- 1Max Planck Weizmann Center for Integrative Archaeology and Anthropology, Max Planck Institute for Evolutionary Anthropology, Leipzig, Germany
- 2Department of Primatology, Max Planck Institute for Evolutionary Anthropology, Leipzig, Germany
- 3Taï Chimpanzee Project, Centre Suisse de Recherches Scientifiques, Abidjan, Côte d'Ivoire
Microscopic tooth wear studies on primates have largely focused on interspecific dietary comparisons, while few have addressed intraspecific variations, such as those among age groups. Here, we examined to what extent dietary shifts during ontogeny can be revealed from microscopic tooth wear in a western chimpanzee population using 3D surface texture (3DST) analysis. To this end, we analyzed feeding observation data of 14 chimpanzees of the Taï National Park (Ivory Coast) and matched them to 3DST data analyzed on two wear facets (f9, f3) of deciduous fourth premolars and permanent molars of 41 specimens (infants, juveniles, adolescents, adults) of the same population. We expected to find an age-dependent increase in texture complexity resulting from the more frequent consumption of seeds and insects in older compared to younger individuals. Furthermore, we expected the introduction of phytolith-producing plants to the diet of post-weaned individuals to result in many small and parallel-orientated 3DST features in juveniles, adolescents and adults compared to infants. We found that the 3DST pattern did not mirror the observed increase in dietary breadth from infants to adults. However, we found that age-dependent differences in the consumption of phytolith-producing plants were reflected to some extent in the 3DST pattern: infants and adolescents who spent more time feeding on phytolith-producing plants than older individuals had more parallel orientated 3DSTs with higher peaks, while adults had flatter and more randomly orientated 3DST features. Our results suggest that phytoliths as small abrasive particles may be of greater importance for the 3DST formation than food categories, such as fruits, leaves or seeds. However, compared to the variation in the feeding data, 3DST results show only little variation among age groups. We conclude that 3DST does not explicitly reflect ontogenetic dietary changes in chimpanzees. Rather, other factors, such as individual- or sex-based feeding habits as well as seasonal variation in dust accumulation, may be of greater importance for 3DST formation.
Introduction
The diet of chimpanzees (Pan troglodytes) includes mostly fruits, but also leaves, piths and other vegetative plant parts as well as animal resources (e.g., Hiraiwa-Hasegawa, 1990a; Tutin et al., 1991; Boesch and Boesch-Achermann, 2000; Morgan and Sanz, 2006; Pruetz, 2006; Fahy et al., 2013). This dietary diversity partly arises from the fact that four subspecies of chimpanzees have been recognized (Wilson and Reeder, 2005; Oates et al., 2008), which occupy a variety of naturally occurring and modified habitats, ranging from tropical rainforests (Boesch and Boesch, 1989) to bamboo forests (Basabose, 2002) and dry open savannas (Conklin-Brittain et al., 2006) in central Africa. While western chimpanzees (P. t. verus), which are most distinctive from the other subspecies (Morin et al., 1994; Becquet et al., 2007), consume fruits with a share of around 62% (Pruetz and Bertolani, 2007; Bogart and Pruetz, 2011), the amount of fruits in central chimpanzees (P. t. troglodytes) varies from 63% (Goualougo Triangle, Democratic Republic of Congo) to 76% (Lope Reserve, Gabon) (Tutin et al., 1991, 1997; Tutin and Fernandez, 1993; Morgan and Sanz, 2006). Nigeria-Cameroon chimpanzees (P. t. ellioti) show with around 91% the highest percentage of fruits in their diet (Dutton and Chapman, 2015), while eastern chimpanzees (P. t. schweinfurthii) show the lowest, ranging from 57% in Mahale (Tanzania) (Hiraiwa-Hasegawa, 1990a) to only 43% in Kahuzi (Demographic Republic of Congo).
Other than the reported differences in fruit content in the diet among chimpanzee subspecies, several authors have reported age-related feeding preferences within populations as well (e.g., Hiraiwa-Hasegawa, 1990a,b; Matsumoto, 2017). Such feeding preferences change throughout a chimpanzee's life, but they seem to be strongest early in life. Thus, infant chimpanzees introduce solid food to their diet at an age of around 4–5 months (Hiraiwa-Hasegawa, 1990a), which almost exclusively consists of ripe fruit (Smith et al., 2013). At an age of around 1.7 years the weaning process starts and encompasses a period up to 4 years (Fahy et al., 2014). This process is accompanied by dietary transitions, such as the introduction of new food items or changes in dietary proclivities. For example, infant eastern chimpanzees (up to 5 years of age) of the Mahale mountains (Tanzania) consume more young leaves and fewer matures leaves compared to their mothers (Hiraiwa-Hasegawa, 1990a), but in general they increase their feeding time on leaves between 2 and 3 years and again after 4 years of age (Matsumoto, 2017). Infant eastern chimpanzees of the Kanyawara community in Kibale National Park (Uganda) have a narrower dietary breadth compared to adolescent and adult chimpanzees. However, even after weaning is completed, at around 5 years of age (Fahy et al., 2014), authors reported on dietary changes. In western chimpanzees (P. t. verus) of the Taï National Park (Ivory Coast) post-weaned individuals commonly feed on phytolith-producing plants, while it was suggested that individuals up to 3 years complement their nutritional needs with a phytolith-free diet (Power et al., 2015). Furthermore, juvenile chimpanzees (5–10 years of age) of the Kanyawara community were observed to feed more often on ripe fruits than their mothers (Bray et al., 2018). In addition, a continuous increase in the feeding bouts on seed-rich Ficus fruits until an age of 10–13 years was documented (Bray et al., 2018). In Mahale, adolescent male chimpanzees feed less often on piths than adult males (Uehara, 1990). Additionally, certain feeding behaviors (e.g., nut cracking and ant dipping) require specific physical and cognitive abilities. It has been shown that the efficiency with which these foods can be accessed increases with age. For example, western chimpanzees begin to crack nuts efficiently at an age of 4 years (Boesch and Boesch-Achermann, 2000). Moreover, hunting and meat consumption is mainly observed in adult male chimpanzees; they only reluctantly share meat with adult females and young individuals (Boesch, 1994; Boesch and Boesch-Achermann, 2000).
Gathering data about feeding behavior and dietary proclivities during ontogeny can provide knowledge about somatic development, cognitive abilities and life history events. However, it is not always possible to obtain direct evidence, such as from focal observations or the analysis of food remains and fecal samples. An alternative approach to inferring the diet of an individual is dental microwear. This method is related to the examination of microscopic signatures on tooth wear facet surfaces that form and modify during an individual's life as the result of tooth use. Microwear signatures have traditionally been described two-dimensionally (2D) as pits and scratches (Gordon, 1984; Teaford and Robinson, 1989). Gordon (1982) analyzed 2D wear patterns in western chimpanzees and found that juveniles had a higher density of pits and scratches compared to adults. She proposed that diet might be not the factor explaining these differences since the dietary variation within one species is supposed to be small. Rather, she speculated that a varying enamel hardness between younger and older individuals could have led to distinct pit and scratch densities among age groups (Gordon, 1982). She proposed that the higher density of microwear features in juveniles might be linked to decreasing enamel hardness in older individuals, since longer exposure to wear might lead to softer inner enamel layers and microwear features are more easily erased or reworked. In a more recent study on buccal microwear, Pérez et al. (2011) found larger scratch densities in infant (2–5 years) Neanderthals than in older ones (6–13 years). The authors argued that these higher scratch densities in infants could be indicative of an abrasive diet, which is accompanied by early weaning. As an alternative explanation they proposed that the enamel of deciduous teeth is less resistant to abrasion, leading to higher scratch densities (Pérez et al., 2011). However, in the same study modern humans showed an increase in feature densities from infants to adolescents, which the authors related to an increase in harder food items after weaning (Pérez et al., 2011).
With the development of new optical three-dimensional (3D) techniques, known as 3D dental microwear texture analysis (SSFA) (Scott et al., 2005) and 3D surface texture analysis (3DST) (Schulz et al., 2010) wear signatures can now be measured in much higher detail and precision. For example, the latter allows for the quantification of various geometric aspects, such as surface height, feature density, and surface complexity. By using SSFA to study inter-individual dietary habits in mandrills Percher et al. (2018) found an indication for a positive correlation between the fill volume of texture features and age, which these authors assumed to be correlated with a slight increase in hard objects and monocotyledons consumed.
Other than microwear signatures caused by internal dietary abrasives, external dust or grit ingested together with the diet may contribute to wear (e.g., Covert and Kay, 1981; Nystrom et al., 2004), by increasing scratch formation frequencies (Romero et al., 2012). Recently, it was shown that the 3DST of chimpanzees of the Taï National Park is influenced by a yearly dust accumulation during dry periods, leading to surface textures with more fine furrows and dales (Schulz-Kornas et al., 2019). While wind-borne dust particles seem to affect the amount and size of surface texture features, at least in those chimpanzees of the Taï National Park, we assume that internal dietary abrasives affect the surface texture in a more versatile way depending on the dietary composition.
Here we investigate whether changes in dietary habits during ontogeny are reflected in the 3D microscopic tooth wear patterns in infant, juvenile, adolescent and adult individuals of a western chimpanzee population from the Taï National Park (Ivory Coast). To that end, we compare data from feeding observations to 3DST analysis data measured on deciduous premolars (dp4) and permanent molars (M1 and M2). While microwear studies frequently have concentrated on M1s and M2s it is unclear whether both permanent and deciduous teeth carry similar microwear signals. Pérez et al. (2011) suggested that differences in enamel hardness between deciduous and permanent teeth may lead to higher scratch densities in infants, which would then reveal structural differences in tooth enamel rather than dietary differences. However, combining teeth would not only contribute to an elevated sample size but also enable to include younger individuals to discover possible dietary transitions during early ontogeny. Furthermore, it has been shown that differences in microwear signals between facet types (phase I vs. phase II) vary among species (Krueger et al., 2008). While in hard-object feeders the dietary signal is more pronounced on phase II facets, in primates with a versatile diet both phase II and phase I facets reflect the diet (Krueger et al., 2008). We therefore test whether teeth- (dp4 and M1/M2) and facet-types (phase II facet 9 and phase I facet 3) show tooth-and facet-type-specific wear patterns or whether they can be combined in future studies.
To test for the relationship of diet and 3DST data we use two sets of predictions. First, we predict that if the dietary composition, especially of more challenging food parts (hard objects) like seeds and insect cuticles (Strait, 1993; Scott et al., 2009), changes throughout a chimpanzee's life, this change will be reflected in the microscopic tooth wear pattern. For example, fig fruits as a common part of a chimpanzee's diet are an assembly of drupes with a high amount of rather small seeds (1–2 mm) per fruit, while other seeds, such as seeds of Calpocalyx sp. or Gilbertiodendron sp., consumed by chimpanzees are up to 2–4 cm in size (Poorter, 2004) and in small number per fruit. Consequently a mixture of smaller and larger seeds, considered as hard objects, produce complex facet surfaces with pits/dales and scratches/valleys varying in size and density (Teaford and Robinson, 1989; Lucas, 2004; Scott et al., 2006). An increase in the amount of such hard objects in the diet has been shown to correlate with an increase in surface texture complexity (Ramdarshan et al., 2016), surface texture height and volume, and a decrease in feature area (Calandra et al., 2012; Schulz-Kornas et al., 2019). Kaiser et al. (2015) proposed that soft diets, i.e., those with low matrix resistance, allow for a closer contact of the occluding teeth during mastication and therefore result in smooth and less complex wear facets with shallow pits/dales and scratches/valleys. Consequently, if juveniles fed mainly on soft ripe fruits and young leaves, we expect to observe less complex, voluminous and isotropic wear facet surfaces combined with shallower dales or valleys. Furthermore, if the proportion of fig fruits (including their small seeds) and other large hard particles (e.g., large seeds and insects) increased with age, then we expect to find complex surfaces with larger reliefs and hills, valleys and dales varying in size in adolescent and adult individuals.
In addition to the above described millimeter-sized hard objects, there are micrometer-sized instrinsic abrasive particles in several monocotyledonous and dicotyledonous plant families, known as phytoliths (Mercader et al., 2000; Piperno, 2006; Power et al., 2015). Previous studies on grass leaf eating mammals have suggested that phytoliths cause a microscopic wear pattern of mainly fine scratches/valleys with an anisotropic orientation (Walker et al., 1978; Solounias and Semprebon, 2002; Ungar et al., 2003; Scott et al., 2006; Schulz et al., 2013b). Therefore, we predict that if infants have a reduced ingestion of phytoliths, their surface texture should clearly differ from juveniles, adolescents and adults. Thus, we expect the surface textures of infants to have less dales and valleys as well as more plateau-like features due to the reduced amount of small abrasive particles in the diet, while we expect to find the opposite in adolescents and adults.
Materials and Methods
Cranial Material
We analyzed a total of 79 molars [upper and lower deciduous fourth premolars (dp4), permanent first (M1), and second molars (M2)] from 41 chimpanzees (P. t. verus) in a free-ranging population from the Taï National Park (Ivory Coast) (Supplemental Data Table S1). The Taï chimpanzee skeletal material is part of the Taï osteological collection of the Taï Chimpanzee Project (Wittig, 2018) based at the Departments of Human Evolution and Primatology of the Max Planck Institute for Evolutionary Anthropology (MPI-EVA Leipzig, Germany). Forty individuals are of known sex (females N = 20, males N = 20) and 36 individuals of known age (2–40 years). Based on a scheme implemented by the Taï Chimpanzee Project (Wittig pers. comm.) we used four age groups classified by major life history characteristics: (1) infants (between birth and weaning, 0–5 years); (2) juveniles (weaned, but still mother dependent, 5–10 years); (3) adolescents (onset of female cycle, 10–13 years; males begin to dominate females and travel with other males, 10–15 years); (4) adults (social maturity and reproductive phase, females > 13 years, males > 15 years).
Long-Term Feeding Observation
Feeding duration and dietary composition of 14 Taï chimpanzees (infants N = 2, adolescents N = 2, adults N = 10) were extracted from a long-term observation database on chimpanzee behavior, which is part of the Taï Chimpanzee Project at the Taï National Park (Wittig, 2018). Feeding sequences were available for a period of up to 12 days prior to death of these individuals and fall within the range of the suggested duration of microwear formation for primates (Teaford and Oyen, 1989). Raw data of adult chimpanzee feeding observations were taken from Schulz-Kornas et al. (2019) (see Supplemental Data Table S1), but the food categories therein were adjusted for our data set. The feeding duration was calculated as the mean feeding time (in %) per day for each individual. This duration was then used to calculate the mean feeding time per age group (in % per day). For 10 individuals both dietary data and 3DST data were available (specimen with “ID,” see Supplemental Data Table S1), Additionally, we added feeding data of 4 living individuals from the Taï population (specimen without “ID,” see Supplemental Data Table S1), which were similar in age, sex and community to four specimens from the osteological collection for which no feeding data were available.
We used two sets of food categorization to describe the feeding behavior. First, we defined food categories (Ncategory = 12) based on different plant parts (e.g., seed, leaf, pith) and animal resources consumed. We subdivided the traditionally used but broad food categories, such as “fruits” and “seeds” into more precise categories, resulting in a more detailed description of the feeding habits. We distinguished between food categories with potential hard objects (e.g., seeds, fruit pulp with seeds) and softer food categories (e.g., fruit pulp, nut endosperm). Second, we classified the plant parts consumed based on presence or absence of phytoliths (Supplemental Data Table S2). We used published data on phytolith content in plant species from the Taï National Park, which are known to be eaten by the 14 chimpanzees analyzed here (see Supplementary Table 6 in Power et al., 2015). For plants not included in the study by Power et al. (2015) we complemented this information with phytolith-producing taxon information from Piperno (2006). Here, we only added plants to the phytolith-producing plant group if they were classified as a high-producing plant (see Table 1.1 in Piperno, 2006).
Surface Texture Analysis
We exclusively selected teeth which exhibited occlusal surfaces with slight to moderate wear, i.e., dentine exposure was limited to the cusp tip or to areas of no interest. Moreover, selected wear facets were clearly delimited from neighboring wear facets. We analyzed 3DSTs on facet 9 which is the standard phase II facet for primate dietary reconstructions. This facet is located on the buccal and slightly posterior facing slope of the protocone of upper molars, and on the lingual facing slope of the hypoconulid of lower molars (Kay and Hiiemae, 1974; Kay, 1977; Maier and Schneck, 1981). Additionally, we analyzed the buccal phase I facet 3, which is located on the lingual slope of the paracone, and the buccal and slightly mesial facing slope of the hypoconulid (Kay and Hiiemae, 1974; Maier and Schneck, 1981). Following the procedure of Schulz et al. (2010, 2013a) and Calandra et al. (2012), we took tooth molds, using a high resolution silicone imprint (Provil® novo Light C.D.2 regular set; Type 3; Heraeus Kulzer, Dormagen, Germany), of permanent upper and lower first and second molars on either the right or left side. For specimens with deciduous dentition we exclusively molded and analyzed the dp4 (also known as the second deciduous molar), because of their similar morphology and facet location to permanent molars (Swindler, 2002). The measurements of the 3DSTs were conducted using the high resolution confocal disc-scanning surface measuring system μsurf mobile (NanoFocus AG, Oberhausen, Germany) at the MPI-EVA. The measurement routine was performed according to Schulz et al. (2010). Three to four non-overlapping scans (each 160 × 160 μm) of each facet were taken using a 100 × long distance objective with a numerical aperture of 0.8. The resolution in x and y were 0.16 μm, noise threshold in z 0.002 μm, and step size in z 0.06 μm. Only measurements with at least 95% surface points and no observable residues were retained for further analyses. The surface texture analysis was performed in the μsoft analysis premium software version 7.4.8076 (NanoFocus AG, Oberhausen, Germany; a derivative of Mountains® Analysis software by Digital Surf, Besançon, France). Twenty-five 3DST parameters, divided into four analysis types, of which two are defined by ISO 25178 (International Organization for Standardization, 2012) and ISO 12781 (International Organization for Standardization, 2011), as well as motif and furrow analyses were selected to describe the wear facets (Supplemental Data Table S3). These parameters relate to aspects, such as height (Sq, Sp, S5p, S5v, FLTv, FLTp, meh, madf, metf ), area (Sha, Sda, mea), density (Spd, Sal, medf ), volume (Vv, Vvc, Vmp, mev), plateau size (Smr, Smc), anisotropy (Str), texture direction (Std), complexity (Sdr), and slope (Sdq).
Statistics
The statistical analyses were performed using the open-source software R 3.4.3 (R Development Core Team, 2017). The test-statistics were conducted following the suggestions of Calandra et al. (2012), using the robust Welch–Yuen heteroscedastic omnibus test (WYT) coupled with a heteroscedastic pairwise comparison test (analog to Dunnett's T3 test) and a heteroscedastic rank based test (according to Cliff's method) to detect significant differences between trimmed (15%) medians. According to Calandra et al. (2012) tests can be applied specifically to small data sets (with at least 3 specimens per group) (Calandra et al., 2012). The WYT tests whether at least one of the groups (infants, juveniles, adolescents or adults) has a different mean than the other groups. Whenever a significant difference was found, a heteroscedastic pair-wise test was subsequently employed to reveal the source of difference. However, the p-values in this test are not controlled for the so-called family-wise error. Therefore, the heteroscedastic rank based test was performed to control for the family-wise error via Hochberg's (1988) method. This test computes, for all pairs of groups, the probability that an observation from one group is smaller than from another group. The R packages xlsx version 0.4.2 (Dragulescu, 2012), doBy version 4.5.3 (Højsgaard et al., 2012), and R.utils version 1.12.1 (Bengtsson, 2015) were used. Group-wise comparison was conducted to test for variation between tooth type (deciduous vs. permanent) and among age groups. The tooth type comparison was only conducted on five juveniles, where both the dp4 and the M1 were fully erupted and present in the same specimen (see Supplemental Data Table S1). For the age group comparison we analyzed specimens that had died during wet and dry periods. We are aware that seasonality in the Taï National Park can affect 3DSTs (Schulz-Kornas et al., 2019), but due to a limited sample size for specimens died during dry periods (see Supplemental Data Table S1) we could not include seasonality as an extra factor in our age group analysis. However, additionally to the age group comparison using the whole data set (data set 1 = specimens from wet and dry period) we conducted the same tests using a reduced data set (data set 2 = specimens from wet periods only) to see if the removal of specimens whose 3DSTs may be affected by external abrasives (e.g., dust during the dry period) change the results from the age group comparison. Additionally, we selected 17 3DST parameters that fit our predictions but were not affected by seasonality in a previous study on 3DSTs in the same population (Schulz-Kornas et al., 2019).
Finally, we performed a Factor Analysis (FA) to compare the effect of facet type (f9 vs. f3). We selected 13 of the initial 25 3DST parameters (Sq, Sp, Vmp, Vv, Sdr, Str, Sal, Sdq, Smc, Smr, meh, madf, metf ) for the final FA using varimax rotation. We selected the 13 3DST parameters due to following criteria: no missing values and approximately normal distribution. The FA was justified as shown by the Kaiser-Meyer-Olkin measure of sampling adequacy (value > 0.5) using the function “paf” of the R package rela (Chajewski, 2009). The FA was calculated using the function “factanal” (for details see Supplementary Data S1).
For all analyses, upper and lower teeth were analyzed separately. Of the permanent molars, the first molar was the preferred tooth. If the first molar was not available, then the second permanent molar was used to increase the number of specimens.
Results
Feeding Observations
We quantified the mean feeding duration that infant, adolescent, and adult Taï chimpanzees spent feeding on selected food sources as a percentage for 24 h. The two major dietary classifications to describe the feeding behavior in each group are shown in Figure 1A for food categories and Figure 1B for phytolith production by different plants.
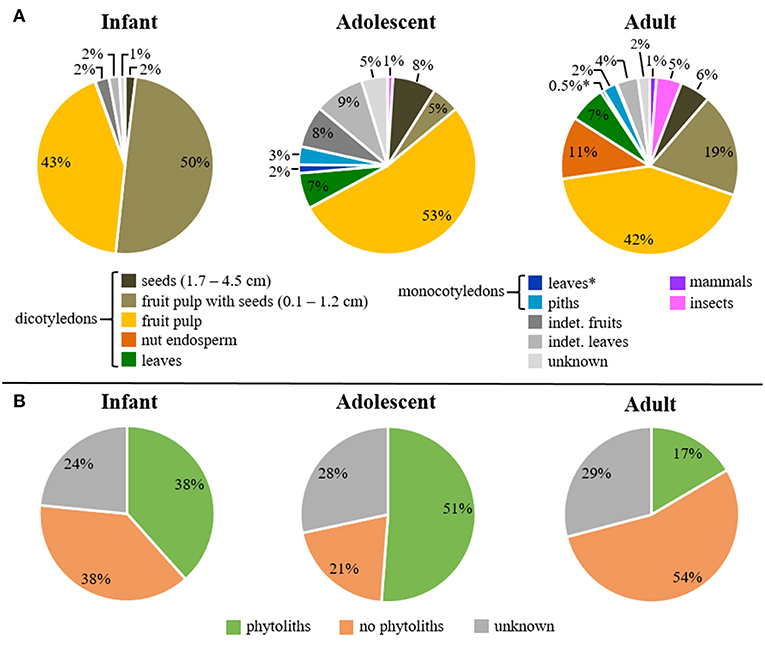
Figure 1. Mean daily feeding duration on (A) food categories and (B) phytolith-producing plants among age groups. Asterisks indicate food categories with percentages below 1% mean feeding duration per day.
Food Categories
Infant feeding time was almost exclusively limited to dicotyledonous fruits (50% fruit pulp with seeds, 43% fruit pulp) (Figure 1A). Likewise, adolescent individuals spent most of their feeding time on dicotyledonous fruits, including softer fruit pulp (53%) and fruit pulp with seeds (5%). They spent less time feeding on dicotyledonous seeds (8%), leaves (7%), indeterminate leaves (9%), and fruits (8%). Adult individuals varied more in their feeding time on dicotyledonous fruits, with 42% fruit pulp, 19% fruit pulp with seeds as well as 11% nut endosperm. Their feeding time on dicotyledonous seeds decreased to 6% while the feeding time on dicotyledonous leaves with 7% was very similar to adolescents. In general, monocotyledons and animal resources were relatively rare in all age groups. Adolescents spent 6% of their time feeding on monocotyledons (3% piths, 2% leaves). This was even lower in adults (3% piths, 1% leaves). Feeding on monocotyledons and animal resources (mammals, insects) was absent in infants. In adolescents, mammal consumption was not observed, but a small amount of insect consumption was present (1%). Adults further increased their feeding time on insects to 5%, and introduced mammal resources (meat and bone) to their diet (1% of the feeding time).
Phytolith Content
Individuals of all age groups spent most of their time feeding on dicotyledonous plants, which vary in the production of phytoliths (Supplemental Data Table S2). Of the three analyzed age groups, adolescents spent the largest proportion of feeding time on plants that produce phytoliths (51%), followed by infants (38%), and then adults (17%) (Figure 1B). Adults spent much more time feeding on non-phytolith-producing plants (54%), while the feeding time in infants is with 38% the same as for phytolith producing plants. Adolescents spent the least time feeding on plant parts that contain no phytoliths (21%).
Surface Texture
Comparison Between Tooth Types
We analyzed upper dp4 and M1 in individuals with mixed dentition (see Supplemental Data Table S1) to test for tooth type specific wear signatures. Table 1 shows the mean, median and standard deviation as well as the test statistics (Welch-Yuen only) of both tooth types on facet 9. Both, deciduous and permanent teeth did not differ in any of the 25 3DST parameters (p > 0.05). The same accounts for the upper facet 3 (Supplemental Data Table S4). Due to similarities in the 3DST parameters, we therefore mixed deciduous and permanent teeth in the subsequent age group comparison to increase specimen number.
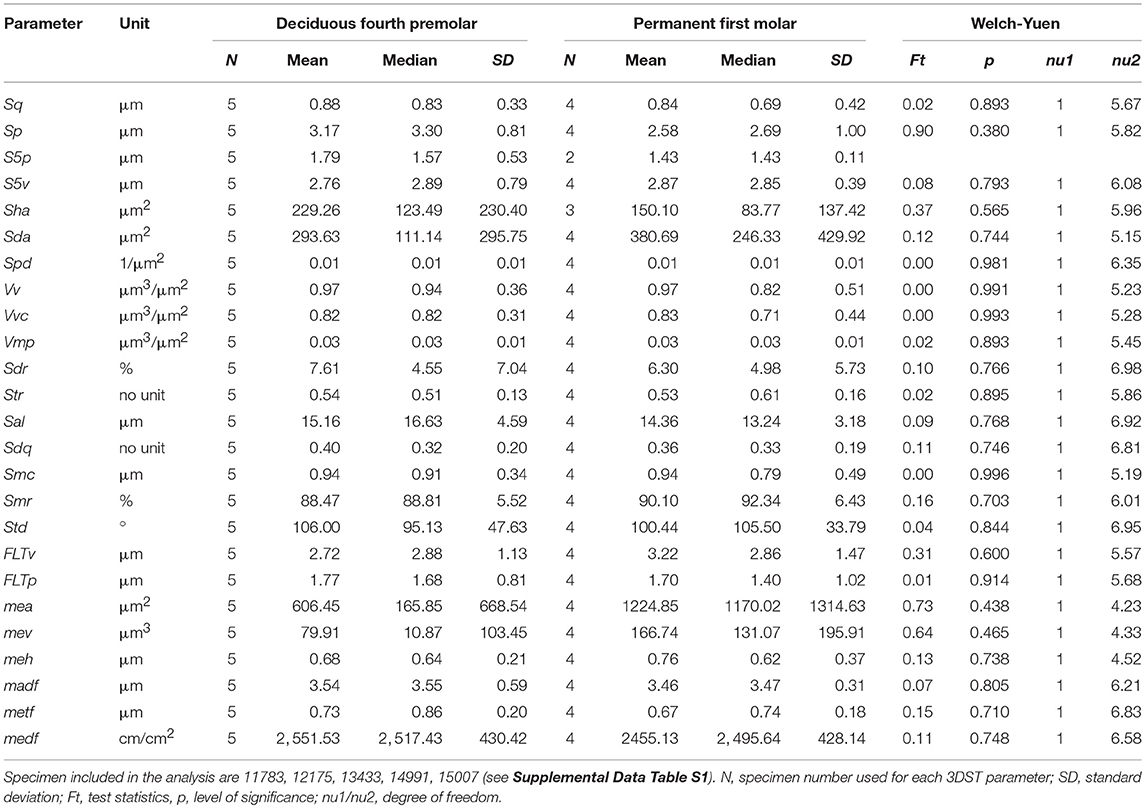
Table 1. Descriptive and test (Welch-Yuen) statistics for 3DST results of the upper facet 9 and compared between deciduous fourth premolars and permanent first molars.
Comparison Among Age Groups
The age group comparisons reveal similar results using both data sets (data set 1 = specimens died during wet and dry periods, Table 2; data set 2 = specimens died during wet periods only, Supplemental Data Table S5). Most distinct age group differences are displayed in isotropy (Str) and maximum peak height (Sp) no matter which of the two data sets was used (see Table 2; Supplemental Data Table S5). Therefore, the following result description refer to the whole data set (data set 1).
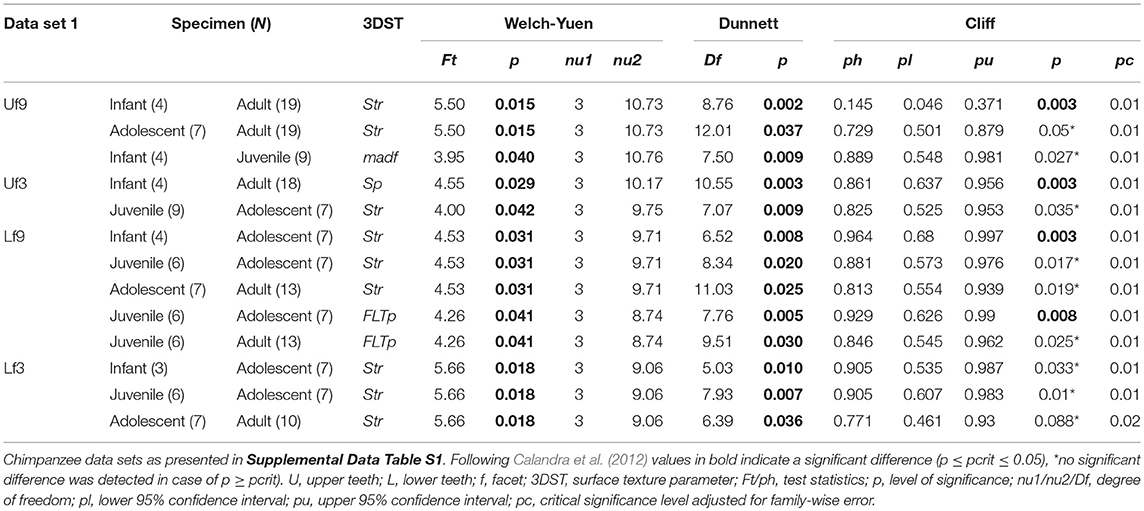
Table 2. Test statistics for the age group comparison given for significant 3DST data and sorted according to data set.
The FA for both upper and lower teeth (Figure 2) shows no separation of facet 9 and facet 3, assuming that both facets are similar in their 3DST. The FA reveals two factors with Eigenvalues ≥ 1, together explaining 80.4% of total variance for upper facets (Figure 2A), and three factors with Eigenvalues ≥ 1, together explaining 82.9% of total variance for lower ones (Figure 2B). For upper facets, the factor loadings (Table 3) suggest that the parameters Sq, Vv, Vmp, Sal, Smc, Smr, and meh contribute most to factor 1, whereas Sdr, Sdq, and metf contribute most to factor 2. For lower facets, the factor loadings (Table 3) suggest similar results with the parameters Sq, Sp, Vv, Vmp, Smc, Smr, and meh contribute most to factor 1, and parameters Sdr and Sdq contribute most to factor 2, whereas all parameters show weak loadings for factor 3.
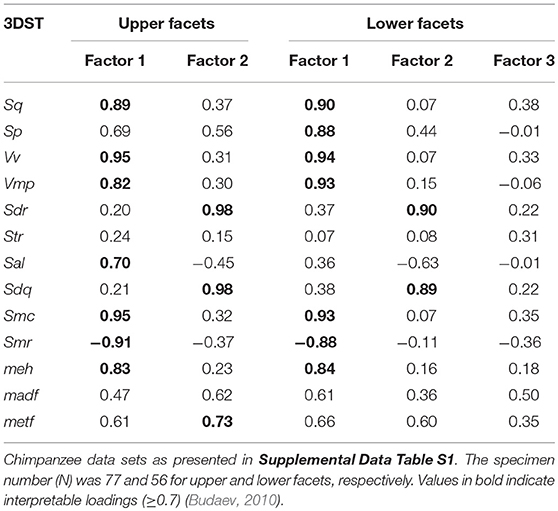
Table 3. Factor loadings for the facet type comparisons given for each 3DST parameter and factors with eigenvalues ≥1.
Of the 25 parameters we tested using the test statistics suggested by Calandra et al. (2012) (for details see section Statistics), only two parameters on facet 9 (Str, FLTp) and one parameter on facet 3 (Sp) show significant differences among age groups (Table 2). In general, facets 9 and 3 were similar in their surface texture pattern on both upper and lower teeth (Figure 3; Supplemental Data Table S6).
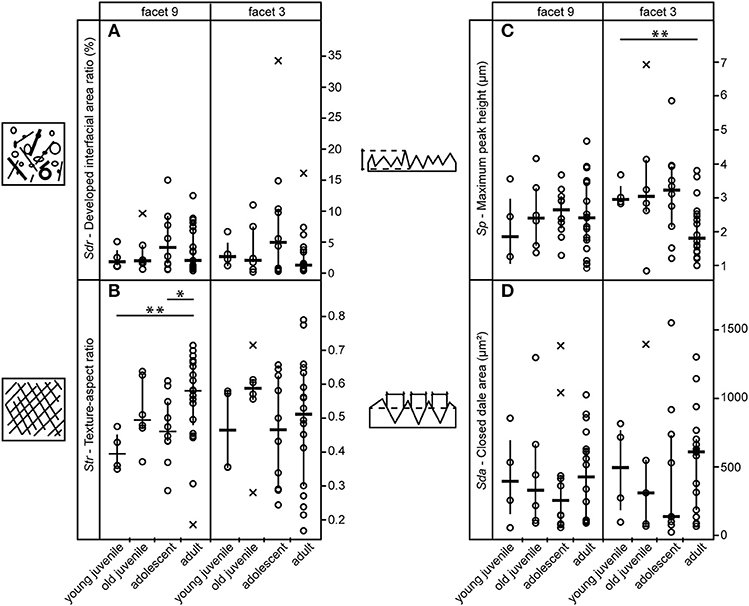
Figure 3. Variation among age groups shown for selected 3DST parameters on upper teeth. (A) Represents the level of complexity, (B) the level of isotropy, (C) the maximum peak height and (D) the area of hills. Strip charts of 3DST parameters indicate data median = horizontal line, interquartile range = vertical line, and outlier = x, significant differences are indicated by asterisks (**p < 0.01).
Contrary to our expectation, age groups did not differ in their surface texture complexity (Sdr) (Figure 3A), density of peaks (Spd), or furrows (medf ) (Supplemental Data Table S6). However, the orientation of the 3DST features (Str) varied among age groups in both upper and lower teeth (Table 2). Infants show a significantly smaller degree of isotropy on the upper facet 9 (smaller Str) than adults (pStr = 0.003) (Figure 3B), as well as a significantly higher degree of isotropy on the lower facet 9 (higher Str) than adolescents (pStr = 0.003). In general, adolescents tend to have more anisotropic orientated 3DST signatures on both facets (facet 9 and 3) compared to adults (Figure 3B; Table 2). Furthermore, infants have significantly larger peaks (Sp) than adults (pSp = 0.003) on the upper facet 3 (Figure 3C), as well as a trend for deeper furrows (madf ) than adolescents on the upper facet 9 (Table 2; Supplemental Data Table S6). Juveniles show larger peaks (FLTp) than adolescents on the lower facet 9 (pFLTp = 0.008), while infants and adults are intermediate (Supplemental Data Table S6). Those peaks additionally have wider areas, as indicated by the closed-hill area (Sha), in both infants and adults compared to juveniles and adolescents (trend only) (Figure 3D).
Discussion
It has been demonstrated that dental microwear analyses can discriminate between specific food items (e.g., fruits, leaves, seeds) (e.g., Scott et al., 2005) and can detect the ingestion of foods containing small abrasive particles, such as phytoliths (e.g., Walker et al., 1978). While such studies have generally focused on dietary proclivities of individuals within a single species using predominantly permanent teeth, the aim of the present study was to assess whether the distinct dietary preferences observed among four age groups (infant, juvenile, adolescent and adult) in the western chimpanzee Taï forest population are reflected in their microscopic tooth wear patterns (3DST data). We additionally investigated whether deciduous and permanent teeth as well as phase I and phase II facets yield similar 3DST signals. This study therefore provides new insights into 3DST formation patterns and their sensitivity to intra-populational behavioral adaptations, such as the introduction of solid food in infants, or dietary transitions after weaning.
Tooth Position and Facet Type (3DST)
Differences in enamel hardness between deciduous and permanent teeth may contribute to tooth-type-specific wear patterns and were suggested to result in higher scratch densities in infant individuals with deciduous teeth compared to individuals with permanent teeth (Pérez et al., 2011). Overall, in the Taï chimpanzees dp4s and M1s are similar in their 3DST pattern (see Table 1; Figure 2), showing no significant differences in feature density or any other 3DST parameters. In humans it was found that with increasing wear, there is an enamel maturation of the outer enamel layer characterized by increasing incorporation of calcium, phosphate and fluoride from saliva or external origins resulting in increased enamel hardness (Fanning et al., 1954). Further, the enamel maturation still occurs after an individual age of >10 years and occurs at a depth of up to 330 μm (Cardoso et al., 2009).
The comparison between the phase II facet 9 and the phase I facet 3 shows great overlap in their 3DST patters on both upper and lower teeth as indicated by the Factor Analysis (see Figure 2). This is in contrast to several microwear studies, which revealed specific microscopic signatures for phase I and phase II facets (e.g., Crompton and Hiiemae, 1969; Gordon, 1982; Krueger et al., 2008). For example it has been shown that in Cebus apella and Lophocebus albigena, which both consume hard objects, such as seeds, bark and palm fronds, phase II facets are often more complex in their microwear pattern compared to phase I facets (Krueger et al., 2008). In contrast, Alouatta palliata, which—akin to chimpanzees—has a highly versatile diet and mainly consumes leaves, stems, fruit seeds and fruit flesh, facet-type-specific wear patterns are less pronounced (Krueger et al., 2008). This therefore could suggest that a highly diverse and generalist diet may minimize differences in wear patterns between facet types compared to those of a dietary specialist.
Feeding Time and Dietary Composition
Dietary proclivities change throughout a chimpanzee's life, but this transition seems to be strongest early in life. Thus, young infant eastern chimpanzees consume mainly ripe fruits (Smith et al., 2013) and have a reduced dietary breath compared to other age groups (Bray et al., 2018). This is in line with our findings for western chimpanzees of the Taï forest: infants (2–3 years), which are still nursed, showed the narrowest dietary breadth and fed on six different food categories only (see Figure 1A). As expected, diet breadth increased in adolescent and adult chimpanzees by adding four and six other food categories to their dietary repertoire, respectively.
Yet, in contrast to observations on eastern chimpanzees of the Mahale mountains (Matsumoto, 2017), leaf consumption was almost absent in infant Taï chimpanzees. Rather, they fed almost exclusively on fruits (fruit pulp and fruit pulp with seeds, see Figure 1A), as observed in eastern chimpanzees at Kanyawara (Smith et al., 2013). The infants' feeding time on the category “fruit pulp with seeds” includes mostly Ficus fruits, concurring with an intake of a large amount of small seeds (mainly 1–2 mm). Other than observed for the eastern chimpanzees of the Kanyawara community (Bray et al., 2018) the consumption of Ficus fruits decreased with age in our data set (see Figure 1A: “fruit pulp with seeds” among age groups). The discrepancies between our results and the findings of Bray et al. (2018) may be due to the fact that these authors counted the items per minute ingested while we calculated the feeding duration independently of ingested items. In addition, it needs to be considered that the feeding observation data for the infant Taï chimpanzees were based on two individuals only. The observation period of these individuals was limited to selected days in May, June and November, and May and June are the 2 months of the year where fruit availability is low in the Taï forest (Boesch and Boesch-Achermann, 2000). Nevertheless, during this time several species of figs are available and hence are the primary food source (Doran, 1997). This may have led to an overrepresentation of feeding time on figs in the feeding observations of infant Taï chimpanzees. During the rest of the year Taï chimpanzees consume a wider range of fruit species, independent of age (see Supplemental Data Table S2), while in the Kanyawara chimpanzees figs are among the preferred fruit sources year round (Wrangham et al., 1993; Potts et al., 2009).
Furthermore, in infant Taï chimpanzees the feeding time on large seeds and insects was considerably low. This might be due to the fact that the permanent teeth with their larger occlusal area are still to erupt and therefore the ability to efficiently process large seeds is limited. Adolescents and adults increased their feeding time on such large seeds, but also on insects. Additionally, the feeding time on fruits (fruit pulp, fruit pulp with seeds), leaves and piths was similar in both age groups as also observed in adolescent and adult eastern chimpanzees (Bray et al., 2018).
Considering the plant genera and species consumed, phytolith-producing plants accounted for a considerable amount in the Taï chimpanzee's diet. Similar findings were reported by Power et al. (2015), who analyzed phytolith remains found on dental calculus of specimens of the same population, although these authors did not find any phytolith remains in individuals up to 3 years of age (corresponding to our “infants” group). Our analysis indicates that the consumption of phytolith-producing plants was highest in adolescents, followed by infants, while adults spent the least time feeding on such plants (see Figure 1B). One explanation for the discrepancies between the studies may be the preservation rate of different phytoliths (Cabanes and Shahack-Gross, 2015). Leaf and stem phytolith assemblages were found to be more stable than inflorescence phytoliths (Cabanes et al., 2011). In our data set, the calculated feeding time on phytolith-producing plants for infants arises almost exclusively from fruits, which were consumed shortly before the individual's death. Since these individuals were still nursed, the introduction of solid food containing phytoliths may date back only weeks or month before their death. It remains unclear whether during this short time period phytoliths would accumulate in the dental calculus sufficiently.
Age Groups (3DST)
We predicted that the 3DST pattern would vary among age groups depending on the amount of large hard objects (e.g., seeds, insect cuticles) consumed as well as on small abrasive particles (phytoliths) in their diet. However, the 3DST pattern was only affected by three of the 25 parameters investigated: (1) texture aspect ratio (Str) which is a measure of isotropy; (2) maximum peak height (Sp), and (3) peak-to-reference flatness deviation (FLTp). Nevertheless, our results reveal that phytoliths embedded in plant parts of several plant species (Piperno, 2006; Power et al., 2015) affect the 3DST in a greater way than large hard objects, such as seeds per se (see also above).
Previous studies showed that the consumption of seeds, considered as large hard particles, produce complex facet surfaces with features varying in size and density (Teaford and Robinson, 1989; Lucas, 2004; Scott et al., 2006). Contrary to our prediction, variation in feeding time that age groups spent on seeds and fruit pulp with seeds did not affect texture complexity and volume. This indicates that variably sized hard objects (1–2 mm seeds vs. 2–4 cm seeds) may be of minor importance for 3DST formation. Moreover, the amount of seeds did not affect texture complexity either. Even though infant chimpanzees in our data set fed on fruit pulp containing many small seeds to around 50% of their time, they do no show higher complexity values than the other age groups. As mentioned above, the category “fruit pulp with seeds” includes mostly Ficus fruits, which contain many seeds. However, these seeds are embedded in soft and fibrous fruit pulp. This may reduce the probability of seeds getting crushed, and consequently reduce the probability that those seeds affect the 3DSTs. Nevertheless, our findings confirm the results of Ramdarshan et al. (2016) showing that the size of seeds does not correlate with microwear complexity values (Ramdarshan et al., 2016).
In addition to the above described millimeter-sized hard objects, it was shown that the consumption of micrometer-sized instrinsic abrasive particles (i.e., phytoliths) cause specific microscopic wear patterns of mainly fine scratches/valleys with an anisotropic (parallel) orientation (e.g., Solounias and Semprebon, 2002; Ungar et al., 2007). The differential effect of phytoliths on microwear signatures is also highlighted by the comparison between P. troglodytes and folivorous Gorilla gorilla. Leaves are often associated with high phytolith content, which is related to a high level of anisotropy (parallel-orientated features) in gorillas. In contrast, chimpanzees are found to have more isotropic orientated wear features as the result of the consumption of fruits which often contain less or no phytoliths (Piperno, 2006; Scott et al., 2012). An alternative explanation for the different microwear pattern between gorillas and chimpanzees might be that leaves need to be sheared which results in a more horizontal and often more controlled chewing movement, while feeding on fruits often requires a more vertical (crushing) chewing movement (Lucas, 2004). Consequently, a more horizontal movement promotes the formation of anisotropic features.
Although there is no chewing kinematics data to support this, it is likely that due to their dietary similarity all age groups engage in more vertical (crushing) chewing movements and less in horizontal (shearing) movements.
Even though previous studies suggested that phytoliths cause a microscopic wear pattern of many fine scratches/valleys with an anisotropic orientation (Walker et al., 1978; Solounias and Semprebon, 2002; Ungar et al., 2003; Scott et al., 2006; Schulz et al., 2013b), feature density (Spd, medf ) did not differ among Taï chimpanzee age groups. However, we found more anisotropic (parallel) orientated texture features (Str values smaller than 0.5) in infants and adolescents compared to juveniles and adults (see Table 2). These differences are most evident between infants and adults, which can be distinguished by the amount of phytolith-producing plants in their diet (see Figure 1B). Juvenile Taï chimpanzees show, similar to adults, more isotropic orientated wear features, but we can only speculate if juveniles had a reduced consumption in phytolith-producing plants as well, since no feeding data were available for this age group. Juveniles are weaned but still mother dependent (pers. comm. Wittig). This leads to the suggestion that both, juveniles and their mothers, utilized the same feeding areas and food sharing may occur at least occasionally (Boesch, 1996), probably leading to a similar amount of phytolith-producing plants in their diet and consequently to a similar orientation of 3DSTs (see Figure 3B).
In addition to the higher level of isotropy in adults, indicating less phytoliths in the diet, we found shallower texture hills (smaller Sp, Figure 3C facet 3) than in infants as well as an indication for larger hill areas (larger Sha, Figure 3D facet 3) than in the other age groups. This is in line with the wear theory proposed by Kaiser et al. (2015), suggesting that a reduced concentration of abrasives results, among other things, in flatter 3DSTs.
Sex and Season Influence the Wear Pattern Stronger Than Age
Several studies reported on varying microwear patterns due to age, sex or seasonality (e.g., Gordon, 1982; Percher et al., 2018; Schulz-Kornas et al., 2019), which are mainly influenced by dietary variation but also by external factors (e.g., dust). For chimpanzees with a versatile diet (e.g., Hiraiwa-Hasegawa, 1990b; Tutin et al., 1991; Boesch and Boesch-Achermann, 2000; Morgan and Sanz, 2006) it raises the question as to whether the actual dietary variation of chimpanzees is too diverse (e.g., Tutin et al., 1991; Boesch and Boesch-Achermann, 2000; Morgan and Sanz, 2006; Pruetz, 2006) to reflect changes in single feeding categories in the 3DST pattern. Especially for the current data we believe, that for some feeding categories the feeding time is too short among age groups (see feeding time on leaves or seeds, Figure 1A) to be reflected in the 3DST parameters. It has been shown that texture signatures caused by a low-abrasive (soft) diet accumulate over a longer period of time—that is to say, the overwriting of the signatures takes longer than in a high-abrasive diet (Schulz et al., 2013b). A hard or high-abrasive diet, on the other hand, causes a high rate of continuous overwriting and consequently plateaus would be interrupted (resulting in narrower hills) by newly forming valleys and dales in a shorter time period (Calandra et al., 2012). Eventually, this may lead to a mixed texture signal if the diet consists of a mixture of such hard and high-abrasive as well as low-abrasive diet, as seen in the Taï chimpanzees.
Recent work has shown that different dietary preferences among male and female adult Taï chimpanzees correspond to differences in their 3DST patterns (Schulz-Kornas et al., 2019). Males tend to consume more meat, while females consume more nuts (Boesch and Boesch-Achermann, 2000). This results in 3DST patterns with more and deeper valleys for males and more plateaus for females (Schulz-Kornas et al., 2019). If such feeding preferences manifest already in younger individuals (older than 4 years of age), when the young chimpanzees efficiently start to crack nuts (Boesch and Boesch-Achermann, 2000), the sex-based signal may be reflected more strongly in the 3DST pattern than the ontogenetic signal. Another interesting point is that breastfeeding females were observed to have varying access to feeding places depending on their dominance rank (Boesch, 1997). Consequently, the diet among infants may also vary based on their mother's dominance rank. Compared to other chimpanzee populations, the Taï chimpanzees associate with each other more frequently (Boesch and Boesch-Achermann, 2000). This includes food sharing or feeding in larger groups (parties). Feeding parties are generally larger during the rainy period than during the dry period (Boesch and Boesch-Achermann, 2000), which is partly related to fruit availability. During ripe fruit scarcity (mainly in June and July) parties becomes smaller and chimpanzees become more solitary (Boesch and Boesch-Achermann, 2000). These behavioral observations may promote similarities in the 3DST pattern among age groups, but also between sexes or single individuals, at least seasonally.
Another seasonal factor that occurs during the dry period is the accumulation of dust particles on food plants in the Taï forest (Ivory Coast) due to a seasonal occurring trade wind (Stoorvogel et al., 1997; Adhvaryu et al., 2016). Although dust originating from the same trade-wind has been contested as the major cause for microwear in neighboring Ghana (Merceron et al., 2016), seasonal variation in dust accumulation plays a fundamental role in microwear formation of the Taï chimpanzees (Schulz-Kornas et al., 2019). The findings of Schulz-Kornas et al. (2019) support previous suggestions, that dust abrades teeth at even relatively low loads (Lucas et al., 2013; van Casteren et al., 2018). In the present analysis we can exclude the possibility that our results are biased by dust accumulation because the 3DST parameters suggesting age group differences (Str, Sp, FLTp) were previously shown to be unaffected by seasonality in the same population (Schulz-Kornas et al., 2019). Furthermore, we analyzed age groups using two data sets (data set 1 = specimens died during wet and dry periods, data set 2 = specimens died during wet periods only), which revealed similar 3DST results.
Conclusion
Understanding 3DST formation patterns and their relationship to diet and functional or morphological (e.g., enamel hardness) characteristics is essential for reconstructing the feeding ecology of a species, a population or among individuals. We found no differences between dp4 and M1 within the same specimens, leading to the suggestion that both tooth types can safely be combined in microscopic tooth wear studies. Furthermore, we found some evidence that the proportion of time spent feeding on phytolith-producing plants has a larger influence on 3DST than large hard objects, such as seeds. However, based on our and the findings of Schulz-Kornas et al. (2019) we conclude that within the Taï chimpanzees 3DST pattern does not explicitly reflect ontogenetic dietary changes. Rather, other factors, such as individual or sex-based feeding habits as well as seasonal variation in dust accumulation may be of greater importance for 3D surface texture formation. Certainly, it needs to be investigated in future studies whether the assumptions we made for the Taï chimpanzees can be affirmed for other chimpanzee populations. This will provide information about whether these findings are population-specific or not. To reduce the possibility of additional factors that might influence the microwear pattern the same subspecies should be tested, ideally one which undergoes similar seasonal changes as the Taï chimpanzees. Addressing this issue in future studies may facilitate interpretations about dietary habits and/or environmental conditions in specimens where no behavioral or ecological data are available.
Data Availability
The data (feeding observation and surface texture) supporting the findings of this study are accessible through the open access data repository of the Max Planck Society (https://dx.doi.org/10.17617/3.2q).
Author Contributions
ES-K, JS, and KK designed the study. JS, ES-K, and RW collected the data. JS and ES-K analyzed the data. JS wrote the paper with input from the other co-authors.
Conflict of Interest Statement
The authors declare that the research was conducted in the absence of any commercial or financial relationships that could be construed as a potential conflict of interest.
Acknowledgments
We thank the Ministère de l'Enseignement Supérieur et de la Recherche Scientifique and the Ministère de Eaux et Fôrests in Côte d'Ivoire, and the Office Ivoirien des Parcs et Réserves for their permission to conduct the long-term observation of the Taï chimpanzees. We are grateful to Christophe Boesch (DP, MPI-EVA) and Jean-Jacques Hublin (DHE, MPI-EVA) for granting us access to the skeletal collection at the MPI-EVA. We also thank the field staff of the Taï Chimpanzee Project for collecting the feeding observation data of the chimpanzees in the field, and Luke Premo (Washington State University) for constructive comments and correction of English language. This research was supported by the Max-Planck-Society.
Supplementary Material
The Supplementary Material for this article can be found online at: https://www.frontiersin.org/articles/10.3389/fevo.2019.00298/full#supplementary-material
References
Adhvaryu, A., Bharadwaj, P., Fenske, J., Nyshadham, A., and Stanley, R. (2016). Dust and Death: Evidence from the West African Harmattan. The Centre for the Study of African Economies (CSAE). Cambridge: University of Oxford.
Basabose, A. K. (2002). Diet composition of chimpanzees inhabiting the montane forest of Kahuzi, Democratic Republic of Congo. Am. J. Primatol. 58, 1–21. doi: 10.1002/ajp.10049
Becquet, C., Patterson, N., Stone, A. C., Przeworski, M., and Reich, D. (2007). Genetic structure of chimpanzee populations. PLoS Genet. 3:e66. doi: 10.1371/journal.pgen.0030066
Boesch, C. (1994). Cooperative hunting in wild chimpanzees. Anim. Behav. 48, 653–667. doi: 10.1006/anbe.1994.1285
Boesch, C. (1996). “Social grouping in Tai chimpanzees,” in Great Ape Societies, eds W. McGrew, L. Marchant, and T. Nishida (Cambridge: Cambridge University Press, 101–113.
Boesch, C. (1997). Evidence for dominant wild female chimpanzees investing more in sons. Anim. Behav. 54, 811–815. doi: 10.1006/anbe.1996.0510
Boesch, C., and Boesch, H. (1989). Hunting behavior of wild chimpanzees in the Taï National Park. Am. J. Phys. Anthropol. 78, 547–573. doi: 10.1002/ajpa.1330780410
Boesch, C., and Boesch-Achermann, H. (2000). The Chimpanzees of the Taï Forest: Behavioural Ecology and Evolution. Oxford: Oxford University Press.
Bogart, S. L., and Pruetz, J. D. (2011). Insectivory of savanna chimpanzees (Pan troglodytes verus) at Fongoli, Senegal. Am. J. Phys. Anthropol. 145, 11–20. doi: 10.1002/ajpa.21452
Bray, J., Emery Thompson, M., Muller, M. N., Wrangham, R. W., and Machanda, Z. P. (2018). The development of feeding behavior in wild chimpanzees (Pan troglodytes schweinfurthii). Am. J. Phys. Anthropol. 165, 34–46. doi: 10.1002/ajpa.23325
Budaev, S. V. (2010). Using principal components and factor analysis in animal behaviour research: caveats and guidelines. Ethology 116, 472–480. doi: 10.1111/j.1439-0310.2010.01758.x
Cabanes, D., and Shahack-Gross, R. (2015). Understanding fossil phytolith preservation: the role of partial dissolution in paleoecology and archaeology. PLoS ONE 10:e0125532. doi: 10.1371/journal.pone.0125532
Cabanes, D., Weiner, S., and Shahack-Gross, R. (2011). Stability of phytoliths in the archaeological record: a dissolution study of modern and fossil phytoliths. J. Archaeol. Sci. 38, 2480–2490. doi: 10.1016/j.jas.2011.05.020
Calandra, I., Schulz, E., Pinnow, M., Krohn, S., and Kaiser, T. M. (2012). Teasing apart the contributions of hard dietary items on 3D dental microtextures in primates. J. Hum. Evol. 63, 85–98. doi: 10.1016/j.jhevol.2012.05.001
Cardoso, C. A. B., Magalhães, A. C., Rios, D., and Lima, J. E. O. (2009). Cross-sectional hardness of enamel from human teeth at different posteruptive ages. Caries Res. 43, 491–494. doi: 10.1159/000264687
Conklin-Brittain, N. L., Knott, C. D., and Wrangham, R. W. (2006). “Energy intake by wild chimpanzees and orangutans: methodological considerations and a preliminary comparison,” in Feeding Ecology in Apes and Other Primates, eds G. Hohmann, M. M. Robbins, and C. Boesch (New York, NY: Cambrigde University Press, 445–471.
Covert, H. H., and Kay, R. F. (1981). Dental microwear and diet: implications for determining the feeding behaviors of extinct primates, with a comment on the dietary pattern of Sivapithecus. Am. J. Phys. Anthropol. 55, 331–336. doi: 10.1002/ajpa.1330550307
Doran, D. (1997). Influence of seasonality on activity patterns, feeding behavior, ranging, and grouping patterns in Taï chimpanzees. Int. J. Primatol. 18, 183–206. doi: 10.1023/A:1026368518431
Dragulescu, A. A. (2012). xlsx: Read, Write, Format Excel 2007 and Excel 97. 2000/XP/2003 files (R package version 042).
Dutton, P., and Chapman, H. (2015). Dietary preferences of a submontane population of the rare Nigerian-Cameroon chimpanzee (Pan troglodytes ellioti) in Ngel Nyaki Forest Reserve, Nigeria. Am. J. Primatol. 77, 86–97. doi: 10.1002/ajp.22313
Fahy, G. E., Richards, M., Riedel, J., Hublin, J.-J., and Boesch, C. (2013). Stable isotope evidence of meat eating and hunting specialization in adult male chimpanzees. Proc. Natl. Acad. Sci. U.S.A. 110, 5829–5833. doi: 10.1073/pnas.1221991110
Fahy, G. E., Richards, M. P., Fuller, B. T., Deschner, T., Hublin, J., and Boesch, C. (2014). Stable nitrogen isotope analysis of dentine serial sections elucidate sex differences in weaning patterns of wild chimpanzees (Pan troglodytes). Am. J. Phys. Anthropol. 153, 635–642. doi: 10.1002/ajpa.22464
Fanning, R. J., Shaw, J. H., and Sognnaes, R. F. (1954). Salivary contribution to enamel maturation and caries resistance. J. Am. Dent. Assoc. 49, 668–671. doi: 10.14219/jada.archive.1954.0197
Gordon, K. D. (1982). A study of microwear on chimpanzee molars: implications for dental microwear analysis. Am. J. Phys. Anthropol. 59, 195–215. doi: 10.1002/ajpa.1330590208
Gordon, K. D. (1984). The assessment of jaw movement direction from dental microwear. Am. J. Phys. Anthropol. 63, 77–84. doi: 10.1002/ajpa.1330630110
Hiraiwa-Hasegawa, M. (1990a). “A note on the ontogeny of feeding,” in The Chimpanzees of Mahale Mountains, ed N. Toshisada (Tokyo: Tokyo University Press, 277–283.
Hiraiwa-Hasegawa, M. (1990b). “Role of food sharing between mother and infant in the ontogeny of feeding behavior,” in The Chimpanzees of Mahale Mountains, ed N. Toshisada (Tokyo: University Press, 267–275.
Hochberg, Y. (1988). A sharper Bonferroni procedure for multiple tests of significance. Biometrika 75, 800–802. doi: 10.1093/biomet/75.4.800
Højsgaard, S., Halekoh, U., Robinson-Cox, J., Wright, K., and Leidi, A. (2012). doBy: Groupwise Summary Statistics, General Linear Contrasts, Population Means (Least-Square-Means), and Other Utilities. R package version 4.5-3.
International Organization for Standardization (2011). ISO 12781-1–Geometrical Product Specifications (GPS)–Flatness–Part 1: Vocabulary and Parameters of Flatness. ISO.
International Organization for Standardization (2012). ISO 25178-2–Geometrical Product Specifications (GPS)–Surface Texture: Areal–Part 2: Terms, Definitions and Surface Texture Parameters. ISO.
Kaiser, T. M., Clauss, M., and Schulz-Kornas, E. (2015). A set of hypotheses on tribology of mammalian herbivore teeth. Surf. Topogr. Metrol. Prop. 4:14003. doi: 10.1088/2051-672X/4/1/014003
Kay, R. F. (1977). The evolution of molar occlusion in the Cercopithecidae and early catarrhines. Am. J. Phys. Anthropol. 46, 327–352. doi: 10.1002/ajpa.1330460213
Kay, R. F., and Hiiemae, K. M. (1974). Jaw movement and tooth use in recent and fossil primates. Am. J. Phys. Anthropol. 40, 227–256. doi: 10.1002/ajpa.1330400210
Krueger, K. L., Scott, J. R., Kay, R. F., and Ungar, P. S. (2008). Technical note: dental microwear textures of “Phase I” and “Phase II” facets. Am. J. Phys. Anthropol. 137, 485–490. doi: 10.1002/ajpa.20928
Lucas, P. W. (2004). Dental Functional Morphology: How Teeth Work. Cambridge: Cambridge University Press.
Lucas, P. W., Omar, R., Al-Fadhalah, K., Almusallam, A. S., Henry, A. G., Michael, S., et al. (2013). Mechanisms and causes of wear in tooth enamel: implications for hominin diets. J. R. Soc. Interface 10, 20120923–20120923. doi: 10.1098/rsif.2012.0923
Maier, W., and Schneck, G. (1981). Konstruktionsmorphologische Untersuchungen am Gebiß der hominoiden Primaten. Z. Morphol. Anthropol. 72, 127–169.
Matsumoto, T. (2017). Developmental changes in feeding behaviors of infant chimpanzees at Mahale, Tanzania: implications for nutritional independence long before cessation of nipple contact. Am. J. Phys. Anthropol. 163, 356–366. doi: 10.1002/ajpa.23212
Mercader, J., Runge, F., Vrydaghs, L., Doutrelepont, H., Ewango, C. E. N., and Juan-Tresseras, J. (2000). Phytoliths from archaeological sites in the tropical forest of Ituri, Democratic Republic of Congo. Quat. Res. 54, 102–112. doi: 10.1006/qres.2000.2150
Merceron, G., Ramdarshan, A., Blondel, C., Boisserie, J.-R., Brunetiere, N., Francisco, A., et al. (2016). Untangling the environmental from the dietary: dust does not matter. Proc. R. Soc. B Biol. Sci. 283:20161032. doi: 10.1098/rspb.2016.1032
Morgan, D., and Sanz, C. (2006). “Chimpanzee feeding ecology and comparisons with sympatric gorillas in the Goualougo Triangle, Republic of Congo,” in Feeding Ecology in Apes and Other Primates, eds H. Gottfried, R. M. Martha, and B. Christophe (Cambridge: Cambridge University Press, 97–122.
Morin, P. A., Moore, J. J., Chakraborty, R., Jin, L., Goodall, J., and Woodruff, D. S. (1994). Kin selection, social structure, gene flow, and the evolution of chimpanzees. Science 265, 1193–1201. doi: 10.1126/science.7915048
Nystrom, P., Phillips-Conroy, J. E., and Jolly, C. J. (2004). Dental microwear in anubis and hybrid baboons (Papio hamadryas, sensu lato) living in Awash National Park, Ethiopia. Am. J. Phys. Anthropol. 125, 279–291. doi: 10.1002/ajpa.10274
Oates, J. F., Dunn, A., Greengrass, E., and Morgan, B. J. (2008). Pan troglodytes ssp. ellioti. The IUCN Red List of Threatened Species. Version 2015.2.2015.
Percher, A. M., Merceron, G., Nsi Akoue, G., Galbany, J., Romero, A., and Charpentier, M. J. E. (2018). Dental microwear textural analysis as an analytical tool to depict individual traits and reconstruct the diet of a primate. Am. J. Phys. Anthropol. 165, 123–138. doi: 10.1002/ajpa.23337
Pérez, B., Romero, A., and Pérez-Pérez, A. (2011). Age-related variability in buccal dental-microwear in Middle and Upper Pleistocene human populations. Anthropol. Rev. 74, 25–37. doi: 10.2478/v10044-010-0005-0
Piperno, D. R. (2006). Phytoliths: A Comprehensive Guide for Archaeologists and Paleoecologists. Oxford: Rowman Altamira.
Poorter, L. (2004). Biodiversity of West African Forests: An Ecological Atlas of Woody Plant Species. Oxon; Cambridge: CABI.
Potts, K. B., Chapman, C. A., and Lwanga, J. S. (2009). Floristic heterogeneity between forested sites in Kibale National Park, Uganda: insights into the fine-scale determinants of density in a large-bodied frugivorous primate. J. Anim. Ecol. 78, 1269–1277. doi: 10.1111/j.1365-2656.2009.01578.x
Power, R. C., Salazar-García, D. C., Wittig, R. M., Freiberg, M., and Henry, A. G. (2015). Dental calculus evidence of Taï Forest Chimpanzee plant consumption and life history transitions. Sci. Rep. 5:15161. doi: 10.1038/srep15161
Pruetz, J. D. (2006). “Feeding ecology of savanna chimpanzees (Pan troglodytes verus) at Fongoli, Senegal,” in Feeding Ecology in Apes and Other Primates, eds H. Gottfried, R. M. Martha, and B. Christophe (Cambridge: Cambridge University Press, 161–182.
Pruetz, J. D., and Bertolani, P. (2007). Savanna chimpanzees, Pan troglodytes verus, hunt with tools. Curr. Biol. 17, 412–417. doi: 10.1016/j.cub.2006.12.042
R Development Core Team (2017). A Language and Environment for Statistical Computing. R Foundation for Statistical Computing. Available online at: https://www.r-project.org/
Ramdarshan, A., Blondel, C., Brunetière, N., Francisco, A., Gautier, D., Surault, J., et al. (2016). Seeds, browse, and tooth wear: a sheep perspective. Ecol. Evol. 6, 5559–5569. doi: 10.1002/ece3.2241
Romero, A., Galbany, J., De Juan, J., and Pérez-Pérez, A. (2012). Brief communication: Short- and long-term in vivo human buccal-dental microwear turnover. Am. J. Phys. Anthropol. 148, 467–472. doi: 10.1002/ajpa.22054
Schulz, E., Calandra, I., and Kaiser, T. M. (2010). Applying tribology to teeth of hoofed mammals. Scanning 32, 162–182. doi: 10.1002/sca.20181
Schulz, E., Calandra, I., and Kaiser, T. M. (2013a). Feeding ecology and chewing mechanics in hoofed mammals: 3D tribology of enamel wear. Wear 300, 169–179. doi: 10.1016/j.wear.2013.01.115
Schulz, E., Piotrowski, V., Clauss, M., Mau, M., Merceron, G., and Kaiser, T. M. (2013b). Dietary abrasiveness is associated with variability of microwear and dental surface texture in rabbits. PLoS ONE 8:e56167. doi: 10.1371/journal.pone.0056167
Schulz-Kornas, E., Stuhlträger, J., Clauss, M., Wittig, R. M., and Kupczik, K. (2019). Dust affects chewing efficiency and tooth wear in forest dwelling western chimpanzees (Pan troglodytes verus). Am. J. Phys. Anthropol. 169, 66–77. doi: 10.1002/ajpa.23808
Scott, J. R., Godfrey, L. R., Jungers, W. L., Scott, R. S., Simons, E. L., Teaford, M. F., et al. (2009). Dental microwear texture analysis of two families of subfossil lemurs from Madagascar. J. Hum. Evol. 56, 405–416. doi: 10.1016/j.jhevol.2008.11.003
Scott, R. S., Teaford, M. F., and Ungar, P. S. (2012). Dental microwear texture and anthropoid diets. Am. J. Phys. Anthropol. 147, 551–579. doi: 10.1002/ajpa.22007
Scott, R. S., Ungar, P. S., Bergstrom, T. S., Brown, C. A., Childs, B. E., Teaford, M. F., et al. (2006). Dental microwear texture analysis: technical considerations. J. Hum. Evol. 51, 339–349. doi: 10.1016/j.jhevol.2006.04.006
Scott, R. S., Ungar, P. S., Bergstrom, T. S., Brown, C. A., Grine, F. E., Teaford, M. F., et al. (2005). Dental microwear texture analysis shows within-species diet variability in fossil hominins. Nature 436, 693–695. doi: 10.1038/nature03822
Smith, T. M., Machanda, Z., Bernard, A. B., Donovan, R. M., Papakyrikos, A. M., Muller, M. N., et al. (2013). First molar eruption, weaning, and life history in living wild chimpanzees. Proc. Natl. Acad. Sci. 110, 2787–2791. doi: 10.1073/pnas.1218746110
Solounias, N., and Semprebon, G. (2002). Advances in the reconstruction of ungulate ecomorphology with application to early fossil equids. Am. Museum Novit. 2002, 1–49. doi: 10.1206/0003-0082(2002)366<0001:AITROU>2.0.CO;2
Stoorvogel, J. J., Van Breemen, N., and Jassen, B. H. (1997). The nutrient input by Harmattan dust to a forest ecosystem in Cote d'Ivoire, Africa. Biogeochemistry 37, 145–157. doi: 10.1023/A:1005734225727
Strait, S. G. (1993). Molar microwear in extant small-bodied faunivorous mammals: an analysis of feature density and pit frequency. Am. J. Phys. Anthropol. 92, 63–79. doi: 10.1002/ajpa.1330920106
Swindler, D. R. (2002). Primate Dentition: An Introduction to the Teeth of Non-Human Primates. Cambridge: Cambridge University Press.
Teaford, M. F., and Oyen, O. J. (1989). In vivo and in vitro turnover in dental microwear. Am. J. Phys. Anthropol. 80, 447–460. doi: 10.1002/ajpa.1330800405
Teaford, M. F., and Robinson, J. G. (1989). Seasonal or ecological differences in diet and molar microwear in Cebus nigrivittatus. Am. J. Phys. Anthropol. 80, 391–401. doi: 10.1002/ajpa.1330800312
Tutin, C. E. G., and Fernandez, M. (1993). Composition of the diet of chimpanzees and comparisons with that of sympatric lowland gorillas in the Lopé Reserve, Gabon. Am. J. Primatol. 30, 195–211. doi: 10.1002/ajp.1350300305
Tutin, C. E. G., Fernandez, M., Rogers, M. E., Williamson, E. A., and McGrew, W. C. (1991). Foraging profiles of sympatric lowland gorillas and chimpanzees in the Lope Reserve, Gabon. Philos. Trans. R. Soc. B Biol. Sci. 334, 179–186. doi: 10.1098/rstb.1991.0107
Tutin, C. E. G., Ham, R. M., White, L. J. T., and Harrison, M. J. S. (1997). The primate community of the Lopé Reserve, Gabon: diets, responses to fruit scarcity, and effects on biomass. Am. J. Primatol. 42, 1–24. doi: 10.1002/(SICI)1098-2345(1997)42:1<1::AID-AJP1>3.0.CO;2-0
Uehara, S. (1990). “A preliminary report on age differences in plant-feeding behaviors among adult and adolescent males,” in The Chimpanzees of the Mahale Mountains, ed N. Toshisada (Tokyo: University of Tokyo Press, 223–236.
Ungar, P. S., Brown, C. A., Bergstrom, T. S., and Walker, A. (2003). Quantification of dental microwear by tandem scanning confocal microscopy and scale-sensitive fractal analyses. Scanning 25, 185–193. doi: 10.1002/sca.4950250405
Ungar, P. S., Merceron, G., and Scott, R. S. (2007). Dental microwear texture analysis of Varswater bovids and early Pliocene paleoenvironments of Langebaanweg, Western Cape Province, South Africa. J. Mamm. Evol. 14, 163–181. doi: 10.1007/s10914-007-9050-x
van Casteren, A., Lucas, P. W., Strait, D. S., Michael, S., Bierwisch, N., Schwarzer, N., et al. (2018). Evidence that metallic proxies are unsuitable for assessing the mechanics of microwear formation and a new theory of the meaning of microwear. R. Soc. Open Sci. 5:171699. doi: 10.1098/rsos.171699
Walker, A., Hoeck, H. N., and Perez, L. (1978). Microwear of mammalian teeth as an indicator of diet. Science 201, 908–910. doi: 10.1126/science.684415
Wilson, D. E., and Reeder, D. M. (2005). Mammal Species of the World: A Taxonomic and Geographic Reference. 3rd Edn. Baltimore: JHU Press.
Wittig, R. M. (2018). “Taï chimpanzees,” in Encyclopedia of Animal Cognition and Behavior, eds V. Jennifer and S. Todd (Cham: Springer International Publishing), 1–7. doi: 10.1007/978-3-319-47829-6_1564-1
Keywords: surface texture, Taï, ontogeny, phytolith, hard object, wear facet
Citation: Stuhlträger J, Schulz-Kornas E, Wittig RM and Kupczik K (2019) Ontogenetic Dietary Shifts and Microscopic Tooth Wear in Western Chimpanzees. Front. Ecol. Evol. 7:298. doi: 10.3389/fevo.2019.00298
Received: 22 March 2019; Accepted: 24 July 2019;
Published: 08 August 2019.
Edited by:
Renoult P. Julien, UMR5175 Centre d'Ecologie Fonctionnelle et Evolutive (CEFE), FranceReviewed by:
Gildas Merceron, UMR7262 Institut de Paléoprimatologie, Paléontologie Humaine Évolution et Paléoenvironnements (IPHEP), FranceAlejandro Romero, University of Alicante, Spain
Copyright © 2019 Stuhlträger, Schulz-Kornas, Wittig and Kupczik. This is an open-access article distributed under the terms of the Creative Commons Attribution License (CC BY). The use, distribution or reproduction in other forums is permitted, provided the original author(s) and the copyright owner(s) are credited and that the original publication in this journal is cited, in accordance with accepted academic practice. No use, distribution or reproduction is permitted which does not comply with these terms.
*Correspondence: Julia Stuhlträger, anVsaWFfc3R1aGx0cmFlZ2VyQGV2YS5tcGcuZGU=