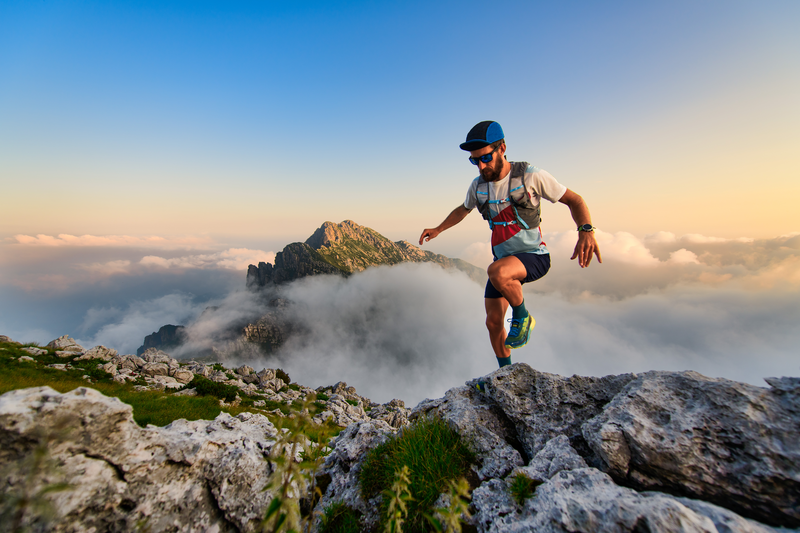
94% of researchers rate our articles as excellent or good
Learn more about the work of our research integrity team to safeguard the quality of each article we publish.
Find out more
ORIGINAL RESEARCH article
Front. Ecol. Evol. , 11 October 2019
Sec. Conservation and Restoration Ecology
Volume 7 - 2019 | https://doi.org/10.3389/fevo.2019.00373
Habitat loss and fragmentation rank high amongst the most pressing threats to biodiversity. Understanding how variation in functional traits is associated with species vulnerability in fragmented landscapes is central to the design of effective conservation strategies. Here, we used a whole-ecosystem ecological experiment in the Central Amazon to investigate which functional traits of aerial-hawking insectivorous bats best predict their sensitivity to forest fragmentation. During 2014, bats were surveyed using passive bat recorders in six continuous forest sites, eight forest fragments, eight fragment edges, and eight forest clearings. The interaction between functional traits, environmental characteristics, and species distribution was investigated using a combination of RLQ and fourth-corner analyses. Our results showed that echolocation call structure, vertical stratification, and wing aspect ratio were the strongest predictors of sensitivity to forest fragmentation. Frequency of maximum energy, body mass, and relative wing loading did not show any correlation with the environmental variables. Bat species with constant-frequency calls were associated with high vegetation density, being more susceptible to forest fragmentation than species with frequency-modulated calls. Vertical stratum preference was also correlated with vegetation structure, indicating that understory species were more sensitive to forest loss than canopy species. Finally, species with high aspect ratio wings were linked to forest edges and clearings. Our findings suggest that species functional traits determine the vulnerability of aerial-hawking insectivorous bats toward fragmentation and, similarly, environmental conditions determine if a species is likely to become locally extinct due to fragmentation. Preserving structurally complex forests will be crucial to ensure the long-term persistence of the most sensitive and vulnerable species of this bat ensemble in fragmented landscapes across the Neotropics.
The exponential increase of the human population and growing per capita consumption of resources are causing widespread habitat loss and degradation, which are threatening the survival of numerous species worldwide (Dirzo et al., 2014; Laurance et al., 2014). In fact, human-caused environmental changes are among the most significant and immediate threats to biodiversity (Ewers and Didham, 2006; Dobrovolski et al., 2011; Haddad et al., 2015), and their impacts on biota are predicted to be more pronounced in species-rich tropical areas (Malhi et al., 2014). Across the tropics, human-induced habitat modification is creating increasingly fragmented landscapes composed of smaller and isolated primary habitat patches (Haddad et al., 2015; Taubert et al., 2018), usually embedded in a matrix that is often a mosaic of different habitats that affect both species diversity and composition (Ewers and Didham, 2006; Kupfer et al., 2006; Kennedy et al., 2011; Loureiro and Gregorin, 2015). Matrix quality and permeability are key determinants of biodiversity persistence in degraded landscapes (Ewers and Didham, 2006; Kennedy et al., 2011; Mendenhall et al., 2014; Rocha et al., 2018). Matrix permeability refers to the capacity of any landscape to promote movement of animal populations and to sustain key ecological services and processes (Ray et al., 2002). In general, low matrix permeability negatively affects local biodiversity as it reduces connectivity and increases the effects of isolation of remnant patches (Ricketts, 2001; Estrada-Villegas et al., 2010).
Understanding how habitat alterations affect biodiversity and ecosystem functioning is crucial for the creation of effective conservation strategies (Cadotte et al., 2011). Since most ecological processes are determined by species functional traits (Cardinale et al., 2012), trait-based approaches have lately become popular to predict the anthropogenic effects on ecosystem functioning in fragmented tropical landscapes. Functional traits are measurable characteristics of organisms or species (morphological, physiological, phenological, or behavioral) that determine their performance, fitness, and ecological functions in a particular habitat (Violle et al., 2007). Because some species are more susceptible to habitat loss than others (Davies et al., 2000; Henle et al., 2004), identifying which traits make species more sensitive to habitat fragmentation is essential to minimize local extinctions (Barbaro and Van Halder, 2009; Hanspach et al., 2012).
The tropics harbor the greatest diversity of bats (Mickleburgh et al., 2002; Altringham, 2011), which fulfill key ecological functions. They act as pollinators and seed dispersers of a large number of plant species, promoting the restoration of forest in disturbed areas. Moreover, they are both important prey and biological control agents of animal populations (Kunz et al., 2011). The recent increase in published studies on tropical bats has considerably advanced our knowledge about the effects of habitat loss and fragmentation on this taxon at different ecological levels (Fenton, 1997). However, most studies have analyzed biodiversity changes from a taxonomic point of view, with few focusing on a functional trait-based dimension (Farneda et al., 2015, 2018a; but see Cisneros et al., 2015; Frank et al., 2017; Wordley et al., 2017).
In the Neotropics, aerial-hawking insectivorous bats (hereafter AIB) represent 30-50% of local bat assemblages (Kalko, 1998; MacSwiney et al., 2008; Estrada-Villegas et al., 2010). Although AIB are usually classified into one ensemble, they exhibit high variability in foraging and behavioral strategies. However, AIB so far have rarely been considered in fragmentation studies as most of them relied on mist-net captures, a sampling method poorly suited for this group (Estrada-Villegas et al., 2010; Silva and Bernard, 2017). Although acoustic methods are the most suitable to sample AIB, the costs and time commitment involved in acoustic surveys is still considerable. Moreover, the echolocation calls of many tropical species and the variation among them have not yet been adequately documented, and reference call libraries for tropical regions are scarce (MacSwiney et al., 2008; López-Baucells et al., 2016). As a result of these limitations, AIB continue to be underrepresented in inventories and ecological studies (Cunto and Bernard, 2012), and there is a lack of data about their vulnerability to habitat fragmentation (but see Estrada-Villegas et al., 2010; Bader et al., 2015a,b). Moreover, other ecological characteristics like hunting strategies remain poorly studied (Marques et al., 2013). Despite the aforementioned limitations, the recent improvement of acoustic recording devices and new data analysis approaches have stimulated the inclusion of AIB in surveys and monitoring programmes (Browning et al., 2017).
Here, we used an ecosystem-wide fragmentation experiment in the Brazilian Amazon to investigate which functional traits are linked to fragmentation sensitivity in AIB. We employed the analytical framework introduced by Dray et al. (2014) that integrates RLQ and fourth-corner methods to examine trait-environment links and their association with patterns of species distribution. RLQ is a multivariate ordination technique that summarizes the joint structure among three data tables (R—environmental characteristics, L—species abundances, Q—species traits), whereas the fourth-corner method tests for trait–environment relationships (Dray et al., 2014).
We predicted that: (i) species with high frequency calls are more sensitive to fragmentation than those with low frequency calls; (ii) the main echolocation call types (modulated/quasi-constant/constant frequency calls) are related to species-specific fragmentation sensitivity; (iii) fragmentation filters bat assemblages according to species' morphological and flight-related traits such as body size or wing morphology; and (iv) preferences with regard to vertical foraging stratum influence the adaptability of bats to deforestation.
The study was conducted at the Biological Dynamics of Forest Fragments Project (BDFFP), located in the Central Amazon, approximately 80 km north of Manaus (2°30′S, 60°W), Brazil (Figure 1). Commencing in 1979, the BDFFP is the longest-running experimental study of habitat fragmentation (Laurance et al., 2018). The study area covers 1,000 km2 of moist tropical forest characterized by a mosaic of primary terra firme rainforest and primary forest fragments (1, 10, and 100 ha) embedded in a matrix of late-stage secondary regrowth (~30 years old). In the early 1980s, the region underwent an intense process of forest clearing, resulting in a set of fragments of primary forest isolated from continuous forest by distances of 80–650 m. Since their creation, these experimental fragments were re-isolated 4 to 5 times by clearing a 100 m-wide strip around each fragment. The most recent re-isolation occurred in 2013–2014, resulting in a landscape comprised of both forest-dominated habitats and small man-made clearings around the fragments (Rocha et al., 2017c). Yearly rainfall fluctuates between 1,900 and 3,500 mm, with a dry season from June to October and a wet season from November to June (Ferreira et al., 2017). Forest canopy height varies from 30 to 37 m, with some emergent trees reaching 55 m (Laurance et al., 2011).
Figure 1. Map of the study area in the central Amazon. Image © DigitalGlobe/CNES/Airbus. Experimental areas within the BDFFP landscape are delineated by a white border (Km 41 and Cabo Frio for continuous forest and Dimona, Colosso, and Porto Alegre for forest fragments); continuous forest is represented in light blue and secondary vegetation matrix in dark blue. The image in the upper right corner shows the location of the study area.
Our experimental design was constrained by the overall landscape context and idiosyncrasies of the BDFFP, especially in terms of the number and locations of fragments and continuous forest sites, which precluded us from following a paired study design, i.e., pairing fragments with nearby continuous forest sites. Although the BDFFP landscape is not ideal in every respect from an experimental design perspective, environmental conditions across the study landscape can be considered sufficiently similar (Laurance et al., 2018). We therefore believe the influence of potentially confounding environmental variables on our results to be negligible, especially in view of the high vagility of the study taxon.
Acoustic surveys were carried out in eight forest fragments during the first year after the re-isolation that took place in 2013–2014 (Rocha et al., 2017c), and in six control sites in continuous primary forest (Figure 1). Automatic bat recorders Song Meter 2 (SM2Bat+) equipped with omnidirectional ultrasonic SMX-US microphones (Wildlife Acoustics, Inc. Massachusetts, USA) were used to acoustically sample bats. Recorders were placed at 1.5 m height, in the interiors (N = 8) and edges (N = 8) of all forest fragments, as well as in the adjacent clearings (N = 8) (for more information regarding the experimental re-isolation of the forest fragments see Rocha et al., 2017c). With the six recorders placed in the interior of continuous forest we had a total of 30 sampling sites. Detectors were installed for three consecutive nights per recording site (from 18:00 to 6:00 h), and all sites were sampled twice during both the dry and wet season in 2014.
Kaleidoscope Pro Software v4.0.4 (Wildlife Acoustics, In. Massachusetts, USA) was used to manually classify all recordings based on López-Baucells et al. (2016, 2019) and a regional reference call library compiled by A. López-Baucells (unpublished). In the case of 14 species it was possible to identify the calls to species level. However, some species had to be grouped into sonotypes (comprising several species with similar calls that could not be reliably distinguished, N = 5; see Table S2). Owing to inherent constraints of acoustic data (i.e., the fact that it is not possible to count individuals), we used “bat activity” as a proxy for bat abundance. The sample unit was a bat pass (Wickramasinghe et al., 2003), which we considered as any sequence/recording with a minimum of two distinguishable echolocation pulses per species during a maximum time of 5 s (Millon et al., 2015). We only considered those pulses whose intensity exceeded 10 dB from the background noise. Data from all fragments were pooled together independently of their size within the habitat category “fragment,” due to the limited number of replicates.
Vegetation structure was assessed at each sampling site within three plots of 100 m2 (5 × 20 m) located next to the detector locations. Four environmental variables were quantified: number of stems, tree height (height in m of all trees ≥10 cm diameter at breast height), shortest distance to water (distance in km between each site and the nearest water body calculated using Google Earth), and habitat type (qualitative variable including four categories: continuous forest interior, fragment interior and fragment edge, clearing).
The relative scarcity of knowledge on AIB, together with the difficulty of gathering reliable information about most of the species, led us to not consider traits such as diet or roosting strategy/guild (Borkin and Parsons, 2011; Bohnenstengel, 2012; Farneda et al., 2015; Coronado et al., 2018), and to focus instead on only six functional traits as potential predictors of AIB vulnerability to habitat fragmentation (Table 1):
Table 1. List of functional traits used as predictors of aerial-hawking insectivorous bat vulnerability to habitat fragmentation in the central Amazon.
Frequency of maximum energy (FME), also known as peak frequency, is the most intense frequency in the call (López-Baucells et al., 2016). Amongst other environmental and acoustic factors, it determines how quickly the intensity of a call will diminish and therefore, will influence the kind of habitat in which the bat will be able to fly and forage. Data on FME for all species were obtained from López-Baucells et al. (2016). Whenever species with similar peak frequencies had to be grouped under the same sonotype, the average FME of all species was calculated and used in subsequent analyses.
This predictor was based on an existing guild classification of bats that links habitat type and foraging mode with their echolocation calls (Schnitzler and Kalko, 2001; Schnitzler et al., 2003). Following this, we established the following categories: (A) frequency-modulated signals (FM): usually emitted by cluttered space foragers; (B) frequency modulated—quasi-constant frequency signals (FM-QCF): typical of edge-foraging species; (C) quasi-constant frequency signals (QCF): mostly emitted by open space foragers; and (D) constant frequency signals (CF): emitted by cluttered space foragers. Species were assigned to each category following López-Baucells et al. (2016).
Body mass plays a key role in the physiology, ecology, and diversification of species and also influences life-history traits of individuals (Safi et al., 2013). Species with large body size tend to have smaller populations and larger home ranges and are therefore more prone to extinction (Purvis et al., 2000). Body mass was measured in captured bats (data collected at the BDFFP between 2012 and 2014; Rocha et al., 2017b,c) and averaged for each species (excluding juveniles and reproductive females). Missing data for Molossops spp., Peropteryx kappleri and P. macrotis, Promops spp., and Pteronotus personatus were obtained from the literature (Eisenberg and Redford, 1989).
Body size has been widely used in trait-based analyses due to its influence on the physiology, ecology, and morphology of organisms (Peters, 1986; Penone et al., 2018). Larger bats tend to emit lower frequency calls and typically forage in more open habitats, while smaller species use higher frequency signals that allow them to move in cluttered spaces (Kalko and Schnitzler, 1993; Thiagavel et al., 2017; Penone et al., 2018). We used forearm length as a measure of body size. Data was obtained from our own data and the literature (Gardner, 2008).
Bat activity and richness differ among tropical forest strata, whereby species are generally divided into canopy, sub-canopy, or understory specialists. However, this classification is mostly based on studies on phyllostomid bats (Carvalho et al., 2013; Marques, 2016). We assigned species to three different strata based on Mas (2014), who studied vertical stratification at the BDFFP using acoustic data: (1) more recordings in the understory than in the other strata, (2) bats flying significantly more often between 20 and 30 m but still under the canopy (sub-canopy), and (3) bats flying above the canopy.
Maneuverability and flight speed in bats are constrained by wing morphology (Marinello and Bernard, 2014), which usually determines access to suitable foraging habitat (Arita and Fenton, 1997). We calculated aspect ratio and relative wing loading of each species following Norberg and Rayner (1987). Measurements were obtained from extended-wing photographs of bats captured at the BDFFP (Rocha et al., 2017b,c; Torrent et al., 2018) and analyzed with ImageJ (National Institute of Health, USA). Missing data were obtained from the literature (see references in Table 1).
Several analytical methods have historically been used to assess the associations between species traits and environmental gradients (Peres-Neto et al., 2017). Amongst them, RLQ and fourth-corner analysis are considered excellent approaches to assess trait–environment relationships at the species level and have been widely used in ecological and conservation research (Kleyer et al., 2012). Dray et al. (2014) recently developed an analytical framework that combines both methods and so far is considered the most appropriate approach to study the relation between environmental variables and functional traits. Applied to our dataset, it tests the relation of the data contained in three different matrices: table L (species activity levels per sampling site), table Q (functional traits of species) and table R (environmental variables per sampling site). A correspondence analysis (CA) is applied to table L, providing a common organization of the samples and the species (Dray et al., 2014). Both the table of environmental variables (R) and traits (Q) contained a mix of qualitative and quantitative variables and were therefore analyzed using Hill-Smith principal component analysis (PCA; Hill and Smith, 1976). The RLQ method maximizes the covariance between the traits and the environmental variables through the species relative abundance (Dray et al., 2014). While RLQ consists of a multivariate analysis of the associations between the three tables, the fourth-corner method tests the significance of bivariate associations. We used two permutation models in the RLQ-fourth corner analysis. Model 2 tests the null hypothesis that there is no relationship between the distribution of species and environmental conditions (i.e., no association between R and L matrices). In contrast, model 4 assumes no association between L and Q and the null hypothesis here is that species composition is independent of species traits (Dray et al., 2014). To adjust p-values for multiple comparisons, we used the false discovery method (Benjamini and Hochberg, 1995; Dray et al., 2014), determining significance based on 50,000 permutations. Given the ecological focus of our study and following the recommendations by De Bello et al. (2015) regarding when to apply phylogenetic corrections in functional trait-based analyses, we decided not to incorporate phylogenetic relatedness. All calculations were carried out using R v3.4.1 (R Core Team, 2017), specifically package ade4 (Dray and Dufour, 2007).
The RLQ analysis showed that the first axis of the ordination (considering the three tables R, L, and Q) explained 87.6% of the variance and the second axis 10.2%. The first and second axes of the Hill-Smith PCA of the functional traits table (Q table) accounted for 35.6 and 28.4% of the variance respectively. Regarding the R table (environmental variables), the first axis of the PCA explained 48.3% of the variance, and the second axis 23.1%. Finally, in the L table (species activity) the first axis of the correspondence analysis (CA) explained 43.1% of the variance and the second 15.3% (Table 2).
In the PCA of the R table (Figure 2), sites grouped according to the gradient made up by the different habitat categories, with all forest interior sites (continuous forest and forest fragments) well-separated from clearings and fragment edges along the first axis. In the PCA of the Q ordination table (Figure 3), no clear species groupings could be distinguished. However, all species were mostly spatially organized based on three of their functional traits, call structure (CF or FM calls), forearm length (FA) and frequency of maximum energy (FME). The eigenvalues of the first axis of the RLQ analysis from species traits and environmental variables (Figure 4) showed that the variables that explained most variability in species occurrence among sites were call structure (CF) and vertical stratification, and two categories of the habitat type variable, forest fragment and continuous forest.
Figure 2. Biplots from the Hill-Smith PCA of the environmental gradients (R table). (A) Environmental variables. (B) Sites. C, clearing; FF, forest fragment; CF, continuous forest; E, edge.
Figure 3. Biplots from the Hill-Smith PCA of the functional traits (Q table). (A) Species. Red, Mormoopidae; Pink, Furipteridae; Green, Vespertilionidae; Orange, Emballonuridae; Blue, Molossidae (see Table S1 for species abbreviations). (B) Traits (see Table 1 for trait abbreviations).
Figure 4. Eigenvalues of species traits and environmental variables along the first axis of the RLQ analysis. See Table 1 for trait abbreviations.
Fourth corner analysis revealed a highly significant relationship between species distribution and environmental variables (model 2, p < 0.0001), and a non-significant association between species composition and functional traits (model 4, p = 0.17). Based on 50,000 iterations, the total inertia of the RLQ analysis indicates a non-significant general relation (p = 0.17) between environmental variables and functional traits in the combined models 2 and 4.
Finally, the combination of RLQ and fourth-corner analysis yielded three significant (p < 0.05) trait-environment relationships (Figure S1): CF call structure showed a positive correlation with the first axis (AxR1) for the environmental gradient; vertical stratification revealed a negative correlation with the AxR1 axis for the environmental gradient; and the assemblages recorded in the forest fragments were significantly associated with the AxQ1 axis that represented the pool of bat functional traits.
The diversity of species functional traits in a given assemblage is a key predictor of an ecosystem's functioning, resistance, and resilience (Petchey and Gaston, 2006), and therefore, functional traits are also likely to influence the vulnerability of species to the ongoing process of habitat destruction and fragmentation. However, trait-mediated environmental filters are still seldom considered in studies based on human-modified tropical landscapes. This is especially the case for poorly known animal groups, such as AIB. We used autonomous bat detectors and a modern statistical approach to identify how the traits of Neotropical aerial-hawking insectivorous bats correlate with environmental features and species distributions in a fragmented landscape. Our results revealed that some traits were significantly linked to environmental variables, potentially affecting species vulnerability to habitat fragmentation. We found a strong association between echolocation call type with features of AIB foraging habitat that are likely to be affected by forest fragmentation.
The responses of phyllostomid bats to habitat modification have been extensively studied across the Neotropics (Meyer et al., 2016; Frank et al., 2017) and in particular at the BDFFP, where recent long-term studies have provided important insights into the dynamics of bat responses to landscape fragmentation associated with matrix regeneration (Farneda et al., 2018a,b; Rocha et al., 2018). Farneda et al. (2015) found that, in the case of Amazonian phyllostomid bats, body mass and trophic level are good predictors of fragmentation sensitivity. However, this issue is poorly studied in AIB, and the present study is one of the few investigating the link between these species' traits and vulnerability to fragmentation.
Although bat echolocation calls are specifically adapted to different habitat types (Schnitzler et al., 2003), only a few studies have analyzed the correlation between echolocation traits and habitat characteristics (but see Wordley et al., 2017). These authors linked echolocation and morphological traits to habitat use in the Paleotropics and found that functional traits filtered bat assemblages in tea plantations.
A recent study tested the relation between echolocation traits and forest structure as quantified through LiDAR technology (Blakey et al., 2017). In accordance with our results, the authors did not find a significant correlation between forest structure variables and frequency of maximum energy. Similarly, Frank et al. (2017) found that this echolocation parameter was not predictive of a bat's habitat usage. Although Wordley et al. (2017) found bats with highest FME to be mostly affiliated with forests, they suggest that this variable might be less useful than others to predict bat vulnerability as bat call libraries in the tropics are not comprehensive. The consistency between our results and findings from similar studies (Blakey et al., 2016; Frank et al., 2017), indicates that this trait overall does not seem to be a key predictor of bat vulnerability to habitat modification.
Froidevaux et al. (2016) studied the relation between bat guilds and forest structure through the combination of LiDAR and acoustic sampling. The authors found that guild-specific activity was strongly influenced by 3D forest structure, highlighting the importance of echolocation in habitat selection. Similarly, Jung et al. (2012) found that bat assemblages in Germany were affected by canopy structure, height, surface roughness and edge fraction.
Bats belonging to the same guild might not use calls with similar frequencies, but unquestionably tend to share similar echolocation call shapes, which are highly related to habitat type and foraging mode (Schnitzler and Kalko, 2001; Denzinger and Schnitzler, 2013). Following a widely used classification of bats into guilds (Schnitzler and Kalko, 2001), we used the term “clutter foragers” for those species in our study that emit CF calls (e.g., Pteronotus alitonus and Pteronotus cf. rubiginosus). They emit pulses of long duration that allow them to obtain accurate information about the environment, and detect small, mobile prey in dense vegetation (Barclay, 1999; Barclay et al., 1999; López-Baucells et al., 2017; Pavan and Marroig, 2017). These species might be more susceptible to the landscape-wide increase in open areas and the reduction of vegetation density associated with forest fragmentation. Our results show that CF species are more abundant in forest interiors than in clearings or fragment edges, regardless of whether the area was continuous forest or a fragment. This suggests that vegetation cover is a major determinant of their habitat use and implies a capacity for these species to persist even in relatively small forest patches. Wordley et al. (2017) obtained similar results in India, with bats using constant frequency calls being much more common in protected forests than in altered habitats. A better knowledge of the extent to which these species can adapt to habitat fragmentation and modification, or which kinds of remnant habitats offer suitable environmental conditions for their long-term persistence is essential to improve their conservation. Larger distances between remnant patches in landscapes that are heavily fragmented would likely constitute a substantial barrier for these species, reducing their survival capacity (Ethier and Fahrig, 2011).
We found a strong link between the preferred stratum in which each bat species tends to forage and the vegetation structure of the forest. Primary tropical forests are characterized by marked vertical complexity that creates a pattern of differential use of space by organisms (Bernard, 2001; Marques et al., 2015). Bats move through space three-dimensionally, and as a consequence, forest structure and vegetation clutter may affect not only horizontal patterns of bat activity but also vertical patterns (Hayes and Gruver, 2000; Jung et al., 2012; Mas, 2014). In fact, most of the foraging activity of bats occurs in the canopy, the upper forest layer (Kalko and Handley, 2001; Vetter et al., 2011; Marques et al., 2015; Marques, 2016). Deforestation may not have a large influence on bats foraging in open spaces because they mostly fly above the forest canopy (Marques et al., 2015). However, for bats that typically forage in cluttered habitats, a reduction of tree cover might force these species to abandon the area due to the lack of suitable foraging habitat.
The fact that we pooled the data from the different fragment sizes (due to the limited number of replicates per size class dictated by the BDFFP experimental design) might have slightly biased the results due to differential responses of some AIB toward fragments of different sizes and associated edge effects (López-Baucells, 2018). However, because of the complexity of the statistical approach employed here, we considered it preferable not to add another layer of complexity by taking fragment size into account, which would have made our results not only statistically less robust but also more difficult to interpret.
Patterns of habitat use in AIB are generally influenced by two main functional traits, wing morphology and echolocation call structure (Almeida et al., 2014; Marques et al., 2015). Bats with high wing loading and aspect ratio (long and pointed wings) tend to emit quasi-constant frequency calls, suitable to detect prey at long distances in open habitats. In contrast, bats with low wing loading and aspect ratio (short and rounded wings) emit either modulated or constant frequency calls, characterized by a shorter range of detection, more suitable for cluttered habitats (Aldridge and Rautenbach, 1987; Emrich et al., 2014). This intricate relation among wing morphology, echolocation call design, habitat type and feeding ecology hampers the clear separation of the influence of each functional trait on fragmentation vulnerability. Studies on tropical bats that have tried to unravel the relation between functional traits and environmental gradients so far mostly targeted phyllostomid species, therefore neglecting the acoustic component (Meyer et al., 2008; Farneda et al., 2015; Garcia-Morales et al., 2016). More research is still needed to reveal to which extent and under which circumstances a particular trait is more important than another.
Farneda et al. (2015) showed that diet type is an important predictor of vulnerability to fragmentation in phyllostomids, and this may also be true for AIB. In fact, insectivorous bats have distinct diets that include a broad diversity of insect groups or adjust their activity patterns to new environmental conditions (Kalka and Kalko, 2006; Lawer and Darkoh, 2016; Kemp et al., 2019; Rocha et al., 2019) and there is evidence that some of these are substantially impacted by forest fragmentation (Didham et al., 1996; Golden and Crist, 1999; Rösch et al., 2013; Benítez-Malvido et al., 2016). Diet type may thus influence the vulnerability of AIB to fragmentation, but we could not include this trait in our study due to the scarcity of knowledge about the diet of the species involved. Another potentially critical trait for bat species that should be included in further studies is roosting preference. Some bat species require mature forests for roosting and therefore will be highly vulnerable to habitat loss, fragmentation and degradation (Voss et al., 2016). In this context, it is important to improve our understanding of the natural history of AIB, to allow studies that include a more complete set of predictors that potentially influence the vulnerability of species to fragmentation.
We found that a combination of species functional traits and environmental characteristics were relevant predictors of the sensitivity of AIB toward fragmentation, a finding that may apply across many human-modified Neotropical landscapes. In this context, the type of echolocation calls used by the species proved to be particularly important. Species with QCF echolocation calls, adapted to open habitats or forest edges are likely to be less affected by forest fragmentation. In contrast, species with CF calls, typically understory foragers, with low wing loading and aspect ratio, are potentially more vulnerable. The latter are thus potentially most threatened by deforestation, although the fact that they persist even in fragments as small as 1 ha is encouraging for those working to prevent local extinctions of these species. Promoting natural forest regeneration and human-assisted restoration seem to be promising management strategies in the Neotropics for maintaining high taxonomic and functional bat diversity at the landscape-scale (Farneda et al., 2018a,b, 2019; Rocha et al., 2018). Our results indicate that preserving the structural complexity of tropical forests is important to facilitate the persistence of the most fragmentation-sensitive species and to avoid general functional trait loss and associated loss in ecosystem services provided by bats in human-modified tropical landscapes.
The datasets generated for this study are available on request to the corresponding author.
All authors listed have made a substantial, direct and intellectual contribution to the work, and approved it for publication.
Funding was provided by the Portuguese Foundation for Science and Technology to CM (PTDC/BIA-BIC/111184/2009), RR (SFRH/BD/80488/2011), and AL-B (PD/BD/52597/2014). FF and PB was supported by a CAPES fellowship (8888.370067/2019-00). This is publication 769 in the Technical Series of the BDFFP.
The authors declare that the research was conducted in the absence of any commercial or financial relationships that could be construed as a potential conflict of interest.
We would like to thank the volunteers and field assistants that participated in data collection as well as the BDFFP for logistical support. We are also thankful to Isabel Núñez for her contribution to figure design and to Sara Fraixedas and Álvaro Fernández-Llamazares for help with proof reading. We further acknowledge comments by two reviewers which helped improve the manuscript. Research was conducted under permit 26877-2 issued by the Instituto Chico Mendes de Conservação da Biodiversidade (ICMBio).
The Supplementary Material for this article can be found online at: https://www.frontiersin.org/articles/10.3389/fevo.2019.00373/full#supplementary-material
Aldridge, H., and Rautenbach, I. L. (1987). Morphology, echolocation and resource partitioning in insectivorous bats. J. Anim. Ecol. 56, 763–778. doi: 10.2307/4947
Almeida, M. H., Ditchfield, A. D., and Tokumaru, R. S. (2014). Habitat characteristics and insectivorous bat activity. Chiroptera Neotropical 20, 1264–1270.
Altringham, J. (2011). Bats. From Evolution to Conservation, 2nd Edn. New York, NY: Oxford University Press.
Arita, H. T., and Fenton, M. B. (1997). Flight and echolocation in the ecology and evolution of bats. Trends Ecol. Evol. 12, 53–58. doi: 10.1016/S0169-5347(96)10058-6
Bader, E., Acácio, M., and Monadjem, A. (2015a). The importance of water bodies for insectivorous bats in a Malagasy dry deciduous forest: a case example from Kirindy (Cnferef). Malagasy Nat. 9, 88–96.
Bader, E., Jung, K., Kalko, E. K. V., Page, R. A., Rodriguez, R., and Sattler, T. (2015b). Mobility explains the response of aerial insectivorous bats to anthropogenic habitat change in the Neotropics. Biol. Conserv. 186, 97–106. doi: 10.1016/j.biocon.2015.02.028
Barbaro, L., and Van Halder, I. (2009). Linking bird, carabid beetle and butterfly life-history traits to habitat fragmentation in mosaic landscapes. Ecography 32, 321–333. doi: 10.1111/j.1600-0587.2008.05546.x
Barclay, R. M. R. (1999). Bats are not birds–a cautionary note on using echolocation calls to identify bats: a comment. J. Mammal. 80, 290–296. doi: 10.2307/1383229
Barclay, R. M. R., Fullard, J. H., and Jacobs, D. S. (1999). Variation in the echolocation calls of the hoary bat (Lasiurus cinereus): influence of body size, habitat structure, and geographic location. Canad. J. Zool. 77, 530–534. doi: 10.1139/z99-008
Benítez-Malvido, J., Dáttilo, W., Martínez-Falcón, A. P., Durán-Barrón, C., Valenzuela, J., López, S., et al. (2016). The multiple impacts of tropical forest fragmentation on arthropod biodiversity and on their patterns of interactions with host plants. PLoS ONE 11:e0146461. doi: 10.1371/journal.pone.0146461
Benjamini, Y., and Hochberg, Y. (1995). Controlling the false discovery rate: a practical and powerful approach to multiple testing. J. R. Stat. Soc. B Methodol. 57, 289–300. doi: 10.1111/j.2517-6161.1995.tb02031.x
Bernard, E. (2001). Vertical stratification of bat communities in primary forests of Central Amazon, Brazil. J. Trop. Ecol. 17, 115–126. doi: 10.1017/S0266467401001079
Blakey, R. V., Law, B. S., Kingsford, R. T., and Stoklosa, J. (2017). Terrestrial laser scanning reveals below-canopy bat trait relationships with forest structure. Remote Sens. Environ. 198, 40–51. doi: 10.1016/j.rse.2017.05.038
Blakey, R. V., Law, B. S., Kingsford, R. T., Stoklosa, J., Tap, P., and Williamson, K. (2016). Bat communities respond positively to large-scale thinning of forest regrowth. J. Appl. Ecol. 53, 1694–1703. doi: 10.1111/1365-2664.12691
Bohnenstengel, T. (2012). Roost selection by the forest-dwelling bat Myotis bechsteinii (Mammalia: Chiroptera): implications for its conservation in managed woodlands. Bull. Soc. Neuchl. Sci. Nat. 132, 47–62. doi: 10.5169/seals-309720
Borkin, K. M., and Parsons, S. (2011). Sex-specific roost selection by bats in clearfell harvested plantation forest: improved knowledge advises management. Acta Chiropterol. 13, 373–383. doi: 10.3161/150811011X624848
Browning, E., Gibb, R., Glover-Kapfer, P., and Jones, K. (2017). Passive Acoustic Monitoring in Ecology and Conservation. Woking: WWF.
Cadotte, M. W., Carscadden, K., and Mirotchnick, N. (2011). Beyond species: functional diversity and the maintenance of ecological processes and services. J. Appl. Ecol. 48, 1079–1087. doi: 10.1111/j.1365-2664.2011.02048.x
Cardinale, B. J., Duffy, J. E., Gonzalez, A., Hooper, D. U., Perrings, C., Venail, P., et al. (2012). Corrigendum: biodiversity loss and its impact on humanity. Nature 489, 326–326. doi: 10.1038/nature11373
Carvalho, F., Fabián, M. E., and Menegheti, J. O. (2013). Vertical structure of an assemblage of bats (Mammalia: Chiroptera) in a fragment of Atlantic Forest in Southern Brazil. Zoologia 30, 491–498. doi: 10.1590/S1984-46702013000500004
Cisneros, L. M., Fagan, M. E., and Willig, M. R. (2015). Effects of human-modified landscapes on taxonomic, functional and phylogenetic dimensions of bat biodiversity. Div. Distrib. 21, 523–533. doi: 10.1111/ddi.12277
Coronado, A., Flaquer, C., Puig-Montserrat, X., Barthe, E., Mas, M., Arrizabalaga, A., et al. (2018). The role of secondary trees in Mediterranean mature forests for the conservation of the forest-dwelling bat Myotis alcathoe. Are current logging guidelines appropriate? Hystrix 28, 240–246. doi: 10.4404/hystrix-00004-2017
Cunto, G. C., and Bernard, E. (2012). Neotropical bats as indicators of environmental disturbance: what is the emerging message? Acta Chiropterol. 14, 143–151. doi: 10.3161/150811012X654358
Davies, K. F., Margules, C. R., and Lawrence, J. F. (2000). Which traits of species predict population declines in experimental forest fragments? Ecology 81, 1450–1461. doi: 10.1890/0012-9658(2000)081[1450:WTOSPP]2.0.CO;2
Davies, K. T., Bates, P. J., Maryanto, I., Cotton, J. A., and Rossiter, S. J. (2013). The evolution of bat vestibular systems in the face of potential antagonistic selection pressures for flight and echolocation. PLoS ONE. 8:e61998. doi: 10.1371/journal.pone.0061998
De Bello, F., Berg, M. P., Dias, A. T. C., Diniz-Filho, J. A. F., Gotzenberger, L., Hortal, J., et al. (2015). On the need for phylogenetic ‘corrections’ in functional trait-based approaches. Folia Geobot. 50, 349–357. doi: 10.1007/s12224-015-9228-6
Denzinger, A., and Schnitzler, U. (2013). Bat guilds, a concept to classify the highly diverse foraging and echolocation behaviors of microchiropteran bats. Front. Physiol. 4:164. doi: 10.3389/fphys.2013.00164
Didham, R. K., Ghazoul, J., Stork, N. E., and Davis, A. J. (1996). Insects in fragmented forests: a functional approach. Trends Ecol. Evol. 11, 255–260. doi: 10.1016/0169-5347(96)20047-3
Dirzo, R., Young, H. S., Galetti, M., Ceballos, G., Isaac, N. J. B., and Collen, B. (2014). Defaunation in the anthropocene. Science 345, 401–406. doi: 10.1126/science.1251817
Dobrovolski, R., Diniz-Filho, J. A. F., Loyola, R. D., and De Marco, P. (2011). Agricultural expansion and the fate of global conservation priorities. Biodiv. Conserv. 20, 2445–2459. doi: 10.1007/s10531-011-9997-z
Dray, S., Choler, P., Dolédec, S., Peres-Neto, P. R., Thuiller, W., Pavoine, S., et al. (2014). Combining the fourth-corner and the RLQ methods for assessing trait responses to environmental variation. Ecology 95, 14–21. doi: 10.1890/13-0196.1
Dray, S., and Dufour, A. B. (2007). The ade4 package: implementing the duality diagram for ecologists. J. Stat. Softw. 22, 1–20. doi: 10.18637/jss.v022.i04
Emrich, M. A., Clare, E. L., Symondson, W. O., Koenig, S. E., and Fenton, M. B. (2014). Resource partitioning by insectivorous bats in Jamaica. Mol. Ecol. 23, 3648–3656. doi: 10.1111/mec.12504
Estrada-Villegas, S., Meyer, C. F., and Kalko, E. K. (2010). Effects of tropical forest fragmentation on aerial insectivorous bats in a land-bridge island system. Biol. Conserv. 143, 597–608. doi: 10.1016/j.biocon.2009.11.009
Ethier, K., and Fahrig, L. (2011). Positive effects of forest fragmentation, independent of forest amount, on bat abundance in eastern Ontario, Canada. Landsc. Ecol. 26, 865–876. doi: 10.1007/s10980-011-9614-2
Ewers, R. M., and Didham, R. K. (2006). Confounding factors in the detection of species responses to habitat fragmentation. Biol. Rev. 81, 117–142. doi: 10.1017/S1464793105006949
Farneda, F. Z., Grelle, C. E. V., Rocha, R., Ferreira, D. F., López-Baucells, A., and Meyer, C. F. J. (2019). Predicting biodiversity loss in island and countryside ecosystems through the lens of taxonomic and functional biogeography. Ecography. 1–10. doi: 10.1111/ecog.04507
Farneda, F. Z., Rocha, R., López-Baucells, A., Groenenberg, M., Silva, I., Palmeirim, J. M., et al. (2015). Trait-related responses to habitat fragmentation in Amazonian bats. J. Appl. Ecol. 52, 1381–1391. doi: 10.1111/1365-2664.12490
Farneda, F. Z., Rocha, R., López-Baucells, A., Sampaio, E. M., Palmeirim, J. M., Bobrowiec, P. E. D., et al. (2018a). Functional recovery of Amazonian bat assemblages following secondary forest succession. Biol. Conserv. 218, 192–199. doi: 10.1016/j.biocon.2017.12.036
Farneda, F. Z., Rocha, R., López-Baucells, A., Sampaio, E. M., Palmeirim, J. M., Bobrowiec, P. E. D., et al. (2018b). The road to functional recovery: temporal effects of matrix regeneration on Amazonian bats. Trop. Conserv. Sci. 11, 1–4. doi: 10.1177/1940082918777185
Fenton, M. B. (1997). Science and the conservation of bats. J. Mammal. 78, 1–14. doi: 10.2307/1382633
Ferreira, D. F., Rocha, R., López-Baucells, A., Farneda, F. Z., Carreiras, J. M. B., Palmeirim, J. M., et al. (2017). Season-modulated responses of Neotropical bats to forest fragmentation. Ecol. Evol. 7, 4059–4071. doi: 10.1002/ece3.3005
Frank, H. K., Frishkoff, L. O., Mendenhall, C. D., Daily, G. C., and Hadly, E. A. (2017). Phylogeny, traits, and biodiversity of a Neotropical bat assemblage: close relatives show similar responses to local deforestation. Am. Nat. 190, 200–212. doi: 10.1086/692534
Freeman, P. W. (1981). A Multivariate Study of the Family Molossidae (Mammalia, Chiroptera): Morphology, Ecology, Evolution. Field Museum of Natural History Lincoln. doi: 10.5962/bhl.title.3128
Froidevaux, J. S. P., Zellweger, F., Bollmann, K., Jones, G., and Obrist, M. K. (2016). From field surveys to LiDAR: shining a light on how bats respond to forest structure. Rem. Sens. Environ. 175, 242–250. doi: 10.1016/j.rse.2015.12.038
Garcia-Morales, R., Moreno, C. E., Badano, E. I., Zuria, I., Galindo-Gonzalez, J., Rojas-Martinez, A. E., et al. (2016). Deforestation impacts on bat functional diversity in tropical landscapes. PLoS ONE 11:e0166765. doi: 10.1371/journal.pone.0166765
Gardner, A. L. (2008). Mammals of South America, Volume 1: Marsupials, Xenarthrans, Shrews, and Bats. Chicago, IL: University of Chicago Press. doi: 10.7208/chicago/9780226282428.001.0001
Golden, D. M., and Crist, T. O. (1999). Experimental effects of habitat fragmentation on old-field canopy insects: community, guild and species responses. Oecologia 118, 371–380. doi: 10.1007/s004420050738
Haddad, N. M., Brudvig, L. A., Clobert, J., Davies, K. F., Gonzalez, A., Holt, R. D., et al. (2015). Habitat fragmentation and its lasting impact on Earth's ecosystems. Sci. Adv. 1:e1500052. doi: 10.1126/sciadv.1500052
Hanspach, J., Fischer, J., Ikin, K., Stott, J., and Law, B. S. (2012). Using trait-based filtering as a predictive framework for conservation: a case study of bats on farms in southeastern Australia. J. Appl. Ecol. 49, 842–850. doi: 10.1111/j.1365-2664.2012.02159.x
Hayes, J. P., and Gruver, J. C. (2000). Vertical stratification of bat activity in an old-growth forest in western Washington. Northw. Sci. 74, 102–108. doi: 10.1046/j.1365-294x.2000.00874-7.x
Henle, K., Davies, K. F., Kleyer, M., Margules, C., and Settele, J. (2004). Predictors of species sensitivity to fragmentation. Biodivers. Conserv. 13, 207–251. doi: 10.1023/B:BIOC.0000004319.91643.9e
Hill, M. O., and Smith, A. J. E. (1976). Principal component analysis of taxonomic data with multi-state discrete characters. Taxon 25, 249–255. doi: 10.2307/1219449
Jung, K., Kaiser, S., Böhm, S., Nieschulze, J., and Kalko, E. K. V. (2012). Moving in three dimensions: effects of structural complexity on occurrence and activity of insectivorous bats in managed forest stands. J. Appl. Ecol. 49, 523–531. doi: 10.1111/j.1365-2664.2012.02116.x
Kalka, M., and Kalko, E. K. V. (2006). Gleaning bats as underestimated predators of herbivorous insects: diet of Micronycteris microtis (Phyllostomidae) in Panama. J. Trop. Ecol. 22, 1–10. doi: 10.1017/S0266467405002920
Kalko, E. (1998). Organisation and diversity of tropical bat communities through space and time. Zoology 101, 281–297.
Kalko, E. K., and Schnitzler, V. (1993). Plasticity in echolocation signals of European pipistrelle bats in search flight: implications for habitat use and prey detection. Behav. Ecol. Sociobiol. 33, 415–428. doi: 10.1007/BF00170257
Kalko, E. K. V., and Handley, C. O. (2001). Neotropical bats in the canopy: diversity, community structure, and implications for conservation. Plant Ecol. 153, 319–333. doi: 10.1023/A:1017590007861
Kemp, J., López-Baucells, A., Rocha, R., Wangensteen, O. S., Andriatafika, Z., Nair, A., et al. (2019). Bats as potential suppressors of multiple agricultural pests: a case study from madagascar. Agric. Ecosyst. Environ. 269, 88–96 doi: 10.1016/j.agee.2018.09.027
Kennedy, C. M., Grant, E. H. C., Neel, M. C., Fagan, W. F., and Marra, P. P. (2011). Landscape matrix mediates occupancy dynamics of Neotropical avian insectivores. Ecol. Appl. 21, 1837–1850. doi: 10.1890/10-1044.1
Kleyer, M., Dray, S., Bello, F., Lepš, J., Pakeman, R. J., Strauss, B., et al. (2012). Assessing species and community functional responses to environmental gradients: which multivariate methods? J. Veget. Sci. 23, 805–821. doi: 10.1111/j.1654-1103.2012.01402.x
Kunz, T. H., de Torrez, E. B., Bauer, D., Lobova, T., and Fleming, T. H. (2011). Ecosystem services provided by bats. Ann. N. Y. Acad. Sci. 1223, 1–38. doi: 10.1111/j.1749-6632.2011.06004.x
Kupfer, J. A., Malanson, G. P., and Franklin, S. B. (2006). Not seeing the ocean for the islands: the mediating influence of matrix-based processes on forest fragmentation effects. Glob. Ecol. Biogeogr. 15, 8–20. doi: 10.1111/j.1466-822X.2006.00204.x
Laurance, W. F., Camargo, J. L. C., Fearnside, P. M., Lovejoy, T. E., Williamson, G. B., Mesquita, R. C. G., et al. (2018). An Amazonian rainforest and its fragments as a laboratory of global change. Biol. Rev. Cam. Philos. Soc. 93, 223–247. doi: 10.1111/brv.12343
Laurance, W. F., Camargo, J. L. C., Luizao, R. C. C., Laurance, S. G., Pimm, S. L., Bruna, E. M., et al. (2011). The fate of Amazonian forest fragments: a 32-year investigation. Biol. Conserv. 144, 56–67. doi: 10.1016/j.biocon.2010.09.021
Laurance, W. F., Sayer, J., and Cassman, K. G. (2014). Agricultural expansion and its impacts on tropical nature. Trends Ecol. Evol. 29, 107–116. doi: 10.1016/j.tree.2013.12.001
Lawer, E. A., and Darkoh, E. L. (2016). Effects of agroecosystems on insect and insectivorous bat activity: a preliminary finding based on light trap and mist net captures. Turkish J. Zool. 40, 423–432. doi: 10.3906/zoo-1507-17
López-Baucells, A. (2018). Assessment of the Effects of Forest Fragmentation on Aerial Insectivorous Bats in the Amazonian Rainforest. University of Lisbon (Manaus: Portugal).
López-Baucells, A., Rocha, R., Bobrowiec, P. E. D., Bernard, E., Palmeirim, J., and Meyer, C. (2016). Field Guide to Amazonian Bats. Manaus: INPA
López-Baucells, A., Torrent, L., Rocha, R., Bobrowiec, P. E., Palmeirim, J. M., and Meyer, C. F. (2019). Stronger together: combining automated classifiers with manual post-validation optimizes the workload vs reliability trade-off of species identification in bat acoustic surveys. Ecol. Inform. 49, 45–53. doi: 10.1016/j.ecoinf.2018.11.004
López-Baucells, A., Torrent, L., Rocha, R., Pavan, A. C., Bobrowiec, P. E. D., Meyer, C. F., et al. (2017). Geographical variation in the high-duty cycle echolocation of the cryptic common mustached bat Pteronotus cf. rubiginosus (Mormoopidae). Bioacoustics J. 27, 341–357. doi: 10.1080/09524622.2017.1357145
Loureiro, L. O., and Gregorin, R. (2015). Structure of a bat assemblage from a fragmented landscape in the state of Minas Gerais, southeastern Brazil. Mastozool. Neotropical. 22, 35–42.
MacSwiney, G. C., Clarke, F. M., and Racey, P. A. (2008). What you see is not what you get: the role of ultrasonic detectors in increasing inventory completeness in Neotropical bat assemblages. J. Appl. Ecol. 45, 1364–1371. doi: 10.1111/j.1365-2664.2008.01531.x
Malhi, Y., Gardner, T. A., Goldsmith, G. R., Silman, M. R., and Zelazowski, P. (2014). Tropical 0. Ann. Rev. Environ. Resour. 39, 125–159. doi: 10.1146/annurev-environ-030713-155141
Marinello, M. M., and Bernard, E. (2014). Wing morphology of Neotropical bats: a quantitative and qualitative analysis with implications for habitat use. Can. J. Zool. 92, 141–147. doi: 10.1139/cjz-2013-0127
Marques, J. T. (2016). Structuring Amazonian Bat Assemblages: Importance of Horizontal and Vertical Dimensions of Habitat. Lisbon: University of Lisbon.
Marques, J. T., Ramos Pereira, M. J., Marques, T. A., Santos, C. D., Santana, J., Beja, P., et al. (2013). Optimizing sampling design to deal with mist-net avoidance in Amazonian birds and bats. PLoS ONE 8:e74505. doi: 10.1371/journal.pone.0074505
Marques, J. T., Ramos Pereira, M. J., and Palmeirim, J. M. (2015). Patterns in the use of rainforest vertical space by Neotropical aerial insectivorous bats: all the action is up in the canopy. Ecography 39, 476–486. doi: 10.1111/ecog.01453
Mas, M. (2014). Vertical stratification on insectivorous bats ensembles in central Amazon (MSc thesis). Universitat de Barcelona, Barcelona, Spain.
Mendenhall, C. D., Karp, D. S., Meyer, C. F. J., Hadly, E. A., and Daily, G. C. (2014). Predicting biodiversity change and averting collapse in agricultural landscapes. Nature 509, 213–217. doi: 10.1038/nature13139
Meyer, C. F. J., Fruend, J., Lizano, W. P., and Kalko, E. K. V. (2008). Ecological correlates of vulnerability to fragmentation in Neotropical bats. J. Appl. Ecol. 45, 381–391. doi: 10.1111/j.1365-2664.2007.01389.x
Meyer, C. F. J., Struebig, M. J., and Willig, M. R. (2016). “Responses of tropical bats to habitat fragmentation, logging, and deforestation,” in Bats in the Anthropocene: Conservation of Bats in a Changing World, eds C. C. Voigt and T. Kingston (New York, NY: Springer, 63–103.
Mickleburgh, S. P., Hutson, A. M., and Racey, P. A. (2002). A review of the global conservation status of bats. Oryx 36, 18–34. doi: 10.1017/S0030605302000054
Millon, L. J.-,Julien, F., Julliard, R., and Kerbiriou, C. (2015). Bat activity in intensively farmed landscapes with wind turbines and offset measures. Ecol. Eng. 75, 250–257. doi: 10.1016/j.ecoleng.2014.11.050
Norberg, U. M. (1987). “Wing form and flight mode in bats,” in Recent Advances in the Study of Bats, eds M. B. Fenton, P. A. Racey, and J. M. V. Rayner (Cambridge University Press), 43–56.
Norberg, U. M., and Fenton, M. B. (1988). Carnivorous bats? Biol. J. Linnean Soc. 33, 383–394. doi: 10.1111/j.1095-8312.1988.tb00451.x
Norberg, U. M., and Rayner, J. M. V. (1987). Ecological morphology and flight in bats (Mammalia, Chiroptera) - wing adaptations, flight performance, foraging strategy and echolocation. Philos. Trans. R. Soc. Lond. B Biol. Sci. 316, 337–419. doi: 10.1098/rstb.1987.0030
Pavan, A. C., and Marroig, G. (2017). Timing and patterns of diversification in the Neotropical bat genus Pteronotus (Mormoopidae). Mol. Phylogenet. Evol. 108, 61–69. doi: 10.1016/j.ympev.2017.01.017
Penone, C., Kerbiriou, C., Julien, J. F., Marmet, J., and Le Viol, I. (2018). Body size information in large-scale acoustic bat databases. PeerJ. 6:e5370. doi: 10.7717/peerj.5370
Peres-Neto, P. R., Dray, S., and ter Braak, C. J.. (2017). Linking trait variation to the environment: critical issues with community-weighted mean correlation resolved by the fourth-corner approach. Ecography 40, 806–816. doi: 10.1111/ecog.02302
Petchey, O. L., and Gaston, K. J. (2006). Functional diversity: back to basics and looking forward. Ecol. Lett. 9, 741–758. doi: 10.1111/j.1461-0248.2006.00924.x
Peters, R. H. (1986). The Ecological Implications of Body Size, Vol. 26. Cambridge: Cambridge University Press.
Purvis, A., Gittleman, J. L., Cowlishaw, G., and Mace, G. M. (2000). Predicting extinction risk in declining species. Proc. R. Soc. Lond. B Biol. Sci. 267, 1947–1952. doi: 10.1098/rspb,.2000.1234
R Core Team (2017). R: A Language and Environment for Statistical Computing. R Foundation for Statistical Computing, Vienna.
Ray, N., Lehmann, A., and Joly, P. (2002). Modeling spatial distribution of amphibian populations: a GIS approach based on habitat matrix permeability. Biodivers. Conserv. 11, 2143–2165. doi: 10.1023/A:1021390527698
Ricketts, T. H. (2001). The matrix matters: effective isolation in fragmented landscapes. Am. Nat. 158, 87–99. doi: 10.1086/320863
Rocha, R., Ferreira, D. F., López-Baucells, A., Farneda, F. Z., Carreiras, J., Palmeirim, J. M., et al. (2017a). Does sex matter? Gender-specific responses to forest fragmentation in Neotropical bats. Biotropica 49, 881–890. doi: 10.1111/btp.12474
Rocha, R., López-Baucells, A., Farneda, F., Ferreira, D., Silva, I., Acácio, M., et al. (2019). Second-growth and small forest clearings have little effect on the temporal activity patterns of Amazonian phyllostomid bats. Curr. Zool. 1, 1–9. doi: 10.1093/cz/zoz042/5561099
Rocha, R., López-Baucells, A., Farneda, F. Z., Groenenberg, M., Bobrowiec, P. E. D., Cabeza, M., et al. (2017b). Consequences of a large-scale fragmentation experiment for Neotropical bats: disentangling the relative importance of local and landscape-scale effects. Landsc. Ecol. 32, 31–45. doi: 10.1007/s10980-016-0425-3
Rocha, R., Ovaskainen, O., López-Baucells, A., Farneda, F. Z., Ferreira, D. F., Bobrowiec, P. E. D., et al. (2017c). Design matters: an evaluation of the impact of small man-made forest clearings on tropical bats using a before-after-control-impact design. Forest Ecol. Manage. 401, 8–16. doi: 10.1016/j.foreco.2017.06.053
Rocha, R., Ovaskainen, O., López-Baucells, A., Farneda, F. Z., Sampaio, E. M., Bobrowiec, P. E. D., et al. (2018). Secondary forest regeneration benefits old-growth specialist bats in a fragmented tropical landscape. Sci. Rep. 8:3819. doi: 10.1038/s41598-018-21999-2
Rösch, V., Tscharntke, T., Scherber, C., and Batary, P. (2013). Landscape composition, connectivity and fragment size drive effects of grassland fragmentation on insect communities. J. Appl. Ecol. 50, 387–394. doi: 10.1111/1365-2664.12056
Safi, K., Meiri, S., and Jones, K. E. (2013). “Evolution of body size in bats,” in Animal body size: Linking pattern and process across space, time, and taxonomic group, eds F. A. Smith and S. K. Lyons (Chicago, IL: University of Chicago Press, 95–151.
Schnitzler, H. U., and Kalko, E. K. V. (2001). Echolocation by insect-eating bats: we define four distinct functional groups of bats and find differences in signal structure that correlate with the typical echolocation tasks faced by each group. Bioscience 51, 557–569. doi: 10.1641/0006-3568(2001)051[0557:EBIEB]2.0.CO;2
Schnitzler, H. U., Moss, C. F., and Denzinger, A. (2003). From spatial orientation to food acquisition in echolocating bats. Trends Ecol. Evol. 18, 386–394. doi: 10.1016/S0169-5347(03)00185-X
Silva, C. R., and Bernard, E. (2017). Bioacoustics as an important complementary tool in bat inventories in the Caatinga drylands of Brazil. Acta Chiropterol. 19, 409–418. doi: 10.3161/15081109ACC2017.19.2.017
Taubert, F., Fischer, R., Groeneveld, J., Lehmann, S., Muller, M. S., Rodig, E., et al. (2018). Global patterns of tropical forest fragmentation. Nature 554, 519–522. doi: 10.1038/nature25508
Thiagavel, J., Santana, S. E., and Ratcliffe, J. M. (2017). Body size predicts echolocation call peak frequency better than gape height in vespertilionid bats. Sci. Rep. 7:828. doi: 10.1038/s41598-017-00959-2
Torrent, L., López-Baucells, A., Rocha, R., Bobrowiec, P. E. D., and Meyer, C. F. J. (2018). The importance of lakes for bat conservation in Amazonian rainforests: an assessment using autonomous recorders. Remote Sens. Ecol. Conserv. 4, 339–351. doi: 10.1002/rse2.83
Vetter, D., Hansbauer, M. M., Végvári, Z., and Storch, I. (2011). Predictors of forest fragmentation sensitivity in Neotropical vertebrates: a quantitative review. Ecography 34, 1–8. doi: 10.1111/j.1600-0587.2010.06453.x
Violle, C., Navas, M. L., Vile, D., Kazakou, E., Fortunel, C., Hummel, I., et al. (2007). Let the concept of trait be functional! Oikos 116, 882–892. doi: 10.1111/j.2007.0030-1299.15559.x
Voigt, C. C. (2013). Bat flight with bad wings: is flight metabolism affected by damaged wings? J. Exp. Biol. 216, 1516–1521. doi: 10.1242/jeb.079509
Voss, S. R., Fleck, D. W., Strauss, R. E., Velazco, P. M., and Simmons, N. B. (2016). Roosting Ecology of Amazonian Bats: Evidence for Guild Structure in Hyperdiverse Mammalian Communities. American Museum Novitates.
Wickramasinghe, L. P., Harris, S., Jones, G., and Vaughan, N. (2003). Bat activity and species richness on organic and conventional farms: impact of agricultural intensification. J. Appl. Ecol. 40, 984–993. doi: 10.1111/j.1365-2664.2003.00856.x
Keywords: passive recorders, bioacoustics, Chiroptera, deforestation, species traits, wing
Citation: Núñez SF, López-Baucells A, Rocha R, Farneda FZ, Bobrowiec PED, Palmeirim JM and Meyer CFJ (2019) Echolocation and Stratum Preference: Key Trait Correlates of Vulnerability of Insectivorous Bats to Tropical Forest Fragmentation. Front. Ecol. Evol. 7:373. doi: 10.3389/fevo.2019.00373
Received: 20 February 2019; Accepted: 17 September 2019;
Published: 11 October 2019.
Edited by:
Mark A. Elgar, The University of Melbourne, AustraliaReviewed by:
Leonardo Ancillotto, University of Naples Federico II, ItalyCopyright © 2019 Núñez, López-Baucells, Rocha, Farneda, Bobrowiec, Palmeirim and Meyer. This is an open-access article distributed under the terms of the Creative Commons Attribution License (CC BY). The use, distribution or reproduction in other forums is permitted, provided the original author(s) and the copyright owner(s) are credited and that the original publication in this journal is cited, in accordance with accepted academic practice. No use, distribution or reproduction is permitted which does not comply with these terms.
*Correspondence: Silvia Fraixedas Núñez, c2lsdmlhLmZyYWl4ZWRhczk5QGdtYWlsLmNvbQ==; Adrià López-Baucells, YWRyaWEuYmF1Y2VsbHNAZ21haWwuY29t
†These authors have contributed equally to this work
Disclaimer: All claims expressed in this article are solely those of the authors and do not necessarily represent those of their affiliated organizations, or those of the publisher, the editors and the reviewers. Any product that may be evaluated in this article or claim that may be made by its manufacturer is not guaranteed or endorsed by the publisher.
Research integrity at Frontiers
Learn more about the work of our research integrity team to safeguard the quality of each article we publish.