- 1Seagrass Ecosystem Research Group and SEACAMS, College of Science, Swansea University, Swansea, United Kingdom
- 2Project Seagrass, Cardiff, United Kingdom
- 3Sustainable Places Research Institute, Cardiff University, Cardiff, United Kingdom
- 4Department of Ecology, Environment and Plant Sciences, Stockholm University, Stockholm, Sweden
Seagrass meadows are an important wetland habitat that have been degraded globally but have an important carbon storage role. In order to expand the restoration of these productive and biodiverse habitats methods are required that can be used for large scale habitat creation across a range of environmental conditions. The spreading of seagrass seeds has been proven to be a successful method for restoring seagrass around the world, however in places where tidal range is large such methods become limited by resultant water movements. Here we describe and test a method for deploying seagrass seeds of the species Zostera marina over large scales using a new, simple method “Bags of Seagrass Seeds Line (BoSSLine).” This method involved planting seeds and sediment using natural fiber hessian bags deployed along strings anchored onto the seabed. When deployed in a suitable environment 94% of bags developed mature seagrass shoots, unfortunately one site subjected to a large storm event resulted in sediment burial of the bags and no seed germination. Bags were filled with 100 seeds with each leading to the development of 2.37 ± 2.41 mature shoots (206 ± 87 mm in length) 10 months after planting. The method was proven successful however the experiments illustrated the need to ensure habitat suitability prior to their use. Low seed success rate was comparable to other restoration studies, however further trials are recommended to ensure ways to improve this rate. In conclusion, this study provides evidence for an effective, simple method “Bags of Seagrass Seeds Line (BoSSLine)” for deploying seeds of the seagrass Zostera marina over large scales.
Introduction
Seagrass meadows provide critically important ecosystem services to our planet, including the storage of carbon (Fourqurean et al., 2012; Röhr et al., 2018), supporting world fisheries production (Unsworth et al., 2018), and amongst many other things, helping prevent beach erosion (James et al., 2019). Unfortunately, seagrass meadows are one of the world's most threatened ecosystems and are rapidly disappearing in many parts of the world (Waycott et al., 2009).
Although seagrass meadows have long been considered the “ugly duckling” of marine conservation (Duarte et al., 2008), the urgent and unprecedented changes that are now required to avoid a climate change catastrophe mean that a dual need for conservation of existing meadows, and the active restoration of previously extirpated seagrass meadows, are emerging as potentially meaningful climate change mitigation strategies (Duarte et al., 2013). This is due to the high rates at which seagrass meadows store carbon. Under the Paris Climate Accord and the Katowice Climate Package, seagrass restoration can be considered with respect to Nationally Determined Contributions (NDCs) and therefore be part of defined emissions reduction targets or traded for carbon credits (Kuwae and Hori, 2019). With increasing interest in habitat restoration through a rewilding lens, there is now ever more interest in seagrass restoration (Ockendon et al., 2018). Seagrass conservation and restoration practices are coming of age and with it so is the need for improved methods in all potential seagrass environments.
In the UK, seagrass loss has been estimated to have been vast. Of Britain's 155 estuaries, only 20 now contain seagrass: an 85% decline since the 1920s (Hiscock et al., 2005), with little natural recovery, and continuing losses and degradation in many parts of the country still being observed (Jones and Unsworth, 2016; Unsworth et al., 2017a,b). Poor water quality, primarily driven by excess nutrients, is one of the largest threats faced by seagrass, both in the UK and globally (Grech et al., 2012; Jones et al., 2018). But with increased efforts to improve UK coastal water quality, there is now interest in taking steps to restore associated coastal ecosystems such as seagrass.
Seagrass restoration efforts in the UK have been limited with only a few minor trials ever taking place (RanwelI et al., 1974; Hockings and Tompsett, 2002). However, this field has grown rapidly around the world over the last 30 years, with many successful large-scale projects now documented (Reynolds et al., 2016; van Katwijk et al., 2016). “The field of dreams hypothesis” or the assumption that “if you build it, they will come” (Palmer et al., 1997) is the presumption of that restoration will engender a desirable ecological response. There is now growing evidence that successful seagrass restoration will result in such ecological improvements (Greiner et al., 2013).
These restoration successes are largely down to a plethora of experimental studies as well as the lessons learnt from numerous failed projects (van Katwijk et al., 2016). Arguably the most important finding from past global restoration efforts is that scale matters; large-scale planting increases plant survival. Scaling up spreads the risks, and the resultant increase in population growth rate enhances positive feedbacks, helping the seagrass to self-facilitate a more affable environment (van Katwijk et al., 2016).
Simple and reliable methods are needed for successful seagrass restoration that can be deployed on a large scale, the results of which can lead to meaningful changes to the coastal environment and act as a major component of broader climate change mitigation strategies. The large over production of seeds by some species of seagrass (e.g., Z. marina) creates significant simple, low cost, and potentially effective opportunities for their use in restoration. As such, meta-analysis of global restoration studies indicate that seed based planting may be amongst the most effective methods of restoration (van Katwijk et al., 2016). In the Chesapeake Bay, US, seagrass restoration has largely utilized seed based techniques and has had extensive success (Marion and Orth, 2010). Additionally, recent successful trials of Z. marina restoration in Sweden have also included the use of seeds (Infantes et al., 2016a,b). Projects planting seeds have used a range of methods such as spreading by hand at the water surface, the use of seed buoys (Pickerell et al., 2005), and more recently planting of seeds in coconut matting (Sousa et al., 2017). In many parts of the UK the effectiveness of any technique that involves the loose spreading of seed (e.g., seed buoys) is likely to be compromised by the very large tidal ranges and resultant fast tidal currents that can rapidly move seeds away from their intended location. Thus, a method is required that is both cost effective and simple, yet will still facilitate deployment of seeds on the seabed without dispersal from the intended restoration site. Previous studies describe the use of hessian bags (burlap) for deploying seagrass seeds (Harwell and Orth, 1999; Zhang et al., 2015). Here we adapt, trial and describe their use over a range of sites of high tidal range in West Wales. We name this method “Bags of Seagrass Seeds Line (BoSSLine)” for deploying seeds of the seagrass Z. marina over large scales.
Methods
Study Sites
Two pilot Proof of Concept (PoC) studies on seagrass “seed bag” use were conducted, one at Porthdinllaen in North Wales and the other in the Helford River, Cornwall (Figure 4). These PoC studies trialed the use of differing types of seed bags under various deployment methods. Based on the findings from these initial PoC studies a seagrass seed bag experiment was then conducted at a further three sites around Wales. The locations for this experiment were based on the use of a simple habitat suitability model to confirm their potential viability for seagrass growth (Brown, 2015). In addition, the three sites were inspected in May 2017 using dropdown video and hand grabs to confirm suitability of the sediment. These sites were Dale and Longoar in the Milford Haven Waterway, and Freshwater East on the Pembrokeshire Coast. Dale was known to have an unconfirmed record of seagrass (Kay, 1998), and Freshwater East is suspected to have a small patch due to fragments commonly washing up on its beach. Longoar Bay has a small meadow of seagrass, with areas surrounding it likely to be good potential seagrass habitat (similar exposure, depth, and sediment). The sites were all in the range of 1–3 m depth (below low water spring) with a maximum tidal range of 7.68 m. Sediment type varied from fine and very fine sand at Dale and Longoar, to course sand at Freshwater East and all sites are fully marine (Carey et al., 2015). Sea surface temperatures typically range from 8 to 17 degrees C. During this project we confirmed the location of a small patch of seagrass (Zostera marina) previously recorded in 1958 by Martin George (Kay, 1998) in Dale (51.704765°N, 5.159228°W). This patch was found to still have dense shoots and cover an area of ~5 m2. At these three locations a series of trials were conducted using seed bags to plant the seagrass Zostera marina.
Seed Collection
During August 2014, for the initial PoC, SCUBA divers were used to collect ripe, seed laden reproductive shoots from seagrass meadows at Durgan in the Helford River (Hockings and Tompsett, 2002). Seed-laden shoots were also collected (August 2015) for the second PoC study within an intertidal seagrass meadow at Porthdinllaen in North Wales (Bertelli and Unsworth, 2014). For the main study, ripe seed laden reproductive shoots were collected in August 2017 at Littlewick Bay in Milford Haven Waterway, West Wales.
For the PoC studies and the main experiment all shoots were randomly collected throughout the natural meadows with care taken to cause no damage to the rhizome. They were collected under permit from Natural Resources Wales (and Natural England for the Helford study). Reproductive shoots were transported to Swansea University (except the Helford seeds) where they were placed in aerated flow through aquaria (8–12°C) within a laboratory. Nylon mesh was used to prevent the loss of seeds and seagrass material. Tanks were regularly maintained and cleaned to reduce algal fouling. Matured seeds were naturally released from the flowering shoots and since seeds are negatively buoyant, they sank to the bottom of the tanks. Seeds were collected from the bottom of the tanks and were sieved to remove dead leaves and larger organic material before further separation in separating funnels (Infantes and Moksnes, 2018). Seeds were then stored in flowing seawater tanks.
Initial Proof of Concept Studies
For the initial PoC study in Helford, large hessian bags (80 × 33 cm) (of the type used as sand bags) were filled with local sediment (amount unquantified) from the site in August 2014 (by SCUBA divers using shovels) and mature seagrass seeds (still within their reproductive shoots) (10 spathes in each bag). Six bags were placed 1 m apart from each other in a line at two locations on the edge of an existing seagrass meadow and their location marked with GPS (Figure 4). The bags and their seedling production were monitored using SCUBA divers in April 2015.
For the second PoC study which was conducted during November 2015 at Porthdinllaen, smaller bags of seeds (13 × 7.5 cm with 1 mm holes in the fabric) were deployed. Ten bags were placed on the bare intertidal sediment at Porthdinllaen but weren't secured (see Figure 1A). These were patches of bare sediment within the local seagrass meadow. The bags used in the second PoC study were a much thicker weave hessian than within the Helford PoC pilot and the hessian had a silicon coating. Approximately 100 seeds were placed into each bag together with 100 cm3 of local sediment.
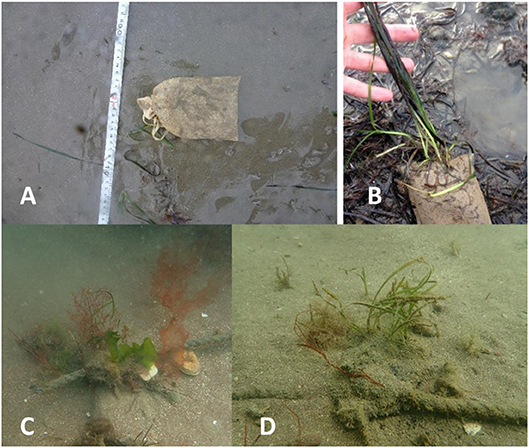
Figure 1. (A) Deployment of PoC seed bags at Porthdinllaen without anchoring. (B) Successful retrieval of 1 of 2 PoC seed bags at Porthdinllaen. (C) Seed bag containing seedlings at Dale with hessian rope degraded at one end. (D) Seed bag at Dale containing an abundance of young seagrass plants.
Seed Bag Experiment
Approximately 100 seeds, were placed into small hessian bags (see Figure 2) along with 100 cm3 of sediment. The uncoated hessian bags were 13 × 7.5 cm with 1 mm holes in the fabric. Surface sediment (top 5 cm) was carefully collected avoiding any sulfurous anoxic layers) within 100 m of an existing seagrass meadow at Littlewick Bay. Due to concerns about the potential for sediment to have low nutrient levels at the recipient site in Dale and to potentially not contain appropriate micro fauna and flora, 50 cm3 of seagrass detritus was added to the sediment bag to assist with microbial inoculation of the seagrass microbiome. The seagrass detritus was the degraded remains of the flowering shoots from which the seeds were collected. Bags were then tied and fixed along hessian rope at 1 m intervals. Each line of rope contained six seed bags (Figure 3). The process for creating seagrass seed bags is visually described in Figure 3.
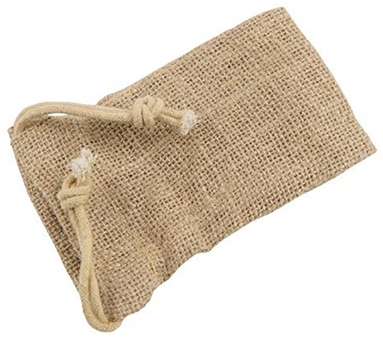
Figure 2. Small hessian bags (13 × 7.5 cm) filled with seagrass seeds. Sediment and seagrass detritus were also added. Bags were strung into lines for deployment at potential restoration sites.
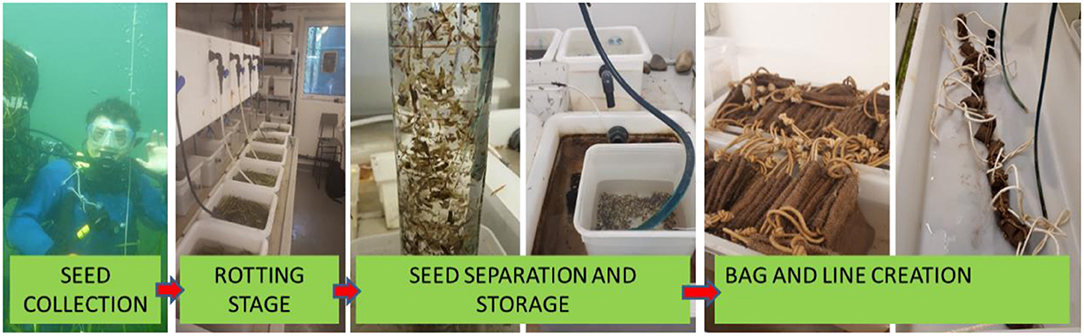
Figure 3. Stages of seed bag creation (1) reproductive shoot collection by SCUBA divers, (2) natural rotting of seagrass to allow for seed dropping, (3) separation of seeds from detritus, and (4) filling of bags with seeds, sediment, and detritus.
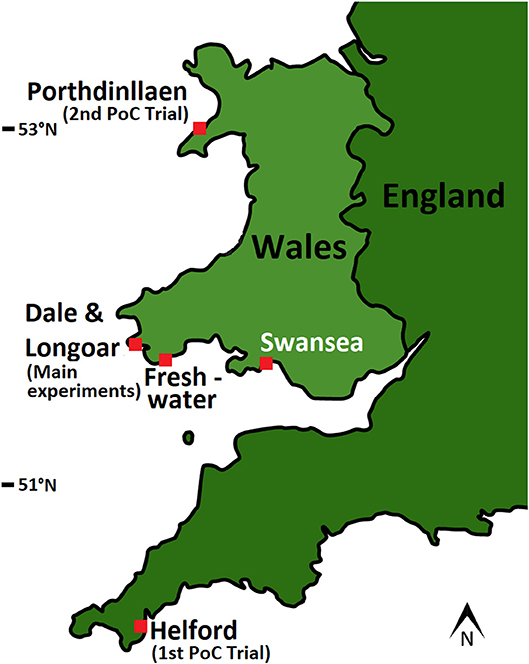
Figure 4. Location of the Proof of Concept (PoC) and main experimental sites used to trial seagrass seed bags.
Bag Deployment and Monitoring
Two lines of seed bags were placed at Dale, and one line was placed at both Longoar Bay and Freshwater East. A total of 24 bags were deployed across the three sites. The lines were held down using steel pegs and the ends of the lines marked approximately from the surface using GPS. All seed bags were deployed in November 2017 and then monitored for the shoot density and canopy height using SCUBA divers in May and August 2018.
Results
Proof of Concept Trial
The initial PoC study conducted in Helford was found to be very time intensive and difficult due to the use of large bags. These were too cumbersome for divers to work with and too large to be deployed from the surface. Assessment of the bags 8 months after deployment revealed that the bags had rapidly broken down and were mostly just observed as fragments. In the areas where the bags were placed, seedling density was determined to be on average (±SD) 5.2 ±3.7 per m2, this was ~4x the density of the seedlings in the surrounding area. Based upon these PoC findings further PoC trials were to be conducted using smaller bags.
Ten smaller seed bags were placed in Porthdinllaen in November 2015, but most of these were lost due to a major easterly storm event (the site faced east) just 2 weeks after deployment. Bags had not been secured to the sediment so were susceptible to displacement. After 3 months only three bags were still in situ, but in all, successful germination had taken place, each containing two seedlings. By October 2016 only two bags remained but contained five and six mature shoots, respectively, with mature rhizome present (see Figure 1B). The seagrass in these bags unfortunately hadn't rooted into the surrounding sediment and the bags were largely intact, possibly as a result of the type of hessian of the bags which were made from thick weave with silicon coated hessian. As a result of these trials, the bags in the following experimental study were changed to be of a lighter hessian comprising 100% natural fibers rather than those with fibers coated in silicon. Bags in the following experimental study were anchored down using pegs and lines.
Seed Bag Experiment
Twenty-four seed bags were deployed across the three sites (Dale, Longoar, and Freshwater East), and 16 of these were recorded by May 2018 to have developed at least one seedling (Figure 1D). In May 2018, 100% of bags at Dale were observed to contain seedlings (Figure 1C), whilst 80% of bags at Longoar contained seedlings (one bag was not observed as the rope had degraded and the bag was lost). All seed bags at Freshwater East failed to develop seedlings, we believe this could have resulted from poor siting of bags leading to sand movement burying them too deep. When the bags were relocated under the sediment, they had become completely anoxic and were blackened by sulfates.
Density of seedlings in May 2018 was on average (±SD) 2.95 ± 3.22 per bag, of those bags where shoots had developed there was an average (±SD) of 3.65 ± 2.09 shoots per bag. By August 2018 there was an overall average (±SD) density of 2.37 ± 2.41 shoots per bag with many of those shoots appearing mature (Figure 5). In May 2018, the average (±SD) shoot length was 41 ± 11 mm and by August 2018 this was 206 ± 87 mm.
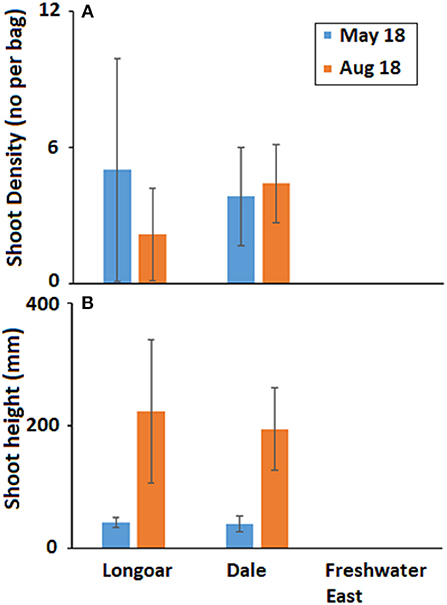
Figure 5. Mean (±SD) (A) seagrass shoot density (no per bag) and (B) shoot height (mm) developing from seed in hessian bags at three locations in South West Wales (UK) on two monitoring visits following deployment of seeds in November 2017.
In order to calculate a % seed success rate we excluded data from Freshwater East, based on the assessment that the failure of the seed bags was the result of a poor site choice rather than the seed bag method itself. The average number of seeds in any given bag was 100 and the average (±SD) shoot density was 3.6 ± 2.1 and so we estimate that seed success was 3.6%. Excluding data from Freshwater East we conclude that seed bags had a 94% success rate.
The hessian rope used in this detailed experiment broke down quickly and by May 2018 was mostly fragmented, making monitoring individual bags difficult, but illustrating the value of using such material for deployment without creating environmental pollution. Importantly, these small bags and simple metal pegs had withstood winter storm(s) without any damage or movement. The bags had also allowed for germination without potential predators (e.g., Carcinus maenas) consuming the abundant seeds and germinating seeds were able to grow through the hessian fabric. By the end of August 2018 all the hessian bags were now fragmented and had mostly broken down, with evidence of rhizomes growing beyond the hessian bags and becoming embedded into the surrounding sediment.
Discussion
There is increasing need for seagrass restoration to be conducted using simple methods over large scales, high reliability, and a low per unit cost. Here we document for the first time the use of the BOSSline method (Bags of Seagrass Seeds Line) for deploying seeds of the seagrass Zostera marina. Seedling establishment rates (the proportion of seeds generating a surviving seedling) was low (3.5%) but typical of an r-strategist species and comparable to many rates recorded in other studies and sites (Marion and Orth, 2010; Govers et al., 2016; Sousa et al., 2017). The reasons for such low rates could be local environmental conditions, health of the seeds, or even disease contamination (Govers et al., 2016). Further experiments are required to separate these low germination rates from the use of this BOSSline method. The BOSSline method is simple, in that beyond the initial collection of seeds, very little (if any) diver based work is required reducing costs and the skills required, however the downside is that in contrast to rudimentary seed based methods (e.g., seed buoys) (Pickerell et al., 2005) laboratory processing is required.
We believe that the complete failure of one of the experimental stations reflected a poor site choice rather than a failed method, this location was possibly too close to the intertidal zone and subject to very mobile sandy substrate. This highlights the need to use a high resolution within any habitat suitability model. It is important to be clear that successful restoration projects require suitable environmental conditions (van Katwijk et al., 2016) and only when conditions can be deemed suitable should projects be conducted.
Whilst success has been observed in the use of seed bags for planting seagrass seeds a more mechanistic understanding is still required as to the processes driving the germination and development of Zostera marina seeds. In the present study we speculatively included detritus into the seed bags, such inclusion may have enhanced the nutrient environment or added microbes that could potentially carry out a broad range of functions that potentially support the health and growth of aquatic plants (Ettinger et al., 2017; Crump et al., 2018). Given the rapidly expanding understanding of the seagrass microbiome (Fahimipour et al., 2017) and its potential mutualistic role in seagrass growth and production (Crump et al., 2018) more information is required as to the relative role of this in germination and seedling development.
Feedbacks, both positive and negative within seagrass systems have significant roles at a meadow scale, particularly with respect to restoration (Maxwell et al., 2017). This study doesn't examine these factors, but we recognize that the use of our BOSSline method does have the potential to reduce potential negative feedbacks present from polychaetes and crustaceans. The hessian bag not only keeps the seeds from dispersing due to tidal movements but also protects the seeds from burial or consumption (Infantes et al., 2016a).
In conclusion we present evidence of the effectiveness of a new seagrass seed planting method referred to as BOSSline. Our experiments show this method to be simple to conduct, and proven to result in successful germination. However, our low germination rates indicate further experimental studies are required to maximize method efficiency and determine factors influencing germination in these bags. An important benefit of this method is its capacity to be upscaled with ease to the meadow creation scale. In the US, large scale mechanized seed collection has enabled the harvesting of 10's of millions of seeds at a time for restoration. In order to potentially facilitate such large-scale restoration in the UK we present a method that could be used to plant such large seed quantities in conditions where tidal currents and adverse seasonal weather conditions necessitate ensuring seeds are not rapidly washed away from the restoration site.
Author Contributions
RU designed the method, took part in fieldwork, analyzed data, and wrote the paper. CB, SR, and NE contributed to the design of the method. All authors contributed to fieldwork and helped to write the paper.
Funding
Funding for this research came from the European Union Regional Development Fund to the project SEACAMS2.
Conflict of Interest Statement
The authors declare that the research was conducted in the absence of any commercial or financial relationships that could be construed as a potential conflict of interest.
The reviewer, MG, declared a shared affiliation, with no collaboration, with one of the authors, BJ, to the handling editor at time of review.
Acknowledgments
The authors wish to acknowledge the financial support of the European Union Regional Development fund through the SEACAMS and SEACAMS2 projects. We thank the following for their assistance with this work: Max Robinson, Keith Naylor, Evie Furness, Alison Palmer-Hargrave, Laura Hughes, and Mark Milburn.
References
Bertelli, C. M., and Unsworth, R. K. F. (2014). Protecting the hand that feeds us: seagrass (Zostera marina) serves as commercial juvenile fish habitat. Mar. Pollut. Bull. 83, 425–429. doi: 10.1016/j.marpolbul.2013.08.011
Brown, G. (2015). Modelling the potential distribution of Zostera marina in Wales (MRes Thesis). Swansea University, Swansea, United Kingdom.
Carey, D. A., Hayn, M., Germano, J. D., Little, D. I., and Bullimore, B. (2015). Marine habitat mapping of the Milford Haven Waterway, Wales, UK: comparison of facies mapping and EUNIS classification for monitoring sediment habitats in an industrialized estuary. J. Sea Res. 100, 99–119. doi: 10.1016/j.seares.2014.09.012
Crump, B. C., Wojahn, J. M., Tomas, F., and Mueller, R. S. (2018). Metatranscriptomics and amplicon sequencing reveal mutualisms in seagrass microbiomes. Front. Microbiol. 9:388. doi: 10.3389/fmicb.2018.00388
Duarte, C. M., Dennison, W. C., Orth, R. J. W., and Carruthers, T. J. B. (2008). The charisma of coastal ecosystems: addressing the imbalance. Estuar. Coasts 31, 233–238. doi: 10.1007/s12237-008-9038-7
Duarte, C. M., Losada, I. J., Hendriks, I. E., Mazarrasa, I., and Marbà, N. (2013). The role of coastal plant communities for climate change mitigation and adaptation. Nat. Climate Change 3, 961. doi: 10.1038/nclimate1970
Ettinger, C. L., Voerman, S. E., Lang, J. M., Stachowicz, J. J., and Eisen, J. A. (2017). Microbial communities in sediment from Zostera marina patches, but not the Z. marina leaf or root microbiomes, vary in relation to distance from patch edge. PeerJ 5:e3246. doi: 10.7717/peerj.3246
Fahimipour, A. K., Kardish, M. R., Lang, J. M., Green, J. L., Eisen, J. A., and Stachowicz, J. J. (2017). Global-scale structure of the eelgrass microbiome. Appl. Environ. Microbiol. 83:e03391-03316. doi: 10.1128/aem.03391-16
Fourqurean, J. W., Duarte, C. M., Kennedy, H., Marba, N., Holmer, M., Mateo, M. A., et al. (2012). Seagrass ecosystems as a globally significant carbon stock. Nat. Geosci. 5, 505–509. doi: 10.1038/ngeo1477
Govers, L. L., Man in ‘t Veld, W. A., Meffert, J. P., Bouma, T. J., van Rijswick, P. C., Heusinkveld, J. H., et al. (2016). Marine Phytophthora species can hamper conservation and restoration of vegetated coastal ecosystems. Proc. R. Soc. B Biol. Sci. 283:20160812. doi: 10.1098/rspb.2016.0812
Grech, A., Chartrand-Miller, K., Erftemeijer, P., Fonseca, M., McKenzie, L., Rasheed, M., et al. (2012). A comparison of threats, vulnerabilities and management approaches in global seagrass bioregions. Environ. Res. Lett. 7:024006. doi: 10.1088/1748-9326/7/2/024006
Greiner, J. T., McGlathery, K. J., Gunnell, J., and McKee, B. A. (2013). Seagrass restoration enhances “blue carbon” sequestration in coastal waters. PLoS ONE 8:e72469. doi: 10.1371/journal.pone.0072469
Harwell, M. C., and Orth, R. J. (1999). Eelgrass (Zostera marina L.) seed protection for field experiments and implications for large-scale restoration. Aquat. Bot. 64, 51–61. doi: 10.1016/S0304-3770(99)00008-X
Hiscock, K., Selwell, J., and Oakley, J. (2005). The Marine Health Check 2005: A Report to Gauge the Health of the UK's sea life. Godalming: WWF-UK.
Hockings, S., and Tompsett, P. E. (2002). The Location and Conservation of Eelgrass Beds in Cornwall and the Isles of ScillyVols 1 and 2. Project Series Number 5. Truro: ERCCIS Cornwall Wildlife Trust.
Infantes, E., Crouzy, C., and Moksnes, P.-O. (2016a). Seed predation by the shore crab Carcinus maenas: a positive feedback preventing eelgrass recovery? PLoS ONE 11:e0168128. doi: 10.1371/journal.pone.0168128
Infantes, E., Eriander, L., and Moksnes, P. O. (2016b). Eelgrass (Zostera marina) restoration on the west coast of Sweden using seeds. Mar. Ecol. Prog. Ser. 546, 31–45. doi: 10.3354/meps11615
Infantes, E., and Moksnes, P.-O. (2018). Eelgrass seed harvesting: flowering shoots development and restoration on the Swedish west coast. Aquat. Bot. 144, 9–19. doi: 10.1016/j.aquabot.2017.10.002
James, R. K., Silva, R., van Tussenbroek, B. I., Escudero-Castillo, M., Mariño-Tapia, I., Dijkstra, H. A., et al. (2019). Maintaining tropical beaches with seagrass and algae: a promising alternative to engineering solutions. Bioscience 69, 136–142. doi: 10.1093/biosci/biy154
Jones, B. L., Cullen-Unsworth, L. C., and Unsworth, R. K. F. (2018). Tracking nitrogen source using δ15N reveals human and agricultural drivers of seagrass degradation across the British Isles. Front. Plant Sci. 9:133. doi: 10.3389/fpls.2018.00133
Jones, B. L., and Unsworth, R. K. F. (2016). The perilous state of seagrass in the British Isles. R. Soc. Open Sci. 3. doi: 10.1098/rsos.150596
Kay, Q. O. N. (1998). A Review of the Existing State of Knowledge of the Ecology and Distribution of Seagrass Beds Around the Coast of Wales. Science Report 296. Countryside Council for Wales, Bangor.
Kuwae, T., and Hori, M. (2019). “The future of blue carbon: addressing global environmental issues,” in Blue Carbon in Shallow Coastal Ecosystems: Carbon Dynamics, Policy, and Implementation, eds T. Kuwae and M. Hori (Singapore: Springer, 347–373.
Marion, S. R., and Orth, R. J. (2010). Innovative techniques for large-scale seagrass restoration using Zostera marina (eelgrass) seeds. Restor. Ecol. 18, 514–526. doi: 10.1111/j.1526-100X.2010.00692.x
Maxwell, P. S., Eklöf, J. S., van Katwijk, M. M., O'Brien, K. R., de la Torre-Castro, M., Boström, C., et al. (2017). The fundamental role of ecological feedback mechanisms for the adaptive management of seagrass ecosystems—‘a review. Biol. Rev. 92, 1521–1538. doi: 10.1111/brv.12294
Ockendon, N., Thomas, D. H. L., Cortina, J., Adams, W. M., Aykroyd, T., Barov, B., et al. (2018). One hundred priority questions for landscape restoration in Europe. Biol. Conserv. 221, 198–208. doi: 10.1016/j.biocon.2018.03.002
Palmer, M. A., Ambrose, R. F., and Poff, N. L. (1997). Ecological theory and community restoration ecology. Restor. Ecol. 5, 291–300. doi: 10.1046/j.1526-100X.1997.00543.x
Pickerell, C. H., Schott, S., and Wyllie-Echeverria, S. (2005). Buoy-deployed seeding: Demonstration of a new eelgrass (Zostera marina L.) planting method. Ecol. Eng. 25, 127–136. doi: 10.1016/j.ecoleng.2005.03.005
RanwelI, D. S., Wyer, D. W., Boorman, L. A., Pizzey, J. M., and Waters, R. J. (1974). Zostera transplants in Norfolk and Suffolk, Great Britain. Aquaculture 4, 185–198.
Reynolds, L. K., Waycott, M., McGlathery, K. J., and Orth, R. J. (2016). Ecosystem services returned through seagrass restoration. Restor. Ecol. 24, 583–588. doi: 10.1111/rec.12360
Röhr, M. E., Holmer, M., Baum, J. K., Björk, M., Chin, D., Chalifour, L., et al. (2018). Blue carbon storage capacity of temperate eelgrass (Zostera marina) meadows. Global Biogeochem. Cycles 32, 1457–1475. doi: 10.1029/2018GB005941
Sousa, A. I., Valdemarsen, T., Lillebø, A. I, Jørgensen, L., and Flindt, M. R. (2017). A new marine measure enhancing Zostera marina seed germination and seedling survival. Ecol. Eng. 104, 131–140. doi: 10.1016/j.ecoleng.2017.04.004
Unsworth, R. K. F., Bertelli, C. M., Robinson, M., and Mendzil, A. F. (2017a). Status Review and Surveillance Recommendations for Seagrass (Zostera spp.) in Milford Haven Waterway. Report for The Milford Haven Waterway Environmental Surveillance Group.
Unsworth, R. K. F., Nordlund, L. M., and Cullen-Unsworth, L. C. (2018). Seagrass meadows support global fisheries production. Conserv. Lett. 12:e12566. doi: 10.1111/conl.12566
Unsworth, R. K. F., Williams, B., Jones, B. L., and Cullen-Unsworth, L. C. (2017b). Rocking the boat: damage to eelgrass by swinging boat moorings. Front. Plant Sci. 8:1309. doi: 10.3389/fpls.2017.01309
van Katwijk, M. M., Thorhaug, A., Marba, N., Orth, R. J., Duarte, C. M., Kendrick, G. A., et al. (2016). Global analysis of seagrass restoration: the importance of large-scale planting. J. Appl. Ecol. 53, 567–578. doi: 10.1111/1365-2664.12562
Waycott, M., Duarte, C. M., Carruthers, T. J. B., Orth, R. J., Dennison, W. C., Olyarnik, S., et al. (2009). Accelerating loss of seagrasses across the globe threatens coastal ecosystems. Proc. Natl. Acad. Sci. U.S.A. 106, 12377–12381. doi: 10.1073/pnas.0905620106
Keywords: seagrass, feedbacks (positive/negative), restoration, seagrass (Zostera), macrophytes (aquatic plants)
Citation: Unsworth RKF, Bertelli CM, Cullen-Unsworth LC, Esteban N, Jones BL, Lilley R, Lowe C, Nuuttila HK and Rees SC (2019) Sowing the Seeds of Seagrass Recovery Using Hessian Bags. Front. Ecol. Evol. 7:311. doi: 10.3389/fevo.2019.00311
Received: 25 January 2019; Accepted: 05 August 2019;
Published: 21 August 2019.
Edited by:
Jonathan Richir, Fonds National de la Recherche Scientifique, BelgiumReviewed by:
Yi Zhou, Institute of Oceanology (CAS), ChinaMartin Gullström, Stockholm University, Sweden
Eduardo Infantes, University of Gothenburg, Sweden
Copyright © 2019 Unsworth, Bertelli, Cullen-Unsworth, Esteban, Jones, Lilley, Lowe, Nuuttila and Rees. This is an open-access article distributed under the terms of the Creative Commons Attribution License (CC BY). The use, distribution or reproduction in other forums is permitted, provided the original author(s) and the copyright owner(s) are credited and that the original publication in this journal is cited, in accordance with accepted academic practice. No use, distribution or reproduction is permitted which does not comply with these terms.
*Correspondence: Richard K. F. Unsworth, r.k.f.unsworth@swansea.ac.uk