- 1Department of Biology, University of Turku, Turku, Finland
- 2Arctic Centre, University of Lapland, Rovaniemi, Finland
Urbanization is negatively affecting biodiversity worldwide, and general ecological patterns may also differ between urban and more natural areas. The main aim of this study was to examine if urbanization has effects on the wintering species occupancy frequency distribution (SOFD) and species abundance–occupancy relationship (SAOR), and if the observed patterns varied between winters, different sizes of towns, and regionally in Finland. In this study, temporal variation of the SOFD and SAOR patterns was studied in 29 town and village centers along a 950-km (60–68°N) latitudinal gradient during mid-winters in Finland. Wintering birds were counted during three winters (1991–1992, 1999–2000, and 2009–2010) from the same study sites and with the same survey methods. A total of 35 wintering bird species and 13,285 individuals were detected. The bimodal symmetric SOFD pattern explained best the distribution of species in the pooled data, and the observed pattern was constant between the study winters, different sizes of towns, and towns located in southern and northern Finland. Three species (Parus major, Pica pica, and Passer domesticus) were core species during all winters, irrespective of the size of town or latitude. There was a slightly higher number of species belonging in satellite species group in the southern than in the northern towns. No changes of species from the core to satellite species were detected, and vice versa. However, the occupancy rate of some species belonging to the intermediate species group either moved toward satellite species or core species across winters. The SAOR pattern was positive and stable over study winters and did not differ between different sizes of towns or town location. Our results indicated that urbanization leads a structure of winter bird community, where there are few widely distributed sedentary core species and many partially migratory or migratory satellite species with a restricted distribution. Our results also demonstrated that urbanization stabilizes between-winter community structure, probably because of intensive winter feeding activities. Our results give support to the metapopulation model, which predicts a bimodal SOFD pattern.
Introduction
Both the number of people living in cities and the area covered by urban areas are increasing worldwide (United Nations, 2015). At the same time, these novel environments present many challenges to the biota via their heterogenic landscape structure, changed climate, frequent human-caused disturbances, and anthropogenic food resources (Adams, 2016). Correspondingly, urbanization might change animal community patterns observed in more natural areas (Francis and Chadwick, 2013; Adams, 2016). For example, opposite to natural communities, winter bird richness does not necessarily decrease with urbanization and toward to the north in heavily urbanized areas (Jokimäki et al., 1996). In addition, bird species composition of urban assemblages is almost similar between southern and northern Finland (Jokimäki et al., 1996).
The spatial variation of urban bird communities is quite well understood (e.g., Marzluff et al., 2001; Chace and Walsh, 2006; Bellocq et al., 2017; Fidino and Magle, 2017). However, temporal variation of the wintering bird assemblages is much less studied. Previous studies have detected only a slight variation in bird species richness and total number of individuals between winters, suggesting that urbanization might stabilize temporal variation of urban bird assemblages (Suhonen et al., 2009; Jokimäki and Kaisanlahti-Jokimäki, 2012; Ciach and Fröhlich, 2017). It has been suggested that urban attributes, such as winter feeding of birds, roads, light, and buildings, will stabilize temporal variation of wintering bird assemblage within city (Ciach and Fröhlich, 2017). However, Ciach (2012) detected that wintering bird species diversity decreased in an urbanizing area in southern Poland during 2005–2010. Apparently, more long-term winter-time studies are needed to understand a more detailed temporal dynamic of bird assemblages, such as species occupancy frequency distribution (SOFD) and species abundance–occupancy relationship (SAOR) patterns.
The shape of the SOFD and a positive SAOR are the two most commonly studied patterns in community ecology (e.g., Gaston et al., 2000; McGeoch and Gaston, 2002; Jenkins, 2011). There are several different, but not mutually exclusive, models that have been coined to account for the SOFD (McGeoch and Gaston, 2002; Jenkins, 2011) and positive SAOR (Gaston et al., 1997b, 2000). The two most common models are the metapopulation dynamic model (MPDM; Hanski, 1982, 1999) and the niche-based model (NBM; Brown, 1984). In natural communities, most of the species occur either at a few sites (satellite species; rare species) or at many sites (core species; common species), forming a bimodal core–satellite species pattern (Hanski, 1982, 1999) or a unimodal mode with a large number of satellite species (Brown, 1984). According to the MPDM (Hanski, 1982), bimodality should result from species stochastic colonization and extinction dynamics in the local communities, in which most species are either vulnerable to extinction (rare satellite species) or relatively stable (abundant core species). According to the alternative NBM, generalist species with wide niches and tolerances of environmental conditions have high local abundances and wide distributions. In contrast, specialist species with their narrow niches and low tolerances of environmental conditions have local abundances and restricted regional distributions (Brown, 1984).
Despite that the general spatial patterns of SOFD and SAOR are quite well reported in natural habitats, only a few studies have analyzed the temporal aspects of these patterns. The results of earlier studies conducted in natural habitats suggest that the temporal variability of the SOFD and SAOR could be rather stable over years (Blackburn and Gaston, 1997; Collins and Glenn, 1997; Blackburn et al., 2006; Heatherly et al., 2007). We investigated the SOFD and SAOR patterns of urban wintering bird communities during three winters (1991–1992, 1999–2000, and 2009–2010) in small and large human settlements located in southern and northern Finland by using the same study plots and survey methods. Some previous studies have examined short-term temporal variability of urban bird assemblages (Suhonen et al., 2009; Ciach, 2012). These studies have found that the diversity of wintering bird assemblages decreased with urbanization (Ciach, 2012), stability of winter bird communities decreased from the center of the town towards the periphery of the town (Jokimäki and Kaisanlahti-Jokimäki, 2012; Leveau et al., 2015), and between-year variability of bird richness and abundance were greatest during the nonbreeding season (Leveau et al., 2015). To the best of our knowledge, this is the first attempt to undertake a joint long-term SOFD and SAOR analyses of urban bird assemblages during winter season.
In this study, we have three main questions. First, we study whether wintering birds will show either a bimodal or a unimodal satellite SOFD pattern. Because the wintering bird species pool is richer in southern than in northern Finland (Jokimäki et al., 1996; Väisänen, 2003), we expected that the relative number of satellite species will be higher in the southern than in the northern regions (Gaston et al., 1998; McGeoch and Gaston, 2002; Korkeamäki et al., 2018, but see Jokimäki et al., 2016). Second, we predicted that the SOFD pattern will differ between large- and small-sized towns as indicated in a previous study of European breeding birds in urban core zone areas (Jokimäki et al., 2016). Finally, we predicted stable SAOR patterns also in urban areas since it is a very common pattern in more natural areas (Blackburn et al., 1998; Webb et al., 2007; Heino, 2008; Zuckerberg et al., 2009). In addition, we considered which of two common models, MPDM or NBM, could explain better the observed patterns in SOFD and SAOR.
Materials and Methods
Study Area
Our data originated from 29 villages and towns (hereafter, we use the term “towns” for simplicity) in northern coniferous forest biome in Finland (Jokimäki et al., 1996; Suhonen et al., 2009, 2010). Most of the study plots were about 30 ha (mean ± SD: 31.2 ± 7.7 ha; range: 13–51 ha). The study areas were situated relatively evenly along a 950-km north–south extent (60–68°N; Jokimäki et al., 1996). The study areas included urban settlements, ranging from large towns to villages; both habitat types were located evenly across Finland. The human populations in these communities ranged from 300 to 159,000 persons (mean ± SD: 21,694 ± 34,018 inhabitants) (Jokimäki et al., 1996). The variation in local habitat structure and edge effects were minimized by selecting each study plot from the most heavily urbanized area of each site. All study plots contained houses, roads, and scattered green areas (woods, parks, and fields) between houses (Jokimäki et al., 1996). The proportion (%) of block-of-flats, single-family house areas and wooded areas (mean ± SD) in the study plots has not changed during the study period [block-of-flats: 32.97 ± 39.40 (1990) and 32.86 ± 39.86 (2014); single-family houses: 33.31 ± 29.98 (1990) and 33.41 ± 30.05 (2014); green areas: 33.24 ± 27.01 (1990) and 33.24 ± 27.01 (2014); Mann–Whitney U-test; P > 0.05 in all cases].
The mean mid-winter temperature (January: mean ± SD) of the study sites differed between study winters, being lowest during 2009–2010 (−11.55°C ± 0.25), intermediate during 1999–2000 (−7.42°C ± 0.25), and highest during 1990–1991 (−4.7°C ± 0.25; F = 184.69; df = 2, 56; P < 0.001; Finnish Meteorological database). There was more snow (cm; mean ± SD) at the study sites during the winter of 1999–2000 (30.80 ± 1.86) than during 1991–1992 (20.15 ± 1.86) or 2009–2010 (19.69 ± 1.96; F = 11.38, df = 2, 56; P < 0.001; Finnish Meteorological database).
Winter Bird Surveys
Winter birds were surveyed by the single-visit study plot method (Bibby et al., 1992). Most of these censuses were made in late December or early January. We counted the birds during three different winters within 8- and 10-years interval: 1991–1992, 1999–2000, and 2009–2010. The census work in each case was done by one person (mainly the same person during each period) in good (windless and no rain) weather conditions at mid-days, when the light availability allowed the surveys to be conducted (in our case between 10.00 and 14.00). Most of the surveys were done during working days, Monday through Friday. The surveys were not done along a single route through the plots but instead as a zigzag walk through the plots. Survey speed was ~30 ha/h. To try to find all the species and individuals from the study plot, we searched birds also from the backyards and gardens of houses during surveys. This kind of a transect count reduces many of the problems associated with counting birds in urban areas, e.g., varying noise level and visibility (Jokimäki and Suhonen, 1998). It is also important to note that wintertime territorial behavior by singing in northern latitudes is lacking. Thus, singing behavior did not affect species detectability in our study. During the winter season, at least in northern areas as in our case, wintering birds are mainly foraging on bird feeders, partly due to the low temperatures, a short daytime (4–6 h) and snow cover (Hurme, 1973). Because feeding sites are more abundant in urban than in more natural sites, most overwintering birds are concentrated in these sites within human settlements (Jokimäki and Suhonen, 1998; Tryjanowski et al., 2015). Also, a scare vegetation cover, due to the absence of leaves on deciduous trees and shrubs in winter, increases the visibility of birds. Therefore, we suppose that the accuracy of the surveys is high. We also assume that the probability of observing birds is about the same for each data that are compared. In general, a single-visit census during wintering enables the detection of ~90% of the species and 80% of the individuals in urban environments (Suhonen, 1984; Jokimäki and Suhonen, 1998). To avoid double counts of individuals, we used a relatively high census rate (10 ha/20 min). All birds were included in the survey, except for overflying individuals, which did not land and stay on the study plot. We excluded aquatic bird species such as the mallard (Anas platyrhynchos), the herring gull (Larus argentatus), and the common gull (Larus canus) from these analysis, since their occurrence is mainly restricted to open water. We also excluded one large flock (over 800 individuals) of the Bohemian waxwing (Bombycilla garrulus) from the Saarenkylä (Jokimäki et al., 1996; Suhonen et al., 2009) survey site during the first winter 1991–1992, because the flock was migratory.
Following the suggestion of McGeoch and Gaston (2002), bird species were defined as core species if they occupied ≥90% of the study sites and satellite species if they occupied ≤10% of the study sites; the rest of the species were defined as intermediate species. Migratory status of bird species was extracted from Väisänen et al. (1998). We included invasive bird species in the partial migratory species category, and, in our case, migratory species included only species migrating to Central Europe or the Mediterranean area since long-distance migratory species were not detected in our study sites during winters.
Statistical Analysis
We tested for the modality of the occupancy frequency distribution in each winter of study. We used 10% occupancy classes and number or percentage of wintering bird species in each class to represent the geographical variation in the SOFD, as recommended by McGeoch and Gaston (2002). We applied the nonlinear regression analysis to the empirically ranked species occupancy curves (RSOCs; Jenkins, 2011). We made separate SOFD using by the pooled data, three winters separately, separate data of large and small towns as well as towns located in the south and north. For the regional SOFD pattern analysis, we grouped study sites for southern (<63°, 30′N) and northern towns (>63°, 30′N). For the town-size analysis, we grouped study sites for large-sized (>500 inhabitants/km2) and small-sized towns (<500 inhabitants/km2; see Jokimäki et al., 1996). For these analyses, in each of the data sets, species were listed in rows and towns as columns, and data were processed separately (Jenkins, 2011). All analyses described below are based on relative species occupancy (presence/absence) data at individual towns. First, we calculated the sum of all towns where a given species was observed. We then divided each species occupancy by the total number of towns to get the relative proportion where each species occupied (Jenkins, 2011). Second, we sorted the species by their relative occupancy values in decreasing order, where Ri is the rank value for species i. We plotted the relative occupancy of the species (Oi) as a function of Ri, resulting in the RSOC. Third, we compared which of the most common core–satellite species patterns (unimodal satellite dominant, bimodal symmetric, or bimodal asymmetric) gave the best fit for the assemblages (Jenkins, 2011).
We fitted each of the following three SOFD patterns:
(1) Unimodal satellite dominant (exponential concave): Oi = y0 + a*exp(–bRi), where the initial parameters were y0 = 0.01, a = 1.0, and b = 0.01.
(2) Bimodal symmetric (sigmoidal symmetric): Oi = a/[1 + exp (–bRi + c)], where the initial parameters were a = 1.0, b = −0.1, and c = −1.0.
(3) Bimodal asymmetric (sigmoidal asymmetric): Oi = a[1–exp (–bRic)], where the initial parameters were a = 1.0, b = −1.0, and c = −1.0,
where y0, a, b, and c were the estimated parameters.
The Levenberg–Marquardt algorithm (999 iterations) was used in the nonlinear regressions analyses following suggestions by Jenkins (2011), and parameters were estimated by means of ordinary least squares (OLS). We graphically evaluated the assumptions of the regressions for normality of residuals, homogeneity of variance, independent error terms, as well as the tails and shoulders of the data and models.
We used the AICc (Akaike information criterion for small sample sizes) to compare the alternative models. The model with the smallest AICc is considered to be the best with respect to the expected Kullback–Leibler information (Burnham and Anderson, 2000). The approach is powerful to detect differences between models if ΔAICc (= AICcmin-AICci) values are higher than 4 (Anderson et al., 2000; Jenkins, 2011).
We then used linear regression analysis to examine the relationships between mean local abundance and regional occupancy frequency, with the former as the independent variable and the latter as the dependent variable. Both mean local abundance and regional occupancy were log10 transformed to meet the assumptions of regression analysis. We used the general linear model to test the temporal variation in the strength and intensity in slope in species occupancy frequencies (log10 transformed). The explanatory variables were study winter (winter), size of town (small and large), and location of town (south and north), and abundance (log10 transformed) (continuous covariate) and interaction term winter × abundance. All the data analyses were performed using the IBM SPSS statistical package, version 23.
Results
A total of 35 species (Table 1) and 13,285 individuals were detected during the whole study period. An average study site had 8.5 (±SD = 2.9) species and 140.6 (±75.8) individuals during the winter 1991–1992; 7.9 (±2.0) species and 168.1 (±88.4) individuals during the winter 1999–2000; and 9.2 (±2.6) species and 149.5 (±85.5) individuals during the winter 2009–2010. In the pooled data, an average species occupied 21 out of 87 study sites (Figure 1). When different study winters were analyzed separately, an average species occupied about 10 out of 29 study sites in each winter (Figure 2).
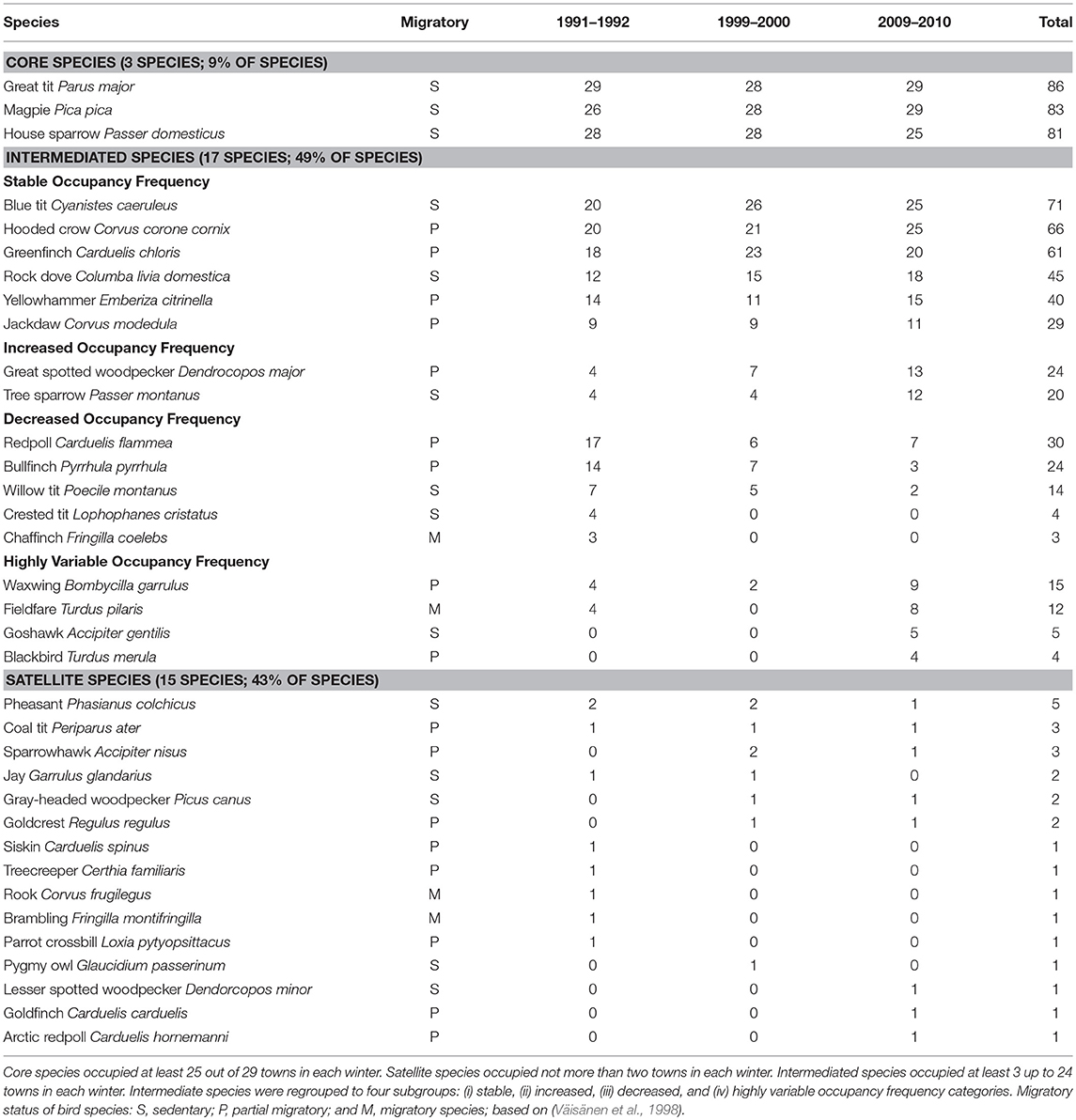
Table 1. Variation of 35 wintering urban bird species occupancy frequencies between three winters (1991–1992, 1999–2000, and 2009–2010) in Finland.
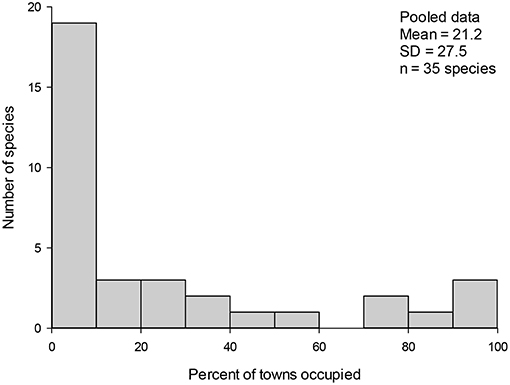
Figure 1. Number of urban wintering bird species (n = 35) in relation to the proportion of the study sites occupied in pooled data (%) in Finland. The proportion of sites occupied was divided to 10 equal size classes. The leftmost class represents satellite species, and the rightmost class represents the core species. Mean number of sites occupied and standard deviation (SD) of regional occupancy as well as the number of species (n) detected in three winters in 29 towns in Finland. Note that there were n = 87 study sites during three winters all together.
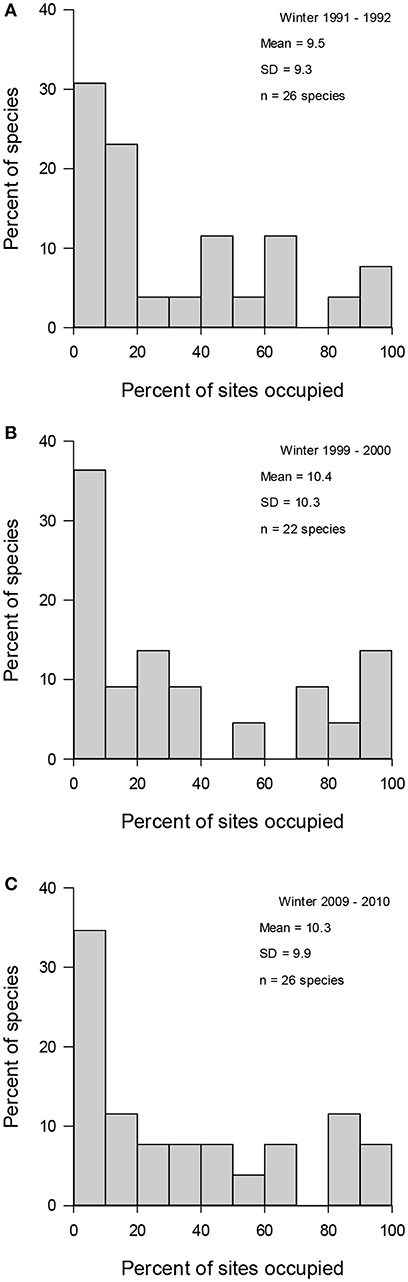
Figure 2. Variation in urban wintering bird species relative occupancy frequency distributions in three winters [(A) 1991–1992, (B) 1999–2000, and (C) 2009–2010]. Mean number of sites occupied and standard deviation (SD) of regional occupancy as well as the number of species (n) detected in each winter in 29 towns in Finland.
On average, each bird species occupied about one third of all study sites in each winter. Three sedentary species, great tit (Parus major), magpie (Pica pica), and house sparrow (Passer domesticus), were the core species; 15 species were satellite species; and 17 species were intermediate species (Table 1 and Figure 1). About 70% of the satellite and intermediate species were either partly migratory or migratory (Table 1). No core species changed its status from core species to satellite species, and vice versa, during the study period (Table 1). However, there were some between-winter variations in the occupancy frequency of individual bird species. For examples, the occupancy frequency of the common redpoll (Carduelis flammea), willow tit (Poecile montanus), and crested tit (Lophophanes cristatus) slightly decreased, whereas the opposite trend was observed for the great spotted woodpecker (Dendrocopos major), tree sparrow (Passer montanus), jackdaw (Corcus monedula), and blackbird (Turdus merula; Table 1 and Figure 3). The occupancy rates of the frugivorous birds, such as the bohemian waxwing (Bombycilla garrulus) and fieldfare (Turdus pilaris), varied greatly between winters (Table 1).
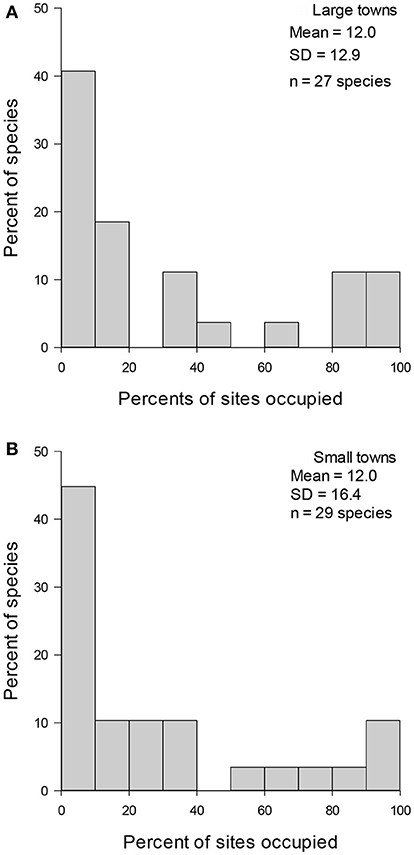
Figure 3. Variation in urban wintering bird species relative occupancy frequency distributions in (A) large (n = 12 towns) and (B) small towns (n = 17 towns). Mean number of sites occupied and standard deviation (SD) of regional occupancy as well as the number of species (n) detected during three winters in Finland.
Pooled data (Figure 1), data from different winters (Figure 2), and subdata of different sizes of towns (Figure 3) and towns located in southern and northern Finland (Figure 4) all followed the bimodal symmetrical SOFD pattern (Table 2). The SOFD patterns were very similar in each study winter (Figure 2) and when data were divided to the large and small towns (Figure 3). However, the proportion of satellite species was slightly greater in the southern towns (45%) than in the northern towns (35%, Figure 4).
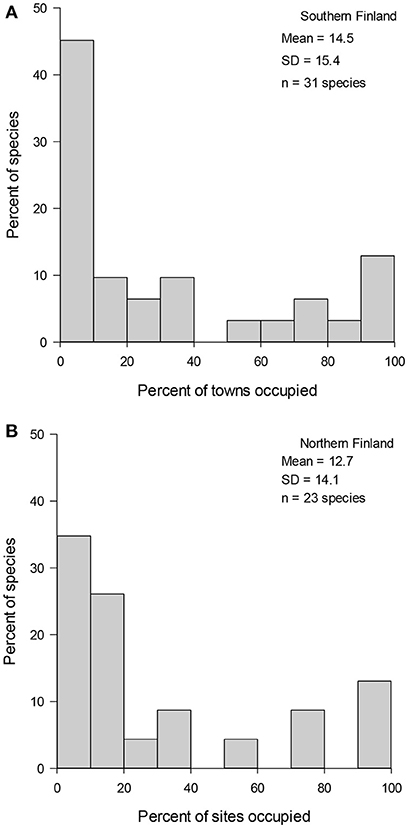
Figure 4. Variation in urban wintering bird species relative occupancy frequency distributions in (A) southern (n = 15 towns) and (B) northern towns (n = 14 towns). Mean number of sites occupied and standard deviation (SD) of regional occupancy as well as the number of species (n) detected during three winters in Finland.
The patterns of SAOR were highly positive in the pooled data (Table 3) and during all the study winters (Table 3 and Figure 5). There were no differences in the strength and slope of the SOAR between winters (Table 4 and Figure 5). The coefficient of determination ranged from 0.55 to 0.65 among the winters and was 0.69 in pooled data (Table 3). The SAOR patterns did not differ between the large and small towns or between towns located in the south or north (Table 4).
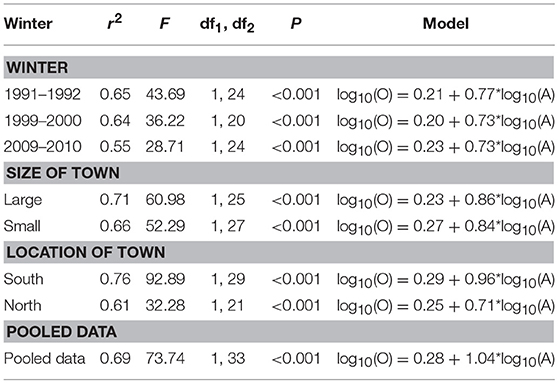
Table 3. Relationship between regional occupancy (dependent variable, log10 transformed) and mean local abundance (independent variable, log10 transformed) of wintering birds in three study winters, different sizes of towns, and location of town and in pooled data in Finland.
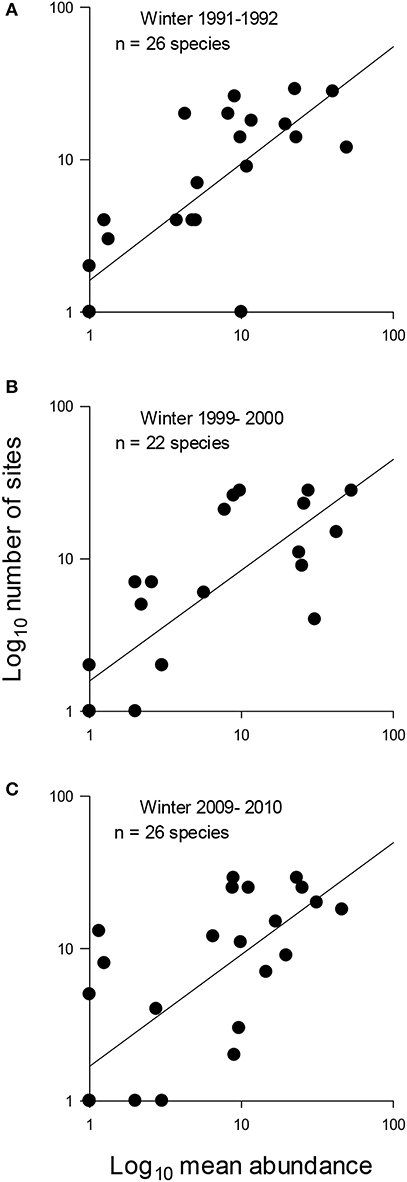
Figure 5. Relationship between regional occupancy and mean local abundance of urban wintering birds in three winters [(A) 1991–1992, (B) 1999–2000, and (C) 2009–2010] in Finland. Both mean local abundance and regional occupancy were log10 transformed. Continuous line is estimated by regression analysis. For regression results, see Table 1. Note that for several bird species, the same data point therefore clumped.
Discussion
Species Richness
We observed a total of 35 wintering bird species in our study towns in Finland, which is ~30% of the total number of bird species wintering in Finland (Lehikoinen and Väisänen, 2014). These results indicate that residential areas are important areas for the many overwintering bird species (Jokimäki and Kaisanlahti-Jokimäki, 2012; Leveau and Leveau, 2012). The proportion of satellite species (43%) in the pooled data was much greater than that observed earlier in a breeding season study conducted in urban core zone areas in Europe (20%; Jokimäki et al., 2016). In addition, southern towns had slightly more satellite species than northern towns, probably due to a greater number of overwintering migratory species occupying in the south than in the north (Lehikoinen and Väisänen, 2014). These results indicate that there are some seasonal differences in bird community composition in urban areas.
We found that the same three sedentary core bird species dominated the winter bird assemblages during every study winters independently of the location and the size of the town. These species (great tit, magpie, and house sparrow) belong also to the species that could be found in most winter feeding sites in Finland (Väisänen, 2018), urban wintering bird assemblages in Poland (Tryjanowski et al., 2015), as well as in breeding bird communities of most European towns (Jokimäki et al., 2016). We did not detect any between-winter cases when a core species changed to satellite species, or vice versa. In practice, there is little empirical evidence that species move between the core and satellite classes in different areas, although there are some observations that a core species may be the same across a variety of habitat types (McGeoch and Gaston, 2002). In our case, two intermediate bird species (blue tit Cyanistes caeruelus and hooded crow Corvus corone cornix) were included in the core species list in some study winters, but no satellite species were changed into the intermediate species group. However, some bird species with intermediate occupancy frequencies showed changes in their relative occupancy rate between the study winters. For example, in two coniferous forest species, the willow tit and the crested tit, and a deciduous forest species, common redpoll, occupation frequencies decreased, whereas the relative occupation frequencies of the tree sparrow and blackbird increased during the study period. The occupancy rates of frugivorous birds such as the bohemian waxwing and fieldfare varied between years due to variation on fruit crop size of rowan berry trees (Sorbus aucuparia; Lehikoinen et al., 2010; Suhonen and Jokimäki, 2015). Our results in species-specific occupancy frequencies and changes in occupancy frequencies are in a good concordance with the long-term winter bird population changes observed both in the Finnish winter bird surveys 1987–2014 (Lehikoinen and Väisänen, 2014; Lehikoinen et al., 2016) and winter feeding site study during 1988–2017 (Väisänen, 2018). Both the satellite and intermediate species have several partial migratory or migratory bird species (Table 1). It is possible that these overwintering migratory bird species might increase species richness and total abundance birds in the winter bird assemblage, especially in the south, and also increase the number of satellite species in the SOFD pattern and decrease the slope in the SAOR pattern in the future.
Species Occupancy Frequency Distribution and Positive Species Abundance–Occupancy Relationship Patterns
Both SOFD and SAOR patterns may possibly depend on sampling artifacts as well as environmental and biological mechanisms such as (i) habitat disturbance, (ii) niche breadth, (iii) dispersal ability, and (iv) sampling site position within geographical range (see Gaston et al., 2000; McGeoch and Gaston, 2002). The sample unit should be kept constant when studying assemblages with a fewer than 20 species (we had at least 22 species), and surveys should be conducted using similar extents. To facilitate comparison, our data came from the core area of each town, and standard sampling procedures were used. The sampling artifact may cause a positive SAOR if locally uncommon species are not detected, although they actually occur at a site (Gaston et al., 1997b, 2000). It is, thus, likely that some locally uncommon bird species were not detected at every site where they actually occurred. The problem of undersampling rare species is to some extent unavoidable (McGeoch and Gaston, 2002), and the longer sampling continues, the more rare species are likely to be added to the satellite mode (McGeoch and Gaston, 2002). To avoid these problems, we standardize our bird counting time to 20 min per 10 ha. Also, samples from large areas are more heterogeneous than samples from smaller areas (McGeoch and Gaston, 2002; Heatherly et al., 2007), but our study sites were almost equal in size (about 30 ha), and we counted birds at each town center to decrease habitat heterogeneity between study sites. Moreover, in most of the cases, the same person surveyed exactly the same study sites in each study winters, which decreases possible interobserver bias related to bird detectability and searching intensity. Thus, the possible artefacts of study size and bird counting methods were probably marginal in this study.
Species Occupancy Frequency Distribution Pattern
We found a bimodal symmetric SOFD pattern that was constant during the study winters. A bimodal SOFD pattern has also been reported in many earlier studies conducted in more natural habitats (Mehranvar and Jackson, 2001). We did not find any differences in SOFD patterns between different sizes of towns and towns located in different latitudes. These results conflict with the breeding season data from the core zones areas of European towns, in which a bimodality SOFD pattern was observed only within large-sized and central European towns (Jokimäki et al., 2016). These differences might be related to both the different extent of these studies (national vs. European level) and season (winter vs. summer). Also, our winter season study did not include very large sized tows with millions of inhabitants. As the scale expand, it is possible that the sample will contain more satellite species. During winter, the regional species pool is smaller and many species are concentrated in urban habitats, at least in northern latitudes like in Finland.
Some earlier studies have found some variability in the SOFD pattern among regions (Heatherly et al., 2007; Jokimäki et al., 2016; Korkeamäki et al., 2018). Our results suggest that the SOFD pattern slightly changed with the latitude, southern towns having more satellite species than the northern towns, probably due to a higher number of migratory species in the south. However, in a breeding bird study, northern regions had more satellite species than southern regions (Jokimäki et al., 2016).
We found that all core species were sedentary generalist species, whereas most (about 70%) satellite and intermediate species were partial migratory or migratory species (Table 1). The NBM did not fit well with our data because it predict that most species are rare, and thus, the SOFD pattern should be a unimodal satellite dominant mode. An earlier study has indicated that local species richness either slightly increased or decreased between winters (Suhonen et al., 2009) as predicted by the MPDM. The temporal stability of the SOFD fits well with the predictions of the MPDM, which predict co-occurrence of many core (common) and satellite (rare) species in the assemblage. If the SOFD pattern is bimodal and species relative occupation rates vary temporally, then MPDM would be shown a better fit to the data than the NBM (Hanski, 1982; Collins and Glenn, 1991). We found that in some species, the relative species occupancy frequency either increased or decreased during the 18-year study period as predicted by the MPDM, although we did not find any case wherein a core species shifted to satellite species and vice versa.
Species Abundance–Occupancy Relationship Pattern
Our data indicated that the temporal variability of the SAOR was rather stable over the years as has been observed in several previous studies conducted in more natural habitats (Blackburn et al., 1998; Webb et al., 2007; Heino, 2008). In addition, the SAOR pattern did not vary between different sizes of towns or towns located in different latitudes. Although both the NBM (e.g., Brown, 1984) and the MPDM (e.g., Hanski, 1982) predict a positive SAOR, our results give more support to the MPDM (Hanski, 1982), which bimodality should result from stochastic species colonization and extinctions. In other words, even common species will go extinct at several plots and become rare (Suhonen and Jokimäki, submitted). On the other hand, rare species can become common via new arrivals (as we observed, e.g., in the case of the great woodpecker and the blackbird), although such shifts should be primarily seen only in long-term studies (e.g., Hanski, 1982). An alternative NBM predicts temporally stable occupancy and abundance patterns for species (Brown, 1984), and our data did not support that.
Conclusions
To conclude, our results indicate that urban wintering bird assemblages follow a bimodal symmetric SOFD pattern with many common sedentary species and many rare and regionally uncommon migratory and partial migratory bird species. In addition, the SOFD and SAOR patterns did not vary between the study winters, indicating that urbanization is stabilizing temporal variation of wintering bird assemblages. However, in some cases, urbanization might cause a rapid decrease in wintering bird species diversity as reported in urbanizing rural areas in Poland (Ciach, 2012). Gaston et al. (1997a) have suggested that birds appear unlikely to exhibit metapopulation dynamics because the dispersal ranges of the vast majority of species are so large. However, some studies have indicated that urban populations can be genetically separated or distinct from their surrounding populations (Evans et al., 2009; Johnson and Munshi-South, 2017; see more references in Schilthuizen, 2018).
Most of the previous studies have only described the pattern, without putting much interest in the direct evaluation of the mechanisms behind the SOFD and SAOR in wintering bird assemblages. However, intensive winter feeding, artificial light, and warmer microclimate (urban heat island phenomena) might reduce temporal variation of urban wintering bird assemblages (Ciach and Fröhlich, 2017). Also, warmer winter climate may be beneficial for southerly distributed bird species, allowing them to colonize our backyards and reformatting winter bird assemblages (Fraixedas et al., 2015; Princé and Zuckerberg, 2015). Therefore, further empirical and theoretical studies are needed to obtain more detailed information regarding how urbanization influences variation in SOFD and SAOR patterns.
Author Contributions
JS and JJ conceived and designed the study, collected the data, and wrote the manuscript. JS analyzed the data.
Conflict of Interest Statement
The handling editor declared a past co-authorship with the authors.
Acknowledgments
We thank Harri Hakkarainen, Teppo Helo, Juhani Honkola, Matti Hovi, Esa Huhta, Kimmo Inki, Simo Jokinen, Marja-Liisa Kaisanlahti-Jokimäki, Esa Korkeamäki, Teppo Mutanen, Olli Osmonen, Ossi Pihajoki, Pekka Rahko, Pentti Rauhala, Pirkko Siikamäki, Esko Sirjola, and Petri Suorsa for their assistance in the wintering bird field surveys.
References
Anderson, D., Burnham, K., and Thompson, W. (2000). Null hypothesis testing: problems, prevalence, and an alternative. J. Wildl. Manage. 64, 912–923. doi: 10.2307/3803199
Bellocq, M. I., Leveau, L. M., and Filloy, J. (2017). “Urbanization and bird communities: spatial and temporal patterns emerging from southern South America,” in Ecology and Conservation of Birds in Urban Environments. eds E. Murgui and M. Hedblom (Cham: Springer), 35–54.
Blackburn, T. M., Cassey, P., and Gaston, K. J. (2006). Variations on a theme: sources of heterogeneity in the form of the interspecific relationship between abundance and distribution. J. Anim. Ecol. 75, 1426–1439. doi: 10.1111/j.1365-2656.2006.01167.x
Blackburn, T. M., Gaston, K., Greenwood, J., and Gregory, R. (1998). The anatomy of the interspecific abundance–range size relationship for the British avifauna: II. Temporal dynamics. Ecol. Lett. 1, 47–55.
Blackburn, T. M., and Gaston, K. J. (1997). A critical assessment of the form of the interspecific relationship between abundance and body size in animals. J. Anim. Ecol. 66, 233–249.
Brown, J. (1984). On the relationship between abundance and distribution of species. Am. Nat. 124, 255–279.
Burnham, P., and Anderson, D. R. (2000). Model Selection and Multimodel Inference. A Practical Information-Theoretic Approach. New York, NY: Springer.
Chace, J. F., and Walsh, J. J. (2006). Urban effects on native avifauna: a review. Landscape Urban Plann. 74, 46–69. doi: 10.1016/j.landurbplan.2004.08.007
Ciach, M. (2012). The winter bird community of rural areas in the proximity of cities: low density and rapid decrease in diversity. Pol. J. Ecol. 60, 193–199.
Ciach, M., and Fröhlich, A. (2017). Habitat type, food resources, noise and light pollution explain the species composition, abundance and stability of a winter bird assemblage in an urban environment. Urban Ecosyst. 20, 547–559. doi: 10.1007/s11252-016-0613-6
Collins, S., and Glenn, S. (1991). Importance of spatial and temporal dynamics in species regional abundance and distribution. Ecology 72, 654–664.
Collins, S., and Glenn, S. (1997). Effects of organismal and distance scaling on analysis of species distribution and abundance. Ecol. Appl. 7, 543–551.
Evans, K. L., Gaston, K. J., Frantz, A. C., Simeoni, M., Sharp, S. P., McGowan, A., et al. (2009). Independent colonization of multiple urban centres by a formerly forest specialist bird species. Proc. R. Soc. B 276, 2403–2410. doi: 10.1098/rspb.2008.1712
Fidino, M., and Magle, S. B. (2017). “Trends in long-term urban bird research,” in Ecology and Conservation of Birds in Urban Environments. eds E. Murgui and M. Hedblom (Cham: Springer), 161–184.
Fraixedas, S., Lehikoinen, A., and Lindén, A. (2015). Impacts of climate and land-use change on wintering bird populations in Finland. J. Avian Biol. 46, 63–72. doi: 10.1111/jav.00441
Francis, R., and Chadwick, M. (2013). Urban Ecosystems: Understanding the Human Environment. London: Routledge.
Gaston, K., Blackburn, T., Greenwood, J., Gregory, R., Quinn, R., and Lawton, J. (2000). Abundance–occupancy relationships. J. Appl. Ecol. 37, 39–59. doi: 10.1046/j.1365-2664.2000.00485.x
Gaston, K., Blackburn, T., and Gregory, R. (1997a). Abundance–range size relationships of breeding and wintering birds in Britain: a comparative analysis. Ecography 20, 569–579.
Gaston, K., Blackburn, T., and Lawton, J. (1997b). Interspecific abundance range size relationships: an appraisal of mechanisms. J. Anim. Ecol. 66, 579–601.
Gaston, K. J., Blackburn, T. M., and Spicer, J. I. (1998). Rapoport's rule: time for an epitaph? Trends Ecol. Evol. 13, 70–74.
Hanski, I. (1982). Dynamics of regional distribution—the core and satellite species hypothesis. Oikos 38, 210–221.
Heatherly, T., Whiles, M. R., Gibson, D. J., Collins, S. L., Huryn, A. D., Jackson, J. K., et al. (2007). Stream insect occupancy-frequency patterns and metapopulation structure. Oecologia 151, 313–321. doi: 10.1007/s00442-006-0596-8
Heino, J. (2008). Temporally stable abundance–occupancy relationships and occupancy frequency patterns in stream insects. Oecologia 157, 337–347. doi: 10.1007/s00442-008-1078-y
Jenkins, D. G. (2011). Ranked species occupancy curves reveal common patterns among diverse metacommunities. Global Ecol. Biogeogr. 20, 486–497. doi: 10.1111/j.1466-8238.2010.00617.x
Johnson, M. T. J., and Munshi-South, J. (2017). Evolution of life in urban environments. Science 358:eaam8327. doi: 10.1126/science.aam8327
Jokimäki, J., and Kaisanlahti-Jokimäki, M. (2012). The role of residential habitat type on the temporal variation of wintering bird assemblages in northern Finland. Ornis Fenn. 89, 20–33.
Jokimäki, J., and Suhonen, J. (1998). Distribution and habitat selection of wintering birds in urban environments. Landscape Urban Plann. 39, 253–263.
Jokimäki, J., Suhonen, J., Inki, K., and Jokinen, S. (1996). Biogeographical comparison of winter bird assemblages in urban environments in Finland. J. Biogeogr. 23, 379–386.
Jokimäki, J., Suhonen, J., and Kaisanlahti-Jokimäki, M. (2016). Urbanization and species occupancy frequency distribution pattern in core zone areas of European towns. Eur. J. Ecol. 2, 23–43. doi: 10.1515/eje-2016-0014
Korkeamäki, E., Elo, M., Sahlen, G., Salmela, J., and Suhonen, J. (2018). Regional variations in occupancy frequency distribution patterns between odonate assemblages in Fennoscandia. Ecospehere 9:e02192. doi: 10.1002/ecs2.2192
Lehikoinen, A., Foppen, R. P., Heldbjerg, H., Lindström, Å., van Manen, W., Piirainen, S., et al. (2016). Large-scale climatic drivers of regional winter bird population trends. Div. Distr. 22, 1163–1173. doi: 10.1111/ddi.12480
Lehikoinen, A., and Väisänen, R. A. (2014). Habitat-specific changes of Finnish winter bird populations in 1987-2014. Linnut-Vuosikirja 2013 (in Finnish with English summary), 78–93.
Lehikoinen, A., Väisänen, R. A., and Hokkanen, T. (2010). Variation of abundance in 14 bird species in relation to the seed and berry crop of trees in Finland in winters 1957–2009. Linnut-vuosikirja 2009 (in Finnish with English summary), 80–93.
Leveau, L. M., Isla, F. I., and Bellocq, M. I. (2015). Urbanization and the temporal homogenization of bird communities: a case study in central Argentina. Urban Ecosyst. 18, 1461–1476. doi: 10.1007/s11252-015-0469-1
Leveau, L. M., and Leveau, C. M. (2012). The role of urbanization and seasonality on the temporal variability of bird communities. Landscape Urban Plann. 106, 271–276. doi: 10.1016/j.landurbplan.2012.03.008
Marzluff, J. M., Bowman, R., and Donnelly, R. (2001). “A historical perspective on urban bird research: trends, terms, and approaches,” in Avian Ecology and Conservation in An Urbanizing World. eds J. M. Marzluff, R. Bowman, and R. Donnelly (Boston, MA: Springer), 1–17.
McGeoch, M. A., and Gaston, K. J. (2002). Occupancy frequency distributions: patterns, artefacts and mechanisms. Bio. Rev. 77, 311–331. doi: 10.1017/S1464793101005887
Mehranvar, L., and Jackson, D. (2001). History and taxonomy: their roles in the core-satellite hypothesis. Oecologia 127, 131–142. doi: 10.1007/s004420000574
Princé, K., and Zuckerberg, B. (2015). Climate change in our backyards: the reshuffling of North America's winter bird communities. Global Change Biol. 21, 572–585. doi: 10.1111/gcb.12740
Schilthuizen, M. (2018). Darwin Comes to Town: How the Urban Jungle Drives Evolution From Urban Ecology and Evolution. New York, NY: Picador.
Suhonen, J. (1984). Sään vaikutuksesta lintujen havaittavuuteen talvilintulaskennassa (in Finnish). Keski-Suomen Linnut 9, 4–8.
Suhonen, J., and Jokimäki, J. (2015). Fruit removal from rowanberry (Sorbus aucuparia) trees at urban and rural areas in Finland: a multi-scale study. Landscape Urban Plann. 137, 13–19. doi: 10.1016/j.landurbplan.2014.12.012
Suhonen, J., Jokimäki, J., Kaisanlahti-Jokimäki, M., Hakkarainen, H., Huhta, E., Inki, K., et al. (2009). Urbanization and stability of a bird community in winter. Ecoscience 16, 502–507. doi: 10.2980/16-4-3280
Suhonen, J., Jokimäki, J., Kaisanlahti-Jokimäki, M., Hakkarainen, H., Huhta, E., Inki, K., et al. (2010). Urbanization and stability of a bird community in winter (vol 16, pg 502, 2009). Ecoscience 17, 121–121. doi: 10.2980/019.017.0102
Tryjanowski, P., Sparks, T. H., Biadun, W., Brauze, T., Hetmanski, T., Martyka, R., et al. (2015). Winter bird assemblages in rural and urban environments: a national survey. PLoS ONE 10:e0130299. doi: 10.1371/journal.pone.0130299
United Nations (2015). World Urbanization Prospects: The 2014 Revision. New York, NY: United Nations.
Väisänen, R. A. (2003). Regional population trends of 33 common bird species in Finland during 27 winters. Linnut-vuosikirja 2002. (in Finnish with English summary), 41–62.
Väisänen, R. A. (2018). Long-term changes in frequency and abundance of bird species at winter feeding sites in Finland during 29 winters 1988/1989–2016/2017. Linnut-vuosikirja 2017 (in Finnish with English summary), 32–47.
Väisänen, R. A., Lammi, E., and Koskimies, P. (1998). Muuttuva pesimälinnusto (Summary: Distribution, Numbers and Population Changes of Finnish Breeding Birds). Helsinki: Otava.
Webb, T. J., Noble, D., and Freckleton, R. P. (2007). Abundance–occupancy dynamics in a human dominated environment: linking interspecific and intraspecific trends in British farmland and woodland birds. J. Anim. Ecol. 76, 123–134. doi: 10.1111/j.1365-2656.2006.01192.x
Keywords: core–satellite hypothesis, metapopulation dynamics, niche limitation, temporal variability, urban birds, winter
Citation: Suhonen J and Jokimäki J (2019) Temporally Stable Species Occupancy Frequency Distribution and Abundance–Occupancy Relationship Patterns in Urban Wintering Bird Assemblages. Front. Ecol. Evol. 7:129. doi: 10.3389/fevo.2019.00129
Received: 16 October 2018; Accepted: 01 April 2019;
Published: 30 April 2019.
Edited by:
Federico Morelli, Czech University of Life Sciences Prague, CzechiaReviewed by:
Michał Ciach, University of Agriculture of Krakow, PolandRaivo Mänd, University of Tartu, Estonia
Copyright © 2019 Suhonen and Jokimäki. This is an open-access article distributed under the terms of the Creative Commons Attribution License (CC BY). The use, distribution or reproduction in other forums is permitted, provided the original author(s) and the copyright owner(s) are credited and that the original publication in this journal is cited, in accordance with accepted academic practice. No use, distribution or reproduction is permitted which does not comply with these terms.
*Correspondence: Jukka Suhonen, anVrc3VoQHV0dS5maQ==