- 1Natural Systems Analysts, Winter Park, FL, United States
- 2Suisan Resource Conservation District, Suisan City, CA, United States
- 3Department of Fish and Wildlife, Olympia, WA, United States
- 4Regional Office for Africa, Food and Agriculture Organization of the United Nations, Accra, Ghana
- 5Department of Microbiology and Plant Biology, University of Oklahoma, Norman, OK, United States
- 6AEC Ltd., Palm Springs, Yuen Long, Hong Kong
- 7The World Wildlife Fund for Nature Hong Kong, Mai Po Marshes Wildlife Education Centre and Nature Reserve, Yuen Long, Hong Kong
- 8U.S. Geological Survey, Patuxent Wildlife Research Center, Beltsville, MD, United States
Avian influenza has advanced from a regional concern to a global health issue with significant economic, trade, and public health implications. Wild birds, particularly waterfowl (Anseriformes), are known reservoirs for low-pathogenic avian influenza viruses (AIV) and recent studies have shown their potential in the spread of highly pathogenic forms of virus. East Asia remains an epicenter for the emergence of novel strains of AIV, however, information on movement ecology of waterfowl, and subsequently a clearer understanding of disease transmission risks in this region has been greatly lacking. To address this, we marked two species of wild waterfowl, northern pintail (Anas acuta) and Eurasian wigeon (Anas penelope), with satellite transmitters on their wintering grounds in Hong Kong, China to study the northward spring migration in the East Asian-Australasian Flyway in relation to disease transmission factors. Northern pintail were found to initiate migration 42 days earlier, travel 2,150 km farther, and perform 4.4 more stopovers than Eurasian wigeon. We found both species used similar stopover locations including areas along the Yangtze River near Shanghai, Bohai Bay and Korea Bay in rapidly developing regions of the Yellow Sea, and the Sea of Okhotsk where the species appeared to funnel through a migratory bottleneck. Both species appeared to exhibit strong habitat selection for rice paddies during migration stopovers, a habitat preference which has the potential to influence risks of AIV outbreaks as rapid land use and land cover changes occur throughout China. Both species had greatest association with H5N1 outbreaks during the early stages of migration when they were at lower latitudes. While Eurasian wigeon were not associated with outbreaks after the mean date of wintering ground departures, northern pintail were associated with outbreaks until the majority of individuals departed from the Yellow Sea, a migratory stopover location. Our results show species-level differences in migration timing and behavior for these common and widespread species, demonstrating the need to consider their unique temporal and spatial movement ecology when incorporating wild birds into AIV risk modeling and management.
Introduction
Avian influenza viruses (AIVs) have been documented as far back as 1878 (Lupiani and Reddy, 2009) but have only recently become established as a worldwide concern with major conservation, economic, and public health implications (OIE, 2017). Following the Guangdong outbreaks of 1996 (Xu et al., 1999), avian influenza has become endemic in East Asia (Chen et al., 2006; Peiris et al., 2016) and multiple novel strains of highly pathogenic avian influenza (HPAI) have surfaced from this region (Nguyen et al., 2014; Chen L. J. et al., 2016). While each passing year is accompanied by the continued spread and reassortment of AIVs worldwide, East Asia remains an epicenter for avian influenza (Liu D. et al., 2013; Fan et al., 2014). Due to the importance of East Asia in the evolution of AIVs and the global threat they pose, a thorough understanding of how AIVs spread, amplify, and persist is critical.
One of the major elements in the natural cycle of low pathogenic avian influenza (LPAI) is the role played by wild waterfowl. Scientists have long recognized that wild waterfowl serve as reservoirs for LPAI which can mutate into HPAI when introduced to flocks of domestic birds (Alexander, 2007). However, HPAI infected waterfowl may travel over distances (Gaidet et al., 2008; Gilbert et al., 2010; Prosser et al., 2011; Jeong et al., 2014) or maintain infections without displaying clinical signs (Gaidet et al., 2008; Nemeth et al., 2013; Spackman et al., 2017; van den Brand et al., 2018). Concurrent outbreaks in domestic poultry and wild waterfowl (Lyecett et al., 2016; Lee et al., 2017) have led scientists to recognize the role of wild birds as potential vectors for HPAI (Takekawa et al., 2010a; Saito et al., 2015; Lee et al., 2017). Due to the role of wild waterfowl in the transmission, evolution, and spread of AIVs, scientists have begun to incorporate wild bird movement ecology into avian influenza outbreak modeling efforts (Newman et al., 2012; Prosser et al., 2016).
In an effort to better understand the migratory ecology of waterfowl throughout the East Asia-Australasian Flyway (EAAF), there is a growing literature base of telemetry studies in this region. Previous work includes mallard (Anas platyrhynchos) from Japan (Yamaguchi et al., 2008), China (Takekawa et al., 2010b), and South Korea (Shin et al., 2015), and northern pintail from Japan (Anas acuta; Yamaguchi et al., 2010; Hupp et al., 2011) and China (Palm et al., 2015). Additionally, telemetry data exists for species such as Baikal teal (Anas formosa), common teal (Anas crecca), Eurasian wigeon (Anas penelope), falcated teal (Anas falcata), garganey (Anas querquedula), and Chinese spotbill (Anas zonorhyncha; Takekawa et al., 2010b). Many of these species migrate through the Yellow Sea region, an area with a recognized importance to waterbirds in general as noted by the collection of RAMSAR sites in this area (Bird Life International, 2009). The Yellow Sea region serves as an important stopover area for migratory waterfowl and other waterbirds (Takekawa et al., 2010b; Palm et al., 2015; Peng et al., 2017; Xia et al., 2017) but rapid land use change is reducing available migration habitats (Murray et al., 2014; Melville et al., 2016; Szabo et al., 2016).
Despite the growing literature for waterfowl movements in the EAAF, lack of movement information for waterfowl in key wintering areas such as Hong Kong where several avian influenza outbreaks have emerged (Guan et al., 2002; Sims et al., 2003; Sturm-Ramirez et al., 2004) places significant limitations on the ability of researchers and managers to incorporate wild bird behavior into disease modeling and management. For instance, infection prevalence varies between species (Hill et al., 2010, 2012b; Gonzalez-Reiche et al., 2016), suggesting that waterfowl density alone may not be enough to predict transmission risk at the domestic-wild bird interface. Instead, knowing the species composition at this interface across space and time may be more informative, and to account for this, species-specific migration data are required. It is important to understand the migratory behavior of a species from their major wintering locations, as migratory endpoints and stopover locations can differ between individuals from the same wintering grounds (Webster et al., 2002). By migrating to different areas, individuals may be exposed to different viral strains which they may transmit to the wintering grounds (Hill et al., 2012b, 2016). An example of variation in migratory strategies has been reported for mallards wintering in California, where birds at a single wintering ground migrated from three different breeding locations separated by large distances (Hill et al., 2012a). Thus, to adequately represent the spatio-temporal distribution of waterfowl and the inherent role this plays in AIV transmission risk, waterfowl species should not be treated as interchangeable or equal contributors.
Incorporating wild birds into AIV transmission models is important in countries where the poultry system allows for extensive interaction and habitat overlap between domestic and wild birds (Muzaffar et al., 2010; Martin et al., 2011). The lack of relevant data on waterfowl migration ecology is only exacerbated by the rapidly changing agricultural landscape in China. For instance, agricultural lands such as rice paddies, which are critical to migratory waterbirds (Elphick, 2010; Fujioka et al., 2010), are shifting to the northeast (Zhang et al., 2011; Liu Z. et al., 2013; Dong et al., 2015; Gilbert et al., 2017). In order to understand how changes in rice paddy distribution alter wild bird migratory behavior and the domestic-wild bird interface, appropriate baseline data on wild bird movement ecology are required (Meyer et al., 2017). Without this information, the ability to predict future transmission risks will be greatly reduced in the face of rapidly changing land cover and land use.
The goal of our study was to gain understanding of the migration and stopover behavior of Eurasian wigeon and northern pintail in the EAAF and to improve understanding of the movement ecology of wild birds in avian influenza modeling. Our specific objectives were to (1) document spring migratory and stopover characteristics and understand how these may relate to avian influenza risk, (2) explore the relationship between wild bird movements and avian influenza outbreaks, and (3) establish baseline information on spatio-temporal rice use to allow for the potential future examination of how changes at the global (climate) and local (land use) scales affect the domestic-wild bird interface.
Methods
Study Site
Ducks were captured at the Mai Po Nature Reserve in Hong Kong (22.491°N, 114.042°E), a 380-ha wildlife preserve located in Inner Deep Bay and operated by the World Wildlife Fund for Nature. Mai Po Nature Reserve and the broader Inner Deep Bay are recognized as a RAMSAR Convention site due to the high ecological value of the area (Bird Life International, 2009) that meets the 1% criterion (area contains more than 1% of a species' total population) for 9 waterbird species. Inner Deep Bay provides significant wintering habitat for migratory waterfowl, supporting over 25,000 waterfowl annually (AFCD, 2011). However, this area is currently under threat from sedimentation (WWF-Hong Kong, 2017) and has had several avian influenza outbreaks nearby (EMPRES-i, 2017). The Bay is a key location for examining the migratory coastal movements of waterfowl and for determining the implications of their spring migration behavior on the spread of avian influenza.
Capture and Marking
During December of 2008 and 2009, 16 Eurasian wigeon and 82 northern pintail were captured under a whoosh-net baited with mixed grains (Gosler, 2004). Both species were abundant winter visitors to Inner Deep Bay (Carey et al., 2001). Upon capture, birds were placed in large holding containers and stored out of direct sunlight until they could be individually processed and released near the capture location. Species, age, flat wing, mass, diagonal tarsus, and culmen length were recorded for each bird. Additionally, some individuals (see Results) were marked with either 12 g (10 EUWI and 11 NOPI) or 18 g (13 NOPI) ARGOS solar powered Platform Terminal Transmitters (PTT; PTT-100), or 22 g (6 EUWI and 7 NOPI) GPS-solar powered PTTs (solar GPS PTT-100, Microwave Telemetry, Inc., Columbia, MD, USA). Transmitters were affixed to birds with a Teflon backpack harness (Bally Ribbon Mills, Bally, PA), and transmitter packages were < 3% of the animal's body weight. All procedures involving live animals were in accordance with the methodology outlined by the Food and Agriculture Organization of the United Nations (FAO, 2007) and approved by the U.S. Geological Survey, Patuxent Wildlife Research Center Animal Care and Use Committee.
Data Collection and Manipulation
Telemetry Data
Argos PTTs were programmed to transmit signals for 8-h periods once every 52 h, and GPS PTTs were scheduled to record locations once every 2 h. Transmissions were received by the Argos satellite tracking system (CLS America Inc., Largo, MD). Prior to analysis, we used the Douglas Argos-Filter Algorithm hybrid filter (Douglas et al., 2012), available within Movebank (www.movebank.org), to censure data for erroneous fixes. Additionally, data were manually inspected in ArcGIS 10 (Environmental Systems Research Institute, Inc., Redlands, CA) to determine the biological end dates for each animal to ensure only data collected while the transmitter was attached to a live bird were used. Departure from wintering grounds and completion of migration, as determined by repeated locations during the period associated with breeding (Ely et al., 2007), were determined via manual inspection of the data for each individual.
Migration Characteristics
Each bird with data for its entire migratory path was categorized as an intermediate or long-distance migrant based upon if the individual migrated to areas indicated as the species breeding range in published range maps (Mackinnon and Phillipps, 2000; Bird Life International, 2018; Carboneras and Kirwan, 2018; Carboneras et al., 2018). We recognize that these range maps are coarse interpretations of breeding ranges, but they do provide a replicable criterion for our analysis. While individuals that did not migrate to the species' reported breeding range may have been impacted by the attached transmitters, substantial movement was observed from each of these individuals after the end of their respective migrations indicating birds remained alive and active well after arrival to these locations.
In order to characterize the migration of Eurasian wigeon and northern pintail, we calculated several descriptive metrics for each individual. Our first metric, migration initiation date, was represented by the date of the last locational fix on the wintering grounds (Trierweiler et al., 2014). While migration initiation date was determined for all individuals that left the wintering grounds, all other migration characteristics were only calculated for birds with data for their complete spring migration, with end dates represented by the date of the first location where the bird ended its migration (Trierweiler et al., 2014; Palm et al., 2015). Another metric, migration distance, was calculated as the summed distance between each locational fix for overall total migration while excluding localized movements. Only consecutive fixes ≥50 km from the previous fix were considered part of active migration, and the cumulative geodesic distance between active migration points was calculated. We used this approach to remove the influence of localized movements during stopovers (Trierweiler et al., 2014). Additionally, the departure date from the Yellow Sea region was recorded for all individuals with locations anywhere within 50 km of the main body of the Yellow Sea. Differences in migration characteristics between and within species were evaluated via linear regression in program R (v3.3.3; R Core Team, 2017).
Stopover Identification
Following the methods of Palm et al. (2015), we created dynamic Brownian Bridge Movement Models (dBBMM) with the “move” package in R (R Core Team, 2017) to estimate a utilization distribution (UD) for each bird that completed spring migration. The 50, 75, and 99% contours of the resulting models were defined as stopover sites, core movement areas, and flight corridors, respectively (Palm et al., 2015; although see Hupp et al., 2011 for an alternative identification method). The number of individual stopover events performed during spring migration was recorded by bird, and the coordinates of each location were recorded at the centroid of the stopover location. Only stopover locations ≥50 km apart were considered to be separate events. We also constructed cumulative UDs for the intermediate and long-distance migrants within each species to identify flight corridors and important habitats by summing the UDs generated for individuals. To account for variation in migration duration between individuals when summing dBBMMs, we weighted each model by the duration of the migration event, multiplying all pixel values in each UD by the number of days in the migration event. We then summed the pixel values of all weighted UDs, and rescaled cumulative pixel values such that they summed to 1. This resulted in a UD that represented the relative use of each pixel during migration by that species across seasons. Differences in stopover use between and within species were evaluated with linear regression.
Avian Influenza Outbreaks and Wild Birds
Following the methods of Newman et al. (2012), telemetry data were separated for each bird into 14-day periods running from 1 February to 1 August with no distinction made between years. These dates included 1 biweekly period prior to the first individual initiating migration and 2 biweekly periods after the last bird completed migration. Individuals were removed from the sample during the time period prior to their end date to ensure that each data sample was representative of use over a full 14-day period. To account for the different number of locations between birds, we used the kernel density estimator in ArcGIS to construct a separate utilization distribution on a fixed 125 km grid for every bird by period, resulting in three dimensional utilization distributions that account for time (Keating and Cherry, 2009). We then averaged all of the UDs within a given period by species and rescaled the pixel values such that they summed to 1. We found the 99% contour for each period with the contour tool in ArcGIS. We also constructed a minimum convex polygon (MCP) around all 99% contours for each species to establish the domain for outbreaks within the EAAF.
The spatial locations of H5N1 outbreaks were downloaded from the EMPRES database for 2007–2012 (± 2 years from observed migrations). These outbreaks were then overlaid with the contours and MCPs to determine the number of outbreaks that fell within each biweekly contour, and the number of outbreaks outside of the contour but within the species MCP. A 7-day lag was applied to outbreak data when pairing with bird locations to allow for incubation and onset of AIV symptoms. Exact binomial tests were used via the R “stats” package (R Core Team, 2017) to compare the observed and expected proportion of outbreaks associated with UDs. When calculating expected values, only the terrestrial areas of the MCPs and contours were used, because outbreaks were not expected over open water. All outbreaks were treated similarly with no distinction between wild bird and domestic poultry outbreaks.
Rice Paddy Use
We obtained a land cover data layer depicting the rice paddies within China at 500-meter resolution during 2009 (see details in Zhang et al., 2011) to compare with the dBBMM model generated for each bird with a full spring migration. The percentage of the flight corridor representing habitat availability and migratory stopover sites representing habitat use were determined with the tabulate area tool in ArcGIS for the dominant agricultural types (rice, wheat, and pastoral) as defined by Pei et al. (2016). These percentages were then compared by species and region with paired t-tests in the “stats”package in R (R Core Team, 2017) to determine if rice use was proportional to availability. Since no comparisons were made between groups, test results were not controlled for alpha inflation. Only individuals that performed a stopover were used in the analysis of that region.
Results
Capture and Marking
Eleven of 16 Eurasian wigeon and 26 of 31 northern pintail migrated from the wintering grounds. Loss of signal for birds marked with transmitters was attributed to radio failure, transmitter loss, or mortality, but the specific cause of failure could not be determined. Six Eurasian wigeon and 10 northern pintails transmitted data for their complete spring migrations.
Migration Characteristics and Stopovers
Eurasian wigeon initiated spring migration on 24 March (Figure 1), and birds took an average of 57 days to migrate with an average of 3.5 stopovers (Table 1). While they migrated as far north as subarctic Siberia (Figure 2A), three individuals followed an intermediate migration strategy and stopped short of the species' reported primary breeding range (Mackinnon and Phillipps, 2000; Bird Life International, 2018; Carboneras and Kirwan, 2018; Carboneras et al., 2018; Figure 2B). Long-distance migrants flew significantly farther (+1,704 km) than the intermediate-distance migrants [F(1, 4) = 17.44, p = 0.014] and took 13 fewer days to complete migration [F(1, 4) = 0.42, p = 0.550]. This resulted in the nearest intermediate and long-distance migrant's migration endpoints being separated by 1,620 km. Long-distance migrants also performed 0.7 more stopovers per bird [F(1, 4) = 0.25, p = 0.643]. Departure dates from the wintering grounds and the Yellow Sea region did not differ significantly between groups (p > 0.05).
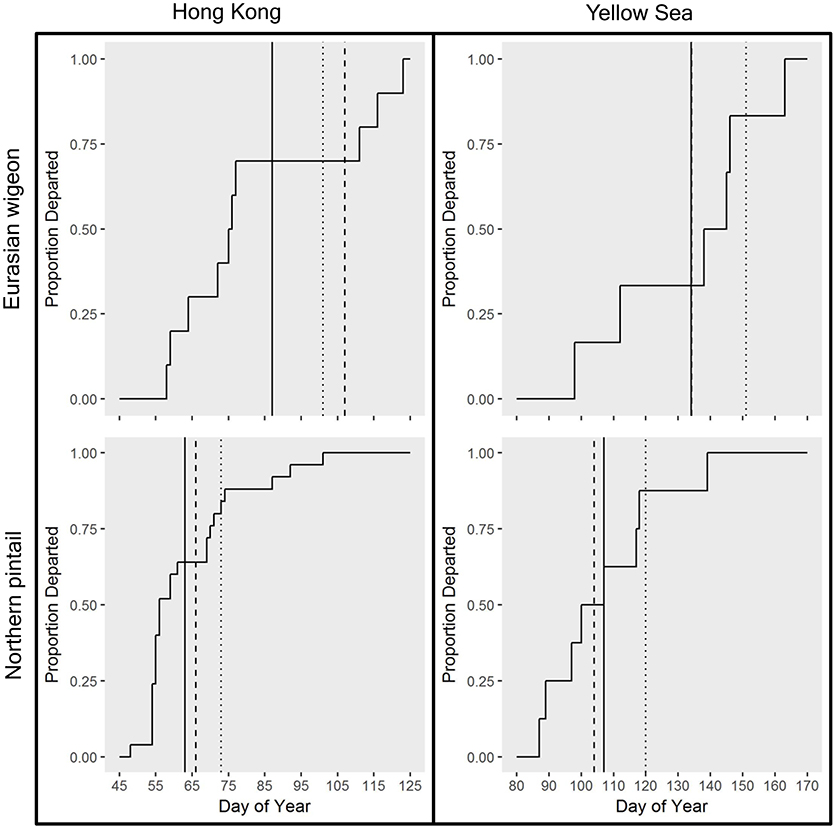
Figure 1. Mean spring initiation migration date and date of departure from the Yellow Sea region for Eurasian wigeon marked at Mai Po Nature Preserve in Hong Kong China from 2008 to 2009. Means were determined for intermediate-distance migrants (dotted lines), and long-distance migrants (dashed lines), and all birds combined (solid lines).
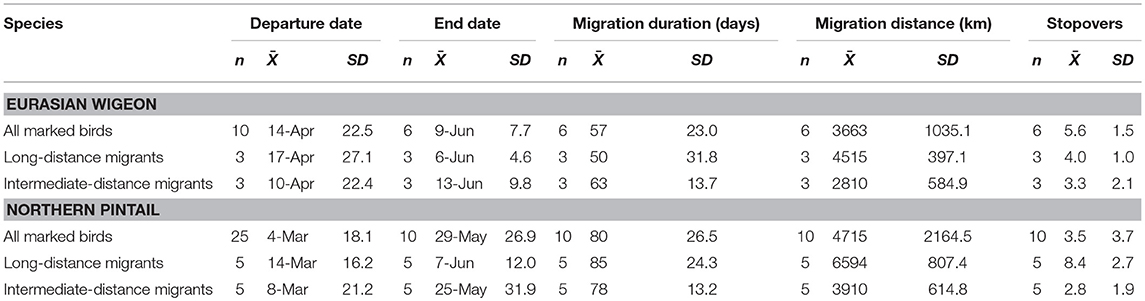
Table 1. Spring migration characteristics of Eurasian wigeon and northern pintail marked at Mai Po Nature Preserve in Hong Kong China from 2008 to 2009.
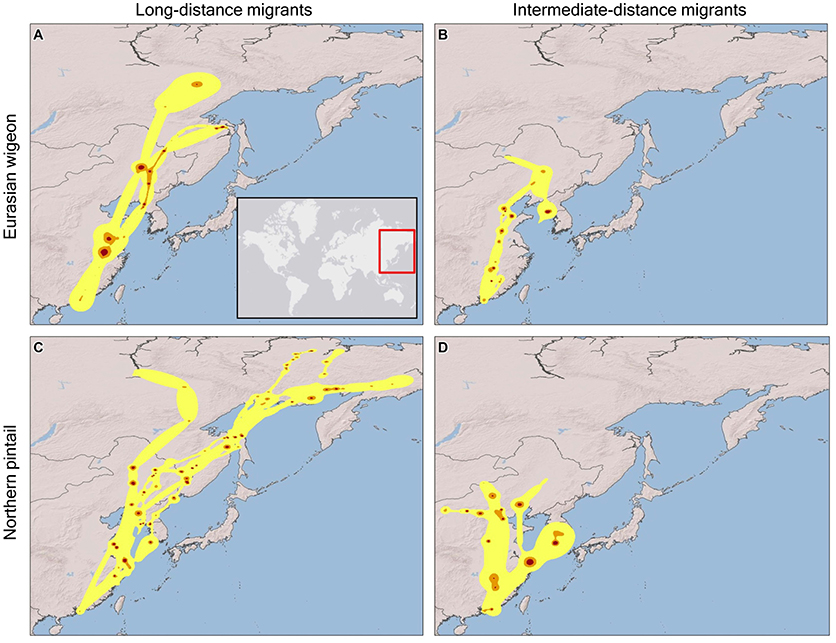
Figure 2. Spring migration utilization distributions determined from dynamic Brownian Bridge Movement Models for Eurasian wigeon [long-distance migrants (A), intermediate-distance migrants (B)] and northern pintail [long-distance migrants (C), intermediate-distance migrants (D)] marked at Mai Po Nature Preserve in Hong Kong China from 2008 to 2009. Flight corridors (99%; yellow), core movement areas (75%; orange), and stopover locations (50%, red) are indicated.
In comparison, northern pintail initiated spring migration on 4 March (Figure 1) with the average migration taking 80 days to cover 4,715 km including 5.6 stopovers (Table 1). Northern pintail migration extended farther west into inland China and farther north into Siberia than did Eurasian wigeon (Figure 2C). Five birds settled short of their reported central breeding grounds (Mackinnon and Phillipps, 2000; Bird Life International, 2018; Carboneras and Kirwan, 2018; Carboneras et al., 2018; Figure 2D). Long-distance migrants flew significantly farther (+3901 km) than intermediate-distance migrants [F(1, 8) = 73.88, p < 0.001] but took 35 days longer to complete their migration [F(1, 8) = 7.57, p = 0.025] and performed 5.6 additional stopovers [F(1, 8) = 14.25, p = 0.005]. This resulted in a minimum separation of migration endpoints of 2,043 km between intermediate and long-distance migrants. Departure dates from the wintering grounds and the Yellow Sea region did not differ significantly between groups (p < 0.05).
Overall, northern pintail initiated migration 23 days prior to Eurasian wigeon [F(1, 34) = 12.58, p = 0.001], although their arrival date [F(1, 14) = 1.06, p = 0.321], migration distance [F(1, 14) = 1.22, p = 0.287], and number of stopovers [F(1, 14) = 1.47, p = 0.246] did not differ significantly between species. Yet, when long-distance migrants were compared, northern pintail were found to migrate 42 days earlier [F(1, 6) = 7.71, p = 0.032], fly 2,150 km farther [F(1, 6) = 17.80, p = 0.006], and perform 4.4 more stopovers [F(1, 6) = 6.981, p = 0.038] than Eurasian wigeon. For intermediate-distance migrants, there were no significant differences in departure dates [F(1, 6) = 3.17, p = 0.125], migration distances [F(1, 6) = 0.01, p = 0.920], arrival dates [F(1, 6) = 2.16, p = 0.192], or the number of stopovers [F(1, 6) = 0.14, p = 0.725] between northern pintail and Eurasian wigeon. Both species used similar stopover locations including areas along the Yangtze River near Shanghai and Bohai and Korea Bays on the Yellow Sea. The Strait of Tartar at the Sea of Okhotsk appeared to serve as a migration bottleneck where 2 Eurasian wigeon settled and 4 northern pintail passed through on their way to Siberia.
Avian Influenza Outbreaks
The spatio-temporal association of both species with H5N1 outbreaks was greatest along the southern portions of their migratory paths but was less correlated as the birds moved northward (Figures 3, 4). However, the significance of the spatio-temporal association with outbreaks may have been confounded by overall reduction in outbreaks as the birds moved northward (Figure 5, Table 2). The core period of association between Eurasian wigeon and H5N1 outbreaks occurred while most individuals were on the wintering grounds. In contrast, northern pintail had a significant association (p ≤ 0.05) with outbreaks until the majority of individuals had departed from the Yellow Sea.
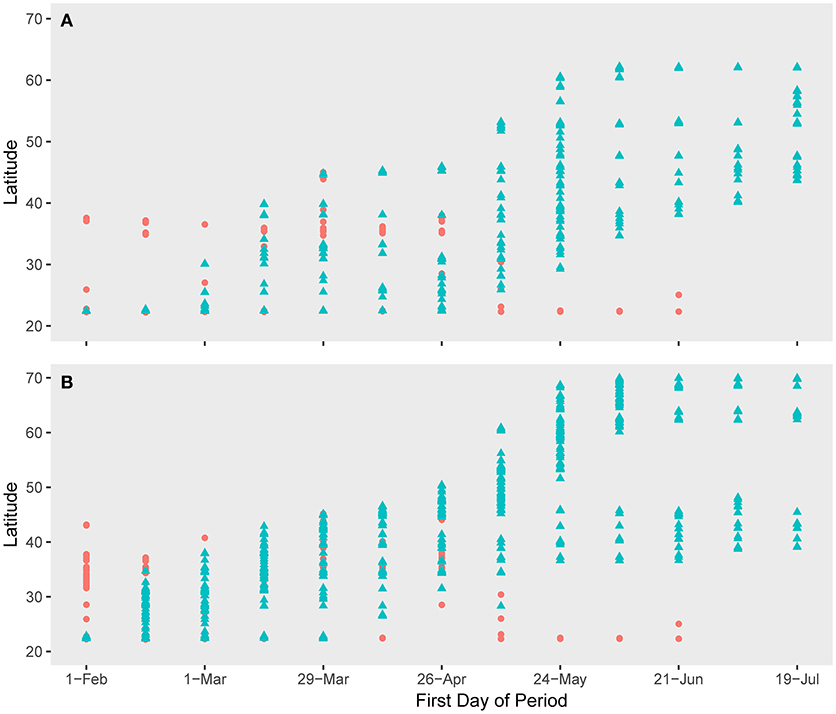
Figure 3. Northward movement of (A) Eurasian wigeon and (B) northern pintail in relation to outbreaks of H5N1 avian influenza along their migration route. Pink circles denote H5N1 outbreaks while blue triangles denote bird locations. The date represents the first day in a 2-week period encompassing bird locations. H5N1 outbreaks were matched to these periods on a 7-day lag to allow for incubation and onset of symptoms after a bird is exposed.
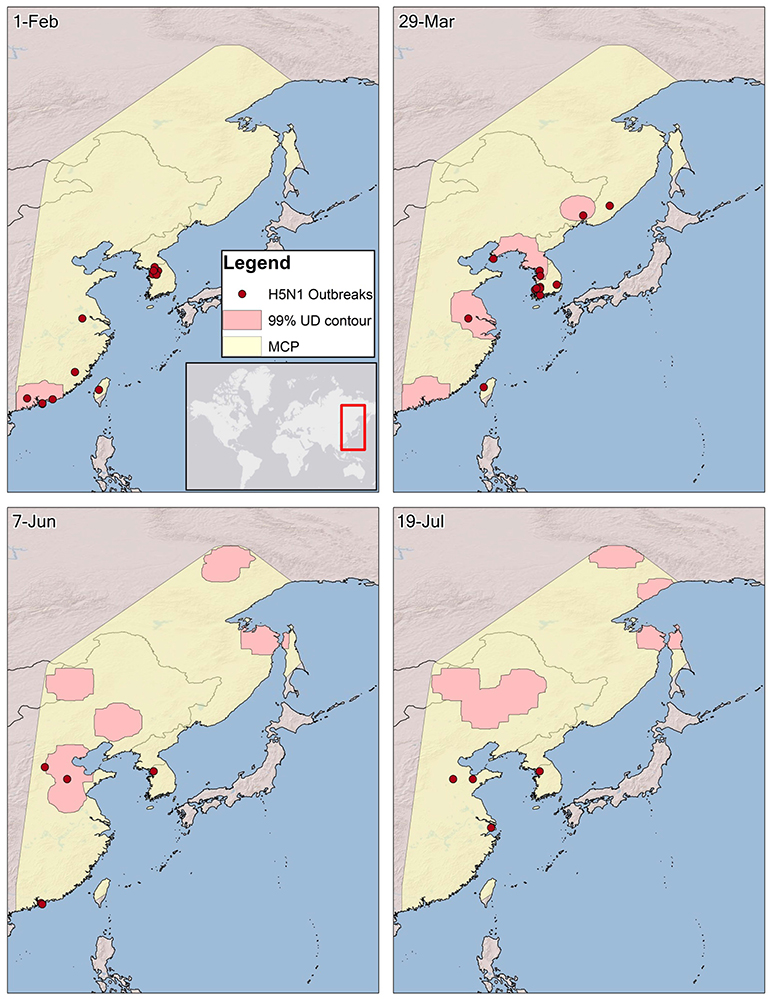
Figure 4. The spatio-temporal association between H5N1 outbreaks and Eurasian wigeon during spring migration from Hong Kong. The 99% utilization distribution (UD) contour, representing bird locations during a 14-day period beginning on a date was derived from a kernel density averaging individual UDs for each bird per time period. A 99% minimum convex polygon (MCP) was created to delineate a domain for outbreaks within the flyway. Areas for both the contours and MCP over the open ocean and bays were removed since outbreaks would not occur in these areas. Outbreaks were grouped on a 7-day lag from bird locations to allow for incubation and onset of symptoms after a bird is infected.
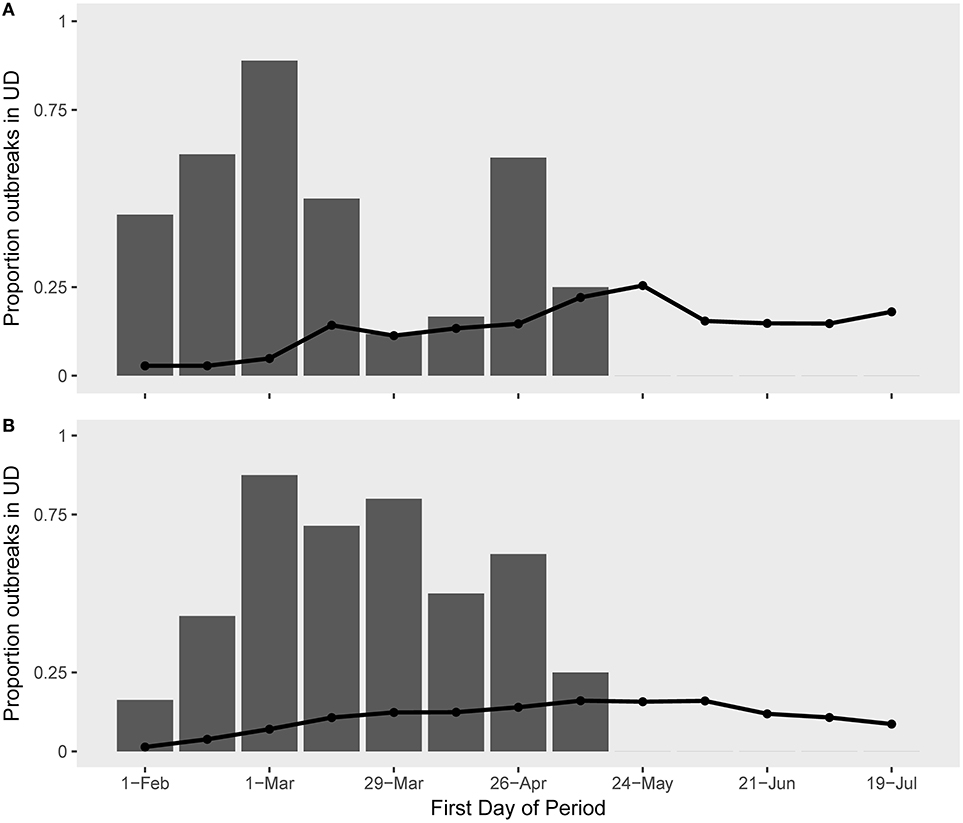
Figure 5. The observed (bars) and expected (lines) proportions of H5N1 along the migratory route of (A) Eurasian wigeon and (B) northern pintail spatio-temporally associated with species presence.
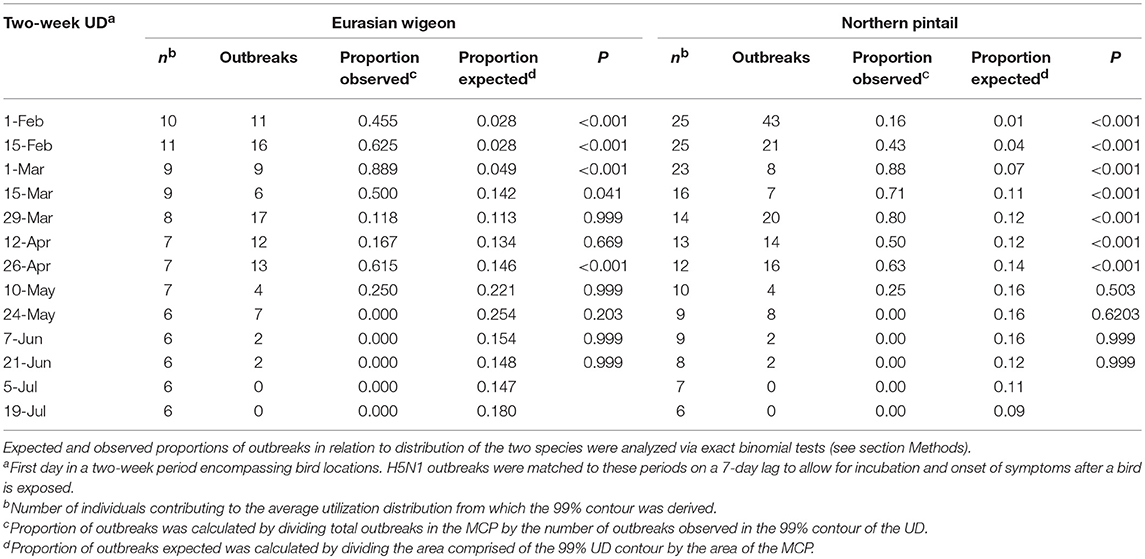
Table 2. Observed and expected spatio-temporal association between H5N1 outbreaks and the utilization distributions of Eurasian wigeon and northern pintail during spring migration from wintering grounds in Hong Kong.
Rice Paddy Use
For both Eurasian wigeon and northern pintail, rice paddies constituted a large proportion of used habitats compared to available habitats in both the flyway and the stopover areas (Figure 6, Table 3). Both Eurasian wigeon [t(4) = 2.86, p = 0.046] and northern pintail [t(7) = 3.43, p = 0.011] demonstrated selection for paddies in the rice region, while there was no significant selection for specific habitats in either the wheat [t(1) = 1.49, p = 0.376; t(1) = 0.06, p = 0.960] or pastoral regions [t(3) = −0.03, p = 0.804; t(7) = 1.91, p = 0.097].
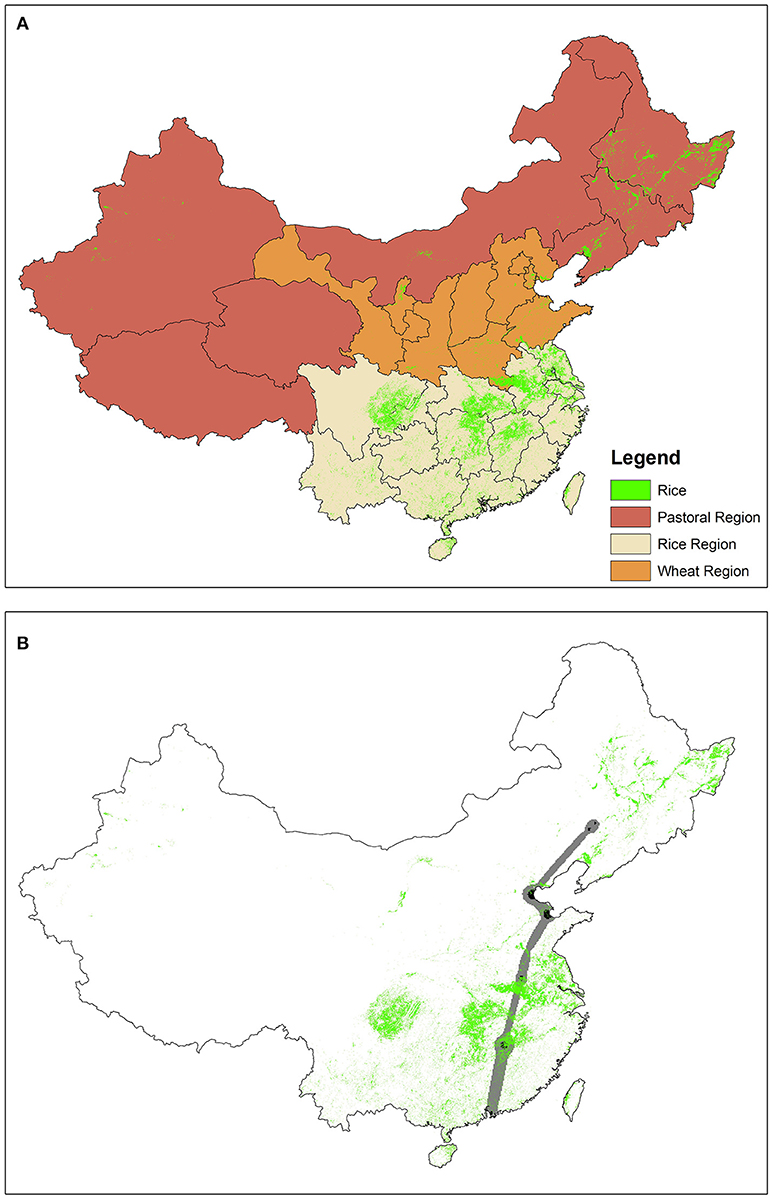
Figure 6. Distribution of rice paddies across (A) China's agricultural zones as defined by Pei et al. (2016), and (B) within the flight corridor (gray) and migration stopover areas (black) of Eurasian wigeon (PTT #44682) during spring migration. While only one individual is depicted in B, analyses were conducted for all individuals with complete spring migration tracks (6 Eurasian wigeon, 10 northern pintail).

Table 3. The mean percentage of Eurasian wigeon and northern pintail stopover sites (use) and flight corridors (available) composed of rice paddies (as depicted by Zhang et al., 2011) within China's three agricultural regions (as defined by Pei et al., 2016).
Discussion
We found that wild waterfowl migrating along coastal areas of the EAAF exhibited a high degree of variability in both stopover locations and distribution to apparent breeding areas. Several Eurasian wigeon and northern pintail settled in areas up to a few thousand kilometers south of their central reported breeding area in Siberia. Use of breeding grounds in lower latitudes where avian influenza is more common (Takekawa et al., 2010b; EMPRES-i, 2017) could have significant implications in the diversity of viral strains transmitted on the wintering grounds (Hill et al., 2012a, 2016) and for the overall potential of outbreaks. The two different migratory strategies by long-distance and intermediate-distance migrants from the same wintering area was consistent with the findings Hill et al. (2012a) reported for mallard in California.
Conversely, since many species such as northern pintail commonly fail to breed every season (Miller et al., 2005; Clark et al., 2014), intermediate-distance migrants may be exhibiting a short-stopping strategy that individuals use in seasons where they will not breed. Range maps of reported breeding ranges for waterfowl species in Asia are generally inferred based on expert knowledge and anecdotal evidence rather than documented with tools such as satellite transmitters, and it has been noted that such breeding ranges and timelines are not always indicative of species behavior (Prosser et al., 2018). Thus, it also is feasible that the intermediate-distance migrants are following a long-standing migration that has not been incorporated into species' range maps. Unfortunately, the majority of intermediate-distance migrants we documented were males who quickly leave their mate after breeding and remain on the breeding grounds only until incubation has ended (Kear, 2005; Clark et al., 2014); we were unable to record enough locations to suggest if reproduction may have occurred (see Ely et al., 2007; Takekawa et al., 2011). However, even if the intermediate-distance migrants are not breeding at these sites, understanding such behavior is still crucial as shorter migrations increase the potential for infected birds to successfully complete migration and serve as meaningful vectors for AIVs (Gaidet et al., 2008; Gilbert et al., 2010).
An alternative explanation for our findings is that we documented changing migration patterns for these species in response to climate change or land use changes. However, the vast majority of shifts related to changing climates have been toward the poles (Guillemain et al., 2013; Potvin et al., 2016) rather than toward the equator. Also, individuals may use separate settling strategies in years when environmental conditions are poor or when the bird itself is in poor condition. Such behavior has been reported for northern pintail migrating along the Pacific Coast of North America where individuals avoided migration routes over the ocean in particularly cold and wet years (Miller et al., 2005).
Also, we are not able to discount the possibility that movements of the monitored individuals were influenced by the tracking devices. Meta-analyses to examine the influence of radio transmitters on avian migration (Barron et al., 2010; De Vries, 2014) have led to some concerns over the interpretation of migratory data from marked birds. However, multiple citizen science reports of unmarked Eurasian wigeon and northern pintail throughout Northeast China and the Korean Peninsula during the summer months (eBird, 2012) corroborate our findings and suggest that intermediate-distance migration may be a regular occurrence. Another variable potentially affected is the migration end date, as this has been previously reported for northern pintail (Hupp et al., 2011). However, our mean arrival date to the breeding grounds of Eurasian wigeon fell within the normally reported values of May-June (Kear, 2005), the arrival date for northern pintail to the breeding grounds was later than expected which also was suggested in a study of northern pintails marked in Japan (Hupp et al., 2011). Additional clarity may have been reached had our sample size been larger, but this was precluded by the failure of multiple individual birds to provide reliable data. Citizen science reports and some published data suggest that our results are representative of the population, but marking of additional birds could help validate our findings.
The differences in migratory ecology between Eurasian wigeon and northern pintail resulted in dramatic differences in their respective domestic poultry-wild bird interfaces across space and time. For instance, while both species had strong associations with H5N1 outbreaks early in the spring, this association was mainly limited to the wintering grounds for Eurasian wigeon as they had not yet initiated migration. Meanwhile, since northern pintail initiate migration earlier than Eurasian wigeon, their association with outbreaks continued along their migratory route to the Yellow Sea. Thus, northern pintail could transmit AIV to other birds at higher latitudes earlier in the spring than Eurasian wigeon. Their earlier initiation of migration may allow for stopovers at wintering and migration sites with exposure to birds with viral strains not present at their original wintering grounds (Hill et al., 2016). Thus, northern pintail may be more likely to introduce AIVs to new environments than Eurasian wigeon, and that finding is consistent with the northern pintails' suggested role in the spread of Asian origin AIVs to North America (Ramey et al., 2010; Pearce et al., 2011). Infection rates can vary greatly between species (Hill et al., 2010, 2012b; Gonzalez-Reiche et al., 2016), and the risk of transmission may vary depending on species composition in wintering or stopover areas.
We found extensive use of stopover areas along the Yellow Sea by both species, and these findings add further evidence to the importance of the Yellow Sea ecosystem for the EAAF. Previous research has found that millions of shorebirds use the intertidal areas as staging habitats (e.g., Barter and Riegen, 2004; Barter, 2006; Bamford et al., 2008; Rogers et al., 2010; Yang et al., 2011; MacKinnon et al., 2012; Xia et al., 2017) with 6 of 16 key shorebird biodiversity areas in the EAAF located around the Yellow Sea (MacKinnon et al., 2012). Yellow Sea stopovers have significant implications for the spread of AIVs, as this behavior allows for intermixing of birds from separate wintering grounds in large numbers, and in stopover areas at lower latitudes, infected birds may still shed virus from wintering areas upon arrival (Gaidet et al., 2008; Gilbert et al., 2010). We found a higher association of wild birds with outbreaks occurred in southern latitudes. Thus, while conservation measures have been proposed to protect the Yellow Sea from rapid urban expansion and habitat loss (Murray et al., 2014) and degradation from damming of major rivers, pollution, wind and tidal power plants, and aquaculture (MacKinnon et al., 2012; Murray et al., 2015), this region may require increased surveillance and control measures as it is at higher risk of AIV outbreaks.
Farther to the north, we observed extensive use of the region where the Strait of Tartar meets the Sea of Okhotsk. Multiple northern pintail passed through this location and some Eurasian wigeon settled here. While use of the Sea of Okhotsk was not as ubiquitous as was the Yellow Sea, it is still a known stopover location for numerous waterbird species (Gerasimov and Gerasimov, 1998; Yamaguchi et al., 2010; Shimada et al., 2014; Chen W. et al., 2016) which indicates it is another migratory bottleneck in the EAAF. While this region is less likely to serve as an area of elevated risk for AIV outbreaks due to its high latitude, conducting surveillance on populations as they pass through this location may help to elucidate trends in the avian influenza cycle.
The final key finding of our study was the preference for rice paddies at stopover sites by both Eurasian wigeon and northern pintail. Rice paddies serve as crucial food sources (Elphick, 2010; Fujioka et al., 2010) during energetically demanding migration periods (Sandberg and Moore, 1996). Increasing urbanization, industrialization, and infrastructure construction have led to substantial decreases in agricultural lands in southern China (Jiang et al., 2013; Liu Z. et al., 2013) while simultaneously pushing remaining agricultural lands to the northeast (Zhang et al., 2011; Liu Z. et al., 2013; Dong et al., 2015). Our results suggest that as rice agriculture becomes more prominent in northeastern China, changes in stopover patterns are likely to occur. However, the overall effect that changes in the distribution of rice agriculture will have on migratory waterfowl is difficult to predict. Increased northeast rice agriculture could lead to greater stopover duration and increased concentration of waterfowl at these locations, but it is equally possible that decreased concentration of food resources could reduce intermixing of large populations. Furthermore, climate change effects may alter the timing of rice production creating a mismatch between food availability and waterfowl migration or forcing waterfowl to alter their migration timing (van Wijk et al., 2012). While the specific effects that changes in rice agriculture and associated poultry will have on migration patterns of wild birds remains unknown, monitoring such changes will be crucial for disease outbreak preparedness (Muzaffar et al., 2010; Wood et al., 2010), as rice paddies serve as a primary location of the wild bird- poultry interface.
Data Availability
The telemetry data supporting the conclusions of this manuscript will be made available by the authors, without undue reservation, to any qualified researcher.
Author Contributions
JT, KS, SN, XX, PL, BS, and DP conceived and designed the study. JS and KS analyzed the data. JS, DP, and JT wrote the paper. All authors read and approved the final manuscript.
Conflict of Interest Statement
The authors declare that the research was conducted in the absence of any commercial or financial relationships that could be construed as a potential conflict of interest.
The handling Editor declared a past co-authorship with one of the authors, JT.
Acknowledgments
All data reported in this manuscript were collected in accordance with protocol approved by the Patuxent Wildlife Research Center Animal Care and Use Committee. We would like to acknowledge E. Bridge, M. Peiris, Katherine Leung, and J. Wall for their contributions in data collection and preparation. We would like to thank our internal reviewer and peer reviewers for their thoughtful revisions during the publication process. This work was supported by the U.S. Geological Survey (Ecosystems Mission Area), the National Institute of Health (NIH) NIAID (1R01AI101028-01A2), and the Food and Agriculture Organization of the United Nations. The use of trade, product, or firm names in this publication is for descriptive purposes only and does not imply endorsement by the U.S. Government. The views expressed in this information product are those of the author(s) and do not necessarily reflect the views or policies of FAO. We would like to thank The Department of Agriculture Fisheries and Conservation of the Government of the Hong Kong SAR who issued the relevant permits relevant to the trapping undertaken in Hong Kong.
References
AFCD (2011). Mai Po Inner Deep Bay Ramsar Site Management Plan. Hong Kong: Agriculture, Fisheries and Conservation Department of the Government of the Hong Kong Special Administrative Region.
Alexander, D. J. (2007). An overview of the epidemiology of avian influenza. Vaccine 25, 5637–5644. doi: 10.1016/j.vaccine.2006.10.051
Bamford, M. J., Watkins, D. G., Bancroft, W., Tischler, G., and Wahl, J. (2008). Migratory Shorebirds of the East Asian Australasian Flyway: Population Estimates and Internationally Important Sites. Canberra: Wetlands International–Oceania.
Barron, D. G., Brawn, J. D., and Weatherhead, P. J. (2010). Meta-analysis of transmitter effects on avian behaviour and ecology. Methods Ecol. Evol. 1, 180–187. doi: 10.1111/j.2041-210X.2010.00013.x
Barter, M. (2006). “The Yellow Sea—A vitally important staging region for migratory shorebirds,” in Waterbirds Around the World, eds G. C. Boere, C. A. Galbraith, and D. A. Stroud (Edinburgh: The Stationery Office), 663–667.
Barter, M., and Riegen, A. (2004). Northward shorebird migration through Yalu Jiang National Nature Reserve. Stilt 46, 9–15.
Bird Life International (2009). Directory of Important Bird Areas in China (Mainland): Key Sites for Conservation. Cambridge: BirdLife International.
Bird Life International (2018). IUCN Red List for birds. Available online at: http://www.birdlife.org (Accessed July 6, 2018).
Carboneras, C., Christie, D. A., and Kirwan, G. M. (2018). “Eurasian Wigeon (Mareca penelope),” Handbook of the Birds of the World Alive, eds J. del Hoyo, A. Elliott, J. Sargatal, D. A. Christie, and E. de Juana (Barcelona: Lynx Edicions). Available online at: https://www.hbw.com/node/52862 (Accessed July 6, 2018).
Carboneras, C., and Kirwan, G. M. (2018). “Northern Pintail (Anas acuta),” in Handbook of the Birds of the World, eds J. Alive del Hoyo, A. Elliott, J. Sargatal, D. A. Christie, and E. de Juana (Barcelona: Lynx Edicions. Available online at: https://www.hbw.com/node/52884 (Accessed July 6, 2018).
Carey, G. J., Chalmers, M. L., Diskin, D. A., Kennerley, P. R., Leader, P. J., Leven, M. R., et al. (2001). The Avifauna of Hong Kong. Hong Kong: Hong Kong Birdwatching Society.
Chen, H., Smith, G. J. D., Li, K. S., Wang, J., Fan, X. H., Rayner, J. M., et al. (2006). Establishment of multiple sublineages of H5N1 influenza virus in Asia: implications for pandemic control. Proc. Nat. Acad. Sci. 103, 2845–2850. doi: 10.1073/pnas.0511120103
Chen, L. J., Lin, X. D., Guo, W. P., Tian, J. H., Wang, W., Ying, X. H., et al. (2016). Diversity and evolution of avian influenza viruses in live poultry markets, free-range poultry and wild wetland birds in China. J General Virol. 97, 844–854. doi: 10.1099/jgv.0.000399
Chen, W., Doko, T., Fujita, G., Hijikata, N., Tokita, K. I., Uchida, K., et al. (2016). Migration of tundra swans (Cygnus columbianus) wintering in Japan using satellite tracking: identification of the Eastern Palearctic Flyway. Zool. Sci. 33, 63–72. doi: 10.2108/zs150066
Clark, R. G., Fleskes, J. P., Guyn, K. L., Haukos, D. A., Austin, J. E., and Miller, M. R. (2014). “Northern Pintail,” The Birds of North America, ed A. F. Poole (Ithaca, NY: Poole Cornell Lab of Ornithology), doi: 10.2173/bna.163
De Vries, M. (2014). The Effect of Telemetric Devices on the Flight and Swimming Performance of Birds. Master's Thesis. Utrecht: Utrecht University.
Dong, J., Xiao, X., Kou, W., Qin, Y., Zhang, G., Li, L., et al. (2015). Tracking the dynamics of paddy rice planting area in 1986–2010 through time series Landsat images and phenology-based algorithms. Rem. Sens. Environ. 160, 99–113. doi: 10.1016/j.rse.2015.01.004
Douglas, D. C., Weinzierl, R., Davidson, S., Kays, R., Wikelski, M., and Bohrer, G. (2012). Moderating Argos location errors in animal tracking data. Methods Ecol. Evol. 3, 999–1007. doi: 10.1111/j.2041-210X.2012.00245.x
eBird (2012). eBird: An Online Database of Bird Distribution and Abundance [Web Application]. Ithaca, NY: eBird. Available online at: http://www.ebird.org (Accessed February 2, 2012).
Elphick, C. S. (2010). Why study birds in rice fields? Waterbirds 33 (Supp1. 1), 1–7. doi: 10.1675/063.033.s101
Ely, C. R., Bollinger, K. S., Densmore, R. V., Rothe, T. C., Petrula, M. J., Takekawa, J. Y., et al. (2007). Reproductive strategies of northern geese: why wait? Auk 124:594. doi: 10.1642/0004-8038(2007)124[594:RSONGW]2.0.CO;2
EMPRES-i (2017). EMPRES-iGlobal Animal Disease Information System. Food and Agriculture Organization of the United Nations. Available online at: http://empres-i.fao.org/eipws3g/
Fan, S., Zhou, L., Wu, D., Gao, X., Pei, E., Wang, T., et al. (2014). A novel highly pathogenic H5N8 avian influenza virus isolated from a wild duck in China. Influenza Other Respir. Viruses 8, 646–653. doi: 10.1111/irv.12289
FAO (2007). “Wild Birds and Avian Influenza: an introduction to applied field research and disease sampling techniques,” FAO Animal Production and Health Manual, No. 5. Rome, eds D. Whitworth, S.H. Newman, T. Mundkur and P. Harris. Available online at: www.fao.org/avianflu
Fujioka, M., Don Lee, S., Kurechi, M., and Yoshida, H. (2010). Bird use of rice fields in Korea and Japan. Waterbirds 33, 8–29. doi: 10.1675/063.033.s102
Gaidet, N., Cattoli, G., Hammoumi, S., Newman, S. H., Hagemeijer, W., Takekawa, J. Y., et al. (2008). Evidence of infection by H5N2 highly pathogenic avian influenza viruses in healthy wild waterfowl. PLoS Pathogens 4:e1000127. doi: 10.1371/journal.ppat.1000127
Gerasimov, N. N., and Gerasimov, Y. N. (1998). The international significance of wetland habitats in the lower Moroshechnaya river, West Kamchatka. Studies 10, 237–242.
Gilbert, M., Newman, S. H., Takekawa, J. Y., Loth, L., Biradar, C., Prosser, D. J., et al. (2010). Flying over an infected landscape: distribution of highly pathogenic avian influenza H5N1 risk in South Asia and satellite tracking of wild waterfowl. EcoHealth 7, 448–458. doi: 10.1007/s10393-010-0672-8
Gilbert, M., Prosser, D. J., Zhang, G., Artois, J., Saharan Dhingra, M., Tildesley, M., et al. (2017). Could changes in the agricultural landscape of northeastern China influence the long-distance transmission of HPAI H5Nx viruses? Front. Vet. Sci. 4:225. doi: 10.3389/fvets.2017.00225
Gonzalez-Reiche, A. S., Müller, M. L., Ortiz, L., Cordón-Rosales, C., and Perez, D. R. (2016). Prevalence and diversity of low pathogenicity avian influenza viruses in wild birds in Guatemala, 2010–2013. Avian Dis. 60, 359–364. doi: 10.1637/11130-050715-Reg
Gosler, A. (2004). “Birds in the hand,” in Bird Ecology and Conservation: A Handbook of Techniques, eds W. J. Sutherland, I. Newton, and R. E. Green, (Oxford, NY: Oxford Univeristy Press), 85–118.
Guan, Y., Peiris, J. S. M., Lipatov, A. S., Ellis, T. M., Dyrting, K. C., Krauss, S., et al. (2002). Emergence of multiple genotypes of H5N1 avian influenza viruses in Hong Kong SAR. Proc. Nat. Acad. Sci. 99, 8950–8955. doi: 10.1073/pnas.132268999
Guillemain, M., Pöysä, H., Fox, A. D., Arzel, C., Dessborn, L., Ekroos, J., et al. (2013). Effects of climate change on European ducks: what do we know and what do we need to know? Wildlife Biol. 19, 404–419. doi: 10.2981/12-118
Hill, N. J., Ma, E. J., Meixell, B. W., Lindberg, M. S., Boyce, W. M., and Runstadler, J. A. (2016). Transmission of influenza reflects seasonality of wild birds across the annual cycle. Ecol. Lett. 19, 915–925. doi: 10.1111/ele.12629
Hill, N. J., Takekawa, J. Y., Ackerman, J. T., Hobson, K. A., Herring, G., Cardona, C. J., et al. (2012a). Migration strategy affects avian influenza dynamics in mallards (Anas platyrhynchos). Mol. Ecol. 21, 5986–5999. doi: 10.1111/j.1365-294X.2012.05735.x
Hill, N. J., Takekawa, J. Y., Cardona, C. J., Ackerman, J. T., Schultz, A. K., Spragens, K. A., et al. (2010). Waterfowl ecology and avian influenza in California: do host traits inform us about viral occurrence? Avian Dis. 54, 426–432. doi: 10.1637/8912-043009-Reg.1
Hill, N. J., Takekawa, J. Y., Cardona, C. J., Meixell, B. W., Ackerman, J. T., Runstadler, J. A., et al. (2012b). Cross-seasonal patterns of avian influenza virus in breeding and wintering migratory birds: a flyway perspective. Vector-Borne Zoonot. Dis. 12, 243–253. doi: 10.1089/vbz.2010.0246
Hupp, J. W., Yamaguchi, N., Flint, P. L., Pearce, J. M., Tokita, K. I., Shimada, T., et al. (2011). Variation in spring migration routes and breeding distribution of Northern Pintails Anas acuta that winter in Japan. J. Avian Biol. 42, 289–300. doi: 10.1111/j.1600-048X.2011.05320.x
Jeong, J., Kang, H. M., Lee, E. K., Song, B. M., Kwon, Y. K., Kim, H. R., et al. (2014). Highly pathogenic avian influenza virus (H5N8) in domestic poultry and its relationship with migratory birds in South Korea during 2014. Vet. Microbiol. 173, 249–257. doi: 10.1016/j.vetmic.2014.08.002
Jiang, L., Deng, X., and Seto, K. C. (2013). The impact of urban expansion on agricultural land use intensity in China. Land Use Policy 35, 33–39. doi: 10.1016/j.landusepol.2013.04.011
Kear, J. (2005). Ducks, geese and swans: species accounts (Cairina to Mergus). Vol. 2. Oxford: Oxford University Press.
Keating, K. A., and Cherry, S. (2009). Modeling utilization distributions in space and time. Ecology 90, 1971–1980. doi: 10.1890/08-1131.1
Lee, D., Bertran, K., Kwon, J., and Swayne, D. E. (2017). Evolution, global spread, and pathogenicity of highly pathogenic avian influenza H5Nx clade 2.3.4.4. J. Vet. Sci. 18, 269–280. doi: 10.4142/jvs.2017.18.S1.269
Liu, D., Shi, W., Shi, Y., Wang, D., Xiao, H., Li, W., et al. (2013). Origin and diversity of novel avian influenza A H7N9 viruses causing human infection: phylogenetic, structural, and coalescent analyses. Lancet 381, 1926–1932. doi: 10.1016/S0140-6736(13)60938-1
Liu, Z., Li, Z., Tang, P., Li, Z., Wu, W., Yang, P., et al. (2013). Change analysis of rice area and production in China during the past three decades. J. Geogr. Sci. 23, 1005–1018. doi: 10.1007/s11442-013-1059-x
Lupiani, B., and Reddy, S. M. (2009). The history of avian influenza. Comp. Immunol. Microbiol. Infect. Dis. 32, 311–323. doi: 10.1016/j.cimid.2008.01.004
Lyecett, S. J., Bodewes, R., Pohlmann, A., Banks, J., Bányai, K., and Boni, M. F. (2016). Role for migratory wild birds in the global spread of avian influenza H5N8. Science 354, 213–217. doi: 10.1126/science.aaf8852
Mackinnon, J., and Phillipps, K. (2000). A Field Guide to the Birds of China. New York, NY: Oxford University Press Inc.
MacKinnon, J., Verkuil, Y. I., and Murray, N.(eds.). (2012). “IUCN situation analysis on East and Southeast Asian intertidal habitats, with particular reference to the Yellow Sea (including the Bohai Sea),” in Occasional Paper of the IUCN Species Survival Commission No. 47. Gland and Cambridge: IUCN.
Martin, V., Pfeiffer, D. U., Zhou, X., Xiao, X., Prosser, D. J., Guo, F., et al. (2011). Spatial distribution and risk factors of highly pathogenic avian influenza (HPAI) H5N1 in China. PLoS Pathogens 7:e1001308. doi: 10.1371/journal.ppat.1001308
Melville, D. S., Chen, Y., and Ma, Z. (2016). Shorebirds along the Yellow Sea coast of China face an uncertain future—A review of threats. Emu-Austral Ornithol. 116, 100–110. doi: 10.1071/MU15045
Meyer, A., Dinh, T. X., Nhu, T. V., Pham, L. T., Newman, S., Nguyen, T. T. T., et al. (2017). Movement and contact patterns of long-distance free-grazing ducks and avian influenza persistence in Vietnam. PLoS ONE 12:e0178241. doi: 10.1371/journal.pone.0178241
Miller, M. R., Takekawa, J. Y., Fleskes, J. P., Orthmeyer, D. L., Casazza, M. L., and Perry, W. M. (2005). Spring migration of Northern Pintails from California's Central Valley wintering area tracked with satellite telemetry: routes, timing, and destinations. Can. J. Zool. 83, 1314–1332. doi: 10.1139/z05-125
Murray, N. J., Clemens, R. S., Phinn, S. R., Possingham, H. P., and Fuller, R. A. (2014). Tracking the rapid loss of tidal wetlands in the Yellow Sea. Front. Ecol. Environ. 12, 267–272. doi: 10.1890/130260
Murray, N. J., Ma, Z., and Fuller, R. A. (2015). Tidal flats of the Yellow Sea: a review of ecosystem status and anthropogenic threats. Austral Ecol. 40, 472–481. doi: 10.1111/aec.12211
Muzaffar, S. B., Takekawa, J. Y., Prosser, D. J., Newman, S. H., and Xiao, X. (2010). Rice production systems and avian influenza: interactions between rice, poultry and wild birds. Waterbirds 33, 219–230. doi: 10.1675/063.033.s116
Nemeth, N. M, Brown, J. D., Stallknecht, D. E., Howerth, E. W., Newman, S. H., and Swayne, D. E (2013). Experimental infection of bar-headed geese (Anser indicus) and ruddy shelducks (Tadorna ferruginea) with a clade 2.3.2 H5N1 highly pathogenic avian influenza virus. Vet. Pathol. 50, 961–970. doi: 10.1177/0300985813490758
Newman, S. H., Hill, N. J., Spragens, K. A., Janies, D., Voronkin, I. O., Prosser, D. J., et al. (2012). Eco-virological approach for assessing the role of wild birds in the spread of avian influenza H5N1 along the Central Asian Flyway. PLoS ONE 7:e30636. doi: 10.1371/journal.pone.0030636
Nguyen, D. T., Bryant, J. E., Davis, C. T., Nguyen, L. V., Pham, L. T., Loth, L., et al. (2014). Prevalence and distribution of avian influenza a (H5N1) virus clade variants in live bird markets of Vietnam, 2011–2013. Avian Dis. 58, 599–608. doi: 10.1637/10814-030814-Reg
OIE (2017). Update on Highly Pathogenic Avian Influenza in Animals: Type H5 and H7. Available online at: http://www.oie.int/animal-health-in-the-world/update-on-avian-influenza/2017/ (Accessed December 5, 2017).
Palm, E. C., Newman, S. H., Prosser, D. J., Xiao, X., Ze, L., Batbayar, N., et al. (2015). Mapping migratory flyways in Asia using dynamic Brownian bridge movement models. Movement Ecol. 3:3. doi: 10.1186/s40462-015-0029-6
Pearce, J. M., Reeves, A. B., Ramey, A. M., Hupp, J. W., Ip, H. S., Bertram, M., et al. (2011). Interspecific exchange of avian influenza virus genes in Alaska: the influence of trans-hemispheric migratory tendency and breeding ground sympatry. Mol. Ecol. 20, 1015–1025. doi: 10.1111/j.1365-294X.2010.04908.x
Pei, Q., Zhang, D. D., and Lee, H. F. (2016). Contextualizing human migration in different agro-ecological zones in ancient China. Quat. Int. 426, 65–74. doi: 10.1016/j.quaint.2015.12.007
Peiris, J. M., Cowling, B. J., Wu, J. T., Feng, L., Guan, Y., Yu, H., et al. (2016). Interventions to reduce zoonotic and pandemic risks from avian influenza in Asia. Lancet Infecti. Dis. 16, 252–258. doi: 10.1016/S1473-3099(15)00502-2
Peng, H. B., Anderson, G. Q., Chang, Q., Choi, C. Y., Chowdhury, S. U., Clark, N. A., et al. (2017). The intertidal wetlands of southern Jiangsu Province, China–globally important for Spoon-billed Sandpipers and other threatened waterbirds, but facing multiple serious threats. Bird Conserv. Int. 27, 305–322. doi: 10.1017/S0959270917000223
Potvin, D. A., Välimäki, K., and Lehikoinen, A. (2016). Differences in shifts of wintering and breeding ranges lead to changing migration distances in European birds. J. Avian Biol. 47, 619–628. doi: 10.1111/jav.00941
Prosser, D. J., Cui, P., Takekawa, J. Y., Tang, M., Hou, Y., Collins, B. M., et al. (2011). Wild bird migration across the Qinghai-Tibetan Plateau: a transmission route for highly pathogenic H5N1. PLoS ONE 6:e17622. doi: 10.1371/journal.pone.0017622
Prosser, D. J., Ding, C., Erwin, R. M., Mundkur, T., Sullivan, J. D., and Ellis, E. C. (2018). Species distribution modeling in regions of high need and limited data: waterfowl of China. Avian Res. 9:7. doi: 10.1186/s40657-018-0099-4
Prosser, D. J., Hungerford, L. L., Erwin, R. M., Ottinger, M. A., Takekawa, J. Y., Newman, S. H., et al. (2016). Spatial modeling of wild bird risk factors for highly pathogenic A(H5N1) avian influenza virus transmission. Avian Dis. 60:329–336. doi: 10.1637/11125-050615-Reg
R Core Team (2017). R: A Language and Environment for Statistical Computing. Vienna: R Foundation for Statistical Computing. Available online at: https://www.R-project.org/
Ramey, A. M., Pearce, J. M., Flint, P. L., Ip, H. S., Derksen, D. V., Franson, J. C., et al. (2010). Intercontinental reassortment and genomic variation of low pathogenic avian influenza viruses isolated from Northern Pintails (Anas acuta) in Alaska: examining the evidence through space and time. Virology 401, 179–189. doi: 10.1016/j.virol.2010.02.006
Rogers, D. I., Yang, H.-Y., Hassell, C. J., Boyle, A. N., Rogers, K. G., Chen, B., et al. (2010). Red Knots (Calidris canutus piersmai and C.c.rogersi) depend on a small threatened staging area in Bohai Bay, China. Emu 110, 307–315. doi: 10.1071/MU10024
Saito, T., Tanikawa, T., Uchida, Y., Takemae, N., Kanehira, K., and Tsunekuni, R. (2015). Intracontinental and intercontinental dissemination of Asian H5 highly pathogenic avian influenza virus (clade 2.3.4.4) in the winter of 2014–2015. Rev. Med. Virol. 25, 388–405. doi: 10.1002/rmv.1857
Sandberg, R., and Moore, F. R. (1996). Fat stores and arrival on the breeding grounds: reproductive consequences for passerine migrants. Oikos 77, 577–581. doi: 10.2307/3545949
Shimada, T., Yamaguchi, N. M., Hijikata, N., Hiraoka, E., Hupp, J. W., Flint, P. L., et al. (2014). Satellite tracking of migrating Whooper Swans Cygnus cygnus wintering in Japan. Ornithol. Sci. 13, 67–75. doi: 10.2326/osj.13.67
Shin, J. H., Lee, K. S., Kim, S. H., Hwang, J. K., Woo, C., Kim, J., et al. (2015). Tracking mallards (Anas platyrhynchos) with GPS satellite transmitters along their migration route through Northeast Asia. Avian Dis. 60, 311–315. doi: 10.1637/11096-042015-Reg
Sims, L. D., Ellis, T. M., Liu, K. K., Dyrting, K., Wong, H., Peiris, M., et al. (2003). Avian influenza in Hong Kong 1997–2002. Avian Dis. 47, 832–838. doi: 10.1637/0005-2086-47.s3.832
Spackman, E., Prosser, D. J., Pantin-Jackwood, M. J., Berlin, A. M., and Stephens, C. B. (2017). The pathogenesis of Clade 2.3.4.4 H5 highly pathogenic avian influenza viruses in ruddy duck (Oxyura jamaicensis) and lesser scaup (Aythya affinis). J Wildl Dis. 53, 832–842. doi: 10.7589/2017-01-003
Sturm-Ramirez, K. M., Ellis, T., Bousfield, B., Bissett, L., Dyrting, K., Rehg, J. E., et al. (2004). Reemerging H5N1 influenza viruses in Hong Kong in 2002 are highly pathogenic to ducks. J. Virol. 78, 4892–4901. doi: 10.1128/JVI.78.9.4892-4901.2004
Szabo, J. K., Battley, P. F., Buchanan, K. L., and Rogers, D. I. (2016). What does the future hold for shorebirds in the East Asian–Australasian Flyway? Emu 116, 95–99. doi: 10.1071/MUv116n2_ED
Takekawa, J. Y., De La Cruz, S. W., Wilson, M. T., Palm, E. C., Yee, J., Nysewander, D. R., et al. (2011). Breeding distribution and ecology of Pacific coast Surf Scoters. Boreal birds of North America: a hemispheric view of their conservation links and significance. Stud. Avian Biol. 41, 41–64.
Takekawa, J. Y., Newman, S. H., Xiao, X., Prosser, D. J., Spragens, K. A., Palm, E. C., et al. (2010b). Migration of waterfowl in the East Asian flyway and spatial relationship to HPAI H5N1 outbreaks. Avian Dis. 54, 466–476. doi: 10.1637/8914-043009-Reg.1
Takekawa, J. Y., Prosser, D. J., Newman, S. H., Muzaffar, S. B., Hill, N. J., Yan, B., et al. (2010a). Victims and vectors: highly pathogenic avian influenza H5N1 and the ecology of wild birds. Avian Biol. Res. 3, 51–73. doi: 10.3184/175815510X12737339356701
Trierweiler, C., Klaassen, R. H., Drent, R. H., Exo, K. M., Komdeur, J., Bairlein, F., et al. (2014). Migratory connectivity and population-specific migration routes in a long-distance migratory bird. Proc. R. Soc. Lond. B Biol. Sci. 281:20132897. doi: 10.1098/rspb.2013.2897
van den Brand, J. M., Verhagen, J. H., Kroeze, E. J. V., Bildt, M. W., Bodewes, R., Herfst, S., et al. (2018). Wild ducks excrete highly pathogenic avian influenza virus H5N8 (2014–2015) without clinical or pathological evidence of disease. Emerg. Microbes Infect. 7:67. doi: 10.1038/s41426-018-0070-9
van Wijk, R. E., Kölzsch, A., Kruckenberg, H., Ebbinge, B. S., Müskens, G. J., and Nolet, B. A. (2012). Individually tracked geese follow peaks of temperature acceleration during spring migration. Oikos 121, 655–664. doi: 10.1111/j.1600-0706.2011.20083.x
Webster, M. S., Marra, P. P., Haig, S. M., Bensch, S., and Holmes, R. T. (2002). Links between worlds: unraveling migratory connectivity. Trends Ecol. Evol. 17, 76–83. doi: 10.1016/S0169-5347(01)02380-1
Wood, C., Qiao, Y., Li, P., Ding, P., Lu, B., and Xi, Y. (2010). Implications of rice agriculture for wild birds in China. Waterbirds 33 (Suppl. 1), 30–43. doi: 10.1675/063.033.s103
WWF-Hong Kong (2017). Mai Po Nature Reserve. Available online at: http://www.wwf.org.hk/en/whatwedo/water_wetlands/mai_po_nature_reserve/ (Accessed June 18, 2018).
Xia, S., Yu, X., Millington, S., Liu, Y., Jia, Y., Wang, L., et al. (2017). Identifying priority sites and gaps for the conservation of migratory waterbirds in China's coastal wetlands. Biol. Conserv. 210, 72–82. doi: 10.1016/j.biocon.2016.07.025
Xu, X., Subbarao, K., Cox, N. J., and Guo, Y. (1999). Genetic characterization of the pathogenic influenza A/Goose/Guangdong/1/96 (H5N1) virus: similarity of its hemagglutinin gene to those of H5N1 viruses from the 1997 outbreaks in Hong Kong. Virology 261, 15–19. doi: 10.1006/viro.1999.9820
Yamaguchi, N., Hiraoka, E., Fujita, M., Hijikata, N., Ueta, M., Takagi, K., et al. (2008). Spring migration routes of mallards (Anas platyrhynchos) that winter in Japan, determined from satellite telemetry. Zool. Sci. 25, 875–881. doi: 10.2108/zsj.25.875
Yamaguchi, N., Hupp, J. W., Higuchi, H., Flint, P. L., and Pearce, J. M. (2010). Satellite-tracking of Northern Pintail Anas acuta during outbreaks of the H5N1 virus in Japan: implications for virus spread. Ibis 152, 262–271. doi: 10.1111/j.1474-919X.2010.01010.x
Yang, H.-Y., Chen, B., Barter, M., Piersma, T., Zhou, C.-F., Li, F., et al. (2011). Impacts of tidal land reclamation in Bohai Bay, China: ongoing losses of critical Yellow Sea waterbird staging and wintering sites. Bird Conserv. Int. 21, 241–259. doi: 10.1017/S0959270911000086
Keywords: migration, stopover, avian influenza virus, H5N1, waterfowl, East Asian-Australasian Flyway
Citation: Sullivan JD, Takekawa JY, Spragens KA, Newman SH, Xiao X, Leader PJ, Smith B and Prosser DJ (2018) Waterfowl Spring Migratory Behavior and Avian Influenza Transmission Risk in the Changing Landscape of the East Asian-Australasian Flyway. Front. Ecol. Evol. 6:206. doi: 10.3389/fevo.2018.00206
Received: 06 July 2018; Accepted: 16 November 2018;
Published: 04 December 2018.
Edited by:
Nichola J. Hill, Tufts University, United StatesReviewed by:
Jose A. Masero, Universidad de Extremadura, SpainInger Suzanne Prange, Ohio University, United States
Copyright © 2018 Sullivan, Takekawa, Spragens, Newman, Xiao, Leader, Smith and Prosser. This is an open-access article distributed under the terms of the Creative Commons Attribution License (CC BY). The use, distribution or reproduction in other forums is permitted, provided the original author(s) and the copyright owner(s) are credited and that the original publication in this journal is cited, in accordance with accepted academic practice. No use, distribution or reproduction is permitted which does not comply with these terms.
*Correspondence: Diann J. Prosser, ZHByb3NzZXJAdXNncy5nb3Y=
†Present Address: Bena Smith, WWT Consulting, Slimbridge, United Kingdom