- Department of Biology, Behavioural Ecology and Ecophysiology Research Group, University of Antwerp, Wilrijk, Belgium
Parents in biparental bird species have a conflict about how much each of them should invest in the current brood to optimize their reproductive success while not being exploited. Recently, it has been hypothesized that parents might attempt to resolve this conflict via taking turns in their provisioning visits. This implies that an individual will increase its working rate when their partner does, and that they will react with a delay in feeding if the partner starts delaying its visit. Experimental studies testing whether turn taking represents a behavioral strategy are surprisingly scarce and focus on the outcome of turn taking which are alternated visits. However, the adaptive significance of turn taking strongly relies on the response to a partner that increases or reduces care. Therefore, we investigate whether parents use the turn taking rules by performing an experimental manipulation on only one of the parents. To this end, we handicapped male blue tits (Cyanistes caeruleus) by feather clipping and recorded parental feeding behavior. Surprisingly, handicapped males did not have lower visit rates or altered turn taking levels, whilst their female partners had higher visit rates and lower turn taking levels when compared to the control. Females responded to the handicap of their partners, which likely reduced the males' parental capacity, but the females' response was independent of the males' rate of provisioning. Our study highlights that behavioral strategies are flexible within pairs and that these can change at the individual level in response to sudden changes in individual state.
Introduction
During biparental care, two unrelated individuals join to raise their offspring, which secures a joint fitness benefit via enhanced offspring growth and survival. However, each parent individually pays the costs of providing care (Trivers, 1972). Thus, it is in both parents' interest that their partner invests more, so that they themselves can retain energy for future reproduction (Stearns, 1989). How parents resolve this sexual conflict over parental care has been studied in several bird species (see e.g., Royle et al., 2012). Various theoretical models have been developed to provide a theoretical framework for this sexual conflict (i.e., Houston and Davies, 1985; McNamara et al., 1999). Most models predict that parents should invest below the most optimal level of care in order to avoid exploitation by their partner, which was interpreted as costs of negotiation (McNamara et al., 1999, 2003; Lessells and McNamara, 2012). However, more recently it was argued that exploitation could be avoided when parents match each other's investment (Hinde, 2006; Johnstone and Hinde, 2006) which they may achieve by coordinating the sequence of their visits (Johnstone et al., 2014).
Such coordination of feeding visits on a temporal scale may originate from conditional cooperation, a behavioral strategy which implies that when one individual invests in a common good, the other individual is also more willing to do so (Gächter, 2007; Johnstone et al., 2014; Johnstone and Savage, 2019). In terms of offspring provisioning, this means that each parent is triggered to feed when their partner fed last. On the contrary, when one parent delays its next nest visit, the partner is supposed to respond by also delaying its next contribution to care (Johnstone et al., 2014; Bebbington and Hatchwell, 2016; Johnstone and Savage, 2019). Thus, parents take turns by adjusting their visit rates in response to each other, which may ultimately be reflected in a high proportion of alternated feeding visits within pairs. Such coordinated feeding visits have been found in a number of observational studies (Johnstone et al., 2014; Bebbington and Hatchwell, 2016; Koenig and Walters, 2016; Iserbyt et al., 2017, 2018; Savage et al., 2017; Leniowski and Wegrzyn, 2018; Wojczulanis-Jakubas et al., 2018), but the number of studies testing the significance of conditional cooperation for conflict resolution remains limited (but see Griffioen et al., 2019; Iserbyt et al., 2019). Experiments are vital for our understanding of conditional cooperation given the analytical difficulties faced in observational studies that may prevent to prove whether parents actively take turns (Schlicht et al., 2016; Ihle et al., 2019; Santema et al., 2019). That is, turn taking could also arise from variation in the refractory period (Johnstone et al., 2014; Savage et al., 2017) or from correlated male and female inter-visit intervals (Schlicht et al., 2016; but see Johnstone et al., 2016; Savage et al., 2017). The only two manipulation studies so far have targeted both parents via brood size manipulations (Griffioen et al., 2019) or via temporal removal of one parent (Iserbyt et al., 2019) and investigated the effect on pair alternation. However, the proportion of alternated feeding visits is supposed to be driven by an active process of turn taking, which is individually adjusting the order and frequency of nest visits to that of the partner. This requires an experimental manipulation of only one of the two pair members.
To investigate this turn taking strategy, individual visit rates could be manipulated experimentally. This could be achieved either by stimulating one parent via begging playbacks to increase its visit rate or by handicapping one parent to lower its visit rate. A playback study by Hinde (2006) was performed such that the begging manipulation was only heard by one of the parents, and showed that individuals stimulated their partner to feed more when they themselves fed the offspring more (Hinde, 2006; see also Lendvai et al., 2018, but see Santema et al., 2007). Handicapping one of the parents, which could be achieved by for example feather clipping or weighting, could also test whether a parent delays its visit rate in response to a reduced visit rate of its partner. Handicapping via feather clipping increases the costs of provisioning for this parent due to higher energetic costs of flying (Pennycuick, 1982), and therefore affects the ability of a parent to maintain a rate of provisioning that corresponds to their partner's rate. Previous studies that handicapped one of the parents (including feather clipping) mainly found that the partners show partial compensation to the reduced care of their manipulated partner (Harrison et al., 2009), which is in line with predictions of the negotiation models but not with the concept of turn taking (Johnstone et al., 2014). Yet, these studies mainly focused on the response of the partners in terms of changes in their feeding rates without considering the chronological order or temporal spacing of feeding visits, and therewith the behavioral mechanism underlying the observed feeding pattern. Furthermore, there are several studies in which parents showed different reactions, such as no response (Slagsvold and Lifjeld, 1990; Whittingham et al., 1994; Schwagmeyer et al., 2002), full compensation (Sanz et al., 2000) and even slight decreases in provisioning as a response to the handicapped partner (Lozano and Lemon, 1996; Sanz et al., 2000). In these cases, parents might well adopt another behavioral strategy such as conditional cooperation. This variation in parental responses may hence relate to among species differences in the role of turn taking for parental care, which requires further study.
In this study, we investigate parental response rules via handicapping male blue tits (Cyanistes caeruleus) by clipping their wing and tail feathers to increase their physical effort of providing care via a reduced flying capacity. Handicapped males are expected to have lower visit rates compared to unmanipulated males. While it remains to be shown how a reduction in flying capacity will impinge on turn-taking, handicapped males are expected to space their nest visits as such that they maximize the number of alternated nest visits when it acts as a strategy to increase their partners' investment. As turn taking implies that neither party can change its strategy unilaterally, the female partners are expected to match their partner's feeding strategy. Finally, if parents evaluate each other's contribution to care based only on the matched feeding visits, parents can still change the value of the feeding itself, i.e., the prey profitability (Whittingham et al., 1994; Sanz et al., 2000; Johnstone et al., 2014). Therefore, our alternative prediction is that in order to keep up with the partner's visit rate, the handicapped male could cheat in its investment by providing the nestlings with smaller prey volumes. This will also allow males to maintain their turn taking level.
Materials and Methods
Study Species and Measurements
The experiment was conducted in a nest-box population of wild blue tits near Antwerp, Belgium (Peerdsbos 51° 16′N, 4° 29′E; Lucass et al., 2016) during the breeding season of 2017 (April-May). Nest boxes were checked weekly to monitor nest building, egg laying and start of incubation. From the expected hatch date, nests were monitored daily to determine the day of hatching (i.e., day 0). On nestling day 14 the individual nestlings were weighted and counted.
Handicapping Experiment
When nestlings were 6 days old, both parents were caught to acquire standard individual measurements (mass, tarsus), a blood sample was taken, and they were banded with a unique metal ring. Nests were randomly assigned to either the control or experimental (handicap) treatment. Males in the experimental treatment were handicapped by clipping their primary wing feathers (5, 7, and 9 counted from the outside) (Slagsvold and Lifjeld, 1990; Sanz et al., 2000). Additionally, their central tail feathers were removed until only the two outermost were left. Removing the feathers only took a few seconds so that handling times were very similar between control and experimental birds. Nests were left undisturbed for two full days and on the morning of day 9, parental behavior was recorded by placing an infrared nest-box camera (420TVL; Pakatak PAK-MIR5, Essex, UK) under the lid (start of experiment (mean [range]) for control nests: 08:10 a.m. [07:47–08:51 a.m.]; handicap nests: 08:01 a.m. [07:38–08:31 a.m.]). Additionally, brood size was noted whilst placing the camera. This study was carried out with approval of the Ethical Committee for animals (ECD) of the University of Antwerp (license number: 2015-85).
Behavioral Measurements
The first half hour of the video recordings was discarded to exclude potential effects of human disturbance. From the video recordings, the visits of the parents were scored until both individuals had a minimum of 10 visits or the scoring was stopped after 2 h [this was found to give reliable estimates on parental care traits—for further details see (Griffioen et al., 2019); observations were 37:44 min (sd ± 26.02) in control pairs (range 00:11:19–01:54:18) and 21:02 min (sd 05:47) in handicapped pairs (range 00:13:32–00:40:54)]. For each of these visits the volume of the prey (1 = small load, 2 = medium load, 3 = large load sensu Kölliker et al., 1998) was estimated (using ObserverXT program version 10.5.572, 2011, Noldus Information Technology, the Netherlands). The proportions of prey volumes (small, medium, and large) were calculated separately per treatment for each parent. Visit rates were calculated as visits per hour for both parents separately. Male and female turn taking rates were calculated from the visit sequences with an approach that is comparable to a Markov analysis. This calculation implements both the nature of the visit (alternated or not) and the duration of the inter-visit intervals, which are divided to acquire a λ (rate of following the partner) and μ (rate of following itself) (as in Johnstone et al., 2014). Dividing λ by μ provides an estimate for a turn taking level (λ / μ; turn taking > 1 indicates that the individual follows its partner more than itself).
Statistical Analyses
A linear mixed effect model (Gaussian distribution) was used to investigate whether variation in visit rates could be explained by treatment (control/handicapped), sex (male/female), or the interaction between treatment and sex. Brood size and Julian date (= date of the experiment) were included as covariates to take the potential influence of the brood value and brood demand, as well as seasonal changes in food availability into account. Nest ID was included as a random factor. The next linear mixed model (Gaussian distribution) investigated whether the turn taking rates varied with treatment, sex and the interaction between treatment and sex. Again, Julian date and brood size were included as covariates and nest ID as the random factor. The next two generalized linear mixed models (binomial distribution) were to investigate if the proportion of small and medium prey volumes varied with treatment, sex and their interaction. These analyses are adjusted for the variation in visit rate via the weights function, while brood size, and Julian date were included as covariates and nest ID as random factor. The effect of handicapping on the average brood mass of the nestlings on day 14 was analyzed using a linear model with treatment as fixed effect and Julian date and brood size as covariates. There were five nests that deceased before day 14 measures could be taken (4 control nests, 1 handicapped nest).
The dataset used for this manuscript can be found in the Supplementary Table 1. The analyses were performed in the statistical program R studio (version 1.1.423 and R version 3.4.3, R core Team, 2018), using the package ‘lme4 (Bates et al., 2015). The significance of the fixed factors was investigated using backward elimination with the step function (and drop1 function for the linear model on average brood mass) and with a critical α level of 0.05. The residuals of all models were normally distributed: Shapiro normality test all W > 0.90.
Results
Visit Rates
The effect of treatment on visit rates was different for both sexes [F(1, 41) = 7.76, P = 0.008; see Figure 1]. No difference was observed in visit rates of males between treatments (differences of LSmeans: df = 61.3, t = 0.179, P = 0.859; mean ± sd: handicap 32.9 ± 7.12, control 32.9 ± 15.5). However, females of handicapped partners had higher visit rates compared to females paired with non-manipulated males (differences of LSmeans: df = 61.3, t = −2.53, P = 0.014; mean ± sd: handicap 39.1 ± 12.6, control 27.9 ± 17.3). Within the treatments, there were no significant sex-differences in visit rates in the control group (differences of LSmeans: df = 41.0, t = −1.74, P = 0.089), whilst females in the handicap group had higher visit rates compared with males (differences of LSmeans: df = 41.0, t = 2.20, P = 0.034). Parental visit rates were positively related with brood size [F(1, 40) = 4.92, P = 0.032], but did not vary with Julian date [F(1, 39) = 0.49, P = 0.490].
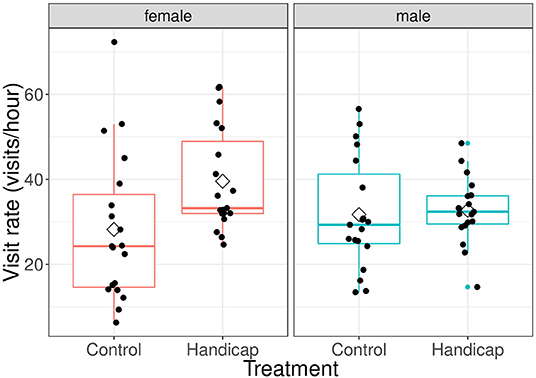
Figure 1. The effect of treatment on visit rates (visits/hour) for both male and female parents when nestlings are 9 days old. Boxplot distribution with mean (diamond) and data points are represented. Sample sizes: females handicap n = 22, control n = 21; males handicap n = 22, control n = 21.
Turn Taking of Provisioning Visits
There was a significant overall effect of treatment [treatment: F(1, 36) = 5.59, P = 0.024; interaction treatment x sex: F(1, 36) = 2.14, P = 0.152; see Figure 2]. However, post-hoc tests revealed that the turn taking level of control females was higher than that of females with a handicapped partner (differences of LSmeans df = 69, t = 2.45, P = 0.016; mean ± sd: handicap 2.16 ± 1.34, control 4.0 ± 3.42). The males of the control and handicap treatment did not differ in their turn taking (difference of LSmeans df = 69, t = 0.48, P = 0.63; mean ± sd: handicap 2.19 ± 1.62, control 2.69 ± 1.42). Furthermore, the turn taking of both parents in the control treatment did not differ (differences of LSmeans df = 36, t = 2.02, P = 0.051). Males and females did not differ in their turn taking level in pairs in which the males were handicapped (differences of LSmeans df = 36, t = −0.05, P = 0.961). Brood size and Julian date had no significant effects [brood size: F(1, 35) = 2.92, P = 0.097; Julian date: F(1, 34) = 1.03, P = 0.316].
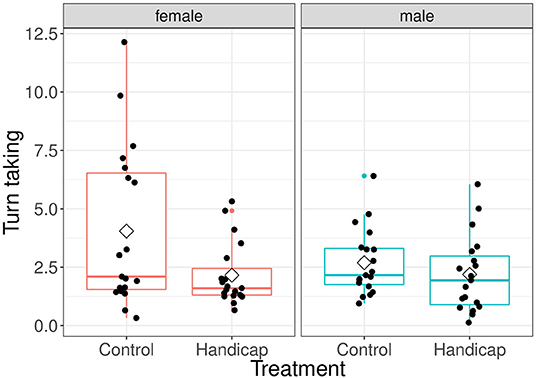
Figure 2. Turn taking levels separated for treatment and for both male and female parents. Boxplot distribution with mean (diamond) and data points are represented. Sample sizes: females handicap n = 19, control n = 19; males handicap n = 19, control n = 19.
Proportions of the Prey Volumes
The proportion of small and medium prey volumes did not differ between treatments (small: χ2 = 0.22, df = 1, P = 0.640; medium: χ2 = 0.009, df = 1, P = 0.924; see Figure 3), a pattern that was consistent for both sexes (interaction treatment * sex small prey volumes: χ2 = 0.34, df = 1, P = 0.559; medium: χ2 = 0.26, df = 1, P = 0.600; sex effect small: χ2 = 2.05, df = 1, P = 0.152; medium: χ2 = 0.19, df = 1, P = 0.659). The proportion of small and medium prey volumes did not vary with brood size (small: χ2 = 2.14, df = 1, P = 0.144; medium: χ2 = 5.27, df = 1, P = 0.217). However, Julian date had a significant positive effect on the small prey volumes (χ2 = 5.78, df = 1, P = 0.016) but not on the proportion of medium prey volumes (χ2 = 0.74, df = 1, P = 0.390).
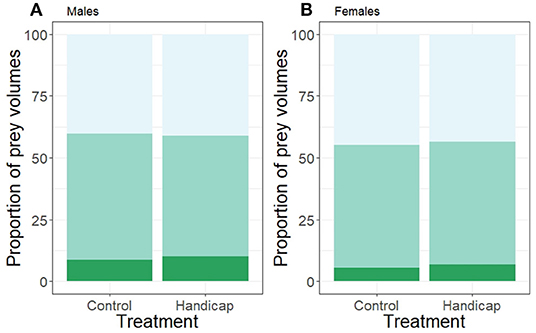
Figure 3. The proportion of prey volumes (calculated for minimal 10 visits/individual) of (A) males and (B) females separated according to treatment (handicap n = 22, control n = 21). Light green bars (top bars) indicate the proportions of small prey items, medium green (middle bars) the medium prey proportions and the dark green (lowest bars) the proportions of the large prey items. Boxplot distribution with mean (diamond) and data points are represented.
Nestling Brood Mass
Average brood mass of day 14 nestlings did not differ between treatments (F = 0.014, df = 1, P = 0.91; see Figure 4). However, both Julian date and brood size had a significant positive effect on the average brood mass of the nestlings (Julian date: F = 17.7, df = 1, P = 0.0002; brood size: F = 13.6, df = 1, P = 0.0008).
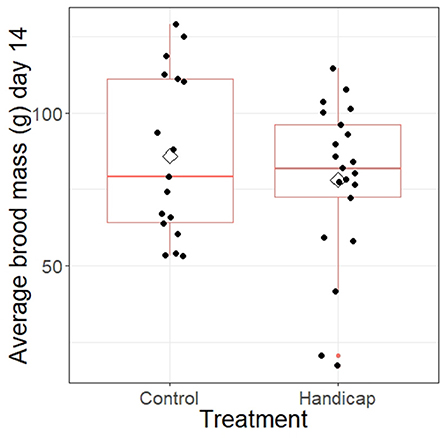
Figure 4. The average brood mass of the nests separated for handicap and control treatment. Boxplot distribution with mean (diamond) and data points are represented. Samples sizes: control n = 17 and handicap n = 21.
Discussion
We used an experimental handicapping approach in male blue tits to investigate how the manipulation of feeding effort by one parent affects the coordination of feeding visits of both parents. We hypothesized that handicapped males would be unable to maintain their levels of feeding (compared to control males) which could affect their turn taking strategy as such that males would strategically space their reduced number of visits so that they are alternated. However, males did not react to the handicapping as expected: neither visit rates nor turn taking differed between handicapped and unmanipulated males. Furthermore, contrary to our expectations that neither party can change its provisioning strategy unilaterally, we found that females had different provisioning rules when their partner was handicapped. We discuss below in detail how these findings fit within the current theory of conditional cooperation.
We hypothesized that the visit rate of handicapped males would be lower than that of unmanipulated males due to the increased flight costs (Pennycuick, 1982). Our results, however, indicate otherwise. There was no difference in the visit rates between unmanipulated and handicapped males. In contrast to other studies that found that individuals lowered their visit rates in response to feather clipping (e.g., blue tits Cyanistes caeruleus, Slagsvold and Lifjeld, 1990; tree swallows Tachycineta bicolor, Whittingham et al., 1994; great tits Parus major, Sanz et al., 2000). There are, however, some handicapping studies that also found no response of the focal individual (e.g., feather clipping: female great tits Parus major, Sanz et al., 2000; male black-legged kittiwakes Rissa tridactyla, Leclaire et al., 2011; female and male great tits Wegmann et al., 2015; weighting: female and male rock sparrows Petronia petronia, Griggio et al., 2005, 2008). Unfortunately, we cannot show whether males actually suffered from increased costs of flying, but considering the number of feathers that were taken it should have an effect on the males. However, another possible explanation why the male blue tits in our study did not lower their feeding rate is that blue tits are a short lived species, with a low probability of future reproduction (Dhondt, 1987; Linden and Møller, 1989; Lendvai et al., 2018). So that it might be more costly for the males in terms of their fitness to lower their visit rates, as this would negatively affect the quality or survival of the offspring, than to keep their feeding rate constant, although at a higher intrinsic cost. For these reasons, reducing or withdrawing care during this period would most likely compromise their lifetime reproductive output. Additionally, the nestlings also did not suffer from the treatment in their body mass when compared to control nests. Furthermore, our results show that females of handicapped partners had higher visit rates than the females of unmanipulated partners. A response of the female partner was also found in other studies in which males were handicapped by feather clipping (Sanz et al., 2000; reviewed in Harrison et al., 2009). Yet, in these studies the change in female behavior was interpreted as partial compensation for the reduction in care by the handicapped male (Harrison et al., 2009). Given the unaltered visit rates of handicapped males in our study, it seems unnecessary for females to compensate. One reason why females of handicapped partners had higher visit rates than females of unmanipulated partners, could be that the females visually noticed a decrease in the state of the handicapped males or that males vocally informed the females about their state (Kavelaars et al., 2019), but that they responded positively to the fact males maintained their feeding levels and were thus likely making a greater investment. Unfortunately, no data on adult mass changes or survival are available to prove whether males increased their investment relative to their capacity. Alternatively, the females in our study could have invested more into the brood to give a positive signal to their partner in order to reduce the propensity of the male to desert (Griggio et al., 2005), or because they are anticipating that the male will desert. Male desertion is in fact common in the polygynous blue tits, and females of handicapped males seem to invest as much as permanent uniparental females in our study population (unpublished data).
We then investigated how the manipulation of feeding effort by the male affects the coordination of feeding visits. To represent an adaptive parental strategy, turn taking should allow parents to adjust the timing and frequency of nest visits to their partners' visiting behavior, which may ultimately result in similar visit rates and thereby ameliorate sexual conflict (Johnstone et al., 2014; Johnstone and Savage, 2019). However, in our study the females with handicapped partners did not seem to follow the rules of turn taking, because they had higher visit rates than their male partner, irrespective of the level of turn taking. The response of the female could not have been triggered by changes in the visit rate or turn taking behavior of the handicapped male considering they were similar to that of control males. However, the same argumentation as explained above may apply, that females notice the lower state of the males, but also the extra effort of the males to maintain their feeding behavior (in terms of visit rate, turn taking and prey volume) which gives the females incentive to raise their visit rate while neglecting the rules of turn taking. This interpretation also could fit a different conditional cooperation concept, because it shows that when one partner (here the male) invests relatively more (given the increased effort by the handicapping for the maintaining its feeding rate), the other (here the female) is also more willing to invest (female increases visit rate). Thus, the reaction of the females can be seen as conditional cooperation—but not via turn-taking, the mechanism as suggested in Johnstone et al. (2014). Finally, understanding conditional cooperation may furthermore require to incorporate the costs and effort of each parental behavior (i.e., provisioning rate and quality, predator defense), rather than focusing on one common parental care trait.
Finally, we argued that the rate of visits is not the only aspect of nestling provisioning and that, for example, load size could vary, if only the number of feeding visits, but no other aspects are monitored. Here, the potential extra effort that the handicapped males had to make to maintain their visit rates, might negatively alter other aspects of the prey. Nevertheless, the males did not change the proportion of prey volumes they brought to the nest, which is in line with a previous study in this species (Griffioen et al., 2019). This suggests that parents cannot cheat by reducing the prey volumes, because they either visually monitor the prey their partner brings or in addition to visual monitoring they also use indirect information on partner care via the hunger levels of the nestling (Johnstone and Hinde, 2006; Johnstone et al., 2014). Thus, this aspect of the feeding visit remains an important part of determining the investment of parents and therefore could play an important role in the resolution of sexual conflict. An alternative possibility that could explain why both parents maintained the proportions of prey volumes is that the environmental conditions were excellent in our study year, perhaps resulting in abundant prey. Indeed, the higher visit rates of the females of handicapped partners did not negatively affect the proportion of prey volumes neither, while elevated visit rates are often associated with smaller prey (see Grieco, 2002; García-Navas and Sanz, 2010; Bowers et al., 2014).
Conclusions
We aimed to investigate whether turn taking is a behavioral strategy by manipulating individual visit rates. Surprisingly, handicapping males by feather clipping did not lead to lower visit rates, so we could not investigate whether individuals respond to reduced visit rates of their partner by delaying their own visits. Intriguingly, females increased the feeding effort in a way which resembled partial compensation, even though male feeding behavior was not reduced. Thus, it may be interesting to study how partial compensation relates to an evaluation of partner state or partner feeding behavior, which could also help to explain why females increased their visit rate and decreased their turn taking. We further speculate that the extra effort of the males might have been an incentive for the females to invest more as well, which is in line with the theory of conditional cooperation. Thus, it seems that the coordination of parental care within pairs is not exclusively related to visit rates, but that partners may use different information streams to co-adjust their behavior.
Data Availability
All datasets generated for this study are included in the manuscript and/or the Supplementary Files.
Ethics Statement
The animal study was reviewed and approved by Ethical Committee for animals (ECD) University of Antwerp (2015-85).
Author Contributions
MG, AI, and WM designed the experiment. MG and AI performed the experiment in the field. MG did the statistical analyses and wrote the first draft of the manuscript. All authors contributed to manuscript revision and approved the final submitted version.
Funding
This study was supported by the Fonds Wetenschappelijk Onderzoek—Vlaanderen (FWO) (Project ID: 1143817N to MG; 1517815N and 1211916N to AI). The funders had no role in the study design, analysis, and writing of the manuscript.
Conflict of Interest Statement
The authors declare that the research was conducted in the absence of any commercial or financial relationships that could be construed as a potential conflict of interest.
Acknowledgments
We thank Tommaso Biglino for assistance in the field. J. L. Savage, Stefan van Dongen, and Vincent Sluydts for statistical advice and providing statistical code.
Supplementary Material
The Supplementary Material for this article can be found online at: https://www.frontiersin.org/articles/10.3389/fevo.2019.00347/full#supplementary-material
Supplementary Table 1. Dataset used for the analyses within the current manuscript.
References
Bates, D., Mächler, M., Bolker, B., and Walker, S. (2015). Fitting Linear Mixed-Effects Models using lme4. J. Stat. Softw. 67, 1–48. doi: 10.18637/jss.v067.i01
Bebbington, K., and Hatchwell, B. J. (2016). Coordinated parental provisioning is related to feeding rate and reproductive success in a songbird. Behav. Ecol. 27, 652–659. doi: 10.1093/beheco/arv198
Bowers, E. K., Nietz, D., Thompson, C. F., and Sakaluk, S. K. (2014). Parental provisioning in house wrens: effects of varying brood size and consequences for offspring. Behav. Ecol. 25, 1485–1493. doi: 10.1093/beheco/aru153
Dhondt, A. A. (1987). Reproduction and survival of polygynous and monogamous Blue Tit Parus caeruleus. Ibis 129, 327–334. doi: 10.1111/j.1474-919X.1987.tb03176.x
Gächter, S. (2007). “Conditional cooperation: behavioral regularities from the lab and the field and their policy implications,” in Psychology and Economics: A Promising New Cross-Disciplinary Field, eds B. S. Frey, and A. Stutzer (Cambridge, MA: MIT Press, 19–50.
García-Navas, V., and Sanz, J. J. (2010). Flexibility in the foraging behavior of blue tits in response to short-term manipulations of brood size. Ethology 116, 744–754. doi: 10.1111/j.1439-0310.2010.01788.x
Grieco, F. (2002). Time constraint on food choice in provisioning blue tits, Parus caeruleus: the relationship between feeding rate and prey size. Anim. Behav. 64, 517–526. doi: 10.1006/anbe.2002.3073
Griffioen, M., Müller, W., and Iserbyt, A. (2019). A fixed agreement—consequences of brood size manipulation on alternation in blue tits. PeerJ. 7:e6826. doi: 10.7717/peerj.6826
Griggio, M., Matessi, G., and Pilastro, A. (2005). Should I stay or should I go? Female brood desertion and male counterstrategy in rock sparrows. Behav. Ecol. 16, 435–441. doi: 10.1093/beheco/ari009
Griggio, M., Mingozzi, T., Bortolin, F., and Pilastro, A. (2008). Trade-off between sexual activities and parental care: an experimental test using handicapped mates. Ethol. Ecol. Evol. 20, 155–164. doi: 10.1080/08927014.2008.9522535
Harrison, F., Barta, Z., Sze, T., Cuthill, I., and Székely, T. (2009). How is sexual conflict over parental care resolved? A meta-analysis. J. Evol. Biol. 22, 1800–1812. doi: 10.1111/j.1420-9101.2009.01792.x
Hinde, C. A. (2006). Negotiation over offspring care? - A positive response to partner-provisioning rate in great tits. Behav. Ecol. 17, 6–12. doi: 10.1093/beheco/ari092
Houston, A. I., and Davies, N. B. (1985). “The evolution of cooperation and life history in the dunnock, Prunella modularis,” in Behavioural Ecology: Ecological Consequences of Adaptive Behaviour, eds R. Sibley and R. S. Smith (Oxford: Blackwell Scientific), 471–487.
Ihle, M., Pick, J. L., Winney, I. S., Nakagawa, S., and Burke, T. (2019). Measuring up to reality: null models and analysis simulations to study parental coordination over provisioning offspring. Front. Ecol. Evol. 7:142. doi: 10.3389/fevo.2019.00142
Iserbyt, A., Fresneau, N., Kortenhoff, T., Eens, M., and Müller, W. (2017). Decreasing parental task specialization promotes conditional cooperation. Sci. Rep. 7:6565. doi: 10.1038/s41598-017-06667-1
Iserbyt, A., Griffioen, M., Borremans, B., Eens, M., and Müller, W. (2018). How to quantify animal activity from radio-frequency identification (RFID) recordings. Ecol. Evol. 8, 10166–10174. doi: 10.1002/ece3.4491
Iserbyt, A., Griffioen, M., Eens, M., and Müller, W. (2019). Enduring rules of care within pairs - how blue tit parents resume provisioning behaviour after experimental disturbance. Sci. Rep. 9:2776. doi: 10.1038/s41598-019-39139-9
Johnstone, R. A., and Hinde, C. A. (2006). Negotiation over offspring care - how should parents respond to each other's efforts? Behav. Ecol. 17, 818–827. doi: 10.1093/beheco/arl009
Johnstone, R. A., Manica, A., Fayet, A. L., Stoddard, M. C., Rodriguez-Girones, M. A., and Hinde, C. A. (2014). Reciprocity and conditional cooperation between great tit parents. Behav. Ecol. 25, 216–222. doi: 10.1093/beheco/art109
Johnstone, R. A., Manica, A., Fayet, A. L., Stoddard, M. C., Rodriguez-Gironés, M. A., and Hinde, C. A. (2016). Evidence for conditional cooperation: a response to Schlicht et al. Behav. Ecol. 27, e6–e7. doi: 10.1093/beheco/arw051
Johnstone, R. A., and Savage, J. L. (2019). Conditional cooperation and turn-taking in parental care. Front. Ecol. Evol. doi: 10.3389/fevo.2019.00335. [Epub ahead of print].
Kavelaars, M. M., Lens, L., and Muller, W. (2019). Sharing the burden: on the division of parental care and voclizations during incubation. Behav. Ecol. 30, 1062–1068. doi: 10.1093/beheco/arz049
Koenig, W. D., and Walters, E. L. (2016). Provisioning patterns in the cooperatively breeding acorn woodpecker: does feeding behaviour serve as a signal? Anim. Behav. 119, 125–134. doi: 10.1016/j.anbehav.2016.06.002
Kölliker, M., Richner, H., Werner, I., and Heeb, P. (1998). Begging signals and biparental care: nestling choice between parental feeding locations. Anim. Behav. 55, 215–222. doi: 10.1006/anbe.1997.0571
Leclaire, S., Bourret, V., Wagner, R. H., Hatch, S. A., Helfenstein, F., Chastel, O., et al. (2011). Behavioral and physiological responses to male handicap in chick-rearing black-legged kittiwakes. Behav. Ecol. 22, 1156–1165. doi: 10.1093/beheco/arr149
Lendvai, Á. Z., Akçay, Ç., Stanback, M., Haussmann, M. F., Moore, I. T., and Bonier, F. (2018). Male parental investment reflects the level of partner contributions and brood value in tree swallows. Behav. Ecol. Sociobiol. 72, 1–31. doi: 10.1007/s00265-018-2594-3
Leniowski, K., and Wegrzyn, E. (2018). Synchronisation of parental behaviours reduces the risk of nest predation in a socially monogamous passerine bird. Sci. Rep. 8:7385. doi: 10.1038/s41598-018-25746-5
Lessells, C. M., and McNamara, J. M. (2012). Sexual conflict over parental investment in repeated bouts: negotiation reduces overall care. Proc. R. Soc. B Biol. Sci. 279, 1506–1514. doi: 10.1098/rspb.2011.1690
Linden, M., and Møller, A. P. (1989). Cost of reproduction and covariation of life history traits in birds. Trends Ecol. Evol. 4, 367–371. doi: 10.1016/0169-5347(89)90101-8
Lozano, G. A., and Lemon, R. E. (1996). Male plumage, paternal care and reproductive success in yellow warblers, Dendroica petechia. Anim. Behav. 51, 265–272. doi: 10.1006/anbe.1996.0027
Lucass, C., Stöwe, M., Eens, M., and Müller, W. (2016). Favored parent–offspring trait combinations? On the interplay of parental and offspring traits. Behav. Ecol. 27, 134–140. doi: 10.1093/beheco/arv134
McNamara, J. M., Gasson, C. E., and Houston, A. I. (1999). Incorporating rules for responding into evolutionary games. Nature 401, 368–371. doi: 10.1038/43869
McNamara, J. M., Houston, A. I., Barta, Z., and Osorno, J. L. (2003). Should young ever be better off with one parent than with two? Behav. Ecol. 14, 301–310. doi: 10.1093/beheco/14.3.301
Pennycuick, C. J. (1982). The flight of petrels and albatrosses (Procellariiformes), observed in South Georgia and its vicinity. Philos. Trans. R. Soc. B Biol. Sci. 300, 75–106. doi: 10.1098/rstb.1982.0158
R Core Team (2018). R: A Language and Environment for Statistical Computing. Vienna: R Foundation for Statistical Computing. Available online at: https://www.R-project.org
Royle, N. J., Smiseth, P. T., and Kolliker, M. (2012). The Evolution of Parental Care. Oxford: Oxford University press. doi: 10.1093/acprof:oso/9780199692576.001.0001
Santema, P., Schlicht, E., and Kempenaers, B. (2019). Testing the conditional cooperation model: what can we learn from parents taking turns when feeding offspring? Front. Ecol. Evol. 7:94. doi: 10.3389/fevo.2019.00094
Santema, P., Schlicht, E., Schlicht, L., and Kempenaers, B. (2007). Blue tits do not return faster to the nest in response to either short- or long-term begging playbacks. Anim. Behav. 123, 117–127. doi: 10.1016/j.anbehav.2016.10.016
Sanz, J. J., Kranenbarg, S., and Tinbergen, J. M. (2000). Differential response by males and females of partner in the great tit manipulation contribution (Parus major). J. Anim. Ecol. 69, 74–84. doi: 10.1046/j.1365-2656.2000.00373.x
Savage, J. L., Browning, L. E., Manica, A., Russell, A. F., and Johnstone, R. A. (2017). Turn-taking in cooperative offspring provisioning: by-product of individual provisioning behaviour or active response rule? Behav. Ecol. Sociobiol. 71:162. doi: 10.1007/s00265-017-2391-4
Schlicht, E., Santema, P., Schlicht, R., and Kempenaers, B. (2016). Evidence for cooperation in biparental care systems? A comment on Johnstone et al. Behav. Ecol. 27, e2–e5. doi: 10.1093/beheco/arw036
Schwagmeyer, P. L., Mock, D. W., and Parker, G. A. (2002). Biparental care in house sparrows: negotiation or sealed bid? Behav. Ecol. 13, 713–721. doi: 10.1093/beheco/13.5.713
Slagsvold, T., and Lifjeld, J. T. (1990). Influence of male and female quality on clutch size in Tits (Parus Spp.). Ecology 71, 1258–1266. doi: 10.2307/1938263
Stearns, S. (1989). Trade-offs in life-history evolution. Funct. Ecol. 3, 259–268. doi: 10.2307/2389364
Trivers, R. L. (1972). “Parental investment and sexual selection,” in Sexual Selection and the Descent of Man 1871-1971 (Chicago, IL: Aldine), 136–207. doi: 10.4324/9781315129266-7
Wegmann, M., Voegeli, B., and Richner, H. (2015). Oxidative status and reproductive effort of great tits in a handicapping experiment. Behav. Ecol. 26, 747–754. doi: 10.1093/beheco/arv006
Whittingham, L. A., Dunn, P. O., and Robertson, R. J. (1994). Female response to reduced male parental care in birds: an experiment in tree swallows. Ethology 96, 260–269. doi: 10.1111/j.1439-0310.1994.tb01014.x
Keywords: conditional cooperation, turn taking, handicapping, sexual conflict, parental investment
Citation: Griffioen M, Iserbyt A and Müller W (2019) Handicapping Males Does Not Affect Their Rate of Parental Provisioning, but Impinges on Their Partners' Turn Taking Behavior. Front. Ecol. Evol. 7:347. doi: 10.3389/fevo.2019.00347
Received: 29 April 2019; Accepted: 02 September 2019;
Published: 18 September 2019.
Edited by:
James Luke Savage, University of Sheffield, United KingdomCopyright © 2019 Griffioen, Iserbyt and Müller. This is an open-access article distributed under the terms of the Creative Commons Attribution License (CC BY). The use, distribution or reproduction in other forums is permitted, provided the original author(s) and the copyright owner(s) are credited and that the original publication in this journal is cited, in accordance with accepted academic practice. No use, distribution or reproduction is permitted which does not comply with these terms.
*Correspondence: Maaike Griffioen, bWFhaWtlLmdyaWZmaW9lbkB1YW50d2VycGVuLmJl