- 1Department of Integrative Biology, University of Guelph, Guelph, ON, Canada
- 2Appalachian Laboratory, University of Maryland Center for Environmental Science, Frostburg, MD, United States
The migratory monarch butterflies (Danaus plexippus) of eastern North America have undergone large-scale declines, which may be attributable to a variety of underlying causes. The uncertainty about the primary cause of declines and whether individual threats are likely to increase in the future presents challenges for developing effective conservation management and policy initiatives that aim to improve population viability. This paper identifies five potential threats and classifies these threats according to the types of studies (observational, experimental, simulation/models) and their current impact and anticipated risk. Broadly, the threats can be classified into five categories: (1) change in suitable abiotic environmental conditions; (2) deforestation in the overwintering range; (3) exposure to contaminants including the bacteria Bacillus thuringiensis, herbicides, and insecticides; (4) loss of breeding habitat; and (5) predation, parasitism, and species-specific pathogens. The vast distribution of the monarch butterfly makes it likely that population declines are attributed to a suite of interacting factors that vary spatially and temporally in their contribution. Nonetheless, the published papers we reviewed suggest the decline in suitable environmental conditions in addition to overwintering (i.e., deforestation) and breeding habitat loss are the most likely threats to continue to affect the population viability of monarch butterflies.
Introduction
Insect populations are experiencing rapid declines globally (Dirzo et al., 2014; Stork et al., 2015) that may have implications for ecosystem function and contributions to economic services (Allen-Wardell et al., 1998; Potts et al., 2010). Changes in the suitability of environmental conditions driven by extreme weather and climate change (Batalden et al., 2007; Barve et al., 2012; Brower et al., 2017), habitat loss (Didham et al., 1996; Fattorini, 2011; Thogmartin et al., 2017b), exposure to contaminants (Stanley-Horn et al., 2001; Thogmartin et al., 2017b), and changes in species interactions (e.g., invasive species, Burghardt and Tallamy, 2015) can have profound effects on biodiversity. The substantial loss of biodiversity within the insect taxon emphasizes their sensitivity to environmental perturbations, but also makes them ideal bioindicators (Lenhard and Witter, 1977; Nummelin et al., 2007) for testing the impact of threats and their downstream effects. By integrating an array of research methods and disciplines, the ramifications of declines on biological systems can be better-anticipated and incorporated into conservation management plans and policy initiatives (Vanbergen and Garratt, 2013).
A multi-disciplinary approach is needed to address the loss of insect diversity and to understand the potential mechanisms driving declines. Extending research initiatives beyond traditional economically significant species may allow for identification of shared mechanisms behind observed declines. In doing so, it is important to consider the type and strength of evidence for threats to a population, emphasizing varying susceptibility at different life stages and across the species range. Therefore, the dynamic and synergistic nature of potential threats can be evaluated and considered when developing conservation strategies, especially for animals that cross national and international boundaries.
The eastern migratory North American population of monarch butterflies (Danaus plexippus) undergoes an annual migration between the Sierra Madre Mountains of Mexico and the northern United States and southern Canada (Urquhart, 1960; Urquhart and Urquhart, 1978; Brower, 1995). In Mexico, monarchs overwinter in large colonies for 4–5 months and remain in a reproductive diapause. In spring, individuals begin mating and migrate north to lay eggs on emerging milkweed (Asclepias spp.) in the southern United States (Brower, 1995). Over successive generations the population colonizes much of the eastern and central United States and parts of southeastern and south central Canada (Brower, 1995; Flockhart et al., 2013). The complex nature of the annual cycle and habitat specialization provides a rare opportunity to investigate ecological pressures across a variety of temporal and geographical scales. Moreover, such an understanding can improve our knowledge of the international cooperation required to preserve this flagship species-at-risk.
Conservation management plans rely on the accurate estimation of species abundance. Over the last 2 decades the eastern population of monarch butterflies has declined more than 80% at overwintering sites (Thogmartin et al., 2017b). Arguably overwintering population size determined by the occupied surface area represents the most reliable estimates and denotes the effective population size (i.e., number of individuals contributing to the next generation, Davis, 2012; Ries et al., 2015b). However, counts taken in the northerly portion of the range during pre-migration do not always correspond with those at overwintering sites, which may suggest either methodological issues in estimating population size or high mortality during migration (Davis, 2012; Badgett and Davis, 2015; Ries et al., 2015b; Inamine et al., 2016; Pleasants et al., 2017). Regardless, despite substantial interannual variation in monarch population size (>10-fold, Swengel, 1995; Rendón-Salinas et al., 2014), summer and winter counts show consistent year-to-year fluctuations (Ries et al., 2015a,b). The discrepancy between population estimates in winter and summer highlights the need to distinguish independent threats contributing to potential declines observed throughout the annual cycle and at different developmental stages.
Changes in suitable environmental conditions (Barve et al., 2012; Thogmartin et al., 2017b) and habitat at both breeding (Pleasants, 2017; Thogmartin et al., 2017a) and overwintering (Oberhauser et al., 2017) sites, as well as contaminant exposure (Oberhauser et al., 2006, 2009; Pecenka and Lundgren, 2015), are thought to be foremost threats to monarch butterfly populations. Suboptimal environmental conditions during the overwintering period, such as unseasonably warm temperatures (Hunt and Tongen, 2017) or cold and wet microclimates that pose a risk of freezing (Anderson and Brower, 1996), can accelerate lipolysis that quickly depletes lipid stores needed for overwinter survival (Alonso-Mejía et al., 1997). Overwintering lipid stores may be further reduced by limited availability of nectar sources due to habitat loss and a northward shift in monarch movements expected with climate change (Batalden et al., 2007; Brower et al., 2015; Lemoine, 2015). The introduction and widespread adoption of glyphosate resistant corn and soybean has greatly increased the use of herbicide, causing up to 68% loss of milkweed in some areas of the central United States (Pleasants and Oberhauser, 2013; Flockhart et al., 2015; Zaya et al., 2017), and logging and rural development have limited suitable habitat for overwintering sites (Brower et al., 2011b). Finally, environmental contaminants, including genetically-modified (GM) pollen (i.e., Bacillus thuringiensis (Bt), Anderson et al., 2004, 2005) and insecticides (e.g., neonicotinoids, Pecenka and Lundgren, 2015; pyrethroids, Oberhauser et al., 2006, 2009), as well as pathogens [notably, Ophryocystis elektroscirrha (OE)], could contribute to elevated rates of mortality. As a species at risk (SARA 2017, US Fish & Wildlife Service 2017) it is imperative that research identifies the foremost threat(s) resulting in decreased fitness and survival and the relative contribution of each threat to the cumulative population decline.
To better understand the main drivers of monarch decline it is important to evaluate the type of study investigating the threat and strength of evidence (i.e., support) for each potential threat in terms of their effect on monarch butterflies and possible future risk to population viability. Though it is probable that other threats exist beyond those identified in this review, we focus on five broad potential threats commonly reported for the eastern migratory North American population of the monarch butterfly: (1) change in suitable abiotic environmental conditions, (2) deforestation in the overwintering range, (3) exposure to contaminants including the bacteria Bt, herbicides, and insecticides, (4) loss of breeding habitat, and (5) predation, parasitism, and pathogens. We modify a previously established scoring system (Godfray et al., 2013, 2014), sorting and summarizing peer-reviewed research papers into three groups based on the type of study. For each research paper we then assign the level of support for the potential threat affecting monarch butterflies and assess the likelihood of the threat persisting and/or increasing. Though a recent review highlighted anthropogenic impacts on monarch populations (Malcolm, 2017), our aim is to evaluate the strength of evidence available for each threats and highlight key gaps in research needed to guide conservation management plans and policy development.
Methods
A systematic review of available peer-reviewed literature was performed following the procedure outlined in Bechshoft et al. (2017), but using monarch-specific terms associated with hypotheses regarding population declines in the Web of Science™ (Reuters, 2016). An initial list of papers was established through a literature search of all databases, including the Web of Science Core Collection, Current Contents Connect, FSTA (The Food Science Resource), KCI (Korean Journal Database), MEDLINE, Russian Science Citation Index, SciELO Citation Index, and Zoological Record, with no limitations placed on the publication date. This list was later refined to retain only peer-reviewed research papers on the eastern migratory North American population of monarch butterflies in English. Search terms (“topic”) were combined in pairs using the Boolean operator “and,” with select terms having wildcard truncation (*) to allow for various word endings (Table 1).
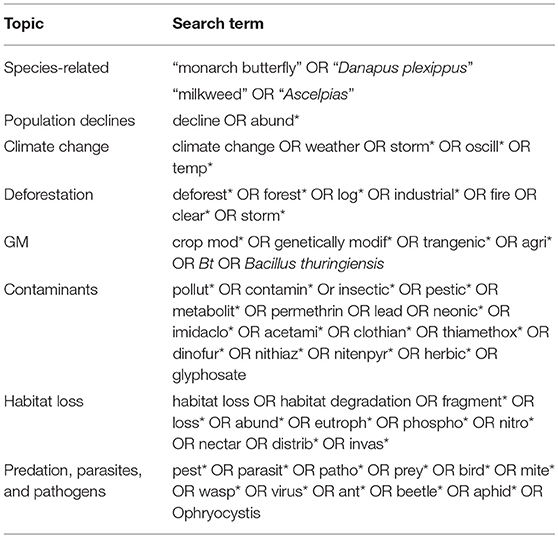
Table 1. Summary of search terms associated with the declines in the eastern migratory North American population of monarch butterflies (Danaus plexippus) used in the comprehensive literature review using Web of Science™.
We sorted and summarized individual peer-reviewed research papers (n = 115) published up to December 2018 by potential threat and assessed the type of study (e.g., [Control_data], [Field_data], [Mod]) using a scoring system modified from Godfray et al. (2013, 2014) with the following unranked categories:
[Control_data] evidence involving controlled experimental studies.
[Field_data] evidence involving data collected in the field but without experimental control.
[Mod] indirect evidence based on previously collected experimental and/or field collected data to assess the impact of threats on the population or make projections of future environmental and conservation scenarios. Models reflect a degree of uncertainty that cannot be fully accounted for as conditions encountered in the future may deviate from those defined in the model.
After classifying the type of study, we then identified whether research papers independently provided support (i.e., whether a threat was supported [S] or not [N]) for the threat as having a current impact on monarch butterflies and whether there was potential for the threat to pose continued risk to population viability. Support for future risk to the eastern migratory North American population of monarch butterflies was assigned based on when impacts were identified from multi-year and/or historical data or from model projections. Studies where no conclusion could be drawn about the future risk to the population were identified by [-]. We then calculated the percentage of research papers (i.e., number of research papers of the total available studies within each type of threat) for: (1) the type of study, (2) whether the study provided support for a threat to monarch butterflies, and (3) the future risk posed to the population. Papers classified in multiple categories (e.g., [Control_data] and [Field_data]) contributed an equivalent number of times to the total number of available studies. Where described in the Results, we also calculated the percentage of represented papers for subcategories within each threat category for the type of study or level of support. For example, within the category of changes in suitable environmental conditions, we calculated the percentage of studies addressing periodic, adverse weather conditions (relative to long-term climate change).
Results
The summary of peer-reviewed literature, sorted by threat and type of study, assessing the impact, and future risk imposed to the eastern migratory North American monarch butterfly population is presented in Supplementary Table 1. The percentage of research papers assigned to each type of study and their support for the specific threat on monarch butterflies and potential risk to the population is presented in Table 2.
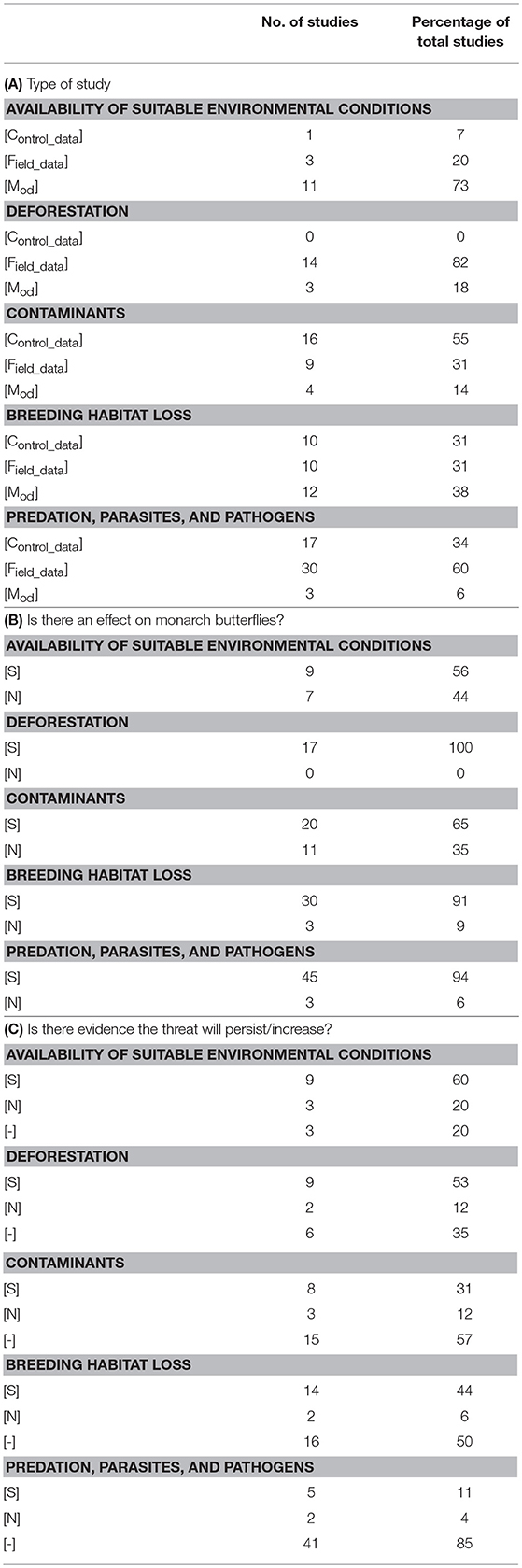
Table 2. The proportion of peer-reviewed research papers classified by the type of study, effect on monarchs, and potential risk imposed by the threat to the eastern migratory North American population of monarch butterflies (Danaus plexippus).
Change in Suitable Abiotic Environmental Conditions
Field studies constituted one of the principal methods documenting the effects of sudden changes in environmental conditions and adverse weather patterns in the decline of monarch butterflies (50% of total studies on adverse weather events, Brower et al., 2015, 2017), but only a single study considered the effect of extreme weather patterns before fall migration (25% of total studies on adverse weather events, Hunt and Tongen, 2017). Field studies examined the physiological response of monarchs to changes in environmental conditions in the southern portion of the migratory range (Brower et al., 2015), but controlled studies that assessed field-realistic, short-term changes in environmental variables such as temperature, humidity, precipitation, or solar radiation on the physiological condition and survival during the breeding season were absent. Similarly, few studies applied modeling techniques that evaluated the impact of weather extremes on monarch population viability (Flockhart et al., 2015; Hunt and Tongen, 2017).
The peer-reviewed literature suggested a negative impact of adverse weather patterns on monarch butterflies (50% of total studies on adverse weather events, Brower et al., 2017; Hunt and Tongen, 2017) and these conditions could impact monarchs at each stage of their life cycle (Hunt and Tongen, 2017). Though sporadic events may result in considerable losses, the timing of the events is also suggested to alter the severity of the impact. Brower et al. (2015) noted that nectar sources available in the southern portion of the migratory range might offset the energetic cost of adverse conditions experienced earlier in migration, therefore having less impact than extreme weather on overwintering populations. Further, though Hunt and Tongen (2017) showed a negative effect of increasing extreme weather events on monarch butterflies, no studies evaluated the effect of adverse weather patterns in long-term datasets or the extent to which populations are capable of recovering afterwards.
Few controlled experiments investigated the effects of predicted long-term climatic conditions on the condition, growth, and reproduction of monarch butterflies (9% of total studies on climate change) and only a single study explored how rising temperatures impacted host plants at different latitudes (9% of total studies on climate change, Couture et al., 2015). Though no multi-year field studies exist, a substantial number of predictive models (82% of total studies on climate change) attempted to disentangle the effects of long-term climate change on breeding habitat (Zipkin et al., 2012; Lemoine, 2015; Zalucki et al., 2015; Thogmartin et al., 2017b), overwintering conditions (Oberhauser and Peterson, 2003; Barve et al., 2012; Sáenz-Romero et al., 2012; Zalucki et al., 2015; Thogmartin et al., 2017b), and overall distribution (Batalden et al., 2007).
Climatic conditions are anticipated to change drastically overtime. In line with the temperature-dependent growth of monarchs (Zalucki, 1982), elevated temperatures are likely to positively affect larval growth and survival during the breeding season (Couture et al., 2015). Couture et al. (2015) predicted that larvae growth will increase under temperature- and water-stressed conditions, though it is unclear whether the shorter generation time will result in a greater number of generations overall during the breeding season. Beyond the direct effects on larval growth, models suggest climate change is anticipated to result in a northward expansion of the breeding range (Batalden et al., 2007; Lemoine, 2015) and that elevated temperatures (Zipkin et al., 2012) are likely to facilitate population growth. As such, other threats likely have a greater potential to drive monarch declines (Flockhart et al., 2015; Zalucki et al., 2015). Nonetheless, the effect of climate change on monarchs at overwintering sites in Mexico may contribute to lower population viability as rising temperatures may generate unsuitable conditions for diapause (Oberhauser and Peterson, 2003; Barve et al., 2012; Sáenz-Romero et al., 2012). Taken together, the majority of studies implied that the threat of climate change is likely to continue (73% of total studies on climate change, Oberhauser and Peterson, 2003; Batalden et al., 2007; Barve et al., 2012; Sáenz-Romero et al., 2012; Flockhart et al., 2015; Lemoine, 2015; Thogmartin et al., 2017b), but the analysis of multi-year datasets suggest that it may affect population viability (Zipkin et al., 2012; Flockhart et al., 2015); (Zalucki et al., 2015).
Deforestation in the Overwintering Range
The level and effect of deforestation is quantified in the peer-reviewed literature primarily by means of field studies (82%, Table 2A). Field observations and aerial surveys assessed the extent of forest canopy loss (Brower et al., 2002; Ramírez et al., 2003; García, 2001; Honey-Rosés et al., 2011; Navarrete et al., 2011; Champo-Jiménez et al., 2012; Vidal and Rendón-Salinas, 2014; Vidal et al., 2014), microclimate suitability (Anderson and Brower, 1996; Alonso-Mejía et al., 1997; Brower et al., 2009, 2011b) and predation levels (Alonso-Mejía et al., 1998) under changing forest conditions. Models (18%, Table 2A), relative to controlled studies (no studies, Table 2A), were used to quantify the likelihood that forest loss contributed to monarch butterfly declines.
All field studies and models suggested that forest loss is a likely contributor to declines in individual condition and population viability of monarch butterflies through its effect on available overwintering habitat (Brower et al., 2002; Ramírez et al., 2003; García, 2001; Honey-Rosés et al., 2011; Navarrete et al., 2011; Champo-Jiménez et al., 2012; Vidal and Rendón-Salinas, 2014; Vidal et al., 2014; Flockhart et al., 2015; Hunt and Tongen, 2017; Oberhauser et al., 2017) and suitable environment conditions (Anderson and Brower, 1996; Alonso-Mejía et al., 1997, 1998; Brower et al., 2009, 2011b). However, studies varied on their assessment of the potential future risk to monarch populations. Multi-year data sets and modeling experiments showed continued forest loss within the Monarch Butterfly Biosphere Reserve (Brower et al., 2002; Ramírez et al., 2003; García, 2001; Navarrete et al., 2011; Champo-Jiménez et al., 2012; Vidal and Rendón-Salinas, 2014; Vidal et al., 2014; Flockhart et al., 2015) that may increase the exposure of monarchs and therefore the probability of a mass mortality event (53%, Table 2C). Improving habitat protection and availability at overwintering sites in Mexico is also considered a potential means to reduce losses (Oberhauser et al., 2017). Yet, other studies suggested that, though illegal logging and deforestation likely contributes to monarch declines, it is not the primary driver (Flockhart et al., 2015; Hunt and Tongen, 2017) and further forest canopy losses would be required to significantly impact monarch populations (Hunt and Tongen, 2017). The results from the remaining studies (Brower et al., 2009, 2011b; Honey-Rosés et al., 2011; 35%, Table 2C, Anderson and Brower, 1996; Alonso-Mejía et al., 1997, 1998) did not suggest a continued threat from deforestation.
Exposure to Contaminants
The type of study assigned to peer-reviewed research papers investigating the effects of environmental contaminants varied depending on the nature of the contaminant. Control (56% of total studies on Bt) and field-based (44% of total studies on Bt) studies were principally used to assess the effects of Bt exposure from GM crops. Control studies (75% of total studies on insecticides) pre-dominated for work on insecticides and modeling experiments examined the effect of insecticides (13% of total studies on insecticides) and herbicides (100% of total studies on herbicides) on population abundance. Though, certain types of studies are notably absent for each contaminant, perhaps most importantly the lack of field-based studies on the effect of contaminants limits extrapolation of results to field-realistic scenarios. Further, the majority of studies did not investigate multi-year datasets (57%, Table 2C) and control and field studies were limited to individual chemicals without considering the wide-range of potential agrochemicals or their interactions.
Controlled laboratory experiments showed a negative effect of Bt on larval development and survival (Losey et al., 1999; Hansen Jesse and Obrycki, 2000; Stanley-Horn et al., 2001; Anderson et al., 2004, 2005; Dively et al., 2004) and reproduction (Tschenn et al., 2001), but effects were dependent on age (Hansen Jesse and Obrycki, 2000; Hellmich et al., 2001) and Bt-transformation event (i.e., specific occurrence of the uptake of genetic material via transformation of cells of Bt, Stanley-Horn et al., 2001). Studies also confirmed that range overlap with Bt-exposed fields (Oberhauser et al., 2001; Pleasants et al., 2001) could contribute to lower reproductive output (Stenoien et al., 2015), though larval mortality is not always associated with proximity to Bt-exposed fields (Zangerl et al., 2001). Similarly, insecticide use showed effects on individual survival (pyrethroids, Oberhauser et al., 2006, 2009; clothianidin, Pecenka and Lundgren, 2015, λ-cyhalothrin, Stanley-Horn et al., 2001) and herbicide application (i.e., glyphosate) is known to influence population size (Thogmartin et al., 2017b). However, the strength of the effects varied depending on the agrochemical (i.e., significant effects not shown for dicamba and 2,4-dichlorophenoxyacetic acid use, Thogmartin et al., 2017b), geographic location (Thogmartin et al., 2017b), and life stage (Pan et al., 2017). Overall, few studies suggested a potential future risk to monarch population viability from Bt-exposure (31% of total studies on Bt, Hansen Jesse and Obrycki, 2000; Pleasants et al., 2001; Dively et al., 2004; Stenoien et al., 2015), dicamba (33% of total studies on herbicides, Thogmartin et al., 2017b), 2,4-dichlorophenoxyacetic acid (33% of total studies on herbicides, Thogmartin et al., 2017b), and glyphosate (33% of total studies on herbicides, Thogmartin et al., 2017b) applications. Neonicotinoids also did not contribute significantly to monarch declines in a population model (25% of total studies on neonicotinoids, Thogmartin et al., 2017b).
Loss of Breeding Habitat
The effects of habitat availability and the influence of urbanization are rarely examined for their impacts on monarch butterflies. In fact, habitat fragmentation was only evaluated in the context of field studies of vehicular collisions or roadsides serving as ecological traps (McKenna et al., 2001; Mueller and Baum, 2014) and through 2 modeling experiments that examined the impact of fragmented landscapes on movement rate (Collingham and Huntley, 2000) and path (Grant et al., 2018). Likewise, only a single study investigated how the availability of nectar resources affected monarch population growth (Oberhauser et al., 2017). Projections on the threat of habitat fragmentation and urbanization were absent, but the reduction in nectar availability (Oberhauser et al., 2017) was suggested to pose a sustained risk.
The literature on optimal breeding habitat for monarch butterflies is dominated by studies on the potential declines in survival on different host plants (35% of total studies on breeding habitat loss) and the declines in common milkweed (Asclepias syriaca) in agricultural fields (47% of total studies on breeding habitat loss). Controlled laboratory experiments investigated the oviposition tendencies on different host plants and the effect on larval growth (DiTommaso and Losey, 2003; Mattila and Otis, 2003; Yeargan and Allard, 2005; Casagrande and Dacey, 2007; Pocius et al., 2017a,b), except for two studies that found higher oviposition on common and swamp milkweed (A. incarnata, Pocius et al., 2018) and greater numbers of immature larvae on tropical milkweed (A. curassavica; Malcolm and Brower, 1986) relative to other milkweed species. The effect of the loss of milkweed, principally on agricultural plots, was limited principally to field studies (40% of total studies on milkweed loss, Hartzler, 2010; Pleasants and Oberhauser, 2013; Inamine et al., 2016; Kasten et al., 2016; Zaya et al., 2017) and modeling experiments (60% of total studies on milkweed loss) relating overwintering population abundance to milkweed availability (Zalucki and Lammers, 2010; Flockhart et al., 2015; Zalucki et al., 2016; Hunt and Tongen, 2017; Oberhauser et al., 2017; Pleasants, 2017; Thogmartin et al., 2017a,c).
Studies provide competing evidence that select species of plants (e.g., dog-strangler vine (Cynanchum rossicum), swallow-worts (Vincetoxicum spp.) resulted in changes in oviposition tendencies (DiTommaso and Losey, 2003; Mattila and Otis, 2003; Casagrande and Dacey, 2007), and larval survival (Mattila and Otis, 2003). Larval survival also varied across milkweed species and was generally higher on common milkweed (Yeargan and Allard, 2005; Pocius et al., 2017a,b). Tropical milkweed posed a more substantial threat as a greater number of larvae are found on this species relative to common milkweed (Malcolm and Brower, 1986) and year-round availability may alter migration patterns (Satterfield et al., 2015, 2018). At the same time, declines in common milkweed was almost unanimously agreed upon as a threat to monarchs, with the exception of a 22-year study that showed monarch population size is predictable along the migratory route and monarchs are capable of recovering during the breeding season (Inamine et al., 2016; but see Pleasants et al., 2017). Studies did not evaluate the sustained risk of the use of different host plant species during oviposition. The threat imposed by milkweed loss (Hartzler, 2010; Pleasants and Oberhauser, 2013; Flockhart et al., 2015; Zalucki et al., 2016; Hunt and Tongen, 2017; Oberhauser et al., 2017; Pleasants, 2017; Pleasants et al., 2017; Thogmartin et al., 2017a,c; Zaya et al., 2017) to the eastern migratory North American population of monarch butterflies is anticipated to continue (80% in support from the total studies on milkweed loss), though present-day loss may occur at a lower rate than historical levels (Pleasants, 2017).
Predation, Parasitism, and Species-Specific Pathogens
Predation and parasitism events experienced by monarch butterflies were recorded in controlled experiments (34%, Table 2A) and in field observations (60%, Table 2A). A variety of species were noted to prey upon monarch butterflies (e.g., flies, Arnaud, 1978; spiders, Borkin, 1982; orioles and grosbeaks, Fink and Brower, 1981). Moreover, a single quantitative risk assessment model determined the impact of the Asian lady beetle (Harmonia axyridis) on monarch butterflies (Koch et al., 2006). The effect of OE on body condition (Altizer and Oberhauser, 1999), flight capacity (Bradley and Altizer, 2005), reproduction (Altizer and Oberhauser, 1999), survival (Altizer and Oberhauser, 1999), and virulence, spore load and transmission (Leong et al., 1997; de Roode et al., 2008a,b, 2009; de Roode and Altizer, 2010; Satterfield et al., 2015) was principally quantified using controlled experiments (63% of total studies on OE), but OE detection was also available through in-field observations (32% of total studies on OE, Urquhart, 1966; Leong et al., 1992). Thogmartin et al. (2017b) provided the sole instance modeling the effect of OE on population size. In-field observations based on counts during migration determined the potential for migratory culling (80% of total studies on migratory culling, Altizer et al., 2000; Bartel et al., 2011; Badgett and Davis, 2015) and a single model determined that there was not a disconnect between monarch population estimates on summering and overwintering grounds, as would be predicted for migratory culling (20% of total studies on migratory culling; Pleasants et al., 2017).
A negative effect of predation and parasitism was found in all peer-review literature studying such events (94%, Table 2B), but few papers examined multi-year datasets or modeled the potential for the threat to pose future risk to the eastern migratory North American population of monarch butterflies (85%, Table 2C). Of those available, parasitism by OE was likely to have a continued negative effect, particularly with the increased availability of year-round tropical milkweed in the South (Satterfield et al., 2015, 2018; Thogmartin et al., 2017b). Though migratory culling due to OE infection may reduce population abundance at overwintering sites (Altizer et al., 2000; Bartel et al., 2011), other studies suggested this is unlikely the case (Pleasants et al., 2017). Badgett and Davis (2015) also highlight that monarch population abundance at survey sites in Michigan remained constant from 1996 to 2014, potentially due to the high concentration of monarchs in this region that were born in the Upper Peninsula and Canada, but also suggesting that larval survival during the breeding season could offset losses observed at overwintering sites.
Discussion
Our review focused on five broad threats to the eastern migratory North American population of monarch butterflies and highlights the dynamic factors that influence monarch butterfly reproduction and survival at different stages of their life cycle and throughout their range. Though evidence exists in support of each threat contributing to the declines in the eastern population of monarch butterflies, based on the potential future risk, we suggest that the change in suitable environmental conditions, specifically that related to climate change, and habitat loss on overwintering (i.e., via deforestation) and breeding grounds are likely the greatest threats.
For each threat, the most common methodology applied was somewhat different. Projections on the decline in the availability of suitable of environmental conditions were evaluated using models (73%, Table 2A) estimating range expansion and optimal abiotic conditions under climate change scenarios for both monarchs and their host plant. Studies quantifying deforestation, as expected, principally used observational field methods (82%, Table 2A). Models (18%, Table 2A) were then used to associate rates of loss and degradation to declines in monarch abundance. The toxicological effects of contaminants on monarch butterflies were principally evaluated using controlled designs (55%, Table 2A) and models (14%, Table 2A), though few studies were conducted and only 9 agrochemicals (i.e., herbicides and insecticides) were assessed. Study type was equally weighted in evaluating the impact and requirements needed for restoration of breeding habitat, though variability existed when assessing the influence of different host species ([Control_data]: 82%, [Field_data]: 18% of total studies on host plant species). More, field studies and models contributed the most in research on the effects of milkweed loss ([Field_data]: 40%, [Mod]: 60% of total studies on milkweed loss). Finally, the effect of predation, parasites, and pathogens on monarch butterflies was determined primarily through field observations (60%, Table 2A), though the effect OE was quantified through controlled experiments (63% of total studies on OE).
Based on the current literature on potential threats in the declines of the eastern migratory North American population of monarch butterflies, the availability of suitable environmental conditions (i.e., climate change) and overwintering and breeding habitat loss arguably have the greatest impact on population viability and potential for continuing risk to monarch populations (Brower et al., 2011a). However, some threats are understudied and should not be discounted in their potential impact to the population. Contaminant exposure may also potentially drive declines based on evidence of the toxicological effects and potential for cumulative sublethal effects, but it is unknown whether the threat will rise given current high level of agrochemical use (Thogmartin et al., 2017b). Risk imposed from predation is also likely to continue given the interaction with climate warming and potential year-round residency by monarchs in the southern US (Sáenz-Romero et al., 2012; Satterfield et al., 2015, 2018).
The five threats highlighted in our review vary temporally (e.g., early vs. late migrants) and spatially (e.g., migrants vs. breeding populations) in their imposed risk. For instance, while exposure to Bt pollen generally reduces survival (Hansen Jesse and Obrycki, 2000; Dively et al., 2004), threat level may be minimized if larval populations do not occur at the same time as pollen shedding (Bartholomew and Yeargan, 2001) and/or contact with toxins is reduced during early development (Hansen Jesse and Obrycki, 2000; Sears et al., 2001). Each factor could interact synergistically, with the strength of effects varying over time. As the availability of milkweed declines around crops, the risk imposed by exposure to agricultural chemicals (e.g., Bt, insecticides, herbicides) is likely to decline in tandem, though no studies have assessed this long-term change. Interactions between threats may also vary in accordance with the pre-dominant threats in a particular region. For instance, climate change may result in phenological mismatch (e.g., milkweed availability during oviposition and nectar sources during breeding and migration) if environmental conditions drive changes in plant growth or the pattern of monarch butterfly migration. Simultaneously, if, as in other species (i.e., honeybees, Mason et al., 2013; Sánchez-Bayo et al., 2015), agrochemical exposure reduces immune system function, the potential elevated risk of exposure to pathogens with climate warming (Altizer et al., 2011) may reduce survival. Thus, a complex array of factors and their interactions must be examined with different methodological protocols to resolve how each potential threat contributes to declines.
Our results are based on the available published peer-reviewed literature, but bias may exist in the publication and dissemination of research that may unintentionally affect meta-analyses and systematic reviews. Though we conducted an extensive literature review, recently completed, unpublished literature may not yet be accessible and thus is unable to be accounted for in the results (Møller and Jennions, 2001). Further, publication bias during the submission, review, and editorial processes may also influence the likelihood of research being available and accessed (Møller and Jennions, 2001). Not only are significant results more likely to be submitted, but novel research with large sample sizes and greater statistical power are more likely to be published (Tregenza, 2002; Joober et al., 2012; Mlinarić et al., 2017). Interestingly, publication record and identity of the author (e.g., gender, nationality, non-English surnames, alphabetical position of the surname in reference list) can also affect the likelihood of publication and subsequent citation rate (Tregenza and Wedell, 1997; Kothiaho, 1999a,b; Møller and Jennions, 2001; Einac and Yariv, 2006). The purpose of the research (e.g., natural history or multi-year modeling experiments) may also influence results. For instance, natural history studies on the effects of predators, parasites, and pathogens highlight the threat to monarchs, but were not intended as long-term studies and we therefore cannot extrapolate from these initial results. As research continues to expand reviews will need to incorporate new knowledge to properly evaluate the strength of evidence and potential threats to the eastern migratory North American population of monarch butterflies.
The threats examined in this review also pose considerable risk to other insect species. We suggest that the monarch butterfly is an ideal candidate to evaluate the contribution and the spatiotemporal interactions of each threat at different stages along the migratory route. Research should contribute to effective conservation management plans aimed at protecting habitat and raising population abundance, while also emphasizing the importance of international cooperation in the protection of species at risk. To accomplish this, studies should tackle questions in an interdisciplinary manner, taking a whole-systems approach, and integrate multiple biological disciplines that address major gaps in methodological procedures (i.e., type of study) and knowledge. An integrated approach to understanding the mechanisms underlying declines will be important in mitigating further losses under escalating and interacting threats and will be vital to developing management responses.
Conclusion
In this review, we sort and summarize 115 peer-reviewed research papers based on the type of study existing within five broad potential threats, evaluating the effect and potential risk imposed by each threat to the eastern migratory North American population of monarch butterflies. We recommend that research initiatives address hypotheses examining the spatiotemporal nature of each risk and how each factor interacts by integrating fields spanning a range of biological disciplines including, though not limited to, ecology, physiology, endocrinology, and conservation management. Without thorough knowledge and management action plans, declines in monarch butterflies may have unintended downstream effects. For example, butterflies are valued for their cultural economic services and socioeconomic benefits in terms of ecotourism in the southern portion of their range (Semmens et al., 2018). We encourage more detailed studies on the mechanisms driving declines, particularly those evaluating the relative contribution of each threat throughout the monarch life cycle and its geographic distribution. We also suggest that studies investigate potential interacting factors that may limit capacity to implement conservation management plans.
Author Contributions
The study was designed and conceived by all authors. AW wrote the initial draft of the manuscript and all other authors contributed to writing. All authors gave final approval to the revisions and approval for publication.
Funding
This study is part of AW's Ph.D. thesis and was supported by scholarships from the University of Guelph and the Natural Sciences and Engineering Research Council (NSERC, Canada).
Conflict of Interest Statement
The authors declare that the research was conducted in the absence of any commercial or financial relationships that could be construed as a potential conflict of interest.
Supplementary Material
The Supplementary Material for this article can be found online at: https://www.frontiersin.org/articles/10.3389/fevo.2019.00099/full#supplementary-material
References
Allen-Wardell, G., Berhardt, P., Bitner, R., Burquez, A., Buchmann, S., Cane, J., et al. (1998). The potential consequences of pollinator declines on the conservation of biodiversity and stability of food crop yields. Conserv. Bio. 12, 8–17. doi: 10.1111/j.1523-1739.1998.97154.x
Alonso-Mejía, A., Montesinos-Patiño, E., Rendon-Salinas, E., Brower, L. P., and Oyama, K. (1998). Influence of forest canopy closure on rates of bird predation on overwintering Monarch butterflies Danaus plexippus L. Biol. Conserv. 85, 151–159. doi: 10.1016/S0006-3207(97)00134-1
Alonso-Mejía, A., Rendon-Salinas, E., Montesinos-Patiño, E., and Brower, L. P. (1997). Use of lipid reserves by monarch butterflies overwintering in Mexico: implications for conservation. Ecol. Appl. 7, 934–947. doi: 10.1890/1051-0761(1997)007[0934:UOLRBM]2.0.CO;2
Altizer, S., Bartel, R., and Han, B. A. (2011). Animal migration and infectious disease risk. Science 331, 296–302. doi: 10.1126/science.1194694
Altizer, S. M., and Oberhauser, K. S. (1999). Effect of the protozoan parasite Ophryocystis elektroscirrha on the fitness of monarch butterflies (Danaus plexippus). J. Invertebr. Pathol. 74, 76–88. doi: 10.1006/jipa.1999.4853
Altizer, S. M., Oberhauser, K. S., and Brower, L. P. (2000). Associations between host migration and the prevalence of a protozoan parasite in natural populations of adult monarch butterflies. Ecol. Entomol. 25, 125–139. doi: 10.1046/j.1365-2311.2000.00246.x
Anderson, J. B., and Brower, L. P. (1996). Freeze-protection of overwintering monarch butterflies in Mexico: critical role of the forest as a blanket and an umbrella. Ecol. Entomol. 21, 107–116. doi: 10.1111/j.1365-2311.1996.tb01177.x
Anderson, P. L., Hellmich, R. L., Prasifka, J. R., and Lewis, L. C. (2004). Effects of Cry1Ab-expressing corn anthers on monarch butterfly larvae. Environ. Entomol. 33, 1109–1115. doi: 10.1603/0046-225X-33.4.1109
Anderson, P. L., Hellmich, R. L., Prasifka, J. R., and Lewis, L. C. (2005). Effects on fitness and behavior of monarch butterfly larvae exposed to a combination of Cry1Ab-expressing corn anthers and pollen. Environ. Entomol. 34, 944–952. doi: 10.1603/0046-225X-34.4.944
Arnaud, P. H. Jr. (1978). A Host-Parasite Catalog of North American Tachinidae (Diptera). Publication no. 1319. Washington, DC: US Department of Agriculture, Science and Education Administration.
Badgett, G., and Davis, A. K. (2015). Population trends of monarchs at a northern monitoring site: analyses of 19 years of fall migration. Ann. Entomol. Soc. Am. 108, 700–706. doi: 10.1093/aesa/sav060
Bartel, R. A., Oberhauser, K. S., de Roode, J. C., and Altizer, S. M. (2011). Monarch butterfly migration and parasite transmission in eastern North America. Ecology 92, 342–351. doi: 10.1890/10-0489.1
Bartholomew, C. S., and Yeargan, K. V. (2001). Phenology of milkweed (Asclepiadaceae) growth and monarch (Lepidoptera: Nymphalidae) reproduction in Kentucky and ovipositional preference between common and honeyvine milkweed. J. Kansas Entomol. Soc. 74, 211–220.
Barve, N., Bonilla, A. J., Brandes, J., Brown, J. C., Brunsell, N., Cochran, F. V., et al. (2012). Climate-change and mass mortality events in overwintering monarch butterflies. Rev. Mex. Biodivers. 83, 817–824. doi: 10.7550/rmb.26460
Batalden, R. V., Oberhauser, K., and Peterson, A. T. (2007). Ecological niches in sequential generations of eastern North American monarch butterflies (Lepidoptera: Danaidae): the ecology of migration and likely climate change implications. Ecol. Appl. 36, 1365–1373. doi: 10.1603/0046-225X(2007)36[1365:ENISGO]2.0.CO;2
Bechshoft, T., Derocher, A. E., Viengkone, M., Routti, H., Aars, J., Letcher, R. J., et al. (2017). On the integration of ecological and physiological variables in polar bear toxicology research: a systematic review. Environ. Rev. 26, 1–12. doi: 10.1139/er-2016-0118
Borkin, S. S. (1982). Notes on shifting distribution patterns and survival of immature Danaus plexippus (Lepidoptera: Danaidae) on the food plant Asclepias syriaca. Great Lakes Entomol. 15, 199–206.
Bradley, C. A., and Altizer, S. (2005). Parasites hinder monarch butterfly flight: implications for disease spread in migratory hosts. Ecol. Lett. 8, 290–300. doi: 10.1111/j.1461-0248.2005.00722.x
Brower, L. P. (1995). Understanding and misunderstanding the migration of the monarch butterfly (Nymphalidae) in North America: 1857–1995. J. Lepid. Soc. 49, 304–385.
Brower, L. P., and Calvert, W. H. (1985). Foraging dynamics of bird predators on overwintering monarch butterflies in Mexico. Evolution 39, 852–868. doi: 10.2307/2408685
Brower, L. P., Castilleja, G., Peralta, A., Lopez-Garcia, J., Bojorquez-Tapia, L., Diaz, S., et al. (2002). Quantitative changes in forest quality in a principal overwintering area of the monarch butterfly in Mexico, 1971-1999. Conserv. Biol. 16, 346–359. doi: 10.1046/j.1523-1739.2002.00572.x
Brower, L. P., Fink, L. S., Kiphart, R. J., Pocius, V., Zubieta, R. R., and Ramírez, M. I. (2015). “Effect of the 2010-2011 drought on the lipid content of monarchs migrating through Texas to overwintering sites in Mexico,” in Monarchs in a Changing World: Biology and Conservation of an Iconic Butterfly, eds K. S. Obserhauser, K. R. Nail, and S. Altizer (Ithaca, NY: Cornell University Press), 117–129.
Brower, L. P., Taylor, O. R., Williams, E. H., Slayback, D. A., Zubieta, R. R., and Ramíerz, M. I. (2011a). Decline of monarch butterflies overwintering in Mexico: is the migratory phenomenon at risk? Insect Conserv. Divers. 5, 95–100. doi: 10.1111/j.1752-4598.2011.00142.x
Brower, L. P., Williams, E. H., Fink, L. S., Slayback, D. A., Ramírez, M. I., Ván Limón García, M., et al. (2011b). Overwintering clusters of monarch butterfly coincide with the least hazardous vertical temperatures in the Oyamel Forest. J. Lepid.' Soc. 65, 27–46. doi: 10.18473/lepi.v65i1.a3
Brower, L. P., Williams, E. H., Jaramillo-López, P., Kust, D. R., Slayback, D. A., and Ramírez, M. I. (2017). Butterfly mortality and salvage logging from the March 2016 storm in the Monarch Butterfly Biosphere Reserve in Mexico. Am. Entomol. 63, 151–164. doi: 10.1093/ae/tmx052
Brower, L. P., Williams, E. H., Slayback, D. A., Fink, L. S., Ramírez, M. I., Zubieta, R. R., et al. (2009). Oyamel fir forest trunks provide thermal advantages for overwintering monarch butterflies in Mexico. Insect Conserv. Divers. 2, 163–175. doi: 10.1111/j.1752-4598.2009.00052.x
Burghardt, K. T., and Tallamy, D. W. (2015). Not all non-natives are equally unequal: reductions in herbivore β-diversity depend on phylogenetic similarity to native plant community. Ecol. Lett. 18, 1087–1098. doi: 10.1111/ele.12492
Calvert, W. H. (1996). Fire ant predation on monarch larvae (Nymphalidae: Danainae) in a central Texas prairie. J. Lepid. Soc. 50, 149–151.
Calvert, W. H. (2004). “The effects of fire ants on monarchs breeding in Texas,” in The Monarch Butterfly: Biology and Conservation, eds K. S. Obserhauser and M. J. Solensky (Ithaca, NY: Cornell University Press), 47–53.
Calvert, W. H., Hedrick, L. E., and Brower, L. P. (1979). Mortality of the monarch butterfly (Danaus plexippus L.): avian predation at five overwintering sites at Mexico. Science 204, 847–851. doi: 10.1126/science.204.4395.847
Carlón-Allende, T., Villaneuva-Días, J., Mendoza, M. E., and Pérez-Salicrup, D. R. (2018). Climatic signal in earlywood and latewood in conifer forests in the monarch butterfly biosphere reserve, Mexico. Tree Ring Res. 74, 63–75. doi: 10.3959/1536-1098-74.1.63
Casagrande, R. A., and Dacey, J. E. (2007). Monarch butterfly oviposition on swallow-worts (Vincetoxicum spp.). Environ. Entomol. 36, 631–636. doi: 10.1603/0046-225X(2007)36[631:MBOOSV]2.0.CO;2
Champo-Jiménez, O., Valderrama-Landeros, L., and España-Boquera, M. L. (2012). Forest cover loss in the monarch butterfly biosphere reserve, Michoacán, México (2006–2010). Ev. Chapingo Ser. Cie 18, 143–157. doi: 10.5154/r.rchscfa.2010.09.074
Collingham, Y. C., and Huntley, B. (2000). Impacts of habitat fragmentation and patch size upon migration rates. Ecol. Appl. 10, 131–144. doi: 10.1890/1051-0761(2000)010[0131:IOHFAP]2.0.CO;2
Couture, J. J., Serbin, S. P., and Townsend, P. A. (2015). Elevated temperature and periodic water stress alter growth and quality of common milkweed (Asclepias syriaca) and monarch (Danaus plexippus) larval performance. Arthropod. Plant Interact. 9, 149–161. doi: 10.1007/s11829-015-9367-y
Davis, A. K. (2012). Are migratory monarchs really declining in eastern North America? Examining evidence from two fall census programs. Insect Conserv. Divers. 5, 101–105. doi: 10.1111/j.1752-4598.2011.00158.x
Davis, A. K., Schroeder, H., Yeager, I., and Pearce, J. (2018). Effects of simulated highway noise on heart rates of larval monarch butterflies, Danaus plexippus: implications for roadside habitat suitability. Biol. Lett. 14:20180018. doi: 10.1098/rsbl.2018.0018
de Roode, J. C., and Altizer, S. (2010). Host-parasite genetic interactions and virulence-transmission relationships in natural populations of monarch butterflies. Evolution 64, 502–514. doi: 10.1111/j.1558-5646.2009.00845.x
de Roode, J. C., Chi, J., Rarick, R. M., and Altizer, S. (2009). Strength in numbers: high parasite burdens increase transmission of a protozoan parasite of monarch butterflies (Danaus plexippus). Oecologia 161, 67–75. doi: 10.1007/s00442-009-1361-6
de Roode, J. C., Gold, L. R., and Altizer, S. (2007). Virulence determinants in a natural butterfly-parasite system. Parasiotology 134, 657–668. doi: 10.1017/S0031182006002009
de Roode, J. C., Pedersen, A. B., Hunter, M. D., and Altizer, S. (2008a). Host plant species affects virulence in monarch butterfly parasites. J. Anim. Ecol. 77, 120–126. doi: 10.1111/j.1365-2656.2007.01305.x
de Roode, J. C., Rarick, R. M., Mongue, A. J., Gerardo, N. M., and Hunter, M. D. (2011). Aphids indirectly increase virulence and transmission potential of a monarch butterfly parasite by reducing defensive chemistry of a shared food plant. Ecol. Lett. 14, 453–461. doi: 10.1111/j.1461-0248.2011.01604.x
de Roode, J. C., Yates, A. J., and Altizer, S. (2008b). Virulence-transmission trade-offs and population divergence in virulence in a naturally occurring butterfly parasite. Proc. Natl. Acad. Sci. USA. 105, 7489–7494. doi: 10.1073/pnas.0710909105
Didham, R. K., Ghazoul, J., Stork, N. E., and Davis, A. J. (1996). Insects in fragmented forests: a functional approach. Trends Ecol. Evol. 11, 255–260. doi: 10.1016/0169-5347(96)20047-3
Dirzo, R., Toung, H. S., Galetti, M., Ceballos, G., Issac, N. J. B., and Collen, B. (2014). Defaunation in the Anthropocene. Science 245, 401–406. doi: 10.1126/science.1251817
DiTommaso, A., and Losey, J. E. (2003). Oviposition preference and larval performance of monarch butterflies (Danaus plexippus) on two invasive swallow-wort species. Entomol. Exp. Appl. 108, 205–209. doi: 10.1046/j.1570-7458.2003.00089.x
Dively, G. P., Rose, R., Sears, M. K., Hellmich, R. L., Stanley-Horn, D. E., Calvin, D. D., et al. (2004). Effects on monarch butterfly larvae (Lepidoptera: Danaidae) after continuous exposure to Cry1Ab-expressing corn during anthesis. Environ. Entomol. 33, 1116–1125. doi: 10.1603/0046-225X-33.4.1116
Einac, L., and Yariv, L. (2006). What's in a surname? The effect of surname initials on academic success. J. Econom. Perspect. 20, 175–188. doi: 10.1257/089533006776526085
Fattorini, S. (2011). Insect extinction by urbanization: a long term study in Rome. Biol. Conserv. 144, 370–375. doi: 10.1016/j.biocon.2010.09.014
Fink, L. S., and Brower, L. P. (1981). Birds can overcome cardenolide defense of monarch butterflies in Mexico. Nature 291, 67–70. doi: 10.1038/291067a0
Fink, L. S., Brower, L. P., Waide, R. B., and Spitzer, P. R. (1983). Overwintering monarch butterflies as food for insectivorous birds in Mexico. Biotropica 15, 151–153. doi: 10.2307/2387962
Flockhart, D. T., Pichancourt, J. B., Norris, D. R., and Martin, T. G. (2015). Unravelling the annual cycle in a migratory animal: breeding-season habitat loss drives population declines of monarch butterflies. J. Anim. Ecol. 84, 155–165. doi: 10.1111/1365-2656.12253
Flockhart, D. T., Wassenaar, L. I., Martin, T. G., Hobson, K. A., Wunder, M. B., and Norris, D. R. (2013). Tracking multi-generational colonization of the breeding grounds by monarch butterflies in eastern North America. Proc. R. Soc. B 280:20131087. doi: 10.1098/rspb.2013.1087
Flockhart, D. T. T., Dabydeen, A., Satterfield, D. A., Hobson, K. A., Wassenaar, L. I., and Norris, D. R. (2018). Patterns of parasitism in monarch butterflies during the breeding season in eastern North America. Ecol. Entomol. 43, 28–36. doi: 10.1111/een.12460
García, J. L. (2001). Deforestation and forest degradation in the Monarch Butterfly Biosphere Reserve, Mexico, 2003–2009. J. Maps 7, 626–633. doi: 10.4113/jom.2011.1163
Geest, E. A., Wolfenbarger, L. L., and McCarty, J. P. (2018). Recruitment, survival, and parasitism of monarch butterflies (Danaus plexippus) in milkweed gardens and conservation areas. J. Insect Conserv. 22, 1–14. doi: 10.1007/s10841-018-0102-8
Godfray, H. C., Blacquiére, T., Field, L. M., Hails, R. S., Petrokofsky, G., Potts, S. G., et al. (2014). A restatement of the natural science evidence base concerning neonicotinoid insecticides and insect pollinators. Proc. R. Soc. B 281:20140558. doi: 10.1098/rspb.2014.0558
Godfray, H. C., Donnelly, C. A., Kao, R. R., Macdonald, D. W., McDonald, R. A., Petrokofsky, G., et al. (2013). A restatement of the natural science evidence base relevant to the control of bovine tuberculosis in Great Britain. Proc. R. Soc. B 280:20131634. doi: 10.1098/rspb.2013.1634
Grant, T. J., Parry, H. R., Zalucki, M. P., and Bradbury, S. P. (2018). Predicting monarch butterfly (Danaus plexippus) movement and egg-laying with a spatially-explicit agent-based model: The role of monarch perceptual range and spatial memory. Ecol. Modell. 374, 37–50. doi: 10.1016/j.ecolmodel.2018.02.011
Hansen Jesse, L. C., and Obrycki, J. J. (2000). Field deposition of Bt transgenic corn pollen: lethal effects on the monarch butterfly. Oecologia 125, 241–248. doi: 10.1007/s004420000502
Hartzler, R. G. (2010). Reduction in common milkweed (Asclepias syriaca) occurrence in Iowa cropland from 1999 to 2009. Crop Protect. 29, 1542–1544. doi: 10.1016/j.cropro.2010.07.018
Hellmich, R. L., Siegfried, B. D., Sears, M. K., Stanley-Horn, D. E., Daniels, M. J., Mattila, H. R., et al. (2001). Monarch larvae sensitivity to Bacillus thuringiensis-purified proteins and pollen. Proc. Natl. Acad. Sci. USA. 98, 11925–11930. doi: 10.1073/ypnas.211297698
Honey-Rosés, J., Baylis, K., and Ramírez, M. I. (2011). A spatially explicit estimate of avoided forest loss. Conserv. Biol. 25, 1032–1043. doi: 10.1111/j.1523-1739.2011.01729.x
Hunt, E., and Tongen, A. (2017). A periodic matrix population model for monarch butterflies. Nat. Resour. Model. 2017:e12123. doi: 10.1111/nrm.12123
Inamine, H., Ellner, S. P., Springer, J. P., and Agrawal, A. A. (2016). Linking the continental migratory cycle of the monarch butterfly to understand its population decline. Oikos 125, 1081–1091. doi: 10.1111/oik.03196
Jennions, M. D., and Møller, A. P. (2002). Publication bias in ecology and evolution: an empirical assessment using the ‘trim and fill’ method. Biol. Rev. 77, 211–222. doi: 10.1017/S1464793101005875
Jesse, L. C. H., and Obrycki, J. J. (2004). “Survival of experimental cohorts of monarch larvae following exposure to transgeneic Bt corn pollen and anthers,” in The Monarch Butterfly: Biology and Conservation, eds. K. S. Obserhauser and M. J. Solensky (Ithaca, NY: Cornell University Press), 69–75.
Joober, R., Schmitz, N., Annable, L., and Boksa, P. (2012). Publication bas: what are the challenges and can they be overcome. J. Psychiatry Neurosci. 37, 149–152. doi: 10.1503/jpn.120065
Kasten, K., Stenoien, C., Caldwell, W., and Oberhauser, K. S. (2016). Can roadside habitat lead monarchs on a recovery route? J. Insect. Conserv. 20, 1047–1057. doi: 10.1007/s10841-016-9938-y
Koch, R. L., Hutchison, W. D., and Venette, R. C. (2003). Survival of monarch butterfly, Danaus plexippus (Nymphalidae), larvae on milkweed near Bt cornfields. J. Lepid. Soc. 57, 92–99.
Koch, R. L., Vemette, R. C., and Hutchison, W. D. (2005). Influence of alternate prey on predation of monarch butterfly (Lepidoptera: Nymphalidae) larvae by the multicolored Asian lady beetle (Coleoptera: Coccinellidae). Environ. Entomol. 34, 410–416. doi: 10.1603/0046-225X-34.2.410
Koch, R. L., Venette, R. C., and Hutchison, W. D. (2006). Predicted impact of an exotic generalist predator on monarch butterfly (Lepidoptera: Nymphalidae) populations: a quantitative risk assessment. Biol. Invas. 8, 1179–1193. doi: 10.1007/s10530-005-5445-x
Kothiaho, J. S. (1999a). Papers vanish in mis-citation black hole. Nature 398:19. doi: 10.1038/17898
Kothiaho, J. S., Tomkins, J. L., and Simmons, L. W. (1999b). Unfamiliar citations breed mistakes. Nature 400:307. doi: 10.1038/22405
Krischik, V., Rogers, M., Gupta, G., and Varshney, A. (2015). Soil-applied imidacloprid translocates to ornamental flowers and reduces survival of adult Coleomegilla maculata, Harmonia axyridis, and Hippodamia convergens Lady Beetles, and larval Danaus plexippus and Vanessa cardui butterflies. PLoS ONE 10:e0119133. doi: 10.1371/journalpone.0119133
Ladner, D. T., and Altizer, S. M. (2003). Oviposition preference and larval performance of North American monarch butterflies on four Asclepias species. Entomol. Exp. Appl. 108, 205–209. doi: 10.1111/j.1570-7458.2005.00308.x
Lemoine, N. P. (2015). Climate change may alter breeding ground distributions of eastern migratory monarchs (Danaus plexippus) via range expansion of Ascelpias host plants. PLoS ONE 10:e0118614. doi: 10.1371/journal.pone.0118614
Lenhard, S. C., and Witter, J. A. (1977). Insects as biological indicators of environmental change. Bull. Entomol. Soc. Am. 23, 191–193. doi: 10.1093/besa/23.3.191
Leong, K. L., Yoshimura, M. A., Kaya, H. K., and Williams, H. (1997). Instar susceptibility of the monarch butterfly (Danaus plexippus) to the neogregarine parasite, Ophryocystis elektroscirrha. J. Invertebr. Pathol. 69, 79–83. doi: 10.1006/jipa.1996.4634
Leong, K. L. H., Kaya, H. K., Yoshimura, M. A., and Frey, D. F. (1992). The occurrence and effect of a protozoan parasite, Ophryocystis elektroscirrha (Neogregarinida: Ophryocystidae) on overwintering monarch butterflies, Danaus plexippus (Lepidoptera: Danaidae) from two California winter sites. Ecol. Entomol. 17, 338–342. doi: 10.1111/j.1365-2311.1992.tb01067.x
Lindsey, E., Mehta, M., Dhulipala, V., Oberhauser, K., and Altizer, S. M. (2009). Crowding and disease: effects of host density on response to infection in a butterfly-parasite interaction. Ecol. Entomol. 34, 551–561. doi: 10.1111/j.1365-2311.2009.01107.x
Losey, J. E., Rayor, L. S., and Carter, M. E. (1999). Transgenic pollen harms monarch larvae. Nature 399:214. doi: 10.1038/20338
Lynch, S. P., and Martin, R. A. (1993). “Milkweed host plant utilization and crdenolooide sequestration by monarch butterflies in Louisiana and Texas,” in Biology and Conservation of the Monarch Butterfly, eds. K. S. B. Malcolm and M. P. Zalucki (Los Angeles, CA: Natural History Museum of Los Angeles County), 107–124.
Malcolm, S. B. (2017). Anthropogenic impacts on mortality and population viability of the monarch butterfly. Ann. Rev. Entomol. 63, 277–302. doi: 10.1146/annurev-ento-020117-043241
Malcolm, S. B., and Brower, L. P. (1986). Selective oviposition by monarch butterflies (Danaus plexippus L.) in a mixed stand of Asclepias curassavica L. and A. incarnata L. in South Florida. J. Lepid. Soc. 40, 255–263.
Mason, R., Tennekes, H., Sánchez-Bayo, F., and Jepsen, P. U. (2013). Immune suppression by neonicotinoid insecticides at the root of global wildlife declines. J. Environ. Immune. Toxicol. 1, 3–12. doi: 10.7178/jeit.1
Mattila, H. R., and Otis, G. W. (2003). A comparison of the host preference of monarch butterflies (Danaus plexippus) for milkweed (Asclepias syriaca) over dog-strangler vine (Vincetoxicum rossicum). Entomol. Exp. Appl. 107, 193–199. doi: 10.1046/j.1570-7458.2003.00049.x
McKenna, D. D., Malcolm, S. B., and Berenbaum, M. R. (2001). Mortality of Lepidoptera along roadways in central Illinois. J. LEpid. Soc. 55, 63–68.
Mlinarić, A., Horvat, M., and Šupak Smolcić, V (2017). Dealing with the positive publication bias: why you should really publish your negative results. Biochem. Med. 27:074005. doi: 10.11613/BM.2017.030201
Møller, A. P., and Jennions, M. D. (2001). Testing and adjusting for publication bias. Trends Ecol. Evol. 16, 580–586. doi: 10.1016/S0169-5347(01)02235-2
Mooney, K. A., and Agrawal, A. A. (2008). Plant genotype shapes ant-aphid interactions: implications for community structure and indirect plant defense. Am Nat. 171, 195–205. doi: 10.1086/587758
Mueller, E. K., and Baum, K. A. (2014). Monarch–parasite interactions in managed and roadside prairies. J. Insect. Conserv. 18, 847–853. doi: 10.1007/s10841-014-9691-z
Navarrete, J. L., Ramírezz, M. I., and Pérez-Salicrup, D. R. (2011). Logging within protected areas: spatial evaluation of the monarch butterfly biosphere reserve, Mexico. Forest Ecol. Manag 262, 646–654. doi: 10.1016/j.foreco.2011.04.033
Nummelin, M., Modenius, M., Tulisalo, E., Hironen, H., and Alanko, T. (2007). Predatory insects as bioindicators of heavy metal pollution. Environ. Pollut. 145, 339–347. doi: 10.1016/j.envpol.2006.03.002
Oberhauser, K. (2012). Tachinid flies and monarch butterflies: citizen scientists document parasitism patterns over broad spatial and temporal scales. Am. Entomol. 58, 19–22. doi: 10.1093/ae/58.1.0019
Oberhauser, K., and Peterson, A. T. (2003). Modeling current and future potential wintering distributions of eastern North American monarch butterflies. Proc. Natl. Acad. Sci. USA. 100, 14063–14068. doi: 10.1073/pnas.2331584100
Oberhauser, K., Wiederholt, R., Diffendorfer, J. E., Semmes, D., Ries, L., Thogmartin, W. E., et al. (2017). A trans-national monarch butterfly population model and implications for regional conservation priorities. Ecol. Entomol. 42, 51–60. doi: 10.1111/een.12351
Oberhauser, K. S., Anderson, M., Anderson, S., Caldwell, W., de Anda, A., Hunter, M., et al. (2015). “Lacewings, wasps, and flies – oh my,” in Monarchs in a Changing World: Biology and Conservation of an Iconic Butterfly, eds K. S. Obserhauser, K. R. Nail, and S. Altizer (Ithaca, NY: Cornell University Press), 71–82.
Oberhauser, K. S., Brinda, S. J., Weaver, S., Moon, R. D., Manweiler, S. A., and Read, N. (2006). Growth and survival of monarch butterflies (Lepidoptera: Danaidae) after exposure to permethrin barrier treatments. Environ. Entomol. 35, 1626–1634. doi: 10.1093/ee/35.6.1626
Oberhauser, K. S., Gebhard, I., Cameron, C., and Oberhauser, S. (2007). Parasitism of monarch butterflies (Danaus plexippus) by Lespesia archippivora (Diptera: Tachinidae). Am. Midl. Nat. 157, 312–328. doi: 10.1674/0003-0031(2007)157[312:POMBDP]2.0.CO;2
Oberhauser, K. S., Maneiler, S. A., Lelich, R., Blank, M., Batalden, R. V., and de Anda, A. (2009). Impacts of ultra-low volume resmethrin applications on non-target insects. J. Am. Mosq. Control Assoc. 25, 83–93. doi: 10.2987/08-5788.1
Oberhauser, K. S., Psyby, M. D., Mattila, H. R., Stanley-Horn, D. E., Sears, M. K., Dively, G., et al. (2001). Temporal and spatial overlap between monarch larvae and corn pollen. Proc. Natl. Acad. Sci. USA. 98, 11913–11918. doi: 10.1073/ypnas.211234298
Pan, H., Yang, X., Bidne, K., Hellmich, R. L., Siegfried, B. D., and Zhou, X. (2017). Dietary risk assessment of v-ATPase A dsRNAs on monarch butterfly larvae. Front. Plant Sci. 8:242. doi: 10.3389/fpls.2017.00242
Pecenka, J. R., and Lundgren, J. G. (2015). Non-target effects of clothianidin on monarch butterflies. Sci. Nat. 102:19. doi: 10.1007/s00114-015-1270-y
Pitman, G. M., Flockhart, D. T. T., and Norris, D. R. (2018). Patterns and causes of oviposition in monarch butterflies: implications for milkweed restoration. Biol. Conserv. 217, 54–65. doi: 10.1016/j.biocon.2017.10.019
Pleasants, J. (2017). Milkweed restoration in the Midwest for monarch butterfly recovery: estimates of milkweeds lost, milkweeds remaining and milkweeds that must be added to increase the monarch population. Insect Conserv. Divers. 10, 42–53. doi: 10.1111/icad.12198
Pleasants, J. M., Hellmich, R. L., Dively, G. P., Sears, M. K., Stanley-Horn, D. E., Mattila, H. R., et al. (2001). Corn pollen deposition on milkweeds in and near cornfields. Proc. Natl. Acad. Sci. USA. 98, 11919–11924. doi: 10.1073/ypnas.211287498
Pleasants, J. M., and Oberhauser, K. S. (2013). Milkweed loss in agricultural fields because of herbicide use: effect on the monarch butterfly population. Insect Conserv. Divers. 6, 135–144. doi: 10.1111/j.1752-4598.2012.00196.x
Pleasants, J. M., Zalucki, M. P., Oberhauser, K. S., Brower, L. P., Taylor, O. R., and Thogmartin, W. E. (2017). Interpreting surveys to estimate the size of the monarch butterfly population: pitfalls and prospects. PLoS ONE 12:e0181245. doi: 10.1371/journal.pone.0181245
Pocius, V. M., Debinski, D. M., Bidne, K. G., Hellmich, R. L., and Hunter, F. K. (2017b). Performance of early instar monarch butterflies (Danaus plexippus L.) on nine milkweed species native to Iowa. J. Lepid. Soc. 71, 153–161. doi: 10.18473/lepi.71i3.a5
Pocius, V. M., Debinski, D. M., Pleasants, J. M., Bidne, K. G., Hellmich, R. L., and Brower, L. P. (2017a). Milkweed matters: monarch butterfly (Lepidoptera: Nymphalidae) survival and development on nine Midwestern milkweed species. Environ. Entomol. 46, 1098–1105. doi: 10.1093/ee/nvx137
Pocius, V. M., Pleasants, J. M., Debinski, D. M., Bidne, K. G., Hellmich, R. L., Bradbury, S. P., et al. (2018). Monarch butterflies show differential utilization of nine Midwestern milkweed species. Front. Evol. Ecol. 2018:00169. doi: 10.3389/fevo.2018.00169
Potts, S. G., Biesmeijer, J. C., Kremen, C., Neumann, P., Schweiger, O., and Kunin, W. E. (2010). Global pollinator declines: trends, impacts and drivers. Trends Ecol. Evol. 25, 345–353. doi: 10.1016/j.tree.2010.01.007
Prysby, M. D. (2004). “Natural enemies and survival of monarch eggs and larvae,” in The Monarch Butterfly: Biology and Conservation, eds. K. S. Obserhauser and M. J. Solensky (Ithaca, NY: Cornell University Press), 27–37.
Rafter, J., Agrawal, A., and Pressier, E. (2013). Chinese mantids gut toxic monarch caterpillars: avoidance of prey defense? Ecol. Entomol. 38, 76–82. doi: 10.1111/j.1365-2311.2012.01408.x
Ramírez, M. I., Azcárate, J. G., and Luna, L. (2003). Effects of human activities on monarch butterfly habitat in protected mountain forests, Mexico. Forest. Chron. 79, 242–246. doi: 10.5558/tfc79242-2
Rayor, L. S. (2004). “Effects of monarch larval host plant chemistry and body size on Polistes wasp predation,” in Monarchs in a Changing World: Biology and Conservation of an Iconic Butterfly, eds K. S. Obserhauser, K. R. Nail, and S. Altizer (Ithaca, NY: Cornell University Press), 39–46.
Rendón-Salinas, E., Fajardo-Arroyo, A., and Tavera-Alonso, G. (2014). Forest Surface Occupied by Monarch Butterfly Hibernation Colonies December 2014 (Report). World Wildlife Federation. Available online at: https://c402277.ssl.cf1.rackcdn.com/publications/768/files/original/REPORT_Monarch_Butterfly_colonies_Winter_2014.pdf?1422378439 (accessed April 09, 2018).
Reuters, T. (2016). Web of Science. New York, NY. Available online at: http://www.webofscience.com (Accessed 09 Apr 2018).
Ries, L., Taron, D. J., and Rendón-Salinas, E. (2015b). The disconnect between summer and winter monarch trends for the eastern migratory population: possible links to differing drivers. Ann. Entomol. Soc. Am. 108, 691–699. doi: 10.1093/aesa/sav055
Ries, L., Taron, D. J., Rendón-Salinas, E., and Oberhauser, K. S. (2015a). “Connecting Eastern monarch population dynamics across their migratory cycle,” in Monarchs in a Changing World: Biology and Conservation of an Iconic Butterfly, eds. K. S. Obserhauser, K. R. Nail, and S. Altizer (Ithaca, NY: Cornell University Press), 268–281.
Sáenz-Romero, C., Rehfeldt, G. E., Duval, P., and Lindig-Cisneros, R. A. (2012). Abies religiosa habitat prediction in climatic change scenarios and implications for monarch butterfly conservation in Mexico. Forest Ecol. Manag. 275, 98–106. doi: 10.1016/j.foreco.2012.03.004
Sakai, W. H. (1994). Avian predation on the monarch butterfly, Danaus plexippus (Nymphalidae: Danaidae), at a California overwintering site. J. Lepid. Soc. 48, 148–156.
Sánchez-Bayo, F., Goulson, D., Pennacchip, F., Nazzi, F., Goka, K., and Desneux, N. (2015). Are bee diseases linked to pesticides? - A brief review. Environ. Int. 89–90, 7–11. doi: 10.1016/j.envint.2016.01.009
Satterfield, D. A., Maerz, J. C., and Altizer, S. (2015). Loss of migratory behaviour increases infection risk for a butterfly host. Proc. R. Soc. B 282:20141734. doi: 10.1098/rspb.2014.1734
Satterfield, D. A., Maerz, J. C., Hunter, M. D., Flockhart, D. T. T., Norris, D. R., Streit, H., et al. (2018). Migratory monarchs that encounter resident monarchs show life-history differences and higher rates of parasite infection. Ecol Lett, 21, 1670–1680. doi: 10.1111/ele.13144
Sears, M. K., Hellmich, R. L., Stanley-Horn, D. E., Oberhauser, K. S., Pleasants, J. M., Mattila, H. R., et al. (2001). Impact of Bt corn pollen on monarch butterfly populations: a risk assessment. Proc. Natl. Acad. Sci. USA. 98, 11937–11942. doi: 10.1073/pnas.211329998
Semmens, D. J., Diffendorfer, J. E., Bagsrad, K. J., Wiederholt, R., Oberhauser, K., Ries, L., et al. (2018). Quantifying ecosystem service flows at multiple scales across the range of a long-distance migratory species. Econ. Serv. 31, 255–264. doi: 10.1016/j.ecoser.2017.12.002
Stanley-Horn, D. E., Dively, G. P., Hellmich, R. L., Mattila, H. R., Sears, M. K., Rose, R., et al. (2001). Assessing the impact of Cry1Ab-expressing corn pollen on monarch butterfly larvae in field studies. Proc. Natl. Acad. Sci. USA. 98, 11931–11936. doi: 10.1073/ypnas.211277798
Stenoien, C., Nail, K. R., and Oberhauser, K. S. (2015). Habitat productivity and temporal patterns of monarch butterfly egg densities in the eastern United States. Ann. Entomol. Soc. Am. 108, 670–679. doi: 10.1093/aesa/sav054
Stork, N. E., McBroom, J., Gely, C., and Hamilton, A. J. (2015). New approaches narrow global species estimates for beetles, insects, and terrestrial arthropods. Proc. Natl. Acad. Sci. USA. 112, 7519–7523. doi: 10.1073/pnas.1502408112
Swengel, A. B. (1995). Population fluctuations of the monarch (Danaus plexippus) in the 4th of July butterfly count 1977-1994. Am. Midl. Nat. 134, 205–214. doi: 10.2307/2426291
Tao, L., Hoang, K. M., and Hunter, M. D. (2018). The effects of milkweed induced defense on parasite resistance in monarch butterflies, Danaus plexippus. J. Chem. Ecol. 44, 1040–1044. doi: 10.1007/s10886-018-1007-4
Thogmartin, W. E., Diffendorfer, J. E., Lópex-Hoffman, L., Oberhauser, K., Pleasants, J., Semmens, B. X., et al. (2017a). Density estimates of monarch butterflies overwintering in central Mexico. PeerJ 5:e3221. doi: 10.7717/peerj.3221
Thogmartin, W. E., López-Hoffman, L., Rohweder, J., Diffendorfer, J., Drum, R., Semmens, D., et al. (2017c). Restoring monarch butterfly habitat in the Midwestern US: “all hands on deck.” Environ. Res. Lett. 12:074005. doi: 10.1088/1748-9326/aa7637
Thogmartin, W. E., Wiederholt, R., Oberhauser, K., Dunn, R. G., Diffendorfer, J. E., Altizer, S., et al. (2017b). Monarch butterfly population decline in North America: identifying the threatening processes. Roy. Soc. Open Sci. 4:170760. doi: 10.6084/m9.figshare.c.3876100
Tregenza, T. (2002). Gender bias in the refereeing process? Trends Ecol. Evol. 17, 349–350. doi: 10.1016/S0169-5347(02)02545-4
Tschenn, J., Losey, J. E., Jesse, L. H., Obrycki, J. J., and Hufbauer, R. (2001). Effects of corn plants and corn pollen on monarch butterfly (Lepidoptera: Danaidae) oviposition behavior. Environ. Entomol. 30, 495–500. doi: 10.1603/0046-225X-30.3.495
Urquhart, F. A. (1960). “Migration,” in The Monarch, (Toronto, ON: University of Toronto Press), 77–94.
Urquhart, F. A. (1966). Virus- caused epizootic as a factor in population fluctuations of the monarch butterfly. J. Invertebr. Pathol. 8, 492–495. doi: 10.1016/0022-2011(66)90076-0
Urquhart, F. A., and Urquhart, N. R. (1978). Autumnal migration routes of the eastern population of the monarch butterfly (Danaus p. plexippus L.; Danaidea; Lepidoptera) in North America to the overwintering site in the Neovolcanic Plateau of Mexico. Can. J. Zool. 56, 1759–1764. doi: 10.1139/z78-240
Vanbergen, A., and Garratt, M. (2013). Threats to an ecosystem service: pressures on pollinators. Front. Ecol. Environ. 16, 251–259. doi: 10.1890/120126
Vidal, O., López-García, J., and Rendón-Salinas, E. (2014). Trends in deforestation and forest degradation after a decade of monitoring in the monarch butterfly biosphere reserve in Mexico. Conserv. Biol. 28, 177–186. doi: 10.1111/cobi.12138
Vidal, O., and Rendón-Salinas, E. (2014). Dynamics and trends of overwintering colonies of the monarch butterfly in Mexico. Biol. Conserv. 180, 165–175. doi: 10.1016/j.biocon.2014.09.041
White, D. S., and Sexton, O. J. (1989). The monarch butterfly (Lepidoptera: Danaidae) as prey for the dragonfly Hagenisu brevistylus (Odantata: Gomphidae). Entomol. News 100, 129–132.
Williams, J. J., Stow, D. A., and Brower, L. (2007). The influence of forest fragmentation on the location of overwintering monarch butterflies in Central Mexico. J. Lepid. Soc. 61, 90–104.
Yeargan, K. V., and Allard, C. M. (2005). Comparison of common milkweed and honeyvine milkweed (Asclepiadaceae) as host plants for monarch larvae (Lepidoptera: Nymphalidae). J. Kansas Entomol. Soc. 78, 247–251. doi: 10.2317/0407.40.1
Zalucki, M. P. (1982). Temperature and rate of development in Danaus plexippus L. and D. chrysippus L. (Lepidoptera: Nymphalidae). J. Aust. Entomol. Soc. 21, 241–246. doi: 10.1111/j.1440-6055.1982.tb01803.x
Zalucki, M. P., Brower, L. P., Malcolm, S. B., and Slager, B. H. (2015). “Estimating the climate signal in monarch population decline: no direct evidence for an impact of climate change?” in Monarchs in a Changing World: Biology and Conservation of an Iconic Butterfly, eds K. S. Obserhauser, K. R. Nail, and S. Altizer (Ithaca, NY: Cornell University Press), 130–142.
Zalucki, M. P., and Lammers, J. H. (2010). Dispersal and egg shortfall in Monarch butterflies: what happens when the matrix is cleaned up? Ecol. Entomol. 35, 84–91. doi: 10.1111/j.1365-2311.2009.01160.x
Zalucki, M. P., Parry, H. R., and Zalucki, J. M. (2016). Movement and egg laying in monarchs: to move or not to move, that is the question. Austr. Ecol. 41, 154–167. doi: 10.1111/aec.12285
Zangerl, A. R., McKenna, D., Wraight, C. L., Carroll, M., Ficarello, P., Warner, R., et al. (2001). Effects of exposure to event 176 Bacillus thuringiensis corn pollen on monarch and black swallowtail caterpillars under field conditions. Proc. Natl. Acad. Sci. USA. 98, 11908–11912. doi: 10.1073/ypnas.171315698
Zaya, D. N., Pearse, I. S., and Spyreas, G. (2017). Long-term trends in Midwestern milkweed abundances and their relevance to monarch butterfly declines. BioScience 67, 343–356. doi: 10.1093/biosci/biw186
Keywords: conservation threats, habitat loss, population declines, migration, Ophryocystis elektroscirrha, contaminants, climate change, deforestation
Citation: Wilcox AAE, Flockhart DTT, Newman AEM and Norris DR (2019) An Evaluation of Studies on the Potential Threats Contributing to the Decline of Eastern Migratory North American Monarch Butterflies (Danaus plexippus). Front. Ecol. Evol. 7:99. doi: 10.3389/fevo.2019.00099
Received: 20 December 2018; Accepted: 12 March 2019;
Published: 05 April 2019.
Edited by:
Jay E. Diffendorfer, United States Geological Survey, United StatesReviewed by:
John Pleasants, Iowa State University, United StatesDouglas Landis, Michigan State University, United States
Copyright © 2019 Wilcox, Flockhart, Newman and Norris. This is an open-access article distributed under the terms of the Creative Commons Attribution License (CC BY). The use, distribution or reproduction in other forums is permitted, provided the original author(s) and the copyright owner(s) are credited and that the original publication in this journal is cited, in accordance with accepted academic practice. No use, distribution or reproduction is permitted which does not comply with these terms.
*Correspondence: Alana A. E. Wilcox, YXdpbGNvMDFAdW9ndWVscGguY2E=