- 1National Centre for Biological Sciences, Tata Institute of Fundamental Research, Bangalore, India
- 2The Earth Institute, Columbia University, New York, NY, United States
- 3The Nature Conservancy, Arlington, VA, United States
- 4School of Biology, University of Leeds, Leeds, United Kingdom
Two dominant biomes that occur across the southern Indian peninsula are dry deciduous “forests” and evergreen forests, with the former occurring in drier regions and the latter in wetter regions, sometimes in close proximity to each other. Here we compare stem and leaf traits of trees from multiple sites across these biomes to show that dry deciduous “forest” species have, on average, lower height: diameter ratios, lower specific leaf areas, higher wood densities and higher relative bark thickness, than evergreen forest species. These traits are diagnostic of these dry deciduous “forests” as open, well-lit, drought-, and fire-prone habitats where trees are conservative in their growth strategies and invest heavily in protective bark tissue. These tree traits together with the occurrence of a C4 grass-dominated understory, diverse mammalian grazers, and frequent fires indicate that large tracts of dry deciduous “forests” of southern India are more accurately classified as mesic deciduous “savannas.”
Introduction
Ecologists have long organized earth's vegetation into biomes or vegetation types that occur predictably under certain combinations of precipitation and temperature (Schimper, 1903; Holdridge, 1947; Walter, 1973; Whittaker, 1975). However, Whittaker (1975); recognized that there were certain climatic zones where vegetation was unpredictable; this zone of “ecosystems uncertain” includes large parts of the tropics where vegetation can be tropical forest, savanna, shrubland, or grassland, depending on seasonality, drought, and other disturbances (Whittaker, 1975; Bond et al., 2005; Bond, 2013). It is also now widely agreed that while temperature and precipitation together define the major biomes at continental scales, local scale turnover in vegetation types can be driven by sharp differences in underlying topographic and edaphic conditions (Ratter, 1992; Esler et al., 2015; Moncrieff et al., 2016; Miatto and Batalha, 2017) but more often by vegetation-disturbance feedbacks that can result in very different ecosystems within comparable climatic conditions (Bond et al., 2005; Staver et al., 2011; Hoffmann et al., 2012; Dantas Vde et al., 2013, 2016; Charles-Dominique et al., 2015; Pausas and Dantas, 2017).
Across the mesic tropics, where both wooded savannas and forests occur, sometimes in close proximity within a landscape, recent evidence clearly establishes a critical role for vegetation-fire feedbacks in maintaining these savanna-forest transitions (Hoffmann et al., 2012; Dantas Vde et al., 2013; Charles-Dominique et al., 2015; Gray and Bond, 2015). Specifically, open lighted environments and frequent grass-fuelled fires are associated with the savanna state, while closed, shaded environments and rare fires are associated with the forest state (Bond and Parr, 2010; Hoffmann et al., 2012). Reflecting these differences, the woody species that are characteristic of these different ecosystems are expected to vary in their morphological and physiological traits in ways that are adapted to their distinctive disturbance-environment regimes (Ratnam et al., 2011; Hoffmann et al., 2012; Dantas Vde et al., 2013).
Forest trees, which rarely encounter fire and are under selection to out-shade competitors are predicted to grow taller and develop wider canopies for a given diameter, and invest little in protective bark tissue (Ratnam et al., 2011). They may also have higher specific leaf area and lower wood density, which are supportive of rapid nutrient acquisition and growth (Poorter and Bongers, 2006; Chave et al., 2009; Miatto and Batalha, 2017). In contrast, savanna trees that grow in fire-prone environments are expected to invest heavily in thick bark that protects them from fire (Gignoux et al., 1997; Hoffmann et al., 2012; Dantas Vde et al., 2013). They may store much of their biomass in belowground tissue that is safe from fire, and aboveground, they may be shorter and have narrower canopies for a given diameter (Ratnam et al., 2011). They may also be expected to have lower specific leaf area and higher wood densities that support the more conservative growth strategies that are favored in their open and desiccating environments (Miatto and Batalha, 2017).
Here, we consider the functional traits of woody trees across different vegetation types in the southern Indian peninsula. Much of this region falls within the zone of “ecosystems uncertain” in the seasonal tropics (Bond, 2013, Figure 16.1); it supports a diversity of vegetation formations ranging from open thorn scrub to wooded grasslands to closed forests. However, a historical problem with the vegetation nomenclature in this region is that all the vegetation formations with some degree of woody cover are classified as “forests” (see Champion and Seth, 1968; Ratnam et al., 2016). Thus, both open and closed woody formations fall within this nomenclature such that savannas are not distinguished from forests. In past work, we have argued that large tracts of the vegetation type in this region classified as “dry deciduous forest” that are characterized by relatively open tree canopies in grassy understories are more correctly viewed as mesic savannas, while vegetation types with dense tree cover and non-grassy understories are true forest formations (Ratnam et al., 2011, 2016; Sankaran and Ratnam, 2013). Here, we extend these arguments to an examination of the traits of tree species in these habitats. If, as we argue, many of the dry deciduous “forests” in southern India are in fact deciduous “savannas” that are adapted to seasonal water-stress and frequent grass-fuelled fires (Ratnam et al., 2016), which is not the case for true forests, we expect traits of tree species characteristic of these habitats to reflect this difference.
Methods
We sampled dry deciduous and evergreen forests across seven sites in southern India (see inset in Figure 1). Rainfall across our sampling points in dry deciduous forests ranged from 516 to 1,260 mm, while rainfall across sampling points in evergreen forests ranged from 1,078 to 5,660 mm. Rainfall across this entire region is monsoonal such that all sites experience long dry seasons of 5–8 months per year (Figure 1).
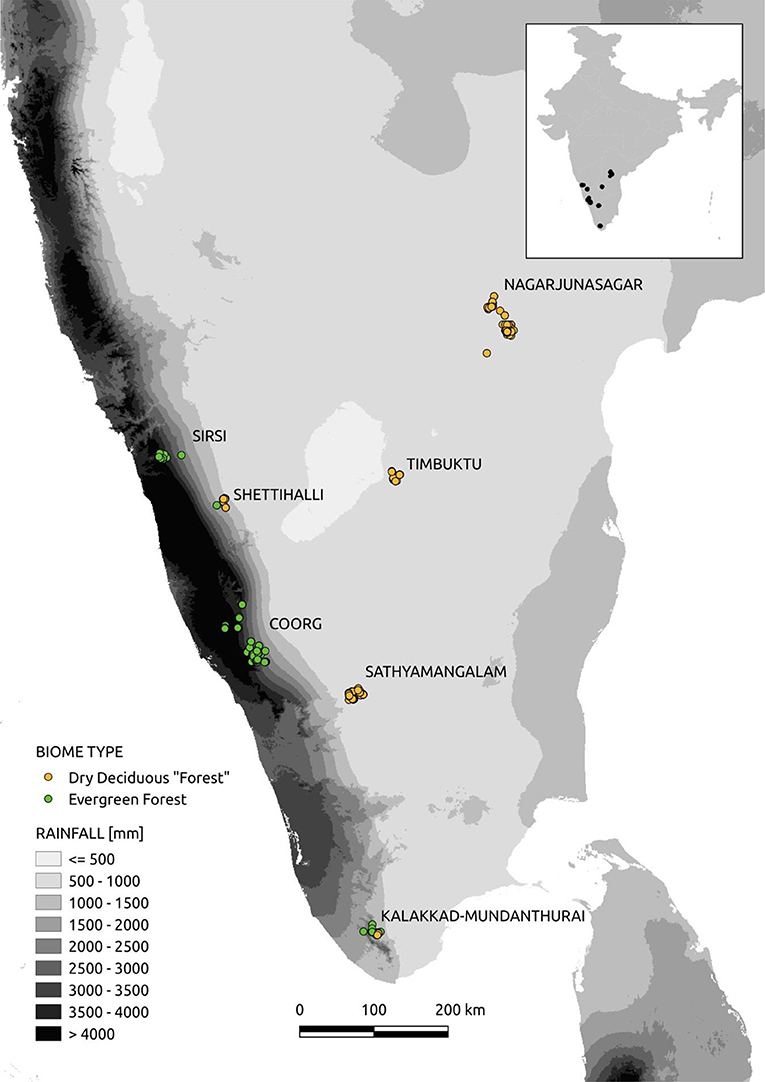
Figure 1. Sampling sites across Southern India. Sites span a rainfall gradient from a little over 500 mm to over 4,000 mm per year. Rainfall is seasonal and all sites have long dry seasons ranging from 5 to 8 months.
We collected functional trait data from 1,350 individual trees of 75 dry deciduous “forest” (DD) species and 92 (EG) evergreen forest species (Supplementary Table 1), with 9 species common to both habitats. Specifically, we measured specific leaf area (SLA), tree height, girth at breast height (GBH), wood density, and bark thickness. For each species, in each habitat, we collected traits from multiple individuals (3–25, depending on the commonness of the species).
For SLA, five mature sun leaves were collected from the canopy of each individual and scanned in a flat-bed scanner on the same day. Leaf areas of scanned leaves were calculated using either Blackspot (Varma and Osuri, 2013) or Image J software (Rasband, 2014). Leaves were then oven-dried and dry weights measured in a precision balance. SLA was calculated as leaf area per unit dry weight. GBH was measured with a tape-measure, 1.3 m above the ground. Tree heights were measured using a clinometer or, alternately, a laser range-finder when the observer could stand at the base of the trunk and sight the top of the tree with the laser beam. Wood cores were collected using an increment borer, and placed into sealed plastic bags with moist cotton. Back in the field station, they rehydrated in water for 1 h. Fresh wood volume was estimated by water displacement, following which samples were oven-dried at 65°C for 72 h and weighed, and wood density estimated as oven-dried weight divided by fresh volume (Chave, 2005). Bark thickness was measured at breast height on the trunk, using a bark gauge. Using the sharp end of the gauge, we gently gouged through the outer layers of bark until it gave way to the thick inner wood which was visibly different tissue, and measured bark thickness to this point. Relative bark thickness was calculated as: (Bark thickness*2/Diameter)*100.
We used Generalized Linear Mixed Models (GLMM) to look at how traits differed between the two vegetation types. Vegetation type (DD, EG) was included as a fixed effect, with species nested within sites as the random effect. Analyses were carried out using the “lme4” package, and the “car” package was used to conduct Type II Wald Chi square tests to assess statistical significance of the fixed effects (Bates et al., 2017). Values for SLA, H:D, and relative bark thickness were log transformed to meet model assumptions.
Results
Consistent with our expectations, dry deciduous “forest” species differed significantly from evergreen forest species for the four traits measured. DD species had, on average, lower height: diameter ratios (Mean ± SE, DD: 0.360 ± 0.01, EG: 0.576 ± 0.017, χ2 = 184.71, df = 1, p < 0.0001) higher relative bark thickness (DD:8.75 ± 0.47%, EG:4.26 ± 0.26%, χ2 = 50.03, df = 1, p < 0.0001), lower SLA (DD: 94.58 ± 2.42 g/cm2, EG:106.31 ± 2.96 g/cm2, χ2 = 5.801, df = 1, p = 0.016), and marginally higher wood densities (DD: 0.619 ± 0.015 g/cm3, EG:0.603 ± 0.011 g/cm3, χ2 = 5.87, df = 1, p = 0.015) than EG species (Figure 2).
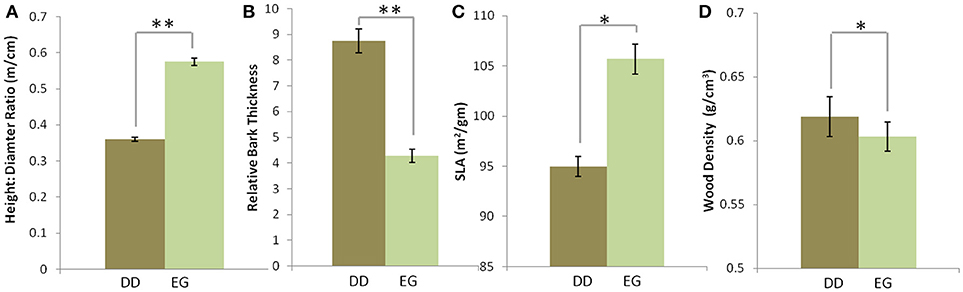
Figure 2. Comparisons of functional traits of trees from dry deciduous “forest” (DD; 79 species) and evergreen forest (EG; 92 species) across seven sites in Southern India. The data shown (Mean ± SE) are for (A) height: diameter ratios, (B) relative bark thickness, (C) specific leaf area, and (D) wood density. Asterisks indicate significant differences between mean trait values in DD and EG habitats (*p < 0.05, **p < 0.001).
Discussion
Our cross-site comparison of the functional traits of tree species from dry deciduous and evergreen forests of southern India indicate that dry deciduous “forests” are fundamentally different in the environments and species traits that they support than are evergreen forests, even though they occur in close proximity in many regions. Dry deciduous “forest” trees have lower height to diameter ratios, produce leaves with lower specific leaf areas, and have higher wood densities. Taken together, this suite of traits is suggestive of slower and more conservative growth strategies in these trees than in their evergreen forest counterparts (Poorter and Bongers, 2006; Chave et al., 2009; Miatto and Batalha, 2017). Such strategies may indicate either relatively lower resource environments, or less competitive ones (Miatto and Batalha, 2017). Clearly, dry deciduous “forests” occur in areas with less rainfall than evergreen forests, although the two forest types overlap at the wetter end of the dry deciduous zone. Seasonal water stress is thus likely to be more pronounced in these habitats. With relatively lower tree densities, dry deciduous “forests” may also be both more desiccating, and less competitive environments for adult trees that grow in them.
Critically, dry deciduous “forest” trees have dramatically higher investment in bark, producing, on average, almost twice as much bark for a given diameter as evergreen forest trees. Thick barks are known to serve multiple protective functions including drought resistance, fire resistance, and prevention of stem damage (Poorter et al., 2014; Schafer et al., 2015). For fire-prone environments, there is overwhelming evidence for a primary role of thick barks in enabling trees to persist through fires (Lawes et al., 2011; Hoffmann et al., 2012; Pausas, 2015; Pellegrini et al., 2017) and several recent analyses across the globe have shown that fire-resistant savanna trees are characterized by thicker bark than fire-sensitive forest trees (Hoffmann et al., 2012; Dantas Vde et al., 2013; Lawes et al., 2013; Pellegrini et al., 2017). Thicker barks may also increase drought resistance in drier environments (Poorter et al., 2014; Schafer et al., 2015) as in these dry deciduous “forests,” but this function may be secondary to a strategy of deciduousness where species avoid drought by being leafless in the dry season.
Several analyses of contemporary fire regimes in the subcontinent confirm that dry deciduous “forests” burn much more frequently (fire return intervals range from 1 to 6 years) and extensively (10–50% of these landscapes burn annually) than do other forest types (Kodandapani et al., 2008; Kodandapani, 2013; Srivastava and Garg, 2013; Mondal and Sukumar, 2016; Reddy et al., 2017). Together with the trait comparisons we report in this study, which are consistent with predicted trait differences between mesic savanna and forest species (Ratnam et al., 2011), these data suggest that large tracts of dry deciduous “forests” in this region, characterized by trees in grass-dominated understories, are in fact deciduous “savannas,” where seasonal water-stress and fire are important drivers. Further, these habitats are also home to an ancient and diverse mammalian herbivore assemblage, ranging from smaller-bodied spotted deer to large-bodied mega-herbivores like the Asian bison and elephant (Bibi and Métais, 2016; Sankaran and Ahrestani, 2016). Large mammalian grazers, which are supported by the forage in grass-dominated understories are characteristic of savannas globally, additional evidence that these “forests” are indeed “savannas.”
These confirmatory findings have important implications for how fire is managed in these ecosystems. At the current time, these habitats are managed under a blanket policy of fire exclusion and suppression and fire-setting is a punishable offense according to the Indian Forest Act (Ratnam et al., 2016; Thekaekara et al., 2017). These policies, which stem from a historical misreading of these ecosystems as “forests” are inimical to the conservation and sustainable management of these savannas (Lehmann and Parr, 2016; Griffith et al., 2017). First, the notion that fires are always undesirable disturbances in these ecosystems, widespread amongst both managers and vegetation scholars, prevents nuanced understanding, and appropriate research on the ecology of these systems. Second, these policies preclude the use of fire as a management tool, an opportunity lost in many areas where fire exclusion has been associated with severe non-native shrub invasions (Sundaram et al., 2012) and associated losses of native biodiversity (Ramaswami and Sukumar, 2011; Sundaram and Hiremath, 2012). Official recognition of these tree-grass ecosystems as drought- and fire-driven mesic savannas will provide multiple opportunities for vital research and effective management.
Author Contributions
JR led and wrote the paper. MS and JR planned the trait comparisons. SKC, SJM, NN, AMO, JR, and MS collected data. All authors commented on the manuscript.
Funding
Funding for this study came from Department of Science and Technology, Government of India (SERB/SR/SO/PS/78/2012), core funds from NCBS-TIFR to MS and Rufford Small Grants Foundation (11086-2) to AMO.
Conflict of Interest Statement
The authors declare that the research was conducted in the absence of any commercial or financial relationships that could be construed as a potential conflict of interest.
Acknowledgments
We thank the Forest Departments of Karnataka, Tamil Nadu, Telangana, and Andhra Pradesh for permits to conduct this study. We thank Dayani Chakaravarthy, Chintan Seth, VijayKumar S, Swapna Nellaballi, and Catherin Arockia for help with data collection and processing.
Supplementary Material
The Supplementary Material for this article can be found online at: https://www.frontiersin.org/articles/10.3389/fevo.2019.00008/full#supplementary-material
References
Bates, D., Maechler, M., Bolker, B., Walker, S., Christensen, R. H. B., Singmann, H., et al. (2017). Package ‘lme4’. Version 1. 1–12. Available online at: https://cran.r-project.org/web/packages/lme4/lme4.pdf
Bibi, F., and Métais, G. (2016). “Evolutionary history of the large herbivores of south and Southeast Asia (Indomalayan Realm),” in The Ecology of Large Herbivores in South and Southeast Asia, eds F. S. Ahrestani and M. Sankaran (Dordrecht: Springer), 15–88.
Bond, W. J. (2013). “Consumer control by megafauna and fire,” in Trophic Cascades: Predators, Prey and the Changing Dynamics of Nature, eds J. Terborgh and A. E. Estes (Washington, DC: Island Press), 275–286.
Bond, W. J., and Parr, C. L. (2010). Beyond the forest edge: ecology, diversity and conservation of the grassy biomes. Biol. Conserv. 143, 2395–2404. doi: 10.1016/j.biocon.2009.12.012
Bond, W. J., Woodward, F. I., and Midgley, G. F. (2005). The global distribution of ecosystems in a world without fire. N. Phytol. 165, 525–538. doi: 10.1111/j.1469-8137.2004.01252.x
Champion, S. H., and Seth, S. K. (1968). A Revised Survey of the Forest Types of India. A Revised Survey of the Forest Types of India. London: Chapman and Hall, 417–429.
Charles-Dominique, T., Staver, A. C., Midgley, G. F., and Bond, W. J. (2015). Functional differentiation of biomes in an African savanna/forest mosaic. South Afr. J. Bot. 101, 82–90. doi: 10.1016/j.sajb.2015.05.005
Chave, J. (2005). Measuring Wood Density for Tropical Forest Trees. Center for Tropical Forest Science, Smithsonian Tropical Research Institute.
Chave, J., Coomes, D., Jansen, S., Lewis, S. L., Swenson, N. G., and Zanne, A. E. (2009). Towards a worldwide wood economics spectrum. Ecol. Lett. 12, 351–366. doi: 10.1111/j.1461-0248.2009.01285.x
Dantas Vde, D. L., Batalha, M. A., and Pausas, J. G. (2013). Fire drives functional thresholds on the savanna–forest transition. Ecology 94, 2454–2463. doi: 10.1890/12-1629.1
Dantas Vde, D. L., Hirota, M., Oliveira, R. S., and Pausas, J. G. (2016). Disturbance maintains alternative biome states. Ecol. Lett. 19, 12–19. doi: 10.1111/ele.12537
Esler, K. J., von Staden, L., and Midgley, G. F. (2015). Determinants of the Fynbos/Succulent Karoo biome boundary: insights from a reciprocal transplant experiment. South Afr. J. Bot. 101, 120–128. doi: 10.1016/j.sajb.2015.02.006
Gignoux, J., Clobert, J., and Menaut, J. C. (1997). Alternative fire resistance strategies in savanna trees. Oecologia 110, 576–583. doi: 10.1007/s004420050198
Gray, E. F., and Bond, W. J. (2015). Soil nutrients in an African forest/savanna mosaic: drivers or driven? South Afr. J. Bot. 101, 66–72. doi: 10.1016/j.sajb.2015.06.003
Griffith, D. M., Lehmann, C. E., Strömberg, C. A., Parr, C. L., Pennington, R. T., Sankaran, M., et al. (2017). Comment on “The extent of forest in dryland biomes”. Science 358:eaao1309. doi: 10.1126/science.aao1309
Hoffmann, W. A., Geiger, E. L., Gotsch, S. G., Rossatto, D. R., Silva, L. C., Lau, O. L., et al. (2012). Ecological thresholds at the savanna-forest boundary: how plant traits, resources and fire govern the distribution of tropical biomes. Ecol. Lett. 15, 759–768. doi: 10.1111/j.1461-0248.2012.01789.x
Holdridge, L. R. (1947). Determination of world plant formations from simple climatic data. Science 105, 267–268. doi: 10.1126/science.105.2727.367
Kodandapani, N. (2013). Contrasting fire regimes in a seasonally dry tropical forest and a savanna ecosystem in the Western Ghats, India. Fire Ecol. 9, 102–115. doi: 10.4996/fireecology.0902102
Kodandapani, N., Cochrane, M. A., and Sukumar, R. (2008). A comparative analysis of spatial, temporal, and ecological characteristics of forest fires in seasonally dry tropical ecosystems in the Western Ghats, India. Forest Ecol. Manag. 256, 607–617. doi: 10.1016/j.foreco.2008.05.006
Lawes, M. J., Midgley, J. J., and Clarke, P. J. (2013). Costs and benefits of relative bark thickness in relation to fire damage: a savanna/forest contrast. J. Ecol. 101, 517–524. doi: 10.1111/1365-2745.12035
Lawes, M. J., Richards, A., Dathe, J., and Midgley, J. J. (2011). Bark thickness determines fire resistance of selected tree species from fire-prone tropical savanna in north Australia. Plant Ecol. 212, 2057–2069. doi: 10.1007/s11258-011-9954-7
Lehmann, C. E., and Parr, C. L. (2016). Tropical grassy biomes: linking ecology, human use and conservation. Philos. Trans. R. Soc. B Biol. Sci. 371:20160329. doi: 10.1098/rstb.2016.0329
Miatto, R. C., and Batalha, M. A. (2017). Are the cerrado and the seasonal forest woody floras assembled by different processes despite their spatial proximity? J. Plant Ecol. 11, 740–750. doi: 10.1093/jpe/rtx044
Moncrieff, G. R., Bond, W. J., and Higgins, S. I. (2016). Revising the biome concept for understanding and predicting global change impacts. J. Biogeogr. 43, 863–873. doi: 10.1111/jbi.12701
Mondal, N., and Sukumar, R. (2016). Fires in seasonally dry tropical forest: testing the varying constraints hypothesis across a regional rainfall gradient. PLoS ONE 11:e0159691. doi: 10.1371/journal.pone.0159691
Pausas, J. G. (2015). Bark thickness and fire regime. Funct. Ecol. 29, 315–327. doi: 10.1111/1365-2435.12372
Pausas, J. G., and Dantas, V. D. L. (2017). Scale matters: fire–vegetation feedbacks are needed to explain tropical tree cover at the local scale. Global Ecol. Biogeogr. 26, 395–399. doi: 10.1111/geb.12562
Pellegrini, A. F., Anderegg, W. R., Paine, C. E., Hoffmann, W. A., Kartzinel, T., Rabin, S. S., et al. (2017). Convergence of bark investment according to fire and climate structures ecosystem vulnerability to future change. Ecol. Lett. 20, 307–316. doi: 10.1111/ele.12725
Poorter, L., and Bongers, F. (2006). Leaf traits are good predictors of plant performance across 53 rain forest species. Ecology 87, 1733–1743. doi: 10.1890/0012-9658(2006)87[1733:LTAGPO]2.0.CO;2
Poorter, L., McNeil, A., Hurtado, V. H., Prins, H. H., and Putz, F. E. (2014). Bark traits and life-history strategies of tropical dry-and moist forest trees. Funct. Ecol. 28, 232–242. doi: 10.1111/1365-2435.12158
Ramaswami, G., and Sukumar, R. (2011). Woody plant seedling distribution under invasive Lantana camara thickets in a dry-forest plot in Mudumalai, southern India. J. Trop. Ecol. 27, 365–373. doi: 10.1017/S0266467411000137
Ratnam, J., Bond, W. J., Fensham, R. J., Hoffmann, W. A., Archibald, S., Lehmann, C. E., et al. (2011). When is a ‘forest’a savanna, and why does it matter? Global Ecol. Biogeogr. 20, 653–660. doi: 10.1111/j.1466-8238.2010.00634.x
Ratnam, J., Tomlinson, K. W., Rasquinha, D. N., and Sankaran, M. (2016). Savannahs of Asia: antiquity, biogeography, and an uncertain future. Philos. Trans. R. Soc. B 371:20150305. doi: 10.1098/rstb.2015.0305
Ratter, J. A. (1992). “Transition between cerrado and forest vegetation in Brazil,” in Nature and Dynamics of Forest-Savanna Boundaries, eds P. A. Furley, J. Proctor, and J. A. Ratter (London: Chapman and Hall), 417–419.
Reddy, C. S., Jha, C. S., Manaswini, G., Alekhya, V. V. L. P., Pasha, S. V., Satish, K. V., et al. (2017). Nationwide assessment of forest burnt area in India using Resourcesat-2 AWiFS data. Curr. Sci. 112, 1521–1532. doi: 10.18520/cs/v112/i07/1521-1532
Sankaran, M., and Ahrestani, F. S. (2016). “The ecology of large herbivores of south and southeast asia: synthesis and future directions,” in The Ecology of Large Herbivores in South and Southeast Asia, eds F. S. Ahrestani and M. Sankaran (Dordrecht: Springer), 237–249.
Sankaran, M., and Ratnam, J. (2013). “African and asian savannas,” in Encyclopedia of Biodiversity, ed S. A. Levin (Cambridge: Academic Press), 58–74.
Schafer, J. L., Breslow, B. P., Hohmann, M. G., and Hoffmann, W. A. (2015). Relative bark thickness is correlated with tree species distributions along a fire frequency gradient. Fire Ecol. 11, 74–87. doi: 10.4996/fireecology.1101074
Srivastava, P., and Garg, A. (2013). Forest fires in India: regional and temporal analyses. J. Trop. Forest Sci. 25, 228–239.
Staver, A. C., Archibald, S., and Levin, S. A. (2011). The global extent and determinants of savanna and forest as alternative biome states. Science 334, 230–232. doi: 10.1126/science.1210465
Sundaram, B., and Hiremath, A. J. (2012). Lantana camara invasion in a heterogeneous landscape: patterns of spread and correlation with changes in native vegetation. Biol. Invas. 14, 1127–1141. doi: 10.1007/s10530-011-0144-2
Sundaram, B., Krishnan, S., Hiremath, A. J., and Joseph, G. (2012). Ecology and impacts of the invasive species, Lantana camara, in a social-ecological system in South India: perspectives from local knowledge. Hum. Ecol. 40, 931–942. doi: 10.1007/s10745-012-9532-1
Thekaekara, T., Vanak, A. T., Hiremath, A. J., Rai, N. D., Ratnam, J., and Sukumar, R. (2017). Notes from the other side of a forest fire. Econ. Politic. Wkly 52, 22–25.
Varma, V., and Osuri, A. M. (2013). Black Spot: a platform for automated and rapid estimation of leaf area from scanned images. Plant Ecol. 214, 1529–1534. doi: 10.1007/s11258-013-0273-z
Keywords: functional traits, fire, savnnas, forests, southern India
Citation: Ratnam J, Chengappa SK, Machado SJ, Nataraj N, Osuri AM and Sankaran M (2019) Functional Traits of Trees From Dry Deciduous “Forests” of Southern India Suggest Seasonal Drought and Fire Are Important Drivers. Front. Ecol. Evol. 7:8. doi: 10.3389/fevo.2019.00008
Received: 01 June 2018; Accepted: 09 January 2019;
Published: 29 January 2019.
Edited by:
Daniel M. Griffith, Oregon State University, United StatesReviewed by:
Juan Ernesto Guevara Andino, Field Museum of Natural History, United StatesKathleen M. Quigley, Michigan State University, United States
Copyright © 2019 Ratnam, Chengappa, Machado, Nataraj, Osuri and Sankaran. This is an open-access article distributed under the terms of the Creative Commons Attribution License (CC BY). The use, distribution or reproduction in other forums is permitted, provided the original author(s) and the copyright owner(s) are credited and that the original publication in this journal is cited, in accordance with accepted academic practice. No use, distribution or reproduction is permitted which does not comply with these terms.
*Correspondence: Jayashree Ratnam, jratnam@ncbs.res.in