- 1Department of Interdisciplinary Studies, University of British Columbia, Vancouver, BC, Canada
- 2Canadian Wildlife Health Cooperative, Animal Health Centre, Abbotsford, BC, Canada
- 3Biodiversity Research Centre, University of British Columbia, Vancouver, BC, Canada
- 4School of Population and Public Health, University of British Columbia, Vancouver, BC, Canada
- 5British Columbia Centre for Disease Control, Vancouver, BC, Canada
- 6Animal Health Centre, British Columbia Ministry of Agriculture, Abbotsford, BC, Canada
Norway and black rats (Rattus norvegicus and Rattus rattus) are ubiquitous urban pests, inhabiting cities worldwide. Despite their close association with people, urban rats remain difficult to control. This can be partly attributed to a general lack of information on basic rat ecology to inform management efforts. In this systematic review and narrative synthesis, we collate the published literature to provide a comprehensive description of what is known about urban rat movement, including information on home range, site fidelity, dispersal, movement patterns, barriers to, and factors impacting, movement. We also discuss the methodologies used to track and infer rat movement, as well as the advantages and limitations of employing these techniques. Our review suggests that the distances traveled by urban rats are location-specific, determined by both local resource availability and barriers to movement such as roadways. Although roads may impede rat movement, genetic techniques suggest that rats traverse roadways more often than revealed by capture-based tools, while long-distance dispersal events by either natural migration or facilitated by humans (i.e., as stowaways in transport vehicles) can maintain connectivity among distant populations. Because rat movement patterns are related to the transmission of rat-associated pathogens and the success of rodent control programs, these results have implications for city planners, pest control efforts, and public health. Therefore, we emphasize the importance of understanding local rat movement patterns in order to devise and deploy efficient and effective rat mitigation initiatives in urban centers.
Introduction
The presence of urban Norway and black rats (Rattus norvegicus and Rattus rattus) is an important and growing issue in cities globally due to their associated health and economic impacts (Feng and Himsworth, 2014). For example, rats pose a risk to public health as they are the source of a variety of zoonotic pathogens (disease-causing microbes transmissible from rats to people, e.g., Leptospira interrogans) responsible for significant human morbidity and mortality (Himsworth et al., 2013b). Infestations can also serve as a chronic stressor, impacting both the mental and physical health of residents (German and Latkin, 2016; Lam et al., 2018). Rats also damage urban infrastructure (due to chewing and burrowing activities) and contaminate foodstuffs. Finally, infestations can result in substantial economic losses, both directly (i.e., costs associated with rat control), and indirectly (i.e., costs associated with mitigating and repairing rat-associated damage) (Pimentel et al., 2005; Almeida et al., 2013). Given rapid urbanization, these issues are likely to increase in future; 55% of the world's population resides in cities, with a projected increase to 68% by 2050. Much of this growth will occur in developing regions (United Nations, 2018) where rat-associated risks are higher due to issues of inadequate housing, infrastructure, and sanitation (Himsworth et al., 2013b). Further, a lack of effective tools to address rat infestations (Parsons et al., 2017) underscores the importance of re-thinking our current approaches to rat control.
To monitor, and mitigate the impact of rats, an understanding of their spatial ecology is paramount. For example, the extent to which animals move within and utilize the environment dictates both the epidemiology of the pathogens they carry (Volkova et al., 2010; Guivier et al., 2011; Quixabeira-Santos et al., 2011) as well as the scale at which pest control efforts will have the greatest success (Bomford and O'Brien, 1995; Robertson and Gemmell, 2004; Adams et al., 2014). One of the most significant remaining knowledge gaps relevant to describing the spatial ecology of urban rats is information regarding the extent of their home range (Desvars-Larrive et al., 2018). The home range represents the area frequented by an individual (Davis et al., 1948) and typically encompasses areas used for food acquisition, mating, and rearing young (Burt, 1943). However, as individuals differentially use areas of their home range according to factors such as age, sex, population density, season, and environmental variability (Wolff, 1985; Cederlund and Sand, 1994; Wiktander et al., 2001; Dahle and Swenson, 2003; Kjellander et al., 2004; Börger et al., 2006; Safi et al., 2007), or may even migrate to new home ranges (Burt, 1943), information on home range size alone may underestimate the true area traversed by rats.
Beyond home range size, spatial ecology requires an understanding of the detailed movements of rats within cities. This includes information on dispersal distances (i.e., movement away from the natal or home area) (Drickamer, 1987), and how features of, or changes to, the urban environment impact movement. For example, a meta-analysis found that terrestrial mammals residing in cities traveled shorter distances than did their non-urban counterparts (Tucker et al., 2018). This reduced structural connectivity may result from the varied quality and size of habitat patches in cities (Dickman and Doncaster, 1987), as well as the physical barriers posed by roadways (Rondinini and Doncaster, 2002). However, as rats can occupy habitats with a diverse set of characteristics (Himsworth et al., 2014a) they may exhibit greater structural connectivity than other urban wildlife.
The objective of this review is to summarize, compare, and evaluate the published literature detailing the movement patterns of urban Norway and black rats. We describe the tools that have been used for studying urban rat movement as well as the challenges of employing these techniques. Finally, we describe how information regarding the spatial ecology of rats may be of relevance to different stakeholders and identify remaining gaps in knowledge to be addressed in future ecological research.
Methods
Search Strategy
From May 2018–July 2018 we performed systematic searches following the approach outlined by Moher et al. (2009). Our search included the databases: Web of Science CORE, CAB Direct, JSTOR, Medline, and Zoological Record. We used keyword combinations pertaining to the following concepts: Rats (Rattus norvegicus,” “Norway rat*,” “brown rat*,” “Rattus rattus,” “black rat*,” “roof rat*”), movement (dispersal, emigration, expansion, immigration, migration, movement, boundaries, distribution, domain, “home range*,” “home area*,” “site fidelity,” territory, zone) and the urban environment (urban, city, cities, municipal, suburban, residential, metropolis, metropolitan). The groups of keywords within each concept were combined using the Boolean operator “OR” and concepts were combined using “AND” (see Supplementary Table 1). We included literature from the earliest cut-off date for each database.
Study Selection
Titles and abstracts were screened for eligibility using the abstract screener function in metagear (Lajeunesse, 2016). This package presents paper titles and abstracts in a graphical user interface for reviewer coding for inclusion or exclusion. Thirty percent of papers were screened by two authors (KAB and MJL) to ensure screening consistency. Articles deemed eligible in the first round of screening were reviewed in full by both KAB and MJL. Papers were excluded if they focused on rural rat populations, global rat migration patterns, or did not measure aspects of rat movement (either directly or indirectly). Literature in languages other than English were excluded. Additional sources were added through citation searching.
Data Collection and Analysis
Included papers were grouped by trapping methodology (i.e., continuous tracking, capture-mark-recapture, genetic techniques, and proxy methods). The content of each paper was summarized using a matrix method (Garrard, 2013) in which a number of categories relevant to describing the study characteristics (i.e., study location, study scale, species studied, sample size, methods used) and rat movement (i.e., home range, dispersal, areas and extent of movement, factors impacting movement, other relevant findings) were determined a priori. Each paper was reviewed and summarized according to these categories, and we compared information within each category across studies. Findings were synthesized using a narrative synthesis methodology which involves summarizing the findings of multiple works in text format (Arai et al., 2007). The following synthesis pertains to Norway and black rat movement patterns in urban ecosystems and is reviewed within six themes derived during the synthesis: study design, home range, site fidelity, dispersal, movement patterns, barriers to movement, and factors impacting movement.
Results
Study Selection and Characteristics
Our initial search resulted in 1665 sources, 105 of which were reviewed in full (Figure 1). Of the final group of 39 papers, two were extensions of other included studies that contained additional relevant information. Therefore, we reviewed 37 unique studies examining the movements of Norway rats (n = 30), black rats (n = 6), or both (n = 1).
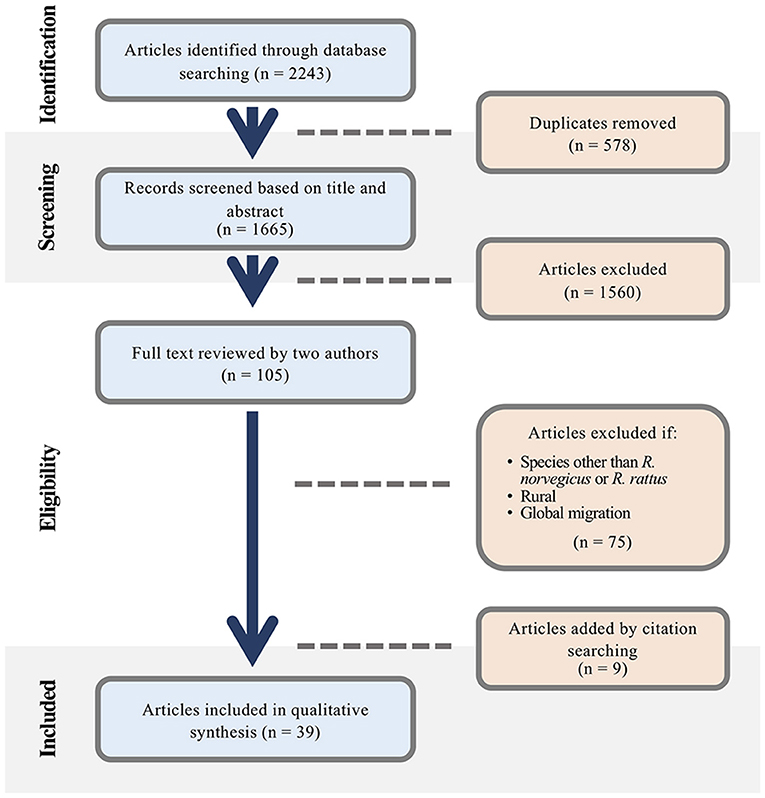
Figure 1. Preferred Reporting Items for Systematic Reviews and Meta-Analyses (PRISMA) flow chart depicting the screening process of articles included in the narrative synthesis.
Although published research on rat movement occurs as early as 1915, nearly half (48.6%; n = 18) of included studies were published in the past decade (Figure 2), and approximately half (51.4%; n = 19) were conducted in North America (Supplementary Table 2). See Supplementary Table 3 for details of the included studies.
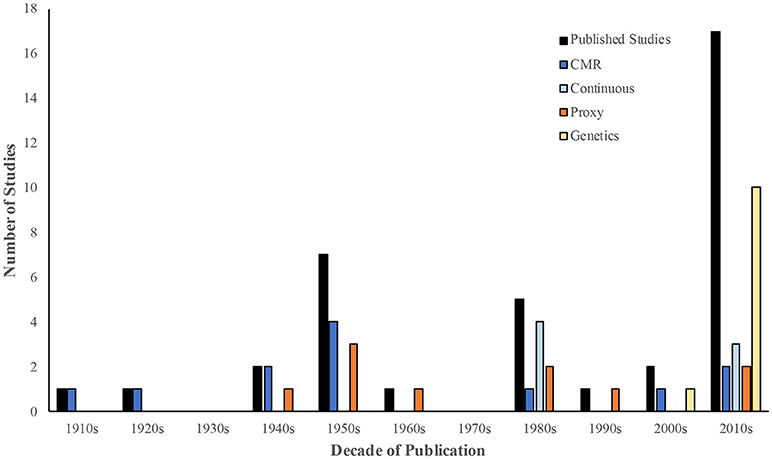
Figure 2. The number of unique published studies (n = 37) included in the review by decade of publication. Within each decade, the number of studies employing direct (Capture-Mark-Recapture (CMR) and continuous tracking), and indirect methods (proxy measures and genetics-based tools) to assess rat movement are indicated.
Study Design
In general, all studies sought to describe urban rat ecology, but most (56.8%; n = 21) explicitly mentioned using this information to inform pest control. Both direct and indirect methods were employed in the study of rat movement (Figure 2). Direct measures included Capture-Mark-Recapture (CMR; n = 12) and continuous tracking (e.g., direct observation, radio-tracking, and Global Positioning Systems (GPS); n = 7). Indirectly, movement was assessed through proxy measures of rat movement (e.g., track pads, rat tracks in snowfall, bait uptake, and feces marked with bait-specific dye; n = 11) and population genetics-based techniques (n = 11). In some instances (n = 3), multiple methods were employed. See Box 1 for an overview of these tools.
Box 1. An overview of methods commonly used for studying urban rat movement, their benefits, and limitations.
Direct Measures: To quantify the movements of urban rats, many studies rely on trapping-based techniques. Capture-Mark-Recapture (CMR) methods involve trapping and tagging individuals (e.g., with numbered ear tags or Passive Integrated Transponder (PIT) tags) for future identification. Following tagging, animals are released at their original point of capture and are later re-caught. Movement is determined by measuring the distances between the traps in which rats are caught, or in the case of PIT tags, by the distances between sensor stations through which rats pass (Parsons et al., 2015). In this way, PIT tags are advantageous as they decrease handling-time of rats. Although these tools allow researchers to track large numbers of rats (e.g., 341 in Petrie and Todd (1923), they are labor-intensive, rely heavily on trap placement, and yield fragmented data as they do not account for movement between capture points (Hayne, 1949; Glass et al., 2016). Moreover, these techniques are hindered by the neophobic nature of rats, which can result in low recapture rates and trapping bias (Barnett, 1963; Tanaka, 1963; Taylor, 1978).
To derive more complete descriptions of movement patterns, researchers have directly observed the behaviors of individual rats (Calhoun, 1963; Takahashi and Lore, 1980; Glass et al., 1989); but, this strategy is limited by the number of individuals which can be tracked at one time and is difficult when animals are not readily visible (Takahashi and Lore, 1980). Two tools that combine trapping and observation are Very-High-Frequency (VHF) radio-telemetry and Global Positioning System (GPS) technologies which require affixing rats with tags that transmit movement data in real-time (VHF), or store location data in the tag for either future retrieval or remote download by the observer (GPS). Both VHF radio-telemetry and GPS-based tools allow for improved spatial resolution of rat movements, but until recently they have been hampered by tag size which is limited to 5% of the animal's weight to minimize negative tag-associated effects (Animal Care Use Committee, 1998). While sample sizes for both methods tend to be smaller than for CMR (Tomkiewicz et al., 2010), GPS-based tools offer advantages over VHF radio-telemetry which requires close-range observation of the animal, potentially influencing natural behaviors (Cooke et al., 2004; Parsons et al., 2014). Further, radio-telemetry is challenging in cities where buildings and other structures can interfere with radio signals (LaPoint et al., 2015). Yet, while GPS-based tools may be more beneficial for these reasons, they remain difficult to deploy on urban rats due to issues of tag removal and tag-satellite line-of-sight obstruction (Byers et al., 2017). Moreover, the costs of GPS tags over radio-telemetry may make this method prohibitive (Cagnacci et al., 2010).
Indirect Measures: Rat movements can also be inferred using indirect measures. Visibly observing the tracks made by rats (e.g., in snow) allows for estimates of space use (Davis et al., 1948), but is naturally limited by the availability of snow. When the environment is not suitable for observing tracks, researchers can deploy track plates which become marked with characteristic “rat signs” when rats travel across them (Hacker et al., 2016). Rat movement may also be determined through bait consumption. This method is common among studies assessing re-infestation of areas where rat eradication efforts were previously enacted (Barnett et al., 1951; Barnett and Bathard, 1953; Greaves et al., 1968; Andrews and Belknap, 1983; Colvin et al., 1998). Bait that is dyed with a compound that colors rats' feces can also be used to calculate the extent of space use around dyed-bait stations (Davis et al., 1948). While these methods are less time consuming to enact than the direct measures previously mentioned, they provide only minimal information on rat activity.
Recently, there has been an increase in the use of population genetics-based methods to infer rat movement (see Figure 2). These tools analyze differences among individuals at specific locations of the genome caused by genetic mutations. These mutations can result in single nucleotide polymorphisms (i.e., SNPs) (Richardson et al., 2017; Combs et al., 2018a,b) or rearrangements resulting in different numbers of small repeating sequences (e.g., microsatellites) (Gardner-Santana et al., 2009; Kajdacsi et al., 2013). Using these tools, researchers can infer historical movements by identifying the distances between relatives (e.g., parents and offspring; Costa et al., 2016; Glass et al., 2016), and identifying potential migrants (e.g., individuals genetically assigned to a population other than the one in which they were caught; Kajdacsi et al., 2013; Berthier et al., 2016; Desvars-Larrive et al., 2017). These methods have the benefit of supporting large sample sizes (e.g., 1220 in Combs et al., 2018a), but they are limited to detecting first-generation migrants and movements during which rats mate and are reproductively successful, underestimating true levels of connectivity among populations (Richardson et al., 2017).
Home Range
For both Norway and black rats (hereafter termed “rats” when referencing both species), the home range size and shape is determined by access to feeding and harborage sites (Davis et al., 1948; Recht, 1982; Low et al., 2013) as well as access to mates (Low et al., 2013; Glass et al., 2016). These associations lead to irregularly shaped home ranges with individuals often moving along narrow pathways connecting harborage and food sources (Davis et al., 1948; Recht, 1982; Recht et al., 1983). The presence of conspecifics may also influence home range size, as some individuals have been found to avoid the home ranges of other rats (Low et al., 2013; Oyedele et al., 2015). Beyond the value of regular access to resources, an intimate familiarity with the features of the home range may serve as a protective measure for rats. For example, individuals within their home range have been recorded entering areas of cover (e.g., a rat hole) more rapidly than those in areas outside of their home range (Davis et al., 1948). This is further evidenced by rats' neophobic behaviors toward new and/or introduced features such as traps (Barnett, 1963).
Rats are familiar with the extent of their home range (Recht, 1982; Recht et al., 1983), but usage is concentrated within a fraction of this area (Low et al., 2013; Oyedele et al., 2015). Termed the “core home range,” this region represents the space where an animal spends 50% of its time (Downs and Horner, 2008). The two studies which calculated core home range size for urban rats estimated its size as 11% of the total home range for Norway rats (Oyedele et al., 2015), and approximately 31% of the space used for black rats (Low et al., 2013). Studies in both species have found that the core home range encompasses important food sources, the home burrow, and areas of dense vegetation (Davis et al., 1948; Recht, 1982; Recht et al., 1983). However, rats will travel throughout the home range to occupy alternate burrows, particularly when their home burrow is disrupted (Recht, 1982; Recht et al., 1983). In fact, rats have been found to switch the location and extent of their home range altogether (Davis et al., 1948; Low et al., 2013), signifying that the size, shape, usage, and location of the home range are flexible for individual rats and dependent on physical and environmental characteristics.
Home range size has also been found to vary by sex, with evidence that male Norway rats occupy larger home ranges than do females (Tanaka and Kawashima, 1951; Oyedele et al., 2015). This may be due to differences in reproductive behavior, whereby males increase their ranges to actively search for mates (Dowding and Murphy, 1994). For example, in Norway rats, the area of the total and the core home range of males was approximately 13X and 5X larger than that of females, respectively (Oyedele et al., 2015). In black rats, the total and core home range area for males was 4X and 3.5X greater than for females, respectively (Low et al., 2013). Indeed, home ranges of male black rats have been shown to overlap with those of other males and females, whereas females had home ranges that were exclusive of each other (Low et al., 2013), further supporting the role of mate-searching in determining the extent of the home range.
Studies also indicate that home range size and shape vary by location (Davis et al., 1948; Recht, 1982; Recht et al., 1983; Oyedele et al., 2015). For example, the home range for Norway rats in Baltimore, Maryland was 30–45 m in diameter (Davis et al., 1948), and in George Town, Malaysia the average home range size was 130 m2 (Oyedele et al., 2015). Similarly, for black rats, home range size did not exceed 30.5 m in diameter in the City of Orange, California (Recht et al., 1983) while on Christmas Island it was 5330 m2 (Low et al., 2013). As home ranges of urban rats are irregularly shaped (Davis et al., 1948; Recht, 1982; Recht et al., 1983), and because home range estimates will vary depending on the methodology used (see Box 1) it is impossible to directly compare these measurements. However, studies in both Norway and black rats have suggested that differences in home range size between study sites may be due to differences in resource availability (Low et al., 2013; Oyedele et al., 2015).
Site Fidelity
Distances traveled by rats are dependent on the presence of harborage and food in the environment (Creel, 1915; Petrie and Todd, 1923; Davis et al., 1948; Heiberg et al., 2012). When these are readily available, rats display a strong site fidelity, rarely leaving their home area. For example, CMR studies in Norway rats have found that 27–63% of rats in residential areas were recaught in the same location as their prior capture (King, 1950; Tanaka and Kawashima, 1951; Glass et al., 1989) although this was less common (e.g., 8% of rats) in urban parklands (Glass et al., 1989). Genetic results support these findings. For example, by DNA fingerprinting methods 95% (Gardner-Santana et al., 2009) and 97% (Glass et al., 2016) of rats were genetically assigned to the area of their capture (i.e., based on genetic similarity to other rats in the vicinity of their capture, they were more likely to have been born in the area in which they were caught than in another sampled site). Strong site fidelity was also revealed by Costa et al. (2016), who genotyped male rats as well as pregnant females and their offspring, and found that males with a high probability of siring offspring (>99%) were within 70 ± 58 m of the pregnant female. Similarly, Richardson et al. (2017) demonstrated that rat movement occurred mostly within the area (i.e., valley) from which rats were sampled. Estimates for site fidelity in urban black rats have not been documented.
Daily movements by rats are typically over short distances. For Norway rats, various CMR studies have documented typical movements ranging from 10 to 20 m (Davis et al., 1948; Tanaka and Kawashima, 1951; Glass et al., 1989; Parsons et al., 2015). In urban parklands, Norway rats have been recorded moving greater average distances of 25 m (Glass et al., 1989). In comparison to CMR, genetic analyses have demonstrated that Norway rats move further still (e.g., 30–150 m), approximately corresponding to the length of a city block (Gardner-Santana et al., 2009; Combs et al., 2018b). Interestingly, limited movement was supported by an analysis of the ectoparasite communities of urban Norway rats. In this study, Angley et al. (2018) found that rats located near each other geographically had more similar assemblages of ectoparasites than did rats located further apart. Because rat ectoparasites are transmitted among individuals via close contact, this implied that rats near each other came into contact with each other more frequently than those further apart.
In urban sewer systems, rats have been found to travel further distances day-to-day than their above ground counterparts. For example, in London, England sewer rates moved up to 77 m (Bentley et al., 1958) while in Copenhagen, Denmark, rats traveled up to 200 m in a day (Heiberg et al., 2012), over 10X the distance recorded for surface populations. In this way, sewers may be more easily traversable, serving as conduits to movement. Interestingly, while daily distances traveled by rats are thought to be greater for males than females (Davis et al., 1948), this does not appear to be the case for sewer populations (Heiberg et al., 2012), suggesting that the environment is a strong determinant of distances traveled.
Dispersal
Dispersal of rats away from their natal site is generally over short distances. For example, mean dispersal distances between parents and offspring have been recorded as 45 m for Norway rats (Combs et al., 2018b) and 496 m for black rats (Mangombi et al., 2016) while distances between putative parents (dams and sires of offspring) ranged from 0 to 353 m for Norway rats (Costa et al., 2016; Glass et al., 2016). Genetic patterns of isolation by distance (whereby individuals are more closely related to rats in neighboring areas than they are to individuals further away) corroborate these trends (Gardner-Santana et al., 2009; Mangombi et al., 2016; Combs et al., 2018a,b). For example, in a multi-city comparison of Norway rat population genetic structures, rats were generally highly related to each other at distances within 500 m (Combs et al., 2018a). However, evidence for isolation by distance has not been found by all studies (Kajdacsi et al., 2013; Berthier et al., 2016).
Less often, dispersal can occur over long distances. For example, dispersal distances have been recorded up to 11.5 km for Norway rats (Gardner-Santana et al., 2009). Although such extended movements are infrequent [e.g., 19 of 230 black rats (8.2%) were classified as migrants in Sahel Niger (Berthier et al., 2016)], evidence of gene flow among Norway rat colonies from 1.5 to 3 km apart suggests that connectivity among populations is maintained by immigration amongst colonies (Gardner-Santana et al., 2009; Combs et al., 2018a). This dispersal may be non-random, whereby individuals move among similar habitat types (e.g., residential areas vs. mixed-used; Angley et al., 2018) and may also be facilitated anthropogenically, such as by commercial transport along road networks (Berthier et al., 2016).
For rats, dispersal has been primarily associated with resource availability and competition, dominance hierarchies, and mating behavior (Calhoun, 1963; Glass et al., 1989). Specifically, when feeding and harborage sites are scare, rats may travel significant distances in search of resources. For example, when in an unfamiliar resource-poor area, Norway rats have been recorded traversing twice the distance as individuals in unfamiliar resource-rich locations (6 vs. 3 km; Creel, 1915). Mate-searching is also an important driver of dispersal, with rats (particularly males) extending their movements in search of mates (Davis et al., 1948; King, 1950; Glass et al., 2016). Sex-biased dispersal has been documented in Norway rats where the majority of migrants are often reproductively mature males (Gardner-Santana et al., 2009; Kajdacsi et al., 2013; Desvars-Larrive et al., 2017). Sex-biased dispersal has been further evidenced by close proximity among related females caught at a fine spatial scale, suggesting that females moved shorter distances than males in the same population (Desvars-Larrive et al., 2017). While these patterns have not been observed in all studies (Gardner-Santana et al., 2009; Combs et al., 2018b) they align with foundational experimental research on Norway rats that found that mature male rats dispersed greater distances than adult females and juveniles (Calhoun, 1963).
In cases where rats immigrate into stable populations, invading rats may be unable to successfully establish home ranges, necessitating extended movements of evicted rats. For instance, the introduction of 112 foreign rats into a city block resulted in the invaders being more likely to emigrate from the site of release than were resident rats in the same area (Calhoun, 1948). Further, the immigration of large numbers of rats into a population may temporarily decrease the reproductive rate of the resident population (Davis and Christian, 1956). Therefore, although dispersal can maintain connectivity among populations, not all immigration events are successful, and can, in some cases, disrupt the regular population dynamics of the resident population.
Movement Patterns
Rats are generally found to be nocturnal (Recht, 1982; Recht et al., 1983) with heightened activity 2–3 h before sunrise and after sunset (Takahashi and Lore, 1980; Recht et al., 1983). However, rats may also be active during the day (Recht, 1982). Indeed, Parsons et al. (2015) found that rats were active between 06:00 and 19:00 with declining activity in the late morning/early afternoon. These activity patterns have also been shown to differ between the sexes, with males generally active longer than females (Parsons et al., 2015), leaving their burrow 1–2 h before females living in the same area (Oyedele et al., 2015). However, as rat activity varies by location, and across differing study methodologies, it is unclear how aspects of the environment and study design contribute to these differences in activity patterns.
During times of activity, rats generally traverse the same pathways (Recht, 1982; Recht et al., 1983; Oyedele et al., 2015). However, they may use alternate routes to adapt to environmental change. For instance, Recht (1982) recorded Norway rats using alternate pathways both to obtain food left over from picnickers and to avoid people. Norway rats typically move along the ground through narrow runways (Davis et al., 1948), near to fences and other cover (Glass et al., 1989), while black rats utilize aerial features such as greenery, pipes, and wires (Worth, 1950). Both species have been found to travel between adjacent buildings (Petrie et al., 1924; King, 1950; Tanaka and Kawashima, 1951; Recht, 1982; Recht et al., 1983; Hacker et al., 2016). Indeed, Tanaka and Kawashima (1951) observed rats moving among three to four houses in a city block over the course of a single week. Additionally, rats may travel between surface and sewer locations (Colvin et al., 1998; Heiberg et al., 2012), but not in all cases (Gras et al., 2012). In contrast, rats do not appear to travel between adjacent, but separate, sewer systems (Heiberg et al., 2012).
Barriers to Movement
Landscape features such as roads, waterways, and “resource-deserts” (areas with very limited resources) may impede the movement of rats throughout cities (Combs et al., 2018a). In general, roadways are reported as the most common barrier to rat movement (Petrie et al., 1924; Davis et al., 1948; King, 1950; Worth, 1950; Traweger and Slotta-Bachmayr, 2005; Richardson et al., 2017). This is supported by findings that Norway rat home ranges rarely overlap with roads (Davis et al., 1948), and by few cases of rats moving among city blocks (Petrie and Todd, 1923; Calhoun, 1948; Davis et al., 1948; Emlen et al., 1949; Worth, 1950). For example, of 146 black rats trapped in Egypt, only one moved between city blocks (Petrie and Todd, 1923). Likewise, in a study which followed Norway rat tracks in fresh snowfall, Davis et al. (1948) estimated the rate of road crossings to vary from one crossing every 66 days to one crossing a day, with the frequency of crossing reliant on resource availability.
The permeability of roadways is dependent on their width. While larger roadways have deterred movement more than smaller roadways (Petrie and Todd, 1923), even the width of an alley may impede rats. For example, Davis et al. (1948) found that almost all dyed feces were located on the same side of the alley as bait stations. Although rats may avoid crossing alleys, they traverse them more frequently than roadways. An observation of rat movement found that rats crossed alleys 80X more often than they crossed roads (Glass et al., 1989). Given that rats may also move greater average distances in underground infrastructure such as sewers (Heiberg et al., 2012), barriers posed by roads may be overcome by alternate means of crossing heavily trafficked spaces.
While CMR studies suggest that movement among city blocks are infrequent, genetic analyses demonstrating gene flow reveal that movement is more frequent (Gardner-Santana et al., 2009; Glass et al., 2016; Mangombi et al., 2016; Combs et al., 2018b). For example, by analyzing the genetics of pregnant females and their offspring, Glass et al. (2016) demonstrated that females mated most often with males trapped in alleys other than their own. The authors suggested that this pattern likely occurred through “mate chases” in which groups of males left their home site to mate with females in neighboring blocks (support for multiple paternity of litters is further supported by Costa et al., 2016). In combination, these results indicate that roads are permeable to rat movement and that movement among blocks may be driven by mate searching (Glass et al., 2016) or resource availability (Davis et al., 1948).
Factors Impacting Movement
Because rat movement patterns are dictated by features of the urban environment, changes in weather and anthropogenic habitat modification alter normal rat movement patterns. For example, Recht (1982) observed that Norway rats cease movement in rain, while black rats continue to forage (Recht et al., 1983). Habitat modification can alter rat movement due to either the removal of areas of harborage and/or by blocking typical movement routes. For example, Recht (1982) found that habitat modification (e.g., trimming of vegetation, and removal of debris) resulted in increased Norway rat activity and exploration, larger home ranges, and movement into previously unvisited areas, while construction caused black rats to move to alternate burrows (Recht et al., 1983).
Control methods (e.g., trapping and poisoning) can also promote rat movement, as individuals migrate to occupy and recolonize previously targeted sites. For example, Kajdacsi et al. (2013) demonstrated that following control efforts, there was both population replacement (i.e., local rat reproduction by surviving rats) and recolonization due to the migration of individuals from surrounding areas. Rat migration may also play a role in the re-infestation of urban sewers systems. For example, following rodent reductions of up to 88% (as indicated by bait uptake), rat populations increased from 3 to 20% per week (Barnett and Bathard, 1953; Bentley et al., 1959; Greaves et al., 1968). This increase was attributed in part to rat immigration from within the same sewer system (Greaves et al., 1968) and from an influx of surface populations (Barnett and Bathard, 1953). Such population rebounds can be rapid, occurring in as little as 4 weeks post-eradication efforts (Hacker et al., 2016).
Discussion
Overall this synthesis highlighted a number of important characteristics of urban rat movement. Specifically, rat movement is dependent on the distribution and availability of important resources such as food and harborage and is limited by the barriers posed by features including roads and waterways. While roads may relegate individuals to home ranges corresponding to the limits of a city block, recent studies suggest that rats cross city streets more often than previously estimated. Further, normal movement patterns may be altered due to environmental change, prompting rats to move greater distances still. These findings have several important implications for city planners, pest control professionals, and public health officials seeking to monitor and mitigate the economic and public health impacts posed by urban rats. However, despite increased scientific attention to describing urban rat ecology in the past decade, this review reveals that the specific details of their spatial ecology remain largely undescribed.
Implications for City Planners
This review illustrates that features of the urban environment influence the spatial ecology of rats. Because city planners determine and design many aspects of cities, they have the potential to create spaces less prone to rat infestation. For example, as features such as urban parkland may be more easily traversed by rats (Glass et al., 1989), approaches to reduce waste and improve infrastructure/building conditions in and around these areas may lower rats' ability to infest surrounding regions. However, while our review suggests that parkland and sewer systems may facilitate rat movement, the specific landscape features and socioeconomic attributes which determine the connectivity of rat populations within cities are still poorly understood (LaPoint et al., 2015). Indeed, the only multi-city comparison of urban rat population structure found that the local environment is a strong determinant of rat movement (Combs et al., 2018a). Therefore, it is becoming increasingly important to identify both common features among cities which influence rat movement as well as local features, which can be used by city planners to target and predict areas prone to rat infestation and re-infestation. Targeting these features is particularly important in under-served and marginalized communities where residents are at heightened risk of exposure to large numbers of rats and their associated health and economic impacts (Himsworth et al., 2013b; Costa et al., 2015b).
Urban centers are continuously expanding and undergoing dramatic habitat modification (Grimm et al., 2008). These issues are enhanced in rapidly urbanizing under-resourced settings (United Nations, 2018) where unplanned urban development and land use changes (e.g., Chitrakar et al., 2016; Pawe and Saikia, 2018) pose additional challenges. Our review suggests that habitat disturbance can instigate long distance movements by rats; this is particularly relevant to city planners as ubiquitous activities such as demolition and construction may both create an environment suitable for rat harborage (e.g., open soil and shelter from equipment) (Colvin and Jackson, 1999) as well as drive rats from disturbed sites to surrounding areas (Richter, 1968; Battersby et al., 2002). To pre-empt the potential for rat colonization and migration, city planners should consider employing Integrated Pest Management approaches to proactively decrease habitat suitability and migration risks. Integrated Pest Management is a multi-faceted approach which incorporates long-term planning goals, data management, as well as partnership among governments, private pest control companies and communities (Colvin and Jackson, 1999). Such an approach may include implementing policies that require the eradication of rats prior to the demolition of a building to minimize the efflux of rats and the degree of subsequent colonization. This requires coordination among developers and private pest control companies to identify areas for control, enact control efforts, and monitor the success of the control campaign. For regions where development is primarily undertaken by urban dwellers as opposed to municipalities (i.e., in urban slums), city planners might instead focus on educating communities about the importance of undertaking pest control before and during construction, as well as providing resources such as traps or deploying private pest control companies to areas with ongoing development. In tandem, actions to reduce food sources and harborage sites in adjacent city blocks or homes can also decrease their attractiveness to rats and their ability to support rat population growth. These initiatives also require community involvement and educational programs to inform residents about the ways to properly dispose of food waste and remove potential harborage sites (Colvin et al., 1998). Although Integrated Pest Management approaches require sustained and significant investment (i.e., in time and personnel), reactive approaches which fail to address the underlying features that promote rat abundance and facilitate rat movement are likely to remain ineffective.
Implications for Pest Control
It is necessary for control methods to account for rat movement patterns. Studies have demonstrated that control campaigns aimed at culling rats alone can be compromised by rapid population rebounds due to reproduction by surviving rats (Barnett and Bathard, 1953; Hacker et al., 2016), and/or immigration of individuals from surrounding areas (Kajdacsi et al., 2013). Given that rats readily cross roads for resources and mating (Davis et al., 1948; Glass et al., 2016), our review suggests that limiting the scale of control to a single property or city block is likely to be ineffective due to reinvasion of the targeted site. Instead, efforts should focus on effectively identifying and targeting areas at the scale of “eradication units.” These areas represent the spatial scale at which rats are interconnected, allowing for recolonization following a control intervention (Abdelkrim et al., 2007). For example, in Salvador, Brazil where the majority of Norway rat movement was found to occur within a valley, targeting rat populations at the level of the valley might be appropriate (Richardson et al., 2017). In contrast, a study evaluating the genetic signatures of black rat populations before and after an eradication campaign on four islets in the French Caribbean, found that control efforts would need to extend to surrounding islands to minimize the potential for re-invasion (Abdelkrim et al., 2007). Because the extent of movement varies by location (Combs et al., 2018a), deriving specific recommendations as to the scale of pest control efforts is difficult. Yet, to design effective control strategies, research that quantifies the contribution of landscape attributes to rat migration is necessary to help pest control professionals define the scale of control and prevention approaches. Further, to support the integration of scientific knowledge into actionable information for pest control professionals, it is necessary that projects evaluate how scaling control efforts to the level of local rat movement (i.e., eradication units) compares to traditional pest control efforts.
An understanding of rat movement is not only necessary for more effectively implementing current pest control practices, but it is also important in developing and deploying future pest control innovations. For example, gene drive technologies have received increasing attention for their potential pest control applications. Gene drive technologies involve genetically engineering individuals so that sets of genes are disseminated within populations through sexual reproduction. For pests, genes which lower the fertility and fecundity of individuals are of particular interest (Moro et al., 2018). Because the spread of these traits throughout a population is reliant on interactions among individuals, understanding local rat movement ecology will be necessary to inform the implementation of these technologies in these species.
Implications for Public Health
Our review supports the long-held position that much of rats' activities remain within the confines of a single city block. Because many of the pathogens carried by rats are transmitted through close contact among conspecifics (Childs et al., 1998; Himsworth et al., 2013b) this limited movement implies that most transmission events are also restricted to within-block populations. These findings support prior research by Himsworth et al. which demonstrated significant heterogeneity in pathogen prevalence across adjacent city blocks (Himsworth et al., 2013a, 2014b, 2015), such that some blocks had many infected rats and other blocks had very few. Similar findings have been demonstrated in Salvador, Brazil, where shedding of the pathogen L. interrogans by Norway rats varied significantly by location (Costa et al., 2015b). If limited movement allows for the clustering of pathogens, then the risk of encountering an infected rat may be site specific. In tandem with these results, our review suggests that activities that disrupt rat colonies could increase movement within and between blocks. Evidence suggests that when rats migrate to surrounding colonies, they fight (Calhoun, 1948), and as aggressive behaviors are the primary mode of transmission of some pathogens (e.g., Streptobacillus monilliformis, and Seoul hantavirus; Himsworth et al., 2013b, migration events could promote disease spread. Indeed, Lee et al. (2018) demonstrated that employing lethal control techniques may increase pathogen prevalence among remaining rats. This increase could be due, in part, to the effects of pest control on rat movement, and underscores the potential role of methods which reduce rat population size without prompting migration (e.g., rat birth control, gene drive technologies). Therefore, to monitor and mitigate the potential health risks posed by rats, public health officials require information on the distribution of rat-associated pathogens, the role of movement in the transmission of pathogens among urban rats, and how different approaches to pest control can minimize these risks.
Limitations
Although one of the aims of this review was to compare and contrast data across study locations, deriving quantitative estimates of urban rat movement patterns is difficult due to the limited number of studies evaluating urban rat movement, and an over-representation of research in developed countries (Supplementary Table 2). These limitations are compounded by differences between, and limitations of, the included studies. First, rat movement estimates have been derived using various methods which have a suite of limitations (see Box 1). These limitations highlight the challenges of studying the movements of not only rats (Parsons et al., 2017; Desvars-Larrive et al., 2018), but urban wildlife in general (LaPoint et al., 2015). Second, even among studies which employ similar techniques to measure movement (i.e., CMR), researchers have used a variety of models for calculating home range size (Low et al., 2013; Oyedele et al., 2015). In tandem, these differences limit the ability to make direct comparisons. Yet, these issues emphasize the importance of employing multiple tools to address methodological limitations (e.g., combining continuous, trapping-, and genetics-based methods) and utilizing either multiple or standardized calculation methods to estimate movement parameters to foster comparability amongst studies.
Conclusions
Overall, this review highlights a number of important features with regards to rat movement and underscores their significance for stakeholders addressing urban rat infestations. Despite the information synthesized in this review, a number of important questions remain (Table 1). To address these knowledge gaps, we suggest that future research prioritize collaborative, multi-jurisdictional research which incorporates multiple methods and standardized approaches to measure rat movement.
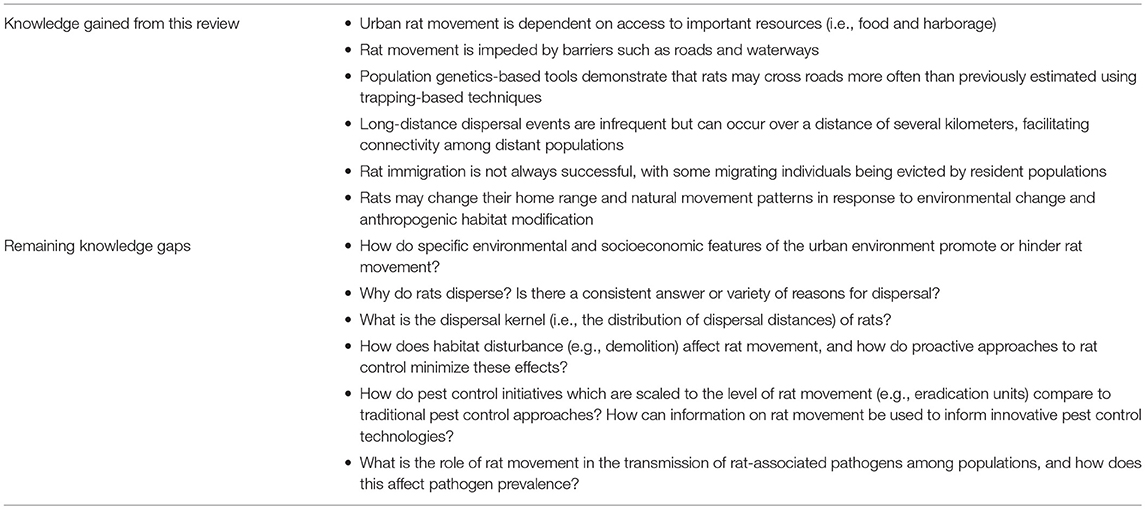
Table 1. Conclusions from a review of the published literature describing Norway and black rat (Rattus norvegicus and Rattus rattus) movement in urban centers.
Data Availability
All data relevant to this review is included in the manuscript and the supplementary file.
Author Contributions
This review was conceived of by KB and designed by KB and CH. Inclusion criteria was determined by KB and ML who also screened and extracted relevant information from all papers. The first draft of the manuscript was written by KB with substantial contributions by ML and CH. DP provided feedback on earlier drafts of the manuscript, and all authors approved the final version for submission.
Funding
Funding for publication of this study was provided by a Natural Sciences and Engineering Research Council (NSERC) grant held by DP, Grant No. 2015-05058.
Conflict of Interest Statement
The authors declare that the research was conducted in the absence of any commercial or financial relationships that could be construed as a potential conflict of interest.
The handling editor and author, CH, declared their involvement as co-editors in the Research Topic, and confirm the absence of any other ongoing collaboration.
Acknowledgments
We would like to thank Dr. Michael Whitlock and Matthew Combs for their review of an earlier version of this manuscript.
Supplementary Material
The Supplementary Material for this article can be found online at: https://www.frontiersin.org/articles/10.3389/fevo.2019.00013/full#supplementary-material
Abbreviations
CMR, capture-mark-recapture.
References
Abdelkrim, J., Pascal, M., and Samadi, S. (2007). Establishing causes of eradication failure based on genetics: case study of ship rat eradication in Ste. Anne Archipelago. Conserv. Biol. 21, 719–730. doi: 10.1111/j.1523-1739.2007.00696.x
Adams, A. L., van Heezik, Y., Dickinson, K. J. M., and Robertson, B. C. (2014). Identifying eradication units in an invasive mammalian pest species. Biol. Invasions 16, 1481–1496. doi: 10.1007/s10530-013-0586-9
Almeida, A., Corrigan, R., and Sarno, R. (2013). The economic impact of commensal rodents on small businesses in Manhattan's chinatown: trends and possible causes. Sub. Sust. 1:2. doi: 10.5038/2164-0866.1.1.2
Andrews, R. V., and Belknap, R. W. (1983). Efficacy of alpha-chlorhydrin in sewer rat control. J. Hyg. 91, 359–366.
Angley, L. P., Combs, M., Firth, C., Frye, M. J., Lipkin, I., Richardson, J. L., et al. (2018). Spatial variation in the parasite communities and genomic structure of urban rats in New York City. Zoonoses Public Health 65, e113–e123. doi: 10.1111/zph.12418
Animal Care Use Committee (1998). Guidelines for the capture, handling, and care of mammals as approved by the American Society of Mammalogists. J. Mammal. 79, 1416–1431. doi: 10.2307/1383033
Arai, L., Britten, N., Popay, J., Roberts, H., Petticrew, M., Rodgers, M., et al. (2007). Testing methodological developments in the conduct of narrative synthesis: a demonstration review of research on the implementation of smoke alarm interventions. Evid. Policy 3, 361–383. doi: 10.1332/174426407781738029
Barnett, S. A., Bathard, A. H., and Spencer, M. M. (1951). Rat populations and control in two English villages. Ann. Appl. Biol. 38, 444–463.
Battersby, S. A., Parsons, R., and Webster, J. P. (2002). Urban rat infestations and the risk to public health. J. Environ. Health Res. 1, 4–12.
Bentley, E. W., Bathard, A. H., and Riley, J. D. (1958). The control of rats living between access points in sewers. J. Hyg. 56, 19–28.
Bentley, E. W., Bathard, A. H., and Riley, J. D. (1959). The rates of recovery of sewer rat populations after poisoning. J. Hyg. 57, 291–298.
Berthier, K., Garba, M., Leblois, R., Navascués, M., Tatard, C., Gauthier, P., et al. (2016). Black rat invasion of inland Sahel: insights from interviews and population genetics in south-western Niger. Biol. J. Linn. Soc. 119, 748–765. doi: 10.1111/bij.12836
Bomford, M., and O'Brien, P. (1995). Eradication or control for vertebrate pests? Wildl. Soc. Bull. 23, 249–255.
Börger, L., Franconi, N., Ferretti, F., Meschi, F., De Michele, G., Gantz, A., et al. (2006). An integrated approach to identify spatiotemporal and individual-level determinants of animal home range size. Am. Nat. 168, 471–485. doi: 10.1086/507883
Burt, W. H. (1943). Territoriality and home range concepts as applied to mammals. J. Mammal. 24, 346–352.
Byers, K. A., Lee, M. J., Donovan, C. M., Patrick, D. M., and Himsworth, C. G. (2017). A novel method for affixing global positioning system (GPS) tags to urban Norway rats (Rattus norvegicus): feasibility, health impacts and potential for tracking movement. J. Urban Ecol. 3:e68496. doi: 10.1093/jue/jux010
Cagnacci, F., Boitani, L., Powell, R. A., and Boyce, M. S. (2010). Animal ecology meets GPS-based radiotelemetry: a perfect storm of opportunities and challenges. Phil. Trans. R. Soc. B. 365, 2157–2162. doi: 10.1098/rstb.2010.0107
Calhoun, J. B. (1948). Mortality and movement of brown rats (Rattus norvegicus) in artificially supersaturated populations. J. Wildl. Manage. 12, 167–172.
Calhoun, J. B. (1963). The Ecology and Sociology of the Norway Rat. Bethesda, MD: US Department of health, Education and Welfare.
Cederlund, G., and Sand, H. (1994). Home-range size in relation to age and sex in moose. J. Mammal. 75, 1005–1012.
Childs, J. E., McLafferty, S. L., Sadek, R., Miller, G. L., Khan, A. S., DuPree, E. R., et al. (1998). Epidemiology of rodent bites and prediction of rat infestation in New York city. Am. J. Epidemiol. 148, 78–87.
Chitrakar, R. M., Baker, D. C., and Guaralda, M. (2016). Urban growth and development of contemporary neighbourhood public space in Kathmandu Valley, Nepal. Habitat Int. 53, 30–38. doi: 10.1016/j.habitatint.2015.11.006
Colvin, B. A., and Jackson, W. B. (1999). “Urban rodent control programs for the 21st century,” in Ecologically-Based Rodent Management, eds G. R. Singleton, H. Liers, and Z. Zhang (Canberra, ACT: Australian Centre for International Agricultural Research), 243–257.
Colvin, B. A., Swift, T. B., and Fothergill, F. E. (1998). “Control of Norway rats in sewer and utility systems using pulsed baiting methods,” in Proceedings of the Eighteenth Vertebrate Pest Conference (Davis, CA), 247–253.
Combs, M., Byers, K. A., Ghersi, B. M., Blum, M. J., Caccone, A., Costa, F., et al. (2018a). Urban rat races: spatial population genomics of brown rats (Rattus norvegicus) compared across multiple cities. Proc. R. Soc. B. 285:20180245. doi: 10.1098/rspb.2018.0245
Combs, M., Puckett, E. E., Richardson, J., Mims, D., and Munshi-South, J. (2018b). Spatial population genomics of the brown rat (Rattus norvegicus) in New York City. Mol. Ecol. 27, 83–98. doi: 10.1111/mec.14437
Cooke, S. J., Hinch, S. G., Wikelski, M., Andrews, R. D., Kuchel, L. J., Wolcott, T. G., et al. (2004). Biotelemetry: a mechanistic approach to ecology. Trends Ecol. Evol. 19, 334–343. doi: 10.1016/j.tree.2004.04.003
Costa, F., Hagan, J. E., Calcagno, J., Kane, M., Torgerson, P., Martinez-Silveira, M. S., et al. (2015a). Global morbidity and mortality of leptospirosis: a systematic review. PLoS Negl. Trop. Dis. 9:e0003898.doi: 10.1371/journal.pntd.0003898
Costa, F., Richardson, J. L., Dion, K., Mariani, C., Pertile, A. C., Burak, M. K., et al. (2016). Multiple paternity in the Norway rat, Rattus norvegicus, from urban slums in Salvador, Brazil. J. Hered. 107, 181–186. doi: 10.1093/jhered/esv098
Costa, F., Wunder, E. A. Jr., De Oliveira, D., Bisht, V., Rodrigues, G., Reis, M. G., et al. (2015b). Patterns in Leptospira shedding in Norway rats (Rattus norvegicus) from Brazilian slum communities at high risk of disease transmission. PLoS Negl. Trop. Dis. 9:e0003819. doi: 10.1371/journal.pntd.0003819
Creel, R. H. (1915). The migratory habits of rats: with special reference to the spread of plague. Public Health Rep. 30, 1679–1685.
Dahle, B., and Swenson, J. E. (2003). Home ranges in adult Scandinavian brown bears (Ursus arctos): effect of mass, sex, reproductive category, population density and habitat type. J. Zool. 260, 329–335. doi: 10.1017/S0952836903003753
Davis, D. E., and Christian, J. J. (1956). Changes in Norway rat populations induced by introduction of rats. J. Wildl. Manage 20, 378–383.
Davis, D. E., Emlen, J. T., and Stokes, A. W. (1948). Studies on home range in the brown rat. J. Mammal. 29, 207–225.
Desvars-Larrive, A., Baldi, M., Walter, T., Zink, R., and Walzer, C. (2018). Brown rats (Rattus norvegicus) in urban ecosystems: are the constraints related to fieldwork a limit to their study? Urban Ecosyst. 21, 951–964. doi: 10.1007/s11252-018-0772-8
Desvars-Larrive, A., Pascal, M., Gasqui, P., Cosson, J.-F., Benoît, E., Lattard, V., et al. (2017). Population genetics, community of parasites, and resistance to rodenticides in an urban brown rat (Rattus norvegicus) population. PLoS ONE 12:e0184015. doi: 10.1371/journal.pone.0184015
Dickman, C. R., and Doncaster, C. P. (1987). The ecology of small mammals in urban habitats. 1. Populations in a patchy environment. J. Anim. Ecol. 56, 629–640. doi: 10.2307/5073
Dowding, J. E., and Murphy, E. C. (1994). Ecology of ship rats (Rattus rattus) in a kauri (Agathis australis) forest in Northland, New Zealand. NZ. J. Ecol. 18, 19–28. doi: 10.2307/24053652
Downs, J. A., and Horner, M. W. (2008). Effects of point pattern shape on home-range estimates. J. Wildl. Manage. 72, 1813–1818. doi: 10.2193/2007-454
Emlen, J. T., Stokes, A. W., and Davis, D. E. (1949). Methods for estimating populations of brown rats in urban habitats. Ecology 30, 430–442.
Feng, A. Y. T., and Himsworth, C. G. (2014). The secret life of the city rat: a review of the ecology of urban Norway and black rats (Rattus norvegicus and Rattus rattus). Urban Ecosyst. 17, 149–162. doi: 10.1007/s11252-013-0305-4
Gardner-Santana, L. C., Norris, D. E., Fornadel, C. M., Hinson, E. R., Klein, S. L., and Glass, G. E. (2009). Commensal ecology, urban landscapes, and their influence on the genetic characteristics of city-dwelling Norway rats (Rattus norvegicus). Mol. Ecol. 18, 2766–2778. doi: 10.1111/j.1365-294X.2009.04232.x
Garrard, J. (2013). Health Sciences Literature Review Made Easy. Burlington, MA: Jones and Bartlett Learning.
German, D., and Latkin, C. A. (2016). Exposure to urban rats as a community stressor among low-income urban residents. J. Community Psychol. 44, 249–262. doi: 10.1002/jcop.21762
Glass, G. E., Childs, J. E., Korch, G. W., and Leduc, J. W. (1989). Comparative ecology and social interactions of Norway rat (Rattus norvegicus) populations in Baltimore, Maryland. Occas. Pap. Mus. Nat. Hist. 130, 1–33.
Glass, G. E., Klein, S. L., Norris, D. E., and Gardner, L. C. (2016). Multiple paternity in urban Norway rats: extended ranging for mates. Vector Borne Zoo. Dis. 16, 342–348. doi: 10.1089/vbz.2015.1816
Gras, L. M., Patergnani, M., and Farina, M. (2012). Poison-based commensal rodent control strategies in urban ecosystems: some evidence against sewer-baiting. EcoHealth 9, 75–79. doi: 10.1007/s10393-012-0748-8
Greaves, J. H., Hammond, L. E., and Bathard, A. H. (1968). The control of re-invasion by rats of part of a sewer network. Ann. Appl. Biol. 62, 341–351.
Grimm, N. B., Faeth, S. H., Golubiewski, N. E., Redman, C. L., Wu, J., Bai, X., et al. (2008). Global change and the ecology of cities. Science 319, 756–760. doi: 10.1126/science.1150195
Guivier, E., Galan, M., Chaval, Y., Xuéreb, A., Ribas Salvador, A., Poulle, M. L., et al. (2011). Landscape genetics highlights the role of bank vole metapopulation dynamics in the epidemiology of Puumala hantavirus. Mol. Ecol. 20, 3569–3583. doi: 10.1111/j.1365-294X.2011.05199.x
Hacker, K. P., Minter, A., Begon, M., Diggle, P. J., Serrano, S., Reis, M. G., et al. (2016). A comparative assessment of track plates to quantify fine scale variations in the relative abundance of Norway rats in urban slums. Urban Ecosyst. 19, 561–575. doi: 10.1007/s11252-015-0519-8
Heiberg, A.-C., Sluydts, V., and Leirs, H. (2012). Uncovering the secret lives of sewer rats (Rattus norvegicus): movements, distribution and population dynamics revealed by a capture-mark-recapture study. Wildl. Res. 39, 202–219. doi: 10.1071/WR11149
Himsworth, C. G., Bai, Y., Kosoy, M. Y., Wood, H., DiBernardo, A., Lindsay, R., et al. (2015). An investigation of Bartonella spp., Rickettsia typhi, and Seoul Hantavirus in rats (Rattus spp.) from an inner-city neighborhood of Vancouver, Canada: is pathogen presence a reflection of global and local rat population structure? Vector Borne Zoo. Dis. 15, 21–26. doi: 10.1089/vbz.2014.1657
Himsworth, C. G., Bidulka, J., Parsons, K. L., Feng, A. Y. T., Tang, P., Jardine, C. M., et al. (2013a). Ecology of Leptospira interrogans in Norway rats (Rattus norvegicus) in an inner-city neighborhood of Vancouver, Canada. PLoS Negl. Trop. Dis. 7:e2270. doi: 10.1371/journal.pntd.0002270.s001
Himsworth, C. G., Parsons, K. L., Feng, A. Y. T., Kerr, T., Jardine, C. M., and Patrick, D. M. (2014a). A mixed methods approach to exploring the relationship between Norway rat (Rattus norvegicus) abundance and features of the urban environment in an inner-city neighborhood of Vancouver, Canada. PLoS ONE 9:e97776. doi: 10.1371/journal.pone.0097776.s004
Himsworth, C. G., Parsons, K. L., Jardine, C., and Patrick, D. M. (2013b). Rats, cities, people, and pathogens: a systematic review and narrative synthesis of literature regarding the ecology of rat-associated zoonoses in urban centers. Vector Borne and Zoo. Dis. 13, 349–359. doi: 10.1089/vbz.2012.1195
Himsworth, C. G., Patrick, D. M., Mak, S., Jardine, C. M., Tang, P., and Weese, J. S. (2014b). Carriage of Clostridium difficile by wild urban Norway rats (Rattus norvegicus) and black rats (Rattus rattus). Appl. Environ. Microbiol. 80, 1299–1305. doi: 10.1128/AEM.03609-13
Kajdacsi, B., Costa, F., Hyseni, C., Porter, F., Brown, J., Rodrigues, G., et al. (2013). Urban population genetics of slum-dwelling rats (Rattus norvegicus) in Salvador, Brazil. Mol. Ecol. 22, 5056–5070. doi: 10.1111/mec.12455
King, O. M. (1950). An ecological study of the Norway rat and the house mouse in a city block in Lawrence, Kansas. Trans. Kansas Acad. Sci. 53, 500–528. doi: 10.2307/3625896
Kjellander, P., Hewison, A. J. M., Liberg, O., Angibault, J. M., Bideau, E., and Cargnelutti, B. (2004). Experimental evidence for density-dependence of home-range size in roe deer (Capreolus capreolus L.): a comparison of two long-term studies. Oecologia 139, 478–485. doi: 10.1007/s00442-004-1529-z
Lajeunesse, M. J. (2016). Facilitating systematic reviews, data extraction and meta-analysis with the METAGEAR package for R. Methods Ecol. Evol. 7, 323–330. doi: 10.1111/2041-210X.12472
Lam, R., Byers, K. A., and Himsworth, C. G. (2018). Beyond zoonosis: the mental health impacts of rat exposure on impoverished urban neighborhoods. J. Environ. Health. 81, 8–12.
LaPoint, S., Balkenhol, N., Hale, J., Sadler, J., and van der Ree, R. (2015). Ecological connectivity research in urban areas. Funct. Ecol. 29, 868–878. doi: 10.1111/1365-2435.12489
Lee, M. J., Byers, K. A., Donovan, C. M., Bidulka, J. J., Stephen, C., Patrick, D. M., et al. (2018). Effects of culling on Leptospira interrogans carriage by rats. Emerg. Infect. Dis. 24, 356–360. doi: 10.3201/eid2402.171371
Low, B. W., Mills, H., Algar, D., and Hamilton, N. (2013). Home ranges of introduced rats on Christmas Island: a pilot study. Ecol. Manag. Restor. 14, 41–46. doi: 10.1111/emr.12024
Mangombi, J. B., Brouat, C., Loiseau, A., Banga, O., Leroy, E. M., Bourgarel, M., et al. (2016). Urban population genetics of the invasive black rats in Franceville, Gabon. J. Zool. 299, 183–190. doi: 10.1111/jzo.12334
Moher, D., Liberati, A., Tetzlaff, J., and Altzman, D. G., and The PRISMA Group (2009). Preferred reporting items for systematic reviews and meta-analyses: the prisma statement. PLoS Med. 6:e1000097. doi: 10.1371/journal.pmed.1000097
Moro, D., Byrne, M., Kennedy, M., Campbell, S., and Tizard, M. (2018). Identifying knowledge gaps for gene drive research to control invasive animal species: the next CRISPR step. Global Ecol. Conserv. 13:e00363. doi: 10.1016/j.gecco.2017.e00363
Oyedele, D. T., Sah, S. A. M., Kairuddin, L., Mohd, W., and Ibrahim, M. W. (2015). Range measurement and a habitat suitability map for the Norway rat in a highly developed urban environment. Trop. Life Sci. Res. 26, 27–44.
Parsons, M. B., Gillespie, T. R., Lonsdorf, E. V., Travis, D., Lipende, I., Gilagiza, B., et al. (2014). Global positioning system data-loggers: a tool to quantify fine-scale movement of domestic animals to evaluate potential for zoonotic transmission to an endangered wildlife population. PLoS ONE 9:110984. doi: 10.1371/journal.pone.0110984
Parsons, M. H., Banks, P. B., Deutsch, M. A., Corrigan, R. F., and Munshi-South, J. (2017). Trends in urban rat ecology: a framework to define the prevailing knowledge gaps and incentives for academia, pest management professionals (PMPs) and public health agencies to participate. J. Urban Ecol. 3, 1–8. doi: 10.1093/jue/jux005
Parsons, M. H., Sarno, R. J., and Deutsch, M. A. (2015). Jump-starting urban rat research: conspecific pheromones recruit wild rats into a behavioral and pathogen-monitoring assay. Front. Ecol. Evol. 3:146. doi: 10.3389/fevo.2015.00146
Pawe, C. K., and Saikia, A. (2018). Unplanned urban growth: land use/land cover change in the Guwahati Metropolitan Area, India. Geogr. Tidsskr Den. 118, 88–100. doi: 10.1080/00167223.2017.1405357
Petrie, G. F., and Todd, R. E. (1923). Observations in Upper Egypt on the range of excursion of the house rodents: R. rattus and Acomys cahirinus. Rep. Notes Pub. Hlth. Lab. Cairo 5, 14–18.
Petrie, G. F., Todd, R. E., Skander, R., and Hilmy, F. (1924). A report on plague investigations in Egypt. J. Hyg. 23, 117–150.
Pimentel, D., Zuniga, R., and Morrison, D. (2005). Update on the environmental and economic costs associated with alien-invasive species in the United States. Ecol. Econ. 52, 273–288. doi: 10.1016/j.ecolecon.2004.10.002
Quixabeira-Santos, J. C., Medaglia, M. L. G., Pescador, C. A., and Damaso, C. R. (2011). Animal movement and establishment of vaccinia virus cantagalo strain in amazon biome, Brazil. Emerg. Infect. Dis. 17, 726–729. doi: 10.3201/eid1704.101581
Recht, M. A. (1982). The fine structure of the home range and activity pattern of free-ranging telemetered urban Norway rats, Rattus norvegicus (Berkenhout). Bull. Soc. Vector Ecol. 7, 29–36.
Recht, M. A., Geck, R., and Challet, G. L. (1983). The fine-structure of the home range and activity-phasing of unrestricted telemetered urban roof rats, Rattus rattus in Orange County, California. Bull. Soc. Vector Ecol. 8, 51–64.
Richardson, J. L., Burak, M. K., Hernandez, C., Shirvell, J. M., Mariani, C., Carvalho-Pereira, T. S. A., et al. (2017). Using fine-scale spatial genetics of Norway rats to improve control efforts and reduce Leptospirosis risk in urban slum environments. Evol. Appl. 10, 323–337. doi: 10.1111/eva.12449
Richter, C. P. (1968). Experiences of a reluctant rat-catcher the common Norway rat - friend or enemy? Proc. Amer. Phil. Soc. 112, 403–415.
Robertson, B. C., and Gemmell, N. J. (2004). Defining eradication units to control invasive pests. J. Appl. Ecol. 41, 1042–1048. doi: 10.1111/j.0021-8901.2004.00984.x
Rondinini, C., and Doncaster, C. P. (2002). Roads as barriers to movement for hedgehogs. Funct. Ecol. 16, 504–509. doi: 10.1046/j.1365-2435.2002.00651.x
Safi, K., König, B., and Kerth, G. (2007). Sex differences in population genetics, home range size and habitat use of the parti-colored bat (Vespertilio murinus, Linnaeus 1758) in Switzerland and their consequences for conservation. Biol. Cons. 137, 28–36. doi: 10.1016/j.biocon.2007.01.011
Takahashi, L. K., and Lore, R. K. (1980). Foraging and food hoarding of wild Rattus norvegicus in an urban environment. Behav. Neural Biol. 29, 527–531.
Tanaka, R. (1963). On the problem of trap-response types of small mammal populations. Res. Popul. Ecol. 5, 139–146.
Tanaka, R., and Kawashima, F. (1951). Movement and population of the brown rat in Kochi City. Annot. Zool. Jpn. 24, 225–233.
Taylor, K. D. (1978). Range of movement and activity of common rats (Rattus norvegicus) on agricultural land. J. Appl. Ecol. 15, 663–677. doi: 10.2307/2402767
Tomkiewicz, S. M., Fuller, M. R., Kie, J. G., and Bates, K. K. (2010). Global positioning system and associated technologies in animal behaviour and ecological research. Phil. Trans. R. Soc. B. 365, 2163–2176. doi: 10.1098/rstb.2010.0090
Traweger, D., and Slotta-Bachmayr, L. (2005). Introducing GIS-modelling into the management of a brown rat (Rattus norvegicus Berk.) (Mamm. Rodentia Muridae) population in an urban habitat. J. Pest Sci. 78, 17–24. doi: 10.1007/s10340-004-0062-5
Tucker, M. A., Böhning-Gaese, K., Fagan, W. F., Fryxell, J. M., Van Moorter, B., Alberts, S. C., et al. (2018). Moving in the Anthropocene: global reductions in terrestrial mammalian movements. Science 359, 466–469. doi: 10.1126/science.aam9712
United Nations (2018). World Urbanization Prospects: The 2018 Revision. New York, NY: United Nations.
Volkova, V. V., Howey, R., Savill, N. J., and Woolhouse, M. E. J. (2010). Sheep movement networks and the transmission of infectious diseases. PLoS ONE 5:e11185. doi: 10.1371/journal.pone.0011185
Wiktander, U., Olsson, O., and Nilsson, S. G. (2001). Seasonal variation in home-range size, and habitat area requirement of the lesser spotted woodpecker (Dendrocopos minor) in southern Sweden. Biol. Cons. 100, 387–395. doi: 10.1016/S0006-3207(01)00045-3
Wolff, J. O. (1985). The effects of density, food, and interspecific interference on home range size in Peromyscus leucopus and Peromyscus maniculatus. Can. J. Zool. 63, 2657–2662.
Keywords: dispersal, ecology, home range, immigration, movement, rat, rattus, urban
Citation: Byers KA, Lee MJ, Patrick DM and Himsworth CG (2019) Rats About Town: A Systematic Review of Rat Movement in Urban Ecosystems. Front. Ecol. Evol. 7:13. doi: 10.3389/fevo.2019.00013
Received: 01 September 2018; Accepted: 14 January 2019;
Published: 30 January 2019.
Edited by:
Mathew Samuel Crowther, University of Sydney, AustraliaReviewed by:
Pablo Tortosa, Université de la Réunion, FranceJames Emory Childs, Yale University, United States
Copyright © 2019 Byers, Lee, Patrick and Himsworth. This is an open-access article distributed under the terms of the Creative Commons Attribution License (CC BY). The use, distribution or reproduction in other forums is permitted, provided the original author(s) and the copyright owner(s) are credited and that the original publication in this journal is cited, in accordance with accepted academic practice. No use, distribution or reproduction is permitted which does not comply with these terms.
*Correspondence: Kaylee A. Byers, S2F5bGVlLmJ5ZXJzQHViYy5jYQ==