- CBGP, Montpellier SupAgro, INRA, CIRAD, IRD, Univ. Montpellier, Montpellier, France
Phytoseiidae mites are efficient predators, able to control pest mites and small arthropods in crops all over the world, using three biological control strategies: (i) augmentation, (ii) classical, and (iii) conservation. This paper focuses on the latter strategy. Most of those predatory mite species are generalist predators; they are naturally present in agro-ecosystems both on crops and adjacent natural vegetation. Because of such characteristics, their occurrence is usually associated with the use of fewer pesticides, providing relief to ecosystem services. As a first baseline for managing their occurrence in agro-ecosystems, a review of the present knowledge of plants and predatory mite interactions and predator dispersal ability is proposed. In addition, based on the author's own occurrence database, the study aims at analyzing (i) plant traits and the potential co-evolutionary relationships between plants and predatory mite species and (ii) how this can be used to forecast favorable plants to key predatory mites. For this, some examples were taken, i.e., vine and citrus crops, and three species, Kampimodromus aberrans, Euseius stipulates, and Typhlodromus (Typhlodromus) pyri. The main conclusion is that the occurrence database can help in determining the probability of finding predatory mite species on crops and non-crop plants. However, because some elements are lacking, especially predatory mite density, plant traits and the true overall distribution, it is currently, difficult to associate plant traits and plant phylogeny to Phytoseiidae diversity. Additional meta-analyses in collaboration with plant specialists would be required. Finally, the paper presents some examples of agroecosystem management at different scales (intercropping, agroforestry, borders management, landscape).
Introduction
Current agricultural practices are increasingly questioned and face two major worldwide challenges to (i) increase crop yields for feeding more humans on the planet, (ii) provide more quality food to address health concerns and (iii) avoid pollution for resource sustainability. In this context, crop protection, especially pesticide use, is an increasing threatening practice. However, crop protection is still necessary to ensure food quality and sufficiently high yield (Oerke, 2006). Several measures have been taken in several countries to limit the use of pesticides. For instance, the European Directive 2009/128/EC (https://eur-lex.europa.eu/eli/dir/2009/128/2009-11-25) aims at achieving the sustainable use of pesticides, reducing health risks and promoting the use of integrated pest management and other alternative plant protection strategies. National plans should be implemented to cope with such objectives (i.e., training of professionals, requirements for the sale of pesticides, pesticide application equipment). In France, the Ecophyto plan aims to halve the application of pesticides by 2025.
Biological control is considered a key solution to control arthropods (Altieri, 1999; Power, 2010). This paper exclusively focuses on predatory mites belonging to the Phytoseiidae family and the term predatory mites is used throughout the text to refer to mites of this family. These predators are used to control mite pests as well as small insects (McMurtry and Croft, 1997; Gerson et al., 2003). The Phytoseiidae family contains more than 2,400 species worldwide (Demite et al., 2018), used in the three biological control strategies. First, the classical biological control aims at controlling invasive pests, introducing natural enemies in the targeted area, from the pest origin zone. These measures are usually expensive and are funded by the government. One example is the successful introduction of Typhlodromalus aripo De Leon, to control Mononychellus tanajoa (Bondar) in Africa (Yaninek et al., 1989). The second strategy, augmentative biological control, consists of a mass release of natural enemies (exotic or endemic) in crops. The natural enemies are usually commercialized by private companies, and producers have to buy them for releasing. These natural enemies are usually specific to their prey and this strategy is essentially applied in greenhouses. One example is the mass-releases of Phytoseiulus persimilis Athias-Henriot in greenhouses, with a world market corresponding to 12% of the total natural enemy market (Van Lenteren, 2006). Finally, the third strategy, conservation biological control, consists of enhancing the occurrence of natural enemies in the agro-ecosystem, through its management (Letourneau et al., 2011; Ratnadass et al., 2012). This biological control strategy applies mainly to controlling endemic pests, essentially in open fields and perennial crops. These studies are usually funded by governmental measures as no economic benefits are expected for private companies. I will focus on this latter biological control strategy, as it is the most promising in the context of agro-environmental management. Furthermore, most predatory mites are food-generalists (able to feed on several prey and also on plant exudates, pollen, and fungi) and they are naturally present in agro-ecosystems, both on crops and adjacent natural vegetation. Such characteristics make them good candidates for providing ecosystem services as natural enemies of pest species, resulting in the reduction of pesticide applications (Prischmann et al., 2006; McMurtry et al., 2015).
For conservation biological control implementation, an integrated knowledge of predatory mite biodiversity is required to determine factors affecting the presence of these natural enemies in reservoir zones and their dispersal between those reservoirs and crops. First, the available knowledge on plant and predatory mite interactions and their dispersal abilities was reviewed. Then, based on the present occurrence knowledge, new approaches to forecast predator occurrence on plants are proposed, assuming that this occurrence is related to plant traits and potential co-evolutionary relationships between plants and mites. Finally, examples of the impact of agro-ecosystem management on predatory mite occurrence are provided for several scales (i) within the crops, (ii) in the near borders of the crops and (iii) at a landscape scale.
Plants as Reservoir Zones for Predatory Mites
Most predatory mites of the Phytoseiidae family are not dependent on a given prey species as they are food-generalists (McMurtry et al., 2013, 2015). However, they are greatly affected by plant characteristics, especially the phylloplan structure (Karban et al., 1995). Some authors reported that the phylloplan (phenotypic characteristics of the leaf surface) features impacted predatory mite densities more than prey availability (Duso, 1992; Karban et al., 1995; Duso et al., 2004b). McMurtry et al. (2013) divided the Phytoseiidae family into four main categories depending on feeding habits: (i) specialized, (ii) selective, (iii) generalists, and (iv) pollen feeders.
Domatia and leaf hairiness are the most documented plant features affecting mite behavior and biology. Schmidt (2014) provided an excellent review of these relationships. The hypotheses proposed to explain such relations are: (i) escape from cannibalism and predation, (ii) maintaining favorable conditions of humidity, especially for egg survival and (iii) pollen retention and alternative food presence in domatia. However, some structures are not favorable to predatory mites, i.e., glandular trichomes of Solanaceae reportedly trap the predators and hinder their movement. The structures affecting predatory mites are not totally characterized. A few studies focused for instance on vein height, presence of nectaries, types of trichomes and domatia. Prischmann et al. (2005a) hypothesized that old galls of Eriophyid mites could serve as refugia for predatory mites. Some authors demonstrated that extrafloral nectaries positively impacted the development of predatory mites (Walter, 1996; van Rijn and Tanigoshi, 1999). Weber et al. (2016) showed that the artificial addition of sugar (imitation of nectaries) on Vitis riparia and Vitis munsoniana enhanced the densities of fungivorous mites (some Phytoseiidae and many Tydeiidae), with an associated negative impact on powdery mildew.
Pollen can also affect predatory mite densities. Some of them even develop better on pollen than on prey (Flechtman and McMutry, 1992). However, a few studies focused on the relationship between predatory mites and the pollen of the plants on which these predators occur. Such a relationship was suggested for citrus (Kennett et al., 1979; Grout and Richards, 1992), avocado (McMurtry and Johnson, 1965; Maoz et al., 2008; Gonzalez-Fernandez et al., 2009) and grapevine (Duso and Vettorazzo, 1999). Daud (2003) studied the effect of pollen of Mabea fistulifera Mart. (Euphorbiaceae) on Euseius citrifolius Denmark and Muma, the main species found on this plant. He showed that M. fistulifera pollen was particularly suitable for E. citrifolius. However, a direct link between predatory mite species and pollen produced by the plants, where these species occur, is not totally evident. The pollen of birch is suitable for the development of Kampimodromus aberrans (Oudemans), whereas this species was never recorded on this plant (Kasap, 2005). The pollen of Typha sp. is currently used for rearing various predatory mites and is sometimes spread in crops to increase the densities of those predators. However, only few species were recorded on Typha sp. and the species known to feed on this pollen, were not reported on this plant (i.e., Amblyseius swirskii Athias-Henriot, Euseius stipulatus (Athias-Henriot) (Maoz et al., 2014; Beltrà et al., 2017). Predatory mite densities in vineyards in Italy, were correlated with the amount of Poaceae pollen found on vine leaves. This correlation was attributed to the great quantity of Poaceae in cover crops (Duso et al., 2009). Karban et al. (1995) assumed to find higher predatory mite densities on male than on female plants because of pollen production. However, such a correlation was not observed.
Several studies also showed that plants with hairy leaves could trap pollen better than those with smooth leaves (i.e., Kreiter et al., 2002; Roda et al., 2003; Duso et al., 2004b). Roda et al. (2003) demonstrated that the hairier the apple leaves are, the higher the densities of Typhlodromus (Typhlodromus) pyri Scheuten are, because of a greater pollen and fungal spore quantity.
Some authors also reported that some predatory mite species could feed on plants. This was observed for several species, as T. (T.) pyri, Typhlodromus (Anthoseius) rhenanus (Oudemans), Euseius finlandicus (Oudemans), K. aberrans, T. aripo, Euseius scutalis (Athias-Henriot), and Euseius hibisci (Chant) (Chant, 1959; Porres et al., 1975; Kreiter et al., 2002; Magalhães and Bakker, 2002; Nomikou et al., 2003; Sengonca et al., 2004; Adar et al., 2012). For this latter species, evidence of plant feeding behavior was observed on avocado but not on citrus leaves, suggesting that plant characteristics affect plant-feeding behavior. Plant tissue might constitute an alternative food and a source of water but does not allow egg production because of its low nutritional value (Nomikou et al., 2003). Thus, it is not clear if those predators would feed on plants when other food sources exist.
The impact of plant compounds on the relationship between plants and predatory mites is not well-documented, except for the impact of VOC (volatile organic compounds) on specialist feeding predators, that are attracted by the compounds emitted by plants once attacked by pests (i.e., Van den Boom et al., 2002). Onzo et al. (2012) showed that cassava cultivars with pubescent leaves were more attractive to T. aripo than glabrous leaves. Ferrero et al. (2014) showed that Phytoseiulus longipes Evans was more attracted to clean tomatoes than clean beans, suggesting that plant volatile compounds could affect P. longipes behavior.
Relationships between plant and predatory mites are complex; all factors affecting their occurrence on plants are not known. There are more than 374,000 plant species (Christenhusz and Byng, 2016) and 2,400 predatory mite species (Demite et al., 2018). This huge number of partners clearly suggests complex and numerous interactions. In a framework of conservation biological control, knowing which plants are favorable to which predatory mites is a key step.
How to Determine the Favorable Plants for the Targeted Predatory Mite Species?
Information on the occurrence of the predatory mite species on plants is available from several publications related to faunistic surveys. The challenge is to retrieve, compile and analyse this information to determine (i) which predator species are present in crops and (ii) the probability of finding these species on non-crop plants.
Tixier et al. (data not publicly available) compiled this information from 1,959 publications, in a database containing 30,684 reports worldwide, of the 2,400 Phytoseiidae species, on 4,900 plant species in 228 countries. This database allows determining the predatory mite species reported for each plant and locality. As an example of the information extracted from the database, Table 1 shows the predatory mite species encountered all over the world on Convolvulus arvensis L., a common weed species. Twenty-five species belonging to 11 genera were recorded. Among them, 16 were reported once. The two species most frequently observed were P. persimilis and Typhlodromus (Typhlodromus) athiasae Porath and Swirski.
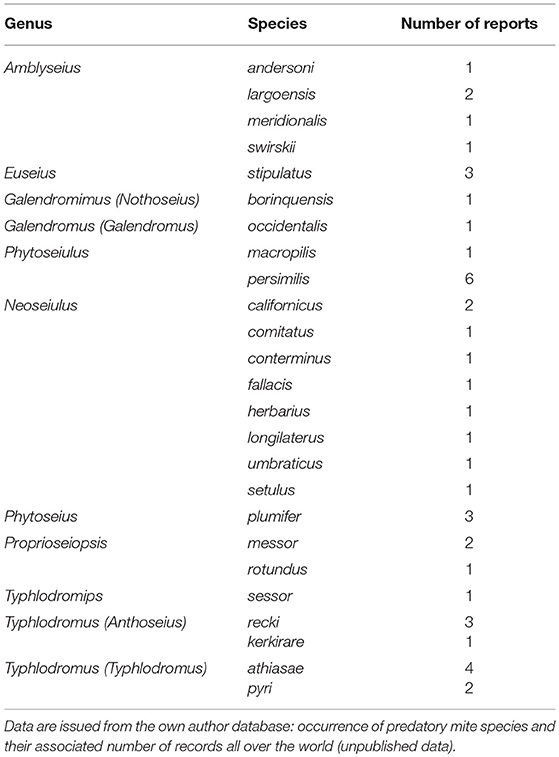
Table 1. Species of predatory mites (Acari: Phytoseiidae) and number of reports on Convolvulus arvensis L. around the world.
The objectives here are to use the information contained in this database to determine/forecast the probability of occurrence of predatory mite species in crops and in non-crop plants. Three approaches are proposed.
First, information will be analyzed to determine how the present predatory mite distribution could help to determine the species most likely to be found on two crops (vine and citrus) in different areas of the world. Then, for the most frequent predatory mite species, the non-crop plants more likely to host them will be investigated.
Second, because plant traits affect predatory mite diversity, an analysis of their occurrence in relation to some plant traits will be provided to determine how plant features can be indicators of the presence of some key predatory mite species.
Finally, because relationships between plants and predatory mites might have an evolutionary basis, an analysis of phylogenetic relationships of plants and associated predatory mite diversity will be provided to determine how plant family or genera could be used to forecast the predator species occurrence.
The common limit for these three approaches is that the densities of predatory mites are not always recorded in publications. Furthermore, the sampling methods and the way to report the results differ. We thus have to assume that the most frequently recorded species are also those found in the highest densities (which is not always the case, see below). The objective here is to propose new ways to investigate the relationships between plants and predatory mite species with an applied objective of conservation biological control: how to manage the plant biodiversity within the agro-ecosystems? This paper thus aims to propose the first baseline for further and deeper analyses. For this, I focused on two crops, vine and citrus (two perennial crops where augmentative biological control is poorly applied) and on generalist predators (Types III and IV) because they might be the most adapted to such a biological control strategy (perennial presence in agro-ecosystems and difficulty to rear the predatory mites for mass-releasing).
Assessing Predatory Mite Diversity Based on Recorded Fauna
Predatory Mites in Crops
Using the database cited before, the examples of two crops, Citrus sp. and Vitis vinifera, were studied.
Citrus Trees
Two hundred and ninety-seven predatory mite species belonging to 38 genera were found in 78 countries (1,231 records in total). On hundred and thirty-five species were recorded once and could be assumed to not play a key role in biological control. The same applies for 134 additional species reported between 2 and 9 times. Thus, among the 297 species, only 28 were found more than 10 times, corresponding to 594 reports in 57 countries (Table 2). The two most frequent species were E. stipulatus and Amblyseius largoensis (Muma). E. stipulatus prevailed in the Palearctic region along with Iphiseius degenerans (Berlese), T. (T.) athiasae, A. swirskii, and E. scutalis. In the Neotropical region, the two most frequent species were Euseius concordis (Chant) and Iphiseiodes zuluagai Denmark & Muma, whereas Amblydromalus limonicus (Garman & McGregor) and Typhlodromalus peregrinus (Muma) were prevalent in the Nearctic citrus fauna region. In the Oriental region, A. largoensis prevailed (20 reports) followed by Neoseiulus californicus (McGregor) and Chanteius contiguus (Chant). General conclusions for the Ethiopian and Australasian regions cannot be drawn because of the low number of records on citrus in these two regions. The analysis of this database therefore provides the probability to find predatory mite species in some world regions. As a consequence, because of the high probability to find E. stipulatus in Europe, crop management would aim to favor this species. More accurate information can also be extracted for countries or for more limited areas, depending on the number of existing reports (to ensure correct forecasting).
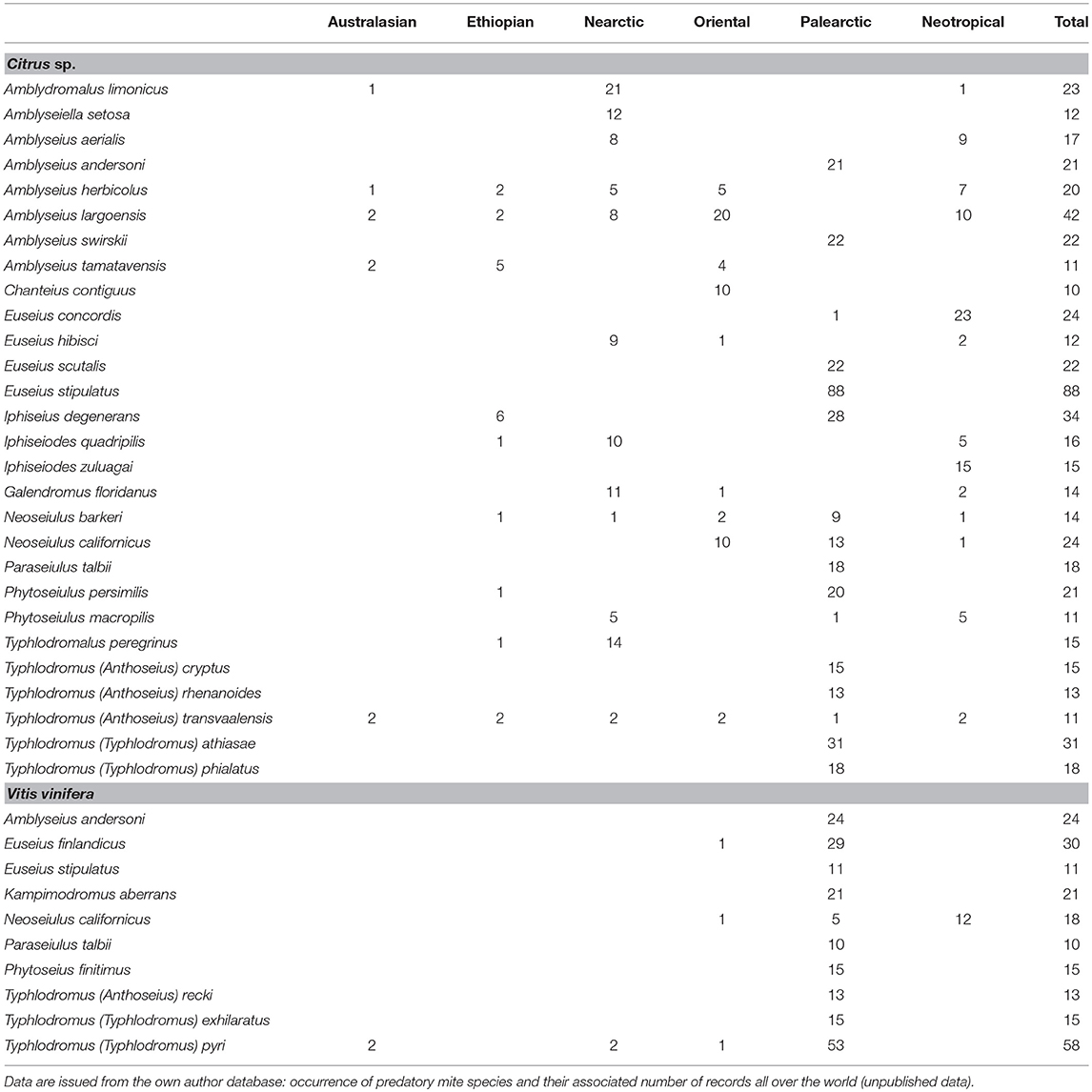
Table 2. The 28 and 10 most frequently reported predatory mite species on Citrus sp. and Vitis vinifera, respectively, and their number of reports in the six biogeographic regions.
Vineyards
One hundred and sixty-seven predatory mite species belonging to 31 genera were found in 40 countries all around the world (558 records in total). Eighty-eight species were recorded once and could be assumed to not play a key role in biological control. The same applies for 69 additional species retrieved <10 times (Table 2). Among the 167 predatory mites, only ten were reported more than 10 times. The four most frequent species were T. (T.) pyri, E. finlandicus, Amblyseius andersoni (Chant), and K. aberrans. These ten species essentially occurred in the Palearctic region, certainly because this region was the most sampled. In the other regions, because of a low number of surveys, it is difficult to forecast predatory mite species occurrence. Furthermore, the present analysis focused on large geographical zones. Yet, fauna diversity depends on the country and more limited geographic zones (Prischmann et al., 2002). In European vineyards, Tixier et al. (2013) showed that only five species were frequently observed (K. aberrans, T. (T.) pyri, T. (T.) exhilaratus, E. finlandicus, and Phytoseius finitimus Ribaga) and that their occurrence differed depending on countries and agricultural practices. For instance, the main species occurring in vineyards in the South of France was K. aberrans whereas T. (T.) pyri prevailed in vineyards in the North of France (Kreiter et al., 2000).
Predatory Mites on Non-crop Plants
The database can help in determining which of the non-crop plants reported the most frequent predatory mite species. Considering the previous examples and focusing mainly on the Palearctic region, the predatory species to be favored would be E. stipulatus in citrus orchards and K. aberrans and T. (T.) pyri in vineyards.
Euseius Stipulatus
Euseius stipulatus was reported on 131 plant species belonging to 102 genera and 54 families (339 reports). Among the 131 plant species, 35 are crops (171 records, 17 genera and 12 families). This species was reported 96 times on non-crop plants belonging to 83 genera and 47 families. The number of reports by plant species was usually low. The highest number of reports was observed on Ficus carica L. (8) and to a lesser extent on species of the genera Rubus, Clematis, Malva, Crataegus, Eriobotrya, Prunus, Quercus, Rosa, and Urtica (Table 3).

Table 3. Number of reports (and occurrence frequency in %) of Euseius stipulatus, Typhlodromus (Typhlodromus) pyri and Kampimodromus aberrans on genera and families of non-crop plants, wordwide.
Kampimodromus Aberrans
Kampimodromus aberrans was reported on 166 plant species belonging to 109 genera and 52 families (289 reports). Among the 166 plant species, 35 were crops (200 records, 19 genera, and 12 families). K. aberrans was reported 133 times on non-crop plants belonging to 86 genera and 45 families. The highest number of reports were observed on Corylus avellana L. (14 reports) and on plants of the genera Quercus, Acer, Celtis, Rubus and Ulmus (Table 3).
Typhlodromus (Typhlodromus) pyri
Typhlodromus (Typhlodromus) pyri was reported on 197 plant species belonging to 112 genera and 53 families (532 reports). Among the 197 plant species, 28 were crops (347 records, 12 genera, and 7 families). This species was recorded 168 times on non-crop plants (100 genera and 50 families). The highest number of reports was observed on plants of the genera Rubus, Quercus, and to a lesser extent Acer, Corylus, Ribes, Tilia, and Ulmus (Table 3).
Plants favorable to E. stipulatus are different to those favorable to K. aberrans and T. (T.) pyri, whereas plants, where these two latter species are the most often recorded, are quite similar (i.e., Ulmus sp., Acer sp., Rubus sp., Quercus sp., Corylus sp.). In an applied point of view, these data indicate that a similar/close agro-ecosystem management can favor both K. aberrans and T. (T.) pyri.
The use of the database allows forecasting some trends on the biodiversity assembling on crops and non-crop plants. However, some limits exist. Predatory mite biodiversity, even if greatly affected by plant characteristics, can also depend on other factors, especially agricultural practices. For instance, surveys carried out in apple orchards in France in 2012 showed that (i) three species prevailed [A. andersoni, T. (T.) pyri, and K. aberrans] and (i) fauna modification occurred as the main species reported 10 years ago, N. californicus, was hardly found in 2012 (Tixier et al., 2014). Fauna in crops can thus change over time, depending on cultural practices, especially pesticide application (Prischmann et al., 2005c). Another factor affecting predatory mite diversity is local climatic conditions. One such illustration is the different fauna on trees of Celtis australis L. planted 1 km apart, on dry soil or near a river (Tixier et al., 2005a, 2007). The main species found in the former condition was T. (T.) phialatus whereas the main species found in the latter condition was K. aberrans. Another limit is that the approach proposed herein is based on the frequency of predatory mite species but not on their abundance. The same weight is given to species found in high densities as to those found in low quantities. As stated before, information on abundance is not always provided in publications and sampling techniques differ depending on the surveys; thus this information is difficult to retrieve and compare. Finally, for forecasting approaches, the number of reports should be high enough. The examples studied before on the distribution of E. stipulatus, K. aberrans, and T. (T.) pyri, showed that it was impossible to provide the probability of occurrence in some areas because of the low number of reports. Clearly, information on predatory mite distribution is lacking, which makes the determination of an occurrence probability difficult.
A modeling approach, to determine a priori favorable plants, based on (i) plant traits, (ii) predatory mite traits, or (iii) taxa, will be therefore proposed in the following paragraphs.
Relationships Between Predatory Mites and Plant Traits for Forecasting Predator Distribution
This approach consists of determining how predatory mite occurrence can be explained by morphological plant and predator traits. For instance, the number of trichomes on leaves (>217 vs. < 217 trichomes/cm2) and the domatia structure (close vs. open) were a key feature discriminating between favorable and unfavorable plants to K. aberrans (Kreiter et al., 2002). Additionally, the main factors explaining high densities of K. aberrans on 11 trees were domatia numbers of the primary axil, domatia structure on the first and secondary veins and trichome densities on the primary vein (Tixier et al., 2005b). Similarly, there was a positive relationship between Typhlodromus (Anthoseius) caudiglans Schuster densities and vine leaf characteristics (density of vein hairs, bristles in leaf axils, and presence of leaf domatia) (Karban et al., 1995). Kreiter et al. (2002) proposed a correlation between trichome densities and predatory mite size.
The examples studied previously on citrus and vineyards showed that predatory mite species encountered on these two crops differ. As some authors (Kreiter et al., 2002) showed that phylloplan hairiness “select” predatory mite species based on their size (smaller predators on plants on hairy leaves and bigger predators on plants with glabrous leaves), we herein investigated how the species found on citrus and V. vinifera differ in body length. The female body length of the 297 and 167 species found on citrus and vine, respectively, was retrieved from original description and/or re-descriptions (data used by Tixier et al., 2012). The weighted mean of the body length of species recorded on citrus was 347.59 μm whereas that of species recorded on vine was 334.69 μm. The same trend was observed when only the main species found on these two crops were considered (28 species on citrus, 10 on vine). The weighted mean of the body length of the 28 predatory mite species reported on citrus was 342.6 μm whereas that of the 10 species mainly retrieved on vine was 322.15 μm. The relation between the frequency of the number of species and records according to body length range, also showed that species found on vine are usually smaller than those observed on citrus (Figures 1A,B). These trends can be associated to phylloplan hairiness, with a smooth surface being more favorable to bigger mites (Kreiter et al., 2002). For a prediction approach, species with a body length <330 μm might be more frequent on V. vinifera and those with a body length more than 330 μm might be more abundant on citrus. However, this result is only a trend, abundance of each species would certainly improve this forecasting.
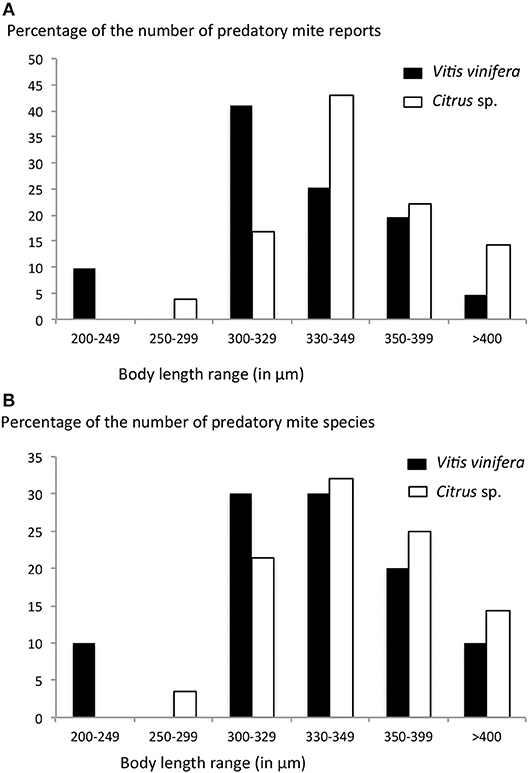
Figure 1. (A) Percentage of the numbers of reports of predatory mites and (B) percentage of the number of species of predatory mites reported on Citrus sp. and Vitis vinifera according to the their body length (range in μm). Data are issued from two own author databases: (i) occurrence of predatory mite species and their associated number of records all over the world (unpublished data), (ii) body lengths of the predatory mite species are issued from original descriptions and the data were those used in Tixier et al. (2012).
E. stipulatus, K. aberrans, and T. (T.) pyri were not reported on the same plant species. To determine how this distribution is associated to plant features, the non-crop plants where these species were reported were classified as follows: (i) low plants (herbaceous plants), (ii) medium high plant (shrubs) and high plants (trees). E. stipulatus was mainly recorded on herbaceous plants (41.9%) whereas T. (T.) pyri and K. aberrans were mainly reported on trees (36.8 and 56%, respectively) (Figure 2). These are only trends and clearly, information on densities would certainly allow for a more accurate determination of the non-crop plants favorable to K. aberrans, T. (T.) pyri, or E. stipulatus. However, based on these first trends, it seems that arboreal plants would be favorable to T. (T.) pyri and K. aberrans whereas grass would rather favor E. stipulatus. This information can be used for agro-ecosystem management: weed management might more affect the occurrence of E. stipulatus than that of the two other species.
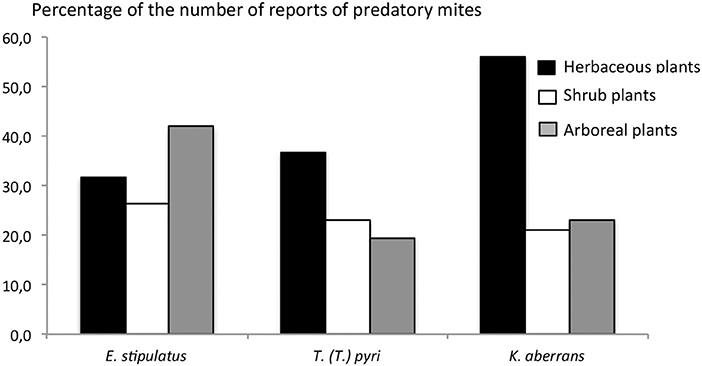
Figure 2. Percentage of the number of reports of Euseius stipulatus, Kampimodromus aberrans, and Typhlodromus (Typhlodromus) pyri recorded on herbaceous, shrubs and arboreal plants. Data are issued from the own author database: occurrence of predatory mite species and their associated number of records all over the world (unpublished data).
To further expand the study of the interaction between plant and predatory mite traits, more information is clearly needed. Collaboration with botanists and plant ecologists would allow more plant traits to be included, as public databases on plant characteristics are rare. Weber et al. (2015) published a database containing the number of species and genera with extrafloral nectaries (EFNs) for each plant family. These structures positively affect predatory mite in providing alternative food (Walter, 1996; van Rijn and Tanigoshi, 1999; Mayuko and Yano, 2008). From my own database, the number of predatory mite species and the number of their reports per plant family were compiled, to investigate a potential link between EFNs and predator occurrence. Few plant species were reported to harbor EFNs (4,017 plant species in 119 families) (http://www.extrafloralnectaries.org/the-list.html). The number of predatory mite reports on these 119 families is 17,899. No correlation was observed between the number of plants with EFNs in each plant family and the number of predatory mite species, nor with the number of predatory mite reports (Tables 4, 5). The correlation tests between the number of plant genera with EFNs, the % of species and genera with EFNs in each plant family and the number of predatory mite reports and species, were not significant either (Table 4). All the plants of a family where predatory mites were reported do not harbor EFNs, which can explain why these simple and global correlations were not significant (information compared was not sufficiently accurate). Furthermore, the biology of predatory mites differ according to species and genera considered. Additional studies considering the predatory mite species associated to plants with EFNs could thus be interesting. Finally, Weber et al. (2012, 2015) stated that the number of unreported cases of plants with EFNs might be as high as the number of species already reported, suggesting an incomplete knowledge of these plant traits. The correlations between the number of plant genera with EFNs and the number of plant genera hosting predatory mites, was also tested. The R2-value (0.35) seems to show that there is a relationship between the plants where predatory mites were reported and EFNs' occurrence. However, the correlation coefficient between the number of genera where predatory mites were found and the total number of genera per plant family was much higher (R2 = 0.64), suggesting that the more genera a family contains (diverse at genus level) the higher the probability to find predatory mite species is (Table 4). To more accurately determine how predatory mite occurrence is associated to EFNs' presence, I focused on the Adoxaceae family where two genera among four, contain EFNs: Sambucus and Viburnum (Table 6). Predatory mites were only reported on these two genera. On the seven Sambucus species where predatory mites were observed, four harbored EFNs (37 predatory mite records, 21 species). On the three Sambucus species without EFN, only a few numbers of records and predatory mite species were observed (3 reports, 3 species). Finally, on six Sambucus species with EFNs, no predatory mite was reported. On the 12 Viburnum species where predatory mites were observed, four harbor EFNs (17 records, 16 species). On the 8 Viburnum species without EFN, much more reports and predatory mite species were recorded (35 reports, 22 species). On 14 Viburnum species with EFNs, no predatory mite was reported. As noted in the previous global analyses, no clear association between EFNs and predatory mite occurrence was observed. However, some cues can be noted (i) EFNs were only present on the genera Sambucus and Viburnum within the family Adoxaceae, and predatory mites were only reported on these two genera as well, (ii) for the genus Sambucus more predatory mite species and reports were observed on plant species with EFNs. As no direct association between EFNs and predatory mite was observed, this seems to show that even if EFNs are favorable to predatory mites, other factors might explain their abundance and diversity on plants. Finally, I analyzed the occurrence of the three species previously studied [E. stipulatus, K. aberrans, and T. (T.) pyri] on the families with EFNs. On the 54 families where E. stipulatus was reported (339 records), 29 families contained plants with EFNs (136 records). On the 53 families where T. (T.) pyri was recorded (532 records), 18 families contained plants with EFNs (201 records). Finally, on the 52 families where K. aberrans was reported (289 records), 25 families contained plants with EFNs (126 records). Again, no clear association with these species and plants with or without EFNs was observed. Incomplete records of predatory mites, as well as lack of accurate information on plants traits can explain such results.
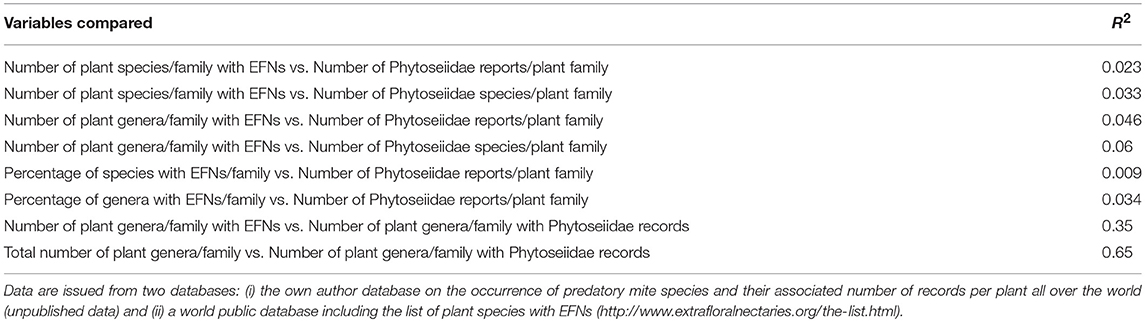
Table 4. Correlation coefficients between several variables concerning (i) the plants with extrafloral nectaries (EFNs) (number and percentage of plant species per family with EFNs, number and percentage of plant genera per family with EFNs, total number of plant genera per family, total number of plant genera per family with EFNs, number of plant genera per family where predatory mites were reported) and (ii) the number of predatory mite species (Phytoseiidae) and reports per plant family.
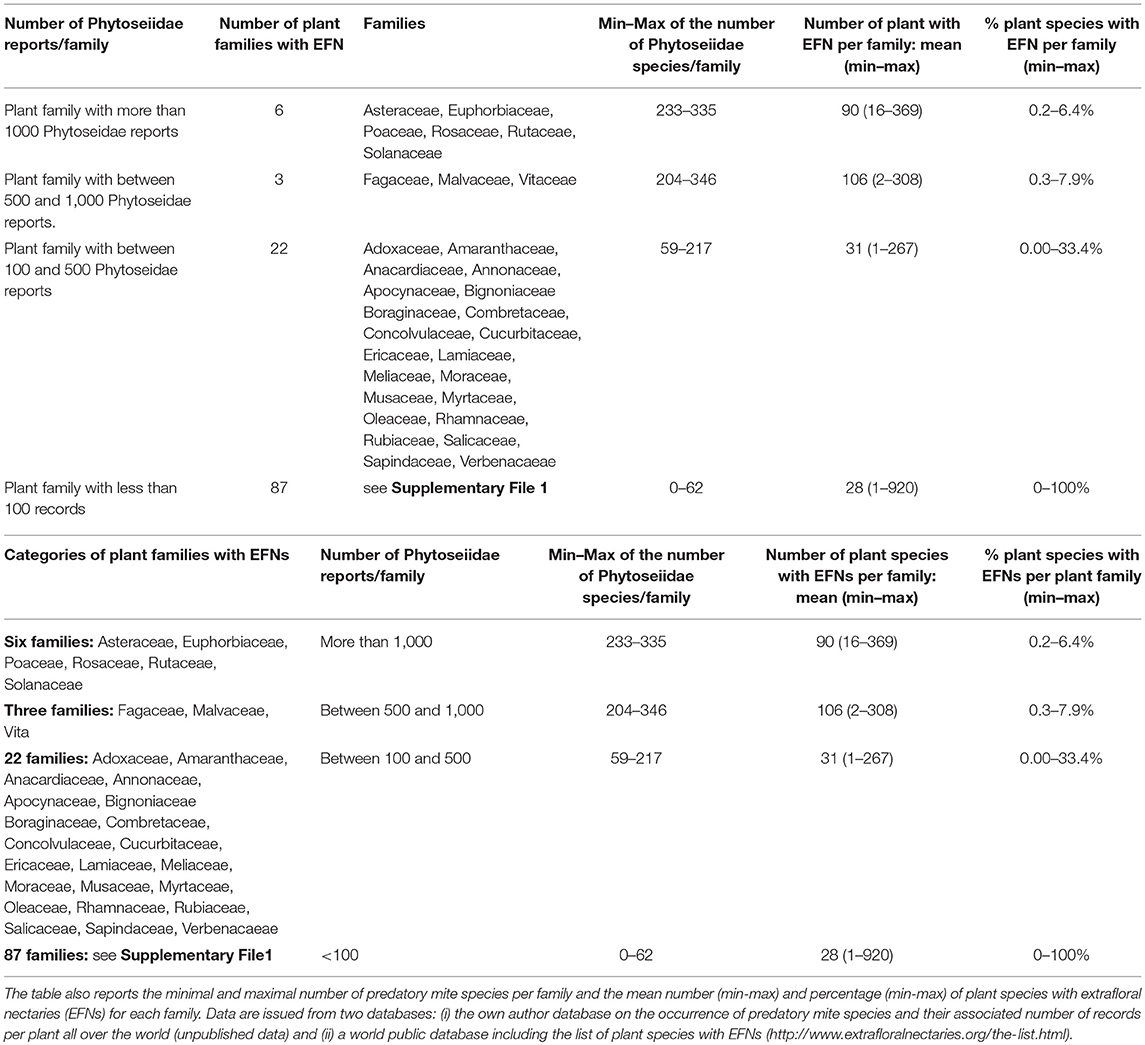
Table 5. Four categories of plant families with EFNs established according to the number of reports of predatory mites (Phytoseiidae) recorded on them.
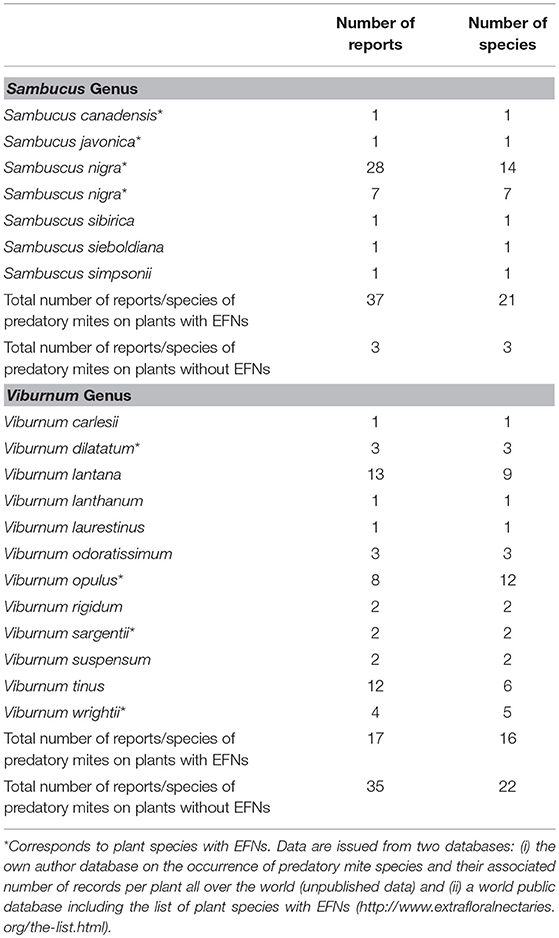
Table 6. Number of reports and number of species of predatory mites reported on plant species of the genera Sambucus and Viburnum (Adoxaceae) with and without extrafloral nectaries (EFNs).
Evolutionary Relationships Between Plants and Predatory Mites
The hypotheses are that (i) plants and predatory mites have a common evolutionary history and (ii) a relationship between plant and predatory mite phylogeny exists. This hypothesis, assuming that plant traits affecting predatory mites are not convergent characters, has not been totally tested yet. Karban et al. (1995) showed no effect of phylogenetic relationships of grape species on predatory mite densities. This latter study focused on densities and not on predatory mite diversity. No study to my knowledge investigated the relationship between plant phylogeny and predatory mite taxa. Weber et al. (2016) showed that 61% of the 87 plant families containing species with domatia were also reported to have species with EFNs, and that the two traits occurred non-randomly in the same clades across Eudicots. Weber et al. (2012), focusing on the genus Viburnum, revealed an EFNs and mite domatia co-occurrence and that these two traits were evolutionarily correlated. One can thus wonder how this plant evolution (and relationships) can affect the predatory mite diversity. Weber et al. (2012) showed an additive effect of habitat (domatia) and food (EFNs) on mite abundance (especially tydeid mites but also to a lesser extent Phytoseiidae). Accessing the database used by plant evolution/ecology specialists and cross-checking data on predatory mite occurrence, might provide elements to assess the relationship between plant and predatory mites.
As a first step of such a large future study, and considering only E. stipulatus, K. aberrans, and T. (T.) pyri, I investigated the evolutionary relationships between the plant genera/family where these three predatory mite species were mainly observed. Euseius sipulatus was the main species reported from Citrus sp. (family Rutaceae, superior taxa: Sapindales). Concerning non-crop plants, E. stipulatus was mainly reported on six plant families (Rosaceae, Moraceae, Urticaceae, Malvaceae, Ranunculaceae, Fagaceae) belonging to four superior taxa (Rosales, Malvales, Papaverales, Fagales) (Figure 3A). No taxonomic relationship was noted between Rutaceae and the other families where this species was mainly found. This can be explained by the fact that agricultural practices affect predatory mite occurrence more than the phylogenetic relations between plants and predators (i.e., Prischmann et al., 2005b). Considering non-crop plants, no clear phylogenetic relationship was observed between the different families and superior taxa when this species was mainly reported. Rosales and Fagales belong to Eurosids I, Malvales belong to Eurosids II whereas Ranunculales does not belong to Eu-Rosids. K. aberrans and T. (T.) pyri were the main species reported on V. vinifera in Europe (family Vitaceae, superior taxa: Vitales). No direct phylogenetic relationship between Vitales and the other plant taxa where these two species were reported were observed (Figures 3B,C). The same conclusions can thus be drawn: agricultural practices mostly affect predatory mite distribution comparing to evolutionary relationships between plants and predatory mites. Considering non-crop plants, K. aberrans mainly occurred on Rosales and Fagales. These taxa are included in the same clade; however, K. aberrans was also reported on Proteales and Sapindales and these two taxa belong to different lineages. The same conclusions can also be drawn for T. (T.) pyri, mainly reported from Rosales and Fagales on one hand and from two close taxa Malvales and Sapindales on the other hand. Finally, these results show that the three predatory mite species were mainly reported on Rosales and then on Fagales for K. aberrans and T. (T.) pyri and on Papaverales for E. stipulatus. As these three predatory mite species belong to different genera and sub-families, no clear phylogenetic relationships between plant and predatory mite evolution can be concluded.
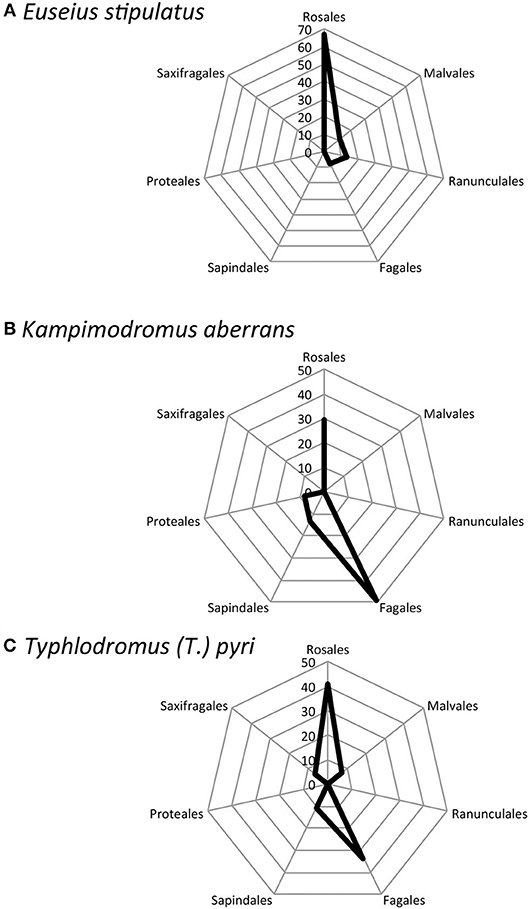
Figure 3. Percentage of the number of reports of (A) Euseius stipulatus, (B) Kampimodromus aberrans, and (C) Typhlodromus (Typhlodromus) pyri recorded on superior plant taxa. Data are issued from the own author database: occurrence of predatory mite species and their associated number of records all over the world (unpublished data). The “Plants database” (https://plants.usda.gov/classification.html) was used to associate the plant families, where predatory mites were found, to the plant orders.
Focusing on the 15 species included in the genus Kampimodromus, I investigated if species of a same genus are reported on phylogenetically related plant taxa. Table 7 shows the number of reports, the number of species found on plant families and superior taxa, as well as the number of plant genera where the 15 Kampimodromus species were observed. The highest number of reports and the highest number of Kampimodromus species and plant genera occupied, were observed on Rosales and Fagales. Rosales and Fabales belong to the clade EuRosids I and are evolutionary related. Then, Kampimodromus species are reported in two other plant groups neither phylogenetically related to each other, nor with Eu-Rosids I: Sapindales (EuRosids II) and Lamiales (Eu-Asterids I) (The Angiosperm Phylogeny Group, 2003). No clear relationship between phylogeny of Phytoseiidae and plant phylogeny was thus noted. However, all the biodiversity and distribution of predatory mites is not known; the phylogeny of the family Phytoseiidae is not stabilized. Furthermore, additional studies on other predatory mite genera could provide different results. At that state and focusing on the examples herein studied, it does not seem appropriate to use phylogenetic relations between plant and predatory mites to forecast their distribution on plants.
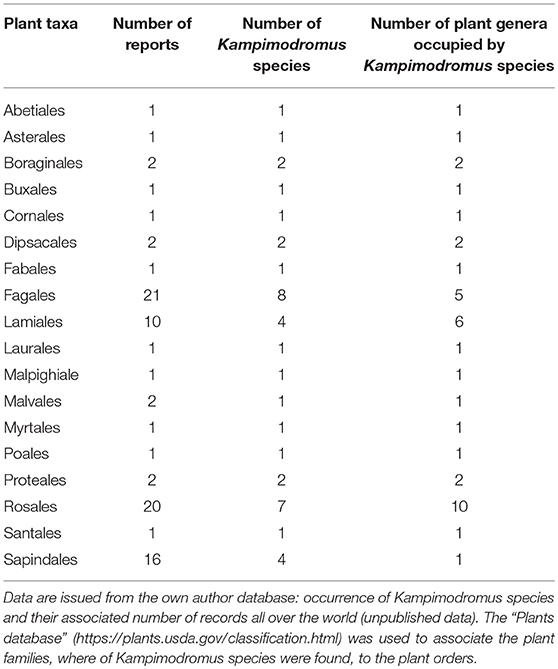
Table 7. Superior plant taxa where species of the genus Kampimodromus were recorded (associated number of reports and number of species) and the number of plant genera hosting Kampimodromus species within those superior plant taxa.
Predatory Mite Dispersal Abilities
The Mode of Dispersal and Factors Affecting Predatory Mite Movement
Predatory mites are wingless organisms. Dispersal ability depends on the species considered, abiotic (temperature, humidity, practical practices) and biotic conditions (i.e., food availability) (Sabelis and Dicke, 1985). In a framework of conservation biological control, it is important to assess why dispersal occurs, for enhancing movements between agro-ecosystem components. Most studies dealing with dispersal were based on the use of traps in field conditions (i.e., Tixier et al., 1998, 2000, 2006; Mailloux et al., 2010; Aguilar-Fenollosa et al., 2011a,b, 2012; Liguori et al., 2011; Sahraoui et al., 2012, 2016), or on the assessment of dispersal behavior (i.e., distance traveled from a release point) in lab conditions. Only one genetic population study was carried out on the dispersal of Neoseiulus womersleyi (Schicha) between tea orchards and between this crop and the non-crop plant, Tithonia rotundifolia Torch, in Japan (Hinomoto et al., 2011) (see below).
Predatory mites can move via ambulatory dispersal. This dispersal applies to low distances, usually from plant to plant, within crops. Ambulatory dispersal of N. californicus and Neoseiulus fallacis (Garman) was observed from cover crops to apple trees (Johnson and Croft, 1976, 1979, 1981; Berry and Holtzer, 1990; Fauvel and Gendrier, 1992; Pratt et al., 1998; Auger et al., 1999; Jung and Croft, 2001a). Berry and Holtzer (1990) reported different walking behaviors of N. fallacis, depending on the densities of prey. An edge-walking behavior seemed to be adopted when prey was scarce. Jung and Croft (2001a) reported that ambulatory dispersal was essentially used by females, and that larvae were the less dispersive stage. However, Sahraoui et al. (2016) showed no difference in sex-ratio and the immature/adult ratio of mites dispersing along citrus trunks. Jung and Croft (2001a) reported a walking speed ranging from 0.1 to 0.4 mm/s for N. fallacis (2.7 h to move through 1 m). Raworth et al. (1994) indicated than N. californicus would be able to walk 10 m within 1 h at 25°C. Lopez et al. (2017) studied the dispersal of A. swirskii to determine how it moved from bank plants to crops. They showed that canopy connectedness increased the dispersal of A. swirskii to the crops, whereas no impact of the prey Polyphagotarsonemus latus Banks was observed. Similarly, Buithenuis et al. (2010) showed a limited dispersal of Neoseiulus cucumeris (Oudemans) in non-continuous plant canopies. Auger et al. (1999) studied the dispersal of N. californicus between infested and non-infested dwarf alfalfa under different abiotic conditions. They showed that the main factors affecting the dispersal of this species were food deprivation and high temperatures (35°C). They also stressed that high light intensities (40,000 lux) and drought-stressed alfalfa increased dispersal. Some studies focused on the impact of agricultural practices on predatory mite dispersal. Sahraoui et al. (2016) showed that plowed plots favored E. stipulatus dispersal from weeds to trees.
Predatory mites can also disperse aerially via the wind (Tuovinen, 1994; Tixier et al., 1998). This dispersal seems to be the main colonization means of Galendromus (Galendromus) occidentalis (Nesbitt) and K. aberrans in orchards in the USA and vineyards in France, respectively (Johnson and Croft, 1979; Whalon and Croft, 1986; Dunley and Croft, 1990; Tixier et al., 1998, 2000; Jung and Croft, 2001a). Aerial dispersal ability seems to depend on the species considered. In aerial traps located under citrus trees canopy, E. stipulatus was not captured (Sahraoui et al., 2016). Tixier et al. (1998, 2000) showed that males, females, and immature stages of K. aberrans dispersed in the same way, whereas Jung and Croft (2001a) reported that the female was the main dispersal stage for N. fallacis. The dispersal rate was correlated to wind speed and starvation for food-specialist species (P. persimilis) (Jung and Croft, 2001b). These authors showed that starved individuals dispersed on a higher distance than well-fed ones. Several studies demonstrated a take-off behavior (Johnson and Croft, 1976, 1981; Sabelis and Afman, 1994). This behavior seems to be more frequent for starved mites (Jung and Croft, 2001b). Some authors reported that wind speed affected dispersal and especially the take-off behavior (2 m/s). The highest dispersal activity of P. persimilis was observed for a wind speed ranging between 4 and 8 m/s (Sabelis and Afman, 1994). Dispersal on a distance higher than 100 m via air currents was demonstrated (Johnson and Croft, 1981; Hoy et al., 1985; Dunley and Croft, 1990); Hoy et al. (1985) documented aerial dispersal of G. (G.) occidentalis for at least 200 m. In the genetic population study carried out in tea orchards in Japan, Hinomoto et al. (2011) showed a dispersal of max. One hundred meters of N. womersleyi between orchards and non-crop plants.
Phoretic dispersal is more assumed than really tested. K. aberrans females were observed on female aphids of Myzocallis coryli (Goeze) (Krantz, 1973). Fain and Krantz (1990) noted the association of Asperoseius species on the body of Diptera.
Dispersal and Predatory Mite Traits
Very few studies focus on the relationship between predatory mite traits and their dispersal ability. The dispersal ability of the majority of the predatory mite species is unknown. Jung and Croft (2001b) demonstrated that in general, specialist species had more walking and aerial dispersal rates than generalist species. These authors studied the “landing process and control” after aerial dispersal for 13 predatory mite species. They showed (i) a positive correlation between the mite body weight and the fallen speed and (ii) a negative correlation between the mite body weight and the distance of dispersal. They also demonstrated that the “landing control” could be affected by mite movement and to a lesser extent by dorsal chaetotaxy. Active mites had a slower falling speed than inactive (anesthetized) mites. Finally, no direct correlation was observed between the fallen speed and morphological features; even if a higher length of the dorsal setae Z5 increased the fallen speed, whereas a smaller length of the setae s4 negatively impacted this parameter (Jung and Croft, 2001b).
Few studies focus on the impact of host plant characteristics (especially hairiness) on predatory mite dispersal. The few existing studies generally deal with ambulatory dispersal and foraging behavior. Sarwar (2014) showed, studying three plant species (Phaseolus lunatus L., Lablab purpureus [L.] and Phaseolus vulgaris), that leaf area, thickness and hairiness significantly affected the abundance of N. womersleyi and its searching behavior. Rezaie et al. (2016) noted different predation rates of N. californicus, in different strawberry cultivars. They explained a higher predation rate by lower trichome densities on leaves, as trichome would protect prey from predation. Koveos and Broufas (2000) reported that due to the dense trichomes covering the lower surface of apple leaves compared to peach leaves, E. finlandicus movement decreased on apple leaves compared to peach leaves, resulting in an increase of prey handling time.
Predatory mite morphological features and taxonomic attributes, cannot be clearly associated since there are very few studies based on the dispersal ability of the species. No prediction of predatory mite dispersal, based on their traits can thus presently be proposed, to improve agro-ecosystem management.
The Agro-Ecosystem Management
In this section, we will present the knowledge that could be used to manage the agro-ecosystem, i.e., which plants should be associated to crops for favoring predatory mite occurrence and biological control, at different scales within the plots (through cover crops and agroforestry management) and out of the plots (through natural neighboring vegetation and landscape management). The objective is not to provide an exhaustive review of the studies carried out on this topic, but to propose, through some examples, elements for answering the following key questions: (i) what plants and what kind of management will favor the predatory mite species desired? (ii) what management will favor dispersal from non-crop plants to trees? It is difficult to address these questions in a single publication, as no general rule exists. However, compiling all elements and evidence discussed, could provide some answers.
Cover Crops/Ground Cover
Many studies showed that cover crops or weeds constitute a reservoir for predatory mites (i.e., Liang and Huang, 1994; Aucejo et al., 2003; Pereira et al., 2006; Mailloux et al., 2010). Cover crops can provide food for predatory mites, especially pollen and prey. They can also provide habitats, and depending on the leaf features of the cover crop plants, the predatory mite abundance and diversity can differ. Finally, cover crops might modify microclimate conditions in the crops, affecting predatory mite development. However, the direct impact of ground cover on the densities and diversity of those predators on the associated crops, is not easy to show. Furthermore, contradictory results exist in literature, depending on cover crop management, the predatory mite species considered and prey densities on crops.
(Markó et al., 2012) showed that densities and diversity of predatory mites in apple orchards increased with flowering ground cover plants in spring and autumn. They attributed this result to the pollen provided by the cover crop plants. They also noted that T. (T.) pyri gradually displaced A. andersoni in the presence of flowers. Similarly, Grafton-Cardwell et al. (1999) tested the effects of pollen of different plants on Euseius tularensis Congdon, in lab conditions and showed that in field conditions, a mixture of these plants (as cover crops) had a positive effect on the densities of E. tularensis in young citrus orchards. Funayama and Sonoda (2014) suggested that conservation of Plantago asiatica L. in apple orchards favored Amblyseius tsugawai Ehara populations, because of the suitable pollen produced by this plant.
Aguilar-Fenollosa et al. (2011a) studied the impact of different soil management on T. urticae and predatory mites on citrus trees. They observed a better regulation when Festuca arundinacea L. was used as cover crop, compared to bare soil or wild cover. Their hypothesis was that this plant, because favorable to T. urticae, hosted specialist predatory mite species (P. persimilis, N. californicus) more efficient for controlling citrus pests than the most species found currently, E. stipulatus. They thus proposed (i) to plant F. arundinacea for favoring P. persimilis and N. californicus and (ii) to avoid flowers in the orchards for disfavouring E. stipulatus. On the opposite end, Alston (1994) indicated the necessity to have floor vegetation plants in apple orchards, that do not harbor spider mites. Aucejo et al. (2003) studied the predatory mite fauna of cover plants in citrus orchards in Spain. They recommended avoiding plants hosting great densities of T. urticae (Equisetum palustre L., C. arvensis L., Tribulus terrestris L., Parietaria officinalis L.) and proposed a list of plants with a benefit ratio for predatory mites. De Villiers and Pringle (2011) studied the occurrence of T. urticae and predatory mites on vines and plant cover in South Africa. They reported an association between predatory mite dynamics on weeds and vineyards depending on the amount of prey present on the cover plants. They concluded that vineyard management could be associated with plants favoring T. urticae. However, they also noted the importance to manage the ratio of T. urticae/predatory mites on cover plants, to avoid T. urticae infestation in vineyards.
Sahraoui et al. (2016) showed that some plant species within citrus orchards are an important reservoir for E. stipulatus (Amarantus retroflexus L., Chenopodium murale L.) the main predatory mite found on the trees. They attributed the presence of I. phiseius degenerans (Berlese) in one part of an orchard on citrus trees to the occurrence of this species, in great densities, on Solanum nigrum L. Even though no global impact of ground cover management was observed on predatory mite diversity and densities on trees, they noted the lowest densities on trees when weeds were chemically controlled. The detrimental impact of herbicides reported in other studies (Liang and Huang, 1994; Pereira et al., 2006) could be due to: (i) the direct lethal effect of glyphosate (Kreiter and Le Menn, 1993) and/or (ii) the indirect herbicide effects through habitat destruction (Gauvrit, 1996).
Cover crops can also be used for winter habitats (Fadamiro et al., 2008). Croft and McGroarty (1977) reported that N. fallacis wintered in the grass under the apple trees and then migrated to the trees in summer, when prey was scarce in the ground cover. Because A. andersoni winters in litter, Szabo and Penzes (2013) proposed a new method to release A. andersoni in apple orchards by bringing litter into a new orchard. Higher densities of this species were observed in the plots where the litter was introduced.
The presence of predatory mites in the cover crop is not sufficient to ensure efficient biological control, as the predators have to disperse to the crops. Very few studies deal with the dispersal of predatory mites from the ground cover to crops. In some cases, no effect of ground cover management was stressed especially for N. fallacis in apple orchards in the USA (i.e., Stanyard et al., 1997), suggesting no movement between these two compartments. Nyrop et al. (1994) showed for instance that the application of pyrethroids on the cover crop did not impact N. fallacis on trees, suggesting limited dispersal from weeds to apple trees. Johnson and Croft (1981) reported that the dispersal of N. fallacis from weeds to apple trees was related to the densities of prey [Panonychus ulmi (Koch)] on apple trees (no dispersal when prey was scarce on trees). Sahraoui et al. (2016) noted that even if E. stipulatus was not the main species dispersing from weeds to citrus, it was the prevailing species on trees. The authors therefore discussed the competition abilities of E. stipulatus on citrus with regards to the other species present on weeds and dispersing along the trunk. They also noted that the densities of predatory mites moving from the ground cover to the citrus canopy along the tree trunk, were higher when the ground was plowed, suggesting that physical weed destruction enhanced predatory mite movement.
Effects other than the reservoir role can also exist. Burgio et al. (2016) studied predatory mite communities in vineyards managed with several cover crops. They observed higher predatory mite densities on vines managed with ground cover. However, no effect of the different ground cover treatments (sweet alyssum, phacelia, buckwheat, faba bean, vetch and oat) was observed on predatory mite densities on vines, where the main species were T. (T.) pyri and K. aberrans. As stated before, these species are mainly found on trees and not on herbaceous plants. Experiments carried out in the South of France, showed that predatory mites were present on herbaceous cover plants; however the main species encountered were not those occurring on vines (Tixier et al., 2015). As in (Burgio et al., 2016), the densities of predatory mites were usually higher in vineyards with cover crop than in vineyards without cover crops. Another hypotheses than simple reservoir effects can thus be drawn: (i) effect of cover crop pollen deposited on the vine leaves, (ii) effect of micro-climate conditions in vineyards managed with cover crops and/or (iii) effect of cover crop on vine physiology. No study was carried out to test the two latter hypotheses, whereas the former is well-documented (Madinelli et al., 2002). Liang and Huang (1994) found high densities of predatory mites in citrus orchards associated with Ageratum conyzoides. They also showed that the presence of such cover crops modified the orchard microclimate, reducing the temperature (−5°C) and increasing relative humidity (+5%). Cover crops could thus create abiotic favorable conditions for the development of predatory mites in orchards.
Agroforestry Management
Few studies were carried out on the impact of agrofrestry management (i.e., plantations of trees within crops) on predatory mite communities. The most documented study was carried out in the South of France, where vines were co-planted with Sorbus domestica L. or Pinus pinea L. (Barbar et al., 2005, 2006, 2009; Liguori et al., 2011; Tixier et al., 2015). A 10-year survey showed that agroforestry management did not increase biodiversity within the plot. Vine cultivar characteristics mostly affected predatory mite densities comparing to agroforestry management. Furthermore, the agroforestry effect was different according to the vine cultivar. On Grenache cv., lower densities were observed on co-planted vines than in monoculture plots, with higher densities in vines co-planted with S. domestica than with P. pinea. On Syrah cv., predatory mite densities in co-planted vines with S. domestica and in the control plot were similar, and much lower than those on vines co-planted with P. pinea. Several hypotheses were proposed: (i) different quality and quantity of pollen produced by the two co-planted trees (P. pinea, anemophilic pollination; S. domestica, entomophilous pollination), (ii) different abilities of Grenache and Syrah cultivars to capture pollen because of their different leaf hairiness and (iii) the impact on predatory mite habitats due to differences in tolerance to drought stress, according to the co-planted trees. After a 10 year-study, no clear conclusion could be drawn on a positive effect of agroforestry management considering the co-planted trees: P. pinea and S. domestica. Complex interactions between plant physiology (stress) and predatory mites might exist. Furthermore, the impact varied according to the co-planted trees and we can assume that other reservoir trees would be more interesting especially in favoring K. aberrans (such as C. australis L. or F. carica L.).
Borders of the Plots
Many studies showed the presence of predatory mites on non-crop plants in vineyard and orchard borders (i.e., Boller et al., 1988; Tuovinen and Rokx, 1991; Coli et al., 1994; Prischmann and James, 2003; Duso et al., 2004a; Demite et al., 2015). For instance, several surveys were carried out in France on the natural vegetation surrounding vineyards (i.e., Tixier et al., 1998, 2000, 2006; Barbar et al., 2005). Those studies often showed great densities of predatory mites (and especially of the most efficient species in vineyards, K. aberrans) on non-crop plants such as C. australis, F. carica, Quercus pubescens Wild. and Cornus sanguinea L. High densities of K. aberrans dispersing via the wind into the vine plots were observed. However, a population genetic study suggested a low gene flow between vines and non-crop habitats (Tixier et al., 2002). The “bridge” between crops and neighboring non-crop plants is therefore not well-understood or documented. Todokoro and Isobe (2010) noted that T. rotundifolia (Mill.) was favorable to N. womersleyi because it hosted great densities of T. urticae. They therefore proposed to use this plant within tea orchards, as T. urticae is not harmful to this crop and N. womersleyi can control Tetranychus kanzawai Kishida, the main pest found on tea orchards. Through a genetic population study, Hinomoto et al. (2011) suggested that T. rotundifolia and tea trees should be planted each 100 m. Genetic population studies would probably bring new elements to better characterize dispersal ability and factors explaining movement from the neighboring natural vegetation. Metabarcoding approaches, are used more frequently to compare predatory mite communities according to different management modalities (i.e., Mollot et al., 2014) also providing elements on factors affecting trophic networks within agro-ecosystems.
Landscape Effects
Landscape effect on predatory mite densities is poorly investigated. A study was recently carried out in France (Sentenac et al., 2018). As previously demonstrated, a clear relationship between the density of pollen and predatory mite densities on vine leaves was observed. The preliminary results showed no landscape effect. The densities of T. (T.) pyri in Burgundy were not related with the proportion of non-crop areas (semi-natural habitats: SNH) for buffers at 100 and 1,000 m. Some meta-analyses noted that densities of generalist predators were related to the proportion of SNH for buffers included between 0 and 6 kms (Chaplin-Kramer et al., 2011; Veres et al., 2013; Landis, 2017). However, predatory mites are wingless organisms and their dispersal is not efficient at high distances. Furthermore, the % SNH can also be a too global indicator, as K. aberrans and T. (T.) pyri usually occur on deciduous trees and SNH includes both trees and grasses. Clearly, the landscape approach needs to be developed for assessing effects at different distances.
Conclusions
Numerous studies focused on the agro-ecosystem management for biological control purposes and associated ecosystem services (less pesticides, well-being, human and animal health, fewer exotic natural enemies …). Many surveys were and are still being carried out to characterize the predatory mite species occurring on non-crop plants and the relationships between this fauna and that found on crops. Even if not complete, a huge amount of information exists on the occurrence of predatory mite species on plants. However, little is known on the parameters that explain this occurrence. The first modeling attempts carried out herein, showed that a prediction could be made to some extent, based on the known distribution. However, because predatory mite occurrence is not well-known, new approaches to forecast plant and predatory mite associations are needed. In the present study, we proposed and illustrated two approaches based on (i) plant traits and (ii) plant phylogeny. The preliminary results obtained are not completely satisfactory especially because of the scarce information on plant traits and the taxonomic levels investigated (too global analyses). The present work therefore constitutes a preliminary baseline for further studies, investigating more accurate taxonomic levels (i.e., species levels) and/or functional traits (i.e., feeding types of Phytoseiidae). For this, more data on plant characteristics (i.e., types of EFNs, domatia, trichomes) should be compiled in collaboration with plant specialists and cross-analyzed with predatory mite diversity and occurrence. Development in automatized plant phenotyping, as well as meta-analyses of data and modeling approaches, would certainly help to develop such studies. Considering the dispersal of predatory mites within agro-ecosystems, progress has been made but factors affecting this dispersal are not clearly understood and studies on predatory mite traits associated with dispersal ability, might be a research track for future applications in biological control. In addition, development of genetic studies for determining the population structures of predatory mites, both in and outside of the crops, would provide answers on agro-ecosystem management impact. Finally, agro-ecosystem management can act as a reservoir for predatory mites (e.g., providing alternative food) but can also impact microclimate conditions. Interactions are thus complex and the presence of predatory mites on non-crop plants does not necessary imply efficient biological control on the adjacent crops. The scale of agro-ecosystem management is therefore also important, and certainly differs depending on the predatory mite species, their habitats (trees vs. herbaceous vegetation) and their feeding habits. Recent development in metabarcoding approaches, for studying communities and trophic networks, might certainly help in deciphering interactions within an agro-ecosystem and the potential impacts of agro-ecosystem management (i.e., cover crops, border management) on these interactions and trophic networks.
Clearly, new methodological (i.e., metabarcoding, population genetics) and analytical (cross-analyses of database) developments as well as interdisciplinary approaches (e.g., botany, plant and mite ecology, agronomy, plant ecophysiology, genetics, etc…) constitute a future outlook, for managing agro-ecosystems better and enhancing biological positive interactions (Figure 4).
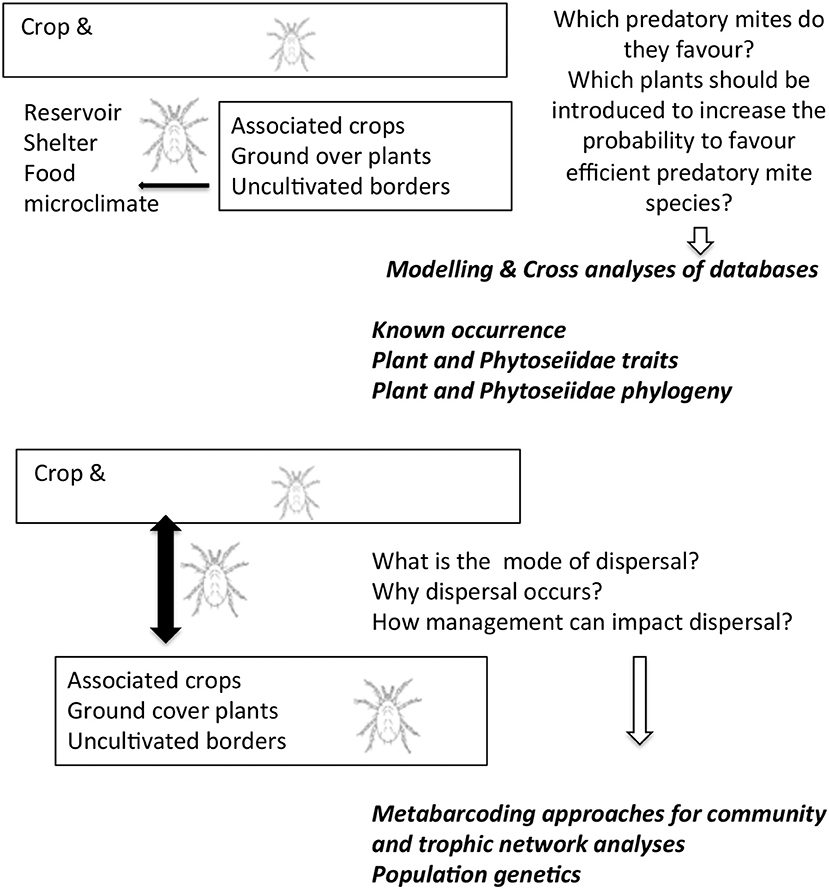
Figure 4. Synthesis of the key questions and further future approaches (in bold and italics) for agro-environmental management of predatory mites in agro-ecosystems.
Author Contributions
M-ST wrote alone the manuscript, based on an analysis of literature and compilation and analysis of databases on Phytoseiidae mites and plants.
Conflict of Interest Statement
The author declares that the research was conducted in the absence of any commercial or financial relationships that could be construed as a potential conflict of interest.
Acknowledgments
The author acknowledge the two reviewers and the manuscript editor for their comments that improved the manuscript.
Supplementary Material
The Supplementary Material for this article can be found online at: https://www.frontiersin.org/articles/10.3389/fevo.2018.00192/full#supplementary-material
Supplementary File 1. Data on the plant families with extrafloral nectaries (EFNs) and the number of predatory mite reports on these families.
References
Adar, E., Inbar, M., Gal, S., Doron, N., Zhang, Z. Q., and Palevsky, E. (2012). Plant-feeding and non-plant feeding phytoseiids: differences in behavior and cheliceral morphology. Exp. Appl. Acarol. 58, 341–357. doi: 10.1007/s10493-012-9589-y
Aguilar-Fenollosa, E. F., Ibanez, G. M. V., Pascual, R. S., Hurtado, M., and Jacas, J. A. (2011a). Effect of ground cover management on spider mites and their phytoseiid natural enemies in Clementine mandarin orchards (II): topdown regulation mechanisms. Biol. Contr. 59, 171–179. doi: 10.1016/j.biocontrol.2011.06.012
Aguilar-Fenollosa, E. F., Pascual-Ruiz, S., Hurtado, M. A., and Jacas, J. A. (2011b). Efficacy and economics of ground cover management as a conservation biological control strategy against Tetranychus urticae in clementine mandarin orchards. Crop Protect. 30, 1328–1333. doi: 10.1016/j.cropro.2011.05.011
Aguilar-Fenollosa, E. F., Pina, T., Gómez-Martínez, M. A., Hurtado, M. A., and Jacas, J. A. (2012). Does host adaptation of Tetranychus urticae populations in clementine orchards with a Festuca arundinacea cover contribute to a better natural regulation of this pest mite? Entom. Exp. Appl. 144, 181–190. doi: 10.1111/j.1570-7458.2012.01276.x
Alston, D. G. (1994). Effect of apple orchard floor vegetation on density and dispersal of phytophagous and predatory mites in Utah. Agric. Ecosys. Environ. 50, 73–84. doi: 10.1016/0167-8809(94)90126-0
Altieri, M. A. (1999). The ecological role of biodiversity in agroecosystems. Agric. Ecosys. Environ. 74, 19–31. doi: 10.1016/S0167-8809(99)00028-6
Aucejo, S., Foó, M., Gimeno, E., Gómez-Cadenas, A., Monfort, R., Obiol, F., et al. (2003). Management of Tetranychus urticae in citrus in Spain: acarofauna associated to weeds. IOBC/WPRS Bull. 26, 213–220.
Auger, P., Tixier, M.-S., Kreiter, S., and Fauvel, G. (1999). Factors affecting ambulatory dispersal in the predaceous mite Neoseiulus californicus (Acari: Phytoseiidae). Exp. Appl. Acarol. 23, 235–250. doi: 10.1023/A:1006019014708
Barbar, Z., Tixier, M.-S., Cheval, B., and Kreiter, S. (2006). Effects of agroforestry on phytoseiid mite communities (Acari: Phytoseiidae) in vineyards in the South of France. Exp. Appl. Acarol. 40, 175–188. doi: 10.1007/s10493-006-9044-z
Barbar, Z., Tixier, M.-S., Kreiter, S., and Cheval, B. (2005). Diversity of phytoseiid mites in uncultivated areas adjacent to vineyards: a case study in the south of France. Acarologia 43, 145–154. Available online at: https://www1.montpellier.inra.fr/CBGP/acarologia/article.php?id=22
Barbar, Z., Tixier, M.-S., Kreiter, S., and Cheval, B. (2009). “Does agroforestry has an influence has an influence on phytoseiid mite communities (Acari: Phytoseiidae) in vineyards in South of France ? Trends in Acarology,” in Proceedings of the 12th International Congress of Acarology, eds M. W. Sabelis and J. Bruin (Amsterdam), 409–412.
Beltrà, B., Calabuig, A. A., Navarro-Campos, C. A., Ramírez-Soria, M.-J., Soto, A., Garcia-Mar,í, F., et al. (2017). Provisioning of food supplements enhances the conservation of phytoseiidmites in citrus. Biol. Contr. 115, 18–22. doi: 10.1016/j.biocontrol.2017.09.007
Berry, J. S., and Holtzer, T. O. (1990). Ambulatory dispersal behavior of Neoseiulus fallacis (Acarina: Phytoseiidae) in relation to prey density and temperature. Exp. Appl. Acarol. 8, 253–274. doi: 10.1007/BF01202136
Boller, E. F., Remund, U., and Candolfi, M. P. (1988). Hedges as potential sources of Typhlodromus pyri, the important predatory mite in vineyards of northern Switzerland. BioControl 33, 249–255.
Buithenuis, R., Shipp, L., and Scott-Dupree, C. (2010). Dispersal of Amblyseius swirskii Athias-Henriot (Acari: Phytoseiidae) on pottedgreenhouse chrysanthemum. Biol. Contr. 52, 110–114. doi: 10.1016/j.biocontrol.2009.10.007
Burgio, G., Marchesini, E., Reggiani, N., Montepaone, G., Schiatti, P., and Sommagio, D. (2016). Habitat management of organic vineyard in Northern Italy: the role of cover plants management on arthropod functional biodiversity. Bull Entomol Res. 106, 759–768. doi: 10.1017/S0007485316000493
Chant, D. A. (1959). Phytoseiid mites (Acarina: Phytoseiidae). Part I. Bionomics of seven species in southeastern England. Part II. A taxonomic review of the family Phytoseiidae, with descriptions of 38 new species. Can. Entomol. (Suppl. 12) 9, 1–166.
Chaplin-Kramer, R., O'Rourke, M. E., Blitzer, E. J., and Kremen, C. (2011). A meta-analysis of crop pest and natural enemy response to landscape complexity. Ecol. Lett. 14, 922–932. doi: 10.1111/j.1461-0248.2011.01642.x
Christenhusz, M. J. M., and Byng, J. W. (2016). The number of known plants species in the world and its annual increase. Phytotaxa 261, 201–217. doi: 10.11646/phytotaxa.261.3.1
Coli, W. M., Ciurlion, R. A., and Hodmer, T. (1994). Effect of understory and border vegetation composition on phytophagous and predatory mites in Massachusetts commercial apple orchards. Agric. Ecosys. Environ. 50, 49–60. doi: 10.1016/0167-8809(94)90124-4
Croft, B. A., and McGroarty, D. L. (1977). The role of Amblyseius fallacis (Acarina: Phytoseiidae) in Michigan apple orchards. Mich. State Agric. Exp. Stn. Res. Rep. 333, 1–22.
Daud, R. D. (2003). Diversity of mites on Mabea fistulifera Mart. (Euphorbiaceae) and efficiency of its pollen as food for phytoseiids (Acari, Phytoseiidae). Biota Neotrop. 5, 227–228. doi: 10.1590/S1676-06032005000100033
De Villiers, M., and Pringle, K. L. (2011). The presence of Tetranychus urticae (Acari: Tetranychidae) and its predators on plants in the ground cover in commercially treated vineyards. Exp. Appl. Acarol. 53, 121–137. doi: 10.1007/s10493-010-9391-7
Demite, P. R., de Moraes, G. J., McMurtry, J. A., Denmark, H. A., and Castilho, R. C. (2018). Phytoseiidae Database. Available online at: www.lea.esalq.usp.br/phytoseiidae (Accessed June 07, 2018).
Demite, P. R., Feres, R. J. F., and Lofego, A. C. (2015). Influence of agricultural environment on the plant mite community in forest fragments. Braz. J. Biol. 75, 396–404. doi: 10.1590/1519-6984.14913
Dunley, J. E., and Croft, B. A. (1990). Dispersal between and colonization of apple by Metaseiulus occidentalis and Typhlodromus pyri (Acarina: Phytoseiidae). Exp. Appl. Acarol. 10, 137–149. doi: 10.1007/BF01194089
Duso, C. (1992). Role of Amblyseius aberrans (Oud.), Typhlodromus pyri Scheuten and Amblyseius andersoni (Chant) (Acari, Phytoseiidae) in vineyards. J. Appl. Entomol. 114, 455–462. doi: 10.1111/j.1439-0418.1992.tb01151.x
Duso, C., Fanti, M., Pozzebon, A., and Angeli, G. (2009). Is the predatory mite Kampimodromus aberrans a candidate for the control of phytophagous mites in European apple orchards? Biocontrol 54, 369–382. doi: 10.1007/s10526-008-9177-6
Duso, C., Fontana, P., and Malagnini, V. (2004a). Diversity and abundance of phytoseiid mites (Acari: Phytoseiidae) in vineyards and the surrounding vegetation in northeastern Italy. Acarologia 44, 31–47. Available online at: https://www1.montpellier.inra.fr/CBGP/acarologia/article.php?id=42
Duso, C., Malagnini, V., Paganelli, A., Aldegheri, L., Bottini, M., and Otto, S. (2004b). Pollen availability and abundance of predatory phytoseiid mites on natural and secondary hedgerows. Biocontrol 49, 397–415. doi: 10.1023/B:BICO.0000034601.95956.89
Duso, C., and Vettorazzo, E. (1999). Mite population dynamics on different grape varieties with or without phytoseiids released (Acari: Phytoseiidae). Exp. Appl. Acarol. 23, 741–763. doi: 10.1023/A:1006297225577
Fadamiro, H. Y., Xiao, Y., Hargroder, T., Nesbitt, M., Umeh, V., and Childers, C. C. (2008). Seasonal occurrence of key arthropod pests and associated natural enemies in Alabama satsuma citrus. Environ. Entomol. 2, 555–567. doi: 10.1093/ee/37.2.555
Fain, A., and Krantz, W. (1990). Notes on the genus Asperoseius Chant, 1957 (Acari, Phytoseiidae), with descriptions of two new species [in phoretic association with biting nematocerous Diptera]. J. Afr. Zool. 104, 213–220.
Fauvel, G., and Gendrier, J. P. (1992). Problems met in the establishment of phytoseiids in apple orchards of Southeastern France. Acta Phytopathol. Entomol. Hung. 27, 223–232.
Ferrero, M., Tixier, M.-S., and Kreiter, S. (2014). Different feeding behaviours in a single predatory mite species. 2. Responses of two populations of Phytoseiulus longipes (Acari: Phytoseiidae) to various prey species, prey stages and plant substrates. Exp. Appl. Acarol. 62, 325–335. doi: 10.1007/s10493-013-9746-y
Flechtman, C., and McMutry, J. A. (1992). Studies of cheliceral and deutosternal morphology of some phytoseiidae (Acari: Mesostigmata) by scanning electron microscopy. Int. J. Acarol. 18, 163–169. doi: 10.1080/01647959208683947
Funayama, K., and Sonoda, S. (2014). Plantago asiatica groundcover supports Amblyseius tsugawai (Acari: Phytoseiidae) populations in apple orchards. Appl. Entomol. Zool. 49, 607–611. doi: 10.1007/s13355-014-0280-0
Gerson, U., Smiley, R. L., and Ochoa, T. (2003). Mites (Acari) for Pest Control. Oxford, UK: Blackwell Science.
Gonzalez-Fernandez, J. J., de La Pena, F., Hormaza, J. I., Boyero, J. R., Vela, J. M., Wong, E., et al. (2009). Alternative food improves the combined effect of an omnivore and a predator on biological pest control, a case study in avocado orchards. Bull. Entomol. Res. 99, 433–444. doi: 10.1017/S000748530800641X
Grafton-Cardwell, E. E., Ouyang, Y., and Bugg, R. L. (1999). Leguminous cover crops to enhance population development of Euseius tularensis (Acari: Phytoseiidae) in citrus. Biol. Contr. 16, 73–80. doi: 10.1006/bcon.1999.0732
Grout, T. G., and Richards, G. I. (1992). The dietary effect of wind break pollens on longevity and fecundity of a predacious mite Euseius addoensis addoensis (Acari: Phytoseiidae) found in citrus orchards in South Africa. Bull. Entomol. Res. 82, 317–320. doi: 10.1017/S0007485300041080
Hinomoto, N., Todokoro, Y., and Higaki, T. (2011). Population structure of the predatory mite Neoseiulus womersleyi in a tea field based on an analysis of microsatellite DNA markers. Exp. Appl. Acarol. 53, 1–15. doi: 10.1007/s10493-010-9384-6
Hoy, M. A., Groot, J. J. R., and Baan, H. E. (1985). Influence of aerial dispersal on persistence and spread of pesticide-resistant Metaseiulus occidentalis in California almond orchards. Entom. Experim. Appl. 37, 17–31. doi: 10.1111/j.1570-7458.1985.tb03448.x
Johnson, D. T., and Croft, B. A. (1976). Laboratory study of the dispersal behavior of Amblyseius fallacis (Acarina: Phytoseiidae). Ann. Entomol. Soc. Am. 69, 1019–1023. doi: 10.1093/aesa/69.6.1019
Johnson, D. T., and Croft, B. A. (1979). “Factors affecting the dispersal of Amblyseius fallacis in an apple ecosystem,” in Recent Advances in Acarology, Vol. I, ed J. G. Rodriguez (Orlando, FL: Academic Press), 477–483. doi: 10.1016/B978-0-12-592201-2.50067-1
Johnson, D. T., and Croft, B. A. (1981). Dispersal of Amblyseius fallacis (Acarina: Phytoseiidae) in an apple ecosystem. Environ. Entomol. 10, 313–319. doi: 10.1093/ee/10.3.313
Jung, C., and Croft, B. A. (2001a). Ambulatory and aerial dispersal among specialist and generalist predatory mites (Acari: Phytoseiidae). Environ. Entomol. 30, 1112–1118. doi: 10.1603/0046-225X-30.6.1112
Jung, C., and Croft, B. A. (2001b). Aerial dispersal of phytoseiid mites (Acari: Phytoseiidae): estimating falling speed and dispersal distance of adult females. Oikos 94, 182–190. doi: 10.1034/j.1600-0706.2001.11044.x
Karban, R., English-Loeb, G., Walker, M. A., and Thaler, J. (1995). Abundance of phytoseiid mites on Vitis species: effects of leaf hairs, domatia, prey abundance and plant phylogeny. Exp. Appl. Acarol. 19, 189–197. doi: 10.1007/BF00130822
Kasap, I. (2005). Life-history traits of the predaceous mite Kampimodromus aberrans (Oudemans) (Acarina: Phytoseiidae) on four different types of food. Biol. Contr. 35, 40–45. doi: 10.1016/j.biocontrol.2005.05.014
Kennett, C. E., Flaherty, D. L., and Hoffmann, R. W. (1979). Effects of wind borne pollens on the population dynamics of Amblyseius hibisci (Acarina: Phytoseiidae). Entomophaga 34, 83–98. doi: 10.1007/BF02377513
Koveos, D. S., and Broufas, G. D. (2000). Functional response of Euseius finlandicus and Amblyseius andersoni to Panonychus ulmi on apple and peach leaves in the laboratory. Exp. Appl. Acarol. 24, 247–256. doi: 10.1023/A:1006431710313
Krantz, G. W. (1973). Dissemination of Kampimodromus aberrans by the filbert aphid. J. Econ. Entomol. 66, 575–576. doi: 10.1093/jee/66.2.575
Kreiter, S., and Le Menn, V. (1993). “Interaction entre le désherbage chimique de la vigne et les populations d'acariens phytophages et prédateurs: résultats de laboratoires,” in Proceeding ANPP, 3ème Conférence Internationale sur les Ravageurs en Agriculture (Montpellier), 821–830.
Kreiter, S., Tixier, M.-S., Auger, P., Muckenstrum, N., Sentenac, G., Doublet, B., et al. (2000). Phytoseiid mites of vineyards in France (Acari: Phytoseiidae). Acarologia 41, 77–96. Available online at: https://www1.montpellier.inra.fr/CBGP/acarologia/article.php?id=120
Kreiter, S., Tixier, M.-S., Croft, B. A., Auger, P., and Barret, D. (2002). Plants and leaf characteristics influencing the predaceous mite, Kampimodromus aberrans (Oudemans) in habitats surrounding vineyards (Acari: Phytoseiidae). Environ. Entomol. 31, 648–660. doi: 10.1603/0046-225X-31.4.648
Landis, A. D. (2017). Designing agricultural landscapes for biodiversity-based ecosystem services. Basic Appl. Ecol. 8, 1–12. doi: 10.1016/j.baae.2016.07.005
Letourneau, D. K., Armbrecht, I., Salguero Rivera, B., Montoya Lerma, J., Jimenez Carmona, E., Constanza Daza, M., et al. (2011). Does plant diversity benefit agroecosystems? A synthetic review. Ecol. Appl. 21, 9–21. doi: 10.1890/09-2026.1
Liang, W., and Huang, M. (1994). Influence of citrus orchard ground cover plants on arthropod communities in China: a review. Agric. Ecosys. Environ. 50, 29–37. doi: 10.1016/0167-8809(94)90122-8
Liguori, M., Tixier, M.-S., Hernandes Akashi, F., Douin, M., and Kreiter, S. (2011). Agroforestry management and phytoseiid communities in vineyards of the South of France. Exp. Appl. Acarol. 54, 1–15. doi: 10.1007/s10493-011-9450-8
Lopez, L., Smith, H. A., Hoy, M. A., and Cave, R. D. (2017). Dispersal of Amblyseius swirskii (Acari: Phytoseiidae) on high-tunnel bell peppers in presence or absence of Polyphagotarsonemus latus (Acari: Tarsonemidae). J. Insect Sci. 17, 1–7. doi: 10.1093/jisesa/iew095
Madinelli, S., Mori, N., and Girolami, V. (2002). The importance of pollen from herbaceous cover for phytoseiid mites. Inform. Agrar. 58, 125–127.
Magalhães, S., and Bakker, F. M. (2002). Plant feeding by a predatory mite inhabiting cassava. Exp. Appl. Acarol. 27, 27–37. doi: 10.1023/A:1021508620436
Mailloux, J., Le Bellec, F., Kreiter, S., Tixier, M.-S., and Dubois, P. (2010). Influence of ground cover management on diversity and density of phytoseiid mites (Acari: Phytoseiidae) in Guadeloupian citrus orchards. Exp. Appl. Acarol. 52, 275–290. doi: 10.1007/s10493-010-9367-7
Maoz, Y., Gal, S., Abrahams, J., Gan-Mor, S., Coll, M., and Palevsky, E. (2008). “Pollen providing enhances Euseius scutalis (Phytoseiidae) populations and improves control of Oligonychus perseae (Tetranychidae),” in Proceedings of the 3rd International Symposium on Biological Control of Arthropods, eds P. G. Mason, D. R. Gillespie, and C. Vincent (Christchurch), 339–346.
Maoz, Y., Gal, S., Argov, Y., Domeratzky, S., Melamed, E., Gan-Mor, S., et al. (2014). Efficacy of indigenous phytoseiids (Acari:Phytoseiidae) against the citrus rust mite (Phyllocoptruta oleivora) (Acari: Eriophyidae): augmentation and conservation biological control in Israeli citrus orchards. Exp. Appl. Acarol. 63, 295–312. doi: 10.1007/s10493-014-9786-y
Markó, V., Jenser, G., Mihályi, K., Hegyi, T., and Balázs, K. (2012). Flowers for better pest control? Effects of apple orchard groundcover management on mites (Acari), leafminers (Lepidoptera, Scitellidae), and fruit pests. Biocontrol Sci. Tech. 22, 39–60. doi: 10.1080/09583157.2011.642337
Mayuko, O., and Yano, S. (2008). Pearl bodies of Cayratia japonica (Thunb.) Gagnep. (Vitaceae) as alternative food for a predatory mite Euseius sojaensis (Ehara) (Acari: Phytoseiidae). Ecol. Res. 24, 257–262. doi: 10.1007/s11284-008-0501-5
McMurtry, J. A., and Croft, B. A. (1997). Life-styles of phytoseiid mites and their roles in biological control. Annu. Rev. Entomol. 42, 291–321. doi: 10.1146/annurev.ento.42.1.291
McMurtry, J. A., De Moraes, G. J., and Sourasso, N. F. (2013). Revision of the lifestyles of phytoseiid mites (Acari: Phytoseiidae) and implications for biological control strategies. Syst. Appl. Acarol. 18, 297–320. doi: 10.11158/saa.18.4.1
McMurtry, J. A., and Johnson, H. G. (1965). Some factors influencing the abundance of the predaceous mite Amblyseius hibisci in southern California (Acarina: Phytoseiidae). Ann. Entomol. Soc. Am. 58, 49–56. doi: 10.1093/aesa/58.1.49
McMurtry, J. A., Sourassou, N. F., and Demite, P. (2015). “The Phytoseiidae (Acari: Mesostigmata) as biological control agents,” in Prospects for Biological Control of Plant Feeding Mites and Other Harmful Organisms. Progress in Biological Control, Vol. 19, eds D. Carrillo, G. de Moraes, and J. Peña (Cham: Springer), 133–149. doi: 10.1007/978-3-319-15042-0_5
Mollot, G., Duyck, P.-F., Lefeuvre, P., Lescourret, F., Martin, J.-F., Piry, S., et al. (2014). Cover cropping alters the diet of arthropods in a banana plantation: a metabarcoding approach. PLoS ONE 9:e93740. doi: 10.1371/journal.pone.0093740
Nomikou, M., Janssen, A., and Sabelis, M. W. (2003). Phytoseiid predators of whiteflies feed and reproduce on non-prey food sources. Exp. Appl. Acarol. 31, 15–26. doi: 10.1023/B:APPA.0000005142.31959.e8
Nyrop, J. P., Minns, J. C., and Herring, C. P. (1994). Influence of ground cover on dynamics of Amblyseius fallacis Garman (Acarina; Phytoseiidae) in New York apple orchards. Agric. Ecosys. Environ. 50, 61–72. doi: 10.1016/0167-8809(94)90125-2
Oerke, E. C. (2006). Crop losses to pests. J. Agric. Sci. 144, 31–43. doi: 10.1017/S0021859605005708
Onzo, A., Hanna, R., and Sabelis, M. W. (2012). The predatory mite Typhlodromalus aripo prefers green-mite induced plant odours from pubescent cassava varieties. Exp. Appl. Acarol. 58, 359–370. doi: 10.1007/s10493-012-9595-0
Pereira, N., Ferreira, M. A., Sousa, M. E., and Franco, J. C. (2006). Mites, lemon trees and ground cover interactions in Mafra region. IOBC/WPRS Bull. 29, 143–150.
Porres, M. A., McMurtry, J. A., and March, R. B. (1975). Investigations of leaf sap feeding by three species of phytoseiid mites by labelling with radioactive phosphoric acid (H332PO4). Ann. Entomol. Soc. Am. 68, 871–872. doi: 10.1093/aesa/68.5.871
Power, A. G. (2010). Ecosystem services and agriculture: tradeoffs and synergies. Philos. Trans. R. Soc. B 365, 2959–2971. doi: 10.1098/rstb.2010.0143
Pratt, P. D., Monetti, L. N., and Croft, B. A. (1998). Withinand between-plant dispersal and distributions of Neoseiulus californicus and N. fallacis (Acari: Phytoseiidae) in simulated bean and apple plant systems. Environ. Entomol. 27, 148–153. doi: 10.1093/ee/27.1.148
Prischmann, D. A., Croft, B. A., and Luh, H.-K. (2002). Biological control of spider mites on grape by phytoseiid mites (Acari: Tetranychidae, Phytoseiidae): emphasis on regional aspects. J. Econ. Entomol. 95, 340–347. doi: 10.1603/0022-0493-95.2.340
Prischmann, D. A., and James, D. G. (2003). Phytoseiidae (Acari) on unsprayed vegetation in southcentral Washington: implications for biological control of spider mites on wine grapes. Int. J. Acarol. 29, 279–287. doi: 10.1080/01647950308684340
Prischmann, D. A., James, D. G., and Snyder, W. E. (2005c): Impact of management intensity on mites (Acari: Tetranychidae, Phytoseiidae) in Southcentral Washington wine grapes. Intern. J. Acarol. 31, 277–288. doi: 10.1080/01647950508684432
Prischmann, D. A., James, D. G., Wright, L. C., and Snyder, W. E. (2006). Effects of generalist phytoseiid mites and grapevine canopy structure on spider mite (Acari: Tetranychidae) biocontrol. Environ. Entomol. 35, 56–67. doi: 10.1603/0046-225X-35.1.56
Prischmann, D. A., James, D. J., and McMurtry, J. A. (2005a). Occurrence of a predatory mite (Acari: Phytoseiidae) within willow galls caused by eriophyid mites. Int. J. Acarol. 31, 433–436. doi: 10.1080/01647950508683686
Prischmann, D. A., James, D. J., Wright, L. C., Teneyck, R. D., and Snyder, W. E. (2005b). Effects of chlorpyrifos and sulfur on spider mites (Acari: Tetranychidae) and their natural enemies. Biol. Contr. 33, 324–334. doi: 10.1016/j.biocontrol.2005.03.008
Ratnadass, A., Fernandez, P., Avelino, J., and Habib, R. (2012). Plant species diversity for sustainable management of crop pests and diseases in agroecosystems: a review. Agron. Sustain. Dev. 32, 273–303. doi: 10.1007/s13593-011-0022-4
Raworth, D. A., Fauvel, G., and Auger, P. (1994). Location, reproduction and movement of Neoseiulus californicus (Acari: Phytoseiidae) during the autumn, winter and spring in orchards in the south of France. Exp. Appl. Acarol. 18, 593–602. doi: 10.1007/BF00051722
Rezaie, R., Baniamerie, V., and Saboori, A. (2016). Functional response and predation interference of (Neoseiulus californicus) (Acari: Phytoseiidae) feeding on the western flower thrips larvae on several commercial strawberry cultivars. Plant Pest Res. 6, Pe1–Pe15. Available online at: https://www.sid.ir/En/Journal/ViewPaper.aspx?ID=542605
Roda, A., Nyrop, J., and English-Loeb, G. (2003). Leaf pubescence mediates the abundance of non-preyfood and the density of the predatory mite Typhlodromus pyri. Exp. Appl. Acarol. 29, 193–211. doi: 10.1023/A:1025874722092
Sabelis, M. W., and Afman, D. P. (1994). Synomone-induced suppression of take-off in the phytoseiid mite Phytoseiulus persimilis Athias-Henriot. Exp. Appl. Acarol. 18, 711–721.
Sabelis, M. W., and Dicke, M. (1985). “Long-range dispersal and searching behaviour,” in n: Spider Mites and Their Control, eds W. Helle and M. W. Sabelis (Amsterdam: Elsevier), 141–160.
Sahraoui, H., Kreiter, S., Lebdi-Grissa, K., and Tixier, M.-S. (2016). Sustainable weed management and predatory mite (Acari: Phytoseiidae) dynamics in Tunisian citrus orchards. Acarologia 56, 517–532. doi: 10.1051/acarologia/20162240
Sahraoui, H., Lebdi-Grissa, K., Kreiter, S., Douin, M., and Tixier, M.-S. (2012). Phytoseiid mites (Acari: Mesostigmata) of Tunisian citrus orchards: catalogue, biogeography and key for identification. Acarologia 52, 433–452. doi: 10.1051/acarologia/20122072
Sarwar, M. (2014). Influence of host plant species on the development, fecundity and population density of pest Tetranychus urticae Koch (Acari: Tetranychidae) and predator Neoseiulus pseudolongispinosus (Xin, Liang and Ke) (Acari: Phytoseiidae). N. Z. J. Crop Hortic. Sci. 42, 10–20. doi: 10.1080/01140671.2013.817444
Schmidt, R. A. (2014). Leaf structures affect predatory mites (Acari: Phytoseiidae) and biological control: a review. Exp. Appl. Acarol. 62, 1–17. doi: 10.1007/s10493-013-9730-6
Sengonca, C., Khan, I. A., and Blaeser, P. (2004). The predatory mite Typhlodromus pyri (Acari: Phytoseiidae) causes feeding scars on leaves and fruits of apple. Exp. Appl. Acarol. 33, 45–53. doi: 10.1023/B:APPA.0000029965.47111.f3
Sentenac, G., Rusch, A., Kreiter, S., Bouvier, J.-C., Thiery, J., Delbac, L., et al. (2018). Biodiversité fonctionnelle : effet de l'environnement paysager d'une parcelle de vigne sur la régulation de ses ravageurs (BIOCONTROL). Innovat. Agron. 63, 139–161.
Stanyard, M. J., Foster, R. E., and Gibb, T. J. (1997). Effects of orchard ground cover and mite management options on the population dynamics of the European red mite (Acari: Tetranychidae) and Amblyseius fallacis (Acari: Phytoseiidae) in apple. J. Econ. Entomol. 90, 595–603. doi: 10.1093/jee/90.2.595
Szabo, A., and Penzes, B. (2013). A new method for the release of Amblyseius andersoni (Chant, 1959) (Acari: Phytoseiidae) in a young apple orchard. Eur. J. Entomol. 110, 477–482. doi: 10.14411/eje.2013.063
The Angiosperm Phylogeny Group (2003). An update of The Angiosperm Phylogeny Group classification for the orders and families of flowering plants: APG II. Bot. J. Linn. Soc. 141, 399–436. doi: 10.1046/j.1095-8339.2003.t01-1-00158.x
Tixier, M.-S., Arnaud, A., Douin, M., and Kreiter, S. (2015). Effects of agroforestry on Phytoseiidae communities (Acari: Mesostigmata) in vineyards. A synthesis of 10-years period of observations. Acarologia 55, 361–375. doi: 10.1051/acarologia/20152182
Tixier, M.-S., Kreiter, S., and Auger, P. (2000). Colonization of vineyards by phytoseiid mites: their dispersal patterns in the plot and their fate. Exp. Appl. Acarol. 24, 191–211. doi: 10.1023/A:1006332422638
Tixier, M.-S., Kreiter, S., Auger, P., and Weber, M. (1998). Colonization of Languedoc vineyards by phytoseiid mites (Acari: Phytoseiidae): influence of wind and crop environment. Exp. Appl. Acarol. 22, 523–542. doi: 10.1023/A:1006085723427
Tixier, M.-S., Kreiter, S., Barrau, J.-N., Cheval, B., and Lecareux, C. (2005b). Phytoseiid communities in southern France on vine cultivars and uncultivated surrounding areas. Acarologia 46, 157–168.
Tixier, M.-S., Kreiter, S., Bourgois, T., and Cheval, B. (2007). Factors affecting abundance and diversity of phytoseiid mite communities in two arboreta in the South of France. J. Egypt. Soc. Parasitol. 37, 493–510.
Tixier, M.-S., Kreiter, S., and Cheval, B. (2005a). Abundance and diversity of phytoseiid mite communities in two arboreta in the South of France. Acarologia 46, 169–179.
Tixier, M.-S., Kreiter, S., Cheval, B., Guichou, S., Auger, P., and Bonafos, R. (2006). Immigration of phytoseiid mites from surrounding uncultivated areas into a newly planted vineyard. Exp. Appl. Acarol. 39, 227–242. doi: 10.1007/s10493-006-9010-9
Tixier, M.-S., Kreiter, S., Croft, B. A., and Auger, P. (2002). Colonization of vineyards by Kampimodromus aberrans (Oudemans) (Acari: Phytoseiidae): dispersal from surrounding plants as indicated by random amplified polymorphism DNA typing. Agric. For. Entomol. 4, 255–264. doi: 10.1046/j.1461-9563.2002.00154.x
Tixier, M.-S., Kreiter, S., Douin, M., and Moraes, G. J. (2012). Rates of description of Phytoseiidae (Acari: Mesostigmata): space, time and body size variations. Biodiv. Conserv. 21, 993–1013. doi: 10.1007/s10531-012-0235-0
Tixier, M.-S., Lopes, I., Blanc, G., Dedieu, J.-L., and Kreiter, S. (2014). Phytoseiidae mites (Acari: Mesostigmata) in French orchards and assessment of their spatial distribution. Acarologia 54, 97–111. doi: 10.1051/acarologia/20142114
Tixier, M. S., Baldassar, A., Duso, C., and Kreiter, S. (2013). Phytoseiidae in European grape (Vitis vinifera L.): bio-ecological aspects and keys to species (Acari: Mesostigmata). Zootaxa 3721, 101–142. doi: 10.11646/zootaxa.3721.2.1
Todokoro, Y., and Isobe, K. (2010). Effectiveness of predatory mites, Neoseiulus womersleyi (Schicha) (Acari: Phytoseiidae) proliferated on natural enemy preservation plants in suppressing the population density of Kanzawa Spider Mite, Tetranychus kanzawai Kishida (Acari: Tetranychidae) on Tea. Jap. J. Appl. Entomol. Zool. 54, 1–12. doi: 10.1303/jjaez.2010.1
Tuovinen, T. (1994). Influence of surrounding trees and bushes on the phytoseiid mite fauna on apple orchard trees in Finland. Agr. Ecosys. Environ. 50, 39–47. doi: 10.1016/0167-8809(94)90123-6
Tuovinen, T., and Rokx, J. A. H. (1991). Phytoseiid mites (Acari: Phytoseiidae) on apple trees and in surrounding vegetation in southern Finland: Densities and species composition. Exp. Appl. Acarol. 12, 35–46. doi: 10.1007/BF01204398
Van den Boom, C. E., van Beek, T. A., and Dicke, M. (2002). Attraction of Phytoseiulus persimilis (Acari: Phytoseiidae) towards volatiles from various Tetranychus urticae-infested plant species. Bull. Entomol. Res. 92, 539–546. doi: 10.1079/BER2002193
Van Lenteren, J.-C. (2006). IOBC Internet Book of Biological Control. Available online at: http://www.iobc-global.org/download/IOBC_InternetBookBiCoVersion6Spring2012.pdf
van Rijn, P. C. J., and Tanigoshi, L. K. (1999). Pollen as food for the predatory mites Iphiseius degenerans and Neoseiulus cucumeris (Acari: Phytoseiidae): dietary range and life history. Exp. Appl. Acarol. 23, 785–802. doi: 10.1023/A:1006227704122
Veres, A., Petit, S., Conord, C., and Lavigne, C. (2013). Does landscape composition affect pest abundance and their control by natural enemies? A review. Agric. Ecosys. Environ. 166, 110–117. doi: 10.1016/j.agee.2011.05.027
Walter, D. E. (1996). Living on leaves: mites, tomentia, and leaf domatia. Annu. Rev. Entomol. 41, 101–114. doi: 10.1146/annurev.en.41.010196.000533
Weber, M. G., Clement, W. L., Donoghue, M. J., and Agrawal, A. A. (2012). Phylogenetic and experimental tests of interactions among mutualistic plant defense traits in Viburnum (Adoxaceae). Am. Nat. 180, 450–463. doi: 10.1086/667584
Weber, M. G., Porturas, L. D., and Keeler, K. H. (2015). World List of Plants with Extrafloral Nectaries. Available online at: www.extrafloralnectaries.org (Accessed June 07, 2018).
Weber, M. G., Porturas, L. D., and Taylor, S. A. (2016). Foliar nectar enhances plant–mite mutualisms: the effect of leaf sugar on the control of powdery mildew by domatia-inhabiting mites. Ann. Bot. 118, 459–466. doi: 10.1093/aob/mcw118
Whalon, M. E., and Croft, B. A. (1986). Immigration and colonization of portable apple trees by arthropod pests and their natural enemies. Crop Prot. 5, 376–338. doi: 10.1016/0261-2194(86)90068-2
Keywords: biological control, natural enemies, agroecosystem management, mites, agro-environmental management
Citation: Tixier M-S (2018) Predatory Mites (Acari: Phytoseiidae) in Agro-Ecosystems and Conservation Biological Control: A Review and Explorative Approach for Forecasting Plant-Predatory Mite Interactions and Mite Dispersal. Front. Ecol. Evol. 6:192. doi: 10.3389/fevo.2018.00192
Received: 31 July 2018; Accepted: 05 November 2018;
Published: 14 December 2018.
Edited by:
George Broufas, Democritus University of Thrace, GreeceReviewed by:
Deirdre Anne Prischmann-Voldseth, North Dakota State University, United StatesAndreas Walzer, University of Natural Resources and Life Sciences Vienna, Austria
Copyright © 2018 Tixier. This is an open-access article distributed under the terms of the Creative Commons Attribution License (CC BY). The use, distribution or reproduction in other forums is permitted, provided the original author(s) and the copyright owner(s) are credited and that the original publication in this journal is cited, in accordance with accepted academic practice. No use, distribution or reproduction is permitted which does not comply with these terms.
*Correspondence: Marie-Stephane Tixier, bWFyaWUtc3RlcGhhbmUudGl4aWVyQHN1cGFncm8uZnI=