- 1Island Ecology and Evolution Research Group, Instituto de Productos Naturales y Agrobiología (CSIC), La Laguna, Spain
- 2Department of Bioscience, Aarhus University, Aarhus, Denmark
Birds and mammals are the only vertebrates which receive comprehensive attention in studies of dispersal of fleshy-fruited plant species. However, recently the importance of fleshy fruit in the diet of lizards (order Squamata: suborder Sauria), and their role as seed dispersers have been recognized in a number of studies, especially in studies from arthropod-poor habitats, such as oceanic islands. Here, we revisit the evidence of fruit-eating lizards on a global scale in order to test if fruit consumption is more common on islands than expected by chance. We constructed a database of 470 lizard species (from a global count of 6,515 species), that have been reported to consume fleshy fruits. This set of lizards belong to 27 families with Scincidae (N = 78 species), Gekkonidae (69), and Dactyloidae (55) having more frugivorous species than other lizard families. We found that 62.4% of these lizards inhabit islands, whereas only one third (35.3%) of all lizard species inhabit islands. These values support the presence of an “insular phenomenon,” however; we also tested if this biogeographical pattern might be driven by body size and evolutionary history of lizards. Thus, we looked for any phylogenetic signals in the distributions of lizard body size, island-presence, and frugivory and calculated phylogenetically corrected correlations among the three variables on a global subset of 2,417 lizard species for which we had detailed phylogenetic information. Both lizard body size and island-presence were weakly influenced by phylogeny; whereas, frugivory was not. In addition, we found that (1) body size and frugivory were weakly positively correlated; (2) body size and island-presence were uncorrelated; and (3) island-presence and frugivory were strongly positively correlated. Thus, we conclude that the main driver of frugivory on islands is the specific island environment and not lizard body size per se. Islands are said to be poor in arthropods and predators, and this may force/allow island lizards to forage for additional food sources, such as fleshy fruits. We also suggest that modern lizards as well as their ancestors may potentially play an important role to many plants as seed dispersers. However, we do not known how tight the correlation is between frugivory and seed dispersal. Thus, lizards repeatedly inspire us to ask new ecological and evolutionary questions.
Introduction
Birds and mammals are the principal seed dispersers of fleshy-fruited plant species (e.g., van der Pijl, 1982; Jordano, 2013). Fruit pulp is an important resource to these animals, which in return may promote an important ecosystem service, the dispersal of their seeds (e.g., Herrera and Pelmyr, 2002; Forget et al., 2011; Bascompte and Jordano, 2014; Wandrag et al., 2018). By dispersing seeds further away from the mother plants, seed mortality gets reduced and survival is favored if colonization happens to new microhabitats (e.g., Janzen, 1970; Howe and Smallwood, 1982; Cousens et al., 2008). In addition, seed dispersal promotes gene flow within and among plant populations (e.g., Rousset, 2004; Pérez-Méndez et al., 2018; Wandrag et al., 2018). Until recently, however, few studies have considered the role of other vertebrates as seed dispersers of fleshy-fruited plant species, e.g., fish, chelonians, crocodilians, and lizards (e.g., Olesen and Valido, 2003, 2004; Liu et al., 2004; Correa et al., 2007; Valido and Olesen, 2007; Platt et al., 2013; Falcón et al., 2018).
Here, we focus on lizards (order Squamata: suborder Sauria), because these animals are usually overlooked or dismissed as frugivores and seed dispersers. The main reason is that most are regarded as primarily feeding on small invertebrates (e.g., Greene, 1982; Cooper and Vitt, 2002; Pianka and Vitt, 2003), whereas only a few species bigger in size are herbivorous, i.e., folivorous (Supplementary Material: Herbivorous lizards). Thus, since Pough (1973) a relation between lizard body size and herbivory (specifically “folivory”) has been noted. In this respect, larger lizards would present some digestive and physiological modifications which favor an herbivorous diet (e.g., Iverson, 1982; Zimmerman and Tracy, 1989). This idea has also been extended to lizards with frugivorous habits, such as Anolis-like species whereas fruit intake has been related to large body size in some species (Herrel et al., 2004a). However, fruit pulp is an easily metabolized plant part, being low in fibers and proteins, and high in soluble carbohydrates (e.g., Valido et al., 2011; Jordano, 2013). Thus, lizards do not require large gape, strong bite or a large digestive system to process these items. In this respect, several studies have shown that small arthropod-eating lizards do consume fruit if their favorite arthropod food is scarce. This is most often observed on islands (e.g., Patterson, 1928; Vinson and Vinson, 1969; Schoener et al., 1982; Cheke, 1984; Whitaker, 1987; Pérez-Mellado and Corti, 1993; Valido and Nogales, 1994; Wotton, 2002; Valido et al., 2003; Hare et al., 2016; Wotton et al., 2016; Melzer et al., 2017; Parejo et al., 2018), where arthropods might be in short supply, but also in arthropod-poor habitats on mainland, such as high mountains and deserts (e.g., Fuentes, 1976; Mautz and Lopez-Forment, 1978; Hódar et al., 1996; Whiting and Greeff, 1997; Kiefer and Sazima, 2002; Espinoza et al., 2004; Valdecantos et al., 2012). By feeding from at least two trophic levels, these lizards become omnivorous. Generally, they do not have any morphological, digestive or physiological modifications related to their frugivorous diet (e.g., Valido and Nogales, 2003; Herrel et al., 2004b; Vidal and Sabat, 2010; but see Sagonas et al., 2015). Alongside their fruit diet choice, they may act as seed dispersers (e.g., Pérez-Mellado and Traveset, 1999; Olesen and Valido, 2003; Godínez-Álvarez, 2004; Valido and Olesen, 2007, 2010).
The full extent of this mutualistic plant-lizard interaction is unknown, because fruit rarely is scored as a separate component in diet studies. In the literature, plant material consumed by lizards is often pooled into one diet component, notwithstanding the wide range in energy content and digestability of various plant parts, e.g., foliage, which is difficult to digest because of the presence of cellulose. In the past, however, mutualistic reptiles were also present in the past (Supplementary Material: Mutualistic reptiles in the past), and the natural history literature is rich in stories about fruit-eating lizards (Supplementary Material: Early records of lizard-fruit interactions), but this knowledge is still not integrated into general ecology and evolutionary biology (see Miranda, 2017). Consequently, most reviews about seed dispersal focus on birds and mammals (Traveset et al., 2013; e.g., Jordano, 2013).
Here, our aim is (1) to present a detailed global overview of the extent of fleshy fruit consumption and seed dispersal in lizards, by mapping their geographical and taxonomical distribution, and (2) to test if insularity favors plant-lizard mutualism, if we control for lizard body size and phylogenetic relationships.
Materials and Methods
Collection Methods
Variation in collecting efforts and nomenclature changes make the global number of lizard species very dynamic, but on the 29th of August 2018, the number was 6,512 species (Uetz et al., 2018; Table 1). The taxonomy of lizards used here follows the European Molecular Biology Laboratory Reptile Database, EMBL. Two species were excluded from our analysis because their geographic range were missing in the EMBL, and, additionally, we included four new species from New Zealand and one from the Canary Islands (Hare et al., 2016; Table S1). Thus, our database included a total of 6,515 species sorted into 38 families, which further belonged to the infraorders Iguania, Gekkota, Scincomorpha, Diploglossa, Dibamia, and Platynota (Squamata: Sauria). Using this database, we classified lizards according to their geographical distribution, i.e., only island (I), only mainland (M), and both island and mainland (IM). We classified Australia as a mainland together with the other continents, whereas New Zealand, Madagascar, New Guinea, Borneo, Japan, and Taiwan were analyzed as islands. The borderline between island and mainland is obviously subjective. However, in island literature, Australia is most often regarded as a mainland, and here, we prefer to keep it that way. Several IM-species are introductions from their native islands to a mainland, e.g., from the Caribbean Islands to USA (e.g., Anolis cristatellus, Dactyloideae), from the Balearic Archipelago to the Iberian Peninsula (Podarcis pityusensis, Lacertidae), or vice versa, from USA to Japanese Ogasawara Islands (A. carolinensis), from North Africa to Menorca Island (Scelarcis perspicillata). Such species were here categorized as IM. Some lizard species are also island-to-island introductions (e.g., the lacertid Teira dugesii from Madeira to the Azores, and Gallotia stehlini from Gran Canaria to Fuerteventura). Finally, near-shore islets were regarded as part of their adjacent mainland or island. We also included the continuous variable lizard body size (maximum snout-vent length “max SVL”; Meiri, 2008, 2018), and the binary trait presence of fruits: 0 (absence) and 1 (presence) (see Supplementary Material: Quality of the data).
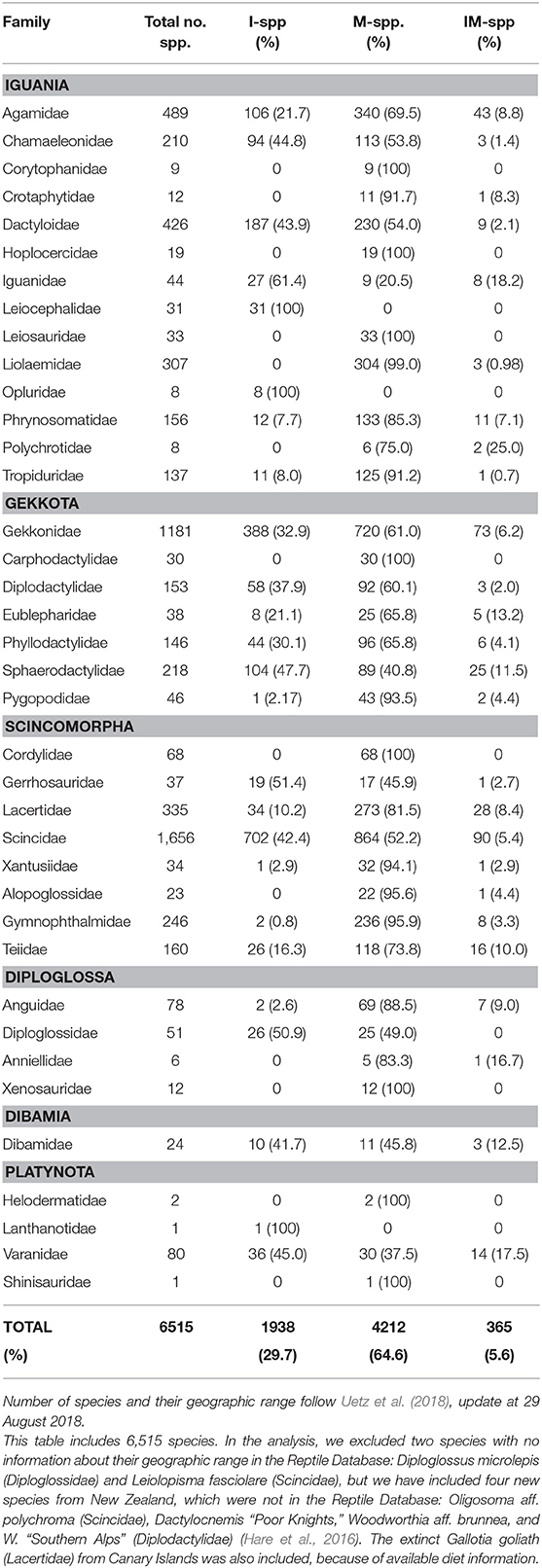
Table 1. Number of lizard species (Sauria) in the families of the infraorders Iguania, Gekkota, Scincomorpha, Diploglossa, Dibamia, and Platynota, sorted according to their geographic distribution: I, island-only species; M, mainland-only species; and IM, species present on both island and mainland.
First, we compared species frequencies of fruit-eating lizards on islands and mainland. Our null hypothesis was that the frugivorous lizards had a geographical distribution similar to lizards in general. This was tested with a Chi squared test of data from each family separately and for the global count of lizards. Globally, 29.7% of all lizard species are I-species, 64.6% only M-species, and 5.6% are IM-species (Table 1; Figure 1).
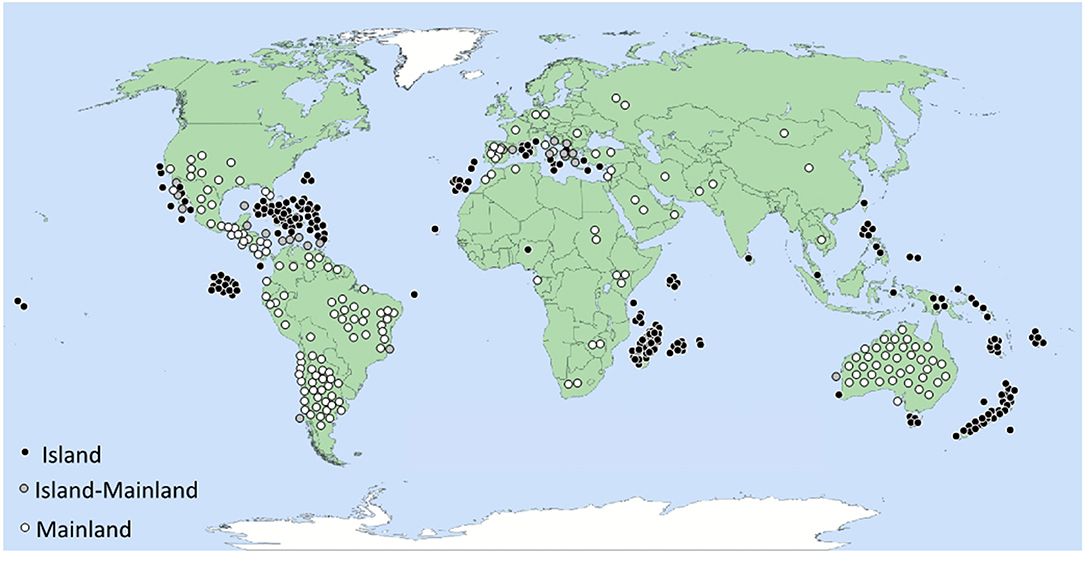
Figure 1. Geographic distribution of 470 fruit-eating lizard species sorted as island-only species (I), mainland-only species (M) and species present on both island and mainland (IM). See also Table S1 in Suplementary Material for a full list of frugivorous lizard species. The map shows not just the distribution of lizard frugivory, but also the heterogeneous sampling efforts. Note, for example, the absence of dots in Japan and Indonesia, which most likely is caused by insufficient field observation.
We also tested if the variables body size, frugivory, and island-presence in their distribution among lizards had a phylogenetic signal (see below). As backbone lizard phylogeny, we used the one published by Pyron et al. (2013). This phylogeny only included 2,847 lizard species. We pruned the phylogeny for those lizard species without any information about their max SVL or geographic distribution, resulting in 2,417 lizard species, which were included in our phylogenetic analysis.
Phylogenetic Signal
The phylogenetic signal of a trait is a measure of the statistical dependency among values of this trait on the phylogenetic relationships among species in the study sample (e.g., Blomberg and Garland, 2002). If other factors than phylogenetical relatedness influence trait variation, for example convergence due to related ecology, this will reduce the phylogenetic signal.
The phylogenetic signal of a continuous trait (max SVL) can be measured by Blomberg's K and Pagel's λ (Pagel, 1999; Blomberg et al., 2003). Both measures react slightly different to number of species included in the phylogeny, amount of information about branch length and number of polytomies (Münkemüller et al., 2012). For both measures, the actual observed value is compared to a null model of trait evolution, a Brownian motion (BM) evolutionary model (Blomberg et al., 2003), which is based upon either pure genetic drift, randomly varying selection, or varying stabilizing selection, but not on directional selection. According to this BM model, trait evolution follows a random walk along the branches of the phylogenetic tree. To test this null hypothesis of no phylogenetic signal, the observed values of the focal trait was compared with values expected under random (1,000 permutations) value distribution by using a likelihood ratio test (Münkemüller et al., 2012).
Both measures (K, λ) vary from 0 to 1, with values close to zero indicating lack of phylogenetic dependency, i.e., the trait has evolved in response to local selective processes. Whereas, a value close to 1 indicates an evolution according to the BM, i.e., a gradual accumulation of changes over time. K may also be larger than one, which indicates a strong phylogenetic signal. At least theoretically, λ might also become slightly larger than one (Münkemüller et al., 2012). The lower and upper bounds of K and λ indicate which of the two scenarios is the most likely.
The phylogenetic signal for binary traits (frugivory and island-presence) can be measured by D (phylogenetic dispersion) (Fritz and Purvis, 2010). D = (dobs – mean db)/(mean dr – mean db), where dobs is the number of trait state changes needed to get the observed trait state distribution in our phylogeny, db is the expected distribution of d under a BM model (1,000 permutations) and dr is the expected distribution of d, if trait states are randomly distributed among species. D typically varies from 0 to 1. D = 0 indicates that the trait evolves according to the Brownian model, BM (i.e., phylogenetic signal). D = 1 indicates that the trait evolves according to a random model (i.e., independent of the phylogeny), D > 1, if the trait is phylogenetically overdispersed, and D < 0, if the trait is more phylogenetically clustered than expected according to a BM model (Nunn, 2011).
The finding of a significant phylogenetic signal in some of these variables requires the use of comparative phylogenetic analysis to test for correlation between traits.
Phylogenetically Corrected Correlations
We tested for correlations between max SVL, island-presence and frugivory, using phylogenetically independent contrasts (PIC), i.e., any influence of statistical dependency among trait values was removed before the correlation analysis. The lizard body size (max SVL) data were log-transformed.
By using the lizard phylogeny (Pyron et al., 2013), and our compiled database including max SVL of lizards (Meiri, 2008, 2018), island-presence and frugivory (Table S1), we answered: 1. Is there, globally, any phylogenetic signal in the distribution of frugivory, body size, and island-presence in lizards; and 2. to what extent do island-presence and max SVL influence frugivory after correcting for any phylogenetical influence. For these phylogenetical analyses we used picante (Kembel et al., 2018), geiger (Harmon et al., 2016), caper (Orme et al., 2018), and ape (Paradis et al., 2018) R packages (R Core Team, 2014).
Results
Fruit-Eating Lizards
We found reports of 470 lizard species from 27 families and 128 genera consuming fleshy fruits (Tables 2, S1). Thus, 7.2% of all lizards use fleshy fruits to some extent in their diet. These species are widely distributed taxonomically, since 71% of all lizard families included some frugivorous members; Scincidae (N = 78 species), Gekkonidae (69), and Dactyloidae (55) being most frugivorous. Other lizard families with a high percentage of frugivorous species are Iguanidae (54.5%), Corytophanidae (44.4%), and Leiocephalidae (41.9%) (Tables 2, S1, Importance and examples of fleshy fruit to the diet of lizards).
Geographic Distribution of Frugivorous Lizards
64.6%, 29.7% and 5.6% of the 6,515 lizard species in the world inhabit mainlands, islands, and both, respectively (Table 1). However, frugivory in lizards were much more frequent among only island species (57.9% of all frugivorous lizards) than among only mainland species (37.6%). Only 4.5% of all frugivorous lizards were found on both island and mainland (Tables 2, S1). First, we tested the null-hypothesis H0: the frequencies of fruit-consuming lizard species on islands, mainland and both could be explained by the global geographical distribution of lizards. In this analysis, only the 27 lizard families with reported frugivory were included (N = 6,113 species). H0 was rejected (P < < 0.001; Table 2). Thus, the high frequency of fruit-consuming lizards on islands could not be explained by the general biogeography of the group. This analysis was repeated at family level. Island species were significantly overrepresented as fruit consumers in Dactyloidae, Gekkonidae, Diplodactylidae, and Lacertidae (Table 2). In contrast, in the Tropiduridae, frugivory was significantly more frequent on mainland than on islands (Table 2). Thus, worldwide fruit-consumption among lizards is almost twice as common on islands than on mainland, but on the family level there is some variation.
Phylogenetic Correlations
The distribution of max SVL showed a significant, but weak phylogenetical signal (K = 0.291; Table 3). Pagel's λ was closer to 1 than 0, but it was still significantly different from both values (95% confidence interval = [0.911; 0.941], and both the lower and upper bound were strongly unlikely; Table 3). Thus, K and λ showed that phylogeny, but also other factors, were important as drivers of body size variation in lizards. That lizard body size, in general, is influenced by both phylogenetic relationships and other factors (ecology) is certainly also what one would expect.
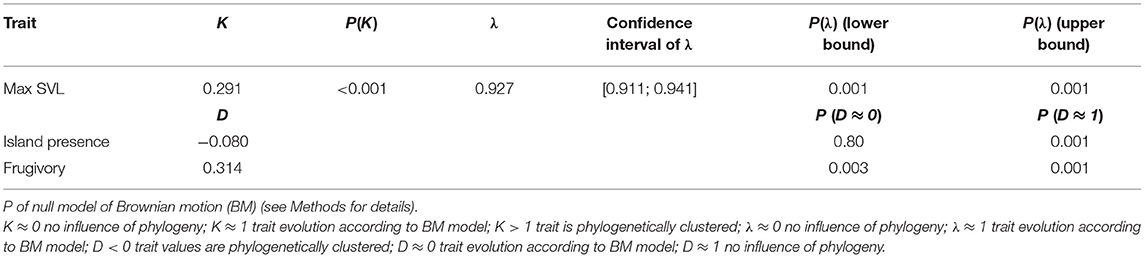
Table 3. Size of phylogenetic signal in the distribution of the continuous trait “max SVL” (K, λ), and the binary traits “island presence” and “frugivory” (D) of 2,417 lizard species, according to the phylogeny of Pyron et al. (2013).
Our null hypothesis, stating that any presence or absence of lizards on islands is influenced of phylogeny, was not rejected (P = 0.80; Table 3), and this binary trait was distributed according to a Brownian motion model (D ≈ 0; Table 3). We would also expect evolution to have played a role here, especially because many lizard radiations are endemic to islands.
For the binary trait frugivory, the null model was rejected (P = 0.003). Thus, the trait was not distributed according to a Brownian motion model of evolution (D ≈ 1). However, D ≈ 1 (no influence of phylogeny) was also rejected (P < 0.001). Thus, both phylogeny and other factors (ecological) play a role in the distribution of frugivory among lizards.
After correcting for any influence of phylogeny (PIC, phylogenetically independent contrasts; Table 4), we found (1) that body size (max SVL) and frugivory were positively, but weakly correlated; (2) that body size and island-presence were uncorrelated; and (3) that island-presence and frugivory were strongly positively correlated. Thus, in our database, frugivory was significantly associated with insular species after correcting for any influence of evolutionary relationships.
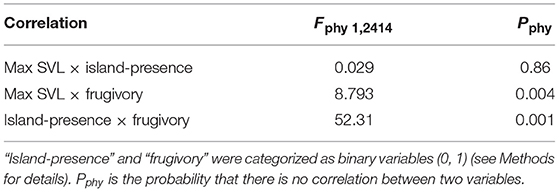
Table 4. Phylogenetic independent contrasts (PIC) correlations between “max SVL,” “frugivory,” and “island-presence” of 2,417 lizard species, based on the phylogeny of Pyron et al. (2013).
Discussion
In this review, we reported that 470 lizard species consume fleshy fruit, many more than previously thought (Cooper and Vitt, 2002; Olesen and Valido, 2003; Godínez-Álvarez, 2004; Valido and Olesen, 2007; Meiri, 2018). However, it is still low compared to equivalent estimates for birds (around 4,000 bird species consume fleshy fruit, Wenny et al., 2016), but similar to mammals (460 species are primarily frugivorous, Fleming and Sosa, 1994). In addition, 182 Neotropical freshwater fish are fruit eaters (Correa et al., 2007), and recently Falcón et al. (2018) reported that up to 72 species of turtles include fleshy fruits in their diet.
However, for several reasons our number of frugivorous lizards is clearly an under-estimate: For example: (1) modern researchers have just recently begun to pay more attention to lizards as frugivores/plant mutualists; (2) many omnivorous (i.e., with 10–90%volume (V) plant matter in their diet) and herbivorous (>90%V) lizard examples reported in e.g., Cooper and Vitt (2002) were not incorporated in our database since the original reports did not specify which vegetative parts were consumed, and these species may use fleshy fruit as well; (3) lizard diet sampling conducted outside the fruiting period will not detect frugivory, and (4) for most lizards we did not find any data about their diet, particularly for endemics inhabiting remote islands, although some of their congenerics are in our database. Thus, we believe that many more fruit-eating and seed-dispersing lizards are waiting to be discovered.
Evolution of Frugivorous Lizards
Irrespectively of recent finding of an herbivorous lizard from early Cretaceous (Evans and Manabe, 2008), carnivory is the ancestral feeding mode in modern lizard species and most species are still exclusively or mainly carnivorous (e.g., Cooper and Vitt, 2002). However, trends toward true herbivory are seen repeatedly (Iverson, 1982; King, 1996; Cooper and Vitt, 2002), e.g., ~18 herbivory origins within the South American Liolaemus (Espinoza et al., 2004). These lizards are smaller (max. SVL <100 mm) than other herbivorous species, and also inhabit mainland cold areas. Thus, small montane lizards may keep a higher body temperature (Vitt, 2004), being necessary for microbial hindgut endosymbionts, by digesting plant fibers. Cooler habitats have fewer insects and selection may favor a switch to herbivory (Pearson, 1954; Fuentes and Di Castri, 1975; Jaksic, 1978). However, the rarity of plant-eating lizards also suggests that herbivory in lizards is constrained (Szarski, 1962; Ostrom, 1963; Sokol, 1967; Pough, 1973; Espinoza et al., 2004). Here, we demonstrate that the use of easily digestible plant matter (i.e., fleshy pulp) is relatively frequent, occurring in several lizard lineages. However, the extent of this shift to frugivory in lizards is affected by insularity and body size.
Insularity
One third of all lizard species live on islands, but two thirds of all the fruit-consuming lizards are reported from islands. This geographical pattern is not caused by a higher number of scientists working both on seed dispersal (e.g., Estrada and Fleming, 1986; Fleming and Estrada, 1993; Levey et al., 2002; Dennis et al., 2007; and references therein) or diet of lizards on islands compared to mainland habitats (Meiri, 2018). In addition, the pattern is not caused by a presence of more fleshy-fruited plant species on islands compared to mainland habitats; on the contrary, islands have fewer fleshy-fruited plant species than comparable mainland habitats (e.g., Heleno and Vargas, 2015). Herrel et al. (2004a) compared the geographic distribution of frugivory among 45 island and 16 mainland Anolis species and also demonstrated that fruit in the diet of lizards is more common on islands (90% of all cases) than on mainland (10%). Analyzing many lizard species, but pooling all dietary plant parts, earlier studies also found this relationship (e.g., van Damme, 1999; Cooper and Vitt, 2002). However, island lizards include fleshy fruit, but also other vegetable food items, into their diet because they may be food limited (Janzen, 1973; Andrews, 1979; Schluter, 1984; Pérez-Mellado and Corti, 1993; van Damme, 1999; Cooper and Vitt, 2002; Polis et al., 2002; Olesen and Valido, 2003; Barret et al., 2005). This recurrent island phenomenon is an example of “niche expansion” or interaction release (sensu Traveset et al., 2015) and was first demonstrated for island birds (Grant, 1966; MacArthur et al., 1972). This pattern is possibly caused by a three-step process: 1) Compared to mainland, islands usually support fewer insect taxa (Gulick, 1932; MacArthur and Wilson, 1967) of a lower overall abundance (Allan et al., 1973; Janzen, 1973); 2) island lizards are less exposed to interspecific competition and predation because of a general low species density (MacArthur et al., 1972; Case, 1975; Andrews, 1979), and 3) some island lizard species respond to 2) by density compensation (MacArthur et al., 1972; Case, 1975; Rodda et al., 2001), resulting in intense intraspecific competition and consequently an expansion of their feeding niche toward the use of alternative resources, such as fleshy fruits (Olesen and Valido, 2003). However, only scant information is available on island arthropod abundance (Allan et al., 1973; Janzen, 1973; Andrews, 1979; Case, 1982; Faeth, 1984), but the impression from the general island biology literature is that arthropods are less abundant on islands and that they do not density compensate. On tiny islets, however, where coastline habitats dominate, flies functioning as decomposers of algae may be so abundant (Polis et al., 2002), that, at least theoretically, arthropod-eating lizards may be less inclined to shift to fruit.
On many islands, lizards have higher densities than related groups from adjacent mainland (Rodda and Dean-Bradley, 2002; Buckley and Jetz, 2007). Exceptionally high densities of lizards on islands have attracted considerable attention (Case, 1975; Case and Bolger, 1991; Rodda et al., 2001; Buckley and Jetz, 2007). The world record seems to be >5 Sphaerodactylus macrolepis individuals/m2 in the Virgin Islands (Rodda et al., 2001). Insular founders may reach such high densities in just 3 years (Schoener, 1989). Density compensation in island lizards is a ubiquitous and global phenomenon, and total island lizard density is an order of magnitude higher than on mainland (128 vs. 1,920 individuals/ha) (Rodda et al., 2001; Buckley and Jetz, 2007). Many of these density-compensating island lizards are also present in our database (genera Anolis, Gallotia, Gehyra, Hemidactylus, Lepidodactylus, Oligosoma, Phelsuma, Podarcis, Xantusia, etc.). Reduction in species richness of predators (e.g., birds of prey) and number of competitors (insectivorous birds) may be the dominant drivers of lizard abundance on islands (Schoener and Schoener, 1978; Andrews, 1979; Wright, 1979; Buckley and Jetz, 2007). Density compensation in fruit-consuming lizards may thus be of high importance to seed dispersal in many plant species, influencing their chance of colonization and establishment on small islands, and their general population structure. Besides, the poor ability of mammals to reach remote islands will leave part of the diet niche dimension empty for other animal groups to explore (Whittaker and Fernández-Palacios, 2007).
Fruit -eating lizards are also observed in mainland habitats poor in arthropods. Frugivory in lizards inhabiting desert-like habitats (e.g., Clark and Comanor, 1976; Vitt et al., 1981; Hódar et al., 1996; Whiting and Greeff, 1997; Belver and Avila, 2002), high mountains (e.g., Hurtubia and Di Castri, 1973; Fuentes, 1976), cerrado habitats (e.g., Vitt, 1993; Mesquita and Colli, 2003) and caves (Mautz and Lopez-Forment, 1978) make up most of the mainland observations. Seasonal scarcity of arthropods and frugivory in lizards are also reported (e.g., Schleich et al., 1996; Duffield and Bull, 1998; Fialho et al., 2000). Thus, the arthropod-scarcity hypothesis can also explain mainland observations of lizards as fruit eaters.
Lizard Body Size
Since the review by Pough (1973) about lizard body size and herbivory diet, the generalization that only lizards >300 g (>217 mm SVL) are truly herbivorous and that medium-sized lizards, 100–300 g (150–217 mm SVL) are omnivorous have become a dogma in lizard ecology. It has been used to explain the paucity of modern, truly herbivorous lizards. However, a debate about this has also been running for more than 50 years (Szarski, 1962; Ostrom, 1963; Sokol, 1967; Pough, 1973; Iverson, 1982; van Devender, 1982; Auffenberg, 1988; King, 1996; van Damme, 1999; Cooper and Vitt, 2002; Cooper, 2003; Espinoza et al., 2004; Herrel et al., 2004a,b, 2008), including issues about predation risk, insularity, lizard body size, and digestive and physiological adaptative modifications. However, Espinoza et al. (2004), and more recently Vervust et al. (2010) demonstrated true herbivory in smaller species (max. SVL <100 mm) than in other known herbivorous species. These species were inhabiting insular (-like) habitats and true islands.
Many small (<150 mm SVL) lizards are being classified as frugivorous, e.g., species of Gallotia, Podarcis (Lacertidae), Anolis (Dactyloidae), Carinascincus, Trachylepis (Scincidae), Gehyra, Phelsuma (Gekkonidae), Platysaurus (Cordylidae), Ameiva, Cnemidophorus (Teiidae), Leiocephalus (Leiocephalidae), Microlophus (Tropiduridae), and Lepidophyma smithii (Xantusidae). Large lizards have large gapes and a strong bite (e.g., Herrel et al., 1999, 2004a,b), and this may be advantageous if the diet is vegetarian, because it allows the lizards to crush the material efficiently (Szarski, 1962; Sokol, 1967). Fleshy fruits, on the other hand, do not require this.
Frugivorous Lizards as Legitimate Seed Dispersers
Lizards do not fully chew their food and seeds passing through their gut may remain intact. Reviewing experimental studies of 40 plant species and 17 lizard species, Traveset (1998) and A. Valido (unpublished) concluded that seeds dispersed by lizards germinated just as well as seeds dispersed by frugivorous birds and mammals. The proportions of experiments in which germination of seeds was enhanced (25%), unaffected (57%) and inhibited (18%) after lizard gut passage were similar to figures for seed-dispersing birds (36, 48, and 16%, respectively), non-flying mammals (39%, 42%, 19%), and bats (25%, 67%, 8%). Here, we summarize some of these results.
Iverson (1985) detected a 6% increase in seed germination of Coccoloba uvifera (Polygonaceae) after passage through the gut of Cyclura compared to controls. Studies of other rock iguanas gave similar results. Within Lacertidae, a significant increase in germination in Gallotia-consumed seeds of Withania aristata (Solanaceae) has been reported (Valido and Nogales, 1994). Other reports from the Canaries and Balearics show similar results (Nogales et al., 1998; Castilla, 2000; Pérez-Mellado and Riera, 2004; Pérez-Mellado et al., 2005; Rodríguez-Pérez et al., 2005). Thus, many of these island lizards may contribute to plant fitness. However, for Cnemidophorus murinus from Bonaire Island, Lesser Antilles, Schall (1996) did not find any germination response for Erithalis fruticosa (Rubiaceae).
In Brazilean Melocactus violaceus (Cactaceae), 36% of the seeds passing through Tropidurus torquatus germinated compared to no germination at all for controls (Côrtes-Figueira et al., 1994). Fruits of Melocactus species appear to be consumed by lizards only (Dearing and Schall, 1992). On Chiloé Island, Chile, Rubiaceae seeds defecated by Liolaemus pictus germinated better or to the same extent as controls (Willson et al., 1996). Seeds of Genipa americana (Rubiaceae) and Cereus peruvianus (Cactaceae) consumed by Salvator merianae germinated significantly better and or to the same extent as controls, respectively (De Castro and Galetti, 2004). Vasconcellos-Neto et al. (2009) showed that seeds of Solanum thomasiifolium (Solanaceae) were dispersed less frequently by lizards (4%) than by birds (77%) and foxes (19%), but with higher germination rate (80%) compared to birds (64%) and foxes (53%).
The digestive treatment of seeds seems to be quite similar among different frugivore groups (Valido and Olesen, 2007). Although examples are still scarce, lizards seem to be in the same seed disperser league as the classical and better studied frugivorous birds and mammals. However, the series of seed germination studies mentioned above suggests that frugivory may not just lead to dispersal of seeds, but also to dispersal of viable seeds, which may germinate and increase plant fitness. However, we do not know how strong frugivory is as a proxy for plant fitness, and we also lack an experimental comparison of seed germination after passage through sympatric native lizards, birds and mammals, with the control treatment of intact fruits (Samuels and Levey, 2005).
Conclusions
Many lizard species are potential seed dispersers, and fruit feeding among lizards is taxonomically and geographically widespread, including 7.2% of all lizard species. It is especially important in species and populations inhabiting islands (62.4% of all reported cases). Finally, compared to other, more classical vertebrate mutualists (birds, mammals) seed dispersal by lizards is relatively poorly documented, but may be just as effective in terms of the quantitative and qualitative component of the seed dispersal effectiveness (sensu Schupp et al., 2010).
We believe that the results presented here are only the tip of the “lizard-plant seed dispersal iceberg.” Thus, we find that fruit is an important supplementary diet component for island lizards. Other easily digestable plant material, like nectar and pollen, may show the same difference between islands and mainland, whereas we expect consumption of fiber-rich plant parts to show a deviating pattern. In general, much about lizard diet and its ecological and evolutionary consequences remain unknown. Our review may encourage ecologists, herpetologists, island biologists and natural historians in general to pay more attention to this type of plant-animal interaction, which may expand our general understanding of the ecology and evolution of mutualisms. For several decades, lizards have offered us outstanding opportunities for many kinds of ecological and evolutionary study (e.g., Pianka and Vitt, 2003; Losos, 2009), and here a new research line about lizard-plant mutualisms is suggested.
Data Availability
The datasets analyzed for this study are available in Supporting Information (Table S1) and the lizard phylogeny in Pyron et al. (2013).
Author Contributions
All authors listed have made a substantial, direct and intellectual contribution to the work, and approved it for publication.
Funding
This research was supported by the Ministerio de Economía y Competitividad (CGL2L013-47429-P), Severo Ochoa Excellence Award (SEV-2012-0262), and by a Ramón y Cajal research program (RYC-20077-00620) to AV, and the Danish Science Research Council and Carlsberg Foundation to JO.
Conflict of Interest Statement
The authors declare that the research was conducted in the absence of any commercial or financial relationships that could be construed as a potential conflict of interest.
The handling editor declared a shared affiliation, though no other collaboration, with one of the authors, AV, at time of review.
Acknowledgments
We are grateful to many researchers around the world, contributing with original data, references, and personal communications about lizards feeding on fleshy fruits (see Table S1 for a complete list of personal communications). We also thank Casper H. Van Leeuwen, Jose Luis Tella, and Andy J. Green for the invitation to participate in this issue. The manuscript benefited from constructive comments by Andy J. Green, Sean T. Giery, and Daniel Wenny.
Supplementary Material
The Supplementary Material for this article can be found online at: https://www.frontiersin.org/articles/10.3389/fevo.2019.00049/full#supplementary-material
References
Allan, J. D., Barnthhouse, L. W., Prestbye, R. A., and Strong, D. R. (1973). On foliage arthropod communities of Puerto Rican second growth vegetation. Ecology 54, 628–632. doi: 10.2307/1935350
Andrews, R. M. (1979). Evolution of life histories: a comparison of Anolis lizards from matched island and mainland habitats. Breviora 454, 1–51.
Barret, K., Anderson, W. B., Wait, D. A., Grismer, L. L., Polis, G. A., and Rose, M. D. (2005). Marine subsidies alter the diet and abundance of insular and coastal lizard populations. Oikos 109, 145–153. doi: 10.1111/j.0030-1299.2005.13728.x
Bascompte, J., and Jordano, P. (2014). Mutualistic Networks. Princeton NJ: Princeton University Press.
Belver, L. C., and Avila, L. J. (2002). Diet composition of Liolaemus bibronii (Iguania: Liolaemidae) in Southern Rio Negro province, Argentine. Herpetol. J. 12, 39–42.
Blomberg, S. P., and Garland, T. (2002). Tempo and mode in evolution: phylogenetic inertia, adaptation and comparative methods. J. Evol. Biol 15, 899–910. doi: 10.1046/j.1420-9101.2002.00472.x
Blomberg, S. P., Garland, T., and Ives, A. R. (2003). Testing for phylogenetic signal in comparative data: behavioral traits are more labile. Evolution 57, 717–745. doi: 10.1111/j.0014-3820.2003.tb00285.x
Buckley, L. B., and Jetz, W. (2007). Insularity and the determinants of lizard population density. Ecol. Lett. 10, 481–489. doi: 10.1111/j.1461-0248.2007.01042.x
Côrtes-Figueira, J. E., Vasconcellos-Neto, J., García, M. A., and Teixeira, A. L. (1994). Saurochory in Melocactus violaceus (Cactaceae). Biotropica 26, 295–301.
Case, T. J. (1975). Species numbers, density compensation, and colonising ability of lizards on islands in the Gulf of California. Ecology 56, 3–18. doi: 10.2307/1935296
Case, T. J. (1982). “Ecology and evolution of the insular gigantic chuckawallas, Sauromalus hispidus and Sauromalus varius,” in Iguanas of the World. Their Behavior, Ecology and Conservation, eds G. M. Burghardt and A. S. Rand (Park Ridge, NJ: Noyes), 184–212.
Case, T. J., and Bolger, D. T. (1991). The role of interspecific competition in the biogeography of island lizards. TREE 6, 135–139. doi: 10.1016/0169-5347(91)90093-D
Castilla, A. M. (2000). Does passage time through the lizard Podarcis lilfordi's guts affect germination performance in the plant Withania frutescens? Acta Oecol. 21, 119–124. doi: 10.1016/S1146-609X(00)00120-X
Cheke, A. S. (1984). “Lizards of the Seychelles,” in Biogeography and Ecology of the Seychelles Islands. eds D. R. Stoddart (Junk, The Hague), 331–360.
Clark, W. H., and Comanor, P. L. (1976). The northern Desert Horned Lizard, Phrynosoma platyrhinos platyrhinos, as a predator of the Western Harvester Ant, Pogonomyrmex occidentalis, and a dispersal agent for Eriogonum baileyi. J. Idaho Acad. Sci. 12, 9–12.
Cooper, W. E. (2003). Correlated evolution of herbivory and food chemical discrimination in iguanian and ambush foraging lizards. Behav Ecol. 14, 409–416. doi: 10.1093/beheco/14.3.409
Cooper, W. E., and Vitt, L. J. (2002). Distribution, extent, and evolution of plant consumption by lizards. J. Zool. 257, 487–517. doi: 10.1017/S0952836902001085
Correa, S. B., Winemiller, K. O., López-Fernández, H., and Galetti, M. (2007). Evolutionary perspectives on seed consumption and dispersal by fishes. BioSciences 57, 748–756. doi: 10.1641/B570907
Cousens, R., Dytham, C., and Law, R. (2008). Dispersal in Plants: A Population Perspective. Oxford: Oxford University Press. doi: 10.1093/acprof:oso/9780199299126.001.0001
De Castro, E., and Galetti, M. (2004). Frugivoria e dispersao de sementes pelo lagarto Teiú Tupinambis merianae (Reptilia: Teiidae). Papéis Avulsos de Zoologia 44, 91–97. doi: 10.1590/S0031-10492004000600001
Dearing, M. D., and Schall, J. J. (1992). Testing models of optimal diet assembly by the generalist herbivorous lizard Cnemidophorus murinus. Ecology 73, 845–858. doi: 10.2307/1940162
Dennis, A. J., Green, R. A., Schupp, E. W., and Westcott, D. A. (2007). Seed Dispersal: Theory and its Application in a Changing World. Wallingford, CT: CABI Publishing. doi: 10.1079/9781845931650.0000
Duffield, G. A., and Bull, C. M. (1998). Seasonal and ontogenetic changes in the diet of the Australian skink Egernia stokesii. Herpetologica 54, 414–419.
Espinoza, R. E., Wiens, J. J., and Tracy, C. R. (2004). Recurrent evolution of herbivory in small, cold-climate lizards: Breaking the ecophysiological rules of reptilian herbivory. Proc. Natl. Acad. Sci. U. S. A. 101, 16819–16824. doi: 10.1073/pnas.0401226101
Estrada, R., and Fleming, T. H. (1986). Frugivores and Seed Dispersal. Dordrecht: Dr W. Junk Publishers. doi: 10.1007/978-94-009-4812-9
Evans, S. E., and Manabe, M. (2008). An early herbivorous lizard from the lower cretaceous of Japan. Palaeontology 51, 487–498. doi: 10.1111/j.1475-4983.2008.00759.x
Faeth, S. H. (1984). “Density compensation in vertebrates and invertebrates: a review and an experiment,” in Ecological Communities: Conceptual Issues and the Evidence. eds D. R. Strong, D. Simberloff, L. G. Abele and A. B. Thistle (Princeton, NJ: Princeton University Press), 491–509.
Falcón, W., Moll, D., and Hansen, D. M. (2018). Frugivory and Seed Dispersal by Chelonians: A Review and Synthesis. bioRxiv [Preprint]. doi: 10.1101/379933
Fialho, R. F., Rocha, C. F. D., and Vrcibradic, D. (2000). Feeding ecology of Tropidurus torquatus: Ontogenetic shift in plant consumption and seasonal trends in diet. J. Herpetol. 34, 325–330. doi: 10.2307/1565437
Fleming, T. H., and Estrada, R. (1993). Frugivory and Seed Dispersal: Ecological and Evolutionary Aspects. Bruxelles: Kluwer Academic Publishers. doi: 10.1007/978-94-011-1749-4
Fleming, T. H., and Sosa, V. J. (1994). Effects of nectarivorous and frugivorous mammals on reproductive success of plants. J. Mammal. 75, 845–851. doi: 10.2307/1382466
Forget, P.-M., Böhning-Gaese, K., Jordano, P., Lambert, J. E., Traveset, A., and Wright, J. S. (2011). Frugivores and seed dispersal: Mechanisms and consequences of a key interaction for biodiversity. Acta Oecologica 37, 517–682. doi: 10.1098/rsbl.2010.0986
Fritz, S. A., and Purvis, A. (2010). Selectivity in mammalian extinction risk and threat types: a new measure of phylogenetic signal strength in binary traits. Conserv. Biol. 24, 1042–1051. doi: 10.1111/j.1523-1739.2010.01455.x
Fuentes, E. R. (1976). Ecological convergence of lizard communities in Chile and California. Ecology 57, 3–17. doi: 10.2307/1936394
Fuentes, E. R., and Di Castri, F. (1975). Ensayo de herbivoría experimental en especies de Liolaemus (Iguanidae) chilenos. Anales del Museo de Historia Natural Valparaíso 8, 66–75.
Godínez-Álvarez, H. (2004). Pollination and seed dispersal by lizards: a review. Rev. Chilena Hist. Nat. 77, 569–577. doi: 10.4067/S0716-078X2004000300015
Grant, P. R. (1966). Ecological compatibility of bird species on islands. Am. Nat. 100, 451–462. doi: 10.1086/282438
Greene, H. W. (1982). “Dietary and phenotypic diversity in lizards: why are some organisms specialised?,” in Environmental Adaptation and Evolution, eds D. Mossakowski and G. Roth (Stuttgart: Fischer Verlag), 107–128.
Gulick, A. (1932). Biological peculiarities of oceanic islands. Q Rev Biol. 7, 405–427. doi: 10.1086/394416
Hare, K. M., Chapple, D. G., Towns, D. R., and van Winkel, D. (2016). “The ecology of New Zealand‘s lizards,” in New Zealand Lizards, ed D. G. Chapple (New York, NY: Springer), 133–168.
Harmon, L., Weir, J., Brock, C., Glor, R., Challenger, W., Hunt, G., et al. (2016). Geiger: Analysis of Evolutionary Diversification. R package, v. 2.4-0 (2017). Available online at: http://cran.r-project.org/package=geiger
Heleno, R., and Vargas, P. (2015). How do islands become green? Global Ecol. Biogeogr. 24, 518–526. doi: 10.1111/geb.12273
Herrel, A., Aerts, P., Fret, J., and De Vree, F. (1999). Morphology of the feeding system in agamid lizards: ecological correlates. Anatomical Record 254, 496–507. doi: 10.1002/(SICI)1097-0185(19990401)254:4<496::AID-AR5>3.0.CO;2-Q
Herrel, A., Huyghe, K., Vanhooydonck, B., Backeljau, T., Breu,-, K., Grbac, I., et al. (2008). Rapid large scale evolutionary divergence in morphology and performance associated with exploitation of a different dietary resource. Proc. Natl. Acad. Sci. U. S. A. 105, 4792–4795. doi: 10.1073/pnas.0711998105
Herrel, A., Vanhooydonck, B., and Joachim, R. (2004a). Frugivory in polychrotid lizards: effects of body size. Oecologia 140, 160–168. doi: 10.1007/s00442-004-1558-7
Herrel, A., Vanhooydonck, B., and van Damme, R. (2004b). Omnivory in lacertid lizards: adaptive evolution or constraint? J. Evol. Biol. 17, 974–984. doi: 10.1111/j.1420-9101.2004.00758.x
Herrera, C. M., and Pelmyr, O. (2002). Plant–animal Interactions: An Evolutionary Approach. Oxford: Blackwell Science.
Hódar, J. A., Campos, F., and Rosales, B. A. (1996). Trophic ecology of the Ocellated Lizard Lacerta lepida in an arid zone of southern Spain: relationships with availability and daily activity of prey. J. Arid Env. 33, 95–107.
Howe, H. F., and Smallwood, J. H. (1982). Ecology of seed dispersal. Ann. Rev. Ecol. Syst. 13, 201–228. doi: 10.1146/annurev.es.13.110182.001221
Hurtubia, J., and Di Castri, F. (1973). “Segregation of lizard niches in the Mediterranean region of Chile,” in Mediterranean Type Ecosystems. Origin and Structure. eds. F. di Castri and H. A. Mooney (Berlin: Springer), 349–360.
Iverson, J. B. (1982). “Adaptations to herbivory in iguanine lizards,” in Iguanas of the World, eds G. M. Burghardt and A. S. Rand (New Jersey, NY: Noyes), 60–76.
Jaksic, F. (1978). ¿A qué tamaño se hacer herbívora una lagartija? Anales del Museo de Historia Natural Valparaíso 11, 113–116.
Janzen, D. H. (1970). Herbivores and the number of tree species in tropical forests. Am. Nat. 104, 501–528. doi: 10.1086/282687
Janzen, D. H. (1973). Sweep samples of tropical foliage insects: effects of seasons, vegetation types, elevation, time of day, and insularity. Ecology 54, 687–708. doi: 10.2307/1935359
Jordano, P. (2013). “Fruits and frugivory,” in Seeds the Ecology of Regeneration in Plant Communities. ed R. S. Gallagher (Oxfordshire, UK: CAB International), 18–61.
Kembel, S. W., Ackerly, D. D., Blomberg, S. P., Cornwell, W. K., Cowan, P. D., Helmus, M. R., et al. (2018). Picante: Integrating Phylogenies and Ecology. R package, v. 2.4-0 (2017). Available online at: http://cran.r-project.org/package=picante
Kiefer, M. C., and Sazima, I. (2002). Diet of juvenile tegu lizard Tupinambis merianae (Teiidae) in southeastern Brazil. Amphibia-Reptilia 23, 105–108.
Levey, D. J., Silva, W. R., and Galetti, M. (2002). Seed Dispersal and Frugivory: Ecology, Evolution and Conservation. Wallingford, CT: CABI Publishing.
Liu, H., Platt, S. G., and Christopher, K. B. (2004). Seed dispersal by the Florida box turtle (Terrapene carolina bauri) in pine rockland forests of the lower Florida Keys, United States. Oecologia 138, 539–546. doi: 10.1007/s00442-003-1445-7
Losos, J. B. (2009). Lizards in an Evolutionary Tree: Ecology and Adaptive Radiation of Anoles. Berkeley, CA: University of California Press.
MacArthur, R. H., Diamond, J. M., and Karr, J. R. (1972). Density compensation in island faunas. Ecology 53, 330–342. doi: 10.2307/1934090
MacArthur, R. H., and Wilson, E. O. (1967). The Theory of Island Bigeography. Princeton, NJ: Princeton University Press.
Mautz, W. J., and Lopez-Forment, W. (1978). Observations on the activity and diet of the cavernicolous lizard Lepidophyma smithii (Sauria: Xantusiidae). Herpetologica 34, 311–313.
Meiri, S. (2008). Evolution and ecology of lizard body sizes. Global Ecol. Biogeogr. 17, 724–734. doi: 10.1111/j.1466-8238.2008.00414.x
Meiri, S. (2018). Traits of lizards of the world: variation around a successful evolutionary design. Global Ecol. Biogeogr. 27, 1168–1172. doi: 10.1111/geb.12773
Melzer, S., Bell, T., and Patterson, G. B. (2017). Hidden conservation vulnerability within a cryptic species complex: taxonomic revision of the spotted skink (Oligosoma lineoocellatum; Reptilia: Scincidae) from New Zealand. Zootaxa 4300, 355–379. doi: 10.11646/zootaxa.4300.3.2
Mesquita, D. O., and Colli, G. R. (2003). The ecology of Cnemidophorus ocellifer (Squamata, Teiidae) in a Neotropical Savanna. J. Herpetol. 37, 498–509. doi: 10.1670/179-02A
Miranda, E. B. P. (2017). The plight of reptiles as ecological actors in the Tropics. Front. Ecol. Evol. 5, 159. doi: 10.3389/fevo.2017.00159
Münkemüller, T., Lavergne, S., Bzeznik, B., Dray, S., Jombart, T., Schiffers, K., et al. (2012). How to measure and test phylogenetic signal. Methods Ecol. Evol. 3, 743–756. doi: 10.1111/j.2041-210X.2012.00196.x
Nogales, M., Delgado, J. D., and Medina, F. M. (1998). Shrikes, lizards and Lycium intricatum (Solanaceae) fruits: a case of indirect seed dispersal on an oceanic island (Alegranza, Canary Islands). J. Ecol. 86, 866–871. doi: 10.1046/j.1365-2745.1998.8650866.x
Nunn, C. L. (2011). The Comparative Method in Evolutionary Anthropology and Biology. Chicago: University of Chicago Press. doi: 10.7208/chicago/9780226090009.001.0001
Olesen, J. M., and Valido, A. (2003). Lizards as pollinators and seed dispersers: an island phenomenon. Tree 18, 177–181. doi: 10.1016/S0169-5347(03)00004-1
Olesen, J. M., and Valido, A. (2004). “Lizards and birds as generalized pollinators and seed dispersers of island plants,” in Island Ecology, eds J. M. Fernández-Palacios and C. Morici (Canary Islands, AEET/Cabildo Insular de La Palma), 229–249.
Orme, D., Freckleton, R., Thomas, G., Petzoldt, T., Fritz, S., Isaac, N., et al. (2018). The Caper Package: Comparative Analysis of Phylogenetics and Evolution in R. R package, v. 1.0.1 (2018). Available online at: http://cran.r-project.org/package=caper
Ostrom, J. H. (1963). Further comments on herbivorous lizards. Evolution 17, 368–369. doi: 10.1111/j.1558-5646.1963.tb03290.x
Pagel, M. (1999). Inferring the historical patterns of biological evolution. Nature 401, 877–884. doi: 10.1038/44766
Paradis, E., Blomberg, S., Bolker, B., Brown, J., Claude, J., Cuong, J. O., et al. (2018). Ape: Analysis of Phylogenetics and Evolution. R package, v. 2.4-0 (2017). Available online at: http://cran.r-project.org/package=ape
Parejo, S. H., Heleno, R., Rumeu, B., Guzmán, G., Vargas, P., Olesen, J. M., et al. (2018). Small size does not restrain frugivory and seed dispersal across the evolutionary radiation of Galápagos lava lizards. Current Zool. zoy066. doi: 10.1093/cz/zoy066
Patterson, K. (1928). Amphibians and Land Reptiles of Porto Rico, With a List of Those Reported From the Virgin Islands. New York, NY: New York Academy of Science.
Pearson, O. (1954). Habits of the lizard Liolaemus multiformis multiformis at high altitudes in Southern Peru. Copeia 1954, 111–116. doi: 10.2307/1440329
Pérez-Mellado, V., and Corti, C. (1993). Dietary adaptations and herbivory in lacertid lizards on the genus Podarcis from western Mediterranean islands (Reptilia: Sauria). Bonn. Zool. Beitr. 44, 193–220.
Pérez-Mellado, V., and Riera, N. (2004). “Unique interactions of insular lizards and plants. The case of the dead Horse Arum (Dracunculus muscivorus) and the Balearic lizard (Podarcis lilfordi),” in Fifth International Symposium on the Lacertids of the Mediterranean Basin, eds C. Corti and P. Lo Cascio (Liparia: Firenze University Press), 33.
Pérez-Mellado, V., Riera, N., Perera, A., and Martín-García, S. (2005). The lizard, Podarcis lilfordi (Squamata: Lacertidae) as a seed disperser of the Mediterranean plant, Phillyrea media (Oleaceae). Amphibia-Reptilia 26, 105–108. doi: 10.1163/1568538053693260
Pérez-Mellado, V., and Traveset, A. (1999). Relationships between plants and Mediterranean lizards. Natura Croatica 8, 275–285.
Pérez-Méndez, N., Jordano, P., and Valido, A. (2018). Persisting in defaunated landscapes: Reduced plant population connectivity after seed dispersal collapse. J. Ecol. 106, 936–947. doi: 10.1111/1365-2745.12848
Pianka, E. R., and Vitt, L. J. (2003). Lizards. Windows to the Evolution of Diversity. Berkeley, LA: University of California Press.
Platt, S. G., Elsey, R. M., Liu, H., Rainwater, T. R., Nifong, J. C., Rosenblatt, A. E., et al. (2013). Frugivory and seed dispersal by crocodilians: an overlooked form of saurochory? J. Zool. 291, 87–99. doi: 10.1111/jzo.12052
Polis, G. A., Rose, M. D., Sánzhez-Piñero, F., Stapp, P. T., and Anderson, W. B. (2002). “Island food webs,” in A New Island Biogeography of the Sea of Cortés, eds T. J. Case, M. L. Cody and E. Ezcurra (Oxford: Oxford University Press), 362–380.
Pyron, R. A., Burbrink, F. T., and Wiens, J. J. (2013). A phylogeny and revised classification of Squamata, including 4161 species of lizards, amphibaenians and snakes. BMC Evol. Biol. 13, 93. doi: 10.1186/1471-2148-13-93
R Core Team (2014). R: A Language and Environment for Statistical Computing. Vienna: R Foundation for Statistical Computing. Available online at: http://www.R-project.org/
Rodda, G. H., and Dean-Bradley, K. (2002). Excess density compensation of island herpetofaunal assemblages. J. Biogeogra. 29, 623–632. doi: 10.1046/j.1365-2699.2002.00711.x
Rodda, G. H., Perry, G., Rondeau, R. J., and Lazell, J. (2001). The densest terrestrial vertebrate. J. Trop. Ecol. 17, 331–338.
Rodríguez-Pérez, J., Riera, N., and Traveset, A. (2005). Effect of seed passage through birds and lizards on emergence rate of Mediterranean species: Differences between natural and controlled conditions. Funct. Ecol. 19, 699–706. doi: 10.1111/j.0269-8463.2005.00971.x
Rousset, F. (2004). Genetic Structure and Selection in Subdivided Populations. Princeton, NJ: Princeton Univ Press.
Sagonas, K., Pafilis, P., and Valakos, E. D. (2015). Effects of insularity on digestion: living on islands induces shifts in physiological and morphological traits in islands reptiles. Sci. Nat. 102, 55–61. doi: 10.1007/s00114-015-1301-8
Samuels, I. A., and Levey, D. J. (2005). Effects of gut passage on seed germination: do experiments answer the question they ask? Funct. Ecol. 19, 365–368. doi: 10.1111/j.1365-2435.2005.00973.x
Schall, J. J. (1996). Cnemidophorus murinus ruthveni (Bonaire Island Whiptail lizard). Seeds in diet. Herpetol. Rev. 27, 142–143.
Schleich, H. H., Kästle, W., and Kabisch, K. (1996). Amphibians and Reptiles of North Africa (Koenigstein: Koeltz).
Schluter, D. (1984). Body size, prey size and herbivory in the Galápagos lava lizard, Tropidurus. Oikos 43, 291–300.
Schoener, T. W. (1989). Food webs from the small to the large. Ecology 70, 1559–1589. doi: 10.2307/1938088
Schoener, T. W., and Schoener, A. (1978). Inverse relation of survival of lizards with island size and avifaunal richness. Nature 274, 685–687. doi: 10.1038/274685a0
Schoener, T. W., Slade, J. B., and Stinson, C. H. (1982). Diet and sexual dimorphism in the very catholic lizard genus Leiocephalus of the Bahamas. Oecologia 53, 160–169. doi: 10.1007/BF00545659
Schupp, E. W., Jordano, P., and Gómez, J. M. (2010). Seed dispersal effectiveness revisited: a conceptual review. New Phytol. 188, 333–353. doi: 10.1111/j.1469-8137.2010.03402.x
Sokol, O. M. (1967). Herbivory in lizards. Evolution 21, 192–194. doi: 10.1111/j.1558-5646.1967.tb00143.x
Szarski, H. (1962). Some remarks on herbivorous lizards. Evolution 16, 529. doi: 10.1111/j.1558-5646.1962.tb03244.x
Traveset, A. (1998). Effect of seed passage through vertebrate frugivore's guts on germination: a review. PPEES 1, 151–190.
Traveset, A., Heleno, R., and Nogales, M. (2013). “The ecology of seed dispersal,” in Seeds the Ecology of Regeneration in Plant Communities, ed R. S. Gallagher (Oxfordshire, UK: CAB International), 62–93.
Traveset, A., Olesen, J. M., Nogales, M., Vargas, P., Jaramillo, P., Antolín, E., et al. (2015). Bird-flower visitation networks in the Galápagos unveil a widespread interaction release. Nat. Comm. 6, 6376. doi: 10.1038/ncomms7376
Uetz, P., Freed, P., and Hosek, J. (Eds.),. (2018). The EMBL Reptile Database. Available online at: http://www.reptile-database.org/ (accessed August 29, 2018).
Valdecantos, M. S., Arias, F., and Espinoza, R. E. (2012). Herbivory in Liolaemus poecilochromus, a small, cold-climate lizard from the Andes of Argentina. Copeia 2012, 203–210. doi: 10.1643/CE-12-001
Valido, A., and Nogales, M. (1994). Frugivory and seed dispersal by the lizard Gallotia galloti (Lacertidae) in a xeric habitat of the Canary Islands. Oikos 70, 403–411. doi: 10.2307/3545778
Valido, A., and Nogales, M. (2003). Digestive ecology of two omnivorous Canarian lizards species (Gallotia, Lacertidae). Amphibia-Reptilia 24, 331–344. doi: 10.1163/156853803322440790
Valido, A., Nogales, M., and Medina, F. M. (2003). Fleshy fruits in the diet of Canarian lizards Gallotia galloti (Lacertidae) in a xeric habitat of the island of Tenerife. J. Herpetol. 37, 741–747. doi: 10.1670/15-02AN
Valido, A., and Olesen, J. M. (2007). “Importance of lizards as frugivores and seed dispersers,” in Seed dispersal: Theory and its Application in a Changing World, eds A. Dennis, R. Green, E. Schupp and D. Westcott (CAB International, Wallingford), 124–147.
Valido, A., and Olesen, J. M. (2010). “Pollination on islands: examples from the Macaronesian archipelagos,” in Terrestrial Arthropods of Macaronesia: Biodiversity, Ecology and Evolution, eds A. R. M. Serrano, P. A. V. Borges, M. Boieiro, and P. Oromí (Sociedade Portuguesa de Entomologia), 249–283.
Valido, A., Schaefer, H. M., and Jordano, P. (2011). Colour, design and reward: phenotypic integration of fleshy fruit displays. J. Evol. Biol. 24, 751–760. doi: 10.1111/j.1420-9101.2010.02206.x
van Damme, R. (1999). Evolution of herbivory in lacertid lizards: effects of insularity and body size. J. Herpetol. 33, 663–647. doi: 10.2307/1565584
van der Pijl, L. (1982). Principles of Dispersal of Higher Plants. Berlin: Springer. doi: 10.1007/978-3-642-87925-8
van Devender, R. W. (1982). “Growth and ecology of Spiny-tailed and Green Iguanas in Costa Rica, with comments on the evolution of herbivory and large body size,” in Iguanas of the World, eds G. M. Burghardt and A. S. Rand (Park Ridge, NJ: Noyes), 162–183.
Vasconcellos-Neto, J., Barbosa, L., and Silva, W. R. (2009). Seed dispersal of Solanum thomasiifolium Sendtner (Solanaceae) in the Linhares Forest, Espírito Santo state, Brazil. Acta Bot. Brasiliensis 23, 1171–1179. doi: 10.1590/S0102-33062009000400027
Vervust, B., Pafilis, P., Valakos, E. D., and van Damme, R. (2010). Anatomical and physiological changes associated with a recent dietary shift in the lizard Podarcis sicula. Physiol. Biochem Zool. 83, 632–642. doi: 10.1086/651704
Vidal, M. A., and Sabat, P. (2010). Stable isotopes document mainland-island divergence in resource use without concomitant physiological changes in the lizard Liolaemus pictus. Comp. Biochem. Physiol., Part B 156, 61–67. doi: 10.1016/j.cbpb.2010.02.005
Vinson, J., and Vinson, J. M. (1969). The saurian fauna of the Mascarene Islands. I: a revision of the fauna. Mauritius Institute Bulletin 6, 203–320.
Vitt, L. J. (1993). Ecology of isolated open-formation Tropidurus (Reptilia: Tropiduridae) in Amazonian lowland rain forest. Can. J. Zool. 71, 2370–2390. doi: 10.1139/z93-333
Vitt, L. J. (2004). Shifting paradigms: Herbivory and body size in lizards. Proc. Natl. Acad. Sci. U.S.A. 101, 16713–16714. doi: 10.1073/pnas.0407439101
Vitt, L. J., van Loben, R. C., and Ohmart, R. D. (1981). Ecological relationships among arboreal desert lizards. Ecology 62, 398–410. doi: 10.2307/1936714
Wandrag, E. M., Dunham, A. E., Duncan, R. P., and Rogers, H. S. (2018). Seed dispersal increases local species richness and reduces spatial turnover of tropical tree seedlings. Proc. Natl. Acad. Sci., U. S. A. 114, 10689–10694. doi: 10.1073/pnas.1709584114
Wenny, D. G., Sekercioglu, C., Cordeiro, N. J., Rogers, H. S., and Kelly, D. (2016). “Seed dispersal by fruit-eating birds,” in Why Birds Matter: Avian Ecological Function and Ecosystem Services, eds C. H. Sekercioglu, D. G. Wenny and C. J. Whelan (University of Chicago Press), 107–145.
Whitaker, A. H. (1987). The roles of lizards in New Zealand plant reproductive strategies. NZ J. Bot. 25, 315–328. doi: 10.1080/0028825X.1987.10410078
Whiting, M. J., and Greeff, J. M. (1997). Facultative frugivory in the Cape Flat Lizard, Platysaurus capensis (Sauria: Cordylidae). Copeia 1997, 811–818. doi: 10.2307/1447298
Whittaker, R. J., and Fernández-Palacios, J. M. (2007). Island Biogeography: Ecology, Evolution, and Conservation, 2nd Edn. Oxford: Oxford University Press.
Willson, M. F., Sabag, C., Figueroa, J., Armesto, J. J., and Caviedes, M. (1996). Seed dispersal by lizards in Chilean rainforest. Rev. Chilena Hist. Nat. 69, 339–342.
Wotton, D. M. (2002). Effectiveness of the Common Gecko (Hoplodactylus maculatus) as a seed disperser on Mana Island, New Zealand. NZ J. Bot. 40, 639–647. doi: 10.1080/0028825X.2002.9512820
Wotton, D. M., Drake, D. R., Powlesland, R. G., and Ladley, J. J. (2016). The role of lizards as seed dispersers in New Zealand. J. Royal. Soc. NZ 46, 40–65. doi: 10.1080/03036758.2015.1108924
Wright, S. J. (1979). Competition between insectivorous lizards and birds in Central Panama. Am. Zool. 19, 1145–1156. doi: 10.1093/icb/19.4.1145
Keywords: frugivory, lizard, mutualism, plant-animal interaction, saurochory, seed dispersal, island, body size
Citation: Valido A and Olesen JM (2019) Frugivory and Seed Dispersal by Lizards: A Global Review. Front. Ecol. Evol. 7:49. doi: 10.3389/fevo.2019.00049
Received: 22 November 2018; Accepted: 08 February 2019;
Published: 05 March 2019.
Edited by:
Andy J. Green, Estación Biológica de Doñana (EBD), SpainReviewed by:
Sean Thomas Giery, University of Arkansas, United StatesDaniel Wenny, San Francisco Bay Bird Observatory, United States
Copyright © 2019 Valido and Olesen. This is an open-access article distributed under the terms of the Creative Commons Attribution License (CC BY). The use, distribution or reproduction in other forums is permitted, provided the original author(s) and the copyright owner(s) are credited and that the original publication in this journal is cited, in accordance with accepted academic practice. No use, distribution or reproduction is permitted which does not comply with these terms.
*Correspondence: Alfredo Valido, YXZhbGlkb0BpcG5hLmNzaWMuZXM=