- Department of Biology, University of Louisville, Louisville, KY, United States
Animals are under strong selective pressures to make correct decisions when attempting to escape an approaching predator, and not surprisingly many studies have shown that animals adjust their flight initiation behavior in response to risk. However, we have a poor understanding of animals' capability to select an appropriate flight trajectory. We investigated whether eastern gray squirrels would adjust their flight trajectory based on the relative locations of the squirrel, the approaching threat, and potential refuges. We used a person running toward a focal squirrel (N = 122) as the threat and considered the three trees nearest the squirrel and taller than 8 m to be potential refuges. Squirrels were strongly affected by the angle (θ) formed by the locations of person, squirrel, and the three nearest trees. A squirrel was less likely to run to the nearest tree (Tree 1) when θ1 was relatively acute, but also less likely to run to Tree 1 when θ2 was obtuse, making Tree 2 a more attractive refuge. A squirrel was more likely to run to Tree 1 if it was close and if Tree 2 was relatively far. Subtle differences in the effects of θ1 vs. θ2 on squirrel refuge choice support the idea that squirrels prefer a nearby refuge. Squirrels were more likely to select Trees 2 and 3 rather than Tree 1 only when θ2 was obtuse (105°). In contrast, most squirrels chose to run to Tree 1 when θ1 was >65°; thus squirrels were more likely to choose Tree 1 even when doing so required running at least partly toward the approaching threat. The decisions made by focal squirrels provide evidence that this species' assessment of risk is highly nuanced. A great deal of variation has been reported in responses to predators within species. While part of the variation may be due to strategic unpredictability on the part of the prey, part of it may also be due to differences in flight trajectory and refuge preferences that have not been well-studied.
Introduction
Fleeing is a common antipredator behavior across a wide range of animal taxa. When a potential predator is approaching, a prey animal must quickly determine both when to flee and which direction to go. A large body of literature on optimal escape theory has established that the decision of when to flee can reflect both an individual's ability to assess risk and the level of risk it is willing to accept. The decision of when to flee is typically measured as flight initiation distance (FID), i.e., the distance between the potential prey and an approaching predator at the moment the prey flees. Varied factors influence FID, including those related to the cost vs. benefits of flight (e.g., food availability or being engaged in interactions with conspecifics), characteristics and behavior of the threat (predator size, gaze, and approach speed), characteristics of the prey (e.g., body size; Blumstein, 2006; Fernandez-Juricic et al., 2006) and habitat or location effects such as distance from refuge, position of the predator relative to a refuge, or amount of cover (Blumstein, 2003; Stankowich and Blumstein, 2005; Samia et al., 2016). In addition, urban populations of a species often have lower FIDs than rural ones (Møller, 2015; Møller et al., 2015).
Flight trajectories have been less studied in the field than FID, but animals similarly appear to make adaptive decisions about the direction in which they flee, as would be predicted given the high fitness cost of choosing incorrectly. In the lab, these decisions have been shown to be constrained by sensory and motor limitations [reviewed in (Domenici et al., 2011a,b)], and they may also be constrained by the advantages of alignment with a geomagnetic field in some species (Obleser et al., 2016). In both laboratory experiments and field studies, individuals from a wide range of taxa usually move relatively directly away from an approaching threat, thus maximizing or nearly maximizing their distance from approaching predators (Domenici et al., 2011a; Cooper, 2016a). However, the exact direction of flight relative to a threat varies within and across species, an inconsistency that may be a strategic unpredictability to decrease a predator's ability to predict prey response (Arnott et al., 1999; Domenici et al., 2011a). For example, in the Trinidadian stream frog Mannophryne trinitatis and two treefrog species (Trachycephalus venulosus and Hypsiboas geographicus), escape angles were generally predictable yet still highly variable; they jumped away from lateral or caudal stimuli but used a broad range of escape angles, and there was no directionality to their trajectory when approached frontally (Royan et al., 2010). Larval zebrafish (Danio rerio) used a mix of random and direct tactics when fleeing depending on the approach direction of the predator model; a zebrafish maximized distance from a threat when approached from the side but used random escape angles when approached by a threat in line with its heading, i.e., directly toward the head or tail (Nair et al., 2017). Further, some species vary their responses according to predator taxon. For example, Túngara frogs (Engystomops pustulosus) flee away from snake models but toward models of bats, as they seek to undercut the bat's flight path. Other exceptions to the general pattern occur in species that similarly try to undercut an approaching predator's path. Moths are most likely to escape pursuing bats when they decrease the escape angle, turning to move toward or perpendicularly to the bat when the bat is close and thus making it difficult for the bat to adjust its pursuit trajectory to reach the prey (Corcoran and Conner, 2016). Columbian black-tailed deer also preferentially selected acute escape angles when fleeing a nearby threat (Stankowich and Coss, 2007).
The presence of a refuge can also have a large effect on flight trajectories, and in some species, the location of the refuge is the primary determinant of flight trajectory (Hemmi and Pfeil, 2010). For example, broad-headed skinks (Eumeces laticeps) typically fled toward the nearest refuge regardless of their location relative to an approaching threat (Cooper, 1997), and side-blotched lizards (Uta stansburiana) fleeing from a model snake fled in a random direction with respect to the predator but toward a refuge (cliff) if it was nearby (Zani et al., 2009). Similarly, Mongolian gerbils (Meriones unguiculatus) in laboratory experiment tended to flee to the nearest refuge regardless of the position of a visual stimulus meant to induce escape behavior (Ellard, 1993). However, in some species the position of an approaching threat relative to a refuge can influence flight trajectory in at least some circumstances. Blue crabs (Callinectes sapidus) in the intertidal zone use the deeper water offshore as a refuge, and they will flee away from a human approaching from the shore in a direction that maximizes their distance offshore before the person will intercept them, although they flee generally toward the person if that person approaches from the sea (Woodbury, 1986). Thus, for these crabs the location of the approaching human can strongly alter escape trajectory, but only when the person is not blocking the path to the refuge. In staghorn sculpin (Leptocottus armatus), the location of a simulated aerial attack influenced flight trajectory. The sculpin fled toward a refuge at a 90° angle from the stimulus if they were already facing that direction. The sculpin also went toward a refuge placed so that they could move directly away from the stimulus to reach it, but their flight trajectories were random if they had to move toward the stimulus to reach a refuge or to turn around to reach a refuge at a 90° angle from the stimulus (Shi et al., 2017).
When a prey species typically has multiple refuges available, selecting one is likely to be a complex problem, and one that must be quickly solved when a predator is rapidly approaching. In this study, we investigated how eastern gray squirrels (Sciurus carolinensis) select among available refuges when approached rapidly by a human in parks or park-like settings. These squirrels often forage on the ground and run to a nearby tree as a refuge if a potential predator approaches. Although an older study that focused on flight initiation distance suggested that squirrels select the nearest tree as a refuge (Dill and Houtman, 1989), our casual observations suggested that this was not always the case. Previous studies of marmots (Kramer and Bonenfant, 1997) demonstrated that flight initiation distance increased when a prey has to move toward an approaching predator to reach a refuge, suggesting that prey perceive moving in the direction of a predator to be risky. This conclusion implies that refuge choice should be influenced not only by distance to refuge but also by the relative locations of prey, predator, and potential refuges, a prediction that was supported by recent theoretical models (Cooper, 2016b; Cooper et al., 2018). Cooper (2016a) consider a case in which one refuge was available and predicted that flight initiation distance would increase sigmoidally as distance to refuge increased, as predator approach speed increased, and as a potential prey was forced to run more directly toward an approaching threat to reach the refuge. (Cooper et al., 2018) modeled a situation in which threatened prey chose between two refuges. Based on the assumption that shorter flight initiation distances would be preferred, they predicted that prey would not always choose the nearer refuge but would be more likely to flee to the farther refuge if their path to the nearer refuge would take them more directly toward the approaching predator.
We tested whether eastern gray squirrels preferred the nearest tree as refuge vs. one of the two next-nearest trees and whether refuge preference was affected by escape angle, i.e., the angle between the path of an approaching threat and the flight trajectories to the three possible refuges. Vulnerable animals like squirrels should minimize predation risk, so they should choose the nearest refuge to minimize costly flight distance, shorten flight initiation distance, and reduce exposure to predators during flight. However, the safety of the path to the nearest refuge may be compromised if it brings the squirrel closer to the predator. Based on this hypothesis, we predicted that squirrels would be more likely to select the nearest refuge but that this preference would be altered if other refuges were at similar distances and could be reached by moving away from the approaching threat. We also examined whether flight initiation distance was influenced by squirrel distance to refuge, escape angle, and the distance between person and squirrel at the start of the person's approach (starting distance). Both starting distance and distance from refuge affect flight initiation distance across a wide range of taxa, with FID typically increasing when starting distance and distance from refuge are longer (Stankowich and Blumstein, 2005).
Methods
We conducted trials to investigate the flight behavior of eastern gray squirrels from September to November in 2014 and 2016 in Louisville, Kentucky. To maintain consistency in squirrels' activity levels, we conducted trials only from late afternoon until dusk and when the temperature was at least 11°C. Each trial met the following criteria: (1) the focal squirrel was foraging on the ground and not evidently responding to our presence, i.e., not alert, and not looking at us or sitting up on its hind legs; (2) the squirrel was at least 2 m from the nearest tree; (3) there were no squirrels within 15 m of the focal squirrel; and (4) there were a minimum of three trees within 25 m of the squirrel that were suitable refuges. The definition of suitable refuges was based on observations of squirrels' flight behavior before trials began; included trees were at least 8 m in height and had a trunk diameter >0.3 m. When these criteria were met, one person then sprinted at full speed toward the position of the focal squirrel from a distance of at least 10 m while the other two collaborators noted the locations of the focal squirrel when the runner started, when the squirrel became alert, and when it initiated flight. We marked the starting point of the runner and the squirrel locations with rocks immediately after the squirrel fled. The two collaborators stood approximately 3–4 m apart on opposite sides of the runner at the starting location to facilitate accurately marking the focal squirrel's locations. We also used small landmarks, e.g., fallen leaves, bare spots, chunks of bark, and sticks, to pinpoint each squirrel's locations. The runner simulated a potential threat of attack by a predator. Flat rocks were dropped by the runner to mark the locations of the runner when the squirrel alerted and when it fled. Focal squirrels were not within 3 m of any path, and the runner did not start from or follow any path toward the squirrel on any trial because varied species (Mainini et al., 1993; Miller et al., 2001; Eason et al., 2006), including Sciurus carolinensis (Bateman and Fleming, 2014), view humans approaching on a path as a lesser threat than humans approaching but not following a path. The mean running speed of the person was 3.1 ± 0.01 (SE) m/sec, which was calculated from 5 sprints of 20 m that were 10 min apart and not part of the trials.
We recorded the distance in meters from the squirrel to the runner at the start of the trial, the alert distance, and flight initiation distance, using a tape measure to measure all distances to the nearest 10 centimeters. We also recorded the distances between the squirrel and the three nearest trees and between the runner and each of those same trees at the moment the squirrel became alert. The nearest tree to the squirrel was defined as Tree 1, the second closest as Tree 2, and the farthest as Tree 3. A sighting compass was used to record the compass direction to each of the three trees from the positions of both the squirrel and the runner, as well as the direction the squirrel was facing. The tree directions were then standardized so that the starting position of runner was always placed at 0°. The standardized directions were used to calculate escape angle, i.e., the angle (θ) between the person, squirrel, and tree for each tree (θ1, θ2, and θ3; Figure 1) at the moment the squirrel became alert. Angles were calculated on a 180° scale, with a θ of 180° representing a squirrel that ran directly away from the runner, and a θ of 0° representing a squirrel that ran directly toward the runner.
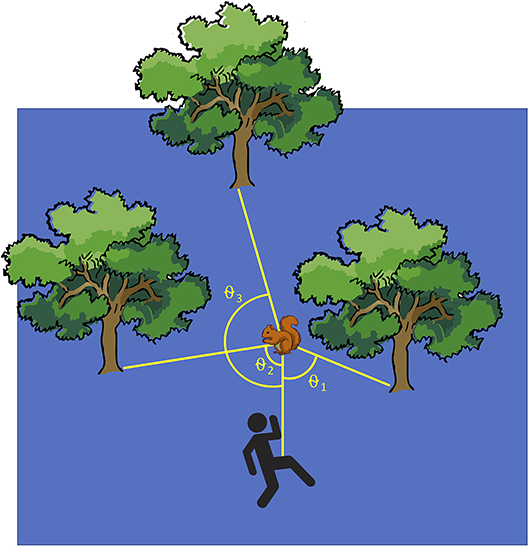
Figure 1. The closest tree to the squirrel is denoted Tree 1 and the farthest is Tree 3. θ indicates the escape angle, i.e., the angle from person to squirrel to a tree.
The squirrels in our trials were not individually identifiable, and accordingly we took measures to reduce the probability of re-sampling individuals. In 2014, we conducted trials on grassy lawns at five sites: the University of Louisville campus (N = 32; 21 ha) and four urban parks, including Central Park (N = 10; 7 ha), George Rogers Clark Park (N = 11; 19 ha), Shawnee Park (N = 5; 128 ha), and Tom Sawyer Park (N = 7; 23 ha). In 2016 we performed trials only at University of Louisville (N = 30) and Central Park (N = 27), the sites that had the highest densities of squirrels. Based on a mark-recapture study from 2010–2013, the number of squirrels on the University of Louisville campus was estimated as 434 ± 46 (±SE; William Persons, pers. com.). At all sites we moved systematically from one side of the site to the other to reduce the probability of re-sampling. At both University of Louisville campus (2014 and 2016) and Central Park (2016), we performed trials over two consecutive days, sampling approximately half the site each day. Approximately 40% of the squirrels we observed in 2014 had ear tags, but in 2016 we saw only two individuals with tags and did not include any tagged individuals in trials. We conducted a total of 122 trials, and all trials were combined for analyses. In two additional trials, which are not included in the above counts or in analyses, one focal squirrel chose the fourth nearest tree and the other chose the fifth nearest tree.
Our alternative hypothesis predicted that squirrels would flee to the closest tree, and accordingly we analyzed squirrel refuge choice as either “Chose Tree 1” or “Did not choose Tree 1.” For statistical regression analyses we used generalized linear models (glm) in R Core Team (2016). We performed a logistic regression to assess squirrel refuge choice. We were most interested in the effects of squirrel and person position relative to the potential refuges. Accordingly, the following explanatory variables and all their interactions were initially included in the logistic regression on squirrel refuge choice: all squirrel distances to trees, person distance to Tree 1, and θ for each of the three trees. In addition, we included the main effects of the following explanatory variables with no interaction terms: flight initiation distance, starting distance, and alert distance. We could not include the interaction terms for this second set of variables because the model became overfitted. We could not include the person distances to Trees 2 and 3 even as main effects because they were co-linear with person distance to Tree 1. Of these three variables, we chose to include person distance to Tree 1 because our study design focused on whether the squirrel selected Tree 1 as a refuge or not. Alert distance and FID were linearly correlated (r = 0.82) so we used a model comparison approach to determine which of these two variables to include. The initial model with alert distance had a lower AIC (150.05) than did the initial model with FID (157.65), so we performed backward elimination using the model containing alert distance.
We performed a linear regression to determine the factors that influenced flight initiation distance, with the explanatory variables squirrel distance to refuge and θrefuge (the escape angle for the tree chosen by a fleeing squirrel) as linear terms and the explanatory variable starting distance as the quadratic term. In a separate analysis, to determine whether refuge choice was independent of the direction a squirrel was facing when they fled, we performed a Chi square goodness-of-fit test on the number of times squirrels chose the tree they were most nearly oriented toward, the tree they were second closest to facing, and the tree they were oriented farthest away from at the time of the threat. All means are given ± SE.
Results
Out of 122 trials, 60 squirrels chose Tree 1 as a refuge and 62 did not, with 45 choosing Tree 2 and 17 choosing Tree 3. Squirrel mean distances to the three potential refuges were 5.3 ± 0.18 m (Tree 1), 8.8 ± 0.23 m (Tree 2), and 12.4 ± 0.29 m (Tree 3). Refuge choice was independent of the direction squirrels were facing (Chi square goodness-of-fit test: X2 = 3.26, N = 122, p = 0.20). Person distance to Tree 1, starting distance, alert distance, and θ3 did not have significant main or interaction effects on squirrel refuge choice and were dropped from the logistic regression model. The final model had an AIC value of 132.79 (see Supplementary Table 1).
Both θ1 and θ2 had significant main effects, but no significant interactions. As θ1 increased, the probability of the squirrel choosing Tree 1 also increased (p < 0.0001; Figure 2A). For θ1, the escape angle at which squirrels were equally likely to choose Tree 1 or a more distant tree was 65°; in other words, at θ1 = 65° the probability that a squirrel would choose Tree 1 as a refuge was 0.5. When θ1 ≤ 65°, only 25% of squirrels chose Tree 1 as a refuge (N = 60; Figure 2B), but when θ1 > 65°, 73% of squirrels (N = 62) chose Tree 1, the nearest refuge. As θ2 increased, i.e., as the trajectory toward Tree 2 allowed the squirrel to run away from rather than toward the approaching threat to reach that refuge, the probability of the squirrel choosing Tree 1 decreased (p = 0.002; Figure 3A). For θ2, the angle at which squirrels were equally likely to choose either Tree 1 or a more distant tree was 105° (Figure 3B). When θ2 ≤ 105°, 67% of squirrels (N = 58) chose Tree 1 as a refuge, but only 33% of squirrels (N = 64) chose Tree 1 when θ2 > 105°. Squirrel distance to Tree 2 interacted with squirrel distance to Tree 1 (p = 0.01; Figure 4) and marginally interacted with squirrel distance to Tree 3 (p = 0.066; Figure 5). Squirrels were more likely to choose Tree 1 when close to it and far from Tree 2, and when very far from both Tree 2 and Tree 3.
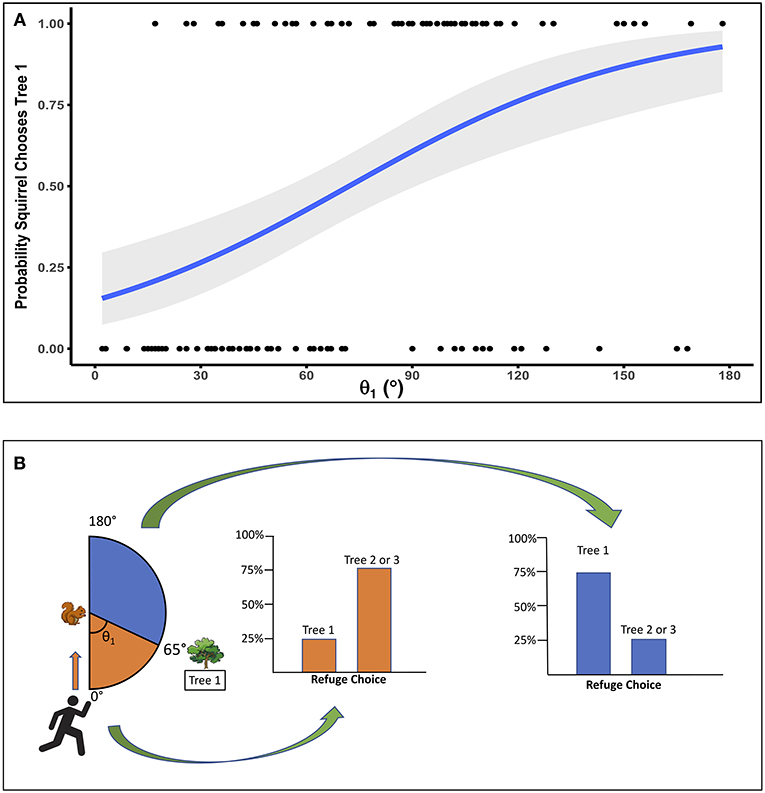
Figure 2. (A) The probability that a squirrel will choose Tree 1 increases as the escape angle to Tree 1 (θ1) increases, so that the squirrel runs more directly away from the approaching threat to reach that refuge. The probability of a squirrel choosing Tree 1 is 0.5 when θ1 is 65°. Gray area indicates SE, and dots show squirrel refuge choices, with a value of 1 for a squirrel that chose Tree 1 and a value of 0 for a squirrel that chose Tree 2 or Tree 3. (B) Squirrels were less likely to select the nearest tree (Tree 1) as a refuge when θ1 was relatively acute, meaning they had to run in the general direction of the approaching person to reach that tree. When Tree 1 angle (θ1) was ≤ 65°, 25% of squirrels chose Tree 1 and 75% chose Tree 2 or 3. When θ1 was > 65°, 73% of squirrels chose Tree 1 and 27% chose Tree 2 or 3.
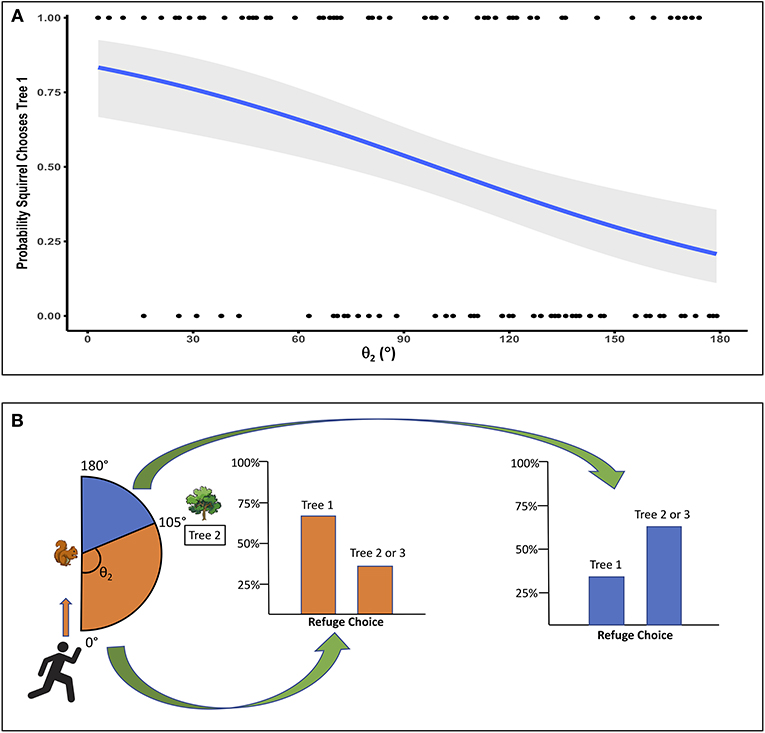
Figure 3. (A) As θ2 increases, Tree 2 becomes a more attractive (less risky) refuge, and the probability that a squirrel will choose Tree 1 decreases. However, a relatively large angle for Tree 2 (θ2 ≥ 105°) is required to make the probability of choosing Tree 1 ≤ 0.5. Gray area indicates SE, and dots show squirrel refuge choices, with a value of 1 for a squirrel that chose Tree 1 and a value of 0 for a squirrel that chose Tree 2 or Tree 3. (B) Squirrels were less likely to select Tree 1 as a refuge when θ2 was relatively large, making the flight trajectory to Tree 2 less risky. When Tree 2 angle (θ2) was ≤ 105°, 67% of squirrels chose Tree 1 and 33% chose Tree 2 or 3. When θ2 was > 105°, 33% of squirrels chose Tree 1 and 67% chose Tree 2 or 3.
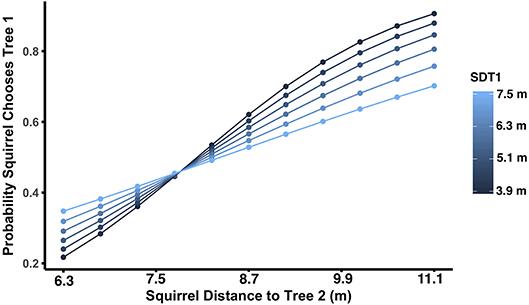
Figure 4. As squirrel distance to Tree 2 increases, the probability of choosing Tree 1 (the nearest tree) as a refuge increases. There is a significant interaction between squirrel distance to Tree 1 (SDT1) and squirrel distance to Tree 2. Lines depict the relationship between squirrel distance to Tree 2 and the probability of choosing Tree 1, with line color indicating the level of squirrel distance to Tree 1. When squirrel distance to Tree 1 is small, squirrel distance to Tree 2 has a stronger effect on the likelihood that the squirrel will choose Tree 1.
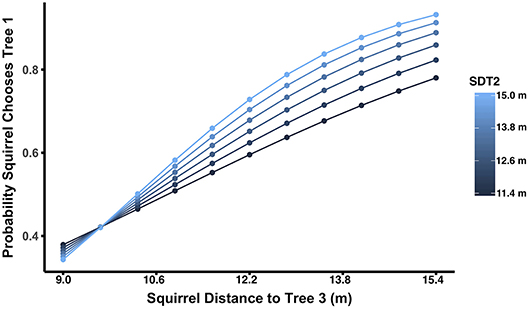
Figure 5. As squirrel distance to Tree 3 increases, the probability of choosing Tree 1 increases. Lines depict the relationship between squirrel distance to Tree 3 and the probability of choosing Tree 1, with line color indicating the level of squirrel distance to Tree 2 (SDT2). When SDT2 is large, squirrel distance to Tree 3 tends to have a stronger effect on the likelihood the squirrel will choose Tree 1, although this was only marginally significant (p = 0.066).
Flight initiation distance ranged from 1.6–18.8 m (mean FID = 9.2 ± 0.33 m). In the linear regression to determine which factors affected FID, squirrel distance to refuge and θrefuge did not have significant effects on FID and were dropped from the model. Flight initiation distance had a significant quadratic relationship with starting distance (p = 0.002; Figure 6), increasing as starting distance increased up to approximately 25 m and then declining (see Supplementary Table 1).
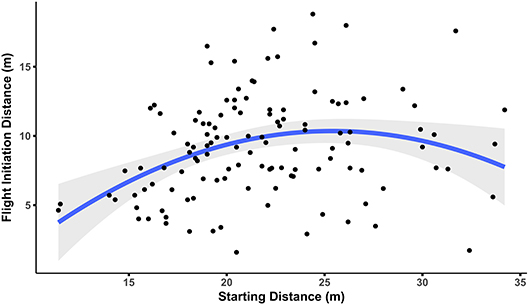
Figure 6. Starting distance has a quadratic relationship with FID. Squirrel FID increases as starting distance increases, but only up to ~25 m after which FID declines. Gray area indicates SE.
Discussion
Squirrels used complex criteria to choose a refuge when fleeing from an approaching threat. A squirrel incorporated the spatial relationships among itself, the approaching threat, and the potential refuges in its flight trajectory decision. Approximately half (49%) of focal squirrels chose the nearest refuge, with 37% of the squirrels fleeing to the next-closest tree and 14% choosing the farthest of the three nearest trees. The escape angles for the two nearest refuges (θ1 and θ2) both had significant main effects on squirrel refuge choice (Figures 2, 3), with squirrels generally preferring larger escape angles so that they would be moving less directly toward the approaching threat. Relative proximity to refuges was also a factor in their decisions: a squirrel was more likely to choose the nearest refuge (Tree 1) if both of the other two refuges were very far away (Figure 5), or if the squirrel was close to Tree 1 and far from Tree 2 (Figure 4). Subtle differences in the effects of escape angles for Tree 1 vs. Tree 2 on squirrel refuge choice also support the idea that squirrels prefer a nearby refuge. Squirrels were more likely to select one of the two more distant refuges rather than the nearest refuge only when the escape angle for Tree 2 (θ2) was obtuse (105°). In contrast, most squirrels chose to run to Tree 1 when its escape angle was >65°; thus squirrels were more likely to choose Tree 1 even when doing so required running at least partly in the direction of the approaching threat. Taken together, these results suggest that proximity of a refuge can modulate the negative effects of a relatively poor escape angle and that a more favorable escape angle can compensate for greater distance to a refuge. This finding provides general support for a prediction in the theoretical model of (Cooper et al., 2018), which addressed the problem of choosing between two refuges and predicted that a fleeing prey would select the more distant refuge if the trajectory to that refuge was more away from the predator and the trajectory to the nearer refuge.
In some studies, flight trajectory has appeared to be random with respect to the approaching threat, behavior that has been explained as creating unpredictability (Arnott et al., 1999; Domenici et al., 2011a), or possibly due to microhabitat differences, where differences in trajectory in different habitats confound overall conclusions (Martín and Lopez, 2000). Most previous studies have suggested that animals are reluctant to run toward an approaching threat (Domenici et al., 2011a; Cooper, 2016a), as was the case in this study for refuges that were farther away. The common preference for obtuse escape angles could be due to a general aversion to moving toward a predator, but may also reflect the greater probability of being cut off from a refuge by the predator if a prey were moving toward both predator and refuge. In contrast, individuals of some species will take an acute escape angle when a predator is very near, undercutting an approaching predator when that predator is unlikely to be able to change direction swiftly enough to capture the prey (Corcoran and Conner, 2016) or causing the predator to make energetically costly changes in direction to be able to follow the prey (Stankowich and Coss, 2007) However, to reach the nearest refuge, squirrels in this study sometimes preferred a trajectory that took them closer to the threat. The density of refuges is also likely to affect escape behavior. Based on our results, we expect that escape angle and distance to refuge would both influence flight trajectory when multiple refuges are close enough to the prey to be suitable refuges and the distances between the prey and the refuges differ enough to be readily distinguishable. However, if refuges are at high densities and thus very near one another, a threatened prey may be unable to determine which refuge is closest or may not need to flee to the closest refuge because travel time is short and differs only slightly among nearby refuges. In this scenario, prey may prioritize flight trajectory over flight distance, more frequently choosing the refuge most directly away from the threat. If refuges are far apart, prey may tend to remain relatively near a refuge and more frequently choose that closest refuge even when the escape angle to reach it is relatively poor, forcing the prey to move at least indirectly toward an approaching threat.
For many species FID is positively correlated with distance from refuge (Cooper and Wilson, 2007), and in some species, variation in indirect risk (distance from cover) is more important to risk-assessment than variation in direct risk [distance from humans; white-browed sparrow-weavers (Plocepasser mahali), (Fong et al., 2009)]. Thus, while they engage in other activities, animals clearly monitor their distance from potential refuges where the likelihood of discovery or capture by a predator would be lessened. This vigilance suggests that animals are also likely to be aware of the direction they should take to reach that refuge. We do not currently have a good understanding of how many potential refuges members of different species typically track or recognize. In the eastern gray squirrel, that number at our study site and under our experimental conditions appeared to be three, with only two individuals of 124 moving to the fourth or fifth nearest tree. The number of potential refuges an animal considers will obviously depend in part on prey taxon, with sensory and cognitive abilities providing limitations. That number will also depend on refuge availability and the kind of refuges a species or individual uses. Some species will have only one refuge in the vicinity, and thus only need to keep track of the direction of one feature to be able to flee efficiently, and in some cases that refuge may be a large landscape feature, which would facilitate orientation if rapid flight is undertaken. For example, lizards that preferentially flee to a cliff (Zani et al., 2009) or blue crabs that flee to deeper waters (Woodbury, 1986) may need to keep track of only one direction in which to flee in the event a threat arises while they are engaged in foraging or other activities, although of course if that direction is unavailable they need to quickly find alternatives or be aware of them beforehand. Slower approaches by a predator are likely to allow consideration of a greater number of refuges, where they are available, although that very slowness may reduce the need to travel to a refuge other than the nearest.
Starting distance had a significant effect on FID, with squirrels fleeing at greater distances when the person started his approach from a greater distance. Starting distance is commonly positively correlated with FID in vertebrate taxa (Blumstein, 2003; Cooper, 2005; Stankowich and Blumstein, 2005; Stankowich and Coss, 2006; Samia and Blumstein, 2015; Holmern et al., 2016; Evans et al., 2017), although these two metrics are sometimes not correlated in urban habitats (birds: Tätte et al., 2018). This effect of starting distance supports the flush early and avoid the rush (FEAR) hypothesis, which posits that a positive correlation between starting distance and FID exists because waiting can be costly for prey, either because of increased risk or decreased benefits due to the need to monitor the approaching predator (Blumstein, 2003; Cooper and Blumstein, 2014). In this study, however, FID decreased slightly with starting distances greater than about 25 m. This suggests that in this urban population, squirrels do not experience increased risk of capture beyond that starting distance for terrestrial predators they would normally contend with. In addition, these squirrels may not monitor their surroundings for threats beyond that distance (Blumstein, 2003) and may not immediately detect predators when they enter the zone within which the squirrels do monitor threats (Stankowich and Coss, 2006). This delay in detection occurs because the prey animal is not constantly scanning for threats (Stankowich and Coss, 2006). In Columbian black-tailed deer (Odocoileus hemionus columbianus), FID decreased at very long starting distances in deer that were not alert to being approached by humans, but in deer that were aware of the approaching threat, FID had a logarithmic relationship with starting distance (Stankowich and Coss, 2006). This result suggests that more wary individuals respond to predators approaching from far away differently than do less reactive individuals and may view such predators as more dangerous (Stankowich and Coss, 2006). As is the case with other species, squirrels in urban environments have moderated responses to humans (Bateman and Fleming, 2014; Uchida et al., 2016). Less habituated squirrels in rural habitats may have longer monitoring distances where visibility permits, and rural squirrels might also have a logarithmic relationship between FID and starting distance rather than the quadratic relationship found in this study.
Although starting distance influenced FID, squirrel distance to refuge did not have a significant effect. Previous studies have typically found that FID is positively correlated with distance to refuge (e.g., Dill, 1990; Cooper and Wilson, 2007; Guay et al., 2013), as optimal escape theory would predict (Ydenberg and Dill, 1986; Cooper and Frederick, 2007). One meta-analysis concluded that the effect of distance to refuge on FID was ubiquitous across taxa and significant (Stankowich and Blumstein, 2005), and a second found broad support for this relationship across 28 lizard species (Samia et al., 2016). There are some exceptions to this pattern. For example, in the lacertid lizard Psammodromus algirus, FID was not correlated with distance to refuge, possibly due to differences in microhabitat and refuge quality (Martín and Lopez, 2000). In our study species, Sciurus carolinensis, a positive correlation between FID and distance to refuge was previously found in a study that used a motorized stuffed cat (Felis domesticus) as a predator, albeit over a small range of distances to refuge (1–5 m; Dill and Houtman, 1989). However, Engelhardt and Weladji (2011), who examined S. carolinensis responses to an approaching human over a wider range of distances to refuge (0.3–26 m), found as we did that distance to refuge did not influence FID in S. carolinensis, and the results in Dill and Houtman (1989) suggested that the relationship between distance to refuge and FID may have been logarithmic, with distance to refuge having little effect above 3 m (Bonenfant and Kramer, 1996).
In sum, the decisions by focal squirrels we observed provide evidence that in this species assessment of risk is highly nuanced. It is also potentially resilient to atypical predator behavior; the squirrels in this study made reasonable choices despite the fact that the human threat sprinted at them in a way that does not reflect the majority of their experience with humans, who are a frequent presence in their environment and are usually only moving at walking speed. A great deal of variation has been reported in responses to predators within species. While part of the variation in FID, for example, may be due to strategic unpredictability on the part of the prey, part of it may also be due to differences in flight trajectory and refuge preferences that have not yet been well-studied. Species that use particular sites or objects as refuges provide a particularly rich opportunity for assessing the effects of flight trajectory on other metrics commonly used to assess flight behavior, including FID, and distance fled.
Data Availability
The raw data supporting the conclusions of this manuscript will be made available by the authors, without undue reservation, to any qualified researcher.
Ethics Statement
No animals were captured or touched during this experiment. The Institutional Animal Care and Use Committee of the University of Louisville approved this project.
Author Contributions
PE initiated and designed the study, observed and measured squirrel responses, wrote the initial draft of the manuscript, and revised later versions. LN observed and measured squirrel responses, performed statistical analyses, and edited the draft of the manuscript. JA participated in the study design, observed and measured squirrel responses, and edited the draft of the manuscript.
Conflict of Interest Statement
The authors declare that the research was conducted in the absence of any commercial or financial relationships that could be construed as a potential conflict of interest.
Acknowledgments
We thank Peter Sherman for chasing squirrels and Louisville Metro and Olmsted Parks for permission to work in the parks they oversee. The Institutional Animal Care and Use Committee of the University of Louisville approved this project.
Supplementary Material
The Supplementary Material for this article can be found online at: https://www.frontiersin.org/articles/10.3389/fevo.2019.00066/full#supplementary-material
References
Arnott, S. A., Neil, D. M., and Ansell, A. D. (1999). Escape trajectories of the brown shrimp Crangon crangon, and a theoretical consideration of initial escape angles from predators. J. Exp. Biol. 202, 193–209.
Bateman, P. W., and Fleming, P. A. (2014). Does human pedestrian behaviour influence risk assessment in a successful mammal urban adapter? J. Zool. 294, 93–98. doi: 10.1111/jzo.12156
Blumstein, D. T. (2003). Flight-initiation distance in birds is dependent on intruder starting distance. J. Wildl. Manage 67, 852–857. doi: 10.2307/3802692
Blumstein, D. T. (2006). Developing an evolutionary ecology of fear: how life history and natural history traits affect disturbance tolerance in birds. Animal Behav. 71, 389–399. doi: 10.1016/j.anbehav.2005.05.010
Bonenfant, M., and Kramer, D. L. (1996). The influence of distance to burrow on flight initiation distance in the woodchuck, Marmota monax. Behav. Ecol. 7, 299–303. doi: 10.1093/beheco/7.3.299
Cooper, W. E. (1997). Escape by a refuging prey, the broad-headed skink (Eumeces laticeps). Can. J. Zool. 75, 943–947. doi: 10.1139/z97-113
Cooper, W. E. (2005). When and how do predator starting distances affect flight initiation distances? Can. J. Zool. 83, 1045–1050. doi: 10.1139/z05-104
Cooper, W. E. (2016a). Directional escape strategy by the striped plateau lizard (Sceloporus virgatus): turning to direct escape away from predators at variable escape angles. Behaviour 153, 401–419. doi: 10.1163/1568539X-00003353
Cooper, W. E., and Blumstein, D. T. (2014). Novel effects of monitoring predators on costs of fleeing and not fleeing explain flushing early in economic escape theory. Behav. Ecol. 25, 44–52. doi: 10.1093/beheco/art083
Cooper, W. E., and Frederick, W. G. (2007). Optimal flight initiation distance. J.Theor. Biol. 244, 59–67. doi: 10.1016/j.jtbi.2006.07.011
Cooper, W. E., Samia, D. S. M., and Foster, S. (2018). Choosing among alternative refuges: distances and directions. Ethology 124, 209–217. doi: 10.1111/eth.12725
Cooper, W. E., and Wilson, D. S. (2007). Beyond optimal escape theory: microhabitats as well as predation risk affect escape and refuge use by the phrynosomatid lizard Sceloporus virgatus. Behaviour 144, 1235–1254. doi: 10.1163/156853907781890940
Cooper, W. E. Jr. (2016b). Fleeing to refuge: escape decisions in the race for life. J. Theor. Biol. 406, 129–136. doi: 10.1016/j.jtbi.2016.06.023
Corcoran, A. J., and Conner, W. E. (2016). How moths escape bats: predicting outcomes of predator-prey interactions. J. Exp. Biol. 219, 2704–2715. doi: 10.1242/jeb.137638
Dill, L. M. (1990). Distance-to-cover and the escape decisions of an African cichlid fish, Melanochromis chipokae. Environ. Biol. Fish. 27, 147–152. doi: 10.1007/BF00001944
Dill, L. M., and Houtman, R. (1989). The influence of distance to refuge on flight initiation distance in the gray squirrel (Sciurus carolinensis). Can. J. Zool. 67, 233–235. doi: 10.1139/z89-033
Domenici, P., Blagburn, J. M., and Bacon, J. P. (2011a). Animal escapology II: escape trajectory case studies. J. Exp. Biol. 214, 2474–2494. doi: 10.1242/jeb.053801
Domenici, P., Blagburn, J. M., and Bacon, J. P. (2011b). Animal escapology I: theoretical issues and emerging trends in escape trajectories. J. Exp. Biol. 214, 2463–2473. doi: 10.1242/jeb.029652
Eason, P., Sherman, P., Rankin, O., and Coleman, B. (2006). Factors affecting flight initiation distance in American robins. J. Wildl. Manage 70, 1796–1800. doi: 10.2193/0022-541X(2006)70[1796:FAFIDI]2.0.CO;2
Ellard, C. G. (1993). Organization of escape movements from overhead threats in the Mongolian gerbil (Meriones unguiculatus). J. Comp. Psychol. 107, 242–249. doi: 10.1037/0735-7036.107.3.242
Engelhardt, S. C., and Weladji, R. B. (2011). Effects of levels of human exposure on flight initiation distance and distance to refuge in foraging eastern gray squirrels (Sciurus carolinensis). Can. J. Zool. 89, 823–830. doi: 10.1139/z11-054
Evans, J. S., Eifler, D. A., and Eifler, M. A. (2017). Sand-diving as an escape tactic in the lizard Meroles anchietae. J. Arid Environ. 140, 1–5. doi: 10.1016/j.jaridenv.2017.01.005
Fernandez-Juricic, E., Blumstein, D. T., Abrica, G., Manriquez, L., Adams, L. B., Adams, R., et al. (2006). Relationships of anti-predator escape and post-escape responses with body mass and morphology: a comparative avian study. Evol. Ecol. Res. 8, 731–752.
Fong, T. E., Delong, T. W., Hogan, S. B., and Blumstein, D. T. (2009). The importance of indirect cues for white-browed sparrow-weaver (Plocepasser mahali) risk assessment. Acta. Ethol. 12, 79–85. doi: 10.1007/s10211-009-0059-4
Guay, P.-J., Lorenz, R. D. A., Robinson, R. W., Symonds, M. R. E., Weston, M. A., and Wright, J. (2013). Distance from water, sex and approach direction influence flight distances among habituated black swans. Ethology 119, 552–558. doi: 10.1111/eth.12094
Hemmi, J. M., and Pfeil, A. (2010). A multi-stage anti-predator response increases information on predation risk. J. Exp. Biol. 213, 1484–1489. doi: 10.1242/jeb.039925
Holmern, T., Setsaas, T. H., Melis, C., Tufto, J., and Røskaft, E. (2016). Effects of experimental human approaches on escape behavior in Thomson's gazelle (Eudorcas thomsonii). Behav. Ecol. 27, 1432–1440. doi: 10.1093/beheco/arw052
Kramer, D. L., and Bonenfant, M. (1997). Direction of predator approach and the decision to flee to a refuge. Animal Behav. 54, 289–295. doi: 10.1006/anbe.1996.0360
Mainini, B., Neuhaus, P., and Ingold, P. (1993). Behavior of marmots Marmota marmota under the influence of different hiking activities. Biol. Cons. 64, 161–164. doi: 10.1016/0006-3207(93)90653-I
Martín, J., and Lopez, P. (2000). Fleeing to unsafe refuges: effects of conspicuousness and refuge safety on the escape decisions of the lizard Psammodromus algirus. Can. J. Zool. 78, 265–270. doi: 10.1139/z99-212
Miller, S. G., Knight, R. L., and Miller, C. K. (2001). Wildlife responses to pedestrians and dogs. Wildl. Soc. Bull. 29, 124–132. doi: 10.2307/3783988
Møller, A. P. (2015). “Birds,” in Escaping From Predators: An Integrative View of Escape Decisions and Refuge Use, ed. J. W. E. Cooper and D. T. Blumstein (Cambridge: Cambridge University Press), 861–865.
Møller, A. P., Tryjanowski, P., Díaz, M., Kwiecinski, Z., Indykiewicz, P., Mitrus, C., et al. (2015). Urban habitats and feeders both contribute to flight initiation distance reduction in birds. Behav. Ecol. 26, 861–865. doi: 10.1093/beheco/arv024
Nair, A., Changsing, K., Stewart, W. J., and McHenry, M. J. (2017). Fish prey change strategy with the direction of a threat. Proc. Biol. Sci. 284:20170393. doi: 10.1098/rspb.2017.0393
Obleser, P., Hart, V., Malkemper, E. P., Begall, S., Holá, M., Painter, M. S., Burda, H., et al. (2016). Compass-controlled escape behavior in roe deer. Behav. Ecol. Sociobiol. 70, 1345–1355. doi: 10.1007/s00265-016-2142-y
R Core Team (2016). R: A Language and Environment for Statistical Computing. Vienna: R Foundation for Statistical Computing. Available online at: https://www.R-project.org/
Royan, A., Muir, A. P., and Downie, J. R. (2010). Variability in escape trajectory in the Trinidadian stream frog and two treefrogs at different life-history stages. Can. J. Zool. 88, 922–934. doi: 10.1139/Z10-059
Samia, D. S., and Blumstein, D. T. (2015). Birds Flush Early and Avoid the Rush: an Interspecific Study. PLos ONE 10:8. doi: 10.1371/journal.pone.0119906
Samia, D. S., Blumstein, D. T., Stankowich, T., and Cooper, W. E. Jr. (2016). Fifty years of chasing lizards: new insights advance optimal escape theory. Biol. Rev. Camb. Philos. Soc. 91, 349–366. doi: 10.1111/brv.12173
Shi, X., Møller, J. S., Højgaard, J., Johansen, J. L., Steffensen, J. F., Liu, D., et al. (2017). The angular position of a refuge affects escape responses in staghorn sculpin Leptocottus armatus. J. Fish. Biol. 90, 2434–2442. doi: 10.1111/jfb.13306
Stankowich, T., and Blumstein, D. T. (2005). Fear in animals: a meta-analysis and review of risk assessment. Proc. Biol. Sci. 272, 2627–2634. doi: 10.1098/rspb.2005.3251
Stankowich, T., and Coss, R. G. (2006). Effects of predator behavior and proximity on risk assessment by Columbian black-tailed deer. Behav. Ecol. 17, 246–254. doi: 10.1093/beheco/arj020
Stankowich, T., and Coss, R. G. (2007). Effects of risk assessment, predator behavior, and habitat on escape behavior in Columbian black-tailed deer. Behav. Ecol. 18, 358–367. doi: 10.1093/beheco/arl086
Tätte, K., Møller, A. P., and Mänd, R. (2018). Towards an integrated view of escape decisions in birds: relation between flight initiation distance and distance fled. Animal Behav. 136, 75–86. doi: 10.1016/j.anbehav.2017.12.008
Uchida, K., Suzuki, K., Shimamoto, T., Yanagawa, H., and Koizumi, I. (2016). Seasonal variation of flight initiation distance in Eurasian red squirrels in urban versus rural habitat. J. Zool. 298, 225–231. doi: 10.1111/jzo.12306
Woodbury, P. B. (1986). The geometry of predator avoidance by the blue crab, Callinectes sapidus rathbun. Animal Behav. 34, 28–37. doi: 10.1016/0003-3472(86)90003-5
Ydenberg, R. C., and Dill, L. M. (1986). The economics of fleeing from predators. Adv. Study Behav. 16, 229–249. doi: 10.1016/S0065-3454(08)60192-8
Keywords: flight initiation, flight trajectory, refuge, escape angle, squirrels
Citation: Eason PK, Nason LD and Alexander JE Jr (2019) Squirrels Do the Math: Flight Trajectories in Eastern Gray Squirrels (Sciurus carolinensis). Front. Ecol. Evol. 7:66. doi: 10.3389/fevo.2019.00066
Received: 31 October 2018; Accepted: 21 February 2019;
Published: 29 March 2019.
Edited by:
Evan Preisser, University of Rhode Island, United StatesReviewed by:
Theodore Stankowich, California State University, Long Beach, United StatesKeith Tarvin, Oberlin College, United States
Kunter Tätte, University of Tartu, Estonia
Copyright © 2019 Eason, Nason and Alexander. This is an open-access article distributed under the terms of the Creative Commons Attribution License (CC BY). The use, distribution or reproduction in other forums is permitted, provided the original author(s) and the copyright owner(s) are credited and that the original publication in this journal is cited, in accordance with accepted academic practice. No use, distribution or reproduction is permitted which does not comply with these terms.
*Correspondence: Perri K. Eason, perri.eason@louisville.edu