- 1Sea Mammal Research Unit, Scottish Oceans Institute, University of St Andrews, St Andrews, United Kingdom
- 2Centre for Ecology and Conservation, University of Exeter, Cornwall, Penryn, United Kingdom
- 3Swansea Lab for Animal Movement, Department of Biosciences, College of Science, Swansea University, Swansea, United Kingdom
- 4Environment and Sustainability Institute, University of Exeter, Cornwall, Penryn, United Kingdom
- 5RSPB Centre for Conservation Science, Inverness, United Kingdom
- 6Centre d'Ecologie Fonctionnelle et Evolutive, UMR 5175, CNRS - Université de Montpellier - Université Paul-Valéry Montpellier - EPHE, Montpellier, France
- 7Percy FitzPatrick Institute of African Ornithology, DST/NRF Centre of Excellence at the University of Cape Town, Cape Town, South Africa
- 8School of Biology, University of Leeds, Leeds, United Kingdom
- 9Réserve Naturelle des Sept-Iles, Ligue pour la Protection des Oiseaux, Pleumeur Bodou, France
- 10Point Blue Conservation Science, Petaluma, CA, United States
- 11Natural Environment Research Council Life Sciences Mass Spectrometry Facility, Scottish Universities Environmental Research Centre, East Kilbride, United Kingdom
- 12School of Environmental Sciences, University of Liverpool, Liverpool, United Kingdom
- 13British Antarctic Survey, Natural Environment Research Council, Cambridge, United Kingdom
- 14Institute of Biodiversity, Animal Health & Comparative Medicine, College of Medical, Veterinary & Life Sciences, University of Glasgow, Glasgow, United Kingdom
- 15School of Biological Sciences, University of Auckland, Auckland, New Zealand
Migration is a fundamental behavioral process prevalent among a wide variety of animal taxa. As individuals are increasingly shown to present consistent responses to environmental cues for breeding or foraging, it may be expected that approaches to migration would present similar among-individual consistencies. Seabirds frequently show consistent individual differences in a range of traits related to foraging and space-use during both the breeding and non-breeding seasons, but the causes and consequences of this consistency are poorly understood. In this study, we combined analysis of geolocation and stable isotope data across multiple years to investigate individual variation in the non-breeding movements and diets of northern gannets Morus bassanus, and the consequences for changes in body condition. We found that individuals were highly repeatable in their non-breeding destination over consecutive years even though the population-level non-breeding distribution spanned >35° of latitude. Isotopic signatures were also strongly repeatable, with individuals assigned to one of two dietary clusters defined by their distinct trophic (δ15N) and spatial (δ13C) position. The only non-breeding destination in which the two dietary clusters co-occurred was off the coast of northwest Africa. The majority of individuals adopted a consistent foraging strategy, as they remained within the same dietary cluster across years, with little variation in body mass corrected for size among these consistent individuals. In contrast, the few individuals that switched clusters between years were in better condition relative to the rest of the population, suggesting there may be benefits to flexibility during the non-breeding period. Our results indicate that a consistent migratory strategy can be effective regardless of wintering region or diet, but that there may be benefits to those individuals able to display flexibility. This appears to be an important behavioral strategy that may enhance individual condition.
Introduction
Animal migration is a fundamental behavioral process that involves seasonal movements between habitats in response to resource heterogeneity. Although prevalent among a wide variety of animal taxa, there is enormous variation in migration strategy in terms of the distance traveled and the degree of seasonal site fidelity, which ranges from strong philopatry to the loose tracking of seasonal resources (Webster et al., 2002; Newton, 2008). Variation among individuals is often attributed to age, sex or morphology (Marra, 2000; Alerstam et al., 2003; Bailleul et al., 2010) but may also be the result of differences in foraging behavior, breeding success or endogenous control (Bradshaw et al., 2004; Phillips et al., 2005; Broderick et al., 2007; Dias et al., 2011). Despite increasing evidence for individual differences in migratory behavior, the degree of consistency or plasticity and their causes and consequences remain incompletely understood (Chapman et al., 2011; Phillips et al., 2017).
A high degree of consistency in migration strategy with strong individual non-breeding site fidelity can be advantageous if this allows access to predictable foraging resources (Bradshaw et al., 2004; Weimerskirch, 2007) and reduces risks associated with exploring novel habitat (McNamara and Dall, 2010). Relatively inflexible strategies are seen in a number of groups, including passerines (Cuadrado et al., 1995), waterfowl (Hestbeck et al., 1991), cetaceans (Calambokidis et al., 2001), pinnipeds (Bradshaw et al., 2004), seabirds (Phillips et al., 2005, 2006), turtles (Broderick et al., 2007), and sharks (Jorgensen et al., 2010). Conversely, if food availability is unpredictable, or environmental conditions are prone to deteriorate in particular regions during the non-breeding period, selection should favor migratory flexibility or nomadism (Andersson, 1980) and facilitate plastic responses within individuals (Switzer, 1993; Sutherland, 1998). Such strategies are seen in groups including seabirds (Dias et al., 2011), waterbirds (Pedler et al., 2014), ungulates (Morrison and Bolger, 2012), and fish (Tibblin et al., 2016). Thus, the extent to which individuals respond to biotic and abiotic variation across time and space can select for clear individual differences in both movement and foraging strategies.
Recent studies of migrant birds show that individual differences in habitat selection and foraging behavior can influence diet quality during the non-breeding season and impact subsequent breeding traits such as body condition, timing of breeding, egg volume, and breeding success (Bearhop et al., 2004; Inger et al., 2008; Sorensen et al., 2009; Hoye et al., 2012), with important fitness consequences (Marra et al., 1998; Crossin et al., 2010; Inger et al., 2010; Harrison et al., 2011). Thus, individuals that pursue a non-breeding strategy that produces strong negative carry-over effects might be expected to preferentially switch strategies in subsequent years, reducing within-individual consistency (Switzer, 1993; Dias et al., 2011; Morrison and Bolger, 2012). Understanding the incidence and implications of individual consistency or flexibility in non-breeding behavior is therefore a key issue in animal ecology, yet there are few long-term studies that quantify these individual differences over multiple seasons or migration periods (Araújo et al., 2011; Phillips et al., 2017).
Marine predators such as seabirds provide an ideal model for examining such questions as they exhibit a broad spectrum of individual differences in behavior (Votier et al., 2010; Patrick et al., 2014). Recent work suggests these differences are likely to develop through ontogeny (Votier et al., 2017) as individuals learn to target profitable habitat (Grecian et al., 2018). In addition, many species display site fidelity to broadly productive regions during the non-breeding period (Grecian et al., 2016; Phillips et al., 2017). Disentangling individual differences in non-breeding foraging behavior and site fidelity may provide insights into how carry-over effects shape the annual cycle of an individual (Furness et al., 2006). For example, when local conditions are poor, individuals may switch non-breeding region while targeting the same preferred prey or, alternatively, may remain within the same preferred non-breeding region and instead switch prey types (Orben et al., 2015).
In this study, we combine multi-year deployments of geolocation loggers with stable carbon and nitrogen isotope analysis of winter-grown feathers to investigate the degree of individual consistency in the non-breeding destination and foraging behavior of a generalist marine predator, the northern gannet (Morus bassanus), tracked from four breeding colonies in the NE Atlantic. Gannets exhibit a southward-oriented chain migration following a flyway running along the coast of western Europe and Africa (Fort et al., 2012). Variation in migratory behavior, the migration path, final non-breeding destination and foraging behavior during these periods, occurs both among and within populations (Kubetzki et al., 2009; Fort et al., 2012; Deakin et al., 2019), and one recent study has shown that individuals in the NW Atlantic exhibit consistent behavioral strategies in successive years (Fifield et al., 2014). Additionally, the non-breeding distributions of gannets may have changed in recent decades (Kubetzki et al., 2009), suggesting a degree of plasticity in migratory behavior. Such shifts could be linked to changes in human fishing activity as many seabirds are attracted to the foraging opportunities afforded by fisheries (Pichegru et al., 2007; Votier et al., 2010; Bodey et al., 2014a; Patrick et al., 2015). This behavior may come at a cost; as well as increasing the risk of bycatch (Bicknell et al., 2013), diets high in discards can have reduced lipid content compared to pelagic fishes, with the potential for adverse effects on body condition and breeding success (Grémillet et al., 2008; Votier et al., 2010). Should dependency on this resource also be evident in the non-breeding season, there may be further fitness consequences via carry-over effects. We therefore examine whether differences in non-breeding destination and diet affect individual body condition (as a short-term fitness proxy) during the subsequent breeding season.
Materials and Methods
Study System and Data Collection
We collected data between 2008 and 2012 from gannets at four colonies in the northeast Atlantic: Bass Rock, Scotland; Grassholm, Wales; Great Saltee, Ireland; and Rouzic, France (Figure 1). In total, 187 breeding adults with chicks aged between 2 and 7 weeks (egg laying is poorly synchronized across the breeding colony) were caught at the nest during changeover of brood-guard duties using a brass noose or crook attached to the end of a carbon fiber pole (Table 1). On capture, the mass (to the nearest 50 g) and bill length (to the nearest 0.1 mm) of each individual was measured, and sex was subsequently assigned from DNA using 2550F, 2718R, or 2757R primers (Griffiths et al., 1998; Fridolfsson and Ellegren, 1999) following Stauss et al. (2012).
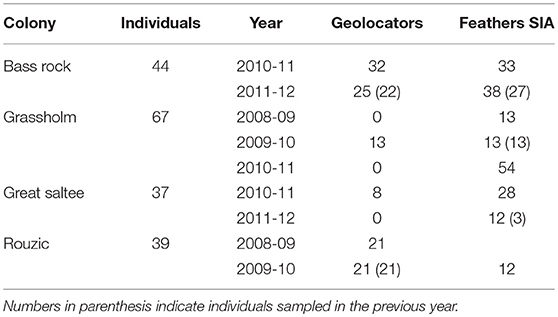
Table 1. Summary of samples collected from 187 northern gannets across four breeding colonies between 2008 and 2012 including geolocation loggers and feather stable isotope analysis (SIA).
Non-breeding Destination
Combined geolocation-immersion loggers (Mk 19, 15, and 5, British Antarctic Survey, Cambridge UK) were deployed on 77 of these individuals across the four colonies. Loggers were attached with two cable ties to a plastic ring, which was then fitted to the tarsus and remained in place for up to 2 years before the bird was recaptured at the breeding colony. The total mass of the attachment did not exceed 10 g, representing <0.35% of average adult body mass, and so unlikely to have any adverse effects (Bodey et al., 2018a). The loggers sampled ambient light every minute and recorded the maximum value every 2, 5, or 10 min (Mk 19, 15, and 5 loggers, respectively).
Positions were calculated from logger data following established methods (Wilson et al., 1992; Phillips et al., 2004). Briefly, the timings of sunset and sunrise were estimated using TransEdit2 (British Antarctic Survey, Cambridge UK) using a light-intensity threshold of 16. A minimum dark period of 4 h was set to remove any light-dark transitions created by shading or cloud cover. Latitude was derived from day length, and longitude from the timing of local midday and midnight, with respect to Greenwich Mean Time and Julian day, providing two positions per day with an accuracy of ~200 km (Phillips et al., 2004). Examination of individual migration tracks revealed latitude to be the major axis of movement, with birds tending to migrate southward from the breeding colonies toward northwest Africa (Fort et al., 2012; Figure 2). Plots of displacement from the colony indicated that all individuals reached their final non-breeding destinations by December and remained in this region for a minimum of 1 month before commencing their return migration. The mean latitude and longitude for December was therefore used as the non-breeding destination of each bird.
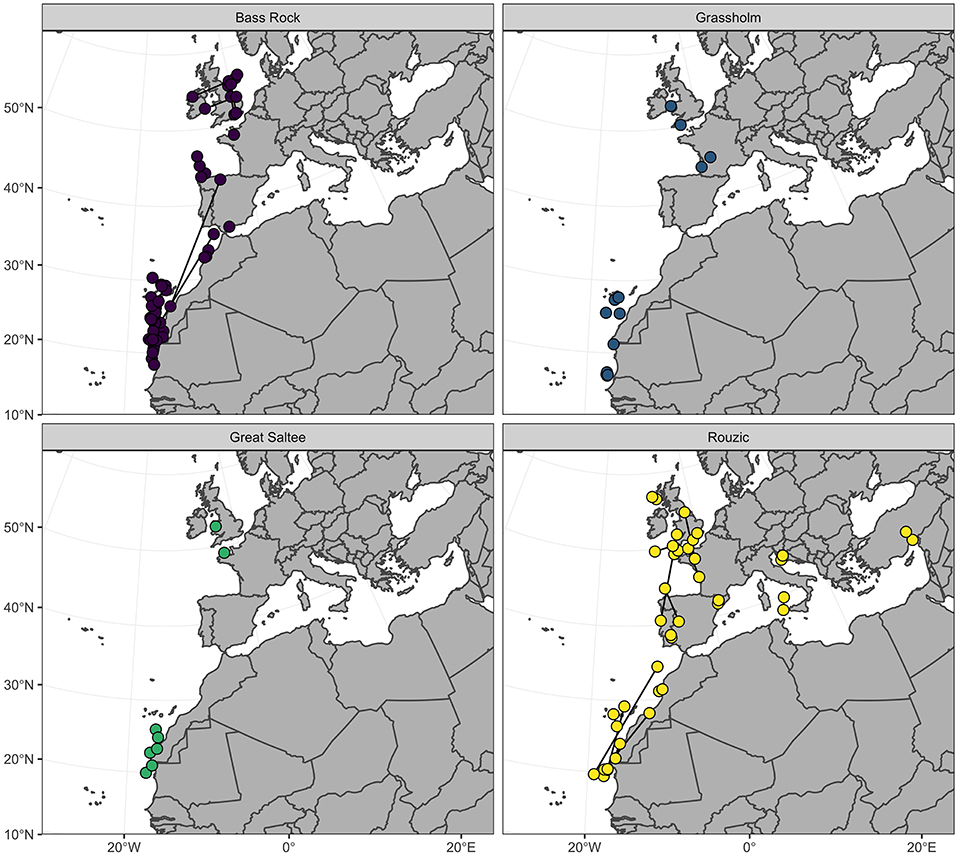
Figure 2. Centroid December location of northern gannets (n = 77) tracked from four colonies in the North East Atlantic. Dark lines connect locations of individuals (n = 43) from Bass Rock and Rouzic tracked over two consecutive non-breeding periods.
Non-breeding Stable Isotopes
Small samples from the 8th primary feather were taken from 148 individuals for stable isotope analysis, with 43 of these individuals sampled a second time when loggers were removed the following year (Table 1). Gannets perform a complete annual molt after the breeding season (from September; Ginn and Melville, 1983), suspending molt by the time the return to the breeding colony (January to March) to invest in nest attendance and foraging trips (see Nelson, 2006). Thus, as feathers are metabolically inert after formation and larger feathers grow over a protracted period, the stable isotope ratios of primary feathers were assumed to largely represent prey consumed at the non-breeding grounds (between October and December).
Feather samples were thoroughly washed with distilled water and placed in a drying oven at ~40°C until dry. The barbules were cut into fine pieces and subsamples of 0.7 ± 0.1 mg were weighed into tin cups. Stable isotope analysis of these subsamples was then conducted at the East Kilbride Node of the Natural Environment Research Council Life Sciences Mass Spectrometry Facility via continuous flow isotope ratio mass spectrometry, using a Thermo Fisher Scientific Delta V Plus with a Costech ECS 4010 elemental analyser configured for simultaneous 13C/12C and 15N/14N isotope analysis. Stable isotope ratios are reported in δ notation, expressed as parts per thousand (%0) deviation according to the equation δX = [(Rsample/Rstandard)-1], where X is 13C or 15N, R is the corresponding ratio 13C/12C or 15N/14N, and Rstandard is the ratio of the international references VPDB for carbon and AIR for nitrogen. At set intervals, standards of GEL, 14N ALA, glycine and tryptophan were analyzed between feather samples in the IRMS. The measurement precision, calculated as the standard deviation of multiple analyses of these standards, was ± 0.1 ‰ for δ13C and ± 0.2 ‰ for δ15N.
Consistency in Non-breeding Strategies and Isotopic Clustering
To examine the consistency of non-breeding destination and stable isotope ratios we calculated the repeatability of these traits based on the intra-class correlation coefficient from linear mixed-effect models fitted with bird ID as a random intercept, using the package “rptR” v. 0.9.21 (Stoffel et al., 2017). We used repeatability as a proxy for behavioral consistency, testing the hypothesis that between-individual variance in a particular trait was greater than within-individual variance (Patrick et al., 2014). To estimate the consistency of non-breeding destinations we calculated the repeatability of mean December latitude and longitude for those individuals from Bass Rock (n = 22) and Rouzic (n = 21), that were tracked over two consecutive years. To estimate the consistency in stable isotope ratios during the non-breeding season we calculated the repeatability of δ13C and δ15N in primary feathers of individuals from Bass Rock (n = 27) and Grassholm (n = 13) sampled in two consecutive years. The three individuals from Great Saltee were excluded from the estimate of isotopic repeatability due to the small, multi-year sample size (Table 1).
To test for the occurrence of distinct dietary clusters in stable isotope ratios we fitted a multivariate normal mixture model to δ13C and δ15N values using the package “mixtools” v. 1.1.0 (Benaglia et al., 2009). The best-fitting model was selected by comparing the log-likelihood of candidate models with differing numbers of clusters. Feather samples were assigned to a dietary cluster based on a probability of assignment >0.5.
Consequences of Non-breeding Strategy
We estimated body condition using a scaled mass index (SMI, Peig and Green, 2009) with bill length as a linear measurement of body size in relation to body mass. However, given that 53 of the individuals were measured in multiple years, we extended this approach to a mixed-effects model with an individual level random intercept, fitted using the package “lme4” v. 1.1-18-1 (Bates et al., 2015). The correlation between body mass and bill length accounting for repeated measures was estimated using the package “rmcorr” v. 0.3.0 (Bakdash and Marusich, 2017). SMI allows for the comparison of the relative size of energy reserves of individuals within a population, avoiding the assumption that larger animals have better body condition due to a higher absolute mass (Peig and Green, 2009). While reproductive success would be a more robust measure of fitness, chick survival from hatching to fledging is over 90% and the majority of offspring mortality occurs during the post-fledging and juvenile period at sea (Nelson, 1966).
The implications of alternative non-breeding strategies (destination and dietary cluster) at the individual level were explored by examining the effects of sex, breeding colony, dietary cluster and non-breeding destination on scaled mass using linear regressions. In cases where there were two observations of an individual's scaled mass in consecutive years, these were fitted as mixed-effects models with individual as a random intercept term. Model selection of linear regressions was based on the F statistic, model selection of mixed-effects linear regression was based on the Chi-squared statistic using likelihood ratio tests. Post-hoc comparisons were made using the package “lsmeans” v. 2.30-0 (Lenth, 2016). All analyses were carried out in R v. 3.4.3 (R Core Team, 2018).
Results
Consistency in Non-breeding Destination and Stable Isotope Ratios
Gannets spent the month of December in one of three regions: a northern region (>36°N), around the British Isles and the Bay of Biscay (n = 26); a southern region (<36°N) from Gibraltar to Mauritania (n = 47); and the Mediterranean Sea (n = 4, all from Rouzic; Figure 2). Individuals from all four colonies were present in both the northern and southern regions during the non-breeding period. The 43 birds from Bass Rock and Rouzic that were tracked over two consecutive years exhibited a high degree of consistency in non-breeding destination and were highly repeatable in both mean December latitude (R = 0.91; CI = 0.83, 0.95; P = 0.001) and longitude (R = 0.92; CI = 0.87, 0.96; P = 0.001; Figure 2).
Stable isotope values in primary feathers from individuals sampled in two consecutive years at Grassholm (n = 13) were repeatable with respect to both δ13C (R = 0.73; CI = 0.35, 0.90; P = 0.004) and δ15N (R = 0.57; CI = 0.06, 0.82; P = 0.028). Individuals from Bass Rock (n = 27) also showed significant repeatability in both δ13C (R = 0.59; CI = 0.27, 0.79; P = 0.002), and δ15N (R = 0.52; CI = 0.20, 0.74; P = 0.002).
Isotopic Clustering
Stable isotope ratios in primary feathers sampled from 148 individuals were best described by a mixture of k = 2 multivariate normal distributions (Table 2). One cluster centered on −13.9 δ13C and 13.2 δ15N, and a second cluster centered on −16.1 δ13C and 15.8 δ15N. The 95% ellipses of the two multivariate normal distributions did not overlap (Figure 3). Seventy-three individuals were assigned to cluster 1 and 75 individuals to cluster 2. Of the 43 individuals (Bass Rock n = 27; Grassholm n = 13; Great Saltee n = 3) that were sampled in consecutive years, most were consistent in their cluster assignment, with 16 assigned to cluster 1 and 20 assigned to cluster 2 in both years. Nevertheless, seven individuals switched between clusters from 1 year to the next (Bass Rock n = 3; Grassholm n = 2; Great Saltee n = 2). Six of these were female and switched from cluster 1 to cluster 2 (lower δ15N to higher δ15N) and one male from Grassholm switched from cluster 2 to cluster 1 (higher δ15N to lower δ15N).
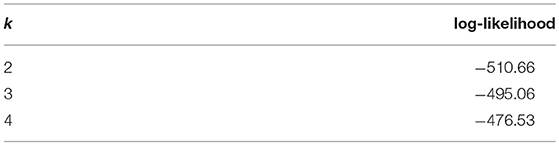
Table 2. Comparison of the log-likelihoods of multivariate normal mixture models fitted with k distributions.
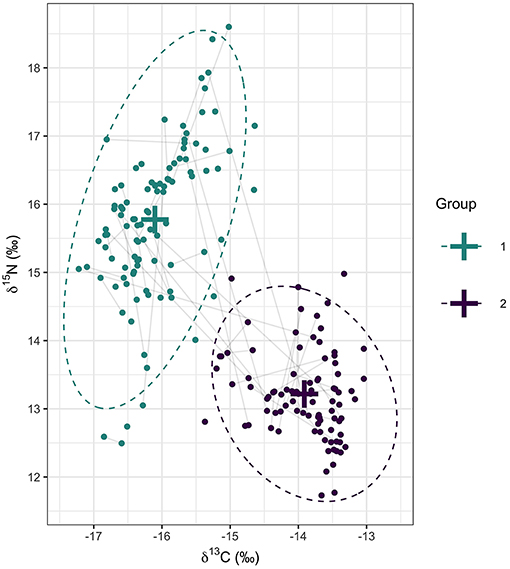
Figure 3. Primary feather δ13C and δ15N values of 148 individual gannets sampled at four colonies in the northeast Atlantic. Crosses represent the mean of the two isotopic clusters identified from a multivariate mixture model and dotted lines represent the 95% ellipses for each distribution. Points are colored based on a probability of group assignment >0.5. Gray lines connect individuals sampled over two consecutive non-breeding periods (n = 43).
Isotopic Clustering Controlling for Winter Destination
Both non-breeding destination and stable isotope data were available for 56 individuals (Bass Rock n = 35, Grassholm n = 13, Great Saltee n = 8). Colony of origin was unrelated to cluster assignment (χ2 = 0.51, P = 0.78) or non-breeding region (χ2 = 0.13, P = 0.94). However, individuals that wintered in the northern region were all assigned to cluster 2 (higher δ15N) whereas individuals that wintered in the southern region were assigned to either isotopic cluster (Figure 4). No isotope data were available for the four individuals that wintered in the Mediterranean.
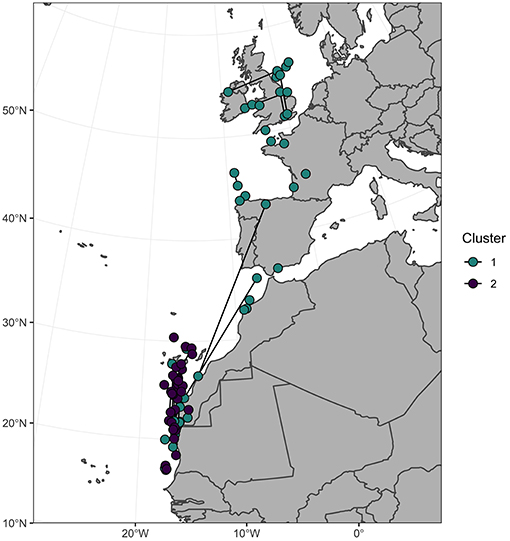
Figure 4. Centroid December locations of 56 northern gannets colored by assignment to one of two isotopic clusters based on a multivariate mixture model of primary feather δ13C and δ15N. Dark lines connect individuals (n = 22) sampled in two consecutive years.
Consequences of Non-breeding Strategy
Female gannets were estimated to be on average 89.4 g (95% CI; 43.9, 134.9) heavier than males ( = 12.9, P < 0.001). Scaled mass differed slightly between the four colonies ( = 9.7, P = 0.02) and post-hoc comparisons indicated that, when averaging over sex differences, individuals sampled at Grassholm were 179.6 g ± 58.0 g lighter than individuals sampled at Bass Rock (z = 3.1, P = 0.01) with no other significant differences between colonies.
There was no difference in scaled mass between individuals using either the northern or southern non-breeding region ( = 0.58, P = 0.44), nor were there differences in scaled mass between birds in the two isotopic clusters ( = 0.0, P = 0.96). Data on scaled mass and feather stable isotope ratios in two consecutive years were available for 34 individuals sampled at Bass Rock (n = 21) and Grassholm (n = 13). In this sample, five individuals switched between the two isotopic clusters and had higher scaled mass (~200 g heavier) compared to those that did not switch isotopic cluster, after accounting for both colony and sex differences (Figure 5). Post-hoc comparisons of marginal means indicated individuals that switched were significantly heavier than individuals in the high δ15N cluster (z = 2.53, P = 0.03). Of these five switching individuals, four were female and switched from the low to high δ15N cluster and one was male and switched from the high to low δ15N cluster. Scaled mass was unavailable for two other switching individuals.
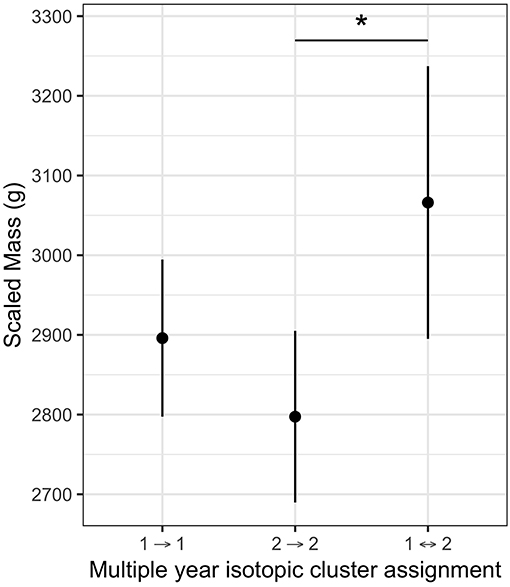
Figure 5. Partial effect of dietary strategy on scaled mass (g) for northern gannets sampled in two consecutive years at Bass Rock and Grassholm (n = 34) after accounting for effect of Colony and Sex. Individuals were assigned to isotopic clusters in each year based on a multivariate normal mixture model of primary feather δ13C and δ15N. Individuals (n = 5) that switched between cluster one and cluster two were heavier relative to those individuals that remained within either cluster one (n = 15) or cluster two (n = 14). Post-hoc comparisons (*) P < 0.05.
Discussion
In this study, we reveal that the non-breeding behavior of individual northern gannets is highly repeatable over consecutive years, with a high degree of site fidelity and consistency in stable isotope ratios during successive non-breeding seasons. Despite substantial differences in destination and variation in foraging strategy among individuals, consistent behaviors during the non-breeding period had no apparent carry-over effect on scaled mass in the subsequent breeding season.
Consistency in Non-breeding Destination and Stable Isotope Ratios
Gannets tracked in this study tended to migrate uniformly southward on a known flyway (Kubetzki et al., 2009; Fort et al., 2012), and spent the non-breeding period in a wide variety of marine habitats including the North Sea, Bay of Biscay, Mediterranean Sea, and Canary Current Upwelling region (Grecian et al., 2016; Figure 2). These three regions differ in their environmental conditions, yet individuals tracked over two consecutive years displayed a high degree of non-breeding site fidelity. The range of ~4 %0 in δ13C and ~6 %0 in δ15N in stable isotope data from the broader sample of the population are larger than the estimates of baseline isotopic variation across the differing non-breeding destinations (McMahon et al., 2013; Magozzi et al., 2017). This suggests that while the population winters across a range of locations, prey are targeted across trophic levels within locations (Inger and Bearhop, 2008).
Adult gannets display consistency in foraging movements and diet within breeding seasons (Patrick et al., 2014; Wakefield et al., 2015; Votier et al., 2017; Bodey et al., 2018b), and the high isotopic consistency observed in individuals in our study that were sampled in consecutive years suggests a similar degree of consistency in both wintering region and the trophic level of prey consumed. Non-breeding site fidelity has been documented in other migratory marine vertebrates (Bradshaw et al., 2004; Broderick et al., 2007; Jorgensen et al., 2010; Phillips et al., 2017) and could allow individuals to increase knowledge of a specific area and thus improve foraging efficiency (Dall et al., 2012).
Isotopic Clustering
Pooling the stable isotope data from all colonies indicated two clusters, indicative of alternative foraging strategies that differed in both spatial (δ13C, δ15N) and trophic (δ15N) characteristics. One cluster was described by higher δ15N and depleted δ13C, consistent with a higher trophic level diet and offshore prey, respectively (Hobson et al., 1994; Post, 2002; Inger and Bearhop, 2008). In contrast, the second cluster was more representative of a diet of inshore (higher δ13C) prey at a lower trophic level (depleted δ15N). Although δ15N can also vary with geographic location (Seminoff et al., 2012; McMahon et al., 2013), the observed difference between these two clusters is greater than the baseline variation between non-breeding destinations (McMahon et al., 2013; Magozzi et al., 2017). In addition, the co-occurrence of individuals from both dietary clusters in the southern wintering area indicates that cluster assignment is not purely driven by the local environment. However, there may be other drivers of the observed isotopic differences, for example individual variation in molt location or feather growth rate could result in a shift in feather isotope signature.
While we lack conventional samples of gannet diet during the winter, the higher trophic level cluster may represent prey obtained primarily as fisheries discards, as δ15N values are elevated in demersal relative to pelagic fish (Votier et al., 2010; Bicknell et al., 2013). In contrast, the second cluster is suggestive of a more inshore diet in pursuit of small forage fish (Garthe et al., 2000; Nelson, 2002). The majority of individuals that were sampled in two consecutive years remained in the same isotopic cluster from one year to the next. Therefore, these clusters may represent foraging strategies that reduce competition among individuals though niche differentiation (Phillips et al., 2009; Young et al., 2010; Bodey et al., 2014b). Foraging specializations have been documented during the breeding season for many seabird species (Annett and Pierotti, 1999; Bearhop et al., 2006; Woo et al., 2008; Phillips et al., 2017) including northern gannets where individuals can vary in the extent of their reliance on high trophic level prey such as fisheries discards (Votier et al., 2010; Patrick et al., 2014; Wakefield et al., 2015;Bodey et al., 2018b).
Consequences of Non-breeding Strategy
Based on our metric of scaled mass, we did not detect any consequences for individuals consistently pursuing different non-breeding strategies. Neither non-breeding destination nor isotopic cluster was significantly related to scaled mass; instead, sex and colony effects drove the observed differences. This is in contrast to patterns seen in thick-billed murres Uria lomvia, where over-wintering foraging strategies are strongly dependant on body size (Orben et al., 2015). Differences in energetic demands over the breeding season may also lead to variation in body condition (Moe et al., 2002) but all individuals in this study were sampled during the chick provisioning period. Sex-linked differences in scaled mass have been observed previously in Northern gannets and may reflect the differing physiological demands of reproduction, and breeding role specialization, as well as more subtle differences between the sexes in prey-capture techniques, nutritional requirements and fine-scale habitat and prey selection (Stauss et al., 2012; Cleasby et al., 2015; Machovsky-Capuska et al., 2016; Bodey et al., 2018b). The difference in scaled mass between individuals at Grassholm and Bass Rock may reflect variation in the prey resources and environmental conditions accessible to individuals from their respective colonies. For example, the North Sea differs in oceanography to the Celtic Sea and supports fewer competing gannet colonies, though the colony at Bass Rock is much larger than at Grassholm and so within-colony competition will be more severe (Nelson, 2002; Wakefield et al., 2013).
Some individuals remained close to the breeding colonies during the non-breeding period and were consistent in this behavior over the two years (Figure 2). Remaining in these areas may decrease energy expenditure by reducing migration costs (Flack et al., 2016), however, this may be offset by the increased energetic requirement for thermoregulation in these colder more northerly waters (Garthe et al., 2012). These individuals were all assigned to the higher δ15N cluster which suggests a greater consumption of fisheries discards or a lack lower δ15N prey available during the winter period (e.g., shoaling forage fish).
The small number of individuals that switched between the two dietary clusters were in better body condition, after accounting for colony and sex effects, than those that were consistent in their cluster assignment. This difference was largest compared to individuals in the higher δ15N cluster, which had relatively low scaled mass. The switching strategy was observed in seven of the 43 individuals (ca. 16%) that were sampled in two consecutive years. Six of these were female and all switched from the lower to higher δ15N cluster. The only individual to switch from the higher to lower δ15N cluster was male. Although foraging on discards brings an additional risk of mortality via incidental bycatch (Bicknell et al., 2013), previous work suggests such a diet may not be detrimental to adult body condition in Cape gannets (Grémillet et al., 2008). Our findings suggest that individuals capable of switching between higher and lower trophic level diets between non-breeding seasons may benefit when compared to individuals specializing in a diet likely to consist of a high proportion of fisheries discards. Alternatively, individual in better condition may be the only ones capable of investing in more risky behaviors (Geary et al., 2019). The majority of individuals switched to the higher δ15N cluster, so this may indicate a short-term benefit of switching to a diet based on fisheries discards or other alternative higher δ15N resources within non-breeding region. The higher δ15N cluster represents one third of those individuals wintering off the coast of northwest Africa, a region known to have experienced a recent intensification of fishing activity (Worm et al., 2009; Grecian et al., 2016).
Conclusions
Our results reveal strong individual consistencies in movement and diet during the non-breeding season, and it is this consistency rather than the strategy itself, that may be important for long-lived species (Ceia et al., 2012; Gilmour et al., 2015). Indeed, such consistency has been demonstrated to result in similar life-time reproductive success among Brünnich's guillemots (Uria lomvia) pursuing different foraging strategies (Woo et al., 2008). Individual repeatability is frequently seen in marine vertebrates despite strong between-year variation in environmental variables and prey fields (Cherel et al., 2007). Importantly however, such consistency could come at a price for highly specialized individuals; for example, changes to anthropogenic subsidies disproportionately affect sub-sections of populations that specialize on such resources (Whitehead and Reeves, 2005; Bicknell et al., 2013). The findings here further highlight the importance of research that links different aspects of behavior between seasons or across annual cycles to understand ecological differentiation among individuals, populations and species (Friesen et al., 2007; Bodey et al., 2014b; Wakefield et al., 2015); and the need to consider the degree of flexibility of individuals and populations to changes in resource availability (Grémillet and Boulinier, 2009).
Data Availability
Telemetry data are available through the BirdLife International Seabird Tracking Database: http://www.seabirdtracking.org. Biometric data are available through the University of St Andrews Research Portal: https://doi.org/10.17630/b3c6dc92-13eb-447d-82d3-acf01d029bc9 (Grecian et al., 2019).
Ethics Statement
Birds were ringed and loggers deployed with permits and approval from the British Trust for Ornithology and Scottish Natural Heritage. Tissue samples were collected under license from the UK Home Office (PPL 30/3065 and 40/3408).
Author Contributions
SV, SB, and KH conceived the study. WG, HW, and TB wrote the first draft of the manuscript. WG, HW, SV, SB, IC, DG, KH, ML, AL, SP, EW, and TB collected data. WG, HW, IC, JN, RP, EW, and TB conducted analyses. All authors contributed to manuscript revision, read and approved the submitted version.
Funding
This study was funded by the UK Natural Environment Research Council (NERC Standard Grant NE/H007466/1). SB is currently funded by an ERC consolidator's grant (310820: STATEMIG). EW is funded by the NERC (Independent Research Fellowship NE/M017990/1). Tissue samples were collected under license from the UK Home Office (PPL 40/3408).
Conflict of Interest Statement
The authors declare that the research was conducted in the absence of any commercial or financial relationships that could be construed as a potential conflict of interest.
Acknowledgments
We thank Sir Hew Hamilton-Dalrymple (Bass Rock), the RSPB (Grassholm), the Neale family (Great Saltee) and the Ligue pour la Protection des Oiseaux and Réserve Naturelle Nationale des Sept- Îles (Rouzic) for permitting and facilitating fieldwork. We are grateful to Fraser Bell, Nadja Christen, Armel Deniau, Richard Inger, Mark Jessopp, Greg & Lisa Morgan, Claudia Stauss, Alyn Walsh, Emma Wood, the Scottish Seabird Center, Maggie Sheddan, and Venture Jet for field assistance.
References
Alerstam, T., Hedenström, A., and Åkesson, S. (2003). Long-distance migration: evolution and determinants. Oikos 103, 247–260. doi: 10.1034/j.1600-0706.2003.12559.x
Andersson, M. (1980). Nomadism and site tenacity as alternative reproductive tactics in birds. J. Anim. Ecol. 49, 175–184. doi: 10.2307/4282
Annett, C. A., and Pierotti, R. (1999). Long-term reproductive output in western gulls: consequences of alternate tactics in diet choice. Ecology 80, 288–297. doi: 10.1890/0012-9658(1999)080<0288:LTROIW>2.0.CO;2
Araújo, M., Bolnick, D. I., and Layman, C. A. (2011). The ecological causes of individual specialisation. Ecol. Lett. 14, 948–958. doi: 10.1111/j.1461-0248.2011.01662.x
Bailleul, F., Authier, M., Ducatez, S., Roquet, F., Charrassin, J. B., Cherel, Y., et al. (2010). Looking at the unseen: combining animal bio-logging and stable isotopes to reveal a shift in the ecological niche of a deep diving predator. Ecography 33, 709–719. doi: 10.1111/j.1600-0587.2009.06034.x
Bakdash, J. Z., and Marusich, L. R. (2017). Repeated measures correlation. Front. Psychol. 8:456. doi: 10.3389/fpsyg.2017.00456
Bates, D., Mächler, M., Bolker, B., and Walker, S. (2015). Fitting linear mixed-effects models using lme4. J. Stat. Softw. 67, 1–48. doi: 10.18637/jss.v067.i01
Bearhop, S., Hilton, G. M., Votier, S. C., and Waldron, S. (2004). Stable isotope ratios indicate that body condition in migrating passerines is influenced by winter habitat. Proc. R. Soc. Lond. Ser. B Biol. Sci. 271, S215–S218. doi: 10.1098/rsbl.2003.0129
Bearhop, S., Phillips, R. A., McGill, R., Cherel, Y., Dawson, D. A., and Croxall, J. P. (2006). Stable isotopes indicate sex-specific and long-term individual foraging specialisation in diving seabirds. Mar. Ecol. Prog. Ser. 311, 157–164. doi: 10.3354/meps311157
Benaglia, T., Chauveau, D., Hunter, D. R., and Young, D. (2009). mixtools : an R Package for analyzing finite mixture models. J. Stat. Softw. 32:25. doi: 10.18637/jss.v032.i06
Bicknell, A. W. J., Oro, D., Camphuysen, K. C. J., and Votier, S. C. (2013). Potential consequences of discard reform for seabird communities. J. Appl. Ecol. 50, 649–658. doi: 10.1111/1365-2664.12072
Bodey, T. W., Cleasby, I. R., Bell, F., Parr, N., Schultz, A., Votier, S. C., et al. (2018a). A phylogenetically controlled meta-analysis of biologging device effects on birds: deleterious effects and a call for more standardized reporting of study data. Methods Ecol. Evol. 9, 946–955. doi: 10.1111/2041-210X.12934
Bodey, T. W., Cleasby, I. R., Votier, S. C., Hamer, K. C., Newton, J., Patrick, S. C., et al. (2018b). Frequency and consequences of individual dietary specialisation in a wide-ranging marine predator, the northern gannet. Mar. Ecol. Prog. Ser. 604, 251–262. doi: 10.3354/meps12729
Bodey, T. W., Jessopp, M. J., Votier, S. C., Gerritsen, H. D., Cleasby, I. R., Hamer, K. C., et al. (2014a). Seabird movement reveals the ecological footprint of fishing vessels. Curr. Biol. 24, R514–R515. doi: 10.1016/j.cub.2014.04.041
Bodey, T. W., Ward, E. J., Phillips, R. A., McGill, R. A. R, and Bearhop, S. (2014b). Species versus guild level differentiation revealed across the annual cycle by isotopic niche examination. J. Anim. Ecol. 83, 470–478. doi: 10.1111/1365-2656.12156
Bradshaw, C. J. A., Hindell, M. A., Sumner, M. D., and Michael, K. J. (2004). Loyalty pays: potential life history consequences of fidelity to marine foraging regions by southern elephant seals. Anim. Behav. 68, 1349–1360. doi: 10.1016/j.anbehav.2003.12.013
Broderick, A. C., Coyne, M. S., Fuller, W. J., Glen, F., and Godley, B. J. (2007). Fidelity and over-wintering of sea turtles. Proc. R. Soc. B Biol. Sci. 274, 1533–1538. doi: 10.1098/rspb.2007.0211
Calambokidis, J., Steiger, G. H., Straley, J. M., Herman, L. M., Cerchio, S., Salden, D. R., et al. (2001). Movements and population structure of humpback whales in the North Pacific. Mar. Mammal Sci. 17, 769–794. doi: 10.1111/j.1748-7692.2001.tb01298.x
Ceia, F. R., Phillips, R. A., Ramos, J. A., Cherel, Y., Vieira, R. P., Richard, P., et al. (2012). Short- and long-term consistency in the foraging niche of wandering albatrosses. Mar. Biol. 159, 1581–1591. doi: 10.1007/s00227-012-1946-1
Chapman, B. B., Brönmark, C., Nilsson, J.-Å., and Hansson, L.-A. (2011). The ecology and evolution of partial migration. Oikos 120, 1764–1775. doi: 10.1111/j.1600-0706.2011.20131.x
Cherel, Y., Hobson, K. A., Guinet, C., and Vanpe, C. (2007). Stable isotopes document seasonal changes in trophic niches and winter foraging individual specialization in diving predators from the Southern Ocean. J. Anim. Ecol. 76, 826–836. doi: 10.1111/j.1365-2656.2007.01238.x
Cleasby, I. R., Wakefield, E. D., Bodey, T. W., Davies, R., Patrick, S. C., Newton, J., et al. (2015). Sexual segregation in a wide-ranging marine predator is a consequence of habitat selection. Mar. Ecol. Prog. Ser. 518, 1–12. doi: 10.3354/meps11112
Crossin, G. T., Trathan, P. N., Phillips, R. A., Dawson, A., Le Bouard, F., and Williams, T. D. (2010). A carryover effect of migration underlies individual variation in reproductive readiness and extreme egg size dimorphism in macaroni Penguins. Am. Nat. 176, 357–366. doi: 10.1086/655223
Cuadrado, M., Senar, J. C., and Copete, J. L. (1995). Do all Blackcaps Sylvia atricapilla show winter site fidelity? Ibis 137, 70–75. doi: 10.1111/j.1474-919X.1995.tb03221.x
Dall, S. R. X., Bell, A. M., Bolnick, D. I., and Ratnieks, F. L. W. (2012). An evolutionary ecology of individual differences. Ecol. Lett. 15, 1189–1198. doi: 10.1111/j.1461-0248.2012.01846.x
Deakin, Z., Hamer, K. C., Sherley, R. B., Bearhop, S., Bodey, T. W., Clark, B., et al. (2019). Sex differences in migration and demography of a wide-ranging seabird, the northern gannet. Mar. Ecol. Progr. Ser. doi: 10.3354/meps12986
Dias, M. P., Granadeiro, J. P., Phillips, R. A., Alonso, H., and Catry, P. (2011). Breaking the routine: individual Cory's shearwaters shift winter destinations between hemispheres and across ocean basins. Proc. R. Soc. B Biol. Sci. 278, 1786–1793. doi: 10.1098/rspb.2010.2114
Fifield, D. A., Montevecchi, W. A., Garthe, S., Robertson, G. J., Kubetzki, U., and Rail, J. F. (2014). Migratory tactics and wintering areas of northern gannets (Morus bassanus) breeding in North America. Ornithol. Monogr. 79, 1–63. doi: 10.1642/aoum.79-1
Flack, A., Fiedler, W., Blas, J., Pokrovsky, I., Kaatz, M., Mitropolsky, M., et al. (2016). Costs of migratory decisions: a comparison across eight white stork populations. Sci. Adv. 2:e1500931. doi: 10.1126/sciadv.1500931
Fort, J., Pettex, E., Tremblay, Y., Lorentsen, S.-H., Garthe, S., Votier, S. C., et al. (2012). Meta-population evidence of oriented chain migration in northern gannets (Morus bassanus). Front. Ecol. Environ. 10, 237–242. doi: 10.1890/110194
Fridolfsson, A.-K., and Ellegren, H. (1999). A simple and universal method for molecular sexing of non-ratite birds. J. Avian Biol. 30:116. doi: 10.2307/3677252
Friesen, V. L., Burg, T. M., and McCoy, K. D. (2007). Mechanisms of population differentiation in seabirds. Mol. Ecol. 16, 1765–1785. doi: 10.1111/j.1365-294X.2006.03197.x
Furness, R. W., Crane, J. E., Bearhop, S., Garthe, S., Käkelä, A., Käkelä, R., et al. (2006). Techniques to link individual migration patterns of seabirds with diet specialization, condition and breeding performance. Ardea 94, 631–638.
Garthe, S., Benvenuti, S., and Montevecchi, W. A. (2000). Pursuit plunging by northern gannets (Sula bassana) feeding on capelin (Mallotus villosus). Proc. R. Soc. Lond. Ser. B Biol. Sci. 267, 1717–1722. doi: 10.1098/rspb.2000.1200
Garthe, S., Ludynia, K., Hüppop, O., Kubetzki, U., Meraz, J. F., and Furness, R. W. (2012). Energy budgets reveal equal benefits of varied migration strategies in northern gannets. Mar. Biol. 159, 1907–1915. doi: 10.1007/s00227-012-1978-6
Geary, B., Walter, S. T., Leberg, P. L., and Karubian, J. (2019). Condition-dependent foraging strategies in a coastal seabird: evidence for the rich get richer hypothesis. Behav. Ecol. 30, 356–363. doi: 10.1093/beheco/ary173
Gilmour, M. E., Lattin, C. R., Romero, L. M., Haussmann, M. F., Mauck, R. A., and Dearborn, D. C. (2015). Finding the best predictor of reproductive performance of Leach's Storm Petrels 132, 191–205. doi: 10.1642/AUK-14-21.1
Grecian, W. J., Lane, J., Michelot, T., Wade, H., and Hamer, K. (2018). Understanding the ontogeny of foraging behaviour: insights from combining marine predator bio-logging with satellite-derived oceanography in hidden Markov models. J. R. Soc. Interface 15:20180084. doi: 10.1098/rsif.2018.0084
Grecian, W. J., Williams, H. J., Votier, S. C., Bearhop, S., Cleasby, I. R., Grémillet, D., et al. (2019). Individual Spatial Consistency and Dietary Flexibility in the Migratory Behaviour of Northern Gannets Wintering in the Northeast Atlantic (dataset). Dataset. University of St Andrews Research Portal. Available online at https://doi.org/10.17630/b3c6dc92-13eb-447d-82d3-acf01d029bc9
Grecian, W. J. J., Witt, M. J. M. J., Attrill, M. J. M. J., Bearhop, S., Becker, P. H. P. H. H., Egevang, C., et al. (2016). Seabird diversity hotspot linked to ocean productivity in the Canary Current Large Marine Ecosystem. Biol. Lett. 12:20160024. doi: 10.1098/rsbl.2016.0024
Grémillet, D., and Boulinier, T. (2009). Spatial ecology and conservation of seabirds facing global climate change: a review. Mar. Ecol. Prog. Ser. 391, 121–137. doi: 10.3354/meps08212
Grémillet, D., Pichegru, L., Kuntz, G., Woakes, A. G., Wilkinson, S., Crawford, R. J. M., et al. (2008). A junk-food hypothesis for gannets feeding on fishery waste. Proc. R. Soc. B Biol. Sci. 275, 1149–1156. doi: 10.1098/rspb.2007.1763
Griffiths, R., Double, M. C., Orr, K., and Dawson, R. J. G. (1998). A DNA test to sex most birds. Mol. Ecol. 7, 1071–1075. doi: 10.1046/j.1365-294x.1998.00389.x
Harrison, X. A., Blount, J. D., Inger, R., Norris, D. R., and Bearhop, S. (2011). Carry-over effects as drivers of fitness differences in animals. J. Anim. Ecol. 80, 4–18. doi: 10.1111/j.1365-2656.2010.01740.x
Hestbeck, J. B., Nichols, J. D., and Malecki, R. A. (1991). Estimates of movement and site fidelity using mark-resight data of wintering canada geese. Ecology 72, 523–533. doi: 10.2307/2937193
Hobson, K. A., Piatt, J. F., and Pitocchelli, J. (1994). Using stable isotopes to determine seabirds trophic relationships. J. Anim. Ecol. 63, 786–798. doi: 10.2307/5256
Hoye, B. J., Hahn, S., Nolet, B. A., and Klaassen, M. (2012). Habitat use throughout migration: linking individual consistency, prior breeding success and future breeding potential. J. Anim. Ecol. 81, 657–66. doi: 10.1111/j.1365-2656.2011.01948.x
Inger, R., and Bearhop, S. (2008). Applications of stable isotope analyses to avian ecology. Ibis 150, 447–461. doi: 10.1111/j.1474-919X.2008.00839.x
Inger, R., Gudmundsson, G. A., Ruxton, G. D., Newton, J., Colhoun, K., Auhage, S., et al. (2008). Habitat utilisation during staging affects body condition in a long distance migrant, Branta bernicla hrota: potential impacts on fitness? J. Avian Biol. 39, 704–708. doi: 10.1111/j.1600-048X.2008.04332.x
Inger, R., Harrison, X. A., Ruxton, G. D., Newton, J., Colhoun, K., Gudmundsson, G. A., et al. (2010). Carry-over effects reveal reproductive costs in a long-distance migrant. J. Anim. Ecol. 79, 974–982. doi: 10.1111/j.1365-2656.2010.01712.x
Jorgensen, S. J., Reeb, C. A., Chapple, T. K., Anderson, S., Perle, C., Van Sommeran, S. R., et al. (2010). Philopatry and migration of Pacific white sharks. Proc. R. Soc. B Biol. Sci. 277, 679–688. doi: 10.1098/rspb.2009.1155
Kubetzki, U., Garthe, S., Fifield, D. A., Mendel, B., and Furness, R. W. (2009). Individual migratory schedules and wintering areas of northern gannets. Mar. Ecol. Ser. 391, 257–265. doi: 10.3354/meps08254
Lenth, R. V. (2016). Least-squares means: the R Package lsmeans. J. Stat. Softw. 69, 1–33. doi: 10.18637/jss.v069.i01
Machovsky-Capuska, G. E., Senior, A. M., Benn, E. C., Tait, A. H., Schuckard, R., Stockin, K. A., et al. (2016). Sex-specific macronutrient foraging strategies in a highly successful marine predator: the Australasian gannet. Mar. Biol. 163, 1–14. doi: 10.1007/s00227-016-2874-2
Magozzi, S., Yool, A., Vander Zanden, H. B., Wunder, M. B., and Trueman, C. N. (2017). Using ocean models to predict spatial and temporal variation in marine carbon isotopes. Ecosphere 8:e01763. doi: 10.1002/ecs2.1763
Marra, P. P. (2000). The role of behavioral dominance in structuring patterns of habitat occupancy in a migrant bird during the nonbreeding season. Behav. Ecol. 11, 299–308. doi: 10.1093/beheco/11.3.299
Marra, P. P., Hobson, K. A., and Holmes, R. T. (1998). Linking winter and summer events in a migratory bird by using stable-carbon isotopes. Science 282, 1884–1886. doi: 10.1126/science.282.5395.1884
McMahon, K. W., Hamady, L. L., and Thorrold, S. R. (2013). A review of ecogeochemistry approaches to estimating movements of marine animals. Limnol. Oceanogr. 58, 697–714. doi: 10.4319/lo.2013.58.2.0697
McNamara, J. M., and Dall, S. R. X. (2010). Information is a fitness enhancing resource. Oikos 119, 231–236. doi: 10.1111/j.1600-0706.2009.17509.x
Moe, B., Langseth, I., Fyhn, M., Gabrielsen, G. W., and Bech, C. (2002), Changes in body condition in breeding kittiwakes Rissa tridactyla. J. Avian Biol. 33:225–234. doi: 10.1034/j.1600-048X.2002.330304.x
Morrison, T. A., and Bolger, D. T. (2012). Wet season range fidelity in a tropical migratory ungulate. J. Anim. Ecol. 81, 543–552. doi: 10.1111/j.1365-2656.2011.01941.x
Nelson, J. B. (1966) The breeding biology of the gannet Sula bassana on the Bass Rock, Scotland. Ibis 108, 584–626. doi: 10.1111/j.1474-919X.1966.tb07210.x.
Nelson, J. B. (2006). Pelicans, Cormorants and their Relatives, the Pelecaniformes. Oxford: Oxford University Press.
Orben, R. A., Paredes, R., Roby, D. D., Irons, D. B., and Shaffer, S. A. (2015). Body size affects individual winter foraging strategies of thick-billed murres in the Bering Sea. J. Anim. Ecol. 84, 1589–1599. doi: 10.1111/1365-2656.12410
Patrick, S. C., Bearhop, S., Bodey, T. W., Grecian, W. J., Hamer, K., Lee, J., et al. (2015). Individual seabirds show consistent foraging strategies in response to predictable fisheries discards. J. Avian Biol. 46, 431–440. doi: 10.1111/jav.00660
Patrick, S. C., Bearhop, S., Grémillet, D., Lescroël, A., Grecian, W. J., Bodey, T. W., et al. (2014). Individual differences in searching behaviour and spatial foraging consistency in a central place marine predator. Oikos 123, 33–40. doi: 10.1111/j.1600-0706.2013.00406.x
Pedler, R. D., Ribot, R. F. H., and Bennett, A. T. D. (2014). Extreme nomadism in desert waterbirds : flights of the banded stilt. Biol. Lett. 10:20140547. doi: 10.1098/rsbl.2014.0547
Peig, J., and Green, A. J. (2009). New perspectives for estimating body condition0 from mass/length data: the scaled mass index as an alternative method. Oikos 118, 1883–1891. doi: 10.1111/j.1600-0706.2009.17643.x
Phillips, R. A., Bearhop, S., McGill, R. A. R., and Dawson, D. A. (2009). Stable isotopes reveal individual variation in migration strategies and habitat preferences in a suite of seabirds during the nonbreeding period. Oecologia 160, 795–806. doi: 10.1007/s00442-009-1342-9
Phillips, R. A., Lewis, S., González-Solís, J., and Daunt, F. (2017). Causes and consequences of individual variability in foraging and migration patterns of seabirds. Mar. Ecol. Prog. Ser. 578, 117–150. doi: 10.3354/meps12217
Phillips, R. A., Silk, J. R. D., Croxall, J. P., and Afanasyev, V. (2006). Year-round distribution of white-chinned petrels from South Georgia: relationships with oceanography and fisheries. Biol. Conserv. 129, 336–347. doi: 10.1016/j.biocon.2005.10.046
Phillips, R. A., Silk, J. R. D., Croxall, J. P., Afanasyev, V., and Bennett, V. J. (2005). Summer distribution and migration of nonbreeding albatrosses: individual consistencies and implications for conservation. Ecology 86, 2386–2396. doi: 10.1890/04-1885
Phillips, R. A., Silk, J. R. D., Croxall, J. P., Afanasyev, V., and Briggs, D. R. (2004). Accuracy of geolocation estimates for flying seabirds. Mar. Ecol. Ser. 266, 265–272. doi: 10.3354/meps266265
Pichegru, L., Ryan, P. G., Van Der Lingen, C. D., Coetzee, J., Ropert-Coudert, Y., and Grémillet, D. (2007). Foraging behaviour and energetics of Cape gannets Morus capensis feeding on live prey and fishery discards in the Benguela upwelling system. Mar. Ecol. Prog. Ser. 350, 127–136. doi: 10.3354/meps07128
Post, D. M. (2002). Using stable isotopes to estimate trophic position: models, methods, and assumptions. Ecology 83, 703–718. doi: 10.1890/0012-9658(2002)083<0703:USITET>2.0.CO;2
R Core Team (2018). R: A Language and Environment for Statistical Computing. Vienna: R Found. Stat. Comput.
Seminoff, J. A., Benson, S. R., Arthur, K. E., Eguchi, T., Dutton, P. H., Tapilatu, R. F., et al. (2012). Stable isotope tracking of endangered sea turtles: validation with satellite telemetry and δ15N analysis of amino acids. PLoS ONE 7:e37403. doi: 10.1371/journal.pone.0037403
Sorensen, M. C., Hipfner, J. M., Kyser, T. K., and Norris, D. R. (2009). Carry-over effects in a Pacific seabird: stable isotope evidence that pre-breeding diet quality influences reproductive success. J. Anim. Ecol. 78, 460–467. doi: 10.1111/j.1365-2656.2008.01492.x
Stauss, C., Bearhop, S., Bodey, T. W., Garthe, S., Gunn, C., Grecian, W. J., et al. (2012). Sex-specific foraging behaviour in northern gannets Morus bassanus: incidence and implications. Mar. Ecol. Prog. Ser. 457, 151–162. doi: 10.3354/meps09734
Stoffel, M. A., Nakagawa, S., and Schielzeth, H. (2017). rptR: repeatability estimation and variance decomposition by generalized linear mixed-effects models. Methods Ecol. Evol. 8, 1639–1644. doi: 10.1111/2041-210X.12797
Sutherland, W. J. (1998). Evidence for flexibility and constraint in migration systems. J. Avian Biol. 29, 441–446. doi: 10.2307/3677163
Switzer, P. V. (1993). Site fidelity in predictable and unpredictable habitats. Evol. Ecol. 7, 533–555. doi: 10.1007/BF01237820
Tibblin, P., Forsman, A., Borger, T., and Larsson, P. (2016). Causes and consequences of repeatability, flexibility and individual fine-tuning of migratory timing in pike. J. Anim. Ecol. 85, 136–145. doi: 10.1111/1365-2656.12439
Votier, S. C., Bearhop, S., Witt, M. J., Inger, R., Thompson, D. R., and Newton, J. (2010). Individual responses of seabirds to commercial fisheries revealed using GPS tracking, stable isotopes and vessel monitoring systems. J. Appl. Ecol. 47, 487–497. doi: 10.1111/j.1365-2664.2010.01790.x
Votier, S. C., Fayet, A. L., Bearhop, S., Bodey, T. W., Clark, B. L., Grecian, J., et al. (2017). Effects of age and reproductive status on individual foraging site fidelity in a long-lived marine predator. Proc. R. Soc. B Biol. Sci. 284:20171068. doi: 10.1098/rspb.2017.1068
Wakefield, E. D., Bodey, T. W., Bearhop, S., Blackburn, J., Colhoun, K., Davies, R., et al. (2013). Space partitioning without territoriality in gannets. Science 341, 68–70. doi: 10.1126/science.1236077
Wakefield, E. D., Cleasby, I. R., Bearhop, S., Bodey, T. W., Davies, R. D., Miller, P. I., et al. (2015). Long-term individual foraging site fidelity—why some gannets don't change their spots. Ecology 96, 3058–3074. doi: 10.1890/14-1300.1
Webster, M. S., Marra, P. P., Haig, S. M., Bensch, S., and Holmes, R. T. (2002). Links between worlds: unraveling migratory connectivity. Trends Ecol. Evol. 17, 76–83. doi: 10.1016/S0169-5347(01)02380-1
Weimerskirch, H. (2007). Are seabirds foraging for unpredictable resources? Deep. Res. Part II Top. Stud. Oceanogr. 54, 211–223. doi: 10.1016/j.dsr2.2006.11.013
Whitehead, H., and Reeves, R. (2005). Killer whales and whaling: the scavenging hypothesis. Biol. Lett. 1, 415–418. doi: 10.1098/rsbl.2005.0348
Wilson, R. P., Ducamp, J. J., Rees, G., Culik, B. M., and Niekamp, K. (1992). “Estimation of location: global coverage using light intensity,” in Wildlife Telemetry: Remote Monitoring and Tracking of Animals, eds I. M. Priede and S. M. Swift (Chichester: Ellis Horward, 131–134.
Woo, K. J., Elliott, K. H., Davidson, M., Gaston, A. J., and Davoren, G. K. (2008). Individual specialization in diet by a generalist marine predator reflects specialization in foraging behaviour. J. Anim. Ecol. 77, 1082–1091. doi: 10.1111/j.1365-2656.2008.01429.x
Worm, B., Hilborn, R., Baum, J. K., Branch, T. A., Collie, J. S., Costello, C., et al. (2009). Rebuilding global fisheries. Science 325, 578–585. doi: 10.1126/science.1173146
Keywords: individual variation, carry-over effects, Geolocator (GLS), stable isotope analysis (SIA), animal migration
Citation: Grecian WJ, Williams HJ, Votier SC, Bearhop S, Cleasby IR, Grémillet D, Hamer KC, Le Nuz M, Lescroël A, Newton J, Patrick SC, Phillips RA, Wakefield ED and Bodey TW (2019) Individual Spatial Consistency and Dietary Flexibility in the Migratory Behavior of Northern Gannets Wintering in the Northeast Atlantic. Front. Ecol. Evol. 7:214. doi: 10.3389/fevo.2019.00214
Received: 14 March 2019; Accepted: 22 May 2019;
Published: 12 June 2019.
Edited by:
Yolanda E. Morbey, University of Western Ontario, CanadaReviewed by:
Kyle Elliott, McGill University, CanadaJaime Albino Ramos, University of Coimbra, Portugal
Copyright © 2019 Grecian, Williams, Votier, Bearhop, Cleasby, Grémillet, Hamer, Le Nuz, Lescroël, Newton, Patrick, Phillips, Wakefield and Bodey. This is an open-access article distributed under the terms of the Creative Commons Attribution License (CC BY). The use, distribution or reproduction in other forums is permitted, provided the original author(s) and the copyright owner(s) are credited and that the original publication in this journal is cited, in accordance with accepted academic practice. No use, distribution or reproduction is permitted which does not comply with these terms.
*Correspondence: W. James Grecian, d2pnNUBzdC1hbmRyZXdzLmFjLnVr; Hannah J. Williams, aC53aWxsaWFtc0Bzd2Fuc2VhLmFjLnVr; Thomas W. Bodey, dC53LmJvZGV5QGV4ZXRlci5hYy51aw==
†These authors have contributed equally to this work