- 1Earth Sciences Department Università di Firenze, Florence, Italy
- 2Laboratory of Evolutionary Biology, Department of Anatomy, College of Medicine, Howard University, Washington, DC, United States
- 3Laboratório de Mastozoologia, Universidade Federal Do Estado Do Rio de Janeiro, Rio de Janeiro, Brazil
- 4Dottorato di Ricerca in Scienze Della Terra, Università di Pisa, Pisa, Italy
- 5Department of Human Evolutionary Biology, Harvard University, Boston, MA, United States
- 6Department of Paleobiology, National Museum of Natural History, Smithsonian Institution, Washington, DC, United States
- 7Museum of Paleontology, The University of Michigan, Ann Arbor, MI, United States
- 8Department of Biology, California State University, San Bernardino, CA, United States
- 9Natural History Museum of Los Angeles County, Los Angeles, CA, United States
Biochronology is important to vertebrate chronology because the primary temporal units developed and applied by vertebrate paleontologists for correlation of terrestrial deposits (Land Mammal Ages, LMA) are all biochronologic units. Specific mammal biochronologic scales have been developed for Europe (MN units or ELMA), Asia (ALMA), North America (NALMA), and South America (SALMA). Each timescale is based on land mammal first appearances and characteristic associations on different continental landmasses. Herein, we summarize and review the bases for recognizing mammalian biochronologic units with the most recent update of the Land Mammal Ages. We correlate these ages with the global magnetochronostratigraphic and geochronologic time scales including the major equid evolutionary events of the last 8 million years.
Introduction
Vertebrate paleontologists have a long tradition of correlating vertebrate occurrences and evolutionary events into stratigraphic and biochronologic contexts. Both have been utilized for the last 50 years together with an increased usage of radioisotopic and magnetostratigraphic correlations. However, the term biochronology was rarely used prior to 1970's when the application of radiometric dating became widespread and the distinction was made between radiochronology and biochronology as different aspects of geochronology (re: Berggren and Van Couvering, 1974; Gradstein et al., 2004)
According to the original definition (Williams, 1901) a biochron is “a time unit whose measure is the endurance of an organic character.” Based on this definition, Berggren and Van Couvering (1974) suggested the application of the term biochron for units of geologic time that are based on paleontologic data without reference to lithostratigraphy or rock units. Thus, the emphasis of mammal occurrences in time rather than in rocks distinguishes biochronology from other chronologic systems (Lindsay and Tedford, 1990). The need for a heuristic method for temporal ordering of the fossil vertebrate record without reference to rock units (biostratigraphy) is crucial for the vertebrate record due to the discontinuous nature of terrestrial stratigraphic sequences (e.g., the case of fissure fillings in karstic systems).
Biochronology is an important concept for geochronology, but it has been compromised by a history of loose definitions and diverse usages. In addition, biochronology has never been discussed in any stratigraphic code because of that “loose and ambiguous” application. Furthermore, biochronology is important to vertebrate chronology because the primary temporal units developed and applied by vertebrate paleontologists for correlation in terrestrial deposits are all biochronologic units.
Specific mammal biochronologic scales (LMA = Land Mammal Ages) have been developed for Europe (ELMA), Asia (ALMA), North America (NALMA) and South America (SALMA) based on the succession of evolutionary stage of faunal assemblages and dispersal events (first occurrence of evolving lineages; Mein, 1975, 1979; Lindsay, 1990, 2003; Lindsay and Tedford, 1990; Berggren et al., 1995; Walsh, 1998). These timescales are variously expressed in terms of conventional mammal biochronologic units (sometime termed “zones”) or land mammal ages, defined by Lindsay (2003: p. 222) as “relatively short interval[s] of geological time that can be recognized and distinguished from earlier and later such units (in a given region or province) by a characterizing assemblage of mammals.” Each timescale is based on land mammals in different continental landmasses and has its own history of development reflecting the uniqueness of the records and the extent to which faunal succession has been resolved. When stratigraphic fortune occurs, these biochronologic units can be tied to biostratigraphic sequences such as the Potwar Plateau, Pakistan (Barry et al., 1982, 2013).
Berggren and Van Couvering developed the concept of a FAD, First Appearance Datum, to identify mammalian lineages that made “a geochronologically instantaneous prochoresis” across intercontinental expanses. Examples of FADs were the “Hipparion” Datum, Proboscidean Datum and later, as exemplified by Lindsay et al. (1980) the Equus Datum. Mein (1975, 1979) defined European MN units based in part on migratory events (FADs) and characteristic associations of faunas that he aggregated into MN “zones” (=units).
The “Datum” concept has been challenged by the dearth of independent ages associated with demonstrably primitive species of invading lineages. Bernor (1983, 1984) recognized that Eurasia and Africa were highly provincial throughout the Neogene and proposed provincial mammal biochronologies that led to age realignment for many Old World localities. Steininger et al. (1996) imposed a set of rules that tied MN unit chronology to independently calibrated faunas whenever possible. Resolution of the taxonomic content of a genuinely primitive species of “Hipparion” with dated rocks led to the refinement of the “Hipparion” Datum demonstrating that the first occurring “Hipparion” in Europe was a species of North American Cormohipparion circa 11.2 Ma (Woodburne, 2007, 2009; Bernor et al., 2017). The “Hipparion” Datum, as such was renamed the Cormohipparion Datum by Bernor et al. (2017) in recognition of the ancestral North American lineage reported from Pakistan, Turkey and North Africa at 10.8 Ma. The original Berggren and Van Couvering (1974) mammalian lineage “Datum Event” has given way to a realization that what FADs are actually accomplishing is demonstrating local earliest occurrences of immigrating taxa. In the case of Old World “Hipparion”, first occurrence discrepancy has narrowed: 11.2 in Central Europe and Spain, 10.8 Ma in Pakistan, Turkey and North Africa (Bernor et al., 2017). Diachroneity in local FADs can be due to physical barriers and/or ecological filters, or to the lack of appropriate sedimentary rocks. Proposed mammalian FADs represent testable hypotheses about invading lineages and their extension over great geographic extent during a short interval of time.
We summarize and review herein a comparative biochronologic framework that provides the most recent update of the Land Mammal Ages characterized, defined and calibrated across different continents (either in Old or New World). We correlate these with magnetochronostratigraphic and geochronologic time scales (Figure 1) and summarize the major equid evolutionary events for the last 8 million years. This contribution is intended to compliment contributions to the Frontiers in Ecology and Evolution work on “Examining Evolutionary Trends in Equus and its Close Relatives from Five Continents,” and has been written with the aim of offering an overview of the chronology of Equus and its close relatives across the geographic/chronologic range where they occur: North and South America, Eurasia and Africa. It is not intended to integrate molecular and DNA interpretations of divergence times in Equus; it addresses only the paleontological data reviewed and revealed in this Frontiers collection of works.
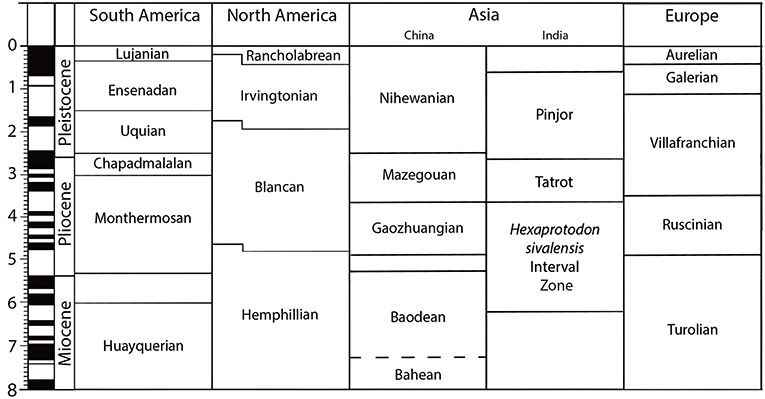
Figure 1. Land Mammal Ages in South America, North America, Asia (China and India) and Europe from 8 Ma with magnetochronostratigraphic and geochronologic time scales.
A Timescale for Horse Evolution in North America
The Hemphillian LMA ranges from approximately 8 Ma up to about 4 Ma, with four faunal stages (Hh1-Hh4), and therefore is late Miocene to early Pliocene in age (Figure 1). It is marked by a continuous loss of autochthonous taxa and by a rising immigration rate including from South America and diverse small mammals and carnivores and some ungulates from Eurasia including the hipparionine Plesiohipparion from China (Hulbert and Harington, 1999). There is a 7–6 Ma turnover event (Hh2/Hh3) with the extinction of Protohippus, Calippus, and “Hipparion” as well as species level turnover in most surviving genera. Equid species richness at many North American late Clarendonian or early Hemphillian sites often ranged between 8 and 11; after this event it was never >6 and most often 3 or 4; monodactyl equids (mostly Dinohippus and Astrohippus) became abundant at this time (Hulbert, personal communication). Hh4 extends across the Mio-Pliocene boundary (5.33 Ma) calibrated as being 5.3–4.9 to 4.6 Ma (Bell et al., 2004; Tedford et al., 2004). Equids recorded from this interval include Dinohippus mexicanus, Neohipparion cf. eurystyle and Astrohippus stocki and with more limited distributions Cormohipparion emsliei, Nannippus aztecus, and Pseudhipparion simpsoni; these last taxa can be considered relictual from the late Miocene.
As cited above, the Hemphillian LMA ranges from the latest Miocene into the early Pliocene, 4.6 Ma (Hh4), but a range of 5.2–4.6 Ma for the earliest Blancan has been proposed (Repenning, 1988; Bell et al., 2004). The succeeding Blancan LMA has been defined by the first appearance In North America of arvicoline rodents, circa 4.8 Ma (Repenning, 2003). Repenning (1988) divided the Blancan into 5 intervals: Blancan I (4.9–4.62 Ma.), Blancan II (4.62–4.1 Ma.), Blancan III (4.1–3.0 Ma.), Blancan IV (3.0–2.5 Ma.), Blancan V (2.5–1.9 or 1.72 Ma.). Subdivision of the Blancan is based largely on small mammal faunas (Repenning, 1988). Bell et al. (2004) reviewed the data suggesting a 5-part subdivision of the Blancan and concluded that Blancan II and IV cannot be distinguished, but accepted Blancan I, III, and V. Blancan II is essentially a continuation (and further characterization) of Blancan I, while Blancan IV is a continuation and further characterization of Blancan III. Revised Blancan chronologic ranges are: Blancan I (~4.9–4.1 Ma), Blancan III (~4.1–2.5 Ma), and Blancan V (~2.5–1.9 or 1.72 Ma) (Bell, personal communication).
Dinohippus is known to occur in the Blancan I interval, while Equus (Plesippus) simplicidens, E. (P.) idahoensis, and E. cumminsii are reported from the Blancan III and later Blancan assemblages. The diminutive hipparionine horse Nannippus peninsulatus is reported from the Blancan V interval but does not survive into the Irvingtonian. The base of Blancan V corresponds closely to the initiation of the Quaternary, i.e., the beginning of the Pleistocene.
The Irvingtonian is divided into three units, Irvingtonian I (1.9–1.7 Ma), Irvingtonian II (0.85–0.4 Ma), and Irvingtonian III (0.4–0.195 Ma) (Bell et al., 2004). Early Irvingtonian Equus includes E. scotti, E. conversidens (sensu Scott, 2004), and Haringtonhippus francisci (possibly including E. calobatus). The Rancholabrean NALMA extends from 0.195 to about 0.011 Ma with the onset of the Holocene. Common Rancholabrean equid species include Equus scotti, E. conversidens, and Haringtonhippus francisci. Equus occidentalis is also abundant in the American southwest during this period. Fossils resembling Equus ferus have also been documented from Rancholabrean faunas.
The Blancan NALMA is defined by the first appearance in North America south of 55 degrees N Latitude of the arvicoline rodents Mimomys and Ophiomys (5.2–4.6 Ma) (Repenning, 1988; Bell et al., 2004). The Irvingtonian NALMA is defined by the first appearance of Mammuthus south of 55 degrees N Latitude. The Rancholabrean NALMA is defined by the first appearance of the bovid Bison in North America.
A Timescale for Horse Evolution in Asia
Pleistocene fossils of Equus are abundant in Asia and overlap those of hipparionine horses. The vast central Asian steppe provided an ideal habitat for these horses adapted to open landscapes. These rich fossil records enabled a long history of studies on the equids (Qiu et al., 1987; Deng and Xue, 1999; Bernor et al., 2018b), but chronological control for a precise timing and sequence of horse evolution, particularly that of Equus, has been neglected until recently (Deng and Sun, in press). The Late Neogene faunal record in East Asia is now based on a greatly improved chronological framework given intense interest in Pleistocene climate oscillations and in the multiple dispersions of hominids (cfr. Li et al., 2019; Zanolli et al., 2019).
East Asian paleoenvironments were intensely influenced by monsoons during the Ice Age and cyclical development of windblown sediments left a paleosol sequence in the Chinese Loess Plateau that archives a high-resolution chronologic record. These fine-grained deposits are ideally suited for paleomagnetic analysis, the dating of which can be further refined by astronomical tuning to the Milankovitch cycles. The resulting chronological framework helps to establish the sequence of several classical Equus-producing localities as well as recently discovered records. From old to young, the following early Pleistocene chronology for Equus represents the current state of knowledge.
Equus eisenmannae (Qiu et al., 2004) is a large primitive stenonine horse from the Longdan loessic section in Linxia Basin, Gansu Province. The Longdan area (35.5°N, 103.5°E) is located in the central part of the Linxia Basin. The locality has been correlated biochronologically with the early Nihewanian (Qiu et al., 2004; Wang and Deng, 2011). Wang and Deng (2011) reported 13 levels in the entire Longdan section and the base of this section is considered about 3.5 Ma (Qiu et al., 2004). The fossils referred to Equus eisenmannae occur in the 5th, 9th, and 11th levels correlating to 2.55, 2.16, and 1.86 Ma, respectively (Wang and Deng, 2011). Wang and Deng combine the 9th and 11th levels into an “upper level” recognizing level 5 as the “lower level,” as was originally proposed by Qiu et al. (2004). Wang and Deng (2011) found no statistical differences among fossils from the upper and lower levels and referred them all to Equus eisenmannae. Equus eisenmannae is one of the largest Old World stenonine horses close in its size to first-occurring European Equus, E. livenzovensis (Bernor et al., 2018a).
Although represented by poorly preserved fossils, Equus sp. from Zanda Basin in southern Tibet (Wang et al., 2013) was correlated to 2.48 Ma, suggesting fast dispersion of Equus even in higher elevations, where modern Tibetan ass, E. kiang, currently lives. Equus sanmeniensis, another large species of Equus, is reported from the Shangshazui stone artifact site in the classical Nihewan Basin (1.7–1.66 Ma) and nearby Majuangou III (1.66 Ma) hominin tool site (Ao et al., 2013). This species was also recorded from the Homo erectus site at Gongwangling, Lantian Area, Shaanxi Province, and magnetically considered slightly younger ~1.54–1.65 Ma (Zhu et al., 2015). E. yunnanensis from the Homo erectus site at Niujianbao in Yuanmou Basin was magnetically correlated as slightly younger than chron C2n, ~1.7 Ma (Zhu et al., 2008).
In South Asia, equids are represented by hipparionines in the Miocene and Pliocene. The diverse Sivalhippus lineage, common in the late Miocene, disappears from the record after 6.0 Ma (Wolf et al., 2013). Within the Hexaprotodon sivalensis Interval Zone in the Siwalik Group (6.2 Ma to 3.6 Ma; Flynn et al., 2013), equid remains are rare. Hipparionines represented by Plesiohipparion huangheense, and Eurygnathohippus sp. appear in the record between 3.6 and 2.6 Ma (Jukar et al., 2018, in press), in an interval of time (correlative to the Gauss chron) commonly called the Tatrot Faunal Zone (Figure 1), or sometimes the Elephas planifrons Interval Zone in the Indian Siwaliks (Nanda, 2002). The youngest indeterminate hipparionine records are correlated paleomagnetically to ~2.6–2.5 Ma, around the same time the first Equus occurs in South Asia (Opdyke et al., 1979; Patnaik, 2013). Equus makes its appearance just above the Gauss-Matuyama chron boundary, which coincides with the Plio-Pleistocene transition (Patnaik, 2013). In the Siwaliks, remains of Equus have been found in sediments ranging from 2.6 to 0.6 Ma, a faunal unit termed the Pinjor Faunal Zone (reviewed by Patnaik, 2013). The Pinjor Faunal Zone is named after the Pinjor Formation near the town of Chandigarh, Northern India (Kumaravel et al., 2005). Temporally equivalent sections are known from the Pabbi Hills and Mirpur areas in Pakistan (Hussain et al., 1992; Dennell et al., 2006). As noted in Bernor et al. (2019), two morphotypes of Equus have been recorded—a large taxon called Equus sivalensis, and a smaller taxon sometimes called Equus sivalensis minor for specimens from the Upper Pinjor Formation near the town of Mirzapur (Gaur and Chopra, 1984), Equus cf. sivalensis from the Pabbi Hills (Dennell et al., 2006), and Equus sp. A (small) for specimens from the Mangla-Samwal anticline (Hussain et al., 1992). Equus sivalensis has been recorded from the entire temporal range of the Pinjor Faunal Zone (Patnaik, 2013), however, the temporal range of the smaller horse appears to be restricted to ~2.2–1.2 Ma (Hussain et al., 1992; Dennell et al., 2006). In the late Pleistocene, Equus namadicus and Equus hemionus are known from the Indian peninsula (Chauhan, 2008).
A Timescale for Horse Evolution in Europe
Equus is not recorded in the Miocene—Pliocene fossil record of Europe: Vallesian, Turolian, Ruscinian, and early Villafranchian in the European Land Mammal Age scheme (Figure 1). European Land Mammal Ages are also referable to the MN (= Mammal Neogene) “zone” or unit, a system used to correlate European mammal-bearing fossil localities: it consists of 16 consecutive zones (numbered MN 1 through MN 17; MN 7 and 8 have been joined into MN 7/8) defined through reference faunas, well-known sites that other localities can be correlated with (Mein, 1975, 1990; Bruijn et al., 1992). These times are characterized by different hipparionine horse evolutionary lineages (Bernor et al., 1996, 2010, 2017). The European Plio-Pleistocene interval is mostly included in the Villafranchian Land Mammal Age (Rook and Martínez-Navarro, 2010). The early Villafranchian correlates with the Late Pliocene, ca. 3.5 to 2.6 Ma, the middle Villafranchian ca. 2.6 to 2.0 Ma (most of the “former” Late Pliocene), and the late Villafranchian is correlative with the remaining Early Pleistocene (Matuyama from Olduvai to Jaramillo subchrons), a time span of ca. 2.0 to 1.0 (that includes the latest part of the former Late Pliocene to most of the former Early Pleistocene). The first appearance of Equus in Europe corresponds to the base of the Middle Villafranchian, ca. 2.6 Ma.
Montopoli was identified by Azzaroli (1970, 1977) as representing an early Middle Villafranchian locality and Lindsay et al. (1980) recognized the Montopoli Equus as representing the local Equus Datum, ca. 2.6 Ma. Bernor et al. (2018a) identified the large horse at Montopoli as being referable to Equus cf. livenzovensis. Earlier, Rook et al. (2017) identified a small hipparion co-occurring at Montopoli as one of the last surviving members of these tridactyl horses. The arrival of Equus in Europe (the so called “elephant-Equus event”) has been classically indicated by Azzaroli (1983) as “a turning point in the history of Eurasia.” Equus livenzovensis appears to be at the base of the radiation of the later lineage of fossils horses, the European Pleistocene Equus stenonis group (= stenonine horses). The European stenonine horses have been revised by Alberdi and Palombo (2013). In addition to Equus livenzovensis, the species (and their chronological ranges) included in this group are Equus stenonis (end of middle Villafranchian to early late Villafranchian; Early Pleistocene, 2.4–1.7 Ma), Equus stehlini (late Villafranchian; Early Pleistocene, 1.8–1.6 Ma), Equus altidens, and Equus suessenbornensis (end of late Villafranchian to early Galerian, Early Pleistocene to early Middle Pleistocene, 1.6–0.6 Ma). Most of these taxa are discussed at greater length by Bernor et al. (2019).
A Timescale for Horse Evolution in Africa
The Miocene to Pleistocene mammal record of Africa is overall less complete than the fossil record on other continents and there is no established land mammal age scheme for Africa at a continental scale (Werdelin, 2010). The completeness of the mammal fossil record across the continent is extremely variable with regions in which the Neogene record is totally missing and others (such as Kenya or Ethiopia) with a relatively densely documented record. Without an established continental-scale biochronology, Africa's “biochronology” is based on the stratigraphic ordering of mammal-bearing localities in different sedimentary basins and is largely dependent on radiochronology with limited use of magnetostratigraphy (Figure 2). Pickford (1981) divided Miocene faunas from Kenyan sites into Faunal Sets I to VII. Pickford (1981) suggested age spans for his late Miocene sets to be 12.0–10.5 Ma (V), 10.5–7.5 (VI), and 7.5–5.5 (VII)
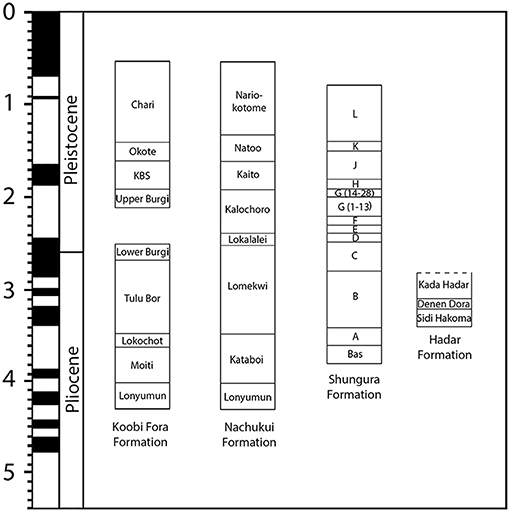
Figure 2. Correlation of the Koobi Fora, Nachukui, Shungura and Hadar Formation with magnetochronostratigraphic and geochronologic time scales. Modified from Werdelin (2010).
The Late Miocene-Early Pliocene boundary is poorly represented in Africa. Hipparionine horses are first found in North and East Africa circa 10.5 Ma (Bernor and White, 2009) the richest sites being the Algerian locality of Bou Hanifia and the Ethiopian locality of Chorora (Bernor et al., 2010). At the end of the Late Miocene, diversification of the hipparionine genus Eurygnathohippus (exhibiting evolutionary relationships to Siwalik hipparionines; Jukar et al., in press) was well under way, as was significant evolution among endemic elephants and marked successes by new bovid tribes and suines arriving from Eurasia.
Again, also for the Plio-Pleistocene of Africa there are no established land mammal ages. This time period has been rigorously studied biochronologically by temporal distributions of elephants (particularly subspecies or stages of Elephas recki) and suids, often in conjunction with the dating of hominin finds (re: Sanders et al., 2010). Equus first occurs in Africa during the early Pleistocene, in lower Member G of the Omo Shungura Formation (2.33 Ma) (Bernor et al., 2010). This age in Africa is delayed relative to Eurasia, where it is 2.6 Ma. First occurring African Equus is apparently related to European E. stenonis and Chinese E. eisenmannae (a detailed morphological comparison is necessary to resolve their relationships; Bernor et al., 2019). Eisenman (1983) named a new species of Equus from Koobi Fora, E. koobiforensis (circa 1.9 Ma) and recognized a smaller horse also from Koobi Fora, E. cf. tabeti. Bernor et al. (2019) distinguished Equus oldowayensis from Member 1, Olorgesailie (0.99 Ma) from E. koobiforensis and E. cf. tabeti. Azzaroli (1982, 2003) suggested that E. koobiforensis was referable to E. grevyi and recent study by Bernor at the Kenya National Museum, Nairobi suggests that E. koobiforensis is morphologically similar to E. grevyi. Equus oldowayensis from Olorgesailie however is derived in its robustly built premaxilla and symphysial region and great cheek tooth crown height (Bernor and Potts, personal observation). Representatives of the genus Equus dominate Eurygnathohippus by a ratio of 2:1 in the Ethiopian locality of Daka (Gilbert and Bernor, 2008) as well as Member 1 of Olorgesailie (Bernor and Potts, personal observation) with Eurygnathohippus sharply declining in its numbers in East and South Africa after 1 Ma. Our current understanding of extant African zebra and ass morphology, combined with our investigations of East African Pleistocene Equus evolution, thus far does not permit us to identify genuine representatives of Equus quagga, Equus grevyi, Equus zebra or Equus africanus in the African Pleistocene record.
A Timescale for Horse Evolution in South America
Two lineages of Equidae occurred in South America during the Pleistocene, Hippidion and Equus. Although there is no fossil record of Hippidion in Central or North America, most evidence suggests that both taxa originated and diversified in North or Central America and then migrated independently to South America during the important biogeographical events known as the Great American Biotic Interchange (GABI, ca. 3.0 Ma; Woodburne, 2010; MacFadden, 2013; Avilla et al., 2015). However, there is no record for Equidae in South America until the beginning of Pleistocene, well after the Pliocene formation of the Isthmus of Panama (around 3 Ma; Woodburne, 2010; MacFadden, 2013). The first known occurrence of Equidae in South America is Hippidion principale from Early Pleistocene deposits (Uquian) of Argentina (Woodburne, 2010; Avilla et al., 2015). However, the age of the first record of Equus in South America is controversial. Traditionally, its earliest record is considered middle Pleistocene (Ensenadan SALMA) for Tarija outcrops in Southern Bolivia, with a biostratigraphic sequence at Tolomosa Formation independently calibrated to between ~0.99 to <0.76 Ma (MacFadden, 2013). Nevertheless, there is no consensus about the age of those deposits and some researchers consider the deposition in Tarija to have occurred only during the Late Pleistocene (Coltorti et al., 2007 and references there in). Recently, it was proposed that only one species of Equus lived in South America during the Pleistocene, E. neogeus (Machado et al., 2017). This species is considered an index-fossil for deposits of Lujanian SALMA (late Pleistocene-earliest Holocene; 0.8 to 0.011 Ma; Figure 1). Although E. neogeus was widely distributed in South America, only a few localities are calibrated by independent chronostratigraphic data, indicating a Lujanian SALMA (Prado and Alberdi, 2017). Therefore, the dispersal of Equus into South America occurred during the GABI (from 3.0 Ma on), but if the Equus earliest record is truly Late Pleistocene, then it falls in the fourth and latest phase of the GABI (SALMA Lujanian, from 0.8 to 0.011 Ma) or possibly Equus migrated to South America during GABI 3 (SALMAs Ensenadan and Bonaerian, from 1.2 to 0.8 Ma), considering its early record in the middle Pleistocene (between ~0.99 and <0.76 Ma; MacFadden, 2013). All equids that occurred in South America during the Pleistocene (Hippidion and Equus) became extinct in the early Holocene (10,710 ± 100 cal ka BP, from Southern Chile; Avilla et al., 2015; Villavicencio et al., 2016; Machado et al., 2017).
Conclusion
Unlike high-resolution biostratigraphic and geochronologic tools available in the marine realm, mammalian biochronology is not permissive of recognizing strictly synchronous events at global scale. The Equus “Datum” event was heralded by Lindsay et al. (1980) as being a synchronous dispersion of Equus from North America to Eurasia and Africa at 2.5 (now 2.6) Ma. We have shown that this extension can be recognized at the genus, not species level, in Europe and rather closely in East and South Asia but was apparently later in Africa (Figure 3). The “Equus Datum” really reflects a first regional-intercontinental scale event with an approximate chronology: 2.6 Ma in Europe (Alberdi and Palombo, 2013) and 2.55 Ma in the Linxia Basin, China (Qiu et al., 2004; Wang and Deng, 2011) and 2.33 Ma in Ethiopia, East Africa (Bernor et al., 2010 and herein). Nevertheless, our review of the currently available evidence of the Land Mammal Ages, defined and calibrated across different continents (both Old and New World), allows us to recognize major faunal change. These changes in some cases correspond the limit between successive Land Mammal Ages, and can be dated by combining biochronologic, radioisotopic and magnetostratigraphic data.
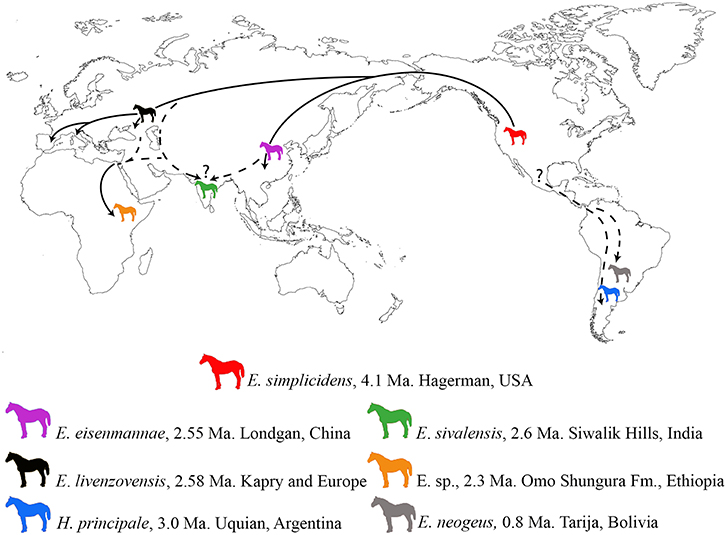
Figure 3. Biogeographic extension of early Equus species across the five continents, with timing of early record of each species.
Author Contributions
LR and RB conceived the paper. LA provided data on South America. RB and ES provided data on North America. AJ, LF, and XW provided data on Asia. LR and OC provided data on Europe. WS, RB, and LR provided data on Africa. LR wrote the paper with input from all authors. All authors contributed to the final version of the manuscript.
Conflict of Interest Statement
The authors declare that the research was conducted in the absence of any commercial or financial relationships that could be construed as a potential conflict of interest.
Acknowledgments
RB's research has been supported by a Visiting Professor fellowship at the Earth Sciences Department of the University of Florence (May-June 2017 and 2018), NSF grants EAR0125009, 1113175, 1138908 and 1558586 for the study of fossil horses and NSF:DBI:ABI Innovation 1759882 FuTRES. This manuscript benefitted from reviews by Chris Bell, Richard Hulbert, and Michael Woodburne. We thank them for their insightful comments.
References
Alberdi, M. T., and Palombo, M. R. (2013). The late Early to early Middle Pleistocene stenonoid horses from Italy. Quarter. Int. 288, 25–44. doi: 10.1016/j.quaint.2011.12.005
Ao, H., Dekkers, M. J., Wei, Q., Qiang, X., and Xiao, G. (2013). New evidence for early presence of hominids in North China. Sci. Reports 3:2403. doi: 10.1038/srep02403
Avilla, L. S., Bernardes, C., and Mothé, D. (2015). A new genus for Onohippidium galushai macfadden and skinner, 1979 (mammalia, equidae), from the late hemphillian of North America. J. Vertebr. Paleontol. 35:e925909. doi: 10.1080/02724634.2014.925909
Azzaroli, A. (1970). Villafranchian correlations based on large mammals. Giornal. Geol. 35, 111–131.
Azzaroli, A. (1977). The Villafranchian stage in Italy and the Plio-Pleistocene boundary. Giornal. Geol. 41, 61–79.
Azzaroli, A. (1982). On villafranchian palaearctic equids and their allies. Palaeontogr. Ital. 72, 74–97.
Azzaroli, A. (1983). Quaternary mammals and the “end-Villafranchian” dispersal event. A turning point in the history of Eurasia. Palaeogeogr. Palaeoclimatol. Palaeoecol. 44, 117–139. doi: 10.1016/0031-0182(83)90008-1
Barry, J. C., Behrensmeyer, A. K., Badgley, C. E., Flynn, L. J., Peltonen, H., Cheema, I. U., et al. (2013). “The neogene siwaliks of the potwar plateau, Pakistan,” in Fossil Mammals of Asia, eds X. Wang, L. J. Flynn, and M. Fortelius (New York, NY: Columbia University Press, 373–399.
Barry, J. C., Lindsay, E. H., and Jacobs, L. L. (1982). A biostratigraphic zonation of the middle and upper siwaliks of the potwar plateau of Northern Pakistan. Palaeogeogr. Palaeoclimatol. Palaeoecol. 37, 95–130. doi: 10.1016/0031-0182(82)90059-1
Bell, C. J., Lundelius, E. L. Jr., Barnosky, A. D., Graham, R. W., Lindsay, E. H., Ruez, D. R. Jr., et al. (2004). “The Blancan, Irvingtonian, and Rancholabrean Mammal Ages,” in Late Cretaceous and Cenozoic Mammals of North America: Biostratigraphy and Geochronology, ed M. O. Woodburne (New York, NY: Columbia University Press), 232–314. doi: 10.7312/wood13040-009
Berggren, W. A., Kent, D. V., Swisher, C. C., and Aubry, M.-P. (1995). “A revised Cenozoic geochronology and chronostratigraphy,” in Geochronology, Time Scales and Stratigraphic Correlation, eds W. A. Berggren, D. V. Kent, M. P. Aubry, and J. Hardenbol (Tulsa, OK: SEPM special publication), 54, 129–212.
Berggren, W. A., and Van Couvering, J. A. (1974). The late Neogene: Biostratigraphy, geochronology and paleoclimatology of the last 15 million years in marine and continental sequences. Palaeogeogr. Palaeoclimatol. Palaeoecol. 16, 1–216.
Bernor, R. L. (1983). “Geochronology and Zoogeographic Relationships of Miocene Hominoidea,” in New Interpretations of Ape and Human Ancestry, eds R. L. Ciochon and R. Corruccini (New York, NY: Plenum Press, 21–64.
Bernor, R. L. (1984). Systematic and evolutionary relationships of the hipparionine horses from Maragheh, Iran, Late Miocene, Turolian age. Palaeovertebrata 15, 173–269.
Bernor, R. L., Armour-Chelu, M., Gilbert, H., Kaiser, T. M., and Schulz, E. (2010). “Equidae,” in Cenozoic Mammals of Africa, eds L. Werdelin and W. J. Sanders (Berkeley, CA: University of California Press, 685–721.
Bernor, R. L., Cirilli, O., Jukar, A. M., Potts, R., Bukhsianidze, M., and Rook, L. (2019). Evolution of Early Equus in Italy, Georgia, the Indian Subcontinent, East Africa and the Origins of African Zebras. Front. Ecol. Evol. 7:166. doi: 10.3389/fevo.2019.00166
Bernor, R. L., Cirilli, O., Wang, S.-Q., and Rook, L. (2018a). Equus cf. livenzovensis from Montopoli, Italy (early Pleistocene; MN 16b; ca. 2.6 Ma). Boll. Soc. Paleont. Ital. 57, 203–216. doi: 10.4435/BSPI.2018.13
Bernor, R. L., Golich, U. B., Harzahauser, M., and Semprebon, G. M. (2017). The Pannonian C hipparions from the Vienna Basin. Palaeogeogr. Palaeoclimatol. Palaeoecol. 476, 28–41. doi: 10.1016/j.palaeo.2017.03.026
Bernor, R. L., Koufos, G. D., Woodbune, M. O., and Fortelius, M. (1996). “The evolutionary history and biochronology of European and southwest Asian late Miocene and Pliocene hipparionine horses,” in The Evolution of Western Eurasian Neogene Mammal Faunas, eds. R. L. Bernor, V. Falbusch and H. W. Mittmann (New York, NY: Columbia University Press, 307–338.
Bernor, R. L., Wang, S.-Q., Liu, Y., Chen, Y., and Sun, B. (2018b). A new hipparion genus Shanxihippus and Chinese hipparion horses with highly specialized nasal-snout regions. Riv. Ital. Paleont. Strat. 124, 361–386.
Bernor, R. L., and White, T. D. (2009). Systematics and biogeography of “Cormohipparion” africanum, early vallesian (MN 9, ca. 10.5 Ma) of Bou Hanifia, Algeria. In: Barry Albright L. III (ed), Papers on geology, vertebrate paleontology, and biostratigraphy in honour of Michael O. Woodburne. Museum Northern Arizona Bull. 65, 635–658.
Bruijn, H., de Daams, R., Daxner-Höck, G., Fahlbusch, V., Ginsburg, L., Mein, P., et al. (1992). Report of the RCMNS working group on fossil mammals, Reisensburg 1990. Newslett. Stratigr. 26, 65–118. doi: 10.1127/nos/26/1992/65
Chauhan, P. R. (2008). Large mammal fossil occurrences and associated archaeological evidence in Pleistocene contexts of peninsular India and Sri Lanka. Quarter. Int. 192, 20–42. doi: 10.1016/j.quaint.2007.06.034
Coltorti, M., Abbazzi, L., Ferretti, M. P., Iacumin, P., Paredes Rios, F., Pellegrini, M., et al. (2007). Last Glacial mammals in South America: a new scenario from the Tarija Basin (Bolivia). Naturwissenschaften 94, 288–299. doi: 10.1007/s00114-006-0196-9
Deng, T., and Xue, X. X. (1999). Chinese Fossil Horses of Equus and Their Environment. Beijing: China Ocean Press.
Dennell, R., Coard, R., and Turner, A. (2006). The biostratigraphy and magnetic polarity zonation of the Pabbi Hills, northern Pakistan: an upper siwalik (pinjor stage) upper pliocene–lower pleistocene fluvial sequence. Palaeogeogr. Palaeoclimatol. Palaeoecol. 234, 168–185. doi: 10.1016/j.palaeo.2005.10.008
Eisenman, V. (1983). “Family Equidae,” in Koobi Fora Research Project Volume 2, The fossil Ungulates: Proboscidea, Perissodactyla and Suidae, ed J. Harris (Oxford, UK: Clarendon Press, 156–214.
Flynn, L. J., Lindsay, E. H., Pilbeam, D., Raza, S. M., Morgan, M. E., Barry, J. C., et al. (2013). “The siwaliks and neogene evolutionary biology in South Asia,” in Fossil Mammals of Asia, eds X. Wang, L. J. Flynn, and M. Fortelius. (New York, NY: Columbia University Press, 353–372.
Gaur, R., and Chopra, S. R. K. (1984). On a new subspecies of Equus from Pinjor Formation of Upper Sivaliks - with remarks on Sivalik Equus. J. Palaeontol. Soc. India 29, 19–25.
Gilbert, H., and Bernor, R. L. (2008). “Equidae,” in Homo erectus – Pleistocene Evidence from the Middle Awash, Ethiopia, eds H. Gilbert and B. Asfaw (Berkeley, CA: University of California Press, 133–166.
Gradstein, F. M., Ogg, J. G., Smith, A. G., Agterberg, F. P., Bleeker, W., Cooper, R. A., et al. (2004). A Geologic Time Scale 2004. Cambridge: Cambridge University Press. doi: 10.4095/215638
Hulbert, R. C. Jr., and Harington, C. R. (1999). An early pliocene hipparionine horse from the Canadian Arctic. Palaeontology 42, 1017–1025. doi: 10.1111/1475-4983.00108
Hussain, S. T., van den Bergh, G., Steensma, K. J., de Visser, J. A., de Vos, J., Mohammad, A., et al. (1992). Biostratigraphy of the plio-pleistocene continental sediments (Upper Siwaliks) of the Mangla-Samwal anticline, Azad Kashmir, Pakistan. Proc. Koninkl. Nederl. Akadem. Wetenschapp. 95, 65–80.
Jukar, A. M., Sun, B., and Bernor, R. L. (2018). The first occurrence of Plesiohipparion huangheense (Qiu, Huang and Guo, 1987) (Equidae, Hipparionini) from the late Pliocene of India. Bolle. Soc. Paleont. Ital. 57, 125–132. doi: 10.4435/BSPI.2018.08
Jukar, A. M., Sun, B., Nanda, A. C., and Bernor, R. L. (in press). The first occurrence of Eurygnathohippus (Mammalia, Perissodactyla, Equidae) outside Africa its biogeographic significance. Boll. Soc. Paleont. Ital.
Kumaravel, V., Sangode, S. J., Kumar, R., and Siddaiah, N. S. (2005). Magnetic polarity stratigraphy of Plio-Pleistocene Pinjor Formation (type locality), Siwalik Group, NW Himalaya, India. Curr. Sci. 88, 1453–1461.
Li, F., Vanwezer, N., Boivin, N., Gao, X., Ott, F., Petraglia, M., et al. (2019). Heading north: late Pleistocene environments and human dispersals in central and eastern Asia. PLoS ONE 14:e0216433. doi: 10.1371/journal.pone.0216433
Lindsay, E. (2003). Chronostratigraphy, biochronology, datum events, land mammal ages, stage of evolution, and appearance event ordination. Bull. Am. Museum Nat. Hist. 279, 212–230. doi: 10.1206/0003-0090(2003)279<0212:C>2.0.CO;2
Lindsay, E. H. (1990). “The setting,” in European Neogene Mammalian Chronology, eds E. H. Lindsay, V. Fahlbush, and P. Mein (New York, NY: Plenum Press, 1–14.
Lindsay, E. H., Opdyke, N. D., and Johnson, N. M. (1980). Pliocene dispersal of the horse Equus and late Cenozoic mammal dispersal events. Nature 287, 135–138. doi: 10.1038/287135a0
Lindsay, H., and Tedford, R. H. (1990). “Development and application of land mammal ages in North America and Europa, and comparison,” in European Neogene Mammalian Chronology, eds E. H. Lindsay, V. Fahlbush, and P. Mein (New York, NY: Plenum Press, 601–624.
MacFadden, B. J. (2013). Dispersal of pleistocene Equus (family Equidae) into South America and calibration of GABI 3 based on evidence from Tarija, Bolivia. PLoS ONE 8:e59277. doi: 10.1371/journal.pone.0059277
Machado, H., Grillo, O., Scott, E., and Avilla, L. (2017). Following the footsteps of the South American Equus: are autopodia taxonomically informative? J. Mammal. Evol. 25, 397–405. doi: 10.1007/s10914-017-9389-6
Mein, P. (1975). “Resultats du groupe de travail des vertébrés,” in Report on Activity of the R.C.M.N.S. Regional Committee on Mediterranean Neogene Stratigraphy Working Groups, ed J. Senes (Bratislava), 78–81.
Mein, P. (1979). Rapport d'activité du groupe de travail vertébrés mise à jour de la biostratigraphic du Néogène basée sur les mammifères. Ann. Géol. Pays Hellén. 3, 1367–1372.
Mein, P. (1990). “Updating the MN zones, “in European Neogene Mammalian Chronology (NATO ASI Series a Life Sciences, 180), eds H. Lindsay, W. Fahlbusch, and P. Mein (New York, NY: Plenum Press, 73–90.
Nanda, A. C. (2002). Upper Siwalik mammalian faunas of India and associated events. J. Asian Earth Sci. 21, 47–58. doi: 10.1016/S1367-9120(02)00013-5
Opdyke, N. D., Lindsay, E., Johnson, G. D., Johnson, N., Tahirkheli, R. A. K., and Mirza, M. A. (1979). Magnetic polarity stratigraphy and vertebrate paleontology of the upper siwalik subgroup of northern Pakistan. Palaeogeogr. Palaeoclimatol. Palaeoecol. 27, 1–34. doi: 10.1016/0031-0182(79)90091-9
Patnaik, R. (2013). “Indian Neogene Siwalik mammalian biostratigraphy: an overview,” in Fossil Mammals of Asia: Neogene Biostratigraphy and Chronology, eds X. Wang, L. J. Flynn, and M. Fortelius (New York, NY: Columbia University Press, 423–444.
Pickford, M. (1981). Preliminary Miocene mammalian biostratigraphy for Western Kenya. J. Hum. Evol. 10, 73–97. doi: 10.1016/S0047-2484(81)80026-7
Prado, J. L., and Alberdi, M. T. (2017). “Biostratigraphy and Biogeography,” in Fossil Horses of South America: Phylogeny, Systematics, and Ecology, eds J. L. Prado and M. T. Alberdi (New York, NY: Springer, 85–99.
Qiu, Z.-X., Deng, T., and Wang, B.-Y. (2004). Early pleistocene mammalian fauna from Longdan, Dongxiang, Gansu, China. Palaeontolog. Sin. N. Ser. C 27, 1–198.
Qiu, Z.-X., Huang, W.-L., and Guo, Z.-H. (1987). The Chinese hipparionine fossils. Palaeontolog. Sin. N. Ser. C 175, 1–243.
Repenning, C. A. (1988). “Biochronology of the microtine rodents of the United States,” in Cenozoic Mammals of North America—Geochronology and Biostratigraphy, ed M. O. Woodburne (Berkeley, CA: University of California Press, 236–268.
Rook, L., Cirilli, O., and Bernor, R. L. (2017). A late occurring “Hipparion” from the middle Villafranchian of Montopoli, Italy (early Pleistocene; MN16b; ca. 2.5 Ma). Boll. Soc. Paleont. Ital. 56, 333–339. doi: 10.4435/BSPI.2017.28
Rook, L., and Martínez-Navarro, B. (2010). Villafranchian: the long story of a Plio-Pleistocene European large mammal biochronologic unit. Quarter. Int. 219, 134–144. doi: 10.1016/j.quaint.2010.01.007
Sanders, W., Gheerbrant, E., Harris, J. M., Saegusa, H., and Delmer, C. (2010). “Proboscidea,” in Cenozoic Mammals of Africa, eds L. Werdelin and W. J. Sanders (Berkeley, CA: University of California Press), 151–252. doi: 10.1525/california/9780520257214.003.0015
Scott, E. (2004). “Pliocene and Pleistocene horses from porcupine cave,” in Biodiversity Response to Environmental Change in the Middle Pleistocene: The Porcupine Cave Fauna from Colorado, ed A. D. Barnosky (Berkeley, CA: University of California Press), 264–279.
Steininger, R. R., Berggren, W. A., Kent, D. V., Bernor, R. L., Sen, S., and Agusti, J. (1996). “Circum mediterranean neogene (miocene and pliocene) marine-continental chronologic correlations of european mammal units and zones,” in Late Neogene European Biotic Evolution and Stratigraphic Correlation, eds R. L. Bernor, V. FahlBusch, and H. W. Mittmann (New York, NY: Columbia University Press, 7–46.
Tedford, R. H., Albright, I., Barnosky, A. D., Ferrusquia-Villafranca, I., Hunt, R. M. Jr, Storer, J. E., et al. (2004). “Mammalian biochronology of the arikareean through hemphillian interval (late oligocene through early pliocene epochs),” in Late Cretaceous and Cenozoic Mammals of North America: Biostratigraphy and Geochronology, ed M. O. Woodburne (New York, NY: Columbia University Press, 169–231.
Villavicencio, N. A., Lindsey, E. L., Martin, F. M., Borrero, L. A., Moreno, P. I., Marshall, C. R., et al. (2016). Combination of humans, climate, and vegetation change triggered Late Quaternary megafauna extinction in the Última Esperanza region, southern Patagonia, Chile. Ecography 39, 125–140. doi: 10.1111/ecog.01606
Walsh, S. L. (1998). Fossil datum and paleobiological event terms, paleontostratigraphy, chronostratigraphy, and the definition of land mammal “age” boundaries. J. Vertebr. Paleontol. 18, 150–179. doi: 10.1080/02724634.1998.10011041
Wang, S.-Q., and Deng, T. (2011). Some evolutionary trends of Equus eisenmannae (mammalia, perissodactyla) in the stratigraphic sequence of Longdan, China, in comparison to modern Equus. J. Vertebr. Paleontol. 31, 1356–1365. doi: 10.1080/02724634.2011.611203
Wang, X., Li, Q., Xie, G., Saylor, J. E., Tseng, Z. J., Takeuchi, G. T., et al. (2013). Mio-Pleistocene Zanda Basin biostratigraphy and geochronology, pre-Ice Age fauna, and mammalian evolution in western Himalaya. Palaeogeogr. Palaeoclimatol. Palaeoecol. 374, 81–95. doi: 10.1016/j.palaeo.2013.01.007
Werdelin, L. (2010). “Chronology of Neogene Mammal localities,” in Cenozoic Mammals of Africa, eds L. Werdelin and W. L. Sanders (Berkeley, CA: University of California Press, 27–43.
Williams, H. S. (1901). The discrimination of time values in geology. J. Geol. 9, 570–585. doi: 10.1086/620952
Wolf, D., Bernor, R. L., and Hussain, S. T. (2013). A systematic, biostratigraphic, and paleobiogeographic reevaluation of the Siwalik hipparionine horse assemblage from the Potwar Plateau, Nothern Pakistan. Palaeontograp. Abteilung A Paläozool. Stratigr. 300, 1–115. doi: 10.1127/pala/300/2013/1
Woodburne, M. O. (2007). Phyletic diversification of the Cormohipparion occidentale complex (mammalia, perissodactyla, equidae), Late Miocene, North America, and the origin of the old world hippotherium datum. Bull. Am. Mus. Nat. Hist. 306, 1–138. doi: 10.1206/0003-0090(2007)306[1:PDOTCO]2.0.CO;2
Woodburne, M. O. (2009). The Early Vallesian vertebrates of Atzelsdorf (Late Miocene, Austria). 9. Hippotherium (mammalia, equidae). Annal. Naturhistor. Mus. Wien Serie A 111, 585–604.
Woodburne, M. O. (2010). The great American biotic interchange: dispersals, tectonics, climate, sea level and holding pens. J. Mammal. Evol. 17, 245–264. doi: 10.1007/s10914-010-9144-8
Zanolli, C., Kullmer, O., Kelley, J., Bacon, A.-M., Demeter, F., Dumoncel, J., et al. (2019). Evidence for increased hominid diversity in the Early to Middle Pleistocene of Indonesia. Nat. Ecol. Evol. 3, 755–764. doi: 10.1038/s41559-019-0860-z
Zhu, R. X., Potts, R., Pan, Y. X., Yao, H. T., Lü, L. Q., Zhao, X., et al. (2008). Early evidence of the genus Homo in East Asia. J. Hum. Evol. 55, 1075–1085. doi: 10.1016/j.jhevol.2008.08.005
Keywords: Equus evolutionary history, biochronology, land mammal ages, Late Neogene, dispersal events
Citation: Rook L, Bernor RL, Avilla LS, Cirilli O, Flynn L, Jukar A, Sanders W, Scott E and Wang X (2019) Mammal Biochronology (Land Mammal Ages) Around the World From Late Miocene to Middle Pleistocene and Major Events in Horse Evolutionary History. Front. Ecol. Evol. 7:278. doi: 10.3389/fevo.2019.00278
Received: 11 February 2019; Accepted: 04 July 2019;
Published: 25 July 2019.
Edited by:
K. Christopher Beard, University of Kansas, United StatesReviewed by:
Richard Charles Hulbert, Florida Museum of Natural History, United StatesChristopher J. Bell, The University of Texas at Austin, United States
Copyright © 2019 Rook, Bernor, Avilla, Cirilli, Flynn, Jukar, Sanders, Scott and Wang. This is an open-access article distributed under the terms of the Creative Commons Attribution License (CC BY). The use, distribution or reproduction in other forums is permitted, provided the original author(s) and the copyright owner(s) are credited and that the original publication in this journal is cited, in accordance with accepted academic practice. No use, distribution or reproduction is permitted which does not comply with these terms.
*Correspondence: Lorenzo Rook, lorenzo.rook@unifi.it