- Department of Ecology, Swedish University of Agricultural Sciences (SLU), Uppsala, Sweden
Zoophytophagous (omnivorous) predators provide valuable pest control services, and offer an advantage over strict carnivores as plant-feeding enables survival during prey shortage. This putative advantage can potentially be their downside, as plant-feeding may entail damage that negatively affects plant growth/yield (i.e., the cost arising from of omnivore plant-feeding). Yet, benefits conferred by predatory services are usually thought to counterbalance any impact of plant damage. In this systematic review, our goal was to determine how often levels of omnivore damage and its consequences for plants (costs) are considered or quantified. We provide a synthesis of publication trends and findings on omnivore plant-feeding levels, plant injury variables, actual (if quantified) and potential effects on growth/yield, the type of study (lab, greenhouse) and the plants/omnivores most often examined. Our search revealed that measures of omnivore plant-feeding are occasionally reported, but seldom are the direct consequences of such damage also considered. Omnivore plant-feeding were reported in 57% of studies (53 of 93 full-text examined); within these, the majority (>80%) indicated moderate to high levels of plant-feeding. However, only 22% of reports (15 of 69) quantified the effects of omnivore-inflicted damage on plant performance. Of these 15 reports, a greater number found negative consequences for plants compared to those showing no effect (8 vs. 4; 3 with both), with consequences for yield relative to growth being more often evaluated (6 vs. 2). Overall, fruit/leaf injuries relative to stem/flower-feeding were most often examined, and lab/greenhouse experiments predominated. Tomatoes (Solanum lycopersicum) and the mirid Nesidiocoris tenuis were the most common species studied (34 and 14 reports, respectively). Our results indicate that costs to plants of omnivore-inflicted damage are often neglected. We argue that predatory benefits need to be simultaneously considered with plant-feeding effects to appropriately evaluate pest control services. Publication trends suggest that more studies are evaluating costs to plants, but a paradigm shift is still needed. Furthermore, we found that our understanding of plant-feeding and its effects is disproportionally based on studies examining tomato plants and its omnivorous biocontrol agents. To confirm the generality of findings thus far, other plant omnivore systems should be further considered.
Introduction
Prey and plant-feeding omnivorous arthropods are increasingly being recognized as providers of valuable and effective pest control services (Albajes and Alomar, 1999; Perdikis et al., 2011; Ågren et al., 2012; Zappala et al., 2013; Pérez-Hedo and Urbaneja, 2015; Beitia et al., 2016; van Lenteren et al., 2018a). Several species of omnivorous predators have proven to be key biocontrol agents of economically-important herbivores, for example in tomato, sweet pepper, and willow crops (Dalin et al., 2011; Calvo et al., 2012; Messelink et al., 2015) and show great promise even for potential invading pests (Pérez-Hedo et al., 2017). One of their main advantages as an alternative or complement to other natural enemies, is that plant-feeding enables their establishment and maintenance before pest infestation or during prey shortage. Consequently, the effects associated with the use of plant-food for omnivore performance/preference and prey suppression have received much attention (Naranjo and Gibson, 1996; Coll and Ruberson, 1998; Sanchez et al., 2004; Lundgren, 2009; Stenberg et al., 2011; Maselou et al., 2014; Perdikis and Arvaniti, 2016; Liman et al., 2017). Indeed, plant-feeding has well-documented positive effects on omnivore development, longevity and fecundity (reviewed by Eubanks and Styrsky, 2005), resulting in larger predator populations and stronger herbivore suppression (e.g., Eubanks and Denno, 2000).
On the other hand, direct interaction of omnivorous predators with plants and the consequences of their phytophagy/herbivory for plant performance have received less attention. The predatory services provided by omnivores are often thought to counterbalance any potential plant damage (Coll and Guershon, 2002; Castañé et al., 2011). However, herbivory entails a cost to plants, for example in the form of loss of photosynthetic capacity (Nabity et al., 2008), mobilization/use of resources for damage repair or compensatory responses (e.g., new or larger leaves; Strauss and Agrawal, 1999), or for inducing defenses (chemical, physical etc.; Heil and Baldwin, 2002; Cipollini et al., 2014). In order for the net outcome of omnivore-mediated protection to serve in favor of plants, benefits should outweigh the costs of plant-food use (Heil, 2008). Benefits to plants are those conferred by the decrease in prey numbers resulting from direct predation by omnivores, and the subsequent reduction in pest-inflicted damage. The cost to plants of such predatory services include any direct negative effects arising from omnivore plant-feeding and the damage they inflict. For example, omnivore feeding damage can result in leaf deformations, necrosis and/or fruit abortion, which can in turn affect plant growth and yield (Raman et al., 1984; Castañé et al., 2011; Adar et al., 2015; Bhatt and Patel, 2018). Thus, to evaluate the “net result” (advantageous, neutral or detrimental) of omnivore-mediated plant protection, assessment of both plant benefits and costs is necessary. Yet, predatory benefits are seldom simultaneously considered with the direct consequences of omnivore-inflicted damage to plants (Puentes and Björkman, 2017). Indirect assessment of costs to plants are common and involve descriptions of crop injury, omnivore feeding preferences, or are even dismissed if there are no obvious signs of plant damage (Castañé et al., 2011; Hamdi et al., 2013; Adar et al., 2015). Thus, costs to plants have often been considered of lesser or negligible importance relative to the predatory services provided by omnivorous predators (Dalin et al., 2011; Bhatt and Patel, 2018).
Accounting for the consequences of plant-feeding is critical given that omnivorous predators can exhibit a diversity of habits ranging from zoophytophagous (mostly prey food, occasional plant-feeding) to phytozoophagous (mostly plant food complemented with prey). For instance, for several zoophytophagous biocontrol agents, plant-feeding does not always decrease when prey is abundant; indicating that phytophagy may be obligatory rather than facultative (Gillespie and McGregor, 2000; Castañé et al., 2011; Aubry et al., 2017). Even within conspecific populations can there be considerable genetic variation in diet preference, with some genotypes specializing on plant resources and others relying mostly on prey (Dumont et al., 2017). Other recent studies have also shown that omnivore plant-feeding can result in similar effects as herbivore damage (Puentes and Björkman, 2017) and even induce plant defenses, thus affecting subsequent interactions (Pappas et al., 2015; Naselli et al., 2016; Bouagga et al., 2018a). Therefore, to minimize risks and maximize benefits of services provided by omnivorous predators, a direct evaluation of costs to plants is necessary.
What evidence is there available for evaluating the extent of plant damage caused by omnivorous predators and its consequences for plant performance? To date, only a review by Castañé et al. (2011) has partly addressed this important question. Their review focuses on reported levels and types of damage to vegetable crops caused by four zoophytophagous species of mirid predators. While some studies reporting the consequences of plant-feeding are mentioned, the review is centered on the circumstances (e.g., predator-prey ratios, predator developmental stage, and stylet morphology) resulting in crop damage and its potential economic (yield loss) consequences. We thus, lack a synthesis and evaluation of the evidence that is available for assessing the direct impact on plants of omnivore-inflicted damage.
In this systematic review, our goal was to compile and summarize findings on the levels of plant-feeding by omnivorous predators and the consequences (costs) for plants. We herein refer to plant costs or cost to plants as any direct effect on their performance (growth, reproduction, crop yield) arising from omnivore plant-feeding. Costs can be null if no effect on plant performance is found. More specifically, we addressed how often levels of omnivore damage and costs to plants are considered or quantified, the plant parts consumed or fed upon by omnivores and the accompanying plant damage variables measured, the actual (if quantified) and potential effects on plant performance of such damage, the type of study (lab, greenhouse, field, etc.) and the plant-omnivore combination for which this information is most commonly reported. In addition, we present overall publication trends for papers studying omnivorous predators, and conduct a comparison within this search of the number of studies reporting, or not, measures of plant-feeding or damage by omnivores. Our systematic review, together with an overview of publication trends, will allow us to determine the extent of neglect of plant costs and examine if their assumed minor importance relative to predatory benefits is supported. Note that our review does not aim to evaluate the net result of simultaneously considering omnivore-provided plant protection services and omnivore-inflicted plant damage. Thus, we do not answer whether the overall outcome of biocontrol is positive, negative or neutral for specific plant-omnivore systems. Our systematic review examines the available literature on direct costs of omnivore phytophagy, but we do not quantitatively compare these results to previously known or estimated benefits for specific omnivore species.
Methods
Literature Search
We performed a systematic literature search, following the steps outlined by Khan et al. (2003), to evaluate the evidence available for determining how much plant damage is usually inflicted by omnivorous predators and its consequences for plant performance. We used Clarivate Analytics' Web of Science platform, and searched in the Web of Science™ core collection using the “field = topic” search field, which searches for terms in the title, abstract or keywords. The document type was limited to Article and Review, all languages, using their Science Citation Index Expanded (SCI-EXPANDED, 1945-present). We were interested in finding publications that examined any form of plant feeding/damage/injury by omnivorous predators, and that potentially examined the consequences of such damage. Thus, the following terms were used in our search:
[(“omnivor*” OR “zoophytophag*” OR “phytozoophag” OR “prey- and plant-feed*” OR “plant- and prey-feed*” OR “plant-feeding predator*” OR “plant and prey” OR “prey and plant” OR “plant bug*” OR “omnivor* predator” OR “omnivor* bodyguard” OR “omnivor* arthropod*” OR “omniv* pest*”) AND (“plant damage” OR “damage to plant*” OR “crop damage*” OR “plant-feeding” OR “plant injur*” OR “injury” OR “damage” OR “phytophagy” OR “plant food” OR “sucking” OR “pierc*” OR “sap-sucking” OR “cost to plant*” OR “plant lesion*” OR “negative effect on plant*” OR “plant performance” OR “plant fitness”)].
The search was refined by excluding several Web of Science categories (see “Supplementary material”) and it was last updated on July 10th, 2018. The process yielded 381 papers to be screened for relevance based on title and abstract (conducted by the author AP). Studies that qualified as relevant were those that indicated that some form of plant-feeding, damage or injury by one or several omnivorous predators had been measured. Studies examining phytophagous/herbivorous insects (even phytophagous plant bugs, e.g., Lygus spp.), ant-plant mutualisms, and other non-arthropod species (e.g., wild boars) were excluded. After this process, 76 of the 381 papers remained for full-text scrutiny. While conducting full-text evaluation of these 76 studies (conducted by all authors), we found additional references within these studies that were relevant to our question. A total of 16 additional publications were added to our original search results. During the review process of the paper, a reviewer suggested an additional article for inclusion in the review. Thus, we conducted full-text examination for a total of 93 papers (see Flow diagram; Figure 1). After evaluation of full-texts, 40 studies were excluded since these did not actually measure any form of plant-feeding or damage. Many of these studies presented an omnivorous predator perspective, for example examining preferences for certain plant-emitted odors, or comparing performance when fed prey- vs. plant-food (see “Supplementary material” for a detailed list of full-text examined studies).
Summary and Classification of Relevant Studies
The remaining 53 studies (Figure 1) were organized in a summary table (Table 1) that included Omnivore species (with Order and Family), Study type (Lab, Field, Greenhouse, Growth chamber), Plant species (with common name), Plant damage variable which was used in the study to evaluate the extent of omnivore plant damage (e.g., leaf or fruit injury), Plant-feeding level by the omnivorous predator reported in the study (Zero to High), Actual (potential) effect on plant (Actual effect if reported by study; Potential: evaluation conducted by authors of potential effect based on damage variable and extent of omnivore-plant feeding) and Reference (authors, year). A more detailed explanation of some of the classification variables follows.
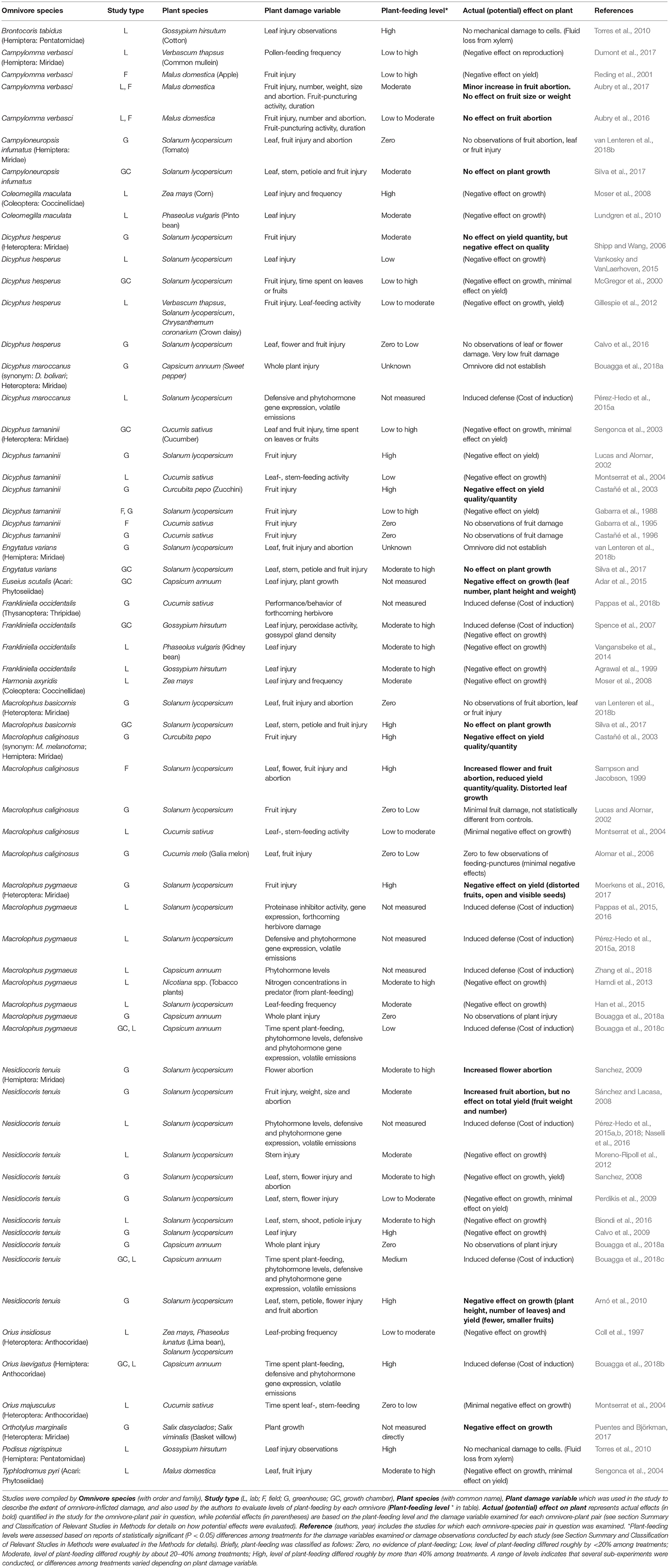
Table 1. Summary of results from 53 studies examining the extent of plant-feeding by omnivorous predators and the actual or potential effect of damage on plant performance.
The summary table category Plant damage variable comprised any measure of plant-feeding or damage by the omnivore. For example, fruit or leaf injury in the form of number of necrotic rings, punctures, scars, or leaf area damaged. Fruit injury also implied dimples, pits, deformations, open fruits or number of fruits injured. Frequency of leaf or fruit-feeding indicated how often predators probed or fed on plant tissue, or how many individuals engaged in this behavior. Time spent on these activities referred to how much of the omnivore activity budget was dedicated to plant feeding. In Table 1, plant damage variables were expressed in a concise form, while in Table S1 a more detailed description of how damage was measured can be found.
The summary table category Plant-feeding level was evaluated by the authors based on actual reports within each study of statistically significant (P < 0.05) differences among treatments for the damage variables examined or damage observations conducted. For example, Calvo et al. (2009) examined the cumulative number of necrotic rings on tomato leaves across 15 weeks, and found statistically significant differences among treatments with densities of 0, 1 and 4 Nesidiocoris tenuis individuals. Differences in number of necrotic rings among treatments were large (over 40% for all treatment comparisons) with greater densities leading to more rings; thus, omnivore plant-feeding level was expressed as high for this study. Our classification of plant-feeding level was based on the differences in damage reported by each study, and ranged from zero to high using the following criteria: Zero, no evidence of plant-feeding; Low, level of plant-feeding differed roughly by < 20% among treatments (e.g., plant-feeding frequency in the presence or absence of prey, time spent feeding on the plant vs. other activities, number of leaf/fruit injuries compared to controls); Moderate: level of plant-feeding differed roughly by about 20–40% among treatments; High, level of plant-feeding differed roughly by more than 40% among treatments. In some instances, several sub-experiments were conducted with different omnivorous species (i.e., more than one specific omnivore-plant species pair per study), or differences in levels of damage among treatments varied depending on plant damage variable (i.e., one omnivore-plant pair can offer multiple reports of an effect on a plant trait); thus, a range of plant-feeding levels are reported were appropriate. It is also important to note that many studies were not directly designed for assessing levels of omnivore plant damage, and our evaluation provides an indication of how much feeding can occur based on the treatments/experimental setting used in each study. We offer suggestions on how to design experiments aimed specifically at examining levels of omnivore plant-feeding and its consequences in section Future prospects.
The summary table category Actual (potential) effect on plant is comprised first of any actual effect on plant growth (e.g., reductions in total height, shoot growth, leaf numbers) and yield quality (fruit blemishes) or quantity (fruit numbers or weight) reported by the study. If no actual effect was reported by the study, we conducted an evaluation of potential effects on plant growth or yield based on the damage variable examined and extent of omnivore-plant feeding reported for each case. For example, if an omnivore fed on flowers or fruits at very high levels, there could potentially be negative effects for reproduction or crop yield. Levels of omnivore damage, and whether it was a reproductive or a trait affecting growth, were used to provide a suggestion for potential consequences of such plant-feeding. For studies examining induction of plant defenses following omnivore-plant feeding, a potential effect for the plant was indicated as a “Cost of induction.” Inducing defenses can involve resources being diverted or allocated away from other functions such as growth, and instead invested in defense. However, the cost of such resource allocation can range from low to high, and varies depending on the plant trait and species (Heil and Baldwin, 2002; Cipollini et al., 2014; Züst and Agrawal, 2017). Our evaluation of costs are meant as suggestions of potential cost to the plants, and these require further investigation. We also included information on omnivorous predator feeding mode (e.g., pierce-sucking, chewing), and prey species provided in the study, but chose to present a simplified version of the table in the main manuscript. See Table S1 for a more detailed classification of each study.
Publication Trends
To examine if consideration of plant costs has changed through time, we conducted a comparison of number of publications per year between studies examining omnivorous predators only, and those also considering some form of plant-feeding/damage. From the search described above, we extracted the number of papers per year resulting from the first level search. In other words, those with only synonyms of omnivores (before the AND connector). We then proceeded to run again the nested second level search (after the AND connector, those with synonyms for plant damage) to also extract number of publications per years, and compared results from the two searches. Secondly, we conducted a comparison of publications per year for those studies found to be potentially relevant to our question (93 studies, full-text examined) and those actually included in the systematic review (53 studies). For both comparisons, the number of studies were log transformed in order to illustrate proportional relationships.
Results
Our systematic search yielded 381 publications that included terms associated with “omnivorous predator” and “plant-feeding” or “plant damage.” A total of 93 papers were full-text examined and 53 of these remained after this process (Figure 1). We used these 53 papers to address how often the extent of plant-feeding by omnivorous predators and its consequences for plants are considered or quantified.
Levels of Omnivore Plant-Feeding, Effects on Plants and Most Common Species
Among the 53 studies examined, some papers presented more than one omnivore-plant relationship (each is considered a report, and hereafter referred to as such) and some examined more than one plant damage variable (hereafter referred to as cases). Thus, sums of reports (69 omnivore-plant reports in total; sum of rows in Table S1) and sums of cases (79 cases examining a specific type of plant variable, excluding “Unknown” in Plant feeding level; Table S1) exceeded the total number of studies. Among the 69 reports, two of the studied omnivore species did not successfully establish (Plant feeding level: “Unknown”; Table 1). For 13 additional reports, no plant-feeding/damage variable was directly examined (Plant feeding level: “Not measured”; Table 1), but plants were exposed to omnivores and allowed to inflict damage. Of the remaining 54 reports, 6 recorded no observations of plant damage, while 13 recorded high levels of omnivore plant-feeding (Plant-feeding level: “Zero” and “High,” respectively; Table 1, Figure 2A). When considering all levels, the majority (31 out of 54) reported at least moderate levels of plant-feeding (Table 1).
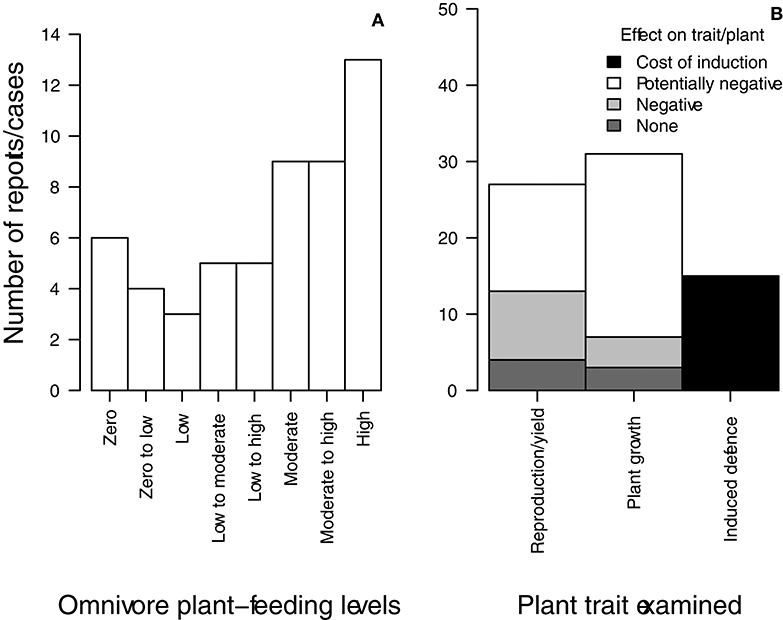
Figure 2. (A) Frequency of reports in 53 studies, which recorded plant-feeding by omnivorous predators ranging in levels from Zero to High (see Table 1; “Unknown” and “Not measured” were excluded). (B) Frequency of cases in 53 studies for which none, actual or potential effects (None, Negative, and Potentially negative in figure) were recorded or estimated for Plant growth and Reproduction/yield, and for which a potential Cost of Induction for Induced defense traits might occur and needs to be quantified (see Methods section Summary and Classification of Relevant Studies and Results section Levels of Omnivore Plant-Feeding, Effects on Plants and Most Common Species for details on the classification of reports). Only cases reporting damage are included (i.e., “Zero” and “Unknown” plant-feeding cases were excluded; Table 1).
Among those reports exposing plants to omnivores and reporting non-zero plant-feeding, only 15 times were the consequences of damage in terms of growth or yield actually measured (Actual effects in bold; Table 1). Of these 15 reports, a greater number found negative consequences for plants compared to those reporting a lack of effect (8 and 4 reports, respectively and 3 reports with both; Table 1). Of these 8 reporting detrimental effects, 6 found negative effects on yield quantity or quality, while 2 report negative effects on growth (Table 1).
In terms of the plant damage variables examined, we found both direct and indirect measures of plant-feeding. Indirect measures included time spent probing or plant-feeding by the omnivore, and frequency of individuals engaging in such behavior (Table S1). Direct measures included different forms of plant injury, with feeding punctures and necrotic rings often quantified, while amount of tissue area damaged was rarely estimated (Table S1). Overall, the majority of cases evaluated fruit or leaf injuries relative to stem- or flower-feeding (Table 1). But among those cases quantifying negative effects on plants (Actual effects in bold; Table 1), examination of fruit injuries were most common relative to leaf injuries (Table 1).
It is important to note that our search results included several cases that examined the consequences of omnivore plant-feeding for plant defense induction against herbivores. A total of 15 cases examined induction of different defense traits, subsequent performance or preference of herbivores and parasitoid attraction (Actual effect: “Induced defense”; Table 1; Figure 2B). These papers do not directly assess the effects on plant growth or yield, but do show that omnivore feeding induces defenses and this could entail a possible cost to plants (Potential effect: “Cost of induction;” Table 1). The cost of inducing defenses against herbivores, however, can vary depending on the plant trait induced (chemical, physical, etc.) and the species in question (Heil and Baldwin, 2002; Cipollini et al., 2014; Züst and Agrawal, 2017). The presence or absence of costs following mirid-mediated induction require actual estimation. Plant-damage variables examined in these papers often included volatile emissions and phytohormone profiling/activity (Table 1). For the remaining cases that did not directly estimate consequences for plants nor induced defense, we also assessed the potential effects of damage on growth and/or yield (Potential effect in parentheses; Table 1). Our evaluation was based on the damage variable examined and extent of omnivore-plant feeding reported for each case (see section Summary and Classification of Relevant Studies), but require actual quantification.
All 69 reports were organized according to whether the study examined traits associated with “Plant growth” (e.g., leaf/stem injuries or feeding, reduced plant height), “Reproduction/yield” (e.g., flower/fruit abortion, fruit distortion, fruit number), or “Induced defense” (e.g., proteinase inhibitor expression, volatile emissions) (Plant trait examined column; Table S1). Based on this classification, we conducted a comparison of the number of cases reporting an actual lack of effect (“None”; Figure 2B), and an actual negative (reported by the study) or potentially negative (evaluated by the authors) on these plant traits (“Negative” and “Potentially negative”; Figure 2B). We found that for both Reproduction/yield and Plant growth, more cases show detrimental rather than no effects on such traits (light gray “Negative” bars vs. dark gray “None” bars, Figure 2B). Also, a greater number of cases evaluated actual effects for Reproduction/yield traits than for Plant growth traits (comparison of dark gray “None” bars between types of traits, Figure 2B). We identified a total of 38 cases with potential negative effects on plant traits (white “Potentially negative” bars, Figure 2B; Table 1); i.e., those excluding “Zero” in the Plant-feeding level column (6 cases; Table S1) as well as those that examined “Induced defense” (15 cases; Table S1) or “Actual effects” (20 cases; Table S1). Among these cases where our evaluation indicated potential negative consequences, plant growth traits were mostly examined relative to reproduction/yield traits (comparison of white “Potentially negative” bars between types of traits, Figure 2B).
Furthermore, a total of 17 out 31 reports presented omnivore feeding for damage variables related to plant growth (e.g., stem injury, leaf area damaged), while 8 out 31 reports for variables associated with reproduction/yield (e.g., fruit-feeding punctures), and 6 out of 31 reported on variables affecting both growth and reproduction (Table 1).
Tomato plants (Solanum lycopersicum) and the omnivorous predator N. tenuis were the most common species studied, with 34 and 14 reports (out of 69) examining these species, respectively (Figures 3A,B). Cucumber plants (Cucumis sativus) and mirids in the genus Macrolophus and Dicyphus were the next most common species studied (Figures 3A,B). Levels of plant-feeding for those omnivore species with more than one occurrence were not consistent among cases, and ranged from zero to high (Omnivore species and Plant-feeding levels; Table 1). Among these omnivore species, plant-feeding was examined on several plant species, except for N. tenuis which was almost exclusively examined on tomatoes (Omnivore and Plant species; Table 1). For those studies quantifying the consequences of damage to plants (15 instances, in bold; Table 1), N. tenuis and Macrolophus spp. plant-feeding often resulted in detrimental effects. Plant-feeding by less-represented species, such as Campylomma verbasci and Engytatus varians, resulted in no negative effects to plants (Actual effects in bold; Table 1). Again, tomato plants were over-represented among these 15 cases, with a few instances finding negative consequences for zucchini and sweet pepper plants (Plant species and Actual effects; Table 1).
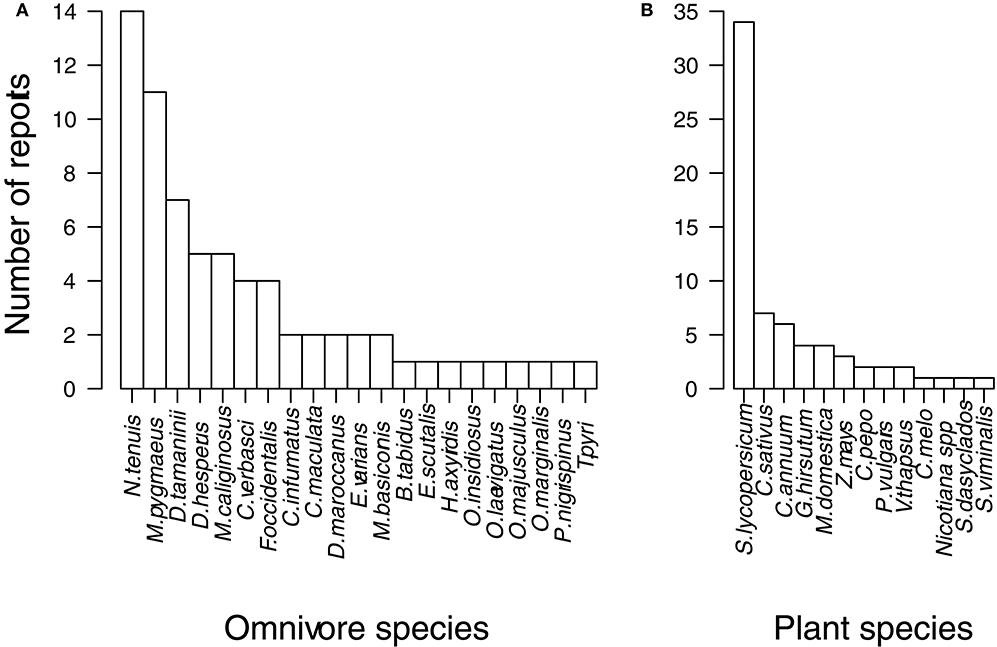
Figure 3. Frequency of reports in 53 studies, which recorded plant-feeding by omnivorous predators, classified according to the (A) Omnivore species and (B) Plant species studied. See Table 1 for full species names.
For each of the 53 studies examined, one or several experiment types (Greenhouse, Field, Growth chamber, or Lab) with omnivores were reported. Lab and greenhouse experiments predominated with 34 and 25 occurrences respectively across all reports, while field studies were few (6 cases, Table 1).
Publication Trends for Studies Considering Plant-Feeding/Damage by Omnivores
Our search on studies examining omnivores without consideration for plant-feeding/damage (See Publication trends in Methods) yielded a total of 3,913 papers between 1945 to present. The number of papers published shows an increasing trend with years (Figure 4, green line). Very few records were found before the 1960s, but a steep increase can be observed from those years onward. Our search for studies examining some form of plant-feeding by omnivores (nested in the search above, see section Publication trends), yielded 381 records. A growing number of studies appear to have been considering plant damage by omnivores (Figure 4A, red line), but few papers did so before the mid to late 1990s. The smoothers used for the lines indicate that studies considering damage have been increasing proportionally to studies addressing only omnivores (Figure 4A).
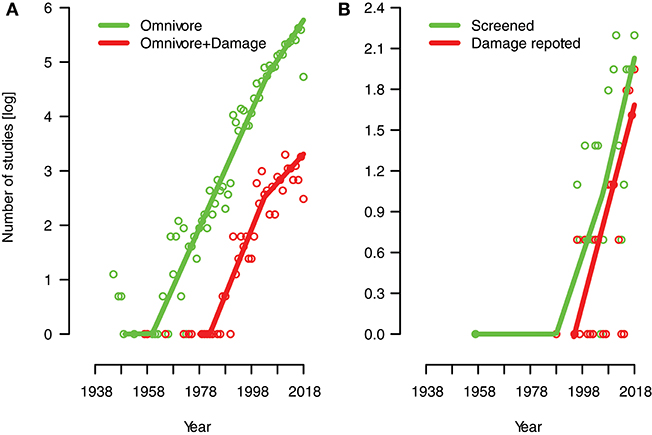
Figure 4. Publication trends (1945-present) for studies examining omnivorous predators without and with consideration for their plant-feeding/damage based on Web of Science systematic searches (see section Publication Trends in Methods). The number of publications per year (log-scale) is presented for (A) the 3,913 studies that were found using several search terms synonymous to omnivorous predators (Omnivore, green line), and those that additionally included terms for plant-feeding/damage (subset of 381 studies; Omnivore + Damage, red line). From these 381 studies, the number of publications per year are presented for (B) the 93 studies for which we conducted full-text screening (Screened, green line), and the 53 studies that reported damage levels to the plant (subset of studies, Damage reported, red line; see Table 1). Lines show additive quantile regression smothers and circles are jittered slightly to increase visibility.
For those 93 studies that we conducted full-text examination of, the publication years ranged from 1957 to 2018 (Figure 4B, green line). Few studies were published before the mid-1990s, and most studies were published in the 2000s. For the 53 studies that we selected, which reported omnivore plant-feeding levels and/or consequences of damage to plants, the range of publication year was 1988–2018 (Table 1; Figure 4B, red line). Of these 53 studies, only 7 were published before the year 2000 (Table 1), indicating that a consideration of omnivore plant damage and its consequence for plants has occurred in more recent years.
Discussion
Summary of Main Findings
Our systematic review addressed how often consequences for plants arising directly from the phytophagy of omnivorous predators, and subsequently affecting plant performance, are considered and/or quantified. We provide a synthesis of the available evidence for assessing plant costs of omnivore-inflicted damage in relation to reductions in plant growth or reproduction/yield. Our search revealed that while some measure of plant-feeding is occasionally reported by studies examining omnivorous predators, it is seldom that the direct consequences of such damage for plants are also considered. Actual measures of plant-feeding levels by omnivores were reported in 57% of studies examined (53 of 93 full-text screened studies). Within these, 24 and 57% of the reports showed high or at least moderate levels of plant-feeding by omnivores, respectively (Table 1; Figure 2A). However, in only 22% of instances were effects of omnivore-inflicted damage on plant performance quantified (15 out of 69 reports, Table 1; Figure 2B). Of these 15 reports, a greater number found negative consequences for plants compared to those reporting a lack of effect (8 and 4 reports, respectively and 3 reports with both; Table 1), with effects on yield quantity/quality being more often evaluated than effects on growth (Table 1; Figure 2B). The effects of omnivore plant-feeding described in these cases suggest that dismissal of plant costs without quantification is not warranted.
We also found that the majority of cases evaluated fruit or leaf injuries relative to stem- or flower-feeding, and among those cases quantifying negative effects on plants, assessment of fruit injuries were most common relative to leaf injuries (Table 1). Lab and greenhouse experiments predominated across all reports, while field studies were few (Table 1). Furthermore, we found a strong bias in the literature toward tomato plants and omnivorous predator species in the mirid family (Nesidiocoris, Macrolophus, Dicyphus spp.) used for biocontrol of tomato pests (Figures 3A,B). Hence, the evidence available for evaluating the extent of plant-feeding damage and its effects, lacks representation from a variety of plant-omnivore systems. Our examination of publication trends, however, suggests an increasing awareness of omnivore plant-feeding effects with more recent papers directly addressing this issue. We hope this indicates an ongoing or upcoming paradigm shift, and simultaneous consideration of benefits and costs of omnivorous predatory services becomes standard practice. Below, we discuss our findings in more detail and provide suggestions for future work.
Extent of Plant-Feeding by Omnivorous Predators
Our systematic search results showed that that the degree of plant-feeding by omnivorous predators is reported every so often, with its quantification being done directly or indirectly in different ways. Out of the 93 studies we screened, 57% provided some measure of how much plant-feeding the omnivore in question engaged in. The selected 53 studies (Table 1) often had an omnivore perspective, examining feeding preferences based on different plant vs. food-prey availabilities, and its effect on pest control or omnivore performance. Nonetheless, a few studies (~1/5 of papers) did set out to directly quantify phytophagy or plant damage by the omnivore in question. For example, phytophagy by the mirid C. verbasci on apples (e.g., Aubry et al., 2016), damage by N. tenuis to tomatoes (e.g., Calvo et al., 2009; Arnó et al., 2010), and damage by the predatory mite Euseius scutalis to sweet peppers (Adar et al., 2015). To measure plant-feeding, a range of different variables were reported, but leaf- and fruit-feeding in contrast to stem- or flower-feeding, were most often evaluated (Table 1). Indirect measures included time spent probing or plant-feeding, and frequency of individuals engaging in such behavior (e.g., Coll et al., 1997; Montserrat et al., 2004; Han et al., 2015). Direct measures included different forms of plant injury, with feeding punctures and necrotic rings often quantified (Table S1), while amount of tissue area damaged/consumed was rarely estimated (e.g., Moser et al., 2008; Lundgren et al., 2010; Vangansbeke et al., 2014; Table S1). It, thus, appears as if less effort has been placed on understanding the plant-omnivore interaction, as exemplified by fewer studies examining actual amounts of plant damage relative to crop quality variables. Overall, these results reflect the traditional greater interest in examining consequences of plant-feeding for omnivore performance or predatory services, rather than its effect on plants.
In addition to the damage variables examined, we also evaluated the levels of plant-feeding reported in each study and classified them between zero to high (Table 1; Figure 2A). Only a small proportion of cases reported zero plant-feeding, relative to the number of studies reporting low to high levels of plant-feeding (Figure 2A). Except for one study (van Lenteren et al., 2018b; Table 1), those reporting zero observations of damage were evaluating predatory services of the omnivores in question and not specifically examining plant injury. Ideally, observations of “no damage” should be confirmed by studies aimed at evaluating plant-feeding, and corroborated several times before being considered general. Indeed, among those zero-damage papers, two separate studies report the mirid Dicyphus tamaninii as inflicting no damage to cucumber plants (Table 1). However, when examining all 7 reports of plant-feeding by D. tamaninii, we can see that 4 of them actually report high levels (Table 1). This inconsistency in the levels of plant-feeding reported is, in fact, observed for all the omnivore species with more than one occurrence in the table (except for Frankliniella occidentalis; Table 1). Thus, no particular omnivorous predator stands out as frequently inflicting low or high levels of damage.
A lack of consistency in degree of plant-feeding can be explained, in some cases, by the plant species examined. For D. tamaninii, high levels of plant damage occur in tomato and zucchinis, yet for other species like Macrolophus caliginous reports of damage vary even within the same plant (Table 1). Another plausible reason for such variation is differences among studies, not only in terms of experimental conditions, but also in omnivore population origin, prey presence/absence and predator:prey ratios. For instance, it has been shown that even within conspecific populations, omnivorous predators can vary genetically in their zoophytophagous vs. phytozoophagous status (Dumont et al., 2017). Even if the majority of studies were lab/greenhouse experiments, authors do report natural infestations or naturally-collected individuals for lab rearing, thus population origin could be important in explaining study variation across different locations. With regard to prey presence/absence, we do summarize whether or not, and which prey was offered to the omnivorous predator for each case (Table S1). However, as our main question addresses the consideration and estimation of plant costs, we were not directly interested in evaluating differences among studies with respect to the prey offered. Castañé et al. (2011) provide an excellent discussion on plant-feeding damage relative to predator:prey ratios at least for D. tamaninii, D. hesperus, M. pygmaeus, and N. tenuis.
Effects of Omnivore Plant-Feeding on Plant Performance (Costs to Plants)
In order to determine whether predatory benefits of omnivorous predators counterbalance plant damage, cost to plants arising from the damage inflicted should be known (Heil, 2008). Such costs encompass any negative effects stemming from omnivore plant-feeding, such as decreases in growth, reproduction or yield due for example to leaf deformations, fruit or flower abortion (Castañé et al., 2011). Our results reveal that such costs have been poorly studied, as they are to a large extent neglected when examining plant-feeding by omnivores. Of those reports evaluating plant-feeding/damage, only 22% quantified the actual consequences of damage to plants (Table 1). Among the cases within these reports, the majority show detrimental effects relative to no effect for different plant traits (Figure 2B). A greater number of these cases evaluated effects on yield quality or quantity, while few examined effects on growth (Table 1). As discussed for findings on plant damage variables, this likely reflects a greater focus on examining effects stemming from omnivore prey consumption relative to those effects stemming from the direct plant-omnivore interaction. Of the 15 reports evaluating plant costs, 4 cases reported no effect on yield and/or growth (Table 1). A lack of negative effects was found even when omnivore plant-feeding levels were estimated as moderate to high (e.g., Silva et al., 2017; Table 1), suggesting that costs cannot be assumed based on plant-feeding levels. However, these cases included species for which our search yielded only one or two instances reporting plant-feeding, such as E. varians, M. basicornis, and Campyloneuropsis infumatus. Thus, it is not possible to determine whether these species often inflict high levels of damage and the generality of non-detrimental effects for plants.
Even though they were few, the studies finding actual negative consequences for plants revealed that yield and growth can be significantly affected by omnivore plant-feeding. Increases in flower or fruit abortion, and reduced height and leaf number were reported in cases examining effects on yield and growth, respectively (Table 1). Consequences for growth were little evaluated, but clearly deserve more attention, especially if leaf area/number reductions are common as this can negatively affect the photosynthetic capacity of plants (e.g., Wisdom et al., 1989; Delaney and Higley, 2006). Among these studies reporting costs (i.e., those finding a negative effect on reproduction/growth; excluding those finding no effect), 5 different plant species were represented (sweet pepper, apple, willows, tomato, and zucchini), but 6 out of 11 reports evaluated effects on tomato plants. Likewise, a total of 7 different omnivore species, all in the Miridae except for E. scutalis in the Phytoseiidae, were represented. Yet, N. tenuis and Macrolophus spp., common biocontrol agents of tomato pests, were used in 6 out of 11 reports (Table 1). Reports of negative plant effects for N. tenuis, and for other mirid species are perhaps not as surprising, given that they have traditionally varied in status as pests or predators (Lu et al., 2010; McColl et al., 2011; Pérez-Hedo and Urbaneja, 2016). However, this bias in the literature makes it difficult to assess generality of results and importance of actual plant costs for other species.
Our evaluation of potential plant consequences (Potential effects in parentheses; Table 1) following omnivore plant-feeding, points out that detrimental effects could be expected for other important crops (e.g., cotton, corn) and non-mirid species. Furthermore, among these studies, we found that omnivore plant-feeding was mostly reported for plant damage variables potentially affecting plant growth relative to those affecting reproduction/yield (“Potentially negative” white bars, Reproduction/yield vs. Plant growth, Figure 2B). This is in contrast to actual reports of negative effects for plants, where reproduction/yield variables were in majority (“Negative” gray bars, Reproduction/yield vs. Plant growth, Figure 2B). While these studies do not directly quantify the negative consequences of omnivore plant-feeding, they do provide valuable insight into possible costs based on the range of plant damage variables examined. For instance, some studies provide detailed estimates of omnivore damage in the form of number and size of leaf- and fruit-feeding punctures (Sengonca et al., 2004; Vangansbeke et al., 2014), amount of leaf-tissue damaged (Moser et al., 2008), necrotic rings on stems and flower clusters (Sanchez, 2008), among others (Table S1). These studies indicate that there is a large potential for negative effects to occur from omnivore plant-feeding, however, whether or not these are manifested needs to be empirically investigated. We hope that our findings encourage actual quantification of direct plant costs for these cases.
Conclusions
So, overall, what does the evidence say about the direct effects of omnivore plant-feeding? The evidence, so far, says that the impact of omnivore plant damage can be substantial and the importance of costs to plants relative to predatory benefits should not be neglected. A reduction in herbivore numbers is of course desirable, of interest and should be examined from a biocontrol perspective. However, with the little evidence available on costs to plants we will be unable to appropriately evaluate if omnivore-provided services often serve (or not) in favor of plants. Even if costs are found to be null or low, such knowledge is valuable and necessary, and we argue that costs to plants should be increasingly considered together with the predatory benefits provided by omnivores. Furthermore, not only is available evidence scarce, but it is strongly overrepresented by studies on tomato plants and omnivorous mirid predators. These results likely mirror the large efforts placed in finding pesticide-free pest management strategies in tomatoes, and in understanding the predatory services provided by different mirid species in this system (e.g., Urbaneja et al., 2012; Pérez-Hedo and Urbaneja, 2016). This research has greatly advanced our knowledge on the net outcome of biocontrol services and laid the foundation for work in other systems. Nonetheless, this bias creates a confounding factor when examining literature on effects of omnivore plant-feeding, as search results are largely skewed toward specific species. This should, thus, be considered when interpreting results from our systematic review.
Future Prospects
Despite a generally low consideration of costs to plants in the literature, publications trends did indicate a more recent awareness of omnivore plant-feeding and its consequences for plants. Indeed, we noticed that several recent papers highlighted some of the knowledge gaps documented by our systematic review (e.g., Hamdi et al., 2013; Aubry et al., 2016; Silva et al., 2017; van Lenteren et al., 2018b) and we hope this is indicative of an ongoing paradigm shift. We, thus, make a timely call for future studies to include a more explicit quantification of costs to plants, and to avoid assuming that they are outweighed by benefits.
Our systematic search also yielded several very recent papers examining the effects of omnivore plant-feeding on induction of plant defenses (Pérez-Hedo et al., 2015b, 2018; Bouagga et al., 2018c; Pappas et al., 2018b; Zhang et al., 2018). Results from these studies present new exciting avenues for increasing the value of omnivorous predators as biocontrol agents. It seems they are able to provide more than predatory services, as their previous plant-feeding can reduce subsequent herbivore performance and even attract other natural enemies (Pappas et al., 2015; Pérez-Hedo et al., 2015a,b). Furthermore, they appear to perceive differential volatile emissions from plants colonized vs. non-colonized by beneficial defense-enhancing endophytic fungi; thus, enabling stronger plant protection as both direct and indirect defenses can be utilized (Pappas et al., 2018a). These novel findings, however, have not addressed any potential costs arising from defense induction (Table 1). That is, costs in terms of resources being diverted away from other functions (such as growth) and invested in defense following omnivore plant-feeding. Studies examining induced defenses after herbivory have shown that resource allocation costs can range from low to high, and vary depending on plant species and trait (Züst and Agrawal, 2017). We thus, recommend greater consideration of such costs to plants in future studies addressing induced defense.
To study costs to plants associated with omnivore plant-feeding, we suggest that studies include several treatments with or without predators, in the absence and presence of prey, and ideally several plant damage (leaf, stem, flower, fruit injuries) and performance (growth, reproduction) variables should be evaluated. Such treatments could include: (1) Control group with plants receiving no damage; (2) Plants with an omnivorous predator that is allowed to reside but not feed on plants (e.g., impairing stylets/mandibles); (3) Plants with an omnivorous predator allowed to feed on plants. These treatments can be replicated both in the absence and presence of prey (e.g., impaired and unimpaired omnivore alone on plant, or together with insect pest as prey). Examination of additional indirect effects resulting from omnivore feeding, for example on parasitoids, could also be included.
Lastly, future efforts should also be aimed at examining a wider range of omnivore-plant systems. In order to be able to make more general conclusions, we need to broaden the base of knowledge on which our current understanding of costs to plants relies on. Perhaps with the exception of N. tenuis on tomato plants, our search results indicate that we cannot conduct complete net effect evaluations (benefits vs. costs) of the pest control services provided by many important omnivorous predators. We strongly believe that it is time to catch-up with the mirid-tomato bias.
Author Contributions
AP, JS, and CB conceived and designed the questions addressed in the systematic review, as well as the search strategy employed both for the review and publication trends. AP conducted the systematic searches and selection of studies to be scrutinized. JS and CB also conducted full-text examination and classification of the selected studies. JS analyzed trends. AP wrote the manuscript following discussion of results with JS and CB, which also provided input during revisions.
Conflict of Interest Statement
The authors declare that the research was conducted in the absence of any commercial or financial relationships that could be construed as a potential conflict of interest.
Supplementary Material
The Supplementary Material for this article can be found online at: https://www.frontiersin.org/articles/10.3389/fevo.2018.00218/full#supplementary-material
References
Ågren, G. I., Stenberg, J. A., and Björkman, C. (2012). Omnivores as plant bodyguards—A model of the importance of plant quality. Basic Appl. Ecol. 13, 441–448. doi: 10.1016/j.baae.2012.07.005
Adar, E., Inbar, M., Gal, S., Issman, L., and Palevsky, E. (2015). Plant cell piercing by a predatory mite: evidence and implications. Exp. Appl. Acarol. 65, 181–193. doi: 10.1007/s10493-014-9860-5
Agrawal, A. A., Kobayashi, C., and Thaler, J. S. (1999). Influence of prey availability and induced host-plant resistance on omnivory by western flower thrips. Ecology 80, 518–523. doi: 10.1890/0012-9658(1999)080[0518:IOPAAI]2.0.CO;2
Albajes, R., and Alomar, Ò. (1999). “Current and potential use of polyphagous predators,” in Integrated Pest and Disease Management in Greenhouse Crops, eds R. Albajes, M. Lodovica Gullino, J. C. van Lenteren, Y. Elad (Dordrecht: Springer), 265–275. Available online at: https://link.springer.com/book/10.1007/0-306-47585-5
Alomar, O., Riudavets, J., and Castañe, C. (2006). Macrolophus caliginosus in the biological control of Bemisia tabaci on greenhouse melons. Biol. Control 36, 154–162. doi: 10.1016/j.biocontrol.2005.08.010
Arnó, J., Castane, C., Riudavets, J., and Gabarra, R. (2010). Risk of damage to tomato crops by the generalist zoophytophagous predator Nesidiocoris tenuis (Reuter) (Hemiptera: Miridae). Bull. Entomol. Res. 100, 105–115. doi: 10.1017/S0007485309006841
Aubry, O., Cormier, D., Chouinard, G., and Lucas, E. (2016). Phytophagy by the mullein bug (Hemiptera: Miridae) on apples: feeding behavior and fruit damage. J. Econ. Entomol. 109, 2463–2471. doi: 10.1093/jee/tow209
Aubry, O., Cormier, D., Chouinard, G., and Lucas, E. (2017). Influence of extraguild prey and intraguild predators on the phytophagy of the zoophytophagous bug Campylomma verbasci. J. Pest Sci. 90, 287–297. doi: 10.1007/s10340-016-0765-4
Beitia, F., Asís, J. D., De Pedro, L., Goula, M., and Tormos, J. (2016). Importance of feeding behaviour on life cycle in the zoophytophagous bug Dicyphus geniculatus. Bull. Insectol. 69, 173–180.
Bhatt, N. A., and Patel, M. V. (2018). Tomato bug Nesidiocoris tenuis (Reuter): a zoophytophagous insect. J. Entomol. Zool. Stud. 6, 1550–1556. doi: 10.13140/RG.2.2.22183.19361
Biondi, A., Zappalà, L., Di Mauro, A., Tropea Garzia, G., Russo, A., Desneux, N., et al. (2016). Can alternative host plant and prey affect phytophagy and biological control by the zoophytophagous mirid Nesidiocoris tenuis? Biocontrol 61, 79–90. doi: 10.1007/s10526-015-9700-5
Bouagga, S., Urbaneja, A., and Pérez-Hedo, M. (2018a). Comparative biocontrol potential of three predatory mirids when preying on sweet pepper key pests. Biol. Control 121, 168–174. doi: 10.1016/j.biocontrol.2018.03.003
Bouagga, S., Urbaneja, A., Rambla, J. L., Flors, V., Granell, A., Jaques, J. A., et al. (2018c). Zoophytophagous mirids provide pest control by inducing direct defences, antixenosis and attraction to parasitoids in sweet pepper plants. Pest Manag. Sci. 74, 1286–1296. doi: 10.1002/ps.4838
Bouagga, S., Urbaneja, A., Rambla, J. L., Granell, A., and Pérez-Hedo, M. (2018b). Orius laevigatus strengthens its role as a biological control agent by inducing plant defenses. J. Pest Sci. 91, 55–64. doi: 10.1007/s10340-017-0886-4
Calvo, F. J., Lorente, M. J., Stansly, P. A., and Belda, J. E. (2012). Preplant release of Nesidiocoris tenuis and supplementary tactics for control of Tuta absoluta and Bemisa tabaci in greenhouse tomato. Entomol. Exp. Appl. 143, 111–119. doi: 10.1111/j.1570-7458.2012.01238.x
Calvo, F. J., Torres-Ruiz, A., Velazquez-Gonzalez, J. C., Rodriguez-Leyva, E., and Lomeli-Flores, J. R. (2016). Evaluation of Dicyphus hesperus for biological control of sweet potato whitefly and potato psyllid on greenhouse tomato. Biocontrol 61, 415–424. doi: 10.1007/s10526-016-9719-2
Calvo, J., Blockmans, K., Stansly, P. A., and Urbaneja, A. (2009). Predation by Nesidiocoris tenuis on Bemisia tabaci and injury to tomato. Biocontrol 54, 237–246. doi: 10.1007/s10526-008-9164-y
Castañé, C., Alomar, O., and Riudavets, J. (1996). Management of western flower thrips on cucumber with Dicyphus tamaninii (Heteroptera: Miridae). Biol. Control 7, 114–120. doi: 10.1006/bcon.1996.0073
Castañé, C., Alomar, O., and Riudavets, J. (2003). Potential risk of damage to zucchinis caused by mirid bugs. IOBC wprs Bull. 26, 135–138.
Castañé, C., Arn,ó, J., Gabarra, R., and Alomar, O. (2011). Plant damage to vegetable crops by zoophytophagous mirid predators. Biol. Control 59, 22–29. doi: 10.1016/j.biocontrol.2011.03.007
Cipollini, D., Walters, D., and Voelckel, C. (2014). “Costs of resistance in plants: from theory to evidence,” in Annual Plant Reviews Book Series: Insect-Plant Interactions Vol. 47, eds C. Voelckel, and G. Jander (Chichester: Wiley), 263–337.
Coll, M., and Guershon, M. (2002). Omnivory in terrestrial arthropods: mixing plant and prey diets. Annu. Rev. Entomol. 47, 267–297. doi: 10.1146/annurev.ento.47.091201.145209
Coll, M., and Ruberson, J. R. (1998). Predatory Heteroptera: Their Ecology and Use in Biological Control. Lanham, MD: Thomas Say Publications, Entomological Society of America.
Coll, M., Smith, L. A., and Ridgway, R. L. (1997). Effect of plants on the searching efficiency of a generalist predator: the importance of predator-prey spatial association. Entomol. Exp. Appl. 83, 1–10. doi: 10.1046/j.1570-7458.1997.00151.x
Dalin, P., Demoly, T., Kabir, M. F., and Björkman, C. (2011). Global land-use change and the importance of zoophytophagous bugs in biological control: coppicing willows as a timely example. Biol. Control 59, 6–12. doi: 10.1016/j.biocontrol.2011.01.010
Delaney, K. J., and Higley, L. G. (2006). An insect countermeasure impacts plant physiology: midrib vein cutting, defoliation and leaf photosynthesis. Plant Cell Environ. 29, 1245–1258. doi: 10.1111/j.1365-3040.2006.01504.x
Dumont, F., Lucas, E., and Réale, D. (2017). Coexistence of zoophytophagous and phytozoophagous strategies linked to genotypic diet specialization in plant bug. PLoS ONE 12:e0176369. doi: 10.1371/journal.pone.0176369
Eubanks, M. D., and Denno, R. F. (2000). Host plants mediate omnivore–herbivore interactions and influence prey suppression. Ecology 81, 936–947. doi: 10.1890/0012-9658(2000)081[0936:HPMOHI]2.0.CO;2
Eubanks, M. D., and Styrsky, J. D. (2005). “Effects of plant feeding on the performance of omnivorous ‘predators',”in Plant-Provided Food for Carnivorous Insects, eds. F. L. Wackers, P. C. J. van Rijn, and J. Bruin (Cambridge: Cambridge University Press), 148–177.
Gabarra, R., Castane, C., and Albajes, R. (1995). The mirid bug Dicyphus tamaninii as a greenhouse whitefly and western flower thrips predator on cucumber. Biocontrol Sci. Technol. 5, 475–488. doi: 10.1080/09583159550039666
Gabarra, R., Castañé, C., Bordas, E., and Albajes, R. (1988). Dicyphus tamaninii as a beneficial insect and pest in tomato crops in Catalonia, Spain. Entomophaga 33, 219–228. doi: 10.1007/BF02372657
Gillespie, D. R., and McGregor, R. R. (2000). The functions of plant feeding in the omnivorous predator Dicyphus hesperus: water places limits on predation. Ecol. Entomol. 25, 380–386. doi: 10.1046/j.1365-2311.2000.00285.x
Gillespie, D. R., VanLaerhoven, S. L., McGregor, R. R., Chan, S., and Roitberg, B. D. (2012). Plant feeding in an omnivorous mirid, Dicyphus hesperus: why plant context matters. Psyche A J. Entomol. 2012, 1–12. doi: 10.1155/2012/495805
Hamdi, F., Chadoeuf, J., and Bonato, O. (2013). Functional relationships between plant feeding and prey feeding for a zoophytophagous bug. Physiol. Entomol. 38, 241–245. doi: 10.1111/phen.12028
Han, P., Dong, Y. C., Lavoir, A. V., Adamowicz, S., Bearez, P., Wajnberg, E., et al. (2015). Effect of plant nitrogen and water status on the foraging behavior and fitness of an omnivorous arthropod. Ecol. Evol. 5, 5468–5477. doi: 10.1002/ece3.1788
Heil, M. (2008). Indirect defence via tritrophic interactions. New Phytol. 178, 41–61. doi: 10.1111/j.1469-8137.2007.02330.x
Heil, M., and Baldwin, I. T. (2002). Fitness costs of induced resistance: emerging experimental support for a slippery concept. Trends Plant Sci. 7, 61–67. doi: 10.1016/S1360-1385(01)02186-0
Khan, K. S., Kunz, R., Kleijnen, J., and Antes, G. (2003). Five steps to conducting a systematic review. J. R. Soc. Med. 96, 118–121. doi: 10.1177/014107680309600304
Liman, A. S., Dalin, P., and Björkman, C. (2017). Enhanced leaf nitrogen status stabilizes omnivore population density. Oecologia 183, 57–65. doi: 10.1007/s00442-016-3742-y
Lu, Y., Wu, K., Jiang, Y., Xia, B., Li, P., Feng, H., et al. (2010). mirid bug outbreaks in multiple crops correlated with wide-scale adoption of Bt cotton in China. Science 328, 1151–1154. doi: 10.1126/science.1187881
Lucas, E., and Alomar, O. (2002). Impact of Macrolophus caliginosus presence on damage production by Dicyphus tamaninii (Heteroptera : Miridae) on tomato fruits. J. Econ. Entomol. 95, 1123–1129. doi: 10.1603/0022-0493-95.6.1123
Lundgren, J. G. (2009). Relationships of Natural Enemies and Non-prey Foods. Vol. 7. Dordrecht: Springer Science and Business Media; Springer Verlag.
Lundgren, J. G., Moser, S. E., Hellmich, R. L., and Seagraves, M. P. (2010). The effects of diet on herbivory by a predaceous lady beetle. Biocontrol Sci. Technol. 21, 71–74. doi: 10.1080/09583157.2010.524917
Maselou, D. A., Perdikis, D. C., Sabelis, M. W., and Fantinou, A. A. (2014). Use of plant resources by an omnivorous predator and the consequences for effective predation. Biol. Control 79, 92–100. doi: 10.1016/j.biocontrol.2014.09.002
McColl, S. A., Khan, M., and Umina, P. A. (2011). Review of the biology and control of Creontiades dilutus (Stål) (Hemiptera: Miridae). Aust. J. Entomol. 50, 107–117. doi: 10.1111/j.1440-6055.2010.00800.x
McGregor, R. R., Gillespie, D. R., Park, C. G., Quiring, D. M. J., and Foisy, M. R. J. (2000). Leaves or fruit? The potential for damage to tomato fruits by the omnivorous predator, Dicyphus hesperus. Entomol. Exp. Appl. 95, 325–328. doi: 10.1046/j.1570-7458.2000.00671.x
Messelink, G. J., Bloemhard, C. M. J., Hoogerbrugge, H., Van Schelt, J., Ingegno, B. L., and Tavella, L. (2015). Evaluation of mirid predatory bugs and release strategy for aphid control in sweet pepper. J. Appl. Entomol. 139, 333–341. doi: 10.1111/jen.12170
Moerkens, R., Berckmoes, E., Van Damme, V., Ortega-Parra, N., Hanssen, I., Wuytack, M., et al. (2016). High population densities of Macrolophus pygmaeus on tomato plants can cause economic fruit damage: interaction with Pepino mosaic virus? Pest Manag. Sci. 72, 1350–1358. doi: 10.1002/ps.4159
Moerkens, R., Berckmoes, E., Van Damme, V., Wittemans, L., Tirry, L., Casteels, H., et al. (2017). Inoculative release strategies of Macrolophus pygmaeus Rambur (Hemiptera: Miridae) in tomato crops: population dynamics and dispersal. J. Plant Dis. Prot. 124, 295–303. doi: 10.1007/s41348-017-0077-9
Montserrat, M., and Albajes, R. (2004). Behavioral responses of three plant-inhabiting predators to different prey densities. Biol. Control 30, 256–264. doi: 10.1016/j.biocontrol.2004.01.006
Moreno-Ripoll, R., Agusti, N., Berruezo, R., and Gabarra, R. (2012). Conspecific and heterospecific interactions between two omnivorous predators on tomato. Biol. Control 62, 189–196. doi: 10.1016/j.biocontrol.2012.04.005
Moser, S. E., Harwood, J. D., and Obrycki, J. J. (2008). Larval feeding on Bt hybrid and non-Bt corn seedlings by Harmonia axyridis (Coleoptera : Coccinellidae) and Coleomegilla maculata (Coleoptera : Coccinellidae). Environ. Entomol. 37, 525–533. doi: 10.1603/0046-225X(2008)37[525:LFOBHA]2.0.CO;2
Nabity, P. D., Zavala, J. A., and DeLucia, E. H. (2008). Indirect suppression of photosynthesis on individual leaves by arthropod herbivory. Ann. Bot. 103, 655–663. doi: 10.1093/aob/mcn127
Naranjo, S. E., and Gibson, R. L. (1996). Phytophagy in predaceous Heteroptera: effects on life history and population dynamics. in Zoophytophagous Heteroptera: Implications For Life History and Integrated Pest Management, eds O. Alomar, and R. N. Wiedenmann (Annapolis, MD: Thomas Say publications in Entomology; Entomological Society of America), 57–93. Available online at: https://www.entsoc.org/Pubs/Books/Thomas_Say
Naselli, M., Urbaneja, A., Siscaro, G., Jaques, J., Zappalà, L., Flors, V., et al. (2016). Stage-related defense response induction in tomato plants by nesidiocoris tenuis. Int. J. Mol. Sci. 17:1210. doi: 10.3390/ijms17081210
Pappas, M. L., Liapoura, M., Papantoniou, D., Avramidou, M., Kavroulakis, N., Weinhold, A., et al. (2018a). The beneficial endophytic fungus Fusarium solani strain K alters tomato responses against spider mites to the benefit of the plant. Front. Plant Sci. 9:1603. doi: 10.3389/fpls.2018.01603
Pappas, M. L., Steppuhn, A., and Broufas, G. D. (2016). The role of phytophagy by predators in shaping plant interactions with their pests. Commun. Integr. Biol. 9:e0127251. doi: 10.1080/19420889.2016.1145320
Pappas, M. L., Steppuhn, A., Geuss, D., Topalidou, N., Zografou, A., Sabelis, M. W., et al. (2015). Beyond predation: the zoophytophagous predator Macrolophus pygmaeus induces tomato resistance against spider mites. PLoS ONE 10:e0127251. doi: 10.1371/journal.pone.0127251
Pappas, M. L., Tavlaki, G., Triantafyllou, A., and Broufas, G. (2018b). Omnivore-herbivore interactions: thrips and whiteflies compete via the shared host plant. Sci. Rep. 8:3996. doi: 10.1038/s41598-018-22353-2
Perdikis, D., and Arvaniti, K. (2016). Nymphal development on plant vs. leaf with and without prey for two omnivorous predators: Nesidiocoris tenuis (Reuter, 1895)(Hemiptera: Miridae) and Dicyphus errans (Wolff, 1804)(Hemiptera: Miridae). Entomol. Gen. 35, 297–306. doi: 10.1127/entomologia/2016/0219
Perdikis, D., Fantinou, A., and Garantonakis, N. (2009). Studies on the damage potential of the predator Nesidiocoris tenuis on tomato plants. Bull. Insectol. 62, 41–46.
Perdikis, D., Fantinou, A., and Lykouressis, D. (2011). Enhancing pest control in annual crops by conservation of predatory Heteroptera. Biol. Control 59, 13–21. doi: 10.1016/j.biocontrol.2011.03.014
Pérez-Hedo, M., Bouagga, S., Jaques, J. A., Flors, V., and Urbaneja, A. (2015a). Tomato plant responses to feeding behavior of three zoophytophagous predators (Hemiptera: Miridae). Biol. Control 86, 46–51. doi: 10.1016/j.biocontrol.2015.04.006
Pérez-Hedo, M., Rambla, J. L., Granell, A., and Urbaneja, A. (2018). Biological activity and specificity of Miridae-induced plant volatiles. Biocontrol 63, 203–213. doi: 10.1007/s10526-017-9854-4
Pérez-Hedo, M., Suay, R., Alonso, M., Ruocco, M., Giorgini, M., Poncet, C., et al. (2017). Resilience and robustness of IPM in protected horticulture in the face of potential invasive pests. Crop Protection 97, 119–127. doi: 10.1016/j.cropro.2016.11.001
Pérez-Hedo, M., and Urbaneja, A. (2015). Prospects for predatory mirid bugs as biocontrol agents of aphids in sweet peppers. J. Pest Sci. 88, 65–73. doi: 10.1007/s10340-014-0587-1
Pérez-Hedo, M., and Urbaneja, A. (2016). “The zoophytophagous predator Nesidiocoris tenuis: a successful but controversial biocontrol agent in tomato crops,” in Advances in Insect Control and Resistance Management, eds A. Horowitz, and I. Ishaaya (Cham:Springer), 121–138.
Pérez-Hedo, M., Urbaneja-Bernat, P., Jaques, J. A., Flors, V., and Urbaneja, A. (2015b). Defensive plant responses induced by Nesidiocoris tenuis (Hemiptera: Miridae) on tomato plants. J. Pest Sci. 88, 543–554. doi: 10.1007/s10340-014-0640-0
Puentes, A., and Björkman, C. (2017). Costs and benefits of omnivore-mediated plant protection: effects of plant-feeding on Salix growth more detrimental than expected. Oecologia 184, 485–496. doi: 10.1007/s00442-017-3878-4
Raman, K., Sanjayan, K. P., and Suresh, G. (1984). Impact of feeding injury of Cyrtopeltis tenuis Reut.(Hemiptera: Miridae) on some biochemical changes in Lycopersicon esculentum Mill.(Solanaceae). Curr. Sci. 53, 1092–1093.
Reding, M. E., Beers, E. H., Brunner, J. F., and Dunley, J. E. (2001). Influence of timing and prey availability on fruit damage to apple by Campylomma verbasci (Hemiptera: Miridae). J. Econ. Entomol. 94, 33–38. doi: 10.1603/0022-0493-94.1.33
Sampson, C., and Jacobson, R. J. (1999). Macrolophus caliginosus wagner (Heteroptera: Miridae): a predator causing damage to UK tomatoes. IOBC/WPRS Bull. 22, 213–316.
Sanchez, J. A. (2008). Zoophytophagy in the plantbug Nesidiocoris tenuis. Agric. For. Entomol. 10, 75–80. doi: 10.1111/j.1461-9563.2007.00357.x
Sanchez, J. A. (2009). Density thresholds for Nesidiocoris tenuis (Heteroptera: Miridae) in tomato crops. Biol. Control 51, 493–498. doi: 10.1016/j.biocontrol.2009.09.006
Sanchez, J. A., Gillespie, D. R., and McGregor, R. R. (2004). Plant preference in relation to life history traits in the zoophytophagous predator Dicyphus hesperus. Entomol. Exp. Appl. 112, 7–19. doi: 10.1111/j.0013-8703.2004.00174.x
Sánchez, J. A., and Lacasa, A. (2008). Impact of the zoophytophagous plant bug Nesidiocoris tenuis (Heteroptera: Miridae) on tomato yield. J. Econ. Entomol. 101, 1864–1870. doi: 10.1603/0022-0493-101.6.1864
Sengonca, C., Khan, I. A., and Blaeser, P. (2004). The predatory mite Typhlodromus pyri (Acari: Phytoseiidae) causes feeding scars on leaves and fruits of apple. Exp. Appl. Acarol. 33, 45–53. doi: 10.1023/B:APPA.0000029965.47111.f3
Sengonca, C., Saleh, A., and Blaeser, P. (2003). Investigations on the potential damage caused to cucumber fruit by the polyphagous predatory bug Dicyphus tamaninii Wagner (Heteroptera : Miridae) under different nutritional conditions. Z. Pflanzenkr. Pflanzenschutz - J. Plant Dis. Prot. 110, 59–65.
Shipp, J. L., and Wang, K. (2006). Evaluation of Dicyphus hersperus (Heteroptera: Miridae) for biological control of Frankliniella occidentalis (Thysanoptera: Thripidae) on greenhouse tomato. J. Econ. Entomol. 99, 414–420. doi: 10.1093/jee/99.2.414
Silva, D. B., Bueno, V. H. P., Calvo, F. J., and van Lenteren, J. C. (2017). Do nymphs and adults of three neotropical zoophytophagous mirids damage leaves and fruits of tomato? Bull. Entomol. Res. 107, 200–207. doi: 10.1017/S0007485316000778
Spence, K. O., Bicocca, V. T., and Rosenheim, J. A. (2007). Friend or foe?: A plant's induced response to an omnivore. Environ. Entomol. 36, 623–630. doi: 10.1603/0046-225X(2007)36[623:FOFAPI]2.0.CO;2
Stenberg, J. A., Lehrman, A., and Björkman, C. (2011). Plant defence: feeding your bodyguards can be counter-productive. Basic Appl. Ecol. 12, 629–633. doi: 10.1016/j.baae.2011.08.007
Strauss, S. Y., and Agrawal, A. A. (1999). The ecology and evolution of plant tolerance to herbivory. Trends Ecol. Evol. 14, 179–185. doi: 10.1016/S0169-5347(98)01576-6
Torres, J. B., Barros, E. M., Coelho, R. R., and Pimentel, R. M. M. (2010). Zoophytophagous pentatomids feeding on plants and implications for biological control. Arthropod. Plant. Interact. 4, 219–227. doi: 10.1007/s11829-010-9095-2
Urbaneja, A., González-Cabrera, J., Arnó, J., and Gabarra, R. (2012). Prospects for the biological control of Tuta absoluta in tomatoes of the Mediterranean basin. Pest Manag. Sci. 68, 1215–1222. doi: 10.1002/ps.3344
van Lenteren, J. C., Bolckmans, K., Köhl, J., Ravensberg, W. J., and Urbaneja, A. (2018a). Biological control using invertebrates and microorganisms: plenty of new opportunities. BioControl 63, 39–59. doi: 10.1007/s10526-017-9801-4
van Lenteren, J. C., Bueno, V. H. P., Calvo, F. J., Calixto, A. M., and Montes, F. C. (2018b). Comparative effectiveness and injury to tomato plants of three neotropical mirid predators of Tuta absoluta (Lepidoptera: Gelechiidae). J. Econ. Entomol. 111, 1080–1086. doi: 10.1093/jee/toy057
Vangansbeke, D., Nguyen, D. T., Audenaert, J., Verhoeven, R., Gobin, B., Tirry, L., et al. (2014). Food supplementation affects interactions between a phytoseiid predator and its omnivorous prey. Biol. Control 76, 95–100. doi: 10.1016/j.biocontrol.2014.06.001
Vankosky, M. A., and VanLaerhoven, S. L. (2015). Plant and prey quality interact to influence the foraging behaviour of an omnivorous insect, Dicyphus hesperus. Anim. Behav. 108, 109–116. doi: 10.1016/j.anbehav.2015.07.019
Wisdom, C. S., Crawford, C. S., and Aldon, E. F. (1989). Influence of insect herbivory on photosynthetic area and reproduction in Gutierrezia species. J. Ecol. 77, 685–692. doi: 10.2307/2260978
Zappala, L., Biondi, A., Alma, A., Al-Jboory, I. J., Arno, J., Bayram, A., et al. (2013). Natural enemies of the South American moth, Tuta absoluta, in Europe, North Africa and Middle East, and their potential use in pest control strategies. J. Pest Sci. 86, 635–647. doi: 10.1007/s10340-013-0531-9
Zhang, N. X., Messelink, G. J., Alba, J. M., Schuurink, R. C., Kant, M. R., and Janssen, A. (2018). Phytophagy of omnivorous predator Macrolophus pygmaeus affects performance of herbivores through induced plant defences. Oecologia 186, 101–113. doi: 10.1007/s00442-017-4000-7
Keywords: biocontrol, omnivore, phytozoophagy, plant indirect defense, plant-feeding, plant injury, tri-trophic interactions, zoophytophagy
Citation: Puentes A, Stephan JG and Björkman C (2018) A Systematic Review on the Effects of Plant-Feeding by Omnivorous Arthropods: Time to Catch-Up With the Mirid-Tomato Bias? Front. Ecol. Evol. 6:218. doi: 10.3389/fevo.2018.00218
Received: 16 July 2018; Accepted: 03 December 2018;
Published: 19 December 2018.
Edited by:
Maria Pappas, Democritus University of Thrace, GreeceReviewed by:
Meritxell Pérez-Hedo, Instituto Valenciano de Investigaciones Agrarias, SpainLene Sigsgaard, University of Copenhagen, Denmark
Copyright © 2018 Puentes, Stephan and Björkman. This is an open-access article distributed under the terms of the Creative Commons Attribution License (CC BY). The use, distribution or reproduction in other forums is permitted, provided the original author(s) and the copyright owner(s) are credited and that the original publication in this journal is cited, in accordance with accepted academic practice. No use, distribution or reproduction is permitted which does not comply with these terms.
*Correspondence: Adriana Puentes, YWRyaWFuYS5wdWVudGVzQHNsdS5zZQ==;YWRyaS5wdWVudGVzQGdtYWlsLmNvbQ==