- Department of Ecology, Evolution and Behavior, University of Minnesota, Saint Paul, MN, United States
Evolutionary loss of traits is common over evolutionary time and occurs in diverse taxa. Sexual signals and other non-signal traits should differ in their likelihood of becoming lost because they experience different selection pressures contributing to their diminution or persistence. In particular, conspicuous sexual signals are often exploited by natural enemies; this significant cost can favor signal reduction or loss. Yet sexual signals should also experience strong selection favoring their persistence because they facilitate communication during sexual encounters and their loss would involve changes in both the signaler and receiver. Most examples of sexual signal loss come from phylogenetic studies, so it is difficult to ascertain the context and key factors responsible for their loss. Here, we describe one of the best documented examples of evolutionary sexual signal loss in real time due to signal exploitation: Teleogryllus oceanicus (the Pacific field cricket) in Hawaii where many males have lost the ability to sing due to natural selection from a deadly, acoustically-orienting parasitoid fly. Using sexual signal loss in T. oceanicus as a model, we identify environmental, social, and genetic factors that appear generally important in driving sexual signal loss due to signal exploitation. We also discuss each putative factor contributing to signal loss more broadly within the context of non-signal trait loss. Overall, the factors that facilitate evolutionary loss of signals and other traits exhibit significant parallels. In general, a significant cost from the environment, weak selection for persistence, and alternative ways of accomplishing the former function appear critical to achieving evolutionary loss of both sexual signals and non-signal traits. However, because few empirical examples of sexual signal loss over contemporary timescales exist, we need more theory and empirical work to better understand the evolutionary dynamics of sexual signal loss.
Evolutionary Loss of Traits and Signals
How biodiversity is generated in nature is a fundamental question in evolutionary biology. Though research tends to focus on understanding how new traits arise within a population, biodiversity is also created when traits are lost (Johnson et al., 2012; Ha and Nehm, 2014). Research in recent decades, particularly through use of comparative phylogenic methods, has demonstrated that trait loss is common over evolutionary time. Lost traits are diverse, and include the loss of flight in insects and birds (Roff, 1990, 1994), photosynthesis in plants (Merckx and Freudenstein, 2010), lipid synthesis in parasitoid insects (Visser et al., 2010), and eyes and pigmentation in cave-dwelling fish (Jeffrey, 2005; Hyacinthe et al., 2018). One consistent message from trait loss literature is that traits do not become reduced or lost simply because they are no longer needed in a Lamarckian “use and disuse” fashion. Trait loss can only occur when the costs incurred by the trait outweigh its benefits, and then must be possible given genetic and physiological constraints (van der Kooi and Schwander, 2014). At a minimum, all traits bear constitutive costs associated with maintenance and expression of the trait that can favor trait disintegration, but traits often experience additional costs beyond basic maintenance (Lahti et al., 2009). Because traits differ in the balance of selective costs and benefits and genetic and physiological constraints, not all traits should be equally subject to loss.
We suggest that sexual signals, traits important in mate localization and mate choice, should be particularly difficult to lose. Signals are by definition used during communication and mediate the transfer of information between a signaler and receiver (Bradbury and Vehrencamp, 1998). Hence, a drastic change in any signal should also require concurrent changes in the receiver, since otherwise communication will be disrupted. Unless the genes associated with signal production and reception are the same or linked, this will require two separate sets of genetic changes. Furthermore, signals generally evolve because they are mutually beneficial to the sender and receiver. As long as the information communicated by the signal is useful, selection should favor signal persistence. But at the same time, sexual signals can be costly and face strong natural selection limiting their evolution (more below), and phylogenetic studies have demonstrated that sexual signal loss is surprisingly common over evolutionary time (Wiens, 2001).
The goal of this review is to shed light on the drivers of sexual signal loss, particularly when these signals are exploited by eavesdropping natural enemies. We begin by discussing the forces that shape sexual signal evolution and examples of sexual signal reduction and loss. We then highlight a particularly well-documented case of sexual signal loss due to exploitation by a deadly eavesdropper that has been observed in real time (the Pacific field cricket, Teleogryllus oceanicus, living in Hawaii). Finally, we synthesize inferences from our empirical case study with other empirical, phylogenetic and theoretical studies of sexual signal loss with work on non-signal trait loss more generally to outline important contributors to the evolution of sexual signal loss in nature.
Sexual Signal Evolution and Exploitation
Sexual signals represent some of the most conspicuous and elaborate traits in nature, and include the iconic peacock train, colorful plumage and mating dances in birds of paradise, and the complex songs of many insects and birds (Andersson, 1994; Zuk and Simmons, 2018). Such signals are critical for securing mates and can play an important role during speciation (Panhuis et al., 2001; Andersson and Simmons, 2006; Safran et al., 2013; Servedio and Boughman, 2017). In general, sexual selection is thought to favor signal elaboration (i.e., bigger, louder, more colorful traits) because the signals are favored by females during mate choice or help males win contests against competitors for access to females (Andersson, 1994). But sexual signals also bear significant costs that can temper this positive, directional selection. For instance, sexual signals are often physiologically costly to produce and maintain, or are difficult to maneuver with when escaping predators (Grafen, 1990; Langerhans et al., 2005; Weaver et al., 2017). Furthermore, because sexual selection tends to favor greater conspicuousness and detectability by conspecifics, sexual signals can also readily capture the attention of unintended receivers such as predators and parasites, which can exploit these signals to localize their prey (Sakaluk and Belwood, 1984; Zuk and Kolluru, 1998; Husak et al., 2006; Heinen-Kay et al., 2015).
Signal exploitation by eavesdropping natural enemies is a major force constraining sexual signal evolution, and includes textbook examples of tradeoffs between natural and sexual selection [reviewed in Zuk and Kolluru (1998)]. For instance, the mating calls of field crickets in North America and Hawaii that attract females is also used by a deadly parasitoid fly to locate its cricket host, which has induced shifts in multiple aspects of cricket song and behavior (Cade, 1975; Zuk et al., 1993; Wagner, 1996). In several species of livebearing fish, males from populations with many predators are less colorful than those in safer populations because the bright colors preferred by females also attract attention from piscivorous fish (Endler, 1980, 1983; Godin and McDonough, 2003; Martin et al., 2014; Heinen-Kay et al., 2015). The vocalizations male túngara frogs use to attract females are also used by frog-eating bats (Tuttle and Ryan, 1981) and blood sucking flies (Bernal et al., 2006) to localize their prey. Sexual signals can even be exploited by eavesdropping humans—bison bellow less in areas where hunting occurs than in protected areas (Sarno et al., 2017). But in most of these cases, even though the signal is reduced or shifted to avoid detection by the eavesdropper, the sexual signal is retained and the communication system remains intact. Does natural selection ever overpower sexual selection and lead to the evolutionary loss of sexual signals?
Looking across phylogenies, both reductions and losses of sexual signals are surprisingly common (Reimchen, 1989; Burns, 1998; Wiens, 2001). This underappreciated trend was highlighted in Wien's (2001) review on the topic, where he showed that loss of sexual signals can be more common than gains over long evolutionary time periods. Since its publication, even more examples of signal reduction and loss have been uncovered. For instance, comparative evidence demonstrates reductions or losses of dorsal crests in newts (Wiens et al., 2011), coo repertoire in doves (de Kort and ten Cate, 2004), song complexity in yellow wagtails (Ödeen and Björklund, 2003), coloration in darters (Gumm and Mendelson, 2011), and digit length in two genera of African frogs (Blackburn, 2009). Such comparative studies using phylogenies are extremely useful in highlighting broad patterns across species lineages. But because these signal loss events happened long ago, we only see the pattern and not the selective forces responsible. In some cases, it is possible to draw inferences based on current species distributions and local ecological conditions. For instance, loss of light flashing in fireflies appears at least partially tied to predation risk (Stanger-Hall and Lloyd, 2015), and loss of sexual ornaments in dragon lizards appears correlated with habitat use (Ord and Stuart-Fox, 2005). But a major limitation of such phylogenetic studies is the inability to ascertain causal agents underlying phenotypic differences with a high level of certainty. It is often impossible to know what causes some sexual signals to disappear while others persist.
What exactly constitutes signal loss is a matter of debate because signal expression within a population occurs along a continuum. For example, signals may merely diminish, rather than completely disappear. Populations may also exhibit polymorphisms, where some individuals express the sexual signal while others do not. For the purpose of this paper, we distinguish between signal reduction and signal loss. In the case of signal reduction, though the trait diminished, it is still present in some form and thus able to be used in communication between the sexes. However, we consider polymorphisms within a population to represent signal loss when some individuals fully lack the trait, yet persist in the population. The complete absence of a signal in at least some individuals is the critical distinction and requires more explanation than trait diminution. Ultimately this can manifest as heritable alternative reproductive tactics. While there has been much informative work on the maintenance of heritable alternative male reproductive morphs (e.g., Sinervo and Lively, 1996), the process by which signals are lost in real time is unclear.
To better understand how sexual signal loss occurs in nature—in particular, when the benefits of sexual signal production fail to outweigh the costs of signal exploitation by natural enemies—we need insight from contemporary systems where sexual signal loss has been observed and the causal driving forces are well-understood. While other ecological agents can impose strong costs that may promote signal loss, including resource limitation and maneuverability within a habitat, we limit our discussion to signal exploitation because this represents a key cost largely restricted to sexual signals. Probably the best documented case of sexual signal loss in real time due to signal exploitation comes from Pacific field crickets (Teleogryllus oceanicus) in Hawaii where many males have lost the ability to sing. This system is especially valuable for understanding how signal loss occurs in the wild because the populations have been studied before, during, and after the evolution of signal loss.
Case Study of Sexual Signal Loss: Pacific Field Crickets (Teleogryllus oceanicus)
Pacific field crickets (Teleogryllus oceanicus) are native to Australia and South Pacific Islands, and have been introduced to the Hawaiian Islands where they have been studied extensively on Kauai, Oahu, and the Big Island of Hawaii (Otte and Alexander, 1983; Otte, 1994). Like most crickets, T. oceanicus males attract females by stridulating, or rubbing together specialized structures on their forewings to produce a long-range calling song and a short-range courtship song, which females use to localize and evaluate potential mates (Zuk and Simmons, 1997; Zuk et al., 2001). In Hawaii, but not elsewhere in its range, T. oceanicus co-occurs with an introduced parasitoid fly from North America, Ormia ochracea, that uses male calling song to locate its cricket host (Cade, 1975; Zuk et al., 1993; Figure 1A). When a gravid female fly locates a calling male, they deposit their free-moving larvae around the cricket, which burrow inside and kill the cricket in approximately a week (Cade, 1975; Adamo et al., 1995). The parasitoid fly presents a major threat to cricket survival—males from parasitized populations tend to be younger on average than crickets from locales where the fly does not occur (Simmons and Zuk, 1994). To cope with this strong natural selection, Pacific field crickets in Hawaii have evolved a number of adaptations to avoid detection by the fly. For example, they exhibit different calling song characteristics and daily calling patterns relative to populations that do not co-exist with the fly (Zuk et al., 1993; Rotenberry et al., 1996).
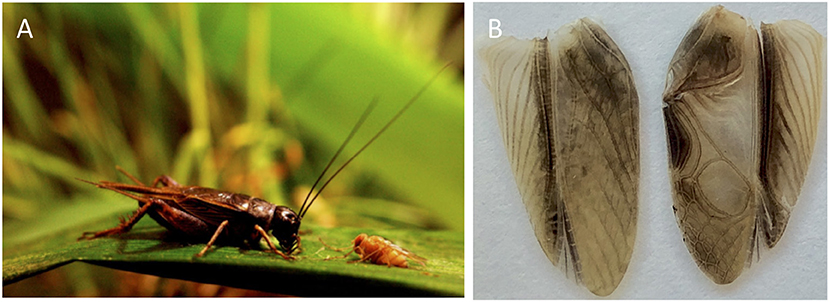
Figure 1. (A) Photograph of Teleogryllus oceanicus (left) and Ormia ochracea (right); Photo courtesy of N. Lee. (B) Photograph of a cricket wing capable of song production (normal-wing; right) and a silent flatwing (left) wing.
Around 2001–2003, a novel wing mutation arose and rapidly spread across the island of Kauai that renders male T. oceanicus obligately silent (Zuk et al., 2006). These silent male morphs are referred to as “flatwings” because they lack sound-producing structures on their forewings (Zuk et al., 2006; Pascoal et al., 2014; Figure 1B). Even though flatwing males still stridulate at approximately the same rate as wild-type males capable of calling (referred to as “normal-wings”), no song is produced (Schneider et al., 2018). By 2003, ~90% of males in the T. oceanicus population on Kauai exhibited the flatwing morphology (Zuk et al., 2006; Pascoal et al., 2014). Flatwing crickets are now known to occur on the three Hawaiian Islands that harbor T. oceanicus, and have stabilized at different proportions of the population: Kauai ~90%, Oahu ~50%, and Hawaii (Big Island) around 2% (Zuk et al., 2018). The silent phenotypes on Kauai and Oahu are caused by independent mutations at different genomic regions, though both mutations segregate as single loci and are located on the X-chromosome (Tinghitella, 2008; Pascoal et al., 2014). Interestingly, a new population of T. oceanicus was recently discovered on the Hawaiian Island of Molokai where many males exhibit an intermediate wing morphology incapable of producing the typical calling song, but instead makes a purring song that is detectable by females (Tinghitella et al., 2018). However, because it is unknown whether O. ochracea is present in Molokai and therefore the role of parasitism in the evolution of purring crickets is unclear (Tinghitella et al., 2018), we disregard this population from our discussion of sexual signal loss in Hawaiian T. oceanicus.
Though other cricket clades with mute males exist, this is often due to the loss of wings rather than an inability to produce sound. The selection pressures that lead to winglessness are likely distinct from those that drive sexual signal loss, and often are linked to habitat stability and lack of dispersal (Roff, 1990; Wagner and Liebherr, 1992). There are also cricket species, such as Gryllus ovisopis in Florida, that lack calling song, but still use courtship song and aggressive chirps to communicate in close proximity, so are not entirely mute (Walker, 1977). This renders the rapid loss of song-production structures in Hawaiian populations of T. oceanicus a unique and informative case study to investigate signal loss.
What allowed signal loss to occur in Hawaiian populations of T. oceanicus? And more generally, in the face of natural selection from sexual signal exploitation, why do some signals persist while others disappear? Below, we describe in detail the environmental, social, and genetic factors that we believe were critical in allowing sexual signal loss to occur in T. oceanicus, and that we further assert should be important in driving sexual signal and non-signal trait loss more broadly.
Contributors to Sexual Signal Loss
Here we discuss key factors that appear to have facilitated sexual signal loss in Hawaiian populations of T. oceanicus. The putative causal factors fall into three primary categories first proposed by Wiens (2001)—environmental, social, and genetic. In Table 1, we summarize these factors and discuss parallels and differences in their likelihood of driving evolutionary loss of sexual signals and non-signal traits. We bolster these suggestions by drawing on other empirical and theoretical work on sexual signal and trait loss. A recent modeling paper (Weigel et al., 2015) was particularly useful in this regard, as the authors explicitly modeled sexual signal loss under a number of scenarios using digital organisms evolved for many generations under different strengths of female preference, flexibility of the mating system (facultative or obligate signaling), population size, and genetic linkage between preference and signal.
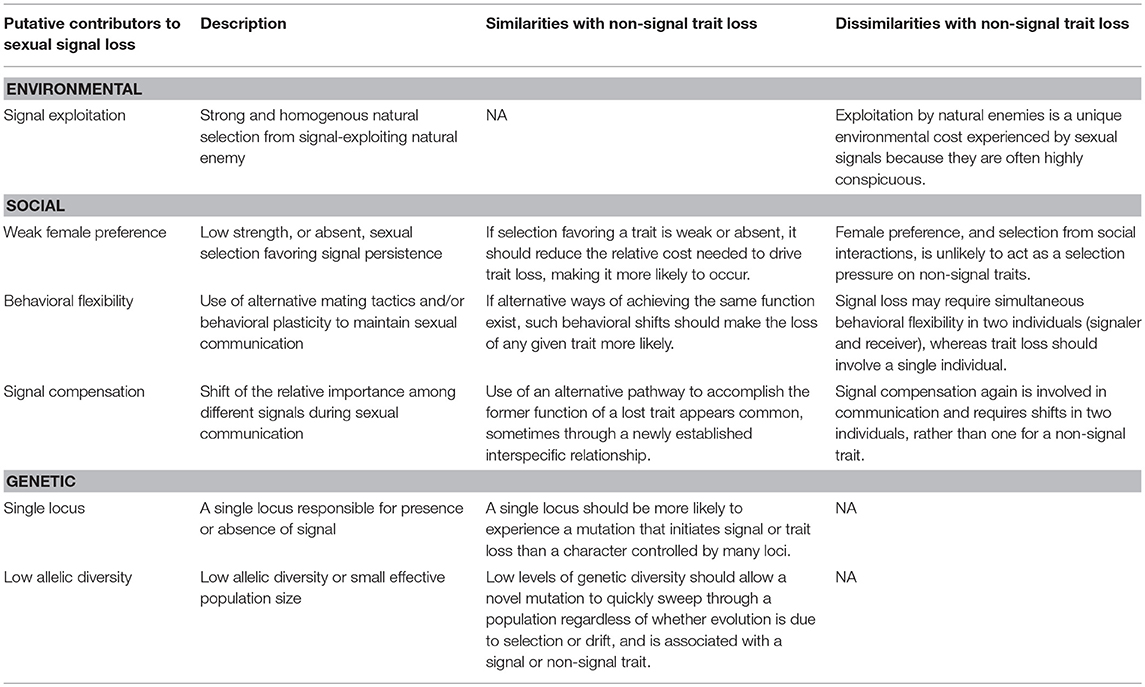
Table 1. Summary of key contributors to sexual signal loss and discussion of their general applicability to evolutionary loss of non-signal traits.
Environmental Factors
Strong, Homogeneous Selection From Signal Exploiter
For a trait to become lost, it must experience significant costs that outweigh the benefits of trait production. In the context of Pacific field crickets, the cost is clear: signal exploitation by a parasitoid fly results in certain death for males. Ormia ochracea are extremely adept at localizing calling male crickets. The hearing ability of O. ochracea is very similar to that of female crickets, and the flies tend to prefer similar song characteristics as female crickets (Cade, 1975; Robert et al., 1992; Wagner, 1996). Field surveys of Hawaiian populations of T. oceanicus prior to the spread of flatwing showed that male crickets experienced high levels of parasitism—for instance, prior to the emergence of flatwing ~25% of calling males captured in Kauai possessed fly larvae (Zuk et al., 1993, 1995). More recent work has confirmed that flatwing males indeed escape parasitism pressure from the fly—only 1 of 121 dissected flatwing males captured in Kauai contained fly larvae (Zuk et al., 2006).
But of course, strong natural selection from signal exploitation does not always lead to signal loss. This is perhaps best exemplified by the fact that other species of field crickets (e.g., Gryllus rubens, G. texensis, and G. lineaticeps) are exploited by O. ochracea in North America (Cade, 1975; Walker and Wineriter, 1991; Wagner, 1996), but to the best of our knowledge, this has not resulted in the evolution of obligately silent (flatwing) male morphs. One key difference between the Hawaiian and North American cricket-fly systems is seasonality of cricket and fly prevalence. In Hawaii, T. oceanicus breed year-round, and the flies are always present. Many North American Gryllids, however, as well as O. ochracea in its native range, show stark seasonality in their abundance. North American Gryllids do not mate continually throughout the year, and the flies tend to occur at low densities in the spring and peak in the fall (Bertram, 2002). The cyclical nature of crickets and flies in North America introduces heterogeneity in the strength of natural selection, with some periods of relaxed natural selection on cricket song (spring) and other times with strong selection (fall). This late season risk of parasitism was suggested as an explanation for why some parasitized field cricket populations in North America have evolved riskier calling songs and preferences than non-parasitized populations (Beckers and Wagner, 2018). Crickets are thought to benefit by investing heavily in signaling in order to mate before the risk of parasitism becomes strong. Such variation in the strength of natural selection from parasites, however, does not occur in Hawaii. Rather, Hawaiian crickets consistently experience strong and persistent selection from the fly that should favor less risky sexual signals. Furthermore, T. oceanicus appears to be the only cricket capable of hosting O. ochracea in Hawaii (Otte, 1994), and these flies are highly responsive to song at the frequency range produced by this species (Gray et al., 2007). Meanwhile, on the mainland O. ochracea can parasitize multiple cricket hosts (Sakaguchi and Gray, 2011). We suggest that strong and consistent selection from the signal exploiter O. ochracea was probably the most important selective force driving sexual signal loss in T. oceanicus in Hawaii.
Social Factors
Weak Female Preference
In the presence of a strong environmental cost, reduced or absent selection in favor of signal retention should further facilitate sexual signal loss. This appears true in Pacific field crickets where females, particularly those from Kauai, express relatively weak preferences for male calling song (Tinghitella et al., 2011). This is consistent with Kaneshiro's (1976; 1980) model of female preference in island populations, which suggested that when population size is small and high-quality males are rare, as may occur after colonization of an island, female choosiness will be selected against. If females are very selective and only willing to mate with high quality males, they may never encounter such a male and subsequently fail to reproduce. Thus, when males are rare, selection should favor females that are less choosey about their mates (Kaneshiro, 1976, 1980). Indeed, female T. oceanicus from islands, including some where the parasitoid does not occur, exhibit significantly more permissive mating behaviors than those from ancestral, mainland populations in Australia (Tinghitella and Zuk, 2009). Population genetic data also indicate that the Hawaiian Island populations show markers of recent genetic bottlenecks, further bolstering this claim (Tinghitella et al., 2011). The Kaneshiro model has been implicated in sexual signal loss in other species, though to our knowledge not in conjunction with signal exploitation. For instance, the Palaearctic yellow wagtail (Motacilla flava) shows dramatic variation in plumage color and song complexity. Reduction in the expression of these sexual signals appears to at least partially result from weak female preferences that emerged during colonization events in conjunction with genetic bottlenecks (Ödeen and Björklund, 2003).
Such permissive female mating behavior in Hawaii is reinforced by socially-mediated behavioral plasticity (see more below). In populations with many silent males, like Kauai, crickets have little exposure to conspecific social cues (i.e., song) that indicate the availability of mates or abundance of competitors an individual can expect to encounter. Female T. oceanicus reared in a song-less acoustic environment, mimicking a high proportion of flatwing males, are less choosy about calling song quality during mate choice than females raised with abundant exposure to calling song (Bailey and Zuk, 2008; Swanger and Zuk, 2015; Lierheimer and Tinghitella, 2017). This creates a positive feedback loop where the song-less environment created by the existence of many flatwing males actually renders females more likely to mate with them, further perpetuating the success of silent males. This social plasticity in female preferences existed prior to the emergence of flatwing (Bailey et al., 2008), and therefore helped facilitate signal loss, and did not emerge as a consequence of signal loss. This is an important discovery because in comparative studies, which often represent the only option to study sexual signal loss, it is difficult to determine whether the signal was lost in response to relaxed selection from females, or if female preference weakened after the signal ceased to exist.
Female preference for a sexual signal has been implicated as a driver of signal loss in Weigel et al. (2015) and some, but not all, comparative studies. Weigel et al. (2015) found that it was very difficult to achieve complete sexual signal loss while females expressed any preference for the sexual signal. The only female preference condition under which sexual signal loss spread to fixation was when female preference was completely absent. If female preference for the male signal was present at even low levels, the strength of preference did not influence the prevalence of signal loss. That is, presence vs. absence of female preference was much more influential than whether the preference was weak or strong—signal loss was just as common at 25% strength as it was at 100%. In livebearing fishes that evolved reduced coloration when sympatric with predators, evidence supporting a role for reduced female preference is mixed. Female guppies from high-predation populations do show weaker preference for male coloration than females from populations that lack predators (Endler and Houde, 1995; Schwartz and Hendry, 2007). However, Bahamas mosquitofish females prefer more colorful males regardless of the predation environment in which they evolved (Heinen-Kay et al., 2015). A similar pattern of weak or absent female preferences was found in swordtail species that lack male sexual signals (Morris et al., 2005; Wong and Rosenthal, 2005). It is unclear why the strength of female preferences varies despite a marked reduction in male signal expression, but it is likely due to different costs incurred or benefits gained by females maintaining such preferences.
Sexual signals are sometimes used during both female choice and male-male competition for access to females (Zuk and Simmons, 2018). If signals are used for multiple functions, the strength of selection favoring their persistence should increase. Even if female preferences are weak, if a signal is important in determining the outcome of intrasexual interactions it could be enough to prevent its loss. In theory, if a sexual signal is used only in male-male competition, the same ideas should apply as we described for female preferences. In Pacific field crickets, males use acoustic signals to communicate during aggressive encounters. While male-male signaling can mitigate the cost of conflict (Logue et al., 2010), it apparently was not enough to halt the evolutionary loss of sound production in this system.
Though mating preferences are unlikely to affect the evolutionary trajectory of traits unrelated to mating, non-signal trait loss in general should be facilitated when selection favoring trait persistence is weak or absent. Trait persistence or loss should largely reflect the net balance of selective pressures favoring persistence or reduction.
Alternative Mating Tactics and Behavioral Flexibility
Loss of a sexual signal requires changes in the mating communication system, while non-signal traits can be lost in relative isolation. Perhaps the easiest way to facilitate a shift in communication is through behavioral flexibility (Zuk and Tinghitella, 2008; Zuk et al., 2014). In most crickets, mate localization and evaluation occur through acoustic signaling, where males produce a call that females are attracted to Zuk and Simmons (1997). Because flatwing males cannot produce the typical sexual signal, this creates an impediment to mating. Flatwings appear to overcome this issue by adopting an alternative mating strategy called satellite behavior, which is common in crickets and existed in the T. oceanicus behavioral repertoire prior to flatwing (Zuk et al., 2006; Tinghitella et al., 2009). Satellite behavior consists of a non-calling male that hovers near a calling male and attempts to intercept females responding to the caller's song. Flatwing males might be particularly successful acting as a satellite because they are differentially attracted to the same male song characteristics that female T. oceanicus prefer in a mate (Olzer and Zuk, 2018). Furthermore, male satellite behavior is enhanced by behavioral plasticity in response to rearing in a song-less environment that occurs as a by-product of the rapid spread of silent males. Males reared in the absence of song are more phonotactic than males reared with exposure to calling song (Bailey et al., 2010). Males from Kauai also walk around more when raised in the absence of calling song, which should increase the likelihood of a chance encounter with a female or calling male (Balenger and Zuk, 2015). As noted above, females reared in a song-less environment are also more phonotactic and express lower mating thresholds (Bailey and Zuk, 2008; Swanger and Zuk, 2015), though they do not show similar flexibility in exploratory behaviors (Heinen-Kay et al., 2018).
Flexibility in the signaling system was also highlighted as an important factor underlying sexual signal loss in Weigel et al.'s (2015) modeling paper. Facultative signaling systems (as opposed to obligate systems, where the signal is required in order to mate), were much more likely to evolve at least some degree of signal loss. This is not surprising, as it highlights the very reason we suggest that sexual signals should be difficult to lose. If the receiver is only willing to mate with individuals that express the signal, even a very costly signal should persist or the population may face local extinction. For instance, if a male signal changes due to strong costs associated with signal exploitation and females are unwilling to mate with males bearing this reduced or absent signal, the population size should dramatically decrease.
Signal Compensation
Another form of behavioral flexibility that may help signal loss occur is signal compensation. Most animals employ multiple sexual signals across a variety of modalities (e.g., acoustic, visual, olfactory), and single signals are often comprised of multiple components (e.g., hue and area of a color patch) that can be differentially targeted by natural and sexual selection (Candolin, 2003; Hebets and Papaj, 2005; Maan and Cummings, 2008). Multiple signals can convey either different or redundant information about the individual bearing the trait (Gomes et al., 2017). The existence of multiple signals, particularly when they are redundant, may provide sufficient flexibility for signal loss to evolve because females could shift the relative importance of the traits they prefer without facing fitness consequences. This could happen if preference for a particular signal weakens for some reason—perhaps if the associated costs begin to outweigh the benefits, or if the information content encoded in the signal becomes unreliable—and the relative importance of a different signal increases as a result. This idea has been referred to as trait switching or trait compensation (Wiens, 2001), and has garnered some empirical support. For example, some Sceloperus lizards have lost a conspicuous colorful belly patch used in intrasexual aggressive encounters, probably due to unwanted attention from predators (Wiens, 1999). Lizards lacking the color patch have evolved more dramatic and complex head bobbing displays (Martins et al., 2015) and more robust chemical signals (Hews and Benard, 2001), suggesting that the modality of signal communication has shifted to accommodate loss of a color signal.
At this point, it is unclear whether flatwing T. oceanicus compensate for lack of song with an alternate sexual signal. Like most insects, T. oceanicus possess cuticular hydrocarbons, long-chain fatty acids expressed on the cuticle that are used for both sexual communication and waterproofing (Ingleby, 2015). Crickets antennate each other prior to copulation and both male and female crickets evaluate cuticular hydrocarbons of potential mates when making mating decisions (Thomas and Simmons, 2009; Simmons et al., 2013). While flatwing males are unable to produce the short-range courtship song typically required in order for the female to mount, if they possessed more attractive chemical cues it could help explain how they are able to achieve matings in the absence of courtship song. Preliminary work suggests that flatwing and normal-wing males express different cuticular hydrocarbon profiles (Simmons et al., 2014), though it is unclear whether females show a preference for singing vs. silent morphs based on these chemical cues (Gray et al., 2014). Cuticular hydrocarbon expression also differs in response to social cues mediated by the acoustic environment experienced during rearing, which could affect the strength of sexual selection on these compounds in the wild (Thomas et al., 2011). However, it is important to note that cuticular hydrocarbons are not detectable over long distances, and therefore would not help females localize males in the absence of calling song. More research is needed to understand whether trait compensation played a role in sexual signal loss of T. oceanicus.
Trait compensation appears generally important in facilitating non-signal trait loss. Ellers et al. (2012) suggested that trait loss often goes undetected in natural populations because organisms do not necessarily lose the function accomplished by the lost trait, particularly in species that exhibit close symbiotic relationships with another species. For instance, some parasitic species of fungi have lost the ability to synthesize lipids, and instead extract these compounds from their host (Visser et al., 2010). Additionally, some plants have lost the ability to photosynthesize when they extract nutrients from other species (Merckx and Freudenstein, 2010). In a way, the alternative mating tactics employed by males are analogous to such an interspecific parasitic relationship—flatwing males essentially parasitize the songs of callers, co-opting their sexual signals to attract females and achieve matings.
Genetic Factors
Single Locus of Large Effect
The genetic architecture of a sexual signal should influence the probability of it becoming lost. In T. oceanicus, the silent male phenotype is due to a mutation on the X-chromosome that is inherited as a single locus (Tinghitella, 2008; Pascoal et al., 2014). Remarkably, the flatwing morphs on Kauai and Oahu are actually the result of two independent mutations in different regions of the X-chromosome, and are not a product of migration between the islands (Pascoal et al., 2014). The genetic underpinning of the flatwing morph on the Big Island of Hawaii has not yet been investigated because few flatwings are present in the population.
In general, trait or signal loss should be more easily accomplished by a mutation at a locus of large effect, rather than via changes at many loci because selection can be diluted. Indeed, a growing number of studies suggests that single loci of large effect tend to be responsible for rapid evolution and trait loss more broadly (Reznick and Ghalambor, 2001). For instance, a single mendelian genetic factor is responsible for the repeated loss of bony armor across stickleback populations released from predation risk (Cresko et al., 2004). In Drosophila, the evolutionary loss of wing pigmentation involved in courtship is caused by a single gene (Prud'homme et al., 2006), and rapid adaptation to a novel thermal environment is due to just two interacting loci (Mallard et al., 2018). Loss of sexual traits due to a transition to asexuality also tends to be caused by a single locus (van der Kooi and Schwander, 2014). While eye loss in cavefish is the product of around 12–15 loci (Protas et al., 2007), the repeated evolution of albinism in cavefish populations is caused by independent mutations in a single gene (Protas et al., 2006). Though evolutionary change in signals should require genetic shifts in both the signaler and the receiver, Weigel et al. (2015) found that genetic linkage between the signaler and receive had little bearing on whether a signal was ultimately lost. This is probably because other factors had a much bigger influence, namely whether the signal was required to initiate mating and whether females exhibited any preference (Weigel et al., 2015).
Low Genetic Diversity in Population
Small population size or low genetic diversity could also create conditions favorable to sexual signal loss because it can increase the chances of a novel mutation sweeping through the population due to selection or drift. In small populations, a trait that is even marginally adaptive could rapidly come to fixation through selection, and deleterious mutations can easily get a foothold through genetic drift. Evidence from T. oceanicus populations suggests recent genetic bottlenecks and low allelic diversity in the Hawaiian Islands relative to mainland populations (Tinghitella et al., 2011; Pascoal et al., 2016). Broadly, small population size with opportunity for rapid growth was identified as an important driver of rapid evolution in an influential review on the topic (Reznick and Ghalambor, 2001). However, it is important to note that the link between genetic variation and signal or trait loss may not be so clear because genetic variation, which should be greater in large populations, is necessary to provide the fodder for selection to act on during the establishment of novel traits. In Weigel et al.'s (2015) modeling study, population size did not affect the likelihood of signal loss becoming established in a population, though greater variability was introduced when populations were small. This argues for a more nuanced view on the role of population size and genetic variation during signal or trait loss, and more empirical evidence from natural populations that have experienced trait loss.
Conclusions
Despite apparent differences in how selection acts on signals and non-signal traits, quite a few commonalities exist regarding the contributors to their loss (Table 1). We identified several factors that likely helped drive sexual signal loss due to signal exploitation based on our case study of Hawaiian T. oceanicus populations and Wiens' (2001) review on sexual signal loss. For each of our highlighted causal factors, parallel examples exist of similar factors being important in driving trait loss more broadly. Most simply, to achieve evolutionary loss of any trait or signal, there needs to be some kind of major cost, weak or absent selection favoring persistence of the trait, and possibly (but not necessarily) an alternative way of maintaining trait functionality. Genetic architecture and population size may also help tip the balance between trait retention and loss, but are unlikely to represent the primary causal agent. Overall, it seems that no one smoking gun exists for sexual signal loss, but rather that it requires a perfect storm of costs, benefits, and sometimes novel ways of accomplishing the same task.
How sexual signals become lost represents an important issue in the evolution of biodiversity. When looking at particularly biodiverse clades, the distinguishing factors between species are often the sexual signals. Though the vast majority of research on sexual signal evolution focuses on elaboration and gains, sexual signals do not always evolve in a single direction, and instead often involves reduction and loss (Wiens, 2001). Given the important role of sexual signals during the maintenance and formation of species boundaries, it is critical to understand how sexual signals evolve, both in terms of how new traits arise and existing traits diminish (Panhuis et al., 2001; Servedio and Boughman, 2017).
Despite many examples of sexual signal loss over long evolutionary durations, sexual signal loss has rarely been documented on a contemporary timescale. In fact, there are relatively few examples of sexual signal evolution—either elaboration or reduction—on short timescales (Svensson and Gosden, 2007). Because of the paucity of examples, we know surprisingly little about the process of rapid sexual signal evolution. Modeling and experimental evolution should prove especially useful in filling this gap because researchers can exert tight control over which variables are manipulated (e.g., heterogeneity and strength of cost and preference, and other factors described in Table 1) to promote sexual signal loss or retention. In the Anthropocene, environmental conditions are changing rapidly and in particular, the composition of ecological communities is shifting due to the rapid spread of invasive and introduced species. Together with rampant habitat fragmentation and loss of genetic diversity in many populations, this may set the stage for new exploitative relationships to develop, and with them, possibly more contemporary examples of sexual signal loss. It is of particular importance that more research capitalizes on these anthropogenic experiments to gain more real-time examples of how sexual signal exploitation influences signal evolution.
Author Contributions
JH-K and MZ together developed the idea and wrote the manuscript.
Funding
MZ is supported by the National Science Foundation (US) and the University of Minnesota College of Biological Sciences.
Conflict of Interest Statement
The authors declare that the research was conducted in the absence of any commercial or financial relationships that could be construed as a potential conflict of interest.
Acknowledgments
We thank Ximena Bernal and Rachel Page for the invitation to contribute to this special edition, and two reviewers who provided thoughtful feedback.
References
Adamo, S. A., Robert, D., and Hoy, R. R. (1995). Effects of a tachinid parasitoid, Ormia ochracea, on the behaviour and reproduction of its male and female cricket hosts (Gryllus spp). J. Insect Phys. 41, 269–277. doi: 10.1016/0022-1910(94)00095-X
Andersson, M., and Simmons, L. W. (2006). Sexual selection and mate choice. Trends Ecol. Evol. 21, 296–302. doi: 10.1016/j.tree.2006.03.015
Bailey, N. W., Gray, B., and Zuk, M. (2010). Acoustic experience shapes alternative mating tactics and reproductive investment in male field crickets. Curr. Biol. 20, 845–849. doi: 10.1016/j.cub.2010.02.063
Bailey, N. W., McNabb, J. R., and Zuk, M. (2008). Preexisting behavior facilitated the loss of a sexual signal in the field cricket Teleogryllus oceanicus. Behav. Ecol. 19, 202–207. doi: 10.1093/beheco/arm123
Bailey, N. W., and Zuk, M. (2008). Acoustic experience shapes female mate choice in field crickets. Proc. R. Soc. B 275, 2645–2650. doi: 10.1098/rspb.2008.0859
Balenger, S. L., and Zuk, M. (2015). Roaming Romeos: male crickets evolving in silence show increased locomotor behaviours. Anim. Behav. 101, 213–219. doi: 10.1016/j.anbehav.2014.12.023
Beckers, O. M., and Wagner, W. E. (2018). Males and females evolve riskier traits in populations with eavesdropping parasitoids. Behav. Ecol. Sociobiol. 72:174. doi: 10.1007/s00265-018-2588-1
Bernal, X. E., Rand, A. S., and Ryan, M. J. (2006). Acoustic preferences and localization performance of blood-sucking flies (Corethrella Coquillett) to túngara frog calls. Behav. Ecol. 17, 709–715. doi: 10.1093/beheco/arl003
Bertram, S. M. (2002). Temporally fluctuating selection of sex-limited signaling traits in the Texas field cricket, Gryllus texensis. Evolution 56, 1831–1839. doi: 10.1111/j.0014-3820.2002.tb00197.x
Blackburn, D. C. (2009). Diversity and evolution of male secondary sexual characters in African squeakers and long-fingered frogs. Biol. J. Linn. Soc. 96, 553–573. doi: 10.1111/j.1095-8312.2008.01138.x
Bradbury, J. W., and Vehrencamp, S. L. (1998). Principles of Animal Communication. Sunderland: Sinauer Associates, Inc.
Burns, K. J. (1998). A phylogenetic perspective on the evolution of sexual dichromatism in tanagers (Thraupidae): the role of female vs. male plumage. Evolution 52, 1219–1224. doi: 10.1111/j.1558-5646.1998.tb01849.x
Cade, W. (1975). Acoustically orienting parasitoids: fly phonotaxis to cricket song. Science 190, 1312–1313. doi: 10.1126/science.190.4221.1312
Candolin, U. (2003). The use of multiple signals during mate choice. Biol. Rev. 78, 575–595. doi: 10.1017/S1464793103006158
Cresko, W. A., Amores, A., Wilson, C., Murphy, J., Currey, M., Phillips, P., et al. (2004). Parallel genetic basis for repeated evolution of loss in Alaskan threespine stickleback populations. Proc. Natl. Aca. Sci. U.S.A 101, 6050–6055. doi: 10.1073/pnas.0308479101
de Kort, S., and ten Cate, C. (2004). Repeated decrease in vocal repertoire size in Streptopelia doves. Anim. Behav. 67, 549–557. doi: 10.1016/j.anbehav.2003.07.004
Ellers, J., Kiers, E. T., Currie, C. R., McDonald, B. R., and Visser, B. (2012). Ecological interactions drive evolutionary loss of traits. Ecol. Lett. 15, 1071–1082. doi: 10.1111/j.1461-0248.2012.01830.x
Endler, J. A. (1980). Natural selection on color patterns in Poecilia reticulata. Evolution 34, 76–91. doi: 10.1111/j.1558-5646.1980.tb04790.x
Endler, J. A. (1983). Natural and sexual selection on color patterns in poeciliid fishes. Envir. Biol. Fish. 9, 173–190. doi: 10.1007/BF00690861
Endler, J. A., and Houde, A. E. (1995). Geographic variation in female preferences for male traits in Poecilia reticulata. Evolution 49, 456–468. doi: 10.1111/j.1558-5646.1995.tb02278.x
Godin, J. G. J., and McDonough, H. E. (2003). Predator preference for brightly colored males in the guppy: a viability cost for a sexually selected trait. Behav. Ecol. 14, 194–200. doi: 10.1093/beheco/14.2.194
Gomes, A. C. R., Funghi, C., Soma, M., Sorenson, M. D., and Cardoso, G. C. (2017). Multimodal signaling in estrildid finches: song, dance and colour are associated with different ecological and life-history traits. J. Evol. Biol. 30, 1336–1346. doi: 10.1111/jeb.13102
Grafen, A. (1990). Biological signals as handicaps. J. Theor. Biol. 144, 517–546. doi: 10.1016/S0022-5193(05)80088-8
Gray, B., Bailey, N. W., Poon, M., and Zuk, M. (2014). Multimodal signal compensation: do field crickets shift sexual selection modality after the loss of acoustic communication? Anim. Behav. 93, 243–248. doi: 10.1016/j.anbehav.2014.04.033
Gray, D. A., Banuelos, C., Walker, S. E., Cade, W. H., and Zuk, M. (2007). Behavioural specialization among populations of the acoustically orienting parasioid fly Ormia ochracea utilizing different cricket species as hosts. Anim. Behav. 73, 99–104. doi: 10.1016/j.anbehav.2006.07.005
Gumm, J. M., and Mendelson, T. C. (2011). The evolution of multi-component visual signals in darters (genus Etheostoma). Curr. Zool. 57, 125–139. doi: 10.1093/czoolo/51.2.125
Ha, M., and Nehm, R. H. (2014). Darwin's difficulties and students' struggles with trait loss: cognitive-historical parallelisms in evolutionary explanation. Sci. Educ. 23, 1051–1074. doi: 10.1007/s11191-013-9626-1
Hebets, E. A., and Papaj, D. R. (2005). Complex signal function: developing a framework of testable hypotheses. Behav. Ecol. 57, 197–214. doi: 10.1007/s00265-004-0865-7
Heinen-Kay, J. L., Morris, K. E., Ryan, N. A., Byerley, S. L., Venezia, R. E., Peterson, M. N., et al. (2015). A tradeoff between natural and sexual selection underlies diversification of a sexual signal. Behav. Ecol. 26, 533–542. doi: 10.1093/beheco/aru228
Heinen-Kay, J. L., Strub, D. B., and Zuk, M. (2018). Limited flexibility in female Pacific field cricket (Teleogryllus oceanicus) exploratory behaviors in response to perceived acoustic environment. Ethology 124, 650–656. doi: 10.1111/eth.12767
Hews, D. K., and Benard, M. F. (2001). Negative association between conspicuous visual display and chemosensory behjavior in two Phrynosomatid lizards. Ethology 107, 839–850. doi: 10.1046/j.1439-0310.2001.00712.x
Husak, J. F., Macedonia, J. M., Fox, S. F., and Sauceda, R. C. (2006). Predation cost of conspicuous male coloration in collared lizards (Crotaphytus collaris): an experimental test using clay-covered model lizards. Ethology 112, 572–580. doi: 10.1111/j.1439-0310.2005.01189.x
Hyacinthe, C., Pierre, C., Retaux, S., Torres-Paz, J., and Retaux, S. (2018). Towards an integrated approach to understand Mexican cavefish evolution. Biol. Lett. 14:20180101. doi: 10.1098/rsbl.2018.0101
Ingleby, F. (2015). Insect cuticular hydrocarbons as dynamic traits in sexual communication. Insects 6, 732–742. doi: 10.3390/insects6030732
Jeffrey, W. R. (2005). Adaptive evolution of eye degeneration in the Mexican blind cavefish. J. Hered. 96, 185–196. doi: 10.1093/jhered/esi028
Johnson, N. A., Lahit, D. C., and Blumstein, D. T. (2012). Combating the assumption of evolutionary progress: lessons from the decay and loss of traits. Evol. Educ. Outreach 5, 128–138. doi: 10.1007/s12052-011-0381-y
Kaneshiro, K. Y. (1976). Ethological isolation and phylogeny in the planitibia subgroup of Hawaiian Drosophila. Evolution 30, 740–745. doi: 10.1111/j.1558-5646.1976.tb00954.x
Kaneshiro, K. Y. (1980). Sexual isolation, speciation and the direction of evolution. Evolution 34, 437–444. doi: 10.1111/j.1558-5646.1980.tb04833.x
Lahti, D. C., Johnson, N. A., Ajie, B. C., Otto, S. P., Hendry, A. P., Blumstein, D. T., et al. (2009). Relaxed selection in the wild. Trends Ecol. Evol. 24, 487–496. doi: 10.1016/j.tree.2009.03.010
Langerhans, R. B., Layman, C. A., and DeWitt, T. J. (2005). Male genital size reflects a tradeoff between attracting mates and avoiding predators in two live-bearing fish species. Proc. Natl. Acad. Sci. U.S.A 102, 7618–7623. doi: 10.1073/pnas.0500935102
Lierheimer, V. F., and Tinghitella, R. M. (2017). Quantity and quality of available mates alters female responsiveness but not investment in the Pacific field cricket, Teleogryllus oceanicus. Behav. Ecol. Sociobiol. 71:80. doi: 10.1007/s00265-017-2298-0
Logue, D. M., Abiola, I. O., Rains, D., Bailey, N. W., Zuk, M., and Cade, W. H. (2010). Does signaling mitigate the cost of agnostic interactions? A test in a cricket that has lost its song. Proc. R. Soc. B 277, 2571–2575. doi: 10.1098/rspb.2010.0421
Maan, M. E., and Cummings, M. E. (2008). Female preferences for aposematic signal components in a polymorphic poison frog. Evolution 62, 2334–2345. doi: 10.1111/j.1558-5646.2008.00454.x
Mallard, F., Nolte, V., Tobler, R., Kapun, M., and Schlötterer, C. (2018). A simple genetic basis of adaptation to a novel thermal environment results in complex metabolic rewiring in Drosophila. Genome Biol. 19:119. doi: 10.1186/s13059-018-1503-4
Martin, R. A, Riesch, R. R., Heinen-Kay, J. L., and Langerhans, R. B. (2014). Evolution of male coloration during a post-Pleistocene radiation of Bahamas mosquitofish (Gambusi hubbsi). Evolution 68, 397–411. doi: 10.1111/evo.12277
Martins, E. P., Ossip-Klein, A. G., Zuniga-Vega, J. J., Vital Garcia, C., Campos, S. M., and Hews, D. K. (2015). Evolving from static to dynamic signals: evolutionary compensation between two communicative signals. Anim. Behav. 102, 223–229. doi: 10.1016/j.anbehav.2015.01.028
Merckx, V., and Freudenstein, J. V. (2010). Evolution of mycoheterotrophy in plants: a phylogenetic perspective. New Phytol. 185, 605–609. doi: 10.1111/j.1469-8137.2009.03155.x
Morris, M. R., Moretz, J. A., Farley, K., and Nicoletto, P. (2005). The role of sexual selection in the loss of sexually selected traits in the swordtail fish Xiphophorus continens. Anim. Behav. 69, 1415–1424. doi: 10.1016/j.anbehav.2004.08.013
Ödeen, A., and Björklund, M. (2003). Dynamics in the evolution of sexual traits: losses and gains, radiation and convergence in yellow wagtails (Motacilla flava). Mol. Ecol. 12, 2113–2130. doi: 10.1046/j.1365-294X.2003.01883.x
Olzer, R. M., and Zuk, M. (2018). Obligate, but not facultative, satellite males prefer the same male sexual signal characteristics as females. Anim. Behav. 144, 37–43. doi: 10.1016/j.anbehav.2018.07.014
Ord, T. J., and Stuart-Fox, D. (2005). Ornament evolution in dragon lizards: multiple gains and widespread losses reveal a complex history of evolutionary change. J. Evol. Biol. 19, 797–808. doi: 10.1111/j.1420-9101.2005.01050.x
Otte, D. (1994). The Crickets of Hawaii: Origin, Systematics, and Evolution. Philadelphia, PA: The Orthopterists' Society; Academy of Natural Sciences.
Otte, D., and Alexander, R. D. (1983). The Crickets of Australia (Orthopter: Gryllidae): Monographs of the Academy of Natural Sciences of Philadelphia, No. 22. Philadelphia, PA: Academy of Natural Sciences.
Panhuis, T. M., Butlin, R., Zuk, M., and Tregenza, T. (2001). Sexual selection and speciation. Trends Ecol. Evol. 16, 364–371. doi: 10.1016/S0169-5347(01)02160-7
Pascoal, S., Cezard, T., Eik-Nes, A., Gharbi, K., Majewska, J., Payne, E., et al. (2014). Rapid convergent evolution in wild crickets. Curr. Biol. 24, 1369–1374. doi: 10.1016/j.cub.2014.04.053
Pascoal, S., Mendrok, M., Mitchell, C., Wilson, A. J., Hunt, J., and Bailey, N. W. (2016). Sexual selection and population divergence I: the influence of socially flexible cuticular hydrocarbon expression in male field crickets (Teleogryllus oceanicus). Evolution 70, 82–97. doi: 10.1111/evo.12839
Protas, M., Conrad, M., Gross, J. B., Tabin, C., and Borowsky, R. (2007). Regressive evolution in the Mexican cave tetra. Curr. Biol. 17, 452–454. doi: 10.1016/j.cub.2007.01.051
Protas, M. E., Hersey, C., Kochanek, D., Zhou, Y., Wilkens, H., Jeffrey, W. R., et al. (2006). Genetic analysis of cavefish reveals molecular convergence in the evolution of albinism. Nat. Genet. 38, 107–111. doi: 10.1038/ng1700
Prud'homme, B., Gompel, N., Rokas, A., Kassner, V. A., Williams, T. M., Yeh, S. D., et al. (2006). Repeated morphological evolution through cis-regulatory changes in a pleiotropic gene. Nature 440, 1050–1053. doi: 10.1038/nature04597
Reimchen, T. E. (1989). Loss of nuptial color in threespine sticklebacks (Gasterosteus aculeatus). Evolution 43, 450–460. doi: 10.1111/j.1558-5646.1989.tb04239.x
Reznick, D. N., and Ghalambor, C. K. (2001). The population ecology of contemporary adaptations: what empirical studies reveal about the conditions that promote adaptive evolution. Genetica 112–113, 183–198. doi: 10.1023/A:1013352109042
Robert, D., Amoroso, J., and Hoy, R. R. (1992). The evolutionary convergency of hearing in a parasitoid fly and its cricket host. Science 258, 1135–1137. doi: 10.1126/science.1439820
Roff, D. A. (1990). Evolution of flightlessness in insects. Ecol. Monogr. 60, 389–321. doi: 10.2307/1943013
Roff, D. A. (1994). The evolution of flightlessness: is history important? Evol. Ecol. 8, 639–657. doi: 10.1007/BF01237847
Rotenberry, J. T., Zuk, M., Simmons, L. W., and Hayes, C. (1996). Phonotactic parasitoids and cricket song structure: an evaluation of alternative hypotheses. Evol. Ecol. 10, 233–243. doi: 10.1007/BF01237681
Safran, R. J., Scordato, E. S. C, Symes, L. B., Rodriguez, R. L., and Mendelson, T. C. (2013). Contributions of natural and sexual selection to the evolution of premating reproductive isolation: a research agenda. Trends Ecol. Evol. 28, 643–650. doi: 10.1016/j.tree.2013.08.004
Sakaguchi, K. M., and Gray, D. A. (2011). Host song selection by an acoustically orienting parasitoid fly exploiting a multispecies assemblage of cricket hosts. Anim. Behav. 81, 851–858. doi: 10.1016/j.anbehav.2011.01.024
Sakaluk, S. K., and Belwood, J. J. (1984). Gecko phonotaxis to cricket calling song: a case of satellite predation. Anim. Behav. 32, 659–662. doi: 10.1016/S0003-3472(84)80141-4
Sarno, R. J., Grigione, M. M., Higa, A., Childers, E., and Ecoffey, T. (2017). The association between continual, year-round hunting and bellowing rate of bison bulls during the rut. PeerJ 5:e3153. doi: 10.7717/peerj.3153
Schneider, W. T., Rutz, C., Hedwig, B., and Bailey, N. W. (2018). Vestigial singing behaviour persists after the evolutionary loss of song in crickets. Biol. Lett. 14:20170654. doi: 10.1098/rsbl.2017.0654
Schwartz, A. K., and Hendry, A. P. (2007). A test for the parallel co-evolution of male coulour and female preference in Trinidadian guppies (Poecilia reticulata). Evol. Ecol. Res. 9, 71–90.
Servedio, M. R., and Boughman, J. W. (2017). The role of sexual selection in local adaptation and speciation. Annu. Rev. Ecol. Evol. Syst. 48:110316-022905. doi: 10.1146/annurev-ecolsys-110316-022905
Simmons, L. W., Thomas, M. L., Gray, B., and Zuk, M. (2014). Replicated evolutionary divergence in the cuticular hydrocarbon profile of male crickets associated with the loss of song in the Hawaiian archipelago. J. Evol. Biol. 27, 2249–2257. doi: 10.1111/jeb.12478
Simmons, L. W., Thomas, M. L., Simmons, F. W., and Zuk, M. (2013). Female preferences for acoustic and olfactory signals during courtship: male crickets send multiple messages. Behav. Ecol. 24, 1099–1107. doi: 10.1093/beheco/art036
Simmons, L. W., and Zuk, M. (1994). Age structure of parasitized and unparasitzed populations of the field cricket Teleogryllus oceanicus. Ethology 98, 333–340. doi: 10.1111/j.1439-0310.1994.tb01081.x
Sinervo, B., and Lively, C. M. (1996). The rock-paper-scissors game and the evolution of alternative male strategies. Nature 380, 240–243. doi: 10.1038/380240a0
Stanger-Hall, K. F., and Lloyd, J. E. (2015). Flash signal evolution in Photinus fireflies: character displacement and signal exploitation in a visual communication system. Evolution 69, 666–682. doi: 10.1111/evo.12606
Svensson, E. I., and Gosden, T. P. (2007). Contemporary evolution of secondary sexual traits in the wild. Funct. Ecol. 21, 422–433. doi: 10.1111/j.1365-2435.2007.01265.x
Swanger, E., and Zuk, M. (2015). Cricket responses to sexual signals are influenced more by adult than juvenile experiences. J. Insect Behav. 28, 328–337. doi: 10.1007/s10905-015-9504-6
Thomas, M. L., Gray, B., and Simmons, L. W. (2011). Male crickets alter the relative expression of cuticular hydrocarbons when exposed to different acoustic environments. Anim. Behav. 82, 49–53. doi: 10.1016/j.anbehav.2011.03.023
Thomas, M. L., and Simmons, L. W. (2009). Sexual selection on cuticular hydrocarbons in the Australian field cricket, Teleogryllus oceanicus. BMC Evol. Biol. 9:162. doi: 10.1186/1471-2148-9-162
Tinghitella, R. M. (2008). Rapid evolutionary change in a sexual signal: genetic control of the mutation, ‘flatwing' that renders male field crickets (Teleogryllus oceanicus) mute. Heredity 100, 261–267. doi: 10.1038/sj.hdy.6801069
Tinghitella, R. M., Broder, E. D., Gurule-Small, G. A., Hallagan, C. J., and Wilson, J. D. (2018). Purring crickets: the evolution of a novel sexual signal. Am. Nat. 192, 773–782. doi: 10.1086/700116
Tinghitella, R. M., Wang, J. M., and Zuk, M. (2009). Preexisting behavior renders a mutation adaptive: flexibility in male phonotaxis behavior and the loss of singing ability in the field cricket Teleogryllus oceanicus. Behav. Ecol. 20, 722–728. doi: 10.1093/beheco/arp052
Tinghitella, R. M., and Zuk, M. (2009). Asymmetric mating preferences accommodated the rapid evolutionary loss of a sexual signal. Evolution 63, 2087–2098. doi: 10.1111/j.1558-5646.2009.00698.x
Tinghitella, R. M., Zuk, M., Beveridge, M., and Simmons, L. W. (2011). Island hopping introduces Polynesian field crickets to novel environments, genetic bottlenecks and rapid evolution. J. Evol. Biol. 24, 1199–1211. doi: 10.1111/j.1420-9101.2011.02255.x
Tuttle, M. D., and Ryan, M. J. (1981). Bat predation and the evolution of frog vocalizations in the neotropics. Science 412, 677–678. doi: 10.1126/science.214.4521.677
van der Kooi, C. J., and Schwander, T. (2014). On the fate of sexual traits under asexuality. Biol. Rev. 89, 805–819. doi: 10.1111/brv.12078
Visser, B., Le Lann, C., den Blanken, F. J., Harvey, J. A., van Alphen, J. J. M., and Ellers, J. (2010). Loss of lipid synthesis as an evolutionary consequence of a parasitic lifestyle. Proc. Natl. Acad. Sci. U.S.A 107, 8677–8682. doi: 10.1073/pnas.1001744107
Wagner, D. L., and Liebherr, J. K. (1992). Flightlessness in insects. Trends Ecol. Evol. 7, 216–220. doi: 10.1016/0169-5347(92)90047-F
Wagner, W. E. (1996). Convergent song preferences between female field crickets and acoustically orienting parasitoid flies. Behav. Ecol. 7, 279–285. doi: 10.1093/beheco/7.3.279
Walker, T. J. (1977). Gryllus ovisopis N. Sp.: a taciturn cricket with a life cycle suggesting allochronic speciation. Flor. Entomol. 57, 13–22. doi: 10.2307/3493823
Walker, T. J., and Wineriter, S. A. (1991). Hosts of a phonotactic parasitoid and levels of parasitism (Diptera: Tachinidae: Ormia ochracea). Flor. Entomol. 74, 554–559. doi: 10.2307/3495408
Weaver, R. J., Koch, R. E., and Hill, G. E. (2017). What maintains signal honesty in animal colour displays used in mate choice? Philos. Trans. R. Soc. B 372:20160343. doi: 10.1098/rstb.2016.0343
Weigel, E. G., Testa, N. D., Peer, A., and Garnett, S. C. (2015). Context matters: sexual signaling loss in digital organisms. Ecol. Evol. 5, 3725–3736. doi: 10.1002/ece3.1631
Wiens, J. J. (1999). Phylogenetic evidence for multiples losses of a sexually selected character in phrynosomatid lizards. Proc. R. Soc. B 266, 1529–1536. doi: 10.1098/rspb.1999.0811
Wiens, J. J. (2001). Widespread loss of sexually selected traits: how the peacock lost its spots. Trends Ecol. Evol. 16, 517–523. doi: 10.1016/S0169-5347(01)02217-0
Wiens, J. J., Sparreboom, M., and Arntzen, J. W. (2011). Crest evolution in newts: implications for reconstruction methods, sexual selection, phenotypic plasticity and the origin of novelties. J. Evol. Biol. 24, 2073–2086. doi: 10.1111/j.1420-9101.2011.02340.x
Wong, B. B., and Rosenthal, G. G. (2005). Female disdain for swords in a swordtail fish. Am. Nat. 167, 136–140. doi: 10.1086/498278
Zuk, M., Bailey, N. W., Gray, B., and Rotenberry, J. T. (2018). Sexual signal loss: the link between behaviour and rapid evolutionary dynamics in a field cricket. J. Anim. Ecol. 87, 623–633. doi: 10.1111/1365-2656.12806
Zuk, M., Bastiaans, E., Langkilde, T., and Swanger, E. (2014). The role of behaviour in the establishment of novel traits. Anim. Behav. 92, 333–344. doi: 10.1016/j.anbehav.2014.02.032
Zuk, M., and Kolluru, G. R. (1998). Exploitation of sexual signals by predators and parasitoids. Q. Rev. Biol. 73, 415–438. doi: 10.1086/420412
Zuk, M., Rotenberry, J. R., and Simmons, L. W. (2001). Geographical variation in calling song of the field cricket Teleogryllus oceanicus: the importance of spatial scale. J. Evol. Biol. 14, 731–741. doi: 10.1046/j.1420-9101.2001.00329.x
Zuk, M., and Simmons, L. W. (1997). “Reproductive strategies of the crickets (Orthoptera: Gryllidae),” in The Evolution of Mating Systems in Insects and Arachnid, eds J. C. Choe and B. J. Crespi (Cambridge: Cambridge University Press, 89–109.
Zuk, M., and Simmons, L. W. (2018). Sexual Selection: A Very Short Introduction. Oxford: Oxford University Press.
Zuk, M., Simmons, L. W., and Cupp, L. (1993). Calling characteristics of parasitized and unparasitized populations of the field cricket Teleogryllus oceanicus. Behav. Ecol. Sociobiol. 33, 339–343. doi: 10.1007/BF00172933
Zuk, M., Simmons, L. W., and Rotenberry, J. R. (1995). Acoustically-orienting parasitoids in calling and silent males of the field cricket Teleogryllus oceanicus. Ecol. Entomol. 20, 380–383. doi: 10.1111/j.1365-2311.1995.tb00471.x
Zuk, M., and Tinghitella, R. M. (2008). “Rapid evolution and sexual selection,” in Sociobiology of Communication, eds P. D. Ettorre and D. P. Hughes (Oxford: Oxford University Press, 139–155.
Keywords: sexual signal loss, trait loss, signal exploitation, signal evolution, sexual selection, natural selection, female mate choice, Teleogryllus oceanicus
Citation: Heinen-Kay JL and Zuk M (2019) When Does Sexual Signal Exploitation Lead to Signal Loss? Front. Ecol. Evol. 7:255. doi: 10.3389/fevo.2019.00255
Received: 18 January 2019; Accepted: 18 June 2019;
Published: 04 July 2019.
Edited by:
Ximena E. Bernal, Purdue University, United StatesReviewed by:
William Wagner, University of Nebraska-Lincoln, United StatesMariella E. Herberstein, Macquarie University, Australia
Copyright © 2019 Heinen-Kay and Zuk. This is an open-access article distributed under the terms of the Creative Commons Attribution License (CC BY). The use, distribution or reproduction in other forums is permitted, provided the original author(s) and the copyright owner(s) are credited and that the original publication in this journal is cited, in accordance with accepted academic practice. No use, distribution or reproduction is permitted which does not comply with these terms.
*Correspondence: Justa L. Heinen-Kay, anVzdGEuaGVpbmVua2F5JiN4MDAwNDA7Z21haWwuY29t