- 1Department of Biosciences, Swansea University, Swansea, United Kingdom
- 2School of Ocean Sciences, Bangor University, Bangor, United Kingdom
- 3Department of Neuroscience, University of Sussex, Brighton, United Kingdom
- 4Department of Estuarine and Delta Systems, Royal Netherlands Institute for Sea Research (NIOZ), Utrecht University, Yerseke, Netherlands
Recent studies in salt marshes have demonstrated the role of plant roots in sediment stabilisation, and hence the importance of marshes in providing coastal protection. However, the relative role of root traits and environmental factors in controlling sediment stability, and how intraspecific variability of root traits vary within and among marshes, remain poorly understood. In this study, we investigated which root trait(s) drive sediment stability (resistance to lateral erosion) in two marsh species with an important role in coastal protection (Spartina anglica and Atriplex portulacoides) and how the environment affects the expression of these traits. We sampled three marshes along salinity gradients in each of two estuaries in Wales (UK), establishing replicate plots in the respective dominant zones of each species. In all plots we sampled abiotic variables (sand, redox potential, pH, salinity) and root traits (root density, specific root density, root volume, root length density); in a subset of these plots (three per species in each marsh) we extracted soil-plant cores and assessed their erosion resistance in a flume. Sediment stability was enhanced by increases in root density and reductions in sand content. Abiotic variables affected root density in different ways depending on species: in S. anglica, redox was the only significant factor, with a positive, linear effect on root density; in A. portulacoides, redox had a non-linear (U-shaped) effect on root density, while sand had a negative effect. Collectively, these results show that (i) intraspecific variability in root density can influence sediment stability in salt marshes, and (ii) sediment properties not only influence sediment stability directly, but also indirectly via root density. These results shed light on spatial variability in the stability of salt marshes to lateral erosion and suggest that root density should be incorporated into coastal vegetation monitoring programs as an easy-to-measure root trait that links the environment to sediment stability and hence to the function and services provided by marshes.
Introduction
Salt marshes are coastal ecosystems that provide humans with valuable services such as carbon storage, forage for livestock, buffers against eutrophication and coastal protection from storms (Barbier et al., 2008; Shepard et al., 2011; Nelson and Zavaleta, 2012; Möller et al., 2014). Several studies demonstrate the ability of salt marsh vegetation to effectively decrease wave energy and stabilise the shoreline (Möller and Spencer, 2002; Bouma et al., 2009, 2010; Shepard et al., 2011; Möller et al., 2014) indicating that marshes are highly beneficial in terms of coastal protection (Costanza et al., 2008; Foster et al., 2013). At the same time, however, researchers have shown the susceptibility of salt marshes to lateral erosion (Mariotti and Fagherazzi, 2010; Marani et al., 2011; Fagherazzi et al., 2013; Leonardi et al., 2016). An increased understanding of what drives the stability of the sediment in salt marshes is a fundamental requirement to the effective integration of salt marshes into coastal management schemes (Feagin et al., 2010; Bouma et al., 2014).
The capacity of salt marshes to resist lateral erosion has received attention recently, with studies establishing that sediment sand content and plant roots are the main drivers of sediment stability (Feagin et al., 2009; Ford et al., 2016; Lo et al., 2017; Wang et al., 2017). In particular, studies in European marshes have demonstrated that increasing root biomass strongly reduces the negative effect of sand on sediment stability (Ford et al., 2016; Wang et al., 2017). Furthermore, variability in root biomass has been shown to affect sediment stability within Spartina spp. (Lo et al., 2017), suggesting that intraspecific variability may play an important role in sediment stabilisation. Yet, little is known about the mechanism by which roots bind the sediment or how the environment drives intraspecific root variability. The response-effect framework of functional traits is a powerful approach for understanding the mechanistic link between the response of organisms to environmental factors and, in turn, the effect on ecosystem functions (Lavorel and Garnier, 2002; Suding et al., 2008; Lavorel et al., 2013). In this framework, variability in environmental factors can modify plant traits (e.g. root length) and, in turn, these changes can affect ecosystem functions (e.g., sediment stability). Thus, understanding the cascade effect from abiotic factors to sediment stability in salt marshes is fundamental to gain insights on marsh lateral resistance to erosion.
In salt marshes, recent studies have investigated only the role of root biomass on sediment stability (Ford et al., 2016; Lo et al., 2017; Wang et al., 2017), while in terrestrial systems wider exploration of a range of traits has shown that root traits underpinning a denser and finer root system reduce soil erosion rates (De Baets et al., 2006; Baets et al., 2007; Burylo et al., 2012; Bardgett and van der Putten, 2014). In particular, studies in terrestrial systems highlight that fine roots are mainly responsible for sediment stabilisation (e.g., Burylo et al., 2012). Furthermore, both work in terrestrial systems and salt marshes has also illustrated the potential for environmental factors to affect root traits that are important for sediment stability. For example, in nutrient poor soils plants invest more biomass in the root system and have higher specific root length (Freschet et al., 2015), which could have a positive effect on soil stability. Similarly, experimental studies in salt marshes have shown that an increasing nutrient load corresponds with a decrease in root biomass and length of first order roots in some species (Bouma et al., 2001a,b; Deegan et al., 2012), which could decrease sediment stability. However, in salt marshes it is unknown how root traits, and fine roots in particular, vary along other key environmental gradients and the consequences for sediment stability. Therefore, understanding the effect of the environment on key root traits has the potential to enhance our ability to predict the stability of marshes to lateral erosion.
Salinity, redox potential (a proxy for anoxia in the sediment) and sand content in soils (a proxy for nutrient levels in the sediment) are known to be strong environmental stressors for salt marsh plants (Armstrong et al., 1985; Olff et al., 1997; Tyler and Zieman, 1999; Crain et al., 2004; Watson and Byrne, 2009), yet how variation in these abiotic factors affects root traits in salt marshes remains largely unknown. Plants show a range of morphological and physiological adaptations to cope with these factors (Naidoo et al., 1992; Colmer and Flowers, 2008; Flowers and Colmer, 2008). For instance, plants can produce glands for salt extrusion in high salinity environments (Tabot and Adams, 2014) and aerenchyma and adventitious roots to allow oxygen transport to the root tips in sediment with low redox (Armstrong, 2000; Nishiuchi et al., 2012). Furthermore, the low nutrient status of sandy soils and their mobility could also affect root development (Olff et al., 1997; Tyler and Zieman, 1999; Schutten et al., 2005; Fourcaud et al., 2008; Freschet et al., 2017). Therefore, when environmental conditions are far from a plant's optimum they can directly reduce overall root growth and induce metabolically expensive adaptations that may affect root trait expression (e.g., fewer fine roots) at the intraspecific level. In this way, adaptations to environmental stresses can have detrimental effects on sediment stability.
We investigated how abiotic factors along environmental gradients directly and indirectly affect the stability of saltmarsh sediment through regulating plant root traits. We tested the stability of extracted cores in a flume system and hypothesised first (H1), that root traits associated with a finer root system will be better predictors of sediment stability than other traits (e.g., root density) because they indicate root biomass is more evenly distributed throughout the sediment, which determines that, second (H2), fine roots will be more important for sediment stability than other below-ground compartments (rhizomes, coarse roots). Furthermore, we also considered the effects of sediment properties on erosion and hypothesised (H3) that increasing sand content would reduce sediment stability. Finally, we investigated the potential for environmental factors to indirectly affect sediment stability via their effects on root traits. We hypothesised (H4) that reduced below-ground plant growth and investment in roots would be associated with stressful sediment conditions (e.g., low redox), indirectly reducing sediment stability. We sampled marshes along two estuaries in South Wales (UK) to encompass natural salinity and redox gradients. We focused on Spartina anglica (C.E. Hubb.) and Atriplex portulacoides (L.) (hereafter Spartina and Atriplex, respectively) because in the UK, both species form large monospecific stands at the marsh edge (Spartina) and along marsh creeks (Atriplex) (Rodwell et al., 2000), thus being directly involved in stabilising sediment against lateral erosion. We analysed the two species separately to understand the importance of intraspecific trait variability for sediment stability in salt marshes.
Materials and Methods
Site Description
Six salt marshes were selected along a salinity gradient in two estuaries in South Wales (UK), the Loughor and the Taf (Figure 1). These marshes showed some variation in community characteristics, but all shared the common feature of extensive monostands of the two target species. In the Loughor estuary, Pembrey Burrows (PB), Penrhyn Gwyn (PNR), and Loughor (LOG) marshes were situated at the mouth, middle, and head of the estuary, respectively. Pembrey contains several zones, with Spartina dominating the pioneer zone and Atriplex occupying the low-mid marsh. Penrhyn Gwyn is characterised by the presence of Spartina and Atriplex, which constitute almost the entire marsh, except for the grazed portion at the landward side; no signs of grazing (browsing marks) were found in the sampling area. Loughor marsh is part of a farm, but no grazing from cattle was observed in the sampled area. Spartina dominates the pioneer zone and Atriplex is present at the low-mid marsh along the creeks; landward of these zones a mixed community is present.
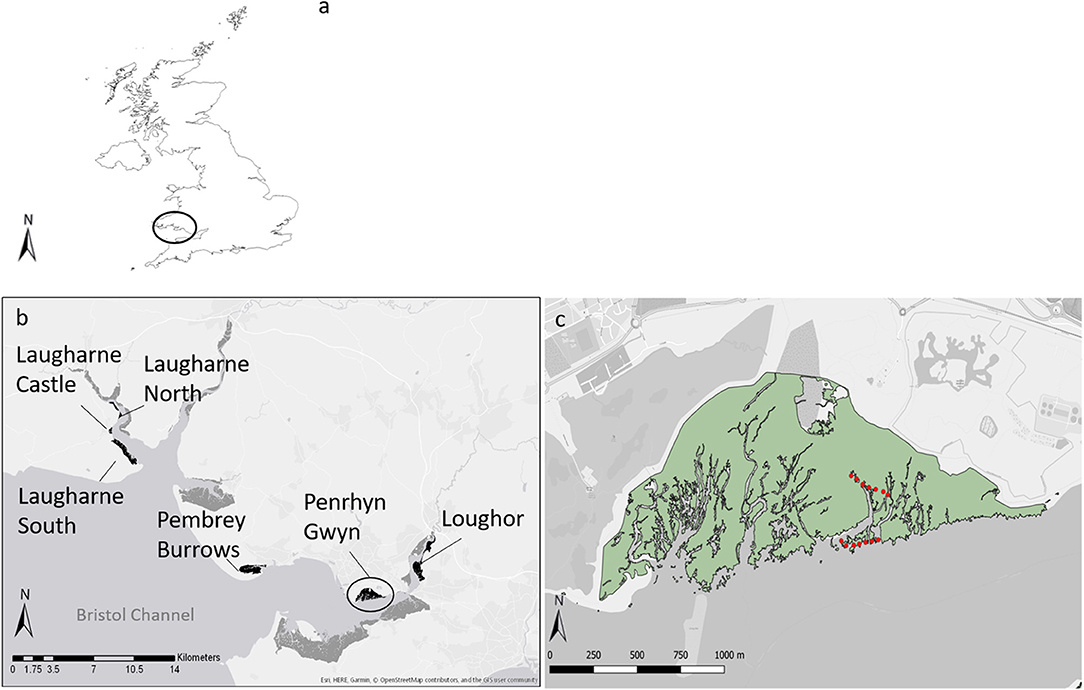
Figure 1. The study sites. In (a), the circle indicates the location of the sampling areas in UK. Panel (b) shows the area inside the circle from (a). Marshes sampled are highlighted in black, other marshes in the estuary are shown in dark grey; from the mouth to the head of the estuary, the position of Pembrey Burrows, Penrhyn Gwyn, and Loughour marshes in the Loughor estuary (lower side panel) and Laugharne South, Laugharne Castle, and Laugharne North marshes in the Taf estuary (left side panel). Panel (c), shows the area inside the circle from (b). The green area represents the salt marshes and red dots represent the GPS coordinates were 1 × 1 meter plots were established; red dots at the edge of the marsh represent plots with Spartina anglica, while the other dots represent plots with Atriplex portulacoides.
Laugharne South (LS), Laugharne Castle (LC), and Laugharne North (LN) are the marshes at the mouth, middle, and head of the Taf estuary, respectively. Laugharne South is dominated by Atriplex although in the pioneer zone Spartina is dominant (with some Salicornia spp. and Suaeda marina). In Laugharne Castle, Spartina is the main species in the pioneer zone with Atriplex present in the low-mid marsh, as a small strip of patchy vegetation. Laugharne North is characterised almost entirely by Atriplex, with the pioneer zone dominated by Spartina.
Study Design
At the end of July 2016, in the areas where Spartina and Atriplex were dominant we established seven 1 × 1 m plots for each species in each salt marsh and recorded GPS positions. Plots were separated by roughly 30 m, except in the Spartina zone in Pembrey where they were 10–15 m apart due to the limited area covered by this species. Plots were positioned to ensure that only the two targeted species were represented with 100% cover and, thus, excavated roots belonged to the species under study. Thus, for a suite of abiotic and root trait parameters (Table A1) we obtained a total of 42 replicates per species (6 marshes × 7 plots per species). In each marsh and for each species, we collected a core of 16 cm in diameter and 30 cm depth from three of the seven plots for a total of 36 cores. Plots were chosen so as to maximise the distance between cores.
Root Traits
In October 2016, sediment samples of 500 cm3 volume (5 × 5 cm surface area and 20 cm depth) were collected adjacent to where the core was extracted for root traits measurements. In plots where cores were not collected, we excavated a piece of marsh to simulate the core extraction and collected the sediment sample as described above. Sediment samples were washed over a sieve (mesh size, 1 mm) to minimise root loss and roots were collected and divided into rhizome, coarse roots (roots >1 mm in diameter) and a mixture of fine roots (roots <1 mm in diameter; Freschet and Roumet, 2017) and dead plant material. Rhizomes and coarse roots were distinguished based on their morphology. Note that, although Atriplex is a dicotyledonous with a tap root system lacking true rhizomes, its shoots have a prostrate growth form and are often buried in the sediment, forming adventitious roots. Thus, from a sediment stability perspective these buried shoots would play a similar role as rhizomes and, for ease of discussion, here are grouped in the rhizome category. The fine roots present in the samples were calculated based of the proportion of fine roots present in three subsamples of ~1 g fresh material.
Root traits were measured on representative subsamples of rhizome, coarse and fine root sub-samples. We placed the root material into a petri dish, scanned all the material (black and white at 1,200 dpi of resolution; Epson Perfection, V550 Photo) and analysed the root length in the scanned images with Rootnav software (Pound et al., 2013). All root and rhizome material was dried at 70°C for 48 h (Pérez-Harguindeguy et al., 2013) and the total specific root length (SRLt) was measured as the sum of the length of all roots (rhizome, coarse roots, and fine roots) divided by the sum of their dry weight. We used SRLt as a proxy of the investment of the plant in rhizome/coarse roots vs. fine roots (Burylo et al., 2012; Freschet and Roumet, 2017). The diameter of ten roots in each image were measured with ImageJ software (Schindelin et al., 2012) and used to calculate total root volume as (r2· π) · ERL, assuming the root is a cylinder; ERL is the estimated length of the entire root system based on the weighted length of scanned roots over the total root weight [(root length/scanned root weight) · total root weight]. Root length density (RLD) and root density (RD) are, respectively, the length and the weight of the entire root system divided by the 500 m3 soil volume sampled (De Baets et al., 2006; Baets et al., 2007). Also, we measured root density for rhizomes (RD.R), coarse roots (RD.C), and fine roots (RD.F) as the weight of each root compartment divided by the 500 m3 soil volume.
Sediment Erosion Rate
Cores (36 in total) were collected in the middle of plots according to Ford et al. (2016) at the end of the growing season (late October 2016). We tested the cores in a flume facility at Bangor University using the methods of Ford et al. (2016), except cores were eroded at only one flow strength (146 Pa). Each core was weighed on a scale, eroded for 5 min and weighed again; we repeated this process five times for a total of 30 min of erosion for each core (examples of eroded cores in Figure A2). This temporal pattern of erosion and measurement allowed us to detect weight loss of clay cores (Atriplex) while avoiding complete erosion of sandy cores (Spartina).
Abiotic Variables
We sampled sediment abiotic variables (Table A1) in plots on three spring tides over July-September 2016 to minimise the influence of variation in tide heights and weather, and plot averages were used for analysis. We inserted Macrorhizones (www.rhizosphere.com) at 15 cm depth, extracted the porewater, and sampled for salinity and pH (Hanna instrument, HI98129). Redox potential was measured at 5 cm soil depth (Hanna instruments, HI 98120). We sampled for sediment in two of the spring tides, using a 10 cm deep, 2.5 cm diameter core; samples were oven dried for 72 h at 70°C and consequently we quantified: sediment moisture content, bulk density, and organic matter content (loss on ignition, 18 h at 440°C) (Feagin et al., 2009). Combusted sediments were sieved to separate the clay-silt fraction (<53 μm), fine sand (53–250 μm), coarse sand (250–1,000 μm), and very coarse sand (>1,000 μm) (Denef et al., 2001).
Statistical Analysis
The core erosion data was described by a mixed effects model (Bates, 2010) with time of erosion (min) both as a fixed explanatory variable and a random effect nested in core; core was a random intercept nested within marsh. This model structure allowed individual cores to vary in their initial mass and erosion rate; it also accounted for the hierarchical nature of the sampling. The response variable (loss of core mass) was log-transformed to account for the non-linear decrease in erosion over time (see example in Figure A2). After fitting the models (one for each species), we extracted the slopes for each core and we used these slopes as a metric of sediment stability (loss of mass/unit of time).
First, a set of a priori mixed-effect models (full models: Table A2) were used to identify root traits that affected sediment stability. Models included parameters for sediment grain size (e.g., sand) and root character (e.g., RLD) because previous studies showed their importance for sediment stabilisation (Ford et al., 2016; Lo et al., 2017; Wang et al., 2017) and marsh as a random factor. Models were ranked with the corrected Akaike Information Criteria (AICc; Akaike, 1973; Burnham et al., 2011) using the MuMIn R package (Barton, 2016). Second, we designed a set of a priori mixed-effect models (Table A3) using RD, the trait selected in the best model from the previous analysis, to understand which root compartment (Rhizome, Coarse roots, and Fine roots) was more important for sediment stability. Because these models were based on the best model selected in the first part of the analysis, results from this model selection has to be considered more exploratory. Third, a priori mixed-effect models (Table A4) were used to understand the effect of the physical environment on the expression of RD, which was the best-model root trait identified in step 1 for both species. As abiotic predictors we included four well-known stressors for salt marsh plants: sand content in the sediment, sediment redox potential, pH, and salinity. Models were designed on expected effects of abiotic variables. We standardised abiotic variables to zero mean and unit variance and fitted these variables as fixed factors and marsh as a random factor. Models were again ranked with AICc and the explanatory power of the best model was evaluated comparing the marginal R2 (hereafter, mR2) with the conditional R2 (hereafter, cR2). Where necessary, we log transformed the response variable to meet the model assumptions. Quadratic terms were included in candidate models to provide a general and flexible approximation of possible non-linear relationships. Because of great differences in sediment characteristics between the two species (Figure A1), we decided to split the analysis. Plots were generated with the visreg package (Breheny and Burchett, 2013). All the analyses were carried out in R (R core team 2015).
Results
Effect of Root Traits and Sediment Grain Size on Core Erosion
The erosion trial was able to account for a high portion of variability in erosion rates in both Spartina and Atriplex (respectively, cR2: 0.96 and 0.99).
We first examined the role of root traits, alongside sediment properties, in explaining sediment stability. For both species, the best model included sand content and a quadratic effect of root density (RD) (Table 1) (Table A5). In Spartina, increasing sand content significantly reduced sediment stability (Table 1, Figure 2A), while RD had a stabilising, though non-linear, effect (Table 1, Figure 2B; model: mR2 = 0.72, cR2 = 0.72). In Atriplex, neither sand content nor RD had significant effects on sediment stability (Table 1, Figures 2C,D), consistent with the low explanatory ability of the fixed effects in this model (mR2 = 0.18, cR2 = 0.63). Beyond RD, there was no support for a role of other root traits (e.g., SRLt) in determining sediment stability in either species (Table A5).
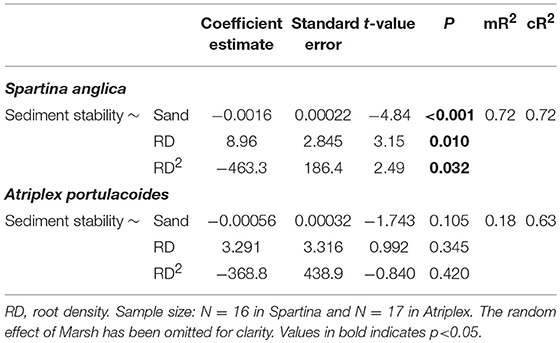
Table 1. Summary results of mixed-effect models of the effect of sand content and root density (RD) on sediment stability for Spartina and Atriplex.
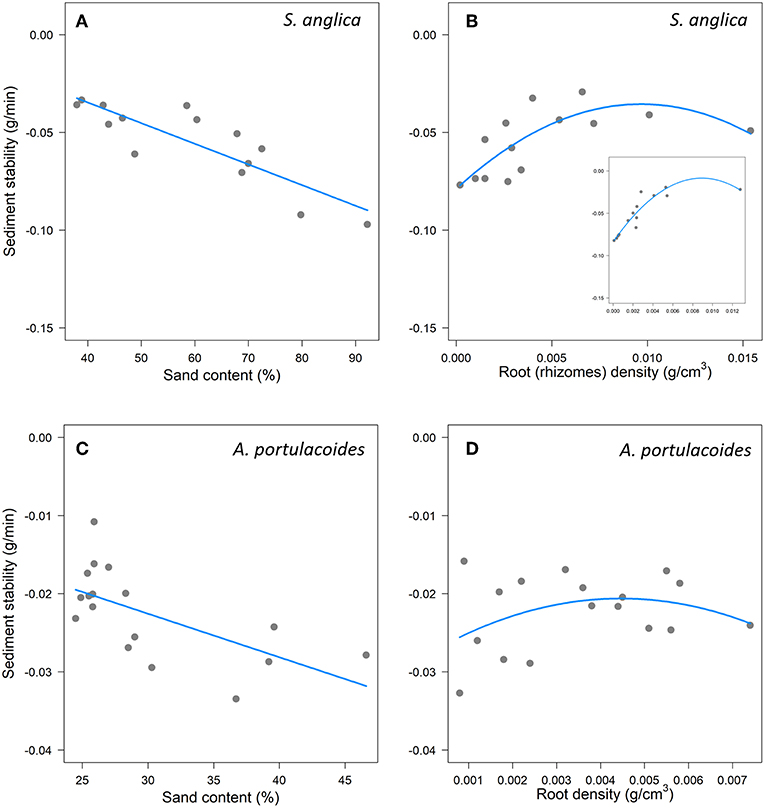
Figure 2. Effects of sand content and root density on marsh resistance to erosion (sediment stability represents a change in the slope of sediment loss; more negative values indicates greater sediment loss,) in experimental erosion cores from Spartina (A,B) and Atriplex (C,D) marshes. In (B), the insert represents marsh resistance to erosion in experimental erosion cores from Spartina when only rhizomes are considered. In (A,C) points indicate partial residuals when root density (RD) was held constant (median). In (B,D), and the insert in (B), points indicate partial residuals when Sand was held constant (median).
We next examined the contributions of different root compartments to sediment stability. In Spartina, the best model included sand content and non-linear effects of both rhizomes and coarse roots (mR2 = 0.79, cR2 = 0.79; Figure 2B, Table A6). This model revealed significant effects of sand content and rhizomes, but not of coarse roots (Table 2). The same analysis for Atriplex showed that the two best, similarly ranked, models had low explanatory ability and none of the parameters included in these models had significant effects on sediment stability (Table 2, Table A7).
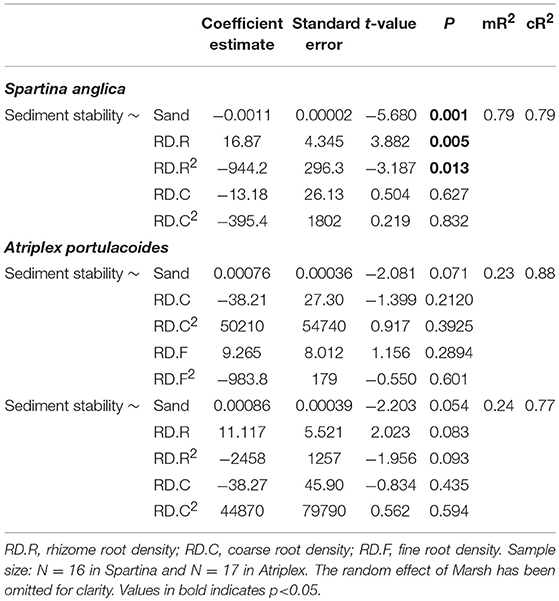
Table 2. Summary results of mixed-effect models of the effect of sand and root density (RD) on sediment stability for Spartina and Atriplex.
Effect of the Environment on Root Density
Since RD was the only trait included in the best models explaining sediment stability, we investigated the effect of environmental factors on this trait (Note that correlations between RD and other root traits are reported in Figure A3). Redox potential and sand content were the main abiotic factors that affected RD, with both retained in the best models, although there were again differences between species (Tables A8, A9). In Spartina, there was no significant effect of sand content (Table 3, Figure 3B), while increasing redox values were significantly associated with increased RD (Table 3, Figure 3A). In Atriplex, sand content had a significant negative effect, while redox had a non-linear, quadratic, effect (Table 3, Figures 3C,D). In the upper half of the redox range, RD increased with increasing redox; in the lower half of the redox range RD appeared to decrease with increasing redox. However, the scarcity of samples calls for a cautious interpretation of the lower half of the relationship. In both species the marginal R2 was relatively low with respect to the conditional R2 (Tables A8, A9), indicating that other factors that vary among marshes are likely to be important for explaining RD variability.
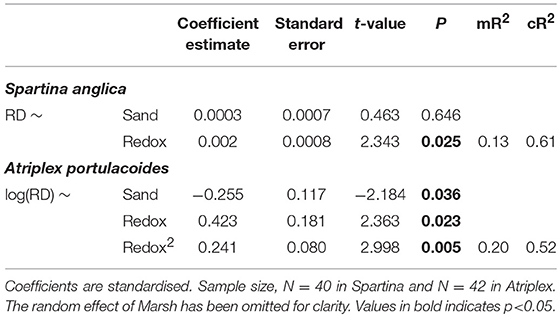
Table 3. Summary table of linear mixed-effect models of the effect of Sand and Redox on Root density (RD) for both Spartina and Atriplex.
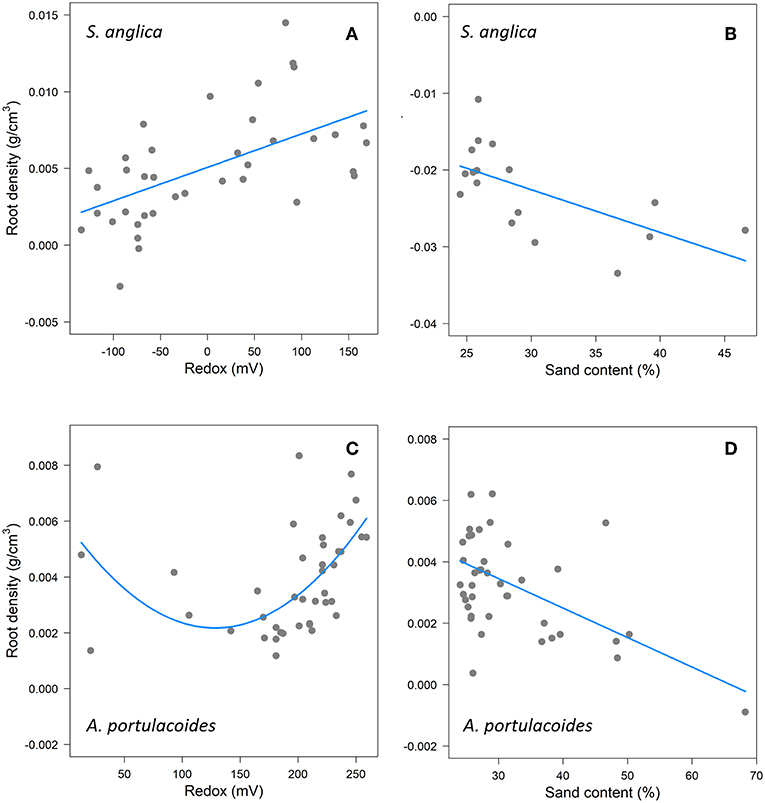
Figure 3. The effects of Redox (left panels) and Sand (right panels) on root density in Spartina (A,B) and Atriplex (C,D). In Atriplex the response variable RD has been log transformed to meet model assumptions, but (C,D) show non-transformed data to allow better comparison between species. Points indicate partial residuals when other abiotic variables are held constant.
Discussion
Our results show that: (i) plant roots increased sediment stability (reduced erosion), particularly in the Spartina zone; (ii) root density (RD) and the fraction of coarse roots/rhizomes—rather than the proportion of fine roots or associated traits, as hypothesized—were responsible for enhanced stability in the Spartina zone; and (iii) root density was greater in sediment with higher redox potential (both species) and was either lower (Atriplex) or unaffected (Spartina) in sediment with higher sand content. Collectively, these results deepen our understanding of the consequences and drivers of variability in belowground traits of salt marsh plants.
Effect of Root Traits on Sediment Stability
Salt marsh lateral erosion is a complex phenomenon regulated by different mechanisms. Marsh lateral erosion depends both on blocks failure, where wave action and water pressure lead to cracks in the sediment and/or subsequent fall of entire marsh blocks (Francalanci et al., 2013; Bendoni et al., 2016), and loss of sediment by sediment erosion, where sediment particles detach from the marsh under wave and water flow action (Bouma et al., 2002, 2009, 2010). At the local scale, field and mesocosm experiments showed that sediment particle erosion well-correlated with lateral marsh retreat and that root biomass played a key role (Wang et al., 2017). Our study strengthens this case and shows that plant roots can increase sediment stability, contributing to reduction in lateral erosion in salt marshes. In Spartina, where evidence of a positive effect of RD was stronger, the non-linear relationship between RD and erosion indicates that small changes in this root trait greatly increase sediment stability until a plateau is reached. This is in accordance with flume studies in terrestrial systems, where roots maximally reduced soil detachment rate at similar values of RD (De Baets et al., 2006; Baets et al., 2007). Interestingly, terrestrial studies look at top soil instead of lateral erosion (e.g., De Baets et al., 2006). Thus, considering that similar RD values lead to comparable erosion reduction in our and their study, suggests that RD effect on sediment erosion is a general mechanism regardless of the flow direction. Spartina is a species wide spread worldwide (Adam, 2002) at the edge of the marsh, thus, the stabilising effect of RD in this species further confirms the importance of roots for sediment stabilisation in salt marshes demonstrated recently at intraspecific (Lo et al., 2017), species (Wang et al., 2017) and community (Ford et al., 2016) levels.
Yet, the lack of strong evidence of a sediment stabilising effect of roots in the Atriplex zone underlines the context dependency of these processes. Sediment composition might be an important factor explaining this result; when sand content is relatively low, as in the Atriplex zone, roots might play a weaker role for sediment stabilisation and sediment cohesiveness is more important (Schutten et al., 2005; Feagin et al., 2009). Indeed, previous studies also showed that root biomass better explained core erosion rates when sand content in the sediment was high (Ford et al., 2016; Lo et al., 2017). In our study, divergent root architecture of the two-focal species (fibrous, rhizomatous root system in Spartina vs. tap root system in Atriplex) may have also contributed to the differences in root effects on sediment stability. Finally, it is possible that cores with low sand content (Atriplex) needed a longer period of erosion to show statistically detectable effects of both sand content and RD. More studies are required to fully elucidate the role of roots in sediment stabilisation in salt marshes across diverse sediment types and plant rooting architectures.
Our results further suggest that sediment stability in the sandy Spartina zone is mainly determined by coarse roots and rhizomes, rather than by fine roots, as argued in terrestrial studies (De Baets et al., 2006; Burylo et al., 2012). In our study, the primary role of coarse roots/rhizomes is suggested by: (i) RD, the trait that we found drove sediment stability, is mainly determined by these compartments; and (ii) rhizomes and coarse roots best explained erosion rates, while fine roots were consistently not included among predictors for sediment stabilisation. Sand content in the Spartina zone reached levels (up to 90%) considerably greater than in analogous terrestrial studies (~50%: Vannoppen et al., 2017). Thus, it is possible that coarser roots become more important for sediment stabilisation in environments with high sand content. However, because model selection of the best root compartments involved in sediment stabilisation was more an exploratory analysis and because of methodological differences in defining root classes between our and terrestrial studies, we cannot generalise these results. In our study the root diameter across the entire root system ranged from 0.5 to 3 mm (rhizomes included), which would be considered either as fine roots (Baets et al., 2007) or coarse roots (Burylo et al., 2012) depending on the terrestrial study considered. Future studies should include a wider sand content gradient and range of root diameters to further elucidate the mechanisms involved in sediment stabilisation (e.g., fine vs. coarse roots) thus allowing reconciliation of the apparent discrepancy between salt marshes and terrestrial systems.
Effect of the Environment on Root Traits and Sediment Stability
Across the two species, root density showed similarities and differences in its responses to environmental factors, and thus the potential for indirect effect of abiotic factors on sediment stabilisation. First, RD in both species appeared invariant to salinity. This indicates that, while high salinities are known to suppress biomass production in salt marsh plants (Cooper, 1982; Crain et al., 2004; Flowers and Colmer, 2008), these dominant, halophytic, salt marsh plants are able to sustain RD, and therefore associated sediment stabilisation, across sites spanning a range of salinities in our study system. Second, notwithstanding the non-linear pattern in Atriplex, both species showed evidence that declining redox, a proxy for low oxygen in the sediment, could suppress RD. This can probably be explained by the metabolic costs associated with mechanisms to cope with low redox (Armstrong, 1979), reviewed in Colmer (2003) and Nishiuchi et al. (2012). While release of oxygen from plant roots (Pezeshki, 2001) may have contributed to the observed relationships, we assume the direction of causality to flow from the abiotic environment to RD given previous experimental evidence in salt marsh plants that: (i) waterlogging can directly reduce growth of salt marsh plants (Cooper, 1982; Bouma et al., 2001a); and (ii) the impact of oxygen release from roots on sediment oxygenation is limited (Koop-Jakobsen et al., 2018). Therefore, factors that influence sediment redox potential, including bioturbation, tidal inundation (and sea-level rise) and livestock grazing, may indirectly affect the stability of salt marsh sediments by altering RD. Third, the species differed in their responses to sand content, and thus nutrient availability. The resistance of RD of Spartina to high sand content might be explained by its ability to acquire resources directly from the water column (Bouma et al., 2002), or a greater capacity for compensatory investment in belowground biomass under low soil nutrients, a mechanism known for terrestrial plants (Freschet et al., 2015). Spartina therefore sustains an important erosion buffering function even where sand content, and thus the erosion vulnerability of the marsh platform, is at its highest. Indeed, the sandier sites at the mouth of the estuaries (Figure A1) did not erode more quickly than those at the heads. Finally, although we investigated a suite of well-known stressors for plant growth (redox, salinity, sand, and pH), in both species the modest portion of variability accounted for by the best models suggest that other factors may drive RD. For instance, variation in wave exposure that exists within and between marshes might affect plants' investment in roots (Coops et al., 1996). Further developing our understanding of the belowground responses of salt marsh plants to environmental factors will be an important task if the future vulnerability of salt marshes to lateral erosion under climate change are to be predicted.
Global Significance and Limitations
Spartina is a pioneer species with a cosmopolitan global distribution (Adam, 2002), thus results of our study highlight the importance of vegetation for reducing lateral erosion in salt marshes. We showed here that marshes with higher sand content in the sediment erode faster, but RD can effectively counteract this negative effect of sand content. Interestingly, despite the differences found here between Spartina and Atriplex, we showed that RD is a good predictor for sediment stability. Thus, the relatively easy investigation of sediment granulometry and RD among marshes could allow managers to map marshes vulnerability to later erosion. These maps, could also be employed in management schemes for coastal protection and for understanding how climate change would impact marsh survival in the long term. Yet, more studies are need to expand our results to wider abiotic gradients and type of marshes, such as barrier island marshes, microtidal marshes, or marsh zones with mixed vegetation communities. Moreover, we stress here that our study extrapolates from a flume experiment, but marsh lateral erosion is a complex phenomenon. Several factors contribute to marsh lateral erosion, with wind exposure and foreshore morphology acting at large and intermediate scales, respectively (Wang et al., 2017). Furthermore, block marsh failure is an important mechanism of marsh retreat (Francalanci et al., 2013; Bendoni et al., 2016), which was beyond the scope of investigation of our study. Although plant roots can play a crucial role in reducing block failure (Bendoni et al., 2016), the role of root density in this regard is yet to be investigated. Overall, future studies should aim at understanding how sediment stabilisation by roots relate to other aspects of marsh erosion (e.g., block failure).
Conclusion
This study shows roots of saltmarsh plants effectively stabilise sediments against erosion, but that root development varies with environmental context, thus generating spatial variation in erosion protection by plants. By addressing both the response of roots to the environment, and, in turn, the effect of roots on sediment stability (“response-effect” approach), we revealed the important role that intraspecific variability plays in marsh resistance to erosion and that environmental factors can propagate through plant traits to influence salt marsh stability. Surprisingly, we found scarce evidence that fine roots—or associated traits—played an important role in sediment stabilisation. Instead, overall root density, and especially the biomass of rhizomes and coarse roots, drove sediment stability. This suggests that different mechanisms of root-sediment stabilisation might exist depending on sand content, and that, in salt marshes, root density can efficiently capture the role of salt marsh plants for sediment stabilisation. More studies are warranted to elucidate the indirect effect of the environment on salt marsh root traits enabling researchers to better forecast salt marsh stability under future climate change and to inform managers on the effective integration of salt marshes into coastal defence schemes.
Author Contributions
DDB and JG developed the idea and led the writing of the manuscript. DDB performed field work, laboratory work, and data analysis. JG, MF, and MR contributed to data analysis. MR contributed to field and laboratory work. MF, SJ, MS, TB, and PN contributed to write the manuscript. All authors contributed to manuscript revision, read, and approved the submitted version.
Funding
This work was provided by Welsh Government and HEFCW through the 384 Sêr Cymru National Research Network for Low Carbon, Energy and Environment RESILCOAST project. JG was supported by Natural Environment Research Council award NE/N013573/1, CoastWEB: Valuing the contribution which COASTal habitats make to human health and WEllBeing, with a focus on the alleviation of natural hazards.
Conflict of Interest Statement
The authors declare that the research was conducted in the absence of any commercial or financial relationships that could be construed as a potential conflict of interest.
The handling editor and author, MS, declared their involvement as co-editors in the Research Topic, and confirm the absence of any other ongoing collaboration.
Acknowledgments
We would like to thank The Wildfowl & Wetlands Trust for access to the Penrhyn Gwyn marsh, Mr. C. Delany for access to marshes on the Taf river, and Mr. D.A. Roberts for access to the Loughor marsh, and Natural Resources Wales for providing authorisation for sampling. We also thank Tom Fairchild, Kate Davidson, Alizée Mauffrey, Laura Cappelatti, Josh Mutter, Olivia Smith, Ella Milne, Gemma Woodhouse, Livius C. Bozga, and Macarius Howard for practical assistance.
Supplementary Material
The Supplementary Material for this article can be found online at: https://www.frontiersin.org/articles/10.3389/fevo.2019.00150/full#supplementary-material
References
Adam, P. (2002). Saltmarshes in a time of change. Environ. Conserv. 29, 39–61. doi: 10.1017/S0376892902000048
Akaike, H. (1973). “Information Theory and an Extension of the Maximum Likelihood Principle,” in Proceedings of the 2nd International Symposium on Information Theory,” eds B. N. Petrov and F. Csaki (Budapest: Akademiai Kiado), 267–281.
Armstrong, W. (2000). Oxygen distribution in wetland plant roots and permeability barriers to gas-exchange with the rhizosphere: a microelectrode and modelling study with Phragmites australis. Ann. Bot. 86, 687–703. doi: 10.1006/anbo.2000.1236
Armstrong, W., Wright, E. J., Lythe, S., and Gaynard, T. J. (1985). Plant zonation and the effects of the spring-neap tidal cycle on soil aeration in a humber salt marsh. J. Ecol. 73:323. doi: 10.2307/2259786
Baets, S. D., Poesen, J., Knapen, A., and Galindo, P. (2007). Impact of root architecture on the erosion-reducing potential of roots during concentrated flow. Earth Surface Processes Landforms 32, 1323–1345. doi: 10.1002/esp.1470
Barbier, E. B., Koch, E. W., Silliman, B. R., Hacker, S. D., Wolanski, E., and Primavera, J., et al. (2008). Coastal ecosystem-based management with nonlinear ecological functions and values. Science 319, 321–323. doi: 10.1126/science.1150349
Bardgett, R. D., and van der Putten, W. H. (2014). Belowground biodiversity and ecosystem functioning. Nature 515, 505–511. doi: 10.1038/nature13855
Barton, K. (2016). MuMIn: Multi-Model Inference. R package version 1.15.6. Available online at: http://CRAN.R-project.org/package=MuMIn
Bendoni, M., Mel, R., Solari, L., Lanzoni, S., Francalanci, S., and Oumeraci, H. (2016). Insights into lateral marsh retreat mechanism through localized field measurements. Water Resour. Res. 52, 1446–1464. doi: 10.1002/2015WR017966
Bouma, T., Stapel, J., van der Heiden, J., Koutstaal, B., van Soelen, J., and van IJzerloo, L. (2002). Relative importance of macrophyte leaves for nitrogen uptake from flood water in tidal salt marshes. Marine Ecol. Progr. Ser. 240, 93–104. doi: 10.3354/meps240093
Bouma, T. J., Friedrichs, M., van Wesenbeeck, B. K., Temmerman, S., Graf, G., and Herman, P. M. J. (2009). Density-dependent linkage of scale-dependent feedbacks: a flume study on the intertidal macrophyte Spartina anglica. Oikos 118, 260–268. doi: 10.1111/j.1600-0706.2008.16892.x
Bouma, T. J., Koutstaal, B. P., van Dongen, M., and Nielsen, K. L. (2001b). Coping with low nutrient availability and inundation: root growth responses of three halophytic grass species from different elevations along a flooding gradient. Oecologia 126, 472–481. doi: 10.1007/s004420000545
Bouma, T. J., Nielsen, K. L., Van Hal, J., and Koutstaal, B. (2001a). Root system topology and diameter distribution of species from habitats differing in inundation frequency. Functi. Ecol. 15, 360–369. doi: 10.1046/j.1365-2435.2001.00523.x
Bouma, T. J., van Belzen, J., Balke, T., Zhu, Z., Airoldi, L., and Blight, A. J., et al. (2014). Identifying knowledge gaps hampering application of intertidal habitats in coastal protection: opportunities & steps to take. Coastal Eng. 87, 147–157. doi: 10.1016/j.coastaleng.2013.11.014
Bouma, T. J., Vries, M. D., and Herman, P. M. J. (2010). Comparing ecosystem engineering efficiency of two plant species with contrasting growth strategies. Ecology 91, 2696–2704. doi: 10.1890/09-0690.1
Breheny, P., and Burchett, W. (2013). Visualization of Regression Models Using Visreg. R Package. University of Kentucky.
Burnham, K. P., Anderson, D. R., and Huyvaert, K. P. (2011). AIC model selection and multimodel inference in behavioral ecology: some background, observations, and comparisons. Behav. Ecol. Sociobiol. 65, 23–35. doi: 10.1007/s00265-010-1029-6
Burylo, M., Rey, F., Mathys, N., and Dutoit, T. (2012). Plant root traits affecting the resistance of soils to concentrated flow erosion: plant root traits and soil stability. Earth Surface Processes Landforms 37, 1463–1470. doi: 10.1002/esp.3248
Colmer, T. D. (2003). Long-distance transport of gases in plants: a perspective on internal aeration and radial oxygen loss from roots. Plant Cell Environ. 26, 17–36. doi: 10.1046/j.1365-3040.2003.00846.x
Colmer, T. D., and Flowers, T. J. (2008). Flooding tolerance in halophytes. New Phytol. 179, 964–974. doi: 10.1111/j.1469-8137.2008.02483.x
Cooper, A. (1982). The effects of salinity and waterlogging on the growth and cation uptake of salt marsh plants. New Phytol. 90, 263–275.
Coops, H., Geilen, N., Verheij, H. J., Boeters, R., and van der Velde, G. (1996). Interactions between waves, bank erosion and emergent vegetation: an experimental study in a wave tank. Aquat. Bot. 53, 187–198. doi: 10.1016/0304-3770(96)01027-3
Costanza, R., Pérez-Maqueo, O., Martinez, M. L., Sutton, P., Anderson, S. J., and Mulder, K. (2008). The value of coastal wetlands for hurricane protection. J. Human Environ. 37, 241–248. doi: 10.1579/0044-7447(2008)37[241:TVOCWF]2.0.CO;2
Crain, C. M., Silliman, B. R., Bertness, S. L., and Bertness, M. D. (2004). Physical and biotic drivers of plant distribution across estuarine salinity gradients. Ecology 85, 2539–2549. doi: 10.1890/03-0745
De Baets, S., Poesen, J., Gyssels, G., and Knapen, A. (2006). Effects of grass roots on the erodibility of topsoils during concentrated flow. Geomorphology 76, 54–67. doi: 10.1016/j.geomorph.2005.10.002
Deegan, L. A., Johnson, D. S., Warren, R. S., Peterson, B. J., Fleeger, J. W., and Fagherazzi, S., et al. (2012). Coastal eutrophication as a driver of salt marsh loss. Nature 490, 388–392. doi: 10.1038/nature11533
Denef, K., Six, J., Bossuyt, H., Frey, S. D., Elliott, E. T., and Merckx, R., et al. (2001). Influence of dry–wet cycles on the interrelationship between aggregate, particulate organic matter, and microbial community dynamics. Soil Biol. Biochem. 33, 1599–1611. doi: 10.1016/s0038-0717(01)00076-1
Fagherazzi, S., Mariotti, G., Wiberg, P., and McGlathery, K. (2013). Marsh collapse does not require sea level rise. Oceanography 26, 70–77. doi: 10.5670/oceanog.2013.47
Feagin, R. A., Lozada-Bernard, S. M., Ravens, T. M., Möller, I., Yeager, K. M., and Baird, A. H. (2009). Does vegetation prevent wave erosion of salt marsh edges? Proc. Nat. Acad. Sci. 106, 10109–10113. doi: 10.1073/pnas.0901297106
Feagin, R. A., Mukherjee, N., Shanker, K., Baird, A. H., Cinner, J., and Kerr, A. M., et al. (2010). Shelter from the storm? Use and misuse of coastal vegetation bioshields for managing natural disasters. Conserv. Lett. 3, 1–11. doi: 10.1111/j.1755-263X.2009.00087.x
Flowers, T. J., and Colmer, T. D. (2008). Salinity tolerance in halophytes. New Phytol. 179, 945–963. doi: 10.1111/j.1469-8137.2008.02531.x
Ford, H., Garbutt, A., Ladd, C., Malarkey, J., and Skov, M. W. (2016). Soil stabilization linked to plant diversity and environmental context in coastal wetlands. J. Veget. Sci. 27, 259–268. doi: 10.1111/jvs.12367
Foster, N. M., Hudson, M. D., Bray, S., and Nicholls, R. J. (2013). Intertidal mudflat and saltmarsh conservation and sustainable use in the UK: a review. J. Environ. Manag. 126, 96–104. doi: 10.1016/j.jenvman.2013.04.015
Fourcaud, T., Ji, J.-N., Zhang, Z.-Q., and Stokes, A. (2008). Understanding the impact of root morphology on overturning mechanisms: a modelling approach. Ann. Bot. 101, 1267–1280. doi: 10.1093/aob/mcm245
Francalanci, S., Bendoni, M., Rinaldi, M., and Solari, L. (2013). Ecomorphodynamic evolution of salt marshes: experimental observations of bank retreat processes. Geomorphology 195, 53–65. doi: 10.1016/j.geomorph.2013.04.026
Freschet, G. T., and Roumet, C. (2017). Sampling roots to capture plant and soil functions. Funct. Ecol. 31, 1506–1518. doi: 10.1111/1365-2435.12883
Freschet, G. T., Swart, E. M., and Cornelissen, J. H. C. (2015). Integrated plant phenotypic responses to contrasting above- and below-ground resources: key roles of specific leaf area and root mass fraction. New Phytol. 206, 1247–1260. doi: 10.1111/nph.13352
Freschet, G. T., Valverde-Barrantes, O. J., Tucker, C. M., Craine, J. M., McCormack, M. L., and Violle, C., et al. (2017). Climate, soil and plant functional types as drivers of global fine-root trait variation. J. Ecol. 105, 1182–1196. doi: 10.1111/1365-2745.12769
Koop-Jakobsen, K., Mueller, P., Meier, R. J., Liebsch, G., and Jensen, K. (2018). Plant-sediment interactions in salt marshes—an optode imaging study of O2, pH, and CO2 gradients in the rhizosphere. Front. Plant Sci. 9:e541. doi: 10.3389/fpls.2018.00541
Lavorel, S., and Garnier, E. (2002). Predicting changes in community composition and ecosystem functioning from plant traits: revisiting the Holy Grail. Funct. Ecol. 16, 545–556. doi: 10.1046/j.1365-2435.2002.00664.x
Lavorel, S., Storkey, J., Bardgett, R. D., de Bello, F., Berg, M. P., and Le Roux, X., et al. (2013). A novel framework for linking functional diversity of plants with other trophic levels for the quantification of ecosystem services. J. Veget. Sci. 24, 942–948. doi: 10.1111/jvs.12083
Leonardi, N., Ganju, N. K., and Fagherazzi, S. (2016). A linear relationship between wave power and erosion determines salt-marsh resilience to violent storms and hurricanes. Proc. Natl. Acad. Sci. U.S.A. 113, 64–68. doi: 10.1073/pnas.1510095112
Lo, V. B., Bouma, T. J., van Belzen, J., Van Colen, C., and Airoldi, L. (2017). Interactive effects of vegetation and sediment properties on erosion of salt marshes in the Northern Adriatic Sea. Marine Environ. Res. 131, 32–42. doi: 10.1016/j.marenvres.2017.09.006
Marani, M., D'Alpaos, A., Lanzoni, S., and Santalucia, M. (2011). Understanding and predicting wave erosion of marsh edges. Geophys. Res. Lett. 38:48995. doi: 10.1029/2011GL048995
Mariotti, G., and Fagherazzi, S. (2010). A numerical model for the coupled long-term evolution of salt marshes and tidal flats. J. Geophys. Res. Earth Surface 115:1326. doi: 10.1029/2009JF001326
Möller, I., Kudella, M., Rupprecht, F., Spencer, T., Paul, M., and van Wesenbeeck, B. K., et al. (2014). Wave attenuation over coastal salt marshes under storm surge conditions. Nat. Geosci. 7, 727–731. doi: 10.1038/ngeo2251
Möller, I., and Spencer, T. (2002). Wave dissipation over macro-tidal saltmarshes: effects of marsh edge typology and vegetation change. J. Coast. Res. 36, 506–521. doi: 10.2112/1551-5036-36.sp1.506
Naidoo, G., McKee, K. L., and Mendelssohn, I. A. (1992). Anatomical and metabolic responses to waterlogging and salinity in Spartina alterniflora and S. patens (Poaceae). Am. J. Bot. 79:765. doi: 10.2307/2444942
Nelson, J. L., and Zavaleta, E. S. (2012). Salt marsh as a coastal filter for the oceans: changes in function with experimental increases in nitrogen loading and sea-level rise. PLoS ONE 7:e38558. doi: 10.1371/journal.pone.0038558
Nishiuchi, S., Yamauchi, T., Takahashi, H., Kotula, L., and Nakazono, M. (2012). Mechanisms for coping with submergence and waterlogging in rice. Rice 5:2. doi: 10.1186/1939-8433-5-2
Olff, H., Leeuw, J. D., Bakker, J. P., Platerink, R. J., and van Wijnen, H. J. (1997). Vegetation succession and herbivory in a salt marsh: changes induced by sea level rise and silt deposition along an elevational gradient. J. Ecol. 85:799. doi: 10.2307/2960603
Pérez-Harguindeguy, N., Díaz, S., Garnier, E., Lavorel, S., Poorter, H., and Jaureguiberry, P., et al. (2013). New handbook for standardised measurement of plant functional traits worldwide. Aust. J. Bot. 61:167. doi: 10.1071/BT12225
Pezeshki, S. R. (2001). Wetland plant responses to soil flooding. Environ. Exp. Bot. 46, 299–312. doi: 10.1016/s0098-8472(01)00107-1
Pound, M. P., French, A. P., Atkinson, J. A., Wells, D. M., Bennett, M. J., and Pridmore, T. (2013). RootNav: navigating images of complex root architectures. Plant Physiol. 162, 1802–1814. doi: 10.1104/pp.113.221531
Rodwell, J. S., Dring, J. C., Averis, A. B. G., Proctor, M. C. F., Malloch, A. J. C., and Schaminée, J. N. J., et al. (2000). Review of coverage of the National Vegetation Classification JNCC Report, No. 302.
Schindelin, J., Arganda-Carreras, I., Frise, E., Kaynig, V., Longair, M., and Pietzsch, T., et al. (2012). Fiji: an open-source platform for biological-image analysis. Nat. Methods 9, 676–682. doi: 10.1038/nmeth.2019
Schutten, J., Dainty, J., and Davy, A. J. (2005). Root anchorage and its significance for submerged plants in shallow lakes. J. Ecol. 93, 556–571. doi: 10.1111/j.1365-2745.2005.00980.x
Shepard, C. C., Crain, C. M., and Beck, M. W. (2011). The protective role of coastal marshes: a systematic review and meta-analysis. PLoS ONE 6:e27374. doi: 10.1371/journal.pone.0027374
Suding, K. N., Lavorel, S., Chapin, F. S., Cornelissen, J. H. C., DíAz, S., and Garnier, E., et al. (2008). Scaling environmental change through the community-level: a trait-based response-and-effect framework for plants. Global Change Biol. 14, 1125–1140. doi: 10.1111/j.1365-2486.2008.01557.x
Tabot, P. T., and Adams, J. B. (2014). Salt secretion, proline accumulation and increased branching confer tolerance to drought and salinity in the endemic halophyte Limonium linifolium. South Afr. J. Bot. 94, 64–73. doi: 10.1016/j.sajb.2014.05.009
Tyler, A., and Zieman, J. (1999). Patterns of development in the creekbank region of a barrier island Spartina alterniflora marsh. Marine Ecol. Progr. Ser. 180, 161–177. doi: 10.3354/meps180161
Vannoppen, W., De Baets, S., Keeble, J., Dong, Y., and Poesen, J. (2017). How do root and soil characteristics affect the erosion-reducing potential of plant species? Ecol. Eng. 109, 186–195. doi: 10.1016/j.ecoleng.2017.08.001
Wang, H., Wal, D., van der, Li, X., Belzen, J., van Herman, P. M. J., and Hu, Z., et al. (2017). Zooming in and out: scale dependence of extrinsic and intrinsic factors affecting salt marsh erosion. J. Geophys. Res. Earth Surface 122, 1455–1470. doi: 10.1002/2016JF004193
Keywords: resilience, sediment stability, response-effect framework, functional root traits, environmental gradients, root density
Citation: De Battisti D, Fowler MS, Jenkins SR, Skov MW, Rossi M, Bouma TJ, Neyland PJ and Griffin JN (2019) Intraspecific Root Trait Variability Along Environmental Gradients Affects Salt Marsh Resistance to Lateral Erosion. Front. Ecol. Evol. 7:150. doi: 10.3389/fevo.2019.00150
Received: 27 September 2018; Accepted: 16 April 2019;
Published: 08 May 2019.
Edited by:
Alberto Vieira Borges, University of Liège, BelgiumReviewed by:
Guillaume Cyril Goodwin, University of Edinburgh, United KingdomVishnu Prahalad, University of Tasmania, Australia
Copyright © 2019 De Battisti, Fowler, Jenkins, Skov, Rossi, Bouma, Neyland and Griffin. This is an open-access article distributed under the terms of the Creative Commons Attribution License (CC BY). The use, distribution or reproduction in other forums is permitted, provided the original author(s) and the copyright owner(s) are credited and that the original publication in this journal is cited, in accordance with accepted academic practice. No use, distribution or reproduction is permitted which does not comply with these terms.
*Correspondence: Davide De Battisti, d.de-battisti.844312@swansea.ac.uk; davide.de85@gmail.com