009, 2013). Ecological considerations and measures of genetic distances showed that B. bavariensis was sufficiently divergent from B. garinii (the closest related species) to deserve species status. Prior to this B. bavariensis had been considered to belong to the species B. garinii. This was based on methods using a serotyping system with monoclonal antibodies and or intergenic spacer typing, respectively: strains identified in I. ricinus in Western Europe were termed B. garinii outer surface protein (Osp) A type 4 (Wilske et al., 1996) whilst those found in I. persulcatus in Eastern Europe or Asia were termed B. garinii NT29-like strains (Korenberg et al., 2002; Miyamoto and Masuzawa, 2002; Masuzawa, 2004).
It had previously been known that Western European B. garinii OspA types (i.e., types 3, 5–8) (Wilske et al., 1996; Fingerle et al., 2008; Taragel'ova et al., 2008) utilize birds as reservoir hosts, while OspA type 4 strains use rodents as reservoir hosts (Hu et al., 2001; Huegli et al., 2002). In Asia, NT29 and related strains also comprised a rodent-associated ecotype within B. garinii, but their relationship to OspA type 4 strains from Europe remained unclear, as European and Asian B. garinii differed not only in their OspA pattern, but also showed differences in the RFLP pattern of the 5S-23S intergenic spacer (Masuzawa et al., 2005). MLSA typing showed that both European and Asian rodent-associated ecotypes belonged to B. bavariensis (Takano et al., 2011; Margos et al., 2013; Scholz et al., 2013). Throughout its very extensive geographic range in Eurasia B. bavariensis is associated only with small mammals, implying that a host switch occurred at some time in the past that allowed, or perhaps even was the cause of the divergence of B. bavariensis from B. garinii. Phylogenetic analysis provides evidence that the European and Asian B. bavariensis sp. nov. form sister clades which cluster next to B. garinii (Margos et al., 2009, 2013; Gatzmann et al., 2015; Figures 3, 4).
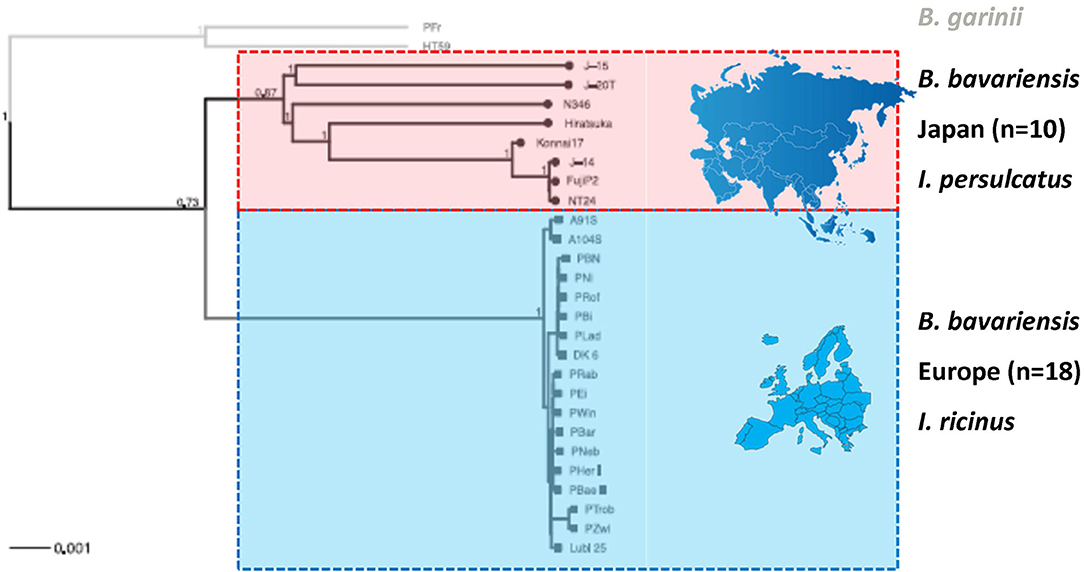
Figure 4. Phylogeny of B. bavariensis from Asia and Europe. The tree was generated using chromosomal single nucleotide polymorphisms. Taxa included are B. bavariensis from I. persulcatus (Japan) and I. ricinus (Europe) dominated regions. The genetic homogeneity in European B. bavariensis stands in strong contrast with the genetic heterogeneity of B. bavariensis from Asia. B. garinii isolates were used as outgroup. The scale bars are expressed in units of substitution per site. With permission from Gatzmann et al. (2015), Ticks and Tick-borne Diseases 6, 344–351.
Vector Adaptation and Geographical Invasion
Previous investigations using RFLP analysis had suggested that strains of ospA type 4 (i.e., Western European B. bavariensis) were genetically highly homogenous (Marconi et al., 1999), a finding that was confirmed using MLSA (Margos et al., 2009) and NGS (Gatzmann et al., 2015). The presence of extensive genetic variation within Asian strains of B. bavariensis (comparable in extent to that within B. garinii itself; Figure 4) implies that the split between B. garinii and B. bavariensis took place relatively long ago, although clearly more recently than the original radiation of the B. burgdorferi s.l. complex as a whole (Becker et al., 2016). The invasion-like range expansion of B. bavariensis into Europe, on the other hand, appears to have happened much more recently, and to have involved a selective sweep that imposed a severe bottleneck on genetic variation in the genome of the surviving European invaders (Figure 4). Since Asian B. bavariensis are associated only with I. persulcatus, a tick species which does not occur in Western Europe, while European B. bavariensis are associated solely with the European species, I. ricinus (Postic et al., 1997; Korenberg et al., 2002; Masuzawa et al., 2005; Fingerle et al., 2008; Takano et al., 2011; Geller et al., 2013; Mukhacheva and Kovalev, 2013, 2014; Scholz et al., 2013), it seems likely that colonization of Europe by the spirochete must have been coincident with, or driven by, a switch in its vector association.
Importantly, the genetic homogeneity of European B. bavariensis, the close genetic relationship of European and Asian B. bavariensis and the recent divergence from B. garinii have all now been confirmed by data derived from next generation sequencing and analyses of the core genome, the main chromosome and the two plasmids lp54 and cp26 (Gatzmann et al., 2015; Becker et al., 2016). In European B. bavariensis no more than 400 single nucleotide polymorphisms (SNPs) were found on the main chromosome between the most divergent isolates while approximately 10 times more chromosomal SNPs were noticed in Asian B. bavariensis, giving rise to the deep branching pattern seen in the phylogeny of the latter population (Figure 4; Gatzmann et al., 2015).
Analyses of the core genome (chromosome, lp54, and cp26) of European and Japanese B. bavariensis revealed genes that were under positive selection in the European population (Gatzmann et al., 2015). Among those were two genes flhB (BG0275) and antigen S1 (BGA04) (a homolog of BBA05) which had previously been shown to be up-regulated under conditions that simulate vector conditions (Ojaimi et al., 2003) or in feeding nymphs (Xu et al., 2010). The results presented by Gatzmann et al. (2015) were based on short-read NGS data which did not allow complete assembly of all plasmids in B. bavariensis and thus our knowledge of the comparative genomics of plasmid encoded genes in this species remains incomplete. This is an avenue for future consideration.
In this context it is important to emphasize that there is so far no direct experimental evidence on the vector competence of different tick species for the different B. bavariensis populations. However, strong support for differential vector adaptation of European vs. Asian B. bavariensis populations comes from field studies. The occurrence of B. burgdorferi s.l. spirochetes in ticks from the European and Asian parts of Russia has been studied since the early 1980s, soon after the description of B. burgdorferi s.s. (Korenberg et al., 2002). Ixodes ricinus, I. persulcatus, and I. trianguliceps ticks were used to culture Borrelia. Intriguingly, B. bavariensis (then designated as B. garinii) NT29-type strains were only ever isolated from I. persulcatus ticks and never from I. ricinus ticks, while B. afzelii and bird associated B. garinii were found in both tick species. Postic et al. (1997) concluded from this work that NT29-type strains (now correctly designated as Asian B. bavariensis) are associated only with I. persulcatus. Remarkably, in terms of vector adaptation, B. burgdorferi s.s., B. lusitaniae, and B. valaisiana were also found only in I. ricinus, and not in I. persulcatus (Korenberg et al., 2002). Subsequent molecular studies conducted in Asia, Mongolia, Russia and Siberia all found only Asian type B. bavariensis in I. persulcatus (Masuzawa et al., 2005; Takano et al., 2011; Mukhacheva and Kovalev, 2013, 2014; Scholz et al., 2013). The most compelling evidence yet for the contrasting vector association/adaptations of the European and Asian populations of B. bavariensis has now been provided by a study conducted in the overlapping zone of I. ricinus and I. persulcatus in Estonia (Geller et al., 2013). These authors reported that Asian type B. bavariensis was associated only with I. persulcatus, and not with I. ricinus. Moreover, a European type of B. bavariensis has recently been found in I. ricinus in Latvia (Mercy Okeyo, NRZ Borrelia, personal communication).
Ixodes Vectors of B. Burgdorferi S.L.
Ticks with more generalist feeding behaviors serve as bridge vectors for agents of human Lyme borreliosis and are considered the most important vectors for Borrelia. They have—for the most part—distinct geographical distribution ranges and include I. pacificus (west of the Rocky Mountains) and I. scapularis (east of the Rocky Mountains—principally the Northeast, Midwest, and Southeast regions of the USA) in North America, I. ricinus in Europe and I. persulcatus in Eastern Europe and Asia (Figure 5; Swanson et al., 2006).
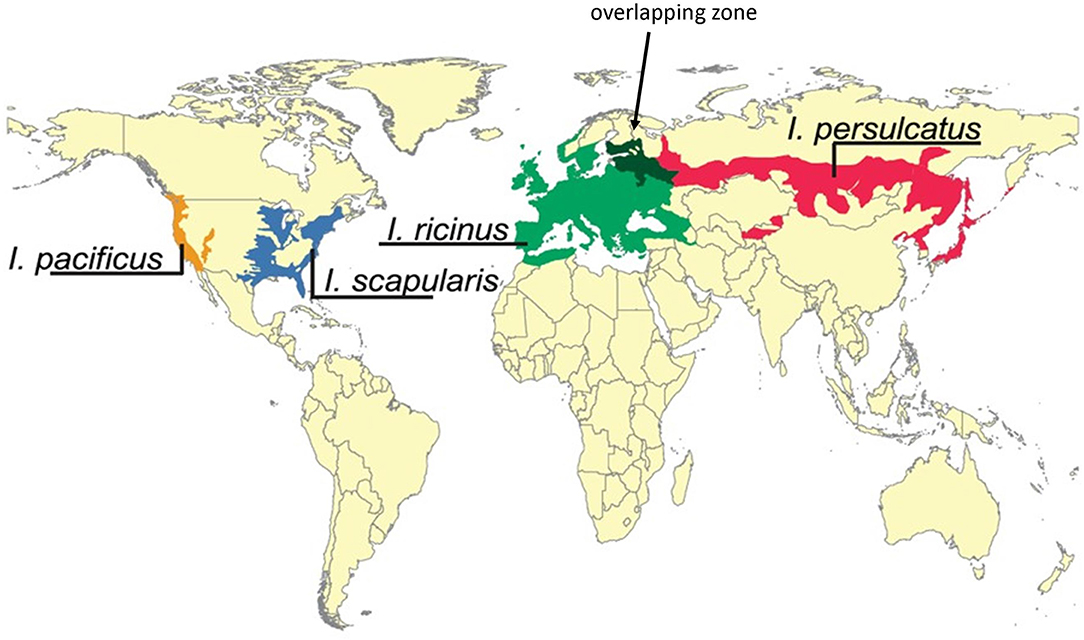
Figure 5. Geographic distribution of main Borrelia vectors Ixodes persulcatus, Ixodes ricinus, Ixodes scapularis, and Ixodes pacificus. Note the overlapping region of I. persulcatus and I. ricinus (dark green zone indicated by an arrow). Modified after and re-used with permission from Swanson et al. (2006), Coinfections Acquired from Ixodes Ticks, Clin Microbiol Rev, 19, 708–727. These ticks have generalist feeding behaviors and serve as bridge vectors for agents of human Lyme borreliosis and are considered the most important vectors for Borrelia. They have—for the most part—distinct geographical distribution ranges and include I. pacificus (west of the Rocky Mountains) and I. scapularis (east of the Rocky Mountains, Northeast, Midwest and Southeast) in North America, I. ricinus in Europe and I. persulcatus in Eastern Europe and Asia.
Experimental studies have shown that many Ixodes species are able to acquire Borrelia from an infected host and harbor live bacteria or bacterial DNA in the midgut trans-stadially (reviewed by Eisen and Lane, 2002). But the ability to efficiently transmit Borrelia to new hosts varies greatly between Ixodes species: for example, the vector competence of I. muris was very poor for B. burgdorferi s.s., I. scapularis, and I. ricinus differ in their vector competence for B afzelii and B. burgdorferi s.s., and ticks other than Ixodes spp. are poor vectors for B. burgdorferi s.l. (reviewed by Lane et al., 1994; Dolan et al., 1997, 1998, 2000; Eisen and Lane, 2002; Eisen et al., 2003). Furthermore, and as noted above, the geographic distribution of Borrelia species such as B. valaisiana, B. spielmanii, and B. burgdorferi s.s. implies that I. persulcatus is not a vector for these Borrelia species (Postic et al., 1997; Korenberg et al., 2002; Bormane et al., 2004; Masuzawa et al., 2005).
So, what confers differential vector competence of Ixodes species on the various Borrelia species? The simple answer is that we do not know, but one can extrapolate from other arthropod-parasite systems (Beerntsen et al., 2000; Lehane et al., 2004; Molina-Cruz et al., 2017) to conclude that genetically encoded traits of both bacterium and vector are likely to be important, probably including (1) Borrelia cell-surface molecules acting as ligands for receptor-molecules, conferring Borrelia resistance to tick anti-microbial defenses, or Borrelia factors that modulate the expression or efficacy of tick defenses, (2) tick physiology; (3) the tick's immune system, and (4) the tick microbiome. Of course, the balanced interplay between all these factors needs to be considered as none of them works in isolation and it has been shown that other microbes present in the tick's microbiome may manipulate the tick's immune system and vice versa (see below) (de la Fuente et al., 2017).
Borrelia Genes
Although several Borrelia genes have been identified that are important for persistence of Borrelia in the tick or for receptor interaction, how these genes differ between species and which genes allow different Borrelia species to utilize certain tick vectors is unknown. To further characterize such genes and to pinpoint their involvement in differential vector compatibility will require comparative genomics studies of Borrelia species that utilize different tick species as vector. Gene upregulation in Borrelia during or following transmission to the vector has been demonstrated and several genes have been shown to be crucial for transmission to, or survival of, Borrelia in the vector (e.g., Pal and Fikrig, 2010; Radolf et al., 2012). A comprehensive study showed that 246 gene transcripts were upregulated and transcribed in Borrelia during or after a blood meal was taken by ticks; 88 of these were present in Borrelia taken up by both, larvae and nymphs, while 17 Borrelia transcripts were only found in Borrelia taken up by larvae during blood feeding (Iyer et al., 2015). Although transcription does not equal presence of proteins, this study does suggest that some of these gene products are likely to be important for survival of Borrelia in the vector. In addition, studies on the gene expression regulation system RpoS identified 41 genes that are repressed in B. burgdorferi in vitro and under conditions representing the vertebrate host. Although a good number encoded hypothetical proteins, many of the identified genes were known to be important for survival in, and interaction with, the vector (Caimano et al., 2019). Whether variations in these molecules contribute to differential vector competence needs to be further investigated.
Some molecules that have been shown to have a function within the tick vector include the Outer surface protein A (OspA) which binds to the vector midgut molecule TROSPA (Pal et al., 2004); BBE31 which binds to the vector molecule Tre31, a protein secreted by tick midgut epithelial cells (Zhang et al., 2011); PdeB, a phosphodiesterase required for survival in, and transmission by, ticks; Lpm1, a surface molecule, depletion of which inhibits Borrelia in the vector (Koci et al., 2018); BB0365, BB0690, BptA, lp6.6, BBk32 are all required for persistence in the tick, while antigen P35 (BBA64) may play a role in tick-to-host transmission (Fikrig et al., 2000; Radolf et al., 2012; Brandt et al., 2014). In a recent study using transposon-based gene interruption approach, >100 genes were shown to be involved in Borrelia survival in larval ticks (Phelan et al., 2019). These included previously reported genes and novel genes important for tick survival of Borrelia. For 46 of these genes complete absence of transmission was observed after knockout, while for 56 other genes a drastic reduction (90%) of transmission occurred. Of the genes inducing complete interruption of transmission 22% were predicted lipoproteins, but for 41% was no predicted function.
Tick Physiology
From the vector's point of view, it is likely that physiological differences between tick species have an impact on vector competence. Generally, Ixodes species are three-host ticks and as such, both biotic and abiotic factors influence where they can survive. On-host feeding periods are short compared to off-host periods, which include periods of diapause (genetically programmed) or quiescence (due to unfavorable conditions). Abiotic factors that influence tick physiology include temperature, humidity, and day-length, all of which have an impact on host seeking phenology, success of egg hatching, overwintering of immature and adult stages. During off-host periods, hot conditions may result in overheating, freezing in sub-zero temperatures, or dehydration in dry conditions. Such conditions require ticks to seek refuges for cooling down or re-hydration and appropriate (micro-)habitats with suitable vegetation or leaf litter layer therefore play an important role in the tick's life cycle (Medlock et al., 2013; Eisen et al., 2016). Ixodes ricinus and I. persulcatus overlap in a region ranging from Estonia to Russia and, although some fluctuation of populations may occur (Filipova, 2017), the general geographic range distribution of the two tick species persists. Differences in host seeking phenology due to differences in tick physiology may affect feeding success in such overlapping zones and it is conceivable that this has an influence on vector competence (Korenberg et al., 1991, 2002; Korenberg, 1995; Alekseev et al., 1998). An impressive example of changed host-seeking phenology was reported for I. scapularis by Gatewood et al. (2009). Ixodes scapularis populations in Midwestern regions of the USA showed more seasonal synchrony of nymphal and larval feeding pattern. Investigations of B. burgdorferi s.s. infection pattern revealed that lineages which carried the 16S-23S rRNA intergenic spacer restriction fragment length polymorphism sequence type 1 were more frequently found in northeastern Ixodes populations (Gatewood et al., 2009). There are several excellent reviews on these topics (Lindgren and Jaenson, 2006; Ogden et al., 2006, 2013b; Medlock et al., 2013; Eisen et al., 2016) and it would go beyond the scope of this review to expand further on this.
Tick Immunity
An attractive hypothesis is that tick immunity plays a role in differential vector competence. In recent years progress has been made in recognizing the complexity of the tick's immune system (reviewed in Hajdusek et al., 2013; Kitsou and Pal, 2018) and it has been shown that Ixodes spp. possess a number of immune effectors and modulators such as recognition molecules, among them thioester-containing proteins (T-TEPS, that serve as lectins labeling cells for immune attack), phagocytotic hemocytes, defensins, lysozymes, antimicrobial peptides, a dityrosine network (DTN), and (atypical) signaling pathways. The signaling pathways regulating the immune system include Toll, IMD (Immunodeficiency) and JAK-STAT (Janus Kinase/Signal Transducers and Activators of Transcription) as well as an indirect, cross-species signaling pathway that recognizes the cytokine interferon gamma in the blood of the vertebrate host (Johns et al., 2001; Hajdusek et al., 2013; Yang et al., 2014; Sonenshine and Macaluso, 2017; Urbanova et al., 2017). RNA interference studies on genes involved in the tick's immune response have shown that depletion of expression may lead to suppression of Borrelia colonization in ticks (Narasimhan et al., 2017) suggesting that Borrelia can exploit tick molecules to its own advantage. In addition, the induction by Borrelia in I. scapularis of a protein with a Reeler domain (PIXR) limits bacterial biofilm formation in the tick's gut, thereby preventing alterations in the microbiome and promoting colonization by Borrelia (Narasimhan et al., 2017). Thus, it is highly likely that immune effectors play an important role in determining the competence of Ixodes species for Borrelia species and vice versa.
The Microbiome of Ticks
In the past decade much attention has been invested to study the tick's microbiome in more detail. These studies have shown that the microbiome of ticks consists of microorganisms associated with the outer surface of ticks, the gut microbiome, and endosymbiontic bacteria (reviewed by Narasimhan and Fikrig, 2015). Initial studies on different Ixodes species (e.g., I. scapurlaris, I. ricinus, I. pacificus, and I. persulcatus) using high throughput sequencing methods have discovered a whole range of bacterial taxa that are associated with ticks. Some studies found differences in bacterial genera contributing to the tick microbiome between geographical locations, tick species and stages (nymphs, female, and males) (Van Treuren et al., 2015), but others did not (Clow et al., 2018). In most studies bacterial genera were found that constitute known tick symbionts like Coxiella, Midichloria, Francisella, Wolbachia, Cardinium, Arsenophonus, Spiroplasma, Rickettsia, Rickettsiella, and Lariskella (Benson et al., 2004; Carpi et al., 2011; Abraham et al., 2017; Bonnet et al., 2017; Mukhacheva and Kovalev, 2017; Swei and Kwan, 2017). Recently in a more refined approach, dissected tick tissues of questing I. scapularis were used to determine what constitutes the “internal” microbiome as opposed to the “surface” microbiome (Ross et al., 2018). This showed that the gut microbiome of I. scapularis was of limited diversity in the majority of adults being dominated by Rickettsia and B. burgdorferi s.s. Only a minority of tick samples contained high microbiome diversity, harboring bacteria of the genera Bacillus and Pseudomonas, and the family Enterobacteriaceae in their midguts.
The distribution of microbial symbionts, in hybrid ticks in the overlapping zone of I. ricinus and I. persulcatus was very interesting. The endosymbiont Midichloria mitochondrii (Sassera et al., 2006) was found to 100% in female I. ricinus ticks. A different endosymbiont, Lariskella arthropodarum, was identified in I. persulcatus (Mediannikov et al., 2004; Mukhacheva and Kovalev, 2017). Hybrid progeny of these ticks harbored only the respective symbionts of their mothers underpinning the specificity of association (Mukhacheva and Kovalev, 2017).
As pointed out below, hybrid offspring of different tick vector species such as I. ricinus and I. persulcatus in regions where these species occur in sympatry may offer an opportunity for vector switching. Such hybrid ticks will have genetic inheritance from both parents and receptor molecules in the midgut or molecules of the immune system may phenotypically represent both parents, which we speculate might allow the survival of an invading Borrelia species and facilitate its adaptation to a new vector.
Possible Mechanisms of Vector Adaptation
We suggest that B. bavariensis is an excellent model in which to examine the underlying mechanisms of invasion-like geographic range expansion of vector-transmitted prokaryotic parasites including vector-switching and the identities of genes underlying vector-adaptation.
Coincident with their colonization of Europe, it appears that Asian B. bavariensis strains that had previously been associated exclusively with the tick I. persulcatus acquired the ability to use I. ricinus as a vector. This may be because the two ixodid ticks had been previously kept apart by a landscape or climatic barrier, perhaps associated with the extensive glaciation of Northern Europe. On the removal of this barrier, adaptation to the new vector would have become possible. But it may also be the case that the distributions of the two tick species had always overlapped to some extent, and that utilization by Asian B. bavariensis of I. ricinus was prevented by the lack of suitable adaptation. The existence of a marked genetic bottleneck in the European population of B. bavariensis certainly argues that adaptation to the new vector was not easily accomplished, and indeed may have taken place on only a single occasion.
In case of a barrier between tick species, the simplest hypothesis would be that I. ricinus constituted an unrealized (because unavailable) dimension within the Hutchinsonian niche of B. bavariensis; this tick would now have revealed itself to be a potential vector, and that no adaptation was necessary to allow B. bavariensis to colonize the new tick species. This then allowed the spirochete to invade the whole of the geographic range of I. ricinus.
But such an evolution-free process does not accord well with the evidence: without the action of natural selection, the realization of the previously unoccupied I. ricinus part of the B. bavariensis fundamental niche would have left no genomic signature, and the extensive genetic variation present within the original Asian population of B. bavariensis would have continued to exist within the strains that now invaded Europe.
In fact, as we have seen, invasion of Europe by B. bavariensis was accompanied by intense genome-wide selection. The resulting population bottleneck led to the massive expansion of a population of closely related spirochetes that contained very little genetic variation (Figure 4). Two hypothetical explanations for such an event (not necessarily mutually exclusive) can be considered.
One of these explanations is that there was a pre-existing clade within the Asian population of B. bavariensis, which in addition to its previous association with I. persulcatus was (unlike the rest of the population) to at least some extent genetically pre-adapted to utilize I. ricinus as a vector; on encountering I. ricinus for the first time, simple population dynamic processes would have led naturally to the expansion of such a clade. But if this were the case, then why did such a pre-adaptation exist, and what maintained it within a population that had never previously come into contact with I. ricinus? Moreover, if the selective sweep that so strongly shaped the genome of the clade that invaded Europe was not the result of selection imposed by the vector switch itself, why did this pre-adapted clade contain so little genetic variation? The only possibility is that colonization of I. ricinus was made possible by an evolutionary event that was the coincidental consequence of an unrelated selective force and which did impose the selective sweep for unrelated reasons. But we suggest that under this scenario it is very hard to account for the very strong genetic bottleneck evidently experienced during vector–switching in B. bavariensis.
The alternative is that no such pre-adaptation was present, and that utilization of I. ricinus as a vector required the recruitment of one or more new genetic variants. We now consider two different scenarios for the acquisition of these adaptive genes.
Competition or Collaboration?
Our first suggestion is that the vector-switch required a mixed infection within a tick between B. bavariensis and some other Borrelia species that was already adapted to use I. ricinus as a vector.
As noted above, different Borrelia species can be found in the same Ixodes tick in mixed infections; the different spirochetes may have been acquired by the tick together at the same time when feeding from a multiply-parasitized host. Whilst standard niche theory would predict that competition between the parasite species would occur in such a scenario, it might also be envisaged that both species may benefit from the secretion by just one of the two species of adaptive “public goods” goods (i.e., secreted proteins that are beneficial to both parasites under these conditions). We note that the evolutionary outcomes of such interactions between parasites in multiple infections may be complex (Alizon and Lion, 2011).
Thus, a simple model for Borrelia adaptation to a new vector would be that co-infection of a single tick with a Borrelia species already adapted to transmission by I. ricinus (e.g., B. garinii, B. afzelii) would enable not only that species, but also B. bavariensis to attach to the midgut of the new tick vector, and/or to be protected against immune attack of the new vector, and/or to be successfully transmitted by the new vector to the reservoir host (Figure 6). This would have enabled B. bavariensis to colonize the new vector, despite lacking the necessary adaptive genes within its own genome. This would not in itself have been enough to make a full transition to the new vector. Importantly, however, the opportunity for B. bavariensis to exploit adaptive genes resident in another Borrelia species during mixed infections in this way would also provide an opportunity whereby those same genes could eventually be acquired by the invading Borrelia strain. This could occur via horizontal gene transfer (HGT) from the other co-infecting species of Borrelia. Phylogenomic studies of Borrelia plasmids have revealed that HGT is common for genes on some plasmids (Qiu et al., 2004; Casjens et al., 2012, 2017, 2018).
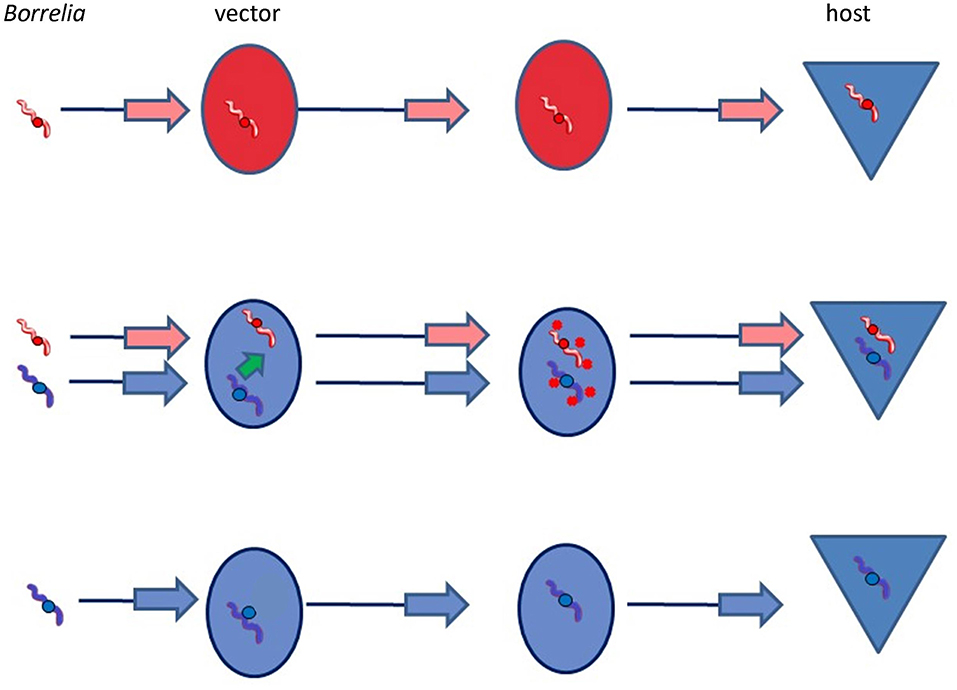
Figure 6. Public goods vector adaptation hypothesis. In this scenario, two Borrelia species that are present in the same vector tick may both benefit from molecules that are secreted by the species that is adapted to this vector species. These molecules may protect both species against adverse conditions encountered during the transmission process in the vector. However, for adaptation to the new vector to be accomplished, appropriate genes permitting adaptation need ultimately to be acquired.
Although it is likely that several genes and gene products are involved in the stepwise adaptation of Borrelia spirochetes to their journey through the vector tick, it should be remembered that every Borrelia species is already well-adapted to utilize at least one species of tick. It is thus conceivable that only one of these steps would actually be deficient in the interaction between B. bavariensis and I. ricinus, much improving the probability that co-infection with another already-adapted Borrelia species would enable B. bavariensis to colonize and exploit the new vector species. Since the acquisition of a single new gene by HGT could theoretically confer the ability to colonize a new vector species with the help of a co-infecting agent, the probability of the evolution of a complete vector switch would also be enhanced. If the necessary HGT event was rare, then this would explain why all B. bavariensis strains that utilize I. ricinus as a vector also show evidence of a historic selective sweep.
Hybrid Ticks?
Our second, speculative suggestion is that adaptation to a new vector required the presence of hybrid I. persulcatus-I. ricinus ticks that allowed the parental strain of B. bavariensis to complete its life cycle. Although the coexistence of the two tick vector species in the Baltic/Western Russian zone would have led to the continually repeated introduction of Asian-type B. bavariensis into I. ricinus ticks, these spirochetes may have been unable to survive in the tick, or (just as likely) were unable successfully to enter the tick's salivary glands and thus to be transferred into the small mammal reservoir hosts utilized by I. ricinus.
The two tick species I. ricinus and I. persulcatus have an overlapping zone in Estonia, Latvia and Western Russia (Figure 5; Swanson et al., 2006). In such overlapping zones cross-species mating occurs, leading to hybrid offspring that likely possess phenotypic traits of both parents. Cross-mating has not only observed between I. ricinus and I. persulcatus but also between I. persulcatus and I. pavlovskyi (Balashov Iu et al., 1998; and references therein Kovalev et al., 2015, 2016) and between I. scapularis and I. cookei in North America (Patterson et al., 2017) suggesting that cross-mating between tick species may be more frequent than once thought.
The presence of hybrid ticks might be a predisposing factor for Borrelia vector -switching. In the Eurasian I. ricinus-I. persulcatus hybrid zone, up to 13% of ticks have been found to be hybrids (Kovalev et al., 2016). Such hybrids presumably arise afresh in each generation through cross-species mating, but it is also possible that hybrids might themselves cross with either parental species for a limited number of generations (Kovalev et al., 2016). Although it has been considered that hybrid offspring produced under laboratory conditions are sterile (Bugmyrin et al., 2015, 2016) the introgression of genes in natural populations suggests that hybrid offspring of these two tick species are at least sometimes fertile (Kovalev et al., 2016). Whether or not hybrid ticks have appreciable fertility might not matter much as far as vector-switching goes, since even sterile hybrid ticks can probably transmit Borrelia.
The significance of tick hybridization for vector switching is that it would allow “mixed” infections to occur in which Borrelia specifically adapted to one tick species can come into intimate contact with another Borrelia species specifically adapted to a different tick vector. This would permit the exchange of bacterial genes conferring colonizing ability between the two Borrelia species, which are not normally able to utilize the “wrong” host (as is the case with B. afzelii adapted to I. ricinus, and Asian populations of B. bavariensis adapted to I. persulcatus) This is illustrated in Figure 7.
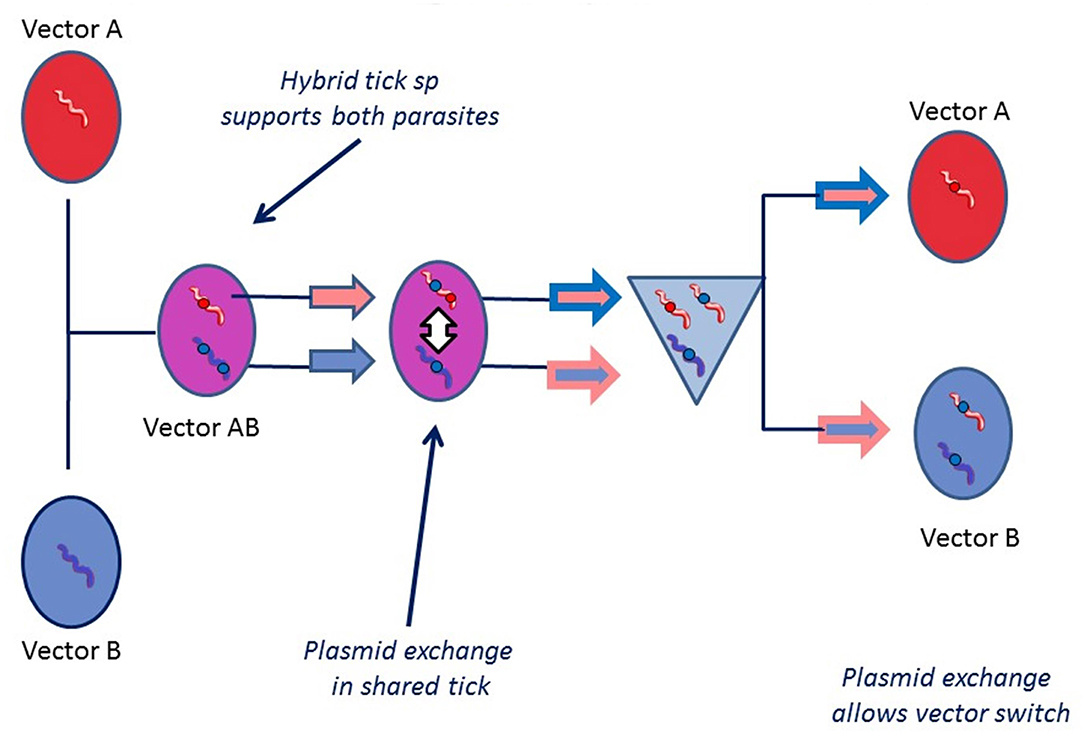
Figure 7. Plasmid exchange vector adaptation hypothesis. This hypothesis supposes that bacterial plasmid exchange may take place inside a vector tick that harbors two different Borrelia species. It may be particularly easy for a Borrelia to adapt to a new environment in a hybrid tick because it will express midgut receptor or immune related proteins from both parents. For plasmid exchange to happen, it is necessary for both species to be present in the same place and at the same time. Even if one of the species is killed (if the tick feeds on a non-permissive host for one of the species), plasmid transfer may still happen. If an Asian strain of B. bavariensis (normally dependent on I. persulcatus as a vector) acquired one or more plasmids from a species of spirochete, for example B. afzelii, that enabled it to colonize the European tick I. ricinus, then the vector switch would be achieved.
Conclusion
In this review we have described ecological factors that are important for geographic invasion by tick-borne bacterial pathogens. To identify factors that make tick-borne parasites potentially “invasive,” we focused on the medically important tick-borne bacteria, the Lyme borreliosis group of spirochetes (reportedly the most frequent source of arthropod-borne human infection in the northern hemisphere, e.g., Bacon et al., 2008; Medlock et al., 2013). We explored the ecological niche concept to explain the importance of hosts and vectors in limiting geographic ranges of these bacteria.
We scrutinized data reporting on vector interactions by the Lyme borreliosis spirochete and identify B. bavariensis as a paradigm case of geographical invasion of a tick-borne pathogen. In doing so, we provide a new perspective as we identify differential vector competence as one important factor for invasion into novel geographic regions by tick-borne pathogens. Our discussion highlights significant trends and areas for potential future research such as:
➢ Obtaining direct experimental evidence for vector competence of I. ricinus and I. persulcatus for the respective B. bavariensis populations through transmission studies.
➢ Different clinical manifestations have been reported from Asia and Europe (Wilske et al., 1993, 1996; Takano et al., 2011). Targeted clinical studies could reveal whether the vector switch may have had an impact on pathogenicity or tissue tropism of B. bavariensis.
➢ NGS with long read methods to allow proper assembly of plasmids in B. bavariensis and B. garinii. So far there is only one B. garinii isolate with the completed set of plasmids. There are two Asian B. bavariensis for which plasmids are available in BorreliaBase.org. For European B. bavariensis we have sequenced two whole genomes using long-read methods permitting the complete assembly of all plasmids but undoubtedly several other genomes need to be sequenced to get better ideas of plasmid presence/absence, plasmid structure.
➢ Comparative analysis of whole genomes including the whole array of plasmids.
Molecular genomics has made major advances in recent years and Ixodes genomes are now being sequenced, and our review points to a research area that will be important in providing new insights in vector-parasite interactions which can be used for developing new prophylactic strategies and for scarce public health resources to be allocated with maximal effect.
Author Contributions
GM and VF experts in tick-borne pathogens, manuscript conceptualization, literature search, and writing of manuscript. SR expert in evolution and ecology, manuscript conceptualization, literature search, and writing of manuscript.
Conflict of Interest
The authors declare that the research was conducted in the absence of any commercial or financial relationships that could be construed as a potential conflict of interest.
The reviewer, SM, declared a past collaboration with one of the authors, GM, to the handling editor.
Acknowledgments
The authors gratefully acknowledge Sabrina Hepner and Durdica Marosevic for useful comments on the manuscript. The final version benefitted from the constructive criticism of two anonymous referees. The National Referenz Centre for Borrelia at the Bavarian Health and Food Safety Authority, Oberschleissheim, Germany is funded by the Robert-Koch-Institute, Berlin, Germany.
References
Abraham, N. M., Liu, L., Jutras, B. L., Murfin, K., Acar, A., Yarovinsky, T. O., et al. (2017). A tick antivirulence protein potentiates antibiotics against Staphylococcus aureus. Antimicrob. Agents Chemother. 61:e00113–17. doi: 10.1128/AAC.00113-17
Alekseev, A. N., Dubinina, H. V., Antykova, L. P., Dzhivanyan, T. I., Rijpkema, S. G., Kruif, N. V., et al. (1998). Tick-borne borrelioses pathogen identification in Ixodes ticks (Acarina, Ixodidae) collected in St. Petersburg and Kaliningrad Baltic regions of Russia. J. Med. Entomol. 35, 136–142. doi: 10.1093/jmedent/35.2.136
Alizon, S., and Lion, S. (2011). Within-host parasite cooperation and the evolution of virulence. Proc. Biol. Sci. 278, 3738–3747. doi: 10.1098/rspb.2011.0471
Auld, S. K., and Tinsley, M. C. (2015). The evolutionary ecology of complex lifecycle parasites: linking phenomena with mechanisms. Heredity 114, 125–132. doi: 10.1038/hdy.2014.84
Bacon, R. M., Kugeler, K. J., and Mead, P. S. (2008). Surveillance for Lyme disease–United States, 1992–2006. MMWR Surveill. Summ. 57, 1–9.
Balashov Iu, S., Grigor'eva, L. A., and Oliver, J. (1998). [Interspecies cross of ixodes ticks from the group Ixodes ricinus-persulcatus]. Dokl. Akad. Nauk 361, 712–714.
Barbour, A. G., and Fish, D. (1993). The biological and social phenomenon of Lyme disease. Science 260, 1610–1616. doi: 10.1126/science.8503006
Becker, N. S., Margos, G., Blum, H., Krebs, S., Graf, A., Lane, R. S., et al. (2016). Recurrent evolution of host and vector association in bacteria of the Borrelia burgdorferi sensu lato species complex. BMC Genomics 17:734. doi: 10.1186/s12864-016-3016-4
Beerntsen, B. T., James, A. A., and Christensen, B. M. (2000). Genetics of mosquito vector competence. Microbiol. Mol. Biol. Rev. 64, 115–137. doi: 10.1128/MMBR.64.1.115-137.2000
Benson, M. J., Gawronski, J. D., Eveleigh, D. E., and Benson, D. R. (2004). Intracellular symbionts and other bacteria associated with deer ticks (Ixodes scapularis) from Nantucket and Wellfleet, Cape Cod, Massachusetts. Appl. Environ. Microbiol. 70, 616–620. doi: 10.1128/AEM.70.1.616-620.2004
Bonnet, S. I., Binetruy, F., Hernandez-Jarguin, A. M., and Duron, O. (2017). The tick microbiome: why non-pathogenic microorganisms matter in tick biology and pathogen transmission. Front. Cell. Infect. Microbiol. 7:236. doi: 10.3389/fcimb.2017.00236
Bormane, A., Lucenko, I., Duks, A., Mavtchoutko, V., Ranka, R., Salmina, K., et al. (2004). Vectors of tick-borne diseases and epidemiological situation in Latvia in 1993-2002. Int. J. Med. Microbiol. 293(Suppl. 37), 36–47. doi: 10.1016/S1433-1128(04)80007-X
Brandt, K. S., Patton, T. G., Allard, A. S., Caimano, M. J., Radolf, J. D., and Gilmore, R. D. (2014). Evaluation of the Borrelia burgdorferi BBA64 protein as a protective immunogen in mice. Clin. Vaccine Immunol. 21, 526–533. doi: 10.1128/CVI.00824-13
Bugmyrin, S. V., Belova, O. A., Bespyatova, L. A., Ieshko, E. P., and Karganova, G. G. (2016). Morphological features of Ixodes persulcatus and I. ricinus hybrids: nymphs and adults. Exp. Appl. Acarol. 69, 359–369. doi: 10.1007/s10493-016-0036-3
Bugmyrin, S. V., Belova, O. A., Ieshko, E. P., Bespyatova, L. A., and Karganova, G. G. (2015). Morphological differentiation of Ixodes persulcatus and I. ricinus hybrid larvae in experiment and under natural conditions. Ticks Tick Borne Dis. 6, 129–133. doi: 10.1016/j.ttbdis.2014.11.001
Burgdorfer, W., Barbour, A. G., Hayes, S. F., Benach, J. L., Grunwaldt, E., and Davis, J. P. (1982). Lyme disease-a tick-borne spirochetosis? Science 216, 1317–1319. doi: 10.1126/science.7043737
Caimano, M. J., Groshong, A. M., Belperron, A., Mao, J., Hawley, K. L., Luthra, A., et al. (2019). The RpoS gatekeeper in Borrelia burgdorferi: an invariant regulatory scheme that promotes spirochete persistence in reservoir hosts and niche diversity. Front. Microbiol. 10:1923. doi: 10.3389/fmicb.2019.01923
Carpi, G., Cagnacci, F., Wittekindt, N. E., Zhao, F., Qi, J., Tomsho, L. P., et al. (2011). Metagenomic profile of the bacterial communities associated with Ixodes ricinus ticks. PLoS ONE 6:e25604. doi: 10.1371/journal.pone.0025604
Casjens, S. R., Di, L., Akther, S., Mongodin, E. F., Luft, B. J., Schutzer, S. E., et al. (2018). Primordial origin and diversification of plasmids in Lyme disease agent bacteria. BMC Genomics 19:218. doi: 10.1186/s12864-018-4597-x
Casjens, S. R., Gilcrease, E. B., Vujadinovic, M., Mongodin, E. F., Luft, B. J., Schutzer, S. E., et al. (2017). Plasmid diversity and phylogenetic consistency in the Lyme disease agent Borrelia burgdorferi. BMC Genomics 18:165. doi: 10.1186/s12864-017-3553-5
Casjens, S. R., Mongodin, E. F., Qiu, W. G., Luft, B. J., Schutzer, S. E., Gilcrease, E. B., et al. (2012). Genome stability of Lyme disease spirochetes: comparative genomics of Borrelia burgdorferi plasmids. PLoS ONE 7:e33280. doi: 10.1371/journal.pone.0033280
Chalkowski, K., Lepczyk, C. A., and Zohdy, S. (2018). Parasite ecology of invasive species: conceptual framework and new hypotheses. Trends Parasitol. 34, 655–663. doi: 10.1016/j.pt.2018.05.008
Charrel, R. N., Brault, A. C., Gallian, P., Lemasson, J. J., Murgue, B., Murri, S., et al. (2003). Evolutionary relationship between Old World West Nile virus strains. Evidence for viral gene flow between Africa, the Middle East, and Europe. Virology 315, 381–388. doi: 10.1016/S0042-6822(03)00536-1
Clover, J. R., and Lane, R. S. (1995). Evidence implicating nymphal Ixodes pacificus (Acari: ixodidae) in the epidemiology of Lyme disease in California. Am. J. Trop. Med. Hyg. 53, 237–240. doi: 10.4269/ajtmh.1995.53.237
Clow, K. M., Weese, J. S., Rousseau, J., and Jardine, C. M. (2018). Microbiota of field-collected Ixodes scapularis and Dermacentor variabilis from eastern and southern Ontario, Canada. Ticks Tick Borne Dis. 9, 235–244. doi: 10.1016/j.ttbdis.2017.09.009
de la Fuente, J., Antunes, S., Bonnet, S., Cabezas-Cruz, A., Domingos, A. G., Estrada-Pena, A., et al. (2017). Tick-pathogen interactions and vector competence: identification of molecular drivers for tick-borne diseases. Front. Cell. Infect. Microbiol. 7:114. doi: 10.3389/fcimb.2017.00114
Delatte, H., Bagny, L., Brengue, C., Bouetard, A., Paupy, C., and Fontenille, D. (2011). The invaders: phylogeography of dengue and chikungunya viruses Aedes vectors, on the South West islands of the Indian Ocean. Infect. Genet. Evol. 11, 1769–1781. doi: 10.1016/j.meegid.2011.07.016
Dolan, M. C., Lacombe, E. H., and Piesman, J. (2000). Vector competence of Ixodes muris (Acari: Ixodidae) for Borrelia burgdorferi. J. Med. Entomol. 37, 766–768. doi: 10.1603/0022-2585-37.5.766
Dolan, M. C., Maupin, G. O., Panella, N. A., Golde, W. T., and Piesman, J. (1997). Vector competence of Ixodes scapularis, I. spinipalpis, and Dermacentor andersoni (Acari:Ixodidae) in transmitting Borrelia burgdorferi, the etiologic agent of Lyme disease. J. Med. Entomol. 34, 128–135. doi: 10.1093/jmedent/34.2.128
Dolan, M. C., Piesman, J., Mbow, M. L., Maupin, G. O., Peter, O., Brossard, M., et al. (1998). Vector competence of Ixodes scapularis and Ixodes ricinus (Acari: Ixodidae) for three genospecies of Borrelia burgdorferi. J. Med. Entomol. 35, 465–470. doi: 10.1093/jmedent/35.4.465
Dunn, A. M. (2009). Parasites and biological invasions. Adv. Parasitol. 68, 161–184. doi: 10.1016/S0065-308X(08)00607-6
Edgerton, B. F., Henttonen, P., Jussila, J., Mannonen, A., Paasonen, P., Taugbol, T., et al. (2004). Understanding the causes of disease in European freshwater crayfish. Conserv. Biol. 18, 1466–1474. doi: 10.1111/j.1523-1739.2004.00436.x
Eisen, L., Dolan, M. C., Piesman, J., and Lane, R. S. (2003). Vector competence of Ixodes pacificus and I. spinipalpis (Acari: Ixodidae), and reservoir competence of the dusky-footed woodrat (Neotoma fuscipes) and the deer mouse (Peromyscus maniculatus), for Borrelia bissettii. J. Med. Entomol. 40, 311–320. doi: 10.1603/0022-2585-40.3.311
Eisen, L., and Lane, R. S. (2002.). “Vectors of Borrelia burgdorferi sensu lato,” in Lyme Borreliosis: Biology, Epidemiology Control, eds J. Gray, O. Kahl, R. S. Lane, G. Stanek (Wallingford: CABI Publishing), 91–115. doi: 10.1079/9780851996325.0091
Eisen, R. J., Eisen, L., Ogden, N. H., and Beard, C. B. (2016). Linkages of Weather and Climate With Ixodes scapularis and Ixodes pacificus (Acari: Ixodidae), Enzootic Transmission of Borrelia burgdorferi, and Lyme Disease in North America. J. Med. Entomol. 53, 250–261. doi: 10.1093/jme/tjv199
Eisenhauer, N., Schulz, W., Scheu, S., and Jousset, A. (2013). Niche dimensionality links biodiversity and invasibility of microbial communities. Funct. Ecol. 27, 282–288. doi: 10.1111/j.1365-2435.2012.02060.x
Fedorova, N., Kleinjan, J. E., James, D., Hui, L. T., Peeters, H., and Lane, R. S. (2014). Remarkable diversity of tick or mammalian-associated Borreliae in the metropolitan San Francisco Bay Area, California. Ticks Tick Borne Dis. 5, 951–961. doi: 10.1016/j.ttbdis.2014.07.015
Fikrig, E., Feng, W., Barthold, S. W., Telford, S. R. III., and Flavell, R. A. (2000). Arthropod- and host-specific Borrelia burgdorferi bbk32 expression and the inhibition of spirochete transmission. J. Immunol. 164, 5344–5351. doi: 10.4049/jimmunol.164.10.5344
Filipova, N. A. (2017). History of the species range of ixodid ticks, vectors of pathogens with natural nidality (Acari, Ixodidae), as a prerequisite of their intraspecific biodiversity. Entomol. Rev. 97, 255–275. doi: 10.1134/S0013873817020117
Fingerle, V., Schulte-Spechtel, U. C., Ruzic-Sabljic, E., Leonhard, S., Hofmann, H., Weber, K., et al. (2008). Epidemiological aspects and molecular characterization of Borrelia burgdorferi s.l. from southern Germany with special respect to the new species Borrelia spielmanii sp. nov. Int. J. Med. Microbiol. 298, 279–290. doi: 10.1016/j.ijmm.2007.05.002
Fritz, M., Taty, R. T., Portella, C., Guimbi, C., Mankou, M., Leroy, E. M., et al. (2019). Re-emergence of chikungunya in the Republic of the Congo in 2019 associated with a possible vector-host switch. Int. J. Infect. Dis. 84, 99–101. doi: 10.1016/j.ijid.2019.05.013
Gaston, K. J. (2003). The Structure and Dynamics of Geographic Ranges. Oxford: Oxford University Press.
Gatewood, A. G., Liebman, K. A., Vourc'h, G., Bunikis, J., Hamer, S. A., Cortinas, M. R., et al. (2009). Climate and tick seasonality predict Borrelia burgdorferi genotype distribution. Appl. Environ. Microbiol. 75, 2476–2483. doi: 10.1128/AEM.02633-08
Gatzmann, F., Metzler, D., Krebs, S., Blum, H., Sing, A., Takano, A., et al. (2015). NGS population genetics analyses reveal divergent evolution of a Lyme Borreliosis agent in Europe and Asia. Ticks Tick Borne Dis. 6, 344–351. doi: 10.1016/j.ttbdis.2015.02.008
Geller, J., Nazarova, L., Katargina, O., and Golovljova, I. (2013). Borrelia burgdorferi sensu lato prevalence in tick populations in Estonia. Parasit. Vectors 6:202. doi: 10.1186/1756-3305-6-202
Gern, L., Estrada-Pena, A., Frandsen, F., Gray, J. S., Jaenson, T. G., Jongejan, F., et al. (1998). European reservoir hosts of Borrelia burgdorferi sensu lato. Zentralbl. Bakteriol. 287, 196–204. doi: 10.1016/S0934-8840(98)80121-7
Gern, L., and Humair, P. (2002). “Ecology of Borrelia burgdorferi sensu lato in Europe,” in Lyme Borreliosis: Biology, Epidemiology and Control, eds J. S. Gray, O. Kahl, R. S. Lane, and G. Stanek (Wallingford: CABI Publishing), 149–174. doi: 10.1079/9780851996325.0149
Granot, I., Shenkar, N., and Belmaker, J. (2017). Habitat niche breadth predicts invasiveness in solitary ascidians. Ecol. Evol. 7, 7838–7847. doi: 10.1002/ece3.3351
Grinnell, J. (1917). The niche-relationships of the California Thrasher. Auk 34, 427–433. doi: 10.2307/4072271
Hajdusek, O., Sima, R., Ayllon, N., Jalovecka, M., Perner, J., de la Fuente, J., et al. (2013). Interaction of the tick immune system with transmitted pathogens. Front. Cell. Infect. Microbiol. 3:26. doi: 10.3389/fcimb.2013.00026
Hamer, S. A., Roy, P. L., Hickling, G. J., Walker, E. D., Foster, E. S., Barber, C. C., et al. (2007). Zoonotic pathogens in Ixodes scapularis, Michigan. Emerging Infect. Dis. 13, 1131–1133. doi: 10.3201/eid1307.070046
Hartemink, N. A., Randolph, S. E., Davis, S. A., and Heesterbeek, J. A. (2008). The basic reproduction number for complex disease systems: defining R(0) for tick-borne infections. Am. Nat. 171, 743–754. doi: 10.1086/587530
Hatcher, M. J., Dick, J. T., and Dunn, A. M. (2006). How parasites affect interactions between competitors and predators. Ecol. Lett. 9, 1253–1271. doi: 10.1111/j.1461-0248.2006.00964.x
Hatcher, M. J., Dick, J. T. A., and Dunn, A. M. (2012). Disease emergence and invasions. Funct. Ecol. 26, 1275–1287. doi: 10.1111/j.1365-2435.2012.02031.x
Hoen, A. G., Margos, G., Bent, S. J., Kurtenbach, K., and Fish, D. (2009). Phylogeography of Borrelia burgdorferi in the eastern United States reflects multiple independent Lyme disease emergence events. Proc. Natl. Acad. Sci. U.S.A. 106, 15013–15018. doi: 10.1073/pnas.0903810106
Hoffmann, B. D., and Courchamp, F. (2016). Biological invasions and natural colonisations: are they that different? Neobiota 29, 1–14. doi: 10.3897/neobiota.29.6959
Hu, C. M., Wilske, B., Fingerle, V., Lobet, Y., and Gern, L. (2001). Transmission of Borrelia garinii OspA serotype 4 to BALB/c mice by Ixodes ricinus ticks collected in the field. J. Clin. Microbiol. 39, 1169–1171. doi: 10.1128/JCM.39.3.1169-1171.2001
Huegli, D., Hu, C. M., Humair, P. F., Wilske, B., and Gern, L. (2002). Apodemus species mice are reservoir hosts of Borrelia garinii OspA serotype 4 in Switzerland. J. Clin. Microbiol. 40, 4735–4737. doi: 10.1128/JCM.40.12.4735-4737.2002
Hutchinson, G. E. (1957). Population studies - animal ecology and demography - concluding remarks. Cold Spring Harb. Symp. Quant. Biol. 22, 415–427. doi: 10.1101/SQB.1957.022.01.039
Iglesias, R., Garcia-Estevez, J. M., Ayres, C., Acuna, A., and Cordero-Rivera, A. (2015). First reported outbreak of severe spirorchiidiasis in Emys orbicularis, probably resulting from a parasite spillover event. Dis. Aquat. Org. 113, 75–80. doi: 10.3354/dao02812
Ivanova, L. B., Tomova, A., Gonzalez-Acuna, D., Murua, R., Moreno, C. X., Hernandez, C., et al. (2014). Borrelia chilensis, a new member of the Borrelia burgdorferi sensu lato complex that extends the range of this genospecies in the Southern Hemisphere. Environ. Microbiol. 16, 1069–1080. doi: 10.1111/1462-2920.12310
Iyer, R., Caimano, M. J., Luthra, A., Axline, D. Jr., Corona, A., Iacobas, D. A., et al. (2015). Stage-specific global alterations in the transcriptomes of Lyme disease spirochetes during tick feeding and following mammalian host adaptation. Mol. Microbiol. 95, 509–538. doi: 10.1111/mmi.12882
Jacquot, M., Gonnet, M., Ferquel, E., Abrial, D., Claude, A., Gasqui, P., et al. (2014). Comparative population genomics of the Borrelia burgdorferi species complex reveals high degree of genetic isolation among species and underscores benefits and constraints to studying intra-specific epidemiological processes. PLoS ONE 9:e94384. doi: 10.1371/journal.pone.0094384
Jahner, J. P., Bonilla, M. M., Badik, K. J., Shapiro, A. M., and Forister, M. L. (2011). Use of exotic hosts by Lepidoptera: widespread species colonize more novel hosts. Evolution 65, 2719–2724. doi: 10.1111/j.1558-5646.2011.01310.x
Johns, R., Ohnishi, J., Broadwater, A., Sonenshine, D. E., De Silva, A. M., and Hynes, W. L. (2001). Contrasts in tick innate immune responses to Borrelia burgdorferi challenge: immunotolerance in Ixodes scapularis versus immunocompetence in Dermacentor variabilis (Acari: Ixodidae). J. Med. Entomol. 38, 99–107. doi: 10.1603/0022-2585-38.1.99
Juhasova, L., Kralova-Hromadova, I., Bazsalovicsova, E., Minarik, G., Stefka, J., Mikulicek, P., et al. (2016). Population structure and dispersal routes of an invasive parasite, Fascioloides magna, in North America and Europe. Parasit. Vectors 9:547. doi: 10.1186/s13071-016-1811-z
Kilpatrick, A. M. (2011). Globalization land use, and the invasion of West Nile virus. Science 334, 323–327. doi: 10.1126/science.1201010
Kilpatrick, A. M., Dobson, A. D. M., Levi, T., Salkeld, D. J., Swei, A., Ginsberg, H. S., et al. (2017). Lyme disease ecology in a changing world: consensus, uncertainty and critical gaps for improving control. Philos. Trans. R. Soc. Lond. B. Biol. Sci. 372:20160117. doi: 10.1098/rstb.2016.0117
Kinnunen, M., Dechesne, A., Proctor, C., Hammes, F., Johnson, D., Quintela-Baluja, M., et al. (2016). A conceptual framework for invasion in microbial communities. ISME J. 10, 2773–2775. doi: 10.1038/ismej.2016.75
Kitsou, C., and Pal, U. (2018). Ixodes immune responses against lyme disease pathogens. Front. Cell. Infect. Microbiol. 8:176. doi: 10.3389/fcimb.2018.00176
Koci, J., Bernard, Q., Yang, X., and Pal, U. (2018). Borrelia burgdorferi surface protein Lmp1 facilitates pathogen dissemination through ticks as studied by an artificial membrane feeding system. Sci. Rep. 8:1910. doi: 10.1038/s41598-018-20208-4
Korenberg, E. (1995). “Ixodid tick-borne borrelioses (ITBBs) infections of the Lyme borreliosis group in Russia: country report,” in Reports of WHO Workshop on Lyme Borreliosis Diagnosis and Surveillance (Warsaw: WHO/CDC/VPH/95.141-1, 128–136.
Korenberg, E., Kovalevskii, Y. V., Kryuchechnikov, V. N., and Gorelova, N. B. (1991). “Tick Ixodes persulcatus schulze 1930 as a vector of Borrelia burgdorferi,” in Modern Acarology, eds F. Dusbabek and V. Bukva (Prague: Academia, 119–123.
Korenberg, E. I., Gorelova, N. B., and Kovalevskii, Y. V. (2002). “Ecology of Borrelia burgdorferi sensu lato in Russia,” in Lyme borreliosis: Biology, Epidemiology and Control, eds J. Gray, O. Kahl, R. S. Lane, and G. Stanek (Wallingford: CABI Publishing), 175–200.
Kovalev, S. Y., Golovljova, I. V., and Mukhacheva, T. A. (2016). Natural hybridization between Ixodes ricinus and Ixodes persulcatus ticks evidenced by molecular genetics methods. Ticks Tick Borne Dis. 7, 113–118. doi: 10.1016/j.ttbdis.2015.09.005
Kovalev, S. Y., Mikhaylishcheva, M. S., and Mukhacheva, T. A. (2015). Natural hybridization of the ticks Ixodes persulcatus and Ixodes pavlovskyi in their sympatric populations in Western Siberia. Infect. Genet. Evol. 32, 388–395. doi: 10.1016/j.meegid.2015.04.003
Kurilshikov, A. M., Fomenko, N. V., Stronin, O. V., Tikunov, A. Y., Kabilov, M. R., Tupikin, A. E., et al. (2014). Complete genome sequencing of Borrelia valaisiana and Borrelia afzelii isolated from Ixodes persulcatus ticks in Western Siberia. Genome Announc. 2:e01315–14. doi: 10.1128/genomeA.01315-14
Kurtenbach, K., Hanincova, K., Tsao, J. I., Margos, G., Fish, D., and Ogden, N. H. (2006). Fundamental processes in the evolutionary ecology of Lyme borreliosis. Nat. Rev. Microbiol. 4, 660–669. doi: 10.1038/nrmicro1475
Kurtenbach, K., Hoen, A. G., Bent, S. J., Vollmer, S. A., Ogden, N. H., and Margos, G. (2010). “Population biology of lyme Borreliosis spirochetes,” in Bacterial Population Genetics in Infectious Disease, eds D. A. Robinson, D. Falush, and E. J. Feil (Hoboken, NJ: John Wiley & Sons, Inc), 217–245. doi: 10.1002/9780470600122.ch12
Kurtenbach, K., Sewell, H. S., Ogden, N. H., Randolph, S. E., and Nuttall, P. A. (1998). Serum complement sensitivity as a key factor in Lyme disease ecology. Infect. Immun. 66, 1248–1251.
Lane, R. S., Brown, R. N., Piesman, J., and Peavey, C. A. (1994). Vector competence of Ixodes pacificus and Dermacentor occidentalis (Acari: Ixodidae) for various isolates of Lyme disease spirochetes. J. Med. Entomol. 31, 417–424. doi: 10.1093/jmedent/31.3.417
Lee, C. E., and Gelembiuk, G. W. (2008). Evolutionary origins of invasive populations. Evol. Appl. 1, 427–448. doi: 10.1111/j.1752-4571.2008.00039.x
Lehane, M. J., Aksoy, S., and Levashina, E. (2004). Immune responses and parasite transmission in blood-feeding insects. Trends Parasitol. 20, 433–439. doi: 10.1016/j.pt.2004.07.002
Li, Y., Zhang, X. X., Mao, R. L., Yang, J., Miao, C. Y., Li, Z., et al. (2017). Ten years of landscape genomics: challenges and opportunities. Front. Plant Sci. 8:2136. doi: 10.3389/fpls.2017.02136
Lindgren, E., and Jaenson, T. G. T. (2006). Lyme Borreliosis in Europe: Influences of Climate and Climate Change, Epidemiology, Ecology and Adaptation Measures. Available online at: http://www.euro.who.int/__data/assets/pdf_file/0006/96819/E89522.pdf
Ling, J., Smura, T., Lundstrom, J. O., Pettersson, J. H., Sironen, T., Vapalahti, O., et al. (2019). Introduction and dispersal of sindbis virus from Central Africa to Europe. J. Virol. 93:e00620–19. doi: 10.1128/JVI.00620-19
Longdon, B., Day, J. P., Alves, J. M., Smith, S. C. L., Houslay, T. M., McGonigle, J. E., et al. (2018). Host shifts result in parallel genetic changes when viruses evolve in closely related species. PLoS Pathog. 14:e1006951. doi: 10.1371/journal.ppat.1006951
Maggi, R. G., Reichelt, S., Toliver, M., and Engber, B. (2010). Borrelia species in Ixodes affinis and Ixodes scapularis ticks collected from the coastal plain of North Carolina. Ticks Tick Borne Dis. 1, 168–171. doi: 10.1016/j.ttbdis.2010.08.003
Mallon, C. A., Elsas, J. D. V., and Salles, J. F. (2015). Microbial invasions: the process, patterns, and mechanisms. Trends Microbiol. 23, 719–729. doi: 10.1016/j.tim.2015.07.013
Marconi, R. T., Hohenberger, S., Jauris-Heipke, S., Schulte-Spechtel, U., LaVoie, C. P., Rossler, D., et al. (1999). Genetic analysis of Borrelia garinii OspA serotype 4 strains associated with neuroborreliosis: evidence for extensive genetic homogeneity. J. Clin. Microbiol. 37, 3965–3970.
Mardulyn, P., Goffredo, M., Conte, A., Hendrickx, G., Meiswinkel, R., Balenghien, T., et al. (2013). Climate change and the spread of vector-borne diseases: using approximate Bayesian computation to compare invasion scenarios for the bluetongue virus vector Culicoides imicola in Italy. Mol. Ecol. 22, 2456–2466. doi: 10.1111/mec.12264
Margos, G., Castillo-Ramirez, S., and Hoen, A. G. (2012a). Phylogeography of Lyme borreliosis-group spirochetes and methicillin-resistant Staphylococcus aureus. Parasitology 139, 1952–1965. doi: 10.1017/S0031182012000741
Margos, G., Lane, R. S., Fedorova, N., Koloczek, J., Piesman, J., Hojgaard, A., et al. (2016). Borrelia bissettiae sp. nov. and Borrelia californiensis sp. nov. prevail in diverse enzootic transmission cycles. Int. J. Syst. Evol. Microbiol. 66, 1447–1452. doi: 10.1099/ijsem.0.000897
Margos, G., Tsao, J. I., Castillo-Ramirez, S., Girard, Y. A., Hamer, S. A., Hoen, A. G., et al. (2012b). Two boundaries separate Borrelia burgdorferi populations in North America. Appl. Environ. Microbiol. 78, 6059–6067. doi: 10.1128/AEM.00231-12
Margos, G., Vollmer, S. A., Cornet, M., Garnier, M., Fingerle, V., Wilske, B., et al. (2009). A new Borrelia species defined by multilocus sequence analysis of housekeeping genes. Appl. Environ. Microbiol. 75, 5410–5416. doi: 10.1128/AEM.00116-09
Margos, G., Vollmer, S. A., Ogden, N. H., and Fish, D. (2011). Population genetics, taxonomy, phylogeny and evolution of Borrelia burgdorferi sensu lato. Infect. Genet. Evol. 11, 1545–1563. doi: 10.1016/j.meegid.2011.07.022
Margos, G., Wilske, B., Sing, A., Hizo-Teufel, C., Cao, W. C., Chu, C., et al. (2013). Borrelia bavariensis sp. nov. is widely distributed in Europe and Asia. Int. J. Syst. Evol. Microbiol. 63(Pt 11), 4284–4288. doi: 10.1099/ijs.0.052001-0
Masuzawa, T. (2004). Terrestrial distribution of the Lyme borreliosis agent Borrelia burgdorferi sensu lato in East Asia. Jpn. J. Infect. Dis. 57, 229–235.
Masuzawa, T., Kharitonenkov, I. G., Kadosaka, T., Hashimoto, N., Kudeken, M., Takada, N., et al. (2005). Characterization of Borrelia burgdorferi sensu lato isolated in Moscow province-a sympatric region for Ixodes ricinus and Ixodes persulcatus. Int. J. Med. Microbiol. 294, 455–464. doi: 10.1016/j.ijmm.2004.09.008
Maupin, G. O., Gage, K. L., Piesman, J., Montenieri, J., Sviat, S. L., VanderZanden, L., et al. (1994). Discovery of an enzootic cycle of Borrelia burgdorferi in Neotoma mexicana and Ixodes spinipalpis from northern Colorado, an area where Lyme disease is nonendemic. J. Infect. Dis. 170, 636–643. doi: 10.1093/infdis/170.3.636
Mechai, S., Feil, E. J., Gariepy, T. D., Gregory, T. R., Lindsay, L. R., Millien, V., et al. (2013). Investigation of the population structure of the tick vector of Lyme disease Ixodes scapularis (Acari: Ixodidae) in Canada using mitochondrial cytochrome C oxidase subunit I gene sequences. J. Med. Entomol. 50, 560–570. doi: 10.1603/ME12178
Mechai, S., Margos, G., Feil, E. J., Lindsay, L. R., Michel, P., Kotchi, S. O., et al. (2018). Evidence for an effect of landscape connectivity on Borrelia burgdorferi sensu stricto dispersion in a zone of range expansion. Ticks Tick Borne Dis. 9, 1407–1415. doi: 10.1016/j.ttbdis.2018.07.001
Mediannikov, O., Ivanov, L. I., Nishikawa, M., Saito, R., Sidel'nikov Iu, N., Zdanovskaia, N. I., et al. (2004). [Microorganism “Montezuma” of the order Rickettsiales: the potential causative agent of tick-borne disease in the Far East of Russia]. Zh. Mikrobiol. Epidemiol. Immunobiol. 1, 7–13.
Medlock, J. M., Hansford, K. M., Bormane, A., Derdakova, M., Estrada-Pena, A., George, J. C., et al. (2013). Driving forces for changes in geographical distribution of Ixodes ricinus ticks in Europe. Parasit. Vectors 6:1. doi: 10.1186/1756-3305-6-1
Metsky, H. C., Matranga, C. B., Wohl, S., Schaffner, S. F., Freije, C. A., Winnicki, S. M., et al. (2017). Zika virus evolution and spread in the Americas. Nature 546, 411–415. doi: 10.1038/nature22402
Miyamoto, K., and Masuzawa, T. (2002). “Ecology of Borrelia burgdorferi sensu lato in Japan and East Asia,” in Lyme Borreliosis: Biology, Epidemiology and Control, eds J. Gray, O. Kahl, R. S. Lane, and G. Stanek (Wallingford: CABI Publishing), 201–222. doi: 10.1079/9780851996325.0201
Molina-Cruz, A., Canepa, G. E., and Barillas-Mury, C. (2017). Plasmodium P47: a key gene for malaria transmission by mosquito vectors. Curr. Opin. Microbiol. 40, 168–174. doi: 10.1016/j.mib.2017.11.029
Mukhacheva, T. A., and Kovalev, S. Y. (2013). Multilocus sequence analysis of Borrelia burgdorferi s.l. in Russia. Ticks Tick Borne Dis. 4, 275–279. doi: 10.1016/j.ttbdis.2013.02.004
Mukhacheva, T. A., and Kovalev, S. Y. (2014). Borrelia spirochetes in Russia: genospecies differentiation by real-time PCR. Ticks Tick Borne Dis. 5, 722–726. doi: 10.1016/j.ttbdis.2014.05.016
Mukhacheva, T. A., and Kovalev, S. Y. (2017). Bacteria of the family ‘Candidatus midichloriaceae’ in sympatric zones of Ixodes ticks: genetic evidence for vertical transmission. Microb. Ecol. 74, 185–193. doi: 10.1007/s00248-017-0932-z
Munro, H. J., Ogden, N. H., Lindsay, L. R., Robertson, G. J., Whitney, H., and Lang, A. S. (2017). Evidence for Borrelia bavariensis infections of Ixodes uriae within seabird colonies of the North Atlantic Ocean. Appl. Environ. Microbiol. 83:e01087–17. doi: 10.1128/AEM.01087-17
Narasimhan, S., and Fikrig, E. (2015). Tick microbiome: the force within. Trends Parasitol. 31, 315–323. doi: 10.1016/j.pt.2015.03.010
Narasimhan, S., Schuijt, T. J., Abraham, N. M., Rajeevan, N., Coumou, J., Graham, M., et al. (2017). Modulation of the tick gut milieu by a secreted tick protein favors Borrelia burgdorferi colonization. Nat. Commun. 8:184. doi: 10.1038/s41467-017-00208-0
Ogden, N. H., Bigras-Poulin, M., Hanincova, K., Maarouf, A., O'Callaghan, C. J., and Kurtenbach, K. (2008). Projected effects of climate change on tick phenology and fitness of pathogens transmitted by the North American tick Ixodes scapularis. J. Theor. Biol. 254, 621–632. doi: 10.1016/j.jtbi.2008.06.020
Ogden, N. H., Bouchard, C., Kurtenbach, K., Margos, G., Lindsay, L. R., Trudel, L., et al. (2010). Active and passive surveillance and phylogenetic analysis of Borrelia burgdorferi elucidate the process of Lyme disease risk emergence in Canada. Environ. Health Perspect. 118, 909–914. doi: 10.1289/ehp.0901766
Ogden, N. H., Lindsay, L. R., and Leighton, P. A. (2013a). Predicting the rate of invasion of the agent of Lyme disease Borrelia burgdorferi. J. Appl. Ecol. 50, 510–518. doi: 10.1111/1365-2664.12050
Ogden, N. H., Maarouf, A., Barker, I. K., Bigras-Poulin, M., Lindsay, L. R., Morshed, M. G., et al. (2006). Climate change and the potential for range expansion of the Lyme disease vector Ixodes scapularis in Canada. Int. J. Parasitol. 36, 63–70. doi: 10.1016/j.ijpara.2005.08.016
Ogden, N. H., Mechai, S., and Margos, G. (2013b). Changing geographic ranges of ticks and tick-borne pathogens: drivers, mechanisms and consequences for pathogen diversity. Front. Cell. Infect. Microbiol. 3:46. doi: 10.3389/fcimb.2013.00046
Ogden, N. H., and Tsao, J. I. (2009). Biodiversity and Lyme disease: dilution or amplification? Epidemics 1, 196–206. doi: 10.1016/j.epidem.2009.06.002
Ojaimi, C., Brooks, C., Casjens, S., Rosa, P., Elias, A., Barbour, A., et al. (2003). Profiling of temperature-induced changes in Borrelia burgdorferi gene expression by using whole genome arrays. Infect. Immun. 71, 1689–1705. doi: 10.1128/IAI.71.4.1689-1705.2003
O'Malley, M. A. (2007). The nineteenth century roots of 'everything is everywhere'. Nat. Rev. Microbiol. 5, 647–651. doi: 10.1038/nrmicro1711
Ostfeld, R. S., Canham, C. D., Oggenfuss, K., Winchcombe, R. J., and Keesing, F. (2006). Climate, deer, rodents, and acorns as determinants of variation in lyme-disease risk. PLoS Biol. 4:e145. doi: 10.1371/journal.pbio.0040145
Pal, U., and Fikrig, E. (2010). “Tick interactions,” in Borrelia - Molecular Biology, Host Interaction and Pathogenesis, eds D. S. Samuels and J. Radolf (Poole: Caister Academic Press, 279–298.
Pal, U., Li, X., Wang, T., Montgomery, R. R., Ramamoorthi, N., Desilva, A. M., et al. (2004). TROSPA, an Ixodes scapularis receptor for Borrelia burgdorferi. Cell 119, 457–468. doi: 10.1016/j.cell.2004.10.027
Patterson, J. W., Duncan, A., McIntyre, K. C., and Lloyd, V. K. (2017). Evidence for genetic hybridization between Ixodes scapularis and Ixodes cookei. Can. J. Zool. 95, 527–537. doi: 10.1139/cjz-2016-0134
Penczykowski, R. M., Laine, A. L., and Koskella, B. (2016). Understanding the ecology and evolution of host-parasite interactions across scales. Evol. Appl. 9, 37–52. doi: 10.1111/eva.12294
Peterson, A. T., Soberon, J., Pearson, R. G., Anderson, R. P., Martinez-Meyer, E., Nakamura, M., et al. (2011). Ecological Niches and Geographic Distributions. Princeton, NJ; Oxford: Princeton University Press. doi: 10.23943/princeton/9780691136868.003.0003
Phelan, J. P., Kern, A., Ramsey, M. E., Lundt, M. E., Sharma, B., Lin, T., et al. (2019). Genome-wide screen identifies novel genes required for Borrelia burgdorferi survival in its Ixodes tick vector. PLoS Pathog. 15:e1007644. doi: 10.1371/journal.ppat.1007644
Piesman, J. (2002). “Ecology of Borrelia burgdorferi sensu lato in Northamerica,” in Lyme Borreliosis: Biology of the Infectious Agents and Epidemiology of Disease, eds J. S. Gray, O. Kahl, R. S. Lane, and G. Stanek (Wallingford: CABI Publishing), 223–249. doi: 10.1079/9780851996325.0223
Postic, D., Korenberg, E., Gorelova, N., Kovalevski, Y. V., Bellenger, E., and Baranton, G. (1997). Borrelia burgdorferi sensu lato in Russia and neighbouring countries: high incidence of mixed isolates. Res. Microbiol. 148, 691–702. doi: 10.1016/S0923-2508(99)80068-0
Poulin, R. (2017). Invasion ecology meets parasitology: advances and challenges. Int. J. Parasitol. Parasites Wildl. 6, 361–363. doi: 10.1016/j.ijppaw.2017.03.006
Qiu, W. G., Schutzer, S. E., Bruno, J. F., Attie, O., Xu, Y., Dunn, J. J., et al. (2004). Genetic exchange and plasmid transfers in Borrelia burgdorferi sensu stricto revealed by three-way genome comparisons and multilocus sequence typing. Proc. Natl. Acad. Sci. U.S.A. 101, 14150–14155. doi: 10.1073/pnas.0402745101
Radolf, J. D., Caimano, M. J., Stevenson, B., and Hu, L. T. (2012). Of ticks, mice and men: understanding the dual-host lifestyle of Lyme disease spirochaetes. Nat. Rev. Microbiol. 10, 87–99. doi: 10.1038/nrmicro2714
Randolph, S. E. (1998). Ticks are not insects: consequences of contrasting vector biology for transmission potential. Parasitol. Today 14, 186–192. doi: 10.1016/S0169-4758(98)01224-1
Randolph, S. E., and Craine, N. G. (1995). General framework for comparative quantitative studies on transmission of tick-borne diseases using Lyme borreliosis in Europe as an example. J. Med. Entomol. 32, 765–777. doi: 10.1093/jmedent/32.6.765
Randolph, S. E., and Rogers, D. J. (2010). The arrival, establishment and spread of exotic diseases: patterns and predictions. Nat. Rev. Microbiol. 8, 361–371. doi: 10.1038/nrmicro2336
Rauter, C., and Hartung, T. (2005). Prevalence of Borrelia burgdorferi sensu lato genospecies in Ixodes ricinus ticks in Europe: a metaanalysis. Appl. Environ. Microbiol. 71, 7203–7216. doi: 10.1128/AEM.71.11.7203-7216.2005
Ross, B. D., Hayes, B., Radey, M. C., Lee, X., Josek, T., Bjork, J., et al. (2018). Ixodes scapularis does not harbor a stable midgut microbiome. ISME J. 12, 2596–2607. doi: 10.1038/s41396-018-0161-6
Sassera, D., Beninati, T., Bandi, C., Bouman, E. A., Sacchi, L., Fabbi, M., et al. (2006). 'Candidatus Midichloria mitochondrii', an endosymbiont of the tick Ixodes ricinus with a unique intramitochondrial lifestyle. Int. J. Syst. Evol. Microbiol. 56(Pt 11), 2535–2540. doi: 10.1099/ijs.0.64386-0
Scholz, H. C., Margos, G., Derschum, H., Speck, S., Tserennorov, D., Erdenebat, N., et al. (2013). High prevalence of genetically diverse Borrelia bavariensis-like strains in Ixodes persulcatus from Selenge Aimag, Mongolia. Ticks Tick Borne Dis. 4, 89–92. doi: 10.1016/j.ttbdis.2012.08.004
Sertour, N., Cotte, V., Garnier, M., Malandrin, L., Ferquel, E., and Choumet, V. (2018). Infection kinetics and tropism of Borrelia burgdorferi sensu lato in mouse after natural (via ticks) or artificial (needle) infection depends on the bacterial strain. Front. Microbiol. 9:1722. doi: 10.3389/fmicb.2018.01722
Slatyer, R. A., Hirst, M., and Sexton, J. P. (2013). Niche breadth predicts geographical range size: a general ecological pattern. Ecol. Lett. 16, 1104–1114. doi: 10.1111/ele.12140
Soberon, J., and Arroyo-Pena, B. (2017). Are fundamental niches larger than the realized? Testing a 50-year-old prediction by Hutchinson. PLoS ONE 12:e0175138. doi: 10.1371/journal.pone.0175138
Sol, D. (2016). “Progresses and controversies in invasion biology,” in Current Trends in Wildlife Research, eds R. Mateo, B. Arroyo, and J. T. Garcia (Basel: Springer International Publishing), 177–200. doi: 10.1007/978-3-319-27912-1_8
Sonenshine, D. E., and Macaluso, K. R. (2017). Microbial invasion vs. tick immune regulation. Front. Cell. Infect. Microbiol. 7:390. doi: 10.3389/fcimb.2017.00390
Swanson, S. J., Neitzel, D., Reed, K. D., and Belongia, E. A. (2006). Coinfections acquired from ixodes ticks. Clin. Microbiol. Rev. 19, 708–727. doi: 10.1128/CMR.00011-06
Swei, A., and Kwan, J. Y. (2017). Tick microbiome and pathogen acquisition altered by host blood meal. ISME J. 11, 813–816. doi: 10.1038/ismej.2016.152
Takano, A., Nakao, M., Masuzawa, T., Takada, N., Yano, Y., Ishiguro, F., et al. (2011). Multilocus sequence typing implicates rodents as the main reservoir host of human-pathogenic Borrelia garinii in Japan. J. Clin. Microbiol. 49, 2035–2039. doi: 10.1128/JCM.02544-10
Taragel'ova, V., Koci, J., Hanincova, K., Kurtenbach, K., Derdakova, M., Ogden, N. H., et al. (2008). Blackbirds and song thrushes constitute a key reservoir of Borrelia garinii, the causative agent of borreliosis in Central Europe. Appl. Environ. Microbiol. 74, 1289–1293. doi: 10.1128/AEM.01060-07
Telford, S. R. III., Mather, T. N., Moore, S. I., Wilson, M. L., and Spielman, A. (1988). Incompetence of deer as reservoirs of the Lyme disease spirochete. Am. J. Trop. Med. Hyg. 39, 105–109. doi: 10.4269/ajtmh.1988.39.105
Thompson, J. N. (1999). Specific hypotheses on the geographic mosaic of coevolution. Am. Natur. 153, 1–14. doi: 10.1086/303208
Toft, C., and Andersson, S. G. (2010). Evolutionary microbial genomics: insights into bacterial host adaptation. Nat. Rev. Genet. 11, 465–475. doi: 10.1038/nrg2798
Tsao, J. I. (2009). Reviewing molecular adaptations of Lyme borreliosis spirochetes in the context of reproductive fitness in natural transmission cycles. Vet. Res. 40, 36. doi: 10.1051/vetres/2009019
Tsetsarkin, K. A., Vanlandingham, D. L., McGee, C. E., and Higgs, S. (2007). A single mutation in chikungunya virus affects vector specificity and epidemic potential. PLoS Pathog. 3:e201. doi: 10.1371/journal.ppat.0030201
Urbanova, V., Hajdusek, O., Honig Mondekova, H., Sima, R., and Kopacek, P. (2017). Tick thioester-containing proteins and phagocytosis do not affect transmission of Borrelia afzelii from the competent vector Ixodes ricinus. Front. Cell. Infect. Microbiol. 7:73. doi: 10.3389/fcimb.2017.00073
Van Treuren, W., Ponnusamy, L., Brinkerhoff, R. J., Gonzalez, A., Parobek, C. M., Juliano, J. J., et al. (2015). Variation in the microbiota of Ixodes Ticks with Regard to Geography, Species, and Sex. Appl. Environ. Microbiol. 81, 6200–6209. doi: 10.1128/AEM.01562-15
Vazquez, D. P. (2006). “Exploring the relationship between niche breadth and invasion success,” in Conceptual Ecology and Invasion Biology: Reciprocal Approaches to Nature, eds M. W.Cadotte, S. M. McMahon, and T. Fukami (Dordrecht: Springer), 307–322. doi: 10.1007/1-4020-4925-0_14
Vollmer, S. A., Bormane, A., Dinnis, R. E., Seelig, F., Dobson, A. D., Aanensen, D. M., et al. (2011). Host migration impacts on the phylogeography of Lyme Borreliosis spirochaete species in Europe. Environ. Microbiol. 13, 184–192. doi: 10.1111/j.1462-2920.2010.02319.x
Vollmer, S. A., Feil, E. J., Chu, C. Y., Raper, S. L., Cao, W. C., Kurtenbach, K., et al. (2013). Spatial spread and demographic expansion of Lyme borreliosis spirochaetes in Eurasia. Infect. Genet. Evol. 14C, 147–155. doi: 10.1016/j.meegid.2012.11.014
Vrålstad, T., Johnsen, S. I., and Taugbøl, T. (2011). NOBANIS – Invasive Alien Species Fact Sheet – Aphanomyces astaci. Online Database of the European Network on Invasive Alien Species – NOBANIS. Available online at: www.nobanis.org.
Wade, M. J. (2007). The co-evolutionary genetics of ecological communities. Nat. Rev. Genet. 8, 185–195. doi: 10.1038/nrg2031
Wilske, B., Busch, U., Eiffert, H., Fingerle, V., Pfister, H. W., Rossler, D., et al. (1996). Diversity of OspA and OspC among cerebrospinal fluid isolates of Borrelia burgdorferi sensu lato from patients with neuroborreliosis in Germany. Med. Microbiol. Immunol. 184, 195–201. doi: 10.1007/BF02456135
Wilske, B., Preac-Mursic, V., Gobel, U. B., Graf, B., Jauris, S., Soutschek, E., et al. (1993). An OspA serotyping system for Borrelia burgdorferi based on reactivity with monoclonal antibodies and ospA sequence analysis. J. Clin. Microbiol. 31, 340–350.
Wilson, J. R. U., García-Díaz, P., Cassey, P., Richardson, D. M., Pyšek, P., and Blackburn, T. M. (2016). Biological invasions and natural colonisations are different – the need for invasion science. NeoBiota 31, 87–98. doi: 10.3897/neobiota.31.9185
Woolhouse, M. E., Webster, J. P., Domingo, E., Charlesworth, B., and Levin, B. R. (2002). Biological and biomedical implications of the co-evolution of pathogens and their hosts. Nat. Genet. 32, 569–577. doi: 10.1038/ng1202-569
Xu, H., He, M., Pang, X., Xu, Z. C., Piesman, J., and Yang, X. F. (2010). Characterization of the highly regulated antigen BBA05 in the enzootic cycle of Borrelia burgdorferi. Infect. Immun. 78, 100–107. doi: 10.1128/IAI.01008-09
Yang, X., Smith, A. A., Williams, M. S., and Pal, U. (2014). A dityrosine network mediated by dual oxidase and peroxidase influences the persistence of Lyme disease pathogens within the vector. J. Biol. Chem. 289, 12813–12822. doi: 10.1074/jbc.M113.538272
Keywords: geographic spread, tick-borne pathogenic bacteria, Borrelia burgdorferi sensu lato, Borrelia bavariensis, vector interaction, Ixodes ricinus complex
Citation: Margos G, Fingerle V and Reynolds S (2019) Borrelia bavariensis: Vector Switch, Niche Invasion, and Geographical Spread of a Tick-Borne Bacterial Parasite. Front. Ecol. Evol. 7:401. doi: 10.3389/fevo.2019.00401
Received: 16 July 2019; Accepted: 08 October 2019;
Published: 23 October 2019.
Edited by:
Luciano Bosso, University of Naples Federico II, ItalyReviewed by:
Katharine Sassandra Walter, Stanford University, United StatesSam Mechai, Public Health Agency of Canada (PHAC), Canada
Copyright © 2019 Margos, Fingerle and Reynolds. This is an open-access article distributed under the terms of the Creative Commons Attribution License (CC BY). The use, distribution or reproduction in other forums is permitted, provided the original author(s) and the copyright owner(s) are credited and that the original publication in this journal is cited, in accordance with accepted academic practice. No use, distribution or reproduction is permitted which does not comply with these terms.
*Correspondence: Gabriele Margos, gabriele.margos@lgl.bayern.de; gmargos1@gmail.com