- 1Department of Biology, Alma College, Alma, MI, United States
- 2Department of Forestry, Michigan State University, East Lansing, MI, United States
Negative plant-soil feedback (PSF), where plant performance is reduced in soils conditioned by conspecifics, is widely documented in plant communities. However, the strength and sometimes direction of PSF can vary widely, presumably not only due to the plant species within the community but also to environmental context. We hypothesized that soil fertility and light availability influence the direction and strength of plant-soil feedback experienced by tree seedlings. We conducted a 10-week greenhouse experiment and assessed survivorship of Northern red oak (Quercus rubra) and black cherry (Prunus serotina) in low (~1% full sun) vs. high (~18% full sun) light availability and in non-sterile vs. sterile soils collected under the canopy of conspecific vs. heterospecific adult trees at five sites that vary in nutrient availability in Manistee National Forest, Michigan, USA. Q. rubra seedlings experienced neutral plant-soil feedback regardless of light level or site as only one seedling died during the course of the experiment. Prunus serotina seedlings experienced microbe-mediated negative PSF in low fertility sites but positive feedback at high fertility sites, but these feedbacks occurred only under high light availability. Consistent with these results, microbe-mediated negative PSF increased with soil Fe3+ and C:N ratios and decreased with NH4+. Our results demonstrate the important role of environmental context, specifically light and soil nutrient availability, on the magnitude and direction of conspecific plant soil feedback, particularly in P. serotina. Since Q. rubra experienced neutral microbial PSF, P. serotina has a relative disadvantage to Q. rubra under lower site fertility, but a relative advantage under higher site fertility. These results are consistent with these species relative abundances in the field and thus PSF could be an important driver of plant community dynamics.
Introduction
A long-standing challenge in ecology is to identify factors regulating plant abundance, co-existence, and community composition. For plant communities, the seedling-establishment phase is a major demographic bottleneck, and thus a critical stage for future community dynamics (Gurevitch et al., 2006). There has been a vast array of hypotheses proposed for how dominance by the most competitive species can be precluded (Palmer, 1994). Janzen (1970) and Connell (1971) proposed that host specific natural enemies could maintain high tree diversity by reducing seed and/or seedling survivorship near conspecific adults and/or at high conspecific densities. Such NDD seedling mortality would favor establishment of heterospecific individuals under an adult tree, and thus promote species coexistence (Mangan et al., 2010; Alvarez-Loayza and Terborgh, 2011). Widespread patterns of plant demography consistent with NDD have been identified (e.g., HilleRisLambers et al., 2002; Comita et al., 2010; Swamy et al., 2011; Johnson et al., 2012). Interactions between adult plants and juveniles that are mediated by root-associated fungi and other symbiotic microorganisms (“biotic-mediated plant soil feedback”) (Bever et al., 2012; Terborgh, 2012) is likely a prime mechanism creating these NDD patterns since plant-soil feedback can reduce seedling survival near conspecific adults while favoring heterospecific seedlings (Mangan et al., 2010; McCarthy-Neumann and Kobe, 2010; Alvarez-Loayza and Terborgh, 2011; McCarthy-Neumann and Ibáñez, 2012).
Plant-soil feedback (PSF) is created when a plant's presence alters soil conditions (biotic, physical, and/or chemical), which in turn affects its own and other plants' performance (Bever, 2003). A key component for these feedbacks to influence community dynamics is that there are species-specific responses to altered soil conditions; for biotic-mediated feedbacks this would result from plants culturing divergent microbial communities surrounding their root systems that exhibit some degree of host-preference (Benítez et al., 2013). Comparisons between the performance of plants in soil conditioned by conspecifics vs. heterospecifics assess the specificity of PSF effects (Brinkman et al., 2010), and have relevance to understanding cases of conspecific inhibition and facilitation due to forms of density-, distance-, and/or frequency-dependent effects. Negative PSF may stabilize species coexistence if a plant modifies its soil biota in a way that inhibits conspecific more than heterospecific recruits, thereby preventing that plant species from dominating the community (Bever et al., 1997). Positive PSF occurs when soil influenced by conspecifics has positive effects (Dickie et al., 2014; Bennett et al., 2017) and may contribute to either clumped distributions or monodominance.
Plant-soil feedback is often found to be widespread and often driven by soil biota in studies with many species (Klironomos, 2002; Petermann et al., 2008; Bennett et al., 2017), and in meta-analysis of published research (Kulmatiski et al., 2008; Crawford et al., 2019). However, there is strong variation in the strength of PSF. For instance, several studies have found strong positive correlation between local adult abundance for a species and the strength of PSF on seedling performance (Klironomos, 2002; Mangan et al., 2010; MacDougall et al., 2011; McCarthy-Neumann and Ibáñez, 2013; Xu et al., 2015; LaManna et al., 2016). However, other empirical studies report locally common species have greater negative PSF (Liu et al., 2015; Zhu et al., 2015), or that PSFs are pervasive across all species regardless of local abundance (Petermann et al., 2008). Variation in direction and strength of PSF has also been linked to mycorrhizal symbiont type, with arbuscular mycorrhizal fungi associated species experiencing negative and ectomycorrhizal fungi species positive feedback (Bennett et al., 2017). A recent meta-analysis found greater negative PSF when species shared the same mycorrhizal guild, were phylogenetically distant and native (Crawford et al., 2019). Species functional traits can also be a strong predictor with shade intolerant tree species experiencing stronger negative feedback than shade tolerant species (McCarthy-Neumann and Kobe, 2008; McCarthy-Neumann and Ibáñez, 2013).
The environmental context in which plants are establishing and growing likely impacts the occurrence and strength of these feedback as well. This influence can act through changes to the soil microbial community (e.g., abundance or composition) or plant response to soil micro-organisms under those environmental conditions. For instance, negative PSF can be stronger or occur only in low light conditions (McCarthy-Neumann and Kobe, 2010; McCarthy-Neumann and Ibáñez, 2013; but see Smith and Reynolds, 2015 which found the opposite pattern). Stronger negative PSF in low light may be due to either increased abundance of soil pathogens (Reinhart et al., 2010; Hersh et al., 2012), mycorrhizal fungi acting parasitically (Ibáñez and McCarthy-Neumann, 2016), and/or a reduction in the plant's physical (Augspurger, 1990) or chemical (Ichihara and Yamaji, 2009) defenses, or ability to re-grow lost tissue (Myers and Kitajima, 2007; Kobe et al., 2010) in low light.
Soil fertility may also influence the soil microbial community or plant response to soil micro-organisms. For instance, soil pathogens are more abundant under high soil resources such as with increased soil moisture (Hersh et al., 2012), and mycorrhizal fungi are beneficial under low-soil resources, but detrimental at high soil resources (Johnson et al., 1997; Grman et al., 2012). These soil biotic responses suggest increased negative PSF as soil fertility increases (as postulated by Revillini et al., 2016; Lekberg et al., 2018). However, in greenhouse experiments, fertilization reduced negative PSF (Petermann et al., 2008) or neutralized either positive or negative PSF (in 't Zandt et al., 2019). As outlined by Smith-Ramesh and Reynolds (2017), negative PSF may be expected to weaken due to a decline in nutrient stress and strengthened physical or chemical defenses (especially those that are N-based) against soil pathogens, and/or ability to re-grow lost tissue from non-structural carbohydrates.
The recent recognition of the importance of environmental context for PSFs [e.g., light and nutrients as described above, herbivory (Bezemer et al., 2013; Heinze and Joshi, 2018) or competition (Casper and Castelli, 2007; Bezemer et al., 2018; Lekberg et al., 2018)], will enable more robust predictions of how PSF regulates plant abundance, promotes co-existence, alters community composition, and impacts ecosystem function. Specifically, understanding the role of light availability on PSF will help us better understand seedling dynamics from small gap formation to large stand disturbance. Understanding the role of soil fertility on PSF will help us better predict how global environmental change (e.g., precipitation and nutrient enrichment) may influence plant coexistence and ecosystem function.
To test effects of environmental context on PSF, we conducted a greenhouse plant-soil feedback experiment with black cherry and red oak seedlings grown in soils from five forest sites (which vary in species local abundance, productivity and fertility), and at low and high light. We asked: (1) Does the direction and strength of biotic-mediated PSF vary across multiple sites? (2) Which factors at the site level (e.g., species local abundance, productivity, and fertility) best correlate with species PSF? (3) Does light environment interact with soil factors in determining the direction and strength of PSFs? We predicted that increasing soil fertility will decrease biotic-mediated negative PSF. We also predicted that increasing light will decrease biotic-mediated negative PSF or even shift the direction of biotic-mediated PSF toward a positive feedback. Both predictions stem from the assumption that robust plants under high resources are better able to survive negative PSF and to take advantage of positive PSF than stressed plants under low resources.
Methods
To assess context dependence for the existence and strength of PSF, we assessed survivorship for Prunus serotina and Quercus rubra seedlings to non-sterile vs. sterile soils collected under the canopy of conspecific vs. heterospecific adult trees at 5 different forest sites that range in species composition, productivity and soil fertility (Tables 1, 2). The seedlings were grown at low and high light in a greenhouse experiment for 10-weeks starting in April 2013.
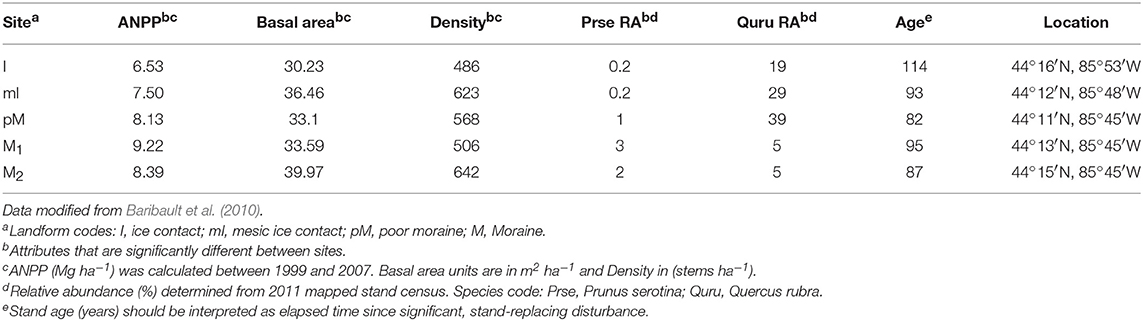
Table 1. Community productivity, structure and composition attributes for forest sites where soil was collected for the plant-soil feedback greenhouse experiment.
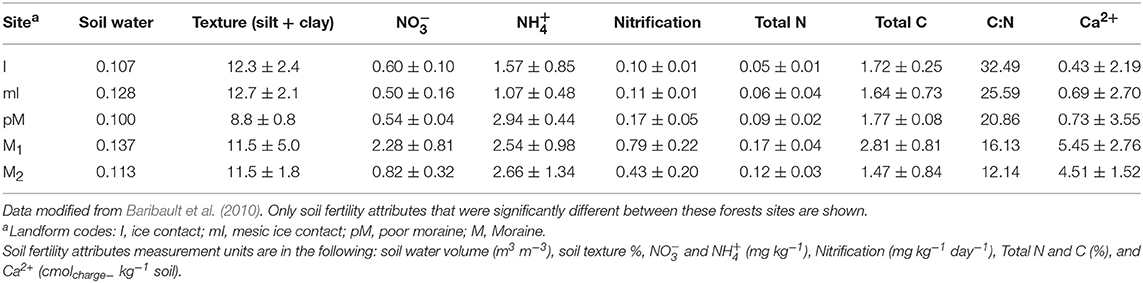
Table 2. Mean soil fertility attributes for forest sites where soil was collected for the plant-soil feedback greenhouse experiment.
Soil Sources and Adult Tree Density Measures
In the first week of April (2013), we collected soil (top 15-cm) within 1 m from the bole of two mature adults for each of our study species at five different mixed hardwood stands located in Manistee National Forest in the lower peninsula of Michigan, USA. This is a region with well-defined associations among glacial landforms, soil fertility, and forest community composition. All sites were within an area of 960 km2 (average distance 15.76 km) and are expected to have very similar climate. Mapped stands were established in 1999 at each of these sites and the most recent census (e.g., species identification, location, measurement of size, and classification of health) of all trees ≥10 cm diameter occurred in Fall of 2011.
To minimize the potential for multiple tree species influencing the soil, we sampled under trees that were at least two crown diameters away from adults of other study species. We diced roots and sifted soil through a 1-cm mesh sieve. To control for individual tree effects, soil from each adult was kept separate throughout the course of the experiment (e.g., Reinhart and Rinella, 2016). We sterilized half of the field and all of the potting soil (Fafard Mix #2, Conrad Fafard Inc., Agawam, MA USA) by gamma irradiation prior to the start of the experiment (~40 KGray; Sterigenics International, Inc., Schaumburg, IL, USA). Sterilization of soil biota through gamma irradiation appeared to be effective as we found no mycorrhizal fungal colonization in a sub-sample of harvested seedling in the sterile treatments.
Planting Methods
Prunus serotina seeds were collected from southeastern Michigan forests, and Q. rubra seeds were purchased from Sheffield's Seed Co. (Locke, NY, USA). To minimize diseases from non-experimental soil sources (e.g., from the collection sites), seeds were surface sterilized (0.6% NaOCl solution) prior to stratification and again prior to germinating in perlite. Seeds with newly emerged radicles were weighed and then planted into 6.4-cm diameter × 25-cm depth pots and grown in greenhouse facilities at Michigan State University's Tree Research Center.
The experiment consisted of a total of 6 replicate seedling pots per experimental treatment (e.g., soil collected from 2 individual adult trees × 3 replicate pots). Experimental treatments were site (5 sites spanning soil fertility) × soil source [conspecific vs. heterospecific soil] x soil status [sterile vs. non-sterile soil] x light availability [1 vs. 18% full sun]). Soil status consisted of a “non-sterile treatment,” a 1:1:2 mixture of non-sterile field soil, sterile field soil, and sterile potting soil, and a “sterile treatment,” a 1:1 mixture of sterile field soil and sterile potting soil. The sterile field soil was always fully composed of either conspecific or heterospecific soil depending upon the soil source treatment. The light availability treatment mimicked conditions from understory (1% full sun) to moderately sized tree-fall gaps (18% full sun) encountered in the Michigan forests at which the soil for this experiment was collected (Schreeg et al., 2005). The lowlight treatment was created by covering benches with an inner layer of black shade cloth and an outer layer of reflective knitted poly-aluminum shade cloth. Light availability as a percentage of full sun levels was confirmed at each bench by calculating the percentage of sunlight measured in the open and with paired photosynthetic active radiation (PAR) measurements at each bench in the greenhouse. PAR was measured on a uniformly overcast day with a LI-COR250A quantum sensor (LI-COR, Lincoln, NE, USA).
Individual pots were set up on two different benches (one low and high light), where all combinations of site, soil source, and soil treatments were represented, and were watered (~50 ml of deionized water) by hand every 3 days for 10 weeks. Emergence and survival were recorded twice weekly, and date of death was assigned as the first census with total leaf and/or stem tissue necrosis.
We used cation and anion PRS™ probes that contain 17.5 cm2 ion-exchange resins (Western Ag Innovations, Saskatoon, Saskatchewan) to assess the effect of site, soil source, sterilization and light on nutrient supply rates in the experiment (Table 3). We measured nutrient supply rates from 40 samples in high light (5 sites × 2 soil source × 2 soil status × 2 replicates) and 20 samples in low light [5 sites × 2 soil source x non-sterile × 2 replicates). We did not assess the effect of species of seedling growing in the soil on nutrient supply rates so replicates are derived from 4 P. serotina and 4 Q. rubra seedlings per treatment combination and in each pot paired cation and anion probes were inserted into the pots starting at week 2 after seedling planting and ending at week 5. Upon removal, the probes were washed with de-ionized water and sent to Western Ag Innovations for analysis of available nitrogen (N, sum of and NH4+), Ca2+, Mg2+, K+, H2, Fe3+, Mn2+, Cu2+, Zn2+, B(OH)4 3+, , Pb2+, and Al3+.
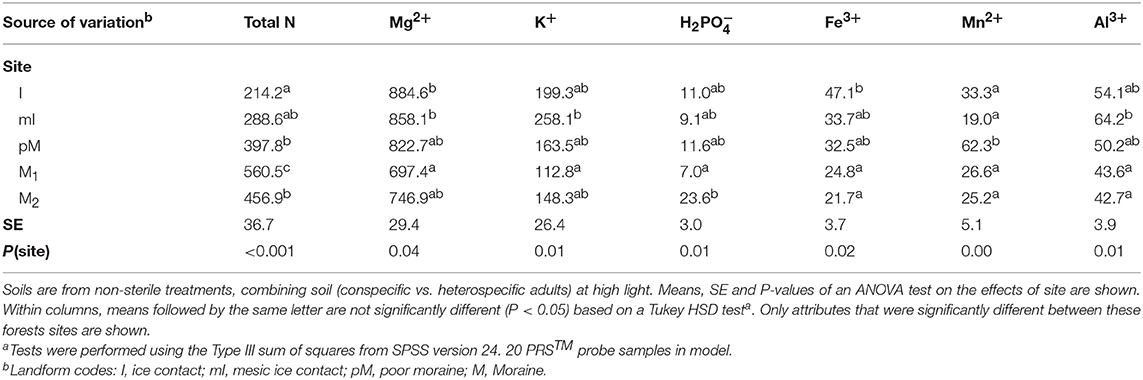
Table 3. Soil nutrient supply rates (μg ion/PRS® Probe/3 week) in the experimental pots across forest site treatments.
Analytical Approach
We analyzed the survival data of seedlings grown in conspecific vs. heterospecific soil from five different forest stands, under sterile vs. non-sterile, and at low and high light levels. Data for each seedling i and each time t, Nit, were coded as 0 until the seedling was found dead, Nit = 1. We used a count process (Poisson distribution) to model the number of failures, in this case death of a seedling at a particular time (Nit), we then estimated the probability of mortality in a Cox survival model that included an intrinsic rate of mortality and an extrinsic risk (Andersen and Gil, 1982). We modeled the likelihood as:
and process model:
where parameter λ is then estimated as a function of the intrinsic rate of mortality (age or time dependent mortality), or hazard h, and the extrinsic risk of mortality or risk μ (mortality due to external factors like site of soil collection, light level, etc.):
Parameters in the model were then estimated at the species level, following a Bayesian approach that allowed us to consider the different sources of uncertainty associated with the data (Clark, 2005). The hazard was estimated for each time step, ht, from a gamma distribution with non-informative parameter values, ht~Gamma (0.01, 0.01). The risk, μit, was estimated as a function of the covariates included in the analysis, μi = XiB. Xi is the matrix of covariates associated to each seedling. B is the vector of fixed effect coefficients associated to each covariate. These coefficients were estimated from normal distributions with non-informative parameter values, Bk~Normal (0, 10,000). Covariates included site of soil collection, soil source (conspecific vs. heterospecific), soil sterilization (non-sterile vs. sterile), light level (low vs. high), and standardized seed size. We did not assess effect of individual tree for each species in this analysis as we were interested in the overall effect of conspecific vs. a heterospecific cultured soil on seedling survival and we did not have large enough replicate size to robustly assess any variation that might exist between individual trees that had cultured the soil.
Models were run only for P. serotina since only one Q. rubra seedling died during the course of the experiment. Models were run in OpenBUGS 1.4 (Thomas et al., 2006). Simulations (three chains) were run until convergence of the parameters was ensured (~50,000 iterations) and then run for another 25,000 iterations from which posterior parameter values and predicted survival were estimated. Using model parameters, means, variances and covariances, we estimated survival (Tables S1, S2), and used these predicted survival values to explicitly assess whether there were differences in how species responded to soil site (five forest stands varying in site fertility), soil source (conspecific vs. heterospecific) and soil treatments (non-sterile vs. sterile) at low vs. high light levels (Tables S3, S4). We also assessed how species responded to microbial mediated PSF [measured by subtracting the PSF effect (survival in conspecific vs. heterospecific soil) on predicted survival in sterile soil from the PSF effect on predicted survival in non-sterile soil] across the five sites and at low vs. high light levels. Differences that did not include zero in their 95% credible intervals were considered statistically significant.
We determined whether nutrient supply rates within the experiment differed between site, soil source, soil status and light availability using ANOVA (SPSS version 24.0; SPSS, Chicago, Illinois, USA). Whether soil was from conspecific vs. heterospecific adults had little impact on nutrient supply rates (Table S5), and there was no significant difference in any nutrient supply rates between low vs. high light in non-sterile soil treatments (we did not have samples to compare light treatment effects in sterile soil; Table S6). What we consider to be microbial mediated PSF, difference between predicted PSF effects on survival in sterile soil vs. non-sterile soil, could be due to both the micro-organisms in the non-sterile soil as well as some other abiotic factor that was altered during sterilization (e.g., Troelstra et al., 2001). However, sterilization had only some relatively minor impact on nutrient supply rates in the experiment, resulting in greater supply rate for H2, Mn2+, Zn2+, and Pb2+ (Table S7), and there were no sign of nutrient deficiency in seedlings planted in the sterile treatments. We then used multiple step-wise regressions (SPSS) to determine which site factors at the stand level (e.g., species composition, productivity and fertility, Tables 1, 2) and at the experimental level (e.g., nutrient supply rates from the PRS probes, Table 3) correlate with PSF in both low and high light environments. Only factors (Tables 1–3) that were significantly different among the sites were included in the analysis.
Results
Environmental Context (i.e., Site Fertility and Light Availability) Alters the Occurrence and Direction of PSFs
In general as soil fertility increased, biotic-mediated plant soil feedbacks in high light went from negative to positive; while effects were less pronounced in low light, there were consistent results with a negative biotic-mediated PSF at the intermediate fertility site and a positive PSF at the highest fertility site (lowest C:N ratio). In low fertility sites at high light, Prunus serotina seedlings experienced a microbe-mediated negative feedback (i.e., the negative effect of conspecific vs. heterospecific soil was greater in non-sterile than sterile soil). In contrast in high fertility sites at high light, the feedback flipped to positive (Figure 1). In low light, seedlings showed a negative feedback at a moderate fertility site (poor Moraine) and positive feedback at higher fertility (Moraine) (Figure 1).
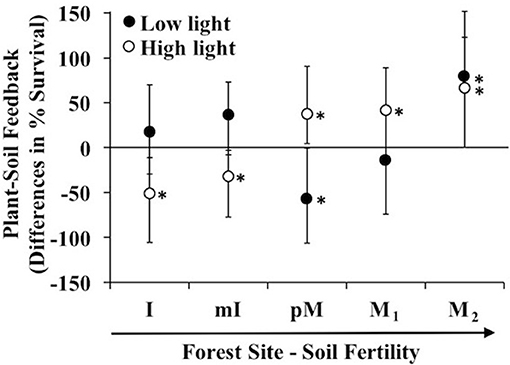
Figure 1. Differences in predicted survival due to microbe mediated PSF (survival in conspecific vs. heterospecific non-sterile soil—survival in conspecific vs. heterospecific sterile soil) in a greenhouse experiment of Prunus serotina seedlings across 5 sites that range in soil fertility and community composition (Tables 1, 2) conducted under low and high light. Sites are in rank order of soil fertility from left (low) to right. Data are means with 95% credible intervals; credible intervals that do not overlap with the zero line are statistically significant. *P < 0.05.
The above microbe-mediated PSF values remove abiotic effects of the soil (survival response to sterile soils cultured by conspecific vs. heterospecific adult trees). In the non-sterile soil treatment, where biotic and abiotic effects both occurred, Prunus serotina seedlings grown at high light experienced negative PSF at low fertility sites, and positive PSF at the intermediate and one of the high fertility sites (Figure 2A). In contrast, under abiotic effects only (results from the sterile soil), P. serotina seedlings grown at high light in low fertility sites experienced positive PSF, but negative PSF at the intermediate and the other high fertility site (M2) (Figure 2B). Thus, under high light, the negative microbe-mediated PSF in low fertility sites and positive microbe-mediated PSF at high fertility sites (Figure 1) is enhanced when abiotic-mediated PSF are explicitly considered and removed in the analysis. In particular, at one of the moraine sites (M2) there was not a significant PSF when comparing just non-sterile soils (Figure 2A). The increased survival in conspecific soils due to soil biota was only apparent at this site (Figure 1) when the large negative abiotic effect of conspecific soil was removed (Figure 2B). In low light, only seedlings at the intermediate fertility site (pM) experienced negative PSF in the non-sterile treatment (Figure 2A), and abiotic-mediated PSF occurred at one of the most fertile sites (M1). Lastly, only at the intermediate fertility site (pM) was there a significant difference in direction of PSF with negative PSF at low light and positive PSF at high light, but this only occurred when soil micro-organisms were included in the feedback (Figures 1, 2A).
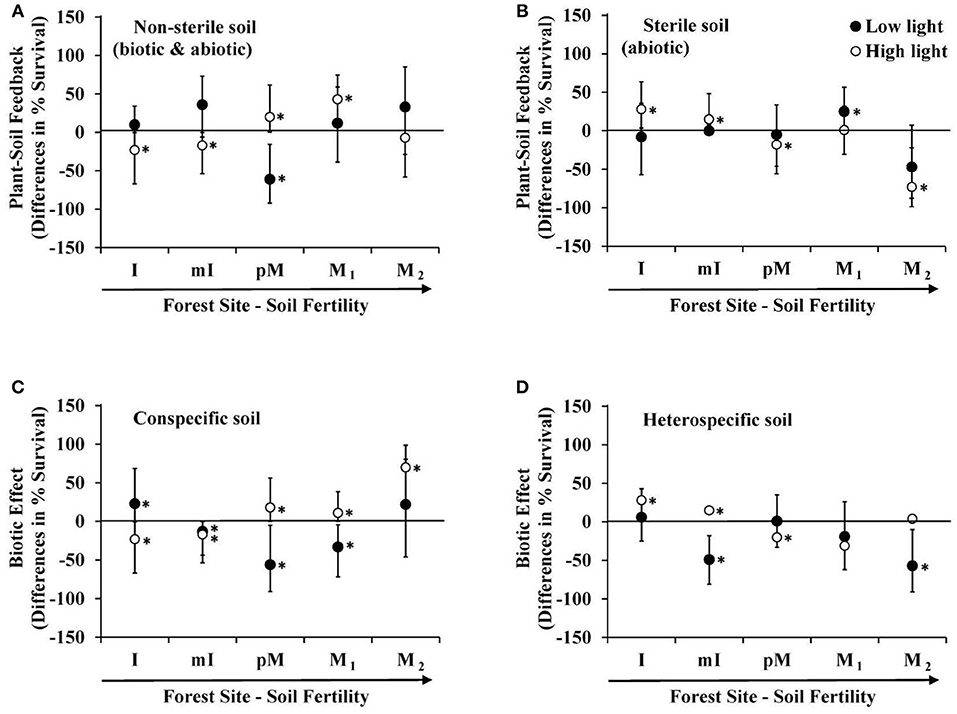
Figure 2. Differences in predicted survival due to: (A) microbe + abiotic mediated PSF (survival in conspecific vs. heterospecific non-sterile soil), (B) abiotic mediated PSF (survival in conspecific vs. heterospecific sterile soil), (C) biotic effect in conspecific soil (survival in non-sterile vs. sterile conspecific soil), and (D) biotic effect in heterospecific soil (survival in non-sterile vs. sterile heterospecific soil) in a greenhouse experiment of Prunus serotina seedlings across 5 sites that range in soil fertility and community composition (Tables 1, 2) conducted under low and high light. Sites are in rank order of soil fertility from left (low) to right. Data are means with 95% credible intervals; credible intervals that do not overlap with the zero line are statistically significant. *P < 0.05.
Negative microbe-mediated feedback could derive solely from detrimental micro-organisms in conspecific soil, beneficial micro-organisms in heterospecific soil or some combination of the two. At high light, P. serotina seedlings experienced negative microbe-mediated PSF in low fertility sites (Figure 1) due to detrimental micro-organisms in conspecific soil (Figure 2C), and beneficial micro-organisms in heterospecific soils (Figure 2D). The positive PSF at higher fertility sites for seedlings grown in high light environments (Figure 1) was due to beneficial micro-organisms in conspecific soil at each of the sites (Figure 2C). In addition, at the intermediate fertility site (pM) micro-organisms in the heterospecific soil were also detrimental (Figure 2D). The light environment affected P. serotina's seedling survival response to soil microbes from conspecific soil with a beneficial response in high light and a detrimental response in low light at the intermediate fertility site (pM) (Figure 2C). For all other sites and for all heterospecific soils, the light level did not influence how seedlings responded to soil microbes (Figures 2C,D).
C:N, NH4+, and Fe3+ Are Correlated With the Occurrence and Direction of PSF, but Only at High Light
A variety of community attributes (Table 1), and soil resource conditions (Tables 2, 3) significantly varied across sites. Mean stand level soil C:N and NH4+ at the sites where soil was collected best predicted microbe-mediated PSF at high light [F(2, 4) = 468.8, p = 0.002, R2 = 0.99]; sites with higher C:N ratios and lower NH4+ experienced more negative PSF. Similarly, microbe-mediated negative PSF at high light strengthened (became more negative) as average Fe3+ increased [F(1, 3) = 11.2, p = 0.04 with an R2 of 0.76]. No site or experimental factors correlated with predicted microbe-mediated negative PSF in low light.
Age and Seed Size Influenced Seedling Survival
While soil fertility and light strongly influenced plant soil feedback over the entire experiment, there were multiple peaks (weeks 4, 6, and 8) of relatively higher seedling mortality (Figure S1). Seed weight significantly affected survival with smaller seeded individuals more likely to die (Table S1).
Discussion
While light and soil fertility interact to influence seedling survivorship and growth (e.g., Kobe, 1996; Peltzer and Wardle, 2016), light and soil fertility also could influence PSF which in turn has an effect on seedling performance. We found that the direction of microbe-mediated PSF varied across sites with highly negative PSF in low fertility sites, but highly positive PSF in higher fertility sites for P. serotina seedlings. High C:N and Fe3+availability and low NH4+ in soils were the factors associated with strong negative PSF. This correlation of PSF going from highly negative to highly positive as soil fertility increased only occurred in high light conditions. In low light, results were idiosyncratic with seedlings experiencing negative PSF (vs. positive PSF in high light) at the intermediate fertility site to positive PSF (found in both low and high light) at the site with the lowest C:N. These findings suggest that PSF is a widespread, important factor in P. serotina seedling recruitment, but its effects are dynamic and can be altered by the soil fertility of the site and the light availability that germinating seedlings experience. Seedling survival in soils cultured by conspecific adults can result in widely diverging outcomes (i.e., from highly detrimental, neutral to highly beneficial) depending upon the local environmental context.
Moreover, these results suggest that the plasticity of microbe-mediated PSF in response to environmental context can shift relative advantages to different species, thereby contributing to species coexistence. In the present study, Prunus serotina has a relative disadvantage to Q. rubra under low fertility but a relative advantage under high fertility. Of course, numerous other factors, such as direct resource effects and herbivory as well as abiotic feedbacks, also will influence growth and survival and the outcome of which species “wins” under a given set of environmental conditions. Nevertheless, because Q rubra had a neutral PSF (same microbe-mediated PSF across all soil fertility levels), the microbial PSF-mediated advantage shifts toward P. serotina's favor relative to Q. rubra, as soil fertility increases. The feedbacks in the field would be an integration of both the biotic and abiotic mechanisms. However, the predictions based on microbe-mediated PSF from our greenhouse experiment are in fact consistent with the actual distribution of these species in the field (see Table 1).
Our findings are the first showing the importance of soil fertility on PSF in a tree species and are mostly consistent with the conceptual framework for PSF context dependence proposed by Smith-Ramesh and Reynolds (2017), and experimental work with herbaceous grassland species that shows stronger negative PSF in low fertility conditions (Petermann et al., 2008; in 't Zandt et al., 2019; but see Harrison and Bardgett, 2010 which found no effect of soil fertility on PSF). However, instead of the PSF becoming less negative (Petermann et al., 2008), absent (in 't Zandt et al., 2019), or weakly positive (Smith-Ramesh and Reynolds, 2017) we found PSF in higher fertility conditions to be equally strong, but in the opposite direction (i.e., seedling survival switching from worse to better in conspecific relative to heterospecific cultured soil). Although, pathogens are often found to be more abundant as nutrients, such as nitrogen, increase (Wei et al., 2018) their impact on seedling survival may be mitigated. For instance, based upon our results we hypothesize that plants with increased overall vigor (i.e., growing in favorable conditions with high levels of nutrients and light) are either better able to defend themselves or tolerate soil-borne pathogens in these environments. Increased survival in conspecific soil in these high resource conditions were due to soil biota. We speculate that mycorrhizal fungi were the biotic agents underlying this result and may have both protected seedlings against species-specific pathogens (Jung et al., 2012) as well as increased nutrient and water uptake.
We found that biotic-mediated PSF was correlated with soil C:N ratio; seedling survival due to soil biota decreased in conspecific soil as soil C:N increased. We are not aware of studies in non-agricultural systems that have examined the effects of organic matter and soil C:N ratio on disease dynamics. In agriculture and horticulture, however, it is common practice to add organic matter to soil to suppress damping-off (Bonanomi et al., 2018). While the importance of C:N ratio of organic matter for disease suppression is contested (Bonanomi et al., 2018), there is some evidence that organic matter additions with low C:N can ameliorate damping-off (Pane et al., 2011). Also, fertilizers with low C:N result in lowered mortality from Pythium-induced damping-off (Al-Azizi et al., 2013). Strong competition by soil microbes in low C:N soils may limit carbon availability to germinating fungal spores or invading hyphae, resulting in stagnation of fungal growth (Bonanomi et al., 2013).
Contrary to our prediction, only at one site (pM) did an increase in light shift biotic-mediated PSF from negative to positive. In fact, biotic-mediated PSF were prevalent across all sites in high light conditions, although the direction of PSF varied based on site fertility. Our results on PSF and light interactions appears to be highly sensitive to timing of soil collection based on comparisons with our earlier work (McCarthy-Neumann and Kobe, 2010; McCarthy-Neumann and Ibáñez, 2012, 2013), when soil was collected during winter when the fungal community was more likely to be present as spores, which enables higher microbe survival during transport and cold storage (Reinhart et al., 2005), which found PSF prevalent in low light rather than high light (Appendix S8). Methodology for soil biota inoculum may also be important since the use of microbial wash (McCarthy-Neumann and Kobe, 2010), which likely reduced or eliminated some pathogenic microbes and excluded AMF spores vs. use of whole soil, resulted in the majority of PSF being abiotic-mediated (Table S8).
Plant-soil feedback is a complex process that incorporates the sum effect of biotic (natural enemies, mutualists, and self-DNA Mazzoleni et al., 2015), and abiotic (nutrient availability and secondary chemicals) interactions between a plant and the soil it is growing in (Bennett and Klrionomos, 2019). We found strong biotic-mediated PSF for P. serotina grown at high light that went from highly negative in low fertility, due to detrimental micro-organisms in conspecific vs. beneficial micro-organisms in heterospecific soils, to highly positive in high fertility sites, due to beneficial micro-organisms in conspecific soils. Interestingly, abiotic-mediated PSF was opposite in nature relative to biotic-mediated PSF with positive effects in low fertility and negative effects in high fertility sites. Thus, if we had solely focused on PSF derived from combined biotic and abiotic mechanisms (e.g., non-sterile treatment), we would have concluded that there was no PSF at the highest fertility site). It is unlikely that the abiotic effects we found in our study are due to nutrient availability since there was rarely any instances where nutrient levels were significantly different between conspecific and heterospecific soils at a site. Thus, secondary chemicals are a likely primary mechanism for abiotic-mediated PSF found in our study. Consistent with this, allelopathic chemicals in heterospecific soil could cause the positive abiotic-mediated PSF at low fertility sites. For example, condensed tannins in Q. rubra leaves increase in low N conditions (Kinney et al., 1997). In contrast, autotoxic chemicals in conspecific soils could be the cause for the negative abiotic-mediated PSF at high fertility sites, but what autotoxic chemicals may be operating for P. serotina in high fertility sites is unknown.
Conclusion
Our results illustrate interactions among PSF (mediated by both biotic and abiotic agents), soil fertility and irradiance. The overall effect of these environmental factors on seedlings appears to be complex, especially since these factors operate at both fine (e.g., irradiance) and coarse (e.g., soil fertility across sites) spatial scales. Our results demonstrate that PSF can be an important driver in plant community dynamics, but their effects could be either promote species coexistence when PSF is negative or promote monodominance when PSF is positive, which depends upon environmental context. A broad understanding of the environmental dependency of PSF is needed to both understand the dynamics of communities and to apply that understanding to ecological challenges such as invasive species management, native species restoration, and climate change adaptation.
Data Availability Statement
The raw data supporting the conclusions of this manuscript will be made available by the authors, without undue reservation, to any qualified researcher.
Author Contributions
SM-N performed all data collection, statistical analysis, and manuscript preparation. RK provided greenhouse and laboratory resources, extra student help, and feedback on the manuscript.
Funding
This work was supported by the USDA National Institute of Food and Agriculture through MSU AgBioResearch (Hatch MICL02611) and the National Science Foundation (DEB 0958943).
Conflict of Interest
The authors declare that the research was conducted in the absence of any commercial or financial relationships that could be construed as a potential conflict of interest.
Acknowledgments
The authors are grateful to Dr. Ines Ibáñez and David Minor for help with the Bayesian survival analysis.
Supplementary Material
The Supplementary Material for this article can be found online at: https://www.frontiersin.org/articles/10.3389/fevo.2019.00383/full#supplementary-material
References
Al-Azizi, A., Al-Sadi, A. M., Dietz, H., Al-Said, F. A., and Deadman, M L. (2013). Influence of carbon/nitrogen ratio on Pythium aphanidermatium and on Pythium-induced damping-off of radish. J. Plant Path. 95, 181–185. doi: 10.4454/JPP.V95I1.011
Alvarez-Loayza, P., and Terborgh, J. (2011). Fates of seedling carpets in an Amazonian floodplain forest: intra-cohort competition or attack by enemies? J. Ecol. 99, 1045–1054. doi: 10.1111/j.1365-2745.2011.01835.x
Andersen, P. K., and Gil, R. D. (1982). Cox's regression model for counting processes: a large sample study. Ann. Stat. 10, 1100–1120. doi: 10.1214/aos/1176345976
Augspurger, C. K. (1990). “Spatial patterns of damping-off disease during seedling recruitment in tropical forests,” in Pests, Pathogens, and Plant Communities, eds J. Burdon, and S. Leather (Oxford: Blackwell Scientific Publications, 131–144.
Baribault, T. W., Kobe, R. K., and Rothstein, D. E. (2010). Soil calcium, nitrogen, and water are correlated with aboveground net primary production in northern hardwood forests. Forest Ecol. Manag. 260, 723–733. doi: 10.1016/j.foreco.2010.05.029
Benítez, M. S., Hersh, M. H., Vilgalys, R., and Clark, J. S. (2013). Pathogen regulation of plant diversity via effective specialization. Trends Ecol. Evolut. 28, 705–711. doi: 10.1016/j.tree.2013.09.005
Bennett, J. A., and Klrionomos, J. (2019). Mechanisms of plant-soil feedback: interactions among biotic and abiotic drivers. New Phytol. 222, 91–96. doi: 10.1111/nph.15603
Bennett, J. A., Maherali, H., Reinhart, K. O., Lekberg, Y., Hart, M. M., and Klironomos, J. (2017). Plant–soil feedbacks and mycorrhizal type influence temperate forest population dynamics. Science 355, 181–184. doi: 10.1126/science.aai8212
Bever, J. D. (2003). Soil community feedback and the coexistence of competitors: conceptual frameworks and empirical tests. New Phytol. 157, 465–473. doi: 10.1046/j.1469-8137.2003.00714.x
Bever, J. D., Platt, T. G., and Morton, E. R. (2012). Microbial population and community dynamics on plant roots and their feedbacks on plant communities. Annu. Rev. Microbiol. 66, 265–283. doi: 10.1146/annurev-micro-092611-150107
Bever, J. D., Westover, K. M., and Antonovics, J. (1997). Incorporating the soil community into plant population dynamics: the utility of the feedback approach. J. Ecol. 85, 561–573. doi: 10.2307/2960528
Bezemer, T. M., Jing, J. J., Bakx-Schotman, J. M. T., and Bijleveld, E. J. (2018). Plant competition alters the temporal dynamics of plant-soil feedbacks. J. Ecol. 106, 2287–2300. doi: 10.1111/1365-2745.12999
Bezemer, T. M., Van der Putten, W. H., Martens, H., Van de Voorde, T. F. J., Mulder, P. P. J., and Kostenko, O. (2013). Above- and below-ground herbivory effects on below-ground plant–fungus interactions and plant–soil feedback responses. J. Ecol. 101, 325–333. doi: 10.1111/1365-2745.12045
Bonanomi, G., Gaglione, S. A., Incerti, G., and Zoina, A. (2013). Biochemical quality of organic amendments affects soil fungistasis. Appl. Soil Ecol. 72, 135–142. doi: 10.1016/j.apsoil.2013.06.007
Bonanomi, G., Lorito, M., Vinale, F., and Woo, S. L. (2018). Organic amendments, beneficial microbes, and soil microbiota: towards a unified framework for disease suppression. Annu. Rev. Phytopathol. 56, 1–20. doi: 10.1146/annurev-phyto-080615-100046
Brinkman, P. E., Van der Putten, W. H., Bakker, E. J., and Verhoeven, K. J. F. (2010). Plant-soil feedback: experimental approaches, statistical analyses and ecological interpretations. J. Ecol. 98, 1063–1073. doi: 10.1111/j.1365-2745.2010.01695.x
Casper, B. B., and Castelli, J. P. (2007). Evaluating plant-soil feedback together with competition in a serpentine grassland. Ecol. Lett. 10, 394–400. doi: 10.1111/j.1461-0248.2007.01030.x
Clark, J. S. (2005). Why environmental scientists are becoming Bayesians. Ecol. Lett. 8, 2–14. doi: 10.1111/j.1461-0248.2004.00702.x
Comita, L. S., Muller-Landau, H. C., Aguilar, S., and Hubbell, S. P. (2010). Asymmetric density dependence shapes species abundances in a tropical tree community. Science 329, 330–332. doi: 10.1126/science.1190772
Connell, J. H. (1971). “On the role of natural enemies in preventing competitive exclusion in some marine animals and in rain forest trees” in Dynamics of Populations, eds P. J. Den Boer and G. R. Gradwell (Wageningen: Centre for Agricultural Publishing and Documentation, 298–312.
Crawford, K. M., Bauer, J. T., Comita, L. S., Eppinga, M. B., Johnson, D. J., Mangan, S. A., et al. (2019). When and where plant-soil feedback may promote plant coexistence: a meta-analysis. Ecol. Lett. 22, 1274–1284. doi: 10.1111/ele.13278
Dickie, I. A., Koele, N., Blum, J. D., Gleason, J. D., and McGlone, M. S. (2014). Mycorrhizas in changing ecosystems. Botany 92, 149–160. doi: 10.1139/cjb-2013-0091
Grman, E., Robinson, T. M. P., and Klausmeier, C. A. (2012). Ecological specialization and trade affect the outcome of negotiations in mutualism. Am. Nat. 179, 567–581. doi: 10.1086/665006
Gurevitch, J. S., Scheiner, M., and Fox, G. A. (2006). The Ecology of Plants, 2nd Edn. Sunderland, MA: Sinauer Associates.
Harrison, K. A., and Bardgett, R. D. (2010). Influence of plant species and soil conditions on plant-soil feedback in mixed grassland communities. J. Ecol. 98, 384–395. doi: 10.1111/j.1365-2745.2009.01614.x
Heinze, J., and Joshi, J. (2018). Plant-soil feedback can be masked by aboveground herbivory under natural conditions. Oecologia 186, 236–246. doi: 10.1007/s00442-017-3997-y
Hersh, M. H., Vilgalys, R., and Clark, J. S. (2012). Evaluating the impacts of multiple generalist fungal pathogens on temperate tree seedling survival. Ecology 93, 511–520. doi: 10.1890/11-0598.1
HilleRisLambers, J., Clark, J. S., and Beckage, B. (2002). Density-dependent mortality and the latitudinal gradient in species diversity. Nature 417, 732–735. doi: 10.1038/nature00809
Ibáñez, I., and McCarthy-Neumann, S. (2016). Effects of mycorrhizal fungi on tree seedling growth: quantifying the parasitism-mutualism transition along a light gradient. Can. J. For. Res. 46, 48–57. doi: 10.1139/cjfr-2015-0327
Ichihara, Y., and Yamaji, K. (2009). Effect of light conditions on the resistance of current-year Fagus crenata seedlings against fungal pathogens causing damping-off in a natural beech forest: fungus isolation and histological and chemical resistance. J. Chem. Ecol. 35, 1077–1085. doi: 10.1007/s10886-009-9687-4
in 't Zandt, D., van den Brink, A., de Kroon, H., and Visser, E. J. W. (2019). Plant-soil feedback is shut down when nutrients come to town. Plant Soil 439, 541–551. doi: 10.1007/s11104-019-04050-9
Janzen, D. H. (1970). Herbivores and the number of tree species in tropical forests. Am. Nat. 104, 501–528. doi: 10.1086/282687
Johnson, D. J., Beaulieu, W. T., Bever, J. D., and Clay, K. (2012). Conspecific negative density dependence and forest diversity. Science 336, 904–907. doi: 10.1126/science.1220269
Johnson, N. C., Graham, J. H., and Smith, F. A. (1997). Functioning of mycorrhizal associations along the mutualism-parasitism continuum. New Phytol. 135, 575–586. doi: 10.1046/j.1469-8137.1997.00729.x
Jung, S. C., Martnex-Medina, A., Lopex-Raex, J. A., and Pozo, M. J. (2012). Mycorrhiza-induced resistance and priming of plant defenses. J. Chem. Ecol. 38, 651–664. doi: 10.1007/s10886-012-0134-6
Kinney, K. K., Lindroth, R. L., Jung, S. M., and Nordheim, E. V. (1997). Effects of CO2 and availability on deciduous trees: phytochemistry and insect performance. Ecology 78, 215–230. doi: 10.1890/0012-9658(1997)078[0215:EOCANA]2.0.CO;2
Klironomos, J. N. (2002). Feedback with soil biota contributes to plant rarity and invasiveness in communities. Nature 417, 67–70. doi: 10.1038/417067a
Kobe, R. K. (1996). Intraspecific variation in sapling mortality and growth predicts geographic variation in forest composition. Ecol. Monogra. 66, 181–201. doi: 10.2307/2963474
Kobe, R. K., Iyer, M., and Walters, M. B. (2010). Optimal partitioning theory revisited: nonstructural carbohydrates dominate root mass responses to nitrogen. Ecology 91, 166–179. doi: 10.1890/09-0027.1
Kulmatiski, A., Beard, K. H., Stevens, J. R., and Cobbold, S. M. (2008). Plant–soil feedbacks: a meta-analytical review. Ecol. Lett. 11, 980–992. doi: 10.1111/j.1461-0248.2008.01209.x
LaManna, J. A., Walton, M. L., Turner, B. L., and Myers, J. A. (2016). Negative density dependence is stronger in resource-rich environments and diversifies communities when stronger for common but not rare species. Ecol. Lett. 19, 657–667, doi: 10.1111/ele.12603
Lekberg, Y., Bever, J. D., Bunn, R. A., Callaway, R. M., Hart, M. M., Kivlin, S. N., et al. (2018). Relative importance of competition and plant-sol feedback, their synergy, context dependency and implications for coexistence. Ecol. Lett. 21, 1268–1281. doi: 10.1111/ele.13093
Liu, Y., Fang, S., Chesson, P., and He, F. (2015). The effect of soil-borne pathogens depends on the abundance of host tree species. Nat. Comun. 6:10017. doi: 10.1038/ncomms10017
MacDougall, A. S., Rillig, M. C., and Klironomos, J. N. (2011). Weak conspecific feedbacks and exotic dominance in a species-rich savannah. Proc. R. Soc. Lond. 278, 2939–2945. doi: 10.1098/rspb.2010.2730
Mangan, S. A., Schnitzer, S. A., Herre, E. A., Mack, K., Valencia, M., Sanchez, E., et al. (2010). Negative plant-soil feedback predicts tree-species relative species abundance in a tropical forest. Nature 466, 752–755. doi: 10.1038/nature09273
Mazzoleni, S., Bonanomi, G., Incerti, G., Chiusano, M. L., Termolino, P., Mingo, A., et al. (2015). Inhibitory and toxic effects of extracellular self-DNA in litter: a mechanism for negative plant–soil feedbacks? New Phytol. 205, 1195–1210. doi: 10.1111/nph.13121
McCarthy-Neumann, S., and Ibáñez, I. (2012). Tree range expansion may be enhanced by escape from negative plant-soil feedbacks. Ecology 93, 2637–2649. doi: 10.1890/11-2281.1
McCarthy-Neumann, S., and Ibáñez, I. (2013). Plant–soil feedback link negative distance dependence and light gradient partitioning during tree seedling establishment. Ecology 94, 780–786. doi: 10.1890/12-1338.1
McCarthy-Neumann, S., and Kobe, R. K. (2008). Tolerance of soil pathogens co-varies with shade tolerance across species of tropical tree seedlings. Ecology 89, 1883–1892. doi: 10.1890/07-0211.1
McCarthy-Neumann, S., and Kobe, R. K. (2010). Conspecific plant-soil feedbacks reduce survivorship and growth of tropical tree seedlings. J. Ecol. 98, 396–407. doi: 10.1111/j.1365-2745.2009.01619.x
Myers, J. A., and Kitajima, K. (2007). Carbohydrate storage enhances seedling shade tolerance in a neotropical forest. J. Ecol. 95, 383–395. doi: 10.1111/j.1365-2745.2006.01207.x
Palmer, M. W. (1994). Variation in species richness: towards a unification of hypotheses. Folia Geobot. Phytotax. 29, 511–530. doi: 10.1007/BF02883148
Pane, C., Spaccini, R., Piccolo, A., Scala, F., and Bonanomi, G. (2011). Compost amendments enhance peat suppressiveness to Pythium ultimum, Rhizoctonia solani and Sclerotinia minor. Biol. Control 56, 115–124. doi: 10.1016/j.biocontrol.2010.10.002
Peltzer, D. A., and Wardle, D. A. (2016). Soil fertility effects on tree seedling performance are light-dependent: evidence from a long-term chronosequence. Oikos 125, 1121–1133. doi: 10.1111/oik.02878
Petermann, J. S., Fergus, A. J. F., Turnbull, L. A., and Schmid, B. (2008). Janzen-Connell effects are widespread and strong enough to maintain diversity in grasslands. Ecology 89, 2399–2406. doi: 10.1890/07-2056.1
Reinhart, K. O., and Rinella, M. J. (2016). A common soil handling technique can generate incorrect estimates of soil biota effects on plants. New Phytol. 210, 786–789. doi: 10.1111/nph.13822
Reinhart, K. O., Royo, A. A., Kageyama, S. A., and Clay, K. (2010). Canopy gaps decrease microbial densities and disease risk for a shade-intolerant tree species. Acta Oecol. 36, 530–536. doi: 10.1016/j.actao.2010.07.006
Reinhart, K. O., Royo, A. A., Van der Putten, W. H., and Clay, K. (2005). Soil feedback and pathogen activity in Prunus serotina throughout its native range. J. Ecol. 93, 890–898. doi: 10.1111/j.1365-2745.2005.01028.x
Revillini, D., Gehring, C. A., and Johnson, N. A. (2016). The role of locally adapted mycorrhizas and rhizobacteria in plant-soil feedback systems. Funct. Ecol. 30, 1086–1098. doi: 10.1111/1365-2435.12668
Schreeg, L. A., Kobe, R. K., and Walters, M. B. (2005). Tree seedling growth, survival and morphology in response to landscape-level variation in soil resource availability in Northern Michigan. Can. J. For. Res. 35, 263–273. doi: 10.1139/x04-168
Smith, L. M., and Reynolds, H. L. (2015). Plant–soil feedbacks shift from negative to positive with decreasing light in forest understory species. Ecology 96, 2523–2532. doi: 10.1890/14-2150.1
Smith-Ramesh, L. M., and Reynolds, H. L. (2017). The next frontier of plant–soil feedback research: unraveling context dependence across biotic and abiotic gradients. J. Veg. Soc. 28, 484–494. doi: 10.1111/jvs.12519
Swamy, V., Terborgh, J., Dexter, K. G., Best, B. D., Alvarez, P., and Cornejo, F. (2011). Are all seeds equal? Spatially explicit comparisons of seed fall and sapling recruitment in a tropical forest. Ecol. Lett. 14, 195–201. doi: 10.1111/j.1461-0248.2010.01571.x
Terborgh, J. (2012). Enemies maintain hyperdiverse tropical forests. Am. Nat. 179, 303–314. doi: 10.1086/664183
Troelstra, S. R., Wagenaar, R., Smant, W., and Peters, B. A. M. (2001). Interpretation of bioassays in the study of interactions between soil organisms and plants: involvement of nutrient factors. New Phytol. 150, 697–706. doi: 10.1046/j.1469-8137.2001.00133.x
Wei, W., Yang, M., Liu, Y. X., Huang, H. C., Ye, C., Cheng, J. F., et al. (2018). Fertilizer N application rate impacts plant-soil feedback in a sanqi production system. Sci.Total Environ. 633, 796–897. doi: 10.1016/j.scitotenv.2018.03.219
Xu, M., Wang, Y., and Yu, S. (2015). Conspecific negative density dependence decreases with increasing species abundance. Ecosphere 6, 1–11. doi: 10.1890/ES15-00144.1
Keywords: C:N, light availability, NH4+, plant-soil feedback, Prunus serotina, Quercus rubra, soil fertility, temperate forest
Citation: McCarthy-Neumann S and Kobe RK (2019) Site Soil-Fertility and Light Availability Influence Plant-Soil Feedback. Front. Ecol. Evol. 7:383. doi: 10.3389/fevo.2019.00383
Received: 19 July 2019; Accepted: 24 September 2019;
Published: 10 October 2019.
Edited by:
Martijn Bezemer, Netherlands Institute of Ecology (NIOO-KNAW), NetherlandsReviewed by:
Kerri Crawford, University of Houston, United StatesDina In 'T Zandt, Radboud University Nijmegen, Netherlands
Copyright © 2019 McCarthy-Neumann and Kobe. This is an open-access article distributed under the terms of the Creative Commons Attribution License (CC BY). The use, distribution or reproduction in other forums is permitted, provided the original author(s) and the copyright owner(s) are credited and that the original publication in this journal is cited, in accordance with accepted academic practice. No use, distribution or reproduction is permitted which does not comply with these terms.
*Correspondence: Sarah McCarthy-Neumann, bmV1bWFubnNtJiN4MDAwNDA7YWxtYS5lZHU=