- 1Centre for the Mathematical Modelling of Infectious Diseases, London School of Hygiene and Tropical Medicine, London, United Kingdom
- 2School of Population and Public Health, University of British Columbia, Vancouver, BC, Canada
- 3Animal Health Centre, British Columbia Ministry of Agriculture, Abbotsford, BC, Canada
- 4Canadian Wildlife Health Cooperative, Animal Health Centre, Abbotsford, BC, Canada
- 5Department of Interdisciplinary Studies, University of British Columbia, Vancouver, BC, Canada
- 6Biodiversity Research Centre, University of British Columbia, Vancouver, BC, Canada
- 7Department of Epidemiology of Microbial Diseases, Yale School of Public Health, Yale University, New Haven, CT, United States
- 8Centro de Pesquisas Gonçalo Moniz, Fundação Oswaldo Cruz, Ministério da Sáúde, Salvador, Brazil
- 9Instituto de Saúde Coletiva, Universidade Federal da Bahia, Salvador, Brazil
Leptospirosis is a zoonotic disease for which rats are the primary reservoir in urban environments. It is transmitted from rats to people via urine, and is responsible for significant human morbidity and mortality in under-resourced settings. To mitigate the risks posed to people, it is important to understand the ecology of the causative agent Leptospira interrogans. The overarching objective of this study was to compare L. interrogans carriage in urban Norway rats in two ecologically distinct urban environments. We trapped Norway rats (Rattus norvegicus) in Vancouver, Canada (N = 525) and Salvador, Brazil (N = 433) to evaluate whether rat characteristics (i.e., sex, weight, sexual maturity, pregnancy, and the presence of wounds) and location of capture were associated with L. interrogans status. Using generalized linear mixed models to control for clustering by trapping location, we found a greater prevalence of L. interrogans in Salvador (79%) than in Vancouver (12%), and greater spatial heterogeneity in pathogen prevalence in Vancouver than in Salvador. In both locations, we found that older rats and rats with more bite wounds had greater odds of L. interrogans carriage, although wounding influenced pathogen status more for younger animals. Additionally, we found that juvenile rats in Salvador were more likely to leave the nest infected with L. interrogans than were rats in Vancouver, suggesting that potential differences in early-life transmission dynamics exist between the two locations. Together, these results elucidate both general L. interrogans ecology, as well as the importance of geographical location in determining transmission among rats.
Introduction
Leptospirosis is a zoonotic disease with a global distribution that is responsible for significant human morbidity and mortality (Himsworth et al., 2013b; Costa et al., 2015a). Residents of resource-poor countries are particularly affected (Costa et al., 2015a). It is caused by the spirochete bacterium Leptospira interrogans, and symptoms can range from undifferentiated febrile illness to fatal liver failure or pulmonary hemorrhage. Rats, particularly Norway rats (Rattus norvegicus), are the primary reservoir for L. interrogans in urban centers (Costa et al., 2014b). The bacterium is carried asymptomatically in the rat kidneys and shed in the urine (Costa et al., 2015b). It is transmitted from rats to humans primarily via direct or indirect contact with rat urine in the urban environment (Evangelista and Coburn, 2010). The incidence and distribution of urban leptospirosis are increasing in association with global urbanization (Hartskeerl et al., 2011), making it an important and growing public health concern.
An understanding of the ecology of L. interrogans in urban rats is critical for monitoring and mitigating human health risks. Past work has shown that carriage of L. interrogans by rats is associated with a variety of factors, including age, sex, sexual maturity, weight, internal fat volume, the presence and severity of bite wounds, and location of capture (Thiermann, 1977; Easterbrook et al., 2007; Krøjgaard et al., 2009; Himsworth et al., 2013a; Costa et al., 2014a, 2015b; Minter et al., 2017; Lee et al., 2018). However, results have varied across studies. For example, Minter et al. (2017) documented evidence of juvenile rats leaving the nest with infection, which could reflect vertical transmission of L. interrogans from mothers to pups (De Oliveira et al., 2016), while others have suggested that transmission occurs almost exclusively among adults (Himsworth et al., 2013a). Additionally, the overall prevalence of L. interrogans carriage has varied markedly among studies (Aviat et al., 2009; Koizumi et al., 2009; Scialfa et al., 2010; Maas et al., 2018). Unfortunately, it is difficult to determine if past findings reflect true variations in the ecology of L. interrogans in different cities, or if it is simply a result of differing study methodologies or areas of focus. For the same reason, the driving forces behind results shared among studies are hard to elucidate. For example, regardless of the city, variation in L. interrogans prevalence by trapping site has been consistently observed (Krøjgaard et al., 2009; Himsworth et al., 2013a; Costa et al., 2015b; Lee et al., 2018); however, differences in both sampling approach and statistical analyses among studies have made it difficult to determine the relative impact of geographical location on the constellation of factors affecting L. interrogans ecology.
Indeed, there has yet to be a study directly comparing L. interrogans carriage between two significantly different urban environments. These sorts of comparisons are essential for determining which elements of L. interrogans ecology are unique to a location, and which can be extrapolated among urban environments, as well as for clarifying the overall ecology of the pathogen. The aim of this study was to compare and contrast the characteristics of L. interrogans carriage in urban Norway rats in Salvador, Brazil, and Vancouver, Canada. This was accomplished through collation and re-analysis of data collected by two methodologically aligned studies (Himsworth et al., 2013a; Minter et al., 2017).
Methods
Ethics Statement
This study was approved by the University of British Columbia's Animal Care Committee (A11-0087) and adhered to national guidelines set out by the Canadian Council on Animal Care. Protocols of this study were also approved by the Institutional Animal Care and Use Committee (IACUC) from Brazil and United States. At Oswaldo Cruz Foundation, Salvador, Brazil, the Comissão de Ética no Uso de Animais (CEUA) do CPqGM-FIOCRUZ-BA approved the protocol number 003/2012.
Study Sites
In Vancouver, the study area was comprised of 43 contiguous city blocks encompassing the core of Vancouver's Downtown Eastside (DTES) (N49°17′ / W123°6′). Also included was an area within the port terminal, which is a center for international shipping that forms the northern border of the DTES (see Figure 1). The DTES is an inner-city area of low socioeconomic status known as “Canada's poorest postal code”. While the built environment is highly developed, with densely populated city blocks (e.g., including residential, commercial, and industrial parcels) surrounded by roadways, buildings are antiquated and often in disrepair. Additionally, there is considerable refuse accumulated in the alleyways, which are also heavily used by residents for injecting drugs, sleeping, loitering, etc (Smith, 2000). Vancouver has a moderate oceanic climate with an average annual temperature of 11°C and average annual precipitation of 1,588 mm.
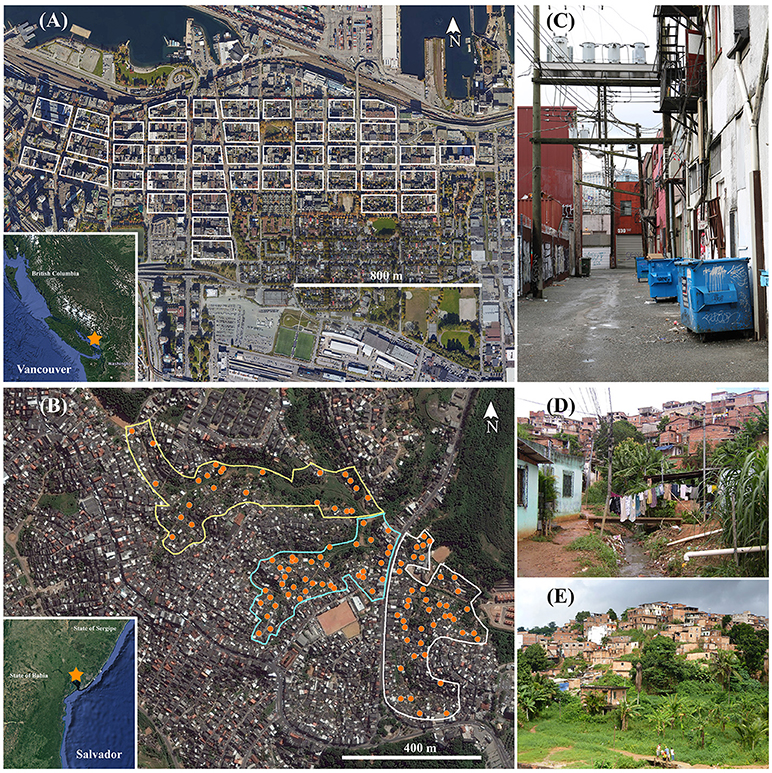
Figure 1. Trapping locations in the 43 city blocks of Vancouver, Canada (A) and three valleys of Salvador, Brazil (B). Images provided through Google Earth Professional (https://www.google.com/earth/download/gep/agree.html). Examples of areas where rats were caught in each location are indicated in (C) (Vancouver), (D,E) (Salvador).
In Salvador, the study area included three valleys within Pau da Lima (S13°32′ / W38°43′), an urban slum. This urban area is characterized by a lack of basic sanitation, open and free flowing sewers as well as poor housing conditions (Felzemburgh et al., 2014) (Figure 1). Salvador has a subtropical climate with an average annual temperature of 25°C and average annual precipitation of 1,781 mm.
Trapping
In Vancouver, each block (and the port site) was randomly assigned to a 3-week study period over the course of 1 year (September 2011–August 2012). Within each block, ~20 Tomahawk Rigid Traps for rats (Tomohawk Live Trap, Hazlelhurst, USA), which trap rats alive, were set out along each side of the back alley that bisected the block. At the port, traps were placed in areas where port staff had observed rats. Traps were pre-baited (filled with bait but fixed open) for 1 week, followed by 2 weeks of active trapping. Baits used included a combination of peanut butter, bacon fat, and oats. Traps were set in the evening and trapped rats were collected the following morning.
In Salvador, 150 sampling points were randomly selected and a 30 m2 buffer zone was placed around each sampling point. Three trapping sites were selected within each sampling point based on signs of rat presence, and two Tomahawk traps were placed at each sampling point. Trapping occurred during four periods of time (May–August 2013, October–December 2013, March–August 2014, September–December 2014). Traps were pre-baited for 2 to 3 days, followed by 4 to 6 consecutive days of active trapping. Traps were baited with sausage. Traps were set at sundown and trapped rats were collected at sunrise the following day.
Data Collection
In Vancouver, trapped rats were anesthetized by isoflurane inhalation prior to pentobarbital euthanasia via intracardiac injection. Rats underwent a complete necropsy during which kidney samples were collected and stored at −80°C. In Salvador, rats were anesthetized by isoflurane inhalation prior to thiopental euthanasia via intraperitoneal injection. Urine was obtained directly from the bladder using a 1 mL syringe and stored at −80°C.
For both cities, the following demographic and morphometric data were collected: species (based on external morphology), sex, weight, sexual maturity (scrotal testes for males and perforate vagina for females), pregnancy, and the presence of bite wounds. The date and location of each rat trapped was also recorded.
The Maxwell 16 System DNA Purification Kit (Promega Corp., USA) and the QiaAMP DNA Mini Kit (Qiagen Inc., Canada), was used to extract DNA from the urine and kidney samples, respectively. DNA extracts were then analyzed using a real-time PCR assay which targets a 242 bp fragment of the lipL32 gene of pathogenic Leptospira species (Stoddard et al., 2009). It should be noted that a near perfect correlation between leptospiral loads in the urine and kidneys of wild, urban Norway rats has been identified, suggesting that urine and kidney samples can likely be used interchangeably for the study of L. interrogans in this species (Costa et al., 2015b).
Statistical Analysis
Previous studies have indicated that age has a strong, positive association with L. interrogans carriage in urban rats (Krøjgaard et al., 2009; Himsworth et al., 2013a; Costa et al., 2015b; Minter et al., 2017). However, the majority of these studies used weight as a proxy for age, which is problematic since weight and age are not linearly associated with each other (Calhoun, 1963). In order to overcome this issue, the von Bertalanffy equation (weight = a[1 – exp{–r(age – c)}], where “a” is the asymptote, “r” is the constant growth rate and “c” is the age at which maximum growth occurs) has been used to more accurately model rodent age curves, including those of urban rats (Burthe et al., 2010; Minter et al., 2017). For this study rats were aged using the von Bertalanffy equation using parameters from Calhoun (1963) as in Minter et al. (2017). In pregnant females, weight was adjusted by the average weight difference between pregnant and non-pregnant, sexually mature females prior to applying the age calculation (Minter et al., 2017).
Explanatory variables included age (in days), sexual maturity (yes/no), sex (male/female), and bite wounds (absent/present) as these variables were previously identified as risk factors for L. interrogans carriage in Vancouver and/or Salvador (Himsworth et al., 2013a; Minter et al., 2017). Chi-squared test or Welch's t-test were used to compare the distribution of the variables between Vancouver and Salvador. Linear regression was used to identify the relationship between age and wounding. Neither weight nor body length (another proxy for age in rats) were included, as these variables were strongly collinear with age, which was the main variable under consideration.
Multivariate generalized linear mixed models (GLMMs) were used to compare the relationships among L. interrogans carriage (positive/negative on PCR) and the aforementioned explanatory variables in Vancouver and Salvador controlling for clustering by trapping location (city block in Vancouver and sampling point in Salvador). These included full models, which contained all explanatory variables (as well as an interaction term between age and wounding), and “final” models obtained through backward selection. For final model selection, non-significant explanatory variables were removed from the full model until all variables were statistically significant at a 5% level. All statistics were conducted in R (R Core Team, 2018) using the lme4 package for GLMMs (Bates et al., 2015).
The full GLMM was used to investigate the risk of rats leaving the nest with infection. Specifically, the probability of infection was calculated for the youngest animals in each cohort (27 days for Salvador and 26 days for Vancouver) that had no wounds and were sexually immature.
Results
The final sample included 525 and 433 Norway rats in Vancouver and Salvador, respectively. In both cities there was approximately an equal sex ratio (see Table 1). However, the Salvador rats were significantly older (based on the von Bertalanffy estimate for age) and more likely to be sexually mature and wounded compared to the Vancouver rats. In both Vancouver and Salvador, there was a significant positive relationship between wounding and both age and sexual maturity (p < 0.001).
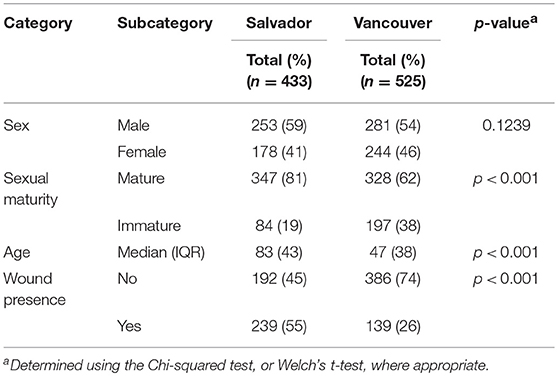
Table 1. Baseline characteristics and associations with Leptospira interrogans PCR status among Norway rats.
The prevalence of L. interrogans carriage was higher in Salvador vs. Vancouver (79% vs. 12%). This difference was consistent even after stratifying by age (Figure 2). In both the Vancouver and Salvador full models, the odds of L. interrogans carriage increased with age and the presence of bite wounds (see Table 2) and there was a significant negative interaction between age and bite wounds (indicating that the relative effect of bite wounds was less for older vs. younger rats). The “final” model for both Vancouver and Salvador also included the aforementioned variables. Sex was not significantly related to L. interrogans carriage in either city. However, sexual maturity was significant in both the full and final models for Salvador but not for Vancouver. Conversely, the random effect of trapping location on the odd of L. interrogans carriage was much greater for Vancouver compared to Salvador (Table 3).
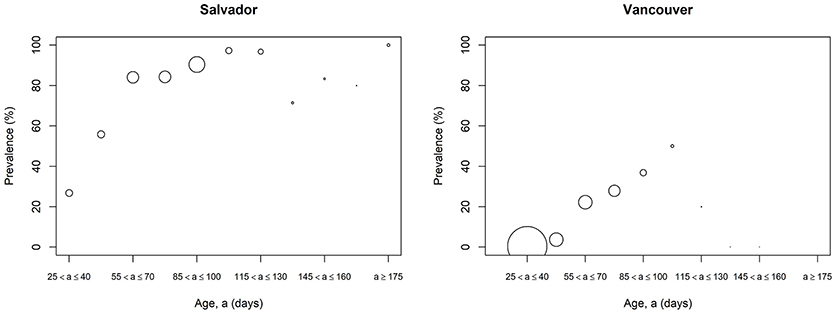
Figure 2. Age-prevalence curve for Leptospira infection in Salvador and Vancouver Norway rats (Rattus norvegicus). The size of the point indicates the number of rats in that age category (larger point = larger sample size).
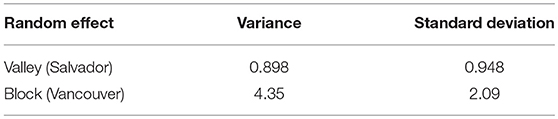
Table 3. Variance and standard deviation of the random effects (of the intercept) for the Vancouver and Salvador full models.
Rats are more likely to be infected with L. interrogans at a younger age in Salvador compared to Vancouver (Figure 2). The probability of a rat leaving the nest with infection was 0.002 (95% CI: 0–0.01) in Vancouver and 0.21 (95% CI: 0.10–0.32) in Salvador.
Discussion
These results reveal a number of similarities and important differences with regard to the ecology of L. interrogans and rats in Vancouver and Salvador. First, it is interesting to note that the rat population in Salvador appeared to be significantly older than that in Vancouver and had a greater proportion of sexually mature rats. The Salvador rats were also more likely to be wounded; however, this may simply be a reflection of population demographics, as older rats and sexually mature rats were more likely to be wounded in both cities. Overall, these results suggest a more rapid population turnover is occurring in Vancouver. Although the reason behind this is unclear, there are several potential explanations. First, rats in Vancouver and Salvador are distinct from each other phylogenetically, and likely arose through independent founder events (Puckett et al., 2016); therefore, there may be heritable differences in lifespan between the two populations. Second, a previous study in Vancouver demonstrated a high prevalence of infectious and degenerative cardiovascular and respiratory disease, particularly in older rats (Rothenburger et al., 2015a,b). While the health status of the Salvador rats is not known, a decreased disease burden in that population compared to Vancouver could account for a longer lifespan. Rats in Salvador and Vancouver exist in two highly disparate environments, and it may be the case that decreased infrastructure and sanitation in Salvador provides greater access to resources (Santos et al., 2017) and supports a longer lifespan in resident rats. Finally, although there are no coordinated rat-control programs in either city, many property and business owners in the DTES conduct lethal rat control (i.e., trapping and poisoning) on their own or through a pest control company, which may contribute to increased population turn over.
Given that L. interrogans carriage is strongly associated with age, the higher overall prevalence of the pathogen in Salvador rats may be partially attributable to the different age structures of the two populations. However, after stratifying by age, rats in Vancouver consistently had a lower prevalence of infection compared to those in Salvador. This phenomenon may be caused by the widespread environmental contamination of soil and water with pathogenic L. interrogans within the urban slums of Salvador (Casanovas-Massana et al., 2018)–contamination that may be facilitated by the higher average annual temperature and precipitation compared to Vancouver. Indeed, transmission of L. interrogans from rats to humans is more common in warmer and wetter climates (Himsworth et al., 2013b). In fact, some studies have suggested that prevalence of L. interrogans in rats decreases with increasing latitude simply as a result of climactic differences (Jensen and Magnussen, 2016). Additionally, differences in the built environment between the Salvador and Vancouver study areas make the former more prone to flooding and accumulation of standing water, both of which facilitate environmental transmission of L. interrogans (Himsworth et al., 2013b; Hagan et al., 2016). Indeed, it is these differences that likely account for the fact that human cases of leptospirosis are common in Salvador, but have yet to be identified in Vancouver (Costa et al., 2017; McVea et al., 2018).
In both Salvador and Vancouver, the odds of L. interrogans infection increased with age. This is a consistent finding among studies of L. interrogans in rats (Vanasco et al., 2003; Johnson et al., 2004; Krøjgaard et al., 2009) and likely reflects an increased cumulative probability of exposure to and infection with this pathogen over time. However, it is interesting to note that rats were significantly more likely to leave the nest carrying Leptospira in Salvador compared to Vancouver. Previous studies have identified the presence of L. interrogans in breastmilk in Salvador rats (De Oliveira et al., 2016), suggesting the possibility of vertical infection. A similar study has not been conducted in Vancouver; however, it seems unlikely that the core biology of the same species in two separate locations would differ sufficiently to account for significantly different rates of vertical transmission. Additionally, the low number of leptospires in breast milk and tissues may not be sufficient for effective transmission of the bacteria (De Oliveira et al., 2016). It therefore seems more likely that this finding reflects a greater degree of environmental contamination and transmission within the nest in Salvador compared to Vancouver. This, in turn may be due to the different environments available for burrowing and nest building in the two cities.
Although age was associated with wounding in both cities, wounding was an important risk factor for infection independent of age. There was also a significant interaction between age and wounding, suggesting that impact of wounding on L. interrogans transmission is greater in younger animals. Yet, as there is no significant shedding of L. interrogans in saliva (Donovan et al., 2018), wounding does not represent a direct route of transmission. Rather, bite wounds may reflect specific social interactions that promote transmission, particularly behaviors associated with dominance and social hierarchies (e.g., fighting). This suggests that, while “random” exposure in the environment may be a source of L. interrogans for rats, specific behaviors are also responsible for L. interrogans infection. This is important because anthropogenic interventions that alter rat population and social structures, and associated intraspecific interactions, may have unforetold impacts on L. interrogans ecology in rats. Indeed, a recent study in Vancouver rats showed that lethal pest control can increase the prevalence of L. interrogans in rats (Lee et al., 2018). The results of this study suggest that behavioral drivers of L. interrogans infection may be common among cities, therefore those undertaking pest control with the goal of reducing public health threats should do so with the understanding of the potential impacts of different control approaches on rats and the pathogens they carry.
Although social factors may be associated with L. interrogans infection in both cities, the specific behaviors and/or interactions that facilitate infection may vary. This possibility is highlighted by the fact that sexual maturity was an important independent risk factor for infection in Salvador but not Vancouver. Vancouver and Salvador also differed significantly with regard to the impact of geography on infection. Specifically, the random variation in the odds of infection by trapping site was much greater in Vancouver than in Salvador. This could be attributed to differences in study design, as trapping was conducted on a finer scale in Vancouver compared to Salvador. The difference in sampling methodology between the two cities, however, was a direct result of differences in the built environment. Specifically, Vancouver's block system allowed a systematic, grid-like approach, which could not be replicated in Salvador. Differences in the built environment may also create differences in rat and pathogen ecology between the two cities. For example, in Vancouver, paved streets heavily used by vehicular traffic separated adjacent city blocks, while in Salvador, trapping points were separated by open spaces and fewer, smaller roadways (i.e., avenues). Genetic evidence suggests that roads may present anthropogenic barriers to rat movement (Richardson et al., 2017; Combs et al., 2018) isolating colonies and creating a markedly heterogeneous pattern of disease distribution even over short geographic distances (Himsworth et al., 2013a, 2015a,b). In the absence of significant roadways, it may be the case that rat colonies co-exist in closer proximity to one another and/or have a greater degree of direct or indirect contact. This, in turn, may facilitate L. interrogans transmission among colonies. Indeed, it is of note that the prevalence of L. interrogans infection in Vancouver ranged from 0 to 66% among blocks (Himsworth et al., 2013a). It may therefore be the case that colony segregation in Vancouver is partially responsible for both the low overall prevalence of the pathogen and the marked random variation in block-level prevalence.
Limitations
One limitation of this study is that, lacking a way to accurately determine the age of rats, we used a weight-derived estimate as a proxy of age. This could lead to miscalculations of age because weight-based age estimates do not account for rat population characteristics that could cause disparities in rat weight, such as genetics, health status, and nutritional condition. For example, rats in poorer health and with lower nutritional status might weigh less, and would therefore be calculated as younger than a healthy rat of the same age in a nutrient-rich environment. However, as the age estimates of rats leaving the nest for both locations were similar, this suggests that age estimates, at least for younger animals, are aligned. Similarly, while rats in Salvador were significantly heavier than rats in Vancouver, they were also more likely to be sexually mature, suggesting that weight is unlikely to have markedly affected differences in age estimates between the two sites.
A second limitation was the variation in the duration of pre-baiting and active trapping between Salvador and Vancouver which was a result of the collaboration between the two study groups being established after the fieldwork had already been completed. It is not known how variations in pre-baiting time might impact the factors included in this study. However, it has been shown that larger, sexually mature rats are more likely to be captured earlier in the trapping period, compared to smaller and immature rats (Himsworth et al., 2014; Byers et al. in unpublished). Although this would not impact analyses at the level of the individual rat, given that mass is positively associated with L. interrogans infection, studies with shorter trapping periods, such as that in Salvador, could produce an inflated estimate of L. interrogans prevalence. However, Byers et al. (in unpublished) found no significant association between L. interrogans infection status and trap day, suggesting that the impact of trapping period on the results of this study are unlikely to be significant.
Finally, it is important to note that the trapping methodology used in this study would not allow us to estimate rat abundance or population density in either city. Indeed, there have yet to be any published studies investigating the impact of these factors on L. interrogans dynamics in urban rats. Given that many diseases are density-dependent (Begon et al., 2002), and density-dependent transmission may influence the efficacy of control measures, such as population reduction, we suggest that this should be a priority for future study.
Conclusions
Overall, this study highlights the similarities and differences in ecology of L. interrogans in rat populations in two ecologically distinct urban environments. We reveal that both increasing age and number of bite wounds heighten the odds that a rat will acquire L. interrogans, and that rats are more likely to leave the nest with infection in Salvador than in Vancouver. Differences between the study areas in pathogen prevalence and spatial heterogeneity of infected rats further suggests that both the local environment and rat social structures/behaviors are important drivers in transmission events. These nuances underscore the importance of geographical location in L. interrogans ecology, and reiterate the necessity of understanding rat-pathogen dynamics both globally and locally to devise effective mitigation techniques. Priorities for future studies include developing and/or validating accurate tools for aging urban rats, as well as repeating these analyses in other urban ecosystems, both similar and different to those included here, in order to determine whether these findings can be supported and/or expanded upon.
Data Availability Statement
The raw data supporting the conclusions of this manuscript will be made available by the authors, without undue reservation, to any qualified researcher.
Author Contributions
This study was conceived of by AM, CH, and FC. Sample collection design was conceived and developed by FC, AK, JC, and CH. Sample collection was performed by FC and CH. Data analysis was performed by AM and CH. Figures were created by AM and KB. Writing of the manuscript was performed by CH, KB, and AM with revisions provided by FC. Funding was acquired by CH and FC.
Funding
This study was supported by the Canadian Institutes of Health Research (MOP-119530 and CGV-104833); Oswaldo Cruz Foundation; Secretariat of Health Surveillance; Brazilian Ministry of Health; Wellcome Trust, Grant/Award Number: 102330/Z/13/Z; NSF-NIH, Grant/Award Number: 5 R01 AI052473, 5 U01 AI088752, 1 R25 TW009338, 1 R01 AI121207, F31 AI114245, R01 AI052473, U01 AI088752, R01 TW009504, and R25 TW009338; Ecology and Evolution of Infection Diseases (EEID), Grant/Award Number: 1 R01 TW009504; and by the Research and Policy for Infectious Disease Dynamics (RAPIDD) program of the Science and Technology Directorate (US Department of Homeland Security).
Conflict of Interest Statement
The authors declare that the research was conducted in the absence of any commercial or financial relationships that could be construed as a potential conflict of interest.
Acknowledgments
We would like to thank Alice Feng, Kirbee Parsons, and the Vancouver Area Network of Drug Users for their assistance in trapping and support of our project. We also thank Elsio Wunder and Lin Zhan for the laboratory analysis of the Salvador samples. We would also like to thank the Salvador field team coordinators for the data collection: Gabriel G. Perda, Soledad Serrano, Daiana De Oliveira and Arsinoe C. Pertile.
Abbreviations
DTES, Downtown Eastside.
References
Aviat, F., Blanchard, B., Michel, V., Blanchet, B., Branger, C., Hars, J., et al. (2009). Leptospira exposure in the human environment in France: a survey in feral rodents and in fresh water. Comp. Immunol. Microb. Infect. Dis. 32, 463–476. doi: 10.1016/j.cimid.2008.05.004
Bates, D., Maechler, M., Bolker, B., and Walker, S. (2015). Fitting linear mixed-effects models using lme4. J. Stat. Softw. 67, 1–48. doi: 10.18637/jss.v067.i01
Begon, M., Bennett, M., Bowers, R. G., French, N. P., and Hazel, S. M. (2002). A clarification of transmission terms in host-microparasite models: numbers, densities and areas. Epidemiol. Infect. 129, 147–153. doi: 10.1017/S0950268802007148
Burthe, S. J., Lambin, X., Telfer, S., Douglas, A., Beldomenico, P., Smith, A., et al. (2010). Individual growth rates in natural field vole, Microtus agrestis, populations exhibiting cyclic population dynamics. Oecologia 162, 653–661. doi: 10.1007/s00442-009-1495-6
Calhoun, J. B. (1963). The Ecology and Sociology of the Norway Rat. Bethesda, MD: US Department of health, Education and Welfare, Bethesda.
Casanovas-Massana, A., Costa, F., Riediger, I. N., Cunha, M., De Oliveira, D., Mota, D. C., et al. (2018). Spatial and temporal dynamics of pathogenic Leptospira in surface waters from the urban slum environment. Water Res. 130, 176–184. doi: 10.1016/j.watres.2017.11.068
Combs, M., Byers, K. A., Ghersi, B. M., Blum, M. J., Caccone, A., Costa, F., et al. (2018). Urban rat races: spatial population genomics of brown rats (Rattus norvegicus) compared across multiple cities. Proc. R. Soc. B. 285, 20180245–20180210. doi: 10.1098/rspb.2018.0245
Costa, F., Carvalho-Pereira, T., Begon, M., Riley, L., and Childs, J. (2017). Zoonotic and vector-borne diseases in urban slums: opportunities for intervention. Trends Parasitol. 33, 660–662. doi: 10.1016/j.pt.2017.05.010
Costa, F., Hagan, J. E., Calcagno, J., Kane, M., Torgerson, P., Martinez-Silveira, M. S., et al. (2015a). Global morbidity and mortality of leptospirosis: a systematic review. PLoS. Negl. Trop. Dis. 9:e0003898. doi: 10.1371/journal.pntd.0003898
Costa, F., Porter, F. H., Rodrigues, G., Farias, H., de Faria, M. T., Wunder, E. A., et al. (2014a). Infections by Leptospira interrogans, Seoul Virus, and Bartonella spp. among Norway rats (Rattus norvegicus) from the urban slum environment in Brazil. Vector Borne Zoo Dis. 14, 33–40. doi: 10.1089/vbz.2013.1378
Costa, F., Ribeiro, G. S., Felzemburgh, R. D., Santos, N., Reis, R. B., Santos, A. C., et al. (2014b). Influence of household rat infestation on Leptospira transmission in the urban slum environment. PLoS. Negl. Trop. Dis. 8:e3338. doi: 10.1371/journal.pntd.0003338
Costa, F., Wunder, E. A., De Oliveira, D., Bisht, V., Rodrigues, G., Reis, M. G., et al. (2015b). Patterns in Leptospira shedding in Norway rats (Rattus norvegicus) from Brazilian slum communities at high risk of disease transmission. PLoS. Negl. Trop. Dis. 9:e0003819. doi: 10.1371/journal.pntd.0003819
De Oliveira, D., Figueira, C. P., Zhan, L., Pertile, A. C., Pedra, G. G., Gusmão, I. M., et al. (2016). Leptospira in breast tissue and milk of urban Norway rats (Rattus norvegicus). Epidemiol Infect. 144, 2420–2429. doi: 10.1017/S0950268816000637
Donovan, C. M., Lee, M. J., Byers, K. A., Bidulka, J., Patrick, D. M., and Himsworth, C. G. (2018). Leptospira spp. in the oral cavity of urban brown rats (Rattus norvegicus) from Vancouver, Canada—Implications for rat-rat and rat-human transmission. J. Wildl. Dis. 54, 635–637. doi: 10.7589/2017-08-194
Easterbrook, J. D., Kaplan, J. B., Vanasco, N. B., Reeves, W. K., Purcell, R. H., Kosoy, M. Y., et al. (2007). A survey of zoonotic pathogens carried by Norway rats in Baltimore, Maryland, USA. Epidemiol. Infect. 135, 1192–1199. doi: 10.1017/S0950268806007746
Evangelista, K. V., and Coburn, J. (2010). Leptospira as an emerging pathogen: a review of its biology, pathogenesis and host immune responses. Future Microbiol. 5, 1413–1425. doi: 10.2217/fmb.10.102
Felzemburgh, R. D., Ribeiro, G. S., Costa, F., Reis, R. B., Hagan, J. E., Melendez, A. X., et al. (2014). Prospective study of leptospirosis transmission in an urban slum community: Role of poor environment in repeated exposures to the Leptospira agent. PLoS. Negl. Trop. Dis. 8:e2927. doi: 10.1371/journal.pntd.0002927
Hagan, J. E., Moraga, P., Costa, F., Capian, N., Ribeiro, G. S., Wunder, E. A., et al. (2016). Spatiotemporal determinants of urban leptospirosis transmission: Four-year prospective cohort study of slum residents in Brazil. PLoS. Negl. Trop. Dis. 10:e0004275. doi: 10.1371/journal.pntd.0004275
Hartskeerl, R. A., Collares-Pereira, M., and Ellis, W. A. (2011). Emergence, control and re-emerging leptospirosis: dynamics of infection in the changing world. Clin. Microbiol. Infect. 17, 494–501. doi: 10.1111/j.1469-0691.2011.03474.x
Himsworth, C. G., Bai, Y., Kosoy, M. Y., Wood, H., DiBernardo, A., Lindsay, R., et al. (2015a). An investigation of Bartonella spp., Rickettsia typhi, and Seoul Hantavirus in rats (Rattus spp.) from an inner-city neighborhood of Vancouver, Canada: Is pathogen presence a reflection of global and local rat population structure? Vector Borne Zoo. Dis. 15, 21–26. doi: 10.1089/vbz.2014.1657
Himsworth, C. G., Bidulka, J., Parsons, K. L., Feng, A. Y., Tang, P., Jardine, C. M., et al. (2013a). Ecology of Leptospira interrogans in Norway rats (Rattus norvegicus) in an inner-city neighborhood of Vancouver, Canada. PLoS. Negl. Trop. Dis. 7:e2270. doi: 10.1371/journal.pntd.0002270.s001
Himsworth, C. G., Jardine, C. M., Parsons, K. L., Feng, A. Y., and Patrick, D. M. (2014). The characteristics of wild rat (Rattus spp.) populations from an inner-city neighborhood with a focus on factors critical to the understanding of rat-associated zoonoses. PLoS ONE 9:e91654. doi: 10.1371/journal.pone.0091654
Himsworth, C. G., Parsons, K. L., Jardine, C., and Patrick, D. M. (2013b). Rats, cities, people, and pathogens: a systematic review and narrative synthesis of literature regarding the ecology of rat-associated zoonoses in urban centers. Vector Borne Zoo. Dis. 13, 349–359. doi: 10.1089/vbz.2012.1195
Himsworth, C. G., Zabek, E., Desruisseau, A., Parmley, E. J., Reid-Smith, R., Jardine, C. M., et al. (2015b). Prevalence and characteristics of Escherichia coli and Salmonella spp. in the feces of wild urban Norway and black rats (Rattus norvegicus and Rattus rattus) from an inner-city neighborhood of Vancouver, Canada. J. Wildl. Dis. 51, 589–600. doi: 10.7589/2014-09-242
Jensen, P. M., and Magnussen, E. (2016). Is it too cold for Leptospira interrrogans transmission on the faroese islands? Infect. Dis. 48, 156–160. doi: 10.3109/23744235.2015.1092579
Johnson, M. A., Smith, H., Joseph, P., Gilman, R. H., Bautista, C. T., Campos, K. J., et al. (2004). Environmental exposure and leptospirosis, Peru. Emerg. Infect. Dis. 10, 1016–1022. doi: 10.3201/eid1006.030660
Koizumi, N., Muto, M., Tanikawa, T., Mizutani, H., Sohmura, Y., Hayashi, E., et al. (2009). Human leptospirosis cases and the prevalence of rats harbouring Leptospira interrogans in urban areas of Tokyo, Japan. J. Med. Microbiol. 58, 1227–1230. doi: 10.1099/jmm.0.011528-0
Krøjgaard, L. H., Villumsen, S., Markussen, M. D. K., Jensen, J. S., Leirs, H., and Heiberg, A. C. (2009). High prevalence of Leptospira spp. in sewer rats (Rattus norvegicus). Epidemiol. Infect. 137, 1586–1592. doi: 10.1017/S0950268809002647
Lee, M. J., Byers, K. A., Donovan, C. M., Bidulka, J. J., Stephen, C., Patrick, D. M., et al. (2018). Effects of culling on Leptospira interrogans carriage by rats. Emerg. Infect. Dis. 24, 356–360. doi: 10.3201/eid2402.171371
Maas, M., De Vries, A., Reusken, C., Buijs, J., Goris, M., Hartskeerl, R., et al. (2018). Prevalence of Leptospira spp. and Seoul hantavirus in brown rats (Rattus norvegicus) in four regions in the Netherlands, 2011-2015. Infect. Ecol. Epidemiol. 8:1490135. doi: 10.1080/20008686.2018.1490135
McVea, D. A., Himsworth, C. G., Patrick, D. M., Lindsay, L. R., Kosoy, M., and Kerr, T. (2018). Exposure to rats and rat-associated Leptospira and Bartonella species among people who use drugs in an impoverished, inner-city neighborhood of Vancouver, Canada. Vector Borne Zoo Dis. 18, 82–88. doi: 10.1089/vbz.2017.2179
Minter, A., Diggle, P. J., Costa, F., Childs, J., Ko, A. I., and Begon, M. (2017). Evidence of multiple intraspecific transmission routes for Leptospira acquisition in Norway rats (Rattus norvegicus). Epidemiol. Infect. 70, 1–11. doi: 10.1017/S0950268817002539
Puckett, E. E., Park, J., Combs, M., Blum, M. J., Bryant, J. E., Caccone, A., et al. (2016). Global population divergence and admixture of the brown rat (Rattus norvegicus). Proc. R. Soc. B. 283:20161762. doi: 10.1098/rspb.2016.1762
R Core Team (2018). R: A Language and Environment for Statistical Computing. Vienna: R Foundation for Statistical Computing. Available online at: https://www.R-project.org/
Richardson, J. L., Burak, M. K., Hernandez, C., Shirvell, J. M., Mariani, C., Carvalho-Pereira, T. S., et al. (2017). Using fine-scale spatial genetics of Norway rats to improve control efforts and reduce Leptospirosis risk in urban slum environments. Evol. Appl. 10, 323–337. doi: 10.1111/eva.12449
Rothenburger, J. L., Himsworth, C. G., Clifford, C. B., Ellis, J., Treuting, P. M., and Leighton, F. A. (2015a). Respiratory pathology and pathogens in wild urban rats (Rattus norvegicus and Rattus rattus). Vet. Pathol. 52, 1210–1219. doi: 10.1177/0300985815593123
Rothenburger, J. L., Himsworth, C. G., Treuting, P. M., and Leighton, F. A. (2015b). Survey of cardiovascular pathology in wild urban Rattus norvegicus and Rattus rattus. Vet Pathol 52, 201–208. doi: 10.1177/0300985814528220
Santos, N. J., Sousa, E., Reis, M. G., Ko, A. I., and Costa, F. (2017). Rat infestation associated with environmental deficiencies in an urban slum community with high risk of leptospirosis transmission. Cad Saúde Pública 33:e00132115. doi: 10.1590/0102-311x00132115
Scialfa, E., Bolpe, J., Bardón, J. C., Ridao, G., Gentile, J., and Gallicchio, O. (2010). Isolation of Leptospira interrogans from suburban rats in Tandill, Buenos Aires, Argentina. Rev. Argent Microbiol. 42, 126–128. doi: 10.1590/S0325-75412010000200012
Smith, H. A. (2000). Where Worlds Collide: Social Polarisation at the Community Level in Vancouver's Gastown/Downtown Eastside. The University of British Columbia, Vancouver, Canada.
Stoddard, R. A., Gee, J. E., Wilkins, P. P., McCaustland, K., and Hoffmaster, A. R. (2009). Detection of pathogenic Leptospira spp. through TaqMan polymerase chain reaction targeting the LipL32 gene. Diagn. Microbiol. Infect. Dis. 64, 247–255. doi: 10.1016/j.diagmicrobio.2009.03.014
Thiermann, A. B. (1977). Incidence of leptospirosis in the Detroit rat population. Am. J. Trop. Med. Hyg. 26, 970–974.
Keywords: age-prevalence, bite wound, intraspecific transmission leptospira, leptospirosis, Norway rat (Rattus norvegicus), urban ecology
Citation: Minter A, Himsworth CG, Byers KA, Childs JE, Ko AI and Costa F (2019) Tails of Two Cities: Age and Wounding Are Associated With Carriage of Leptospira interrogans by Norway Rats (Rattus norvegicus) in Ecologically Distinct Urban Environments. Front. Ecol. Evol. 7:14. doi: 10.3389/fevo.2019.00014
Received: 31 October 2018; Accepted: 15 January 2019;
Published: 30 January 2019.
Edited by:
Serge Morand, Center for the National Scientific Research (CNRS), FranceReviewed by:
Kim Rebecca Blasdell, Commonwealth Scientific and Industrial Research Organisation (CSIRO), AustraliaMiriam Maas, National Institute for Public Health and the Environment, Netherlands
Copyright © 2019 Minter, Himsworth, Byers, Childs, Ko and Costa. This is an open-access article distributed under the terms of the Creative Commons Attribution License (CC BY). The use, distribution or reproduction in other forums is permitted, provided the original author(s) and the copyright owner(s) are credited and that the original publication in this journal is cited, in accordance with accepted academic practice. No use, distribution or reproduction is permitted which does not comply with these terms.
*Correspondence: Chelsea G. Himsworth, Y2hlbHNlYS5oaW1zd29ydGhAZ292LmJjLmNh
†These authors have contributed equally to this work