- Department of Chemical Ecology, Bielefeld University, Bielefeld, Germany
Mating between closely related individuals is often associated with fitness declines. However, less is known about consequences of inbreeding for (sexual) chemical signaling traits and for mate choice and acceptance. Thus, we investigated effects of inbreeding on the chemical phenotype, i.e., the profile and diversity of putative cuticular hydrocarbons (CHCs) in the leaf beetle Phaedon cochleariae Fabricius. Moreover, we tested mating probabilities in no choice bioassays with different combinations of outbred and inbred beetles. Finally, we tested male preferences and female aggression in a dual choice bioassay with one outbred male, one outbred, and one inbred female. The chemical phenotype was altered in inbred females, which showed a male-like CHC profile. In no choice bioassays, inbred individuals had a decreased mating probability than outbred individuals. Changes in the chemical phenotype of inbred females might be involved in the negative inbreeding effects on mating acceptance. When having the choice, males did not show any preference between outbred and inbred females. However, inbred females were more aggressive in these situations, potentially to raise their chances to be mated, because due to inbreeding depression they have lower reproductive perspectives than outbred females. Overall, inbreeding effects on the chemical phenotype, mating acceptance and female aggression might have consequences for the population dynamics and development of the individuals. In conclusion, these results suggest that inbreeding events have the potential to affect sexual selection of chemical signaling traits and evolutionary processes underlying mating strategies.
Introduction
The mating between close relatives, i.e., inbreeding, is often associated with a loss of individual fitness in inbred compared to outbred offspring, called inbreeding depression (Charlesworth and Charlesworth, 1987). Inbreeding depression results from increased genome-wide homozygosity, whereby the risk of phenotypic expression of recessive deleterious mutations increases and advantageous heterozygosity is reduced (Keller and Waller, 2002; Charlesworth and Willis, 2009). Negative inbreeding effects can become evident solely at specific life stages (Meunier and Kölliker, 2013) or throughout the ontogeny of these individuals (Peng et al., 2015; Müller et al., 2018). However, inbreeding does not necessarily lead to fitness declines and can either be tolerated or, under certain conditions, even be adaptive, e.g., in terms of inclusive fitness benefits (Kokko and Ots, 2006; Kuriwada et al., 2011; Szulkin et al., 2013).
A few, mostly recent studies revealed that inbreeding influences various sexual signaling traits in animals. Examples range from inbreeding impacts on chemical (Thom et al., 2008; van Bergen et al., 2013; Menzel et al., 2016) to acoustic traits (Aspi, 2000; Drayton et al., 2007, 2010). In vertebrates, mating preferences for more heterozygous than homozygous individuals can be mediated through chemicals (Ilmonen et al., 2009), whereby major urinary proteins and/or the major histocompatibility complex play an important role (Thom et al., 2008; Griggio et al., 2011). In many arthropods, cuticular hydrocarbons (CHCs), cuticular lipids and sex pheromones trigger mating behavior and preferences (Howard and Blomquist, 2005; Lihoreau and Rivault, 2009; van Bergen et al., 2013; Keppner et al., 2017). For example, the sex pheromone amount of male Bicyclus anynana butterflies is lower in inbred than in outbred individuals, which reduces the mating success of inbred males (van Bergen et al., 2013). Similarly, in Tenebrio molitor beetles the odor of males is affected by inbreeding and females discriminate against this odor (Pölkki et al., 2012). Moreover, inbreeding decreases the CHC diversity of the ant Hypoponera opacior, which potentially leads to an adjustment of reproduction strategies in this species (Menzel et al., 2016).
To prevent inbreeding costs, many animals avoid sibling matings (Pusey and Wolf, 1996; Penn and Frommen, 2010). However, less studies focus on mating tactics of outbred vs. inbred individuals. In dependence of their inbreeding status, insect females are species-specifically either more (in Tribolium castaneum) (Michalczyk et al., 2011) or less promiscuous (in Drosophila melanogaster) (Dolphin and Carter, 2016). Moreover, the preference for outbred, and thus individuals of higher genetic quality, compared to inbred individuals depends on the level of intra-sexual competition and on the inbreeding status of the choosing sex (Valtonen et al., 2014; Ala-Honkola et al., 2015; Pilakouta and Smiseth, 2017). Partly, the discrimination of inbred individuals could directly be related to sex pheromone odors (Pölkki et al., 2012; van Bergen et al., 2013). However, empirical examples that disentangle the relationship between inbreeding effects on putative chemical (sexual) signaling traits, such as CHCs, and on mating behavior, such as preferences and aggression, are lacking.
In this study, we investigated inbreeding effects on the chemical phenotype as well as on mating-related behavioral traits in a no choice and a dual choice situation. As study organism, we used mustard leaf beetles (Phaedon cochleariae F.; Coleoptera: Chrysomelidae), descending in the third generation from individuals caught in the wild. As chemical traits involved in mate recognition (Geiselhardt et al., 2009) we analyzed the profiles and diversity of compounds present on the cuticle surface (putative CHCs) of virgin outbred and inbred adults. Moreover, the mating probability of outbred and inbred partners was tested in a fully reciprocal no choice set-up. In a dual choice situation, the mate choice of outbred males between outbred and inbred females as well as female aggression were investigated. Previous studies of inbreeding effects on P. cochleariae revealed that wild and laboratory strains suffer from inbreeding depression throughout their ontogeny (Müller and Müller, 2016; Müller and Juškauskas, 2018; Müller et al., 2018). Moreover, the personalities, i.e., behavioral traits that are consistent over time and across several contexts (Dall et al., 2004; Stamps and Groothuis, 2010), of wild outbred and inbred P. cochleariae beetles differ. For example, inbred beetles are bolder in an unprotected environment than outbred individuals (Müller and Juškauskas, 2018).
We hypothesized that inbred beetles express a distinct CHC profile compared to outbred beetles, because CHCs have a genetic basis (Dronnet et al., 2006; Dembeck et al., 2015) and are thus prone to inbreeding (van Bergen et al., 2013; Menzel et al., 2016). We expected a decreased CHC diversity in inbred compared to outbred individuals, as has been detected in an ant species (Menzel et al., 2016). We hypothesized that the mating probability of outbred pairs in a no choice situation is higher than in pairs, in which inbred beetles are involved. Moreover, we expected that outbred males prefer outbred over inbred females in a dual choice situation, because it should be advantageous to choose an outbred female, which has a higher reproductive output (Müller and Juškauskas, 2018). Furthermore, we hypothesized that inbred females are more aggressive than outbred females, because inbred females might invest more in outcompeting outbred females to win a mating opportunity, as inbred have lower future reproductive perspectives than outbred females (Müller et al., 2018).
Materials and Methods
Leaf Beetle Origin and Rearing
The capturing procedure of wild beetles and the following breeding set-up were performed as in Müller and Juškauskas (2018). More than 100 adult P. cochleariae beetles were caught close to Bielefeld, Germany, along the creek Furlbach in 2017. The beetles were collected at three distinct watercress (Nasturtium officinale; Brassicaceae) patches, which were about 30 m apart from each other. The watercress plant patches were standing in the creek and thus beetle subpopulations were isolated by water. We never observed the beetles flying, so we assumed that there was little genetic exchange between these three subpopulations. These wild beetles (parental [P] generation) were transferred to the laboratory and reared in three ventilated plastic boxes (20 × 20 × 6.5 cm), separated by the capturing patch. The boxes were placed in a climate cabinet with constant conditions (20°C, 65% r.h., L16:D8) and beetles fed ad libitum with a mixture of leaves of watercress (seeds from Volmary GmbH, Münster, Germany) and cabbage plants (Brassica rapa L. ssp. pekinensis var. Michihili; Brassicaceae; seeds from Kiepenkerl, Bruno Nebelung GmbH, Konken, Germany), which were 6–8 weeks old and did not flower. The host plants were grown in a greenhouse (60% r.h., L16:D8). The first laboratory generation (F1) of the wild-caught beetles was kept separated by the capturing patch of the parents and solely fed with middle-aged cabbage leaves. Pupae of this generation were isolated and kept in small Petri dishes (5.5 cm diameter) to ensure that emerging adults could not mate freely.
The present study was carried out in accordance with the institutional guidelines at Bielefeld University to work with invertebrate animals in science. No permits were required to perform the experiments described in the present study.
Experimental Set-Up
To investigate inbreeding effects on the chemical phenotype, i.e., the putative CHC profile, and the mating behavior of P. cochleariae, outbred and inbred beetles were generated. Therefore, breeding pairs consisting of one virgin female and one virgin male of the F1 generation, which descended from different capturing patches, were randomly mated.
The offspring (F2 generation) of each breeding pair (F1 generation) was reared in a large Petri dish (9.5 cm diameter), with separate Petri dishes per pair. From the pupal stage on, individuals were isolated and kept in small Petri dishes to prevent unintended matings. After hardening of the cuticle of adults and sex determination, breeding pairs of one female and one male were arranged and the pairs placed in separate small Petri dishes. All Petri dishes were lined with moistened filter papers and the beetles were fed ad libitum with discs (2.5 cm diameter) of middle-aged cabbage leaves, which were exchanged every to every other day. The breeding pairs (F2 generation) consisted of either two siblings (same parents) or of two non-siblings (distinct parents), whereby the parents were considered as “families.” Leaves with eggs of each breeding pair were collected and once the larvae (F3 generation) hatched, they were kept in large Petri dishes, separated by family. After pupation, individuals were separated and kept individually in small Petri dishes. The experiments (chemical phenotype analysis and mating assays) were performed with 14–18 days old outbred and inbred virgin adults of the F3 generation. The beetles are sexually mature from day 5–7 of the adult lifetime onwards and have the highest reproductive output around days 14–18 (Müller and Müller, 2016; Müller and Juškauskas, 2018). The above-described breeding design for three generations ensured that the parents (F2) of individuals considered as offspring (F3) of non-siblings were neither full nor half siblings nor cousins (here defined as “outbred” individuals) and the parents of individuals considered as offspring of siblings were all full siblings (defined as “inbred” individuals). In the outbreeding treatment, 18 distinct families and in the inbreeding treatment 16 distinct families were used to produce the F3 generation.
Beetle Chemical Phenotype Analysis
To determine the chemical phenotypes of adult outbred and inbred beetles, the cuticle surfaces of 14 days old, virgin beetles were extracted and chemically analyzed. In total, the cuticles of 9–10 adult beetles per breeding status (outbred and inbred) and sex were analyzed. Only one randomly chosen male and one female per family were used. Prior to the extraction, beetles were starved for 7 h and thereafter separately frozen at −20°C in 1.5 ml Eppendorf tubes. Before extraction, beetles were thawed for 10 min at room temperature. Subsequently, 60 μl dichloromethane and 5 μl n-eicosane solution (0.1 mg/ml in hexane) as internal standard (IS) were added to each tube, fully covering the beetle, and samples shaken on a vortexer for 15 min at room temperature. Afterwards, extracts were analyzed on a gas-chromatograph coupled with a flame ionization detector (GC-2010 Plus, Shimadzu Corporation, Tokyo, Japan) and equipped with a VF-5 ms column (30 m × 0.25 mm i.d., with 10 m guard column, Agilent Technologies, Santa Clara, United States). Per sample 1 μl was injected with a split ratio of 8 at a constant nitrogen flow of 1.02 ml/min. The temperature program of the oven started at 100°C and was then heated at a rate of 5°C/min to 320°C, which was hold for 22 min. Additionally to the samples, blanks consisting of dichloromethane and the IS were analyzed, as well as a mixture of n-alkanes (C8-C40, Sigma Aldrich, Karlsruhe, Germany) which was used to determine the retentions indices (RI) of all compounds (Kováts, 1958). As CHCs are the most abundant compounds in dichloromethane extracts of beetles (Geiselhardt et al., 2009), we considered all peaks as putative CHCs, although this could not be confirmed. Moreover, for every beetle the relative quantity of putative CHC compounds, which were detectable in at least 50% of the individuals per treatment and sex, was computed on the basis of the integrated peak areas. To detect the CHC diversity of every beetle, the Shannon index was calculated [H' = –Σ pi * ln pi] (Shannon and Weaver, 1949), where pi was the proportional quantity of compound i for the relative quantity of all compounds per beetle.
Mating Behavior
To test for inbreeding effects on the mating behavior of the beetles, a no choice and a dual choice assay were set up with virgin beetles at an age of 14–18 days after adult emergence. Each beetle was used only once, either in the no choice or in the dual choice test. These tests were performed in a photo tent inside an air-conditioned room at 20°C without daylight to exclude unintended external (light) stimuli. The tent was uniformly lit by three lamps (20 W). Both mating tests took place in small Petri dishes, lined with filter paper, which were replaced after every test. Furthermore, Petri dishes were cleaned with ethanol after every test to exclude effects of previous beetle contacts on the next test outcome. After the mating tests, the body mass of each female was measured on a microbalance (ME36S, accuracy 0.001 mg; Sartorius AG, Göttingen, Germany). This was done to account for possible female body mass effects on male mating decision and female aggression behavior in the statistical analyses.
No Choice Mating Assay
To investigate inbreeding effects on the mating probability of the beetles, a no choice assay was set up. One adult male was placed into a small Petri dish and, after an acclimatization time of about 30 s, a female was added. In the following 45 min, it was observed whether a mating occurred. In total, four different pair combinations (N = 15 replicates per combination) were set up, two outbred adults (O/O), an outbred male and an inbred female (O/I), an inbred male and an outbred female (I/O) and two inbred adults (I/I).
Dual Choice Mating Assay and Female Aggression
To test for inbreeding effects on the male mating decision and on female aggression, one outbred male was set up together with one outbred and one inbred female in a dual choice assay (N = 13). First, both females were placed into a Petri dish. After an acclimatization time of about 30 s, one male was added. In the following 60 min, it was observed whether a mating occurred with one female (either outbred or inbred), with both females or with none of the females. Moreover, it was noted when one of the females, both females or none of them were aggressive toward the other female. Aggressive behavior in this species is characterized by biting or blocking other females in order to inhibit the mating of the male with a distinct female. In P. cochleariae, males start the mating attempts (Müller and Müller, 2016). Because we never observed male-male aggression in our rearing, dual choice experiments were not performed with one female and two males.
To distinguish between the females during the test, all females were marked with nail polish (Wilde Cosmetics GmbH, Oestrich-Winkel, Germany). Therefore, half of the outbred and of the inbred females were marked with the same color, either crème or light orange. The front third of the elytra was marked with a small dot of nail polish using a needle. In a pre-experiment, the effect of nail polish on male mating decision in a dual choice test was determined. A male had a choice between an unmarked and a nail polish-marked female (both females outbred, N = 14). The males did not discriminate between the unmarked and marked females and mated either one or the other more readily in similar frequency (data not shown). To prevent effects of the volatile ingredients of the nail polish on the mating decision, the females were marked 2 days prior to the dual choice assay.
Statistical Analyses
The statistical analyses and figures were done with the program R, version 3.2.3 (R Development Core Team, 2015). For visualization of the chemical phenotype (profile of all detected putative CHCs) of outbred and inbred (factor breeding status) males and females (factor sex), a non-metric multidimensional scaling (NMDS) with a Wisconsin double standardization of the square root of the data was calculated based on Bray-Curtis distance matrices (R-package: “vegan”; Oksanen et al., 2017). To test for the impacts of the factors breeding status, sex and their interaction on the CHC profiles, a permutational multivariate analysis of variance using distance matrices (ADONIS) based on Bray-Curtis matrices was performed with 50,000 permutations. A linear model (LM) was calculated to test for the effects of the factors breeding status, sex and their interaction on the Shannon index of the CHCs per beetle, i.e., CHC diversity, as response variable, because this response variable had a normal error distribution.
The data of all mating assays were analyzed with generalized linear mixed-effects models (GLMM; R-package: “lme4”; Bates et al., 2014), because the response variables had binomial error distributions. The model for the response factor mating probability in a no choice assay (mating: yes or no) included the fixed effects breeding status of the male and breeding status of the female mating partner, body mass of females (covariate) and their interactions. The family of the males and females were added as random effects. To quantify inbreeding costs of a decreased mating probability, the coefficient of inbreeding depression [δ = ( – ) / ] was calculated in accordance with Hedrick and Kalinowski (2000). Based on this formula, the mean value, i.e., mating probability, of outbred () and inbred () pairs could be compared, and thus the extent of inbreeding depression was evaluated.
The model for the response factor mating decision of an outbred male in a dual choice assay (mating with an outbred and/or with an inbred female: yes or no) comprised the fixed effects of female breeding status, female body mass used as covariate and their interaction. The model for the response factor female aggression in a dual choice assay (aggression: yes or no) included the fixed effects of female breeding status, female body mass and their interaction. In both models on data of the dual choice assays, the ID of the male and the family of the female were included as random effects.
After ensuring that models exhibit variance homogeneity and normal distribution of the residuals, a maximum likelihood approach was used. Step-wise backward model selections were applied to get the minimal adequate models, i.e., based on likelihood ratio tests (R-package: “MASS”, Venables and Ripley, 2000) interactions of the fixed effects were removed when P > 0.05. All models were fitted with the default link function. For visualization of the effects of breeding status and sex, least square means with their standard errors were extracted from the respective minimal adequate models (R-package: “lsmeans,” Lenth, 2016). These model estimates account for the specific error distribution of the response factors, for the effects of covariates and for random effects. All data are accessible at the public storage platform of Bielefeld University (https://pub.uni-bielefeld.de/).
Results
Inbreeding Effects on Chemical Phenotype
In total, 19 putative CHCs could be detected, ranging from RI 1822 to RI 3993 (Table 1). The chemical phenotype, i.e., the CHC profile, of adult beetles was significantly affected by the sex of the individuals and the interaction of the breeding status and the sex (Table 2). Notably, the CHC profiles of outbred females were most distinct from the CHC profiles of the other treatment groups (Figure 1). The CHC profiles of inbred females were more similar to those of males than to those of the outbred females, with two compounds (RI 2918 and 3500) co-occurring in males and inbred females, but not in outbred females, and several compounds, e.g., RI 1822, RI 2026, and RI 2863, being much more abundant in all males and inbred females than in outbred females.
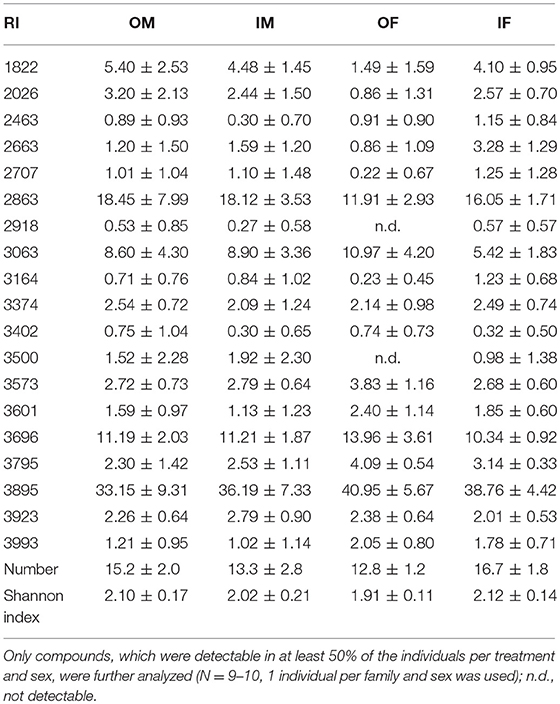
Table 1. Relative quantities (mean % peak are ± SD), number and Shannon index of putative cuticular hydrocarbons, indicated by their retention index (RI), of outbred (O) and inbred (I) adult Phaedon cochleariae males (M) and females (F).
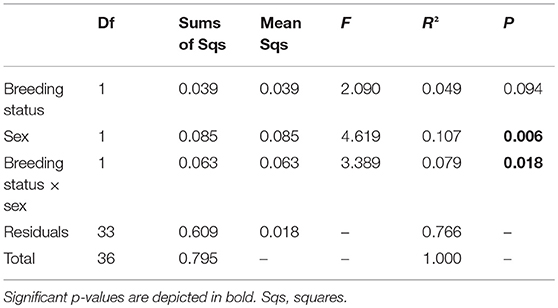
Table 2. Analysis of variance using distance matrices (ADONIS) with 50,000 permutations based on Bray-Curtis distances on the effects of the breeding status (outbred and inbred individuals), sex and their interaction on the chemical phenotype, i.e., profile of putative cuticular hydrocarbons, of adult Phaedon cochleariae (N = 9–10 per breeding status and sex).
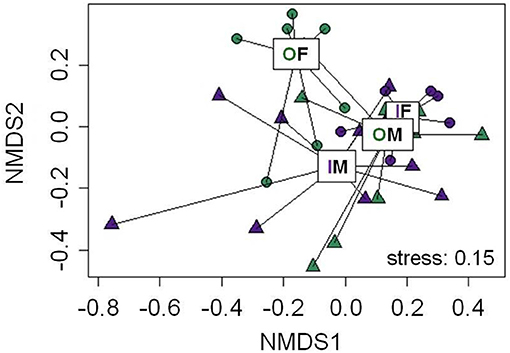
Figure 1. Non-metric multidimensional scaling (NMDS) plot for the chemical phenotype, i.e., profile of putative cuticular hydrocarbons, of adult Phaedon cochleariae females (F, circles) and males (M, triangles), which either were outbred (O, green) or inbred (I, purple). Ordispiders connect individual data points with lines to displayed treatment medians (boxes). The stress-level is depicted at the bottom right of the plot. Statistical results of an ADONIS are given in Table 2. In total, 19 compounds, which were detectable in at least 50% of the individuals per treatment and sex (Table 1), were included in the NMDS plot.
The CHC diversity was significantly affected by the interaction of the breeding status and the sex (LM: df = 1, F = 6.590; p = 0.015; Figure 2). The Shannon indices of the CHC profiles of inbred females and outbred males were the highest and the Shannon index of outbred females was the lowest.
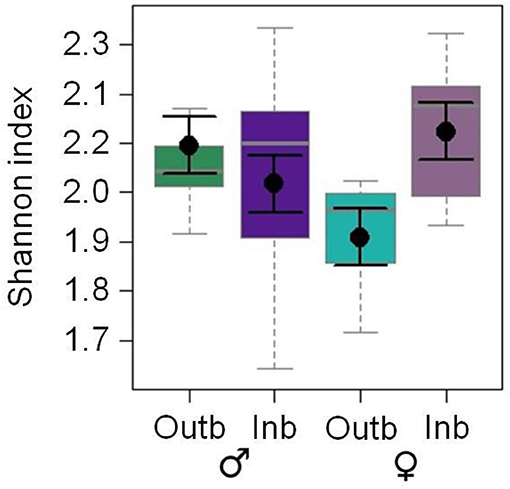
Figure 2. Effects of the factor breeding status [outbred (Outb; green) and inbred (Inb; purple)] and sex [males (dark) and females (light)] on the Shannon index of putative cuticular hydrocarbons of adult Phaedon cochleariae (N = 9–10 adults per breeding status and sex; analyzed with a LM). The box-whisker plots show the medians (solid gray lines), interquartile ranges (boxes), 1.5 * lower/upper quartiles (whiskers) and least square means with their standard errors (black dots with error bars) extracted from the minimal adequate LM. One outlier above 2.5 for the outbred males is not shown in the figure but was included in the statistical analysis.
Inbreeding Effects on Mating Behavior in a No Choice Situation
The mating probability within 45 min in a no choice assay was significantly affected by the breeding status of the male (GLMM: df = 1, X2 = 5.266; p = 0.022; Figure 3, Figure S1) and by the breeding status of the female (df = 1, X2 = 10.405; P = 0.001) mating partner. Outbred pairs had the highest mating probability (mean of 86.7%) and inbred pairs the lowest (20%), resulting in an inbreeding depression coefficient of δ = 0.77. Pairs consisting of an inbred male and an outbred female (66.6%) and of an outbred male and an inbred female (53.3%) had intermediate mating probabilities. The mating probability was not affected by the mass of the female mating partner (df = 1, X2 = 0.052, p = 0.820) or by any of the two or three way interactions of male and/or female breeding status and/or female body mass.
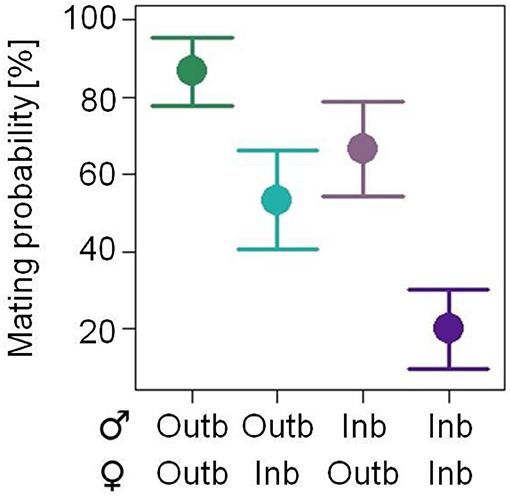
Figure 3. Effects of the factor mating combination of either two outbred (Outb) mating partners (dark green), an outbred male and an inbred (Inb) female (light green), an inbred male and an outbred female (light purple) or two inbred mating partners (dark purple) of Phaedon cochleariae on the mating probability within 45 min in a no choice situation (N = 15 per combination; analyzed with a GLMM). The least square means with their standard errors (black dots with error bars) extracted from the minimal adequate GLMM are shown.
Inbreeding Effects on Mating Behavior and Female Aggression in a Dual Choice Situation
The mating decision of an outbred male in a dual choice situation between an outbred and an inbred female was not affected by the breeding status of the female (GLMM: df = 1, X2 = 0.117, p = 0.732; Figure S2). Outbred males mated on average with 69% of the outbred and 46% of the inbred females. One male did not mate with any of the females, 69% of the males mated one of the females and 23% both females. Moreover, the male mating decision was not affected by the mass of the females (df = 1, X2 = 1.995, p = 0.158) or the interaction of the breeding status and the mass of the females (df = 1, X2 = 2.206, p = 0.138).
Female aggression was significantly impacted by the breeding status of the female (GLMM: df = 1, X2 = 12.718, p < 0.001; Figure S2). Inbred females were more aggressive than outbred females. On average, 62% of the inbred females were aggressive, whereas only 15% of the outbred females were aggressive. Female aggression was not affected by their body mass (GLMM: df = 1, X2 = 0.056, p = 0.814) or by the interaction of their breeding status and mass (GLMM: df = 1, X2 = 2.006, p = 0.157).
Discussion
The present study disclosed that the chemical phenotype, mating probability and female aggression are affected by inbreeding in P. cochleariae. Inbreeding effects on the profile of putative CHCs and their diversity were only partly in line with our hypotheses. In line with our expectation, the CHC profile of females differed in dependence of breeding status, whereby inbred females showed a “male-like” chemical phenotype. This is surprising, because CHC profiles are usually sex-specific in P. cochleariae (Geiselhardt et al., 2009) and in many other insect species (Singer, 1998; Weddle et al., 2012). In our study, several CHCs were expressed in males and inbred, but not, or in a much lower relative quantity, in outbred females. A masculinization of female CHCs may be achieved by genetic manipulation of a sex determination factor of the oenocytes, cells that are involved in CHC production, as found in Drosophila melanogaster (Savarit and Ferveur, 2002; de la Paz Fernández et al., 2010). Moreover, transcriptomic masculinization can occur in conditions, in which male-biased genes are upregulated and female-biased or unbiased genes are not modified (Veltsos et al., 2017).
In contrast to our expectation, we found an increased CHC diversity in inbred compared to outbred females. CHCs are shaped by a combination of intrinsic and external environmental factors (Otte et al., 2018). Due to the genetic basis of CHCs (Foley et al., 2007; Weddle et al., 2013; Dembeck et al., 2015), inbreeding effects on the CHC composition might rely on a decreased homozygosity in inbred compared to outbred individuals (Menzel et al., 2016). Interestingly, as suggested in the present study, a decreased heterozygosity as consequence of inbreeding might not necessarily lead to a decreased CHC number and diversity. Species-specific effects of inbreeding on the CHC diversity (this study; Menzel et al., 2016) may be due to distinct genetic architectures or social lifestyles of the study organisms. The genetic mechanisms behind these inbreeding-dependent sex-specific changes of the CHC profile and diversity as well as the relevance for distinct arthropod species need to be further explored.
CHCs are important for the waterproofing of the insect cuticle and function as defense against microbial parasites (Lockey, 1988; Pedrini et al., 2013). So far, it remains unclear whether inbreeding-induced changes in CHC profiles might alter the susceptibility of inbred individuals to desiccation and parasite infestations in P. cochleariae or other arthropods. Moreover, CHCs mediate intra- and interspecific communication in many arthropod communities (Howard and Blomquist, 2005). Thus, inbreeding effects on the chemical phenotype might have far-reaching consequences on an intraspecific level, e.g., in terms of mate choice (van Bergen et al., 2013), and possibly also on an interspecific level, e.g., in terms of predator-prey interactions.
As hypothesized, the mating probability in a no choice situation was significantly reduced with inbred partners. These detrimental inbreeding effects on mating probability are likely not explainable by a potentially reduced activity of inbred compared to outbred individuals, because activity patterns do not differ between inbred and outbred P. cochleariae beetles (Müller and Juškauskas, 2018), as also observed in Drosophila littoralis (Ala-Honkola et al., 2015). However, other behavioral alterations, for example, in boldness or exploration, may be induced by inbreeding, affecting mating. Moreover, the decreased mating probability of inbred P. cochleariae may be related to the altered composition of the chemical surface compounds of the females. CHCs mediate mating decisions in many arthropod species (Howard and Blomquist, 2005), including P. cochleariae. In this chrysomelid the food plays an important role in shaping the CHC profiles and consequently influences mating preferences (Geiselhardt et al., 2012). To test the role of CHCs for mating in outbred vs. inbred individuals, female extracts might be applied on glass dummies and offered to males. However, beetles of the wild population used in the present study did not accept glass dummies as “mating partners” (personal observation), contrary to laboratory strains of this species (Geiselhardt et al., 2009, 2012) and wild strains of the raspberry weevil (Mutis et al., 2009). Moreover, inbreeding can affect morphological traits (DeRose and Roff, 1999), such as the antennal length, as, for example, found in the aphid Prociphilus oriens (Akimoto, 2006). This may go along with a disrupted functioning of the antennae, i.e., the perception of chemical signaling traits. Such possible changes may also explain the lower mating probability of inbred compared to outbred individuals. Overall, irrespective of the underlying mechanisms, inbreeding has the potential to impair the mating acceptance of males (Mariette et al., 2006; Ala-Honkola et al., 2009), females (Ala-Honkola et al., 2015) or of both sexes (present study) in distinct species. This so far underestimated aspect of inbreeding depression might exacerbate threats of wild populations that suffer from inbreeding.
Interestingly, in a dual choice situation outbred males did not preferentially mate outbred compared to inbred females, in contrast to our expectation. If the experiment would have lasted longer, males probably would have mated both females to increase their reproductive output. In our experimental set-up, males might have preferentially mated with the female that first crossed their path. This “take what you get principle” might enhance male fitness in a non-competitive situation as long as the male can mate both females. Indeed, inbreeding effects on mating decisions can depend on the presence of competitors. In Drosophila melanogaster, a competitive situation leads to negative inbreeding effects on male attractiveness, which is not observable in a non-competitive situation (Valtonen et al., 2014). In a competitive situation, the behavior of the competing individuals of the same sex can influence mating decisions. Inbred P. cochleariae females are bolder (Müller and Juškauskas, 2018) and more aggressive (this study) than outbred females, which may also influence the mating preference of males, potentially leading to a reduced mating with outbred females.
The increased aggression of inbred compared to outbred females might be a result of inbreeding depression that leads to behavioral disorders or could be interpreted as consequence of terminal investment. An increased competitive level of inbred compared to outbred females for a present reproductive opportunity might be based on lower fitness perspectives, which have indeed been revealed in inbred P. cochleariae (Müller et al., 2018). Similarly, inbred burying beetle males show higher aggression and have a higher mortality and thus an expectable lower reproductive perspective than outbred males (Richardson and Smiseth, 2017). Furthermore, the results of the present study can be discussed in the light of indirect genetic effects (IGEs), that is, how genes of one individual influence (behavioral) traits of another individual via social interactions (Bailey et al., 2017). The role of IGEs in behavioral ecology becomes increasingly apparent (Bailey et al., 2017). Genes that are expressed in social interaction partners and affect their phenotype can contribute to the environment that shapes the phenotype of other individuals. With regard to the present study, the high aggression of inbred females might lead to negative effects on the fitness of outbred females, either due to injuries or to prevented copulations. In line with that, inbred burying beetle males were more aggressive and thus impaired the reproductive success (and thus fitness) of outbred males in competitive situations (Richardson and Smiseth, 2017), highlighting the severity of IGEs under such conditions. However, same-sex contests are not necessarily detrimental for the fitness of one or both interaction partners. For example, burying beetle females might increase their post-hatching brood-care in response to a juvenile contest experience with a distinct female (Pilakouta et al., 2016). In the present study, outbred females of P. cochleariae, which had a contest experience, might likewise increase their effort to find a more suitable oviposition site for their offspring. Thus, depending on the context, IGEs can either be detrimental or beneficial (Bailey et al., 2017; Schneider et al., 2017). In conclusion, if IGEs on phenotypic traits frequently occur, also evolutionary dynamics underlying these fitness or behavioral traits can be altered (Bailey et al., 2017; Schneider et al., 2017).
Both a no and a dual choice situation of potential mating partners can display realistic scenarios in nature. In a non-competitive situation with just one potential mating partner, the decision is to accept or reject the mating partner (Shackleton et al., 2005; Geiselhardt et al., 2012). If the mating partner is rejected, reproductive resources can be saved for an upcoming mating opportunity. However, after a rejection, individuals run the risk of not facing a further mating partner. In a dual choice situation, the choosing sex can choose the mate that is expected to have the higher (genetic) quality (Berglund and Rosenqvist, 2001; Fisher and Cockburn, 2005). The competing sex has to deal with same-sex opponents, which might influence the mate choice of the choosy sex (Doutrelant and McGregor, 2000; Nilsson and Nilsson, 2000; Hunt et al., 2009). Consequently, outcomes of mating assays can drastically differ between no and multiple choice situations (this study; Shackleton et al., 2005; Valtonen et al., 2014) and studies run the risk to miss relevant information if only one approach is conducted.
Inbreeding can affect personalities (Manenti et al., 2015; Richardson and Smiseth, 2017; Müller and Juškauskas, 2018) and various mating-related behavioral traits (this study; Aspi, 2000; Drayton et al., 2007; Ala-Honkola et al., 2009), partly explainable by changes in chemical or acoustic (sexual) signaling traits. On a wider scale, population dynamics may change if the proportion of inbred individuals is increasing. Changes might be due to a decreased fitness (Keller and Waller, 2002; Peng et al., 2015) and/or behavioral alterations, i.e., distinct boldness or aggression behavior, of inbred compared to outbred individuals (Eklund, 1996; Richardson and Smiseth, 2017; Müller and Juškauskas, 2018). If inbreeding leads to population size declines (Agnarsson et al., 2013; Peng et al., 2015), this may influence several trophic levels of a food chain, potentially expressed through interspecific IGEs. Indeed, examples of such effects exist in insect predator-prey and herbivore-plant interactions (Khudr et al., 2013; Rebar and Rodríguez, 2014; Bailey et al., 2017). Ultimately, evolutionary processes of ecological communities can be influenced by IGEs between distinct species (Genung et al., 2013a,b).
In conclusion, the severe effects of inbreeding on mating probability, at least in no choice situations, might be influenced by the altered, male-like CHC profile of inbred P. cochleariae females. Moreover, behavioral changes in aggression (this study; Richardson and Smiseth, 2017) and boldness behavior (Müller and Juškauskas, 2018) of inbred compared to outbred individuals likely contribute to inbreeding depression in mating-related behavioral traits. As suggested in the present study, males might benefit by preferentially choosing outbred females based on these chemical and/or behavioral traits, gaining good quality mates. Thus, sexual selection likely contributes to the evolution of chemical signaling traits (Steiger and Stökl, 2014). However, to shed new light on this neglected research field, the combination of behavioral, chemical and evolutionary approaches is urgently required. More empirical data will help to understand whether and how inbreeding-related alterations of signaling processes drive evolutionary processes underlying sexual selection and mating strategies.
Author Contributions
TM designed the study. LL and TM conducted the breeding and mating experiments and collected the data. TM conducted the chemical analyses. TM analyzed the data and prepared the figures. TM and CM wrote the manuscript.
Conflict of Interest Statement
The authors declare that the research was conducted in the absence of any commercial or financial relationships that could be construed as a potential conflict of interest.
Acknowledgments
We thank Ruth Jakobs for help in GC-FID maintenance and the gardeners of Bielefeld University for their help in plant rearing. We acknowledge support for the article processing charge by the Deutsche Forschungsgemeinschaft and the Open Access Publication Fund of Bielefeld University.
Supplementary material
The Supplementary Material for this article can be found online at: https://www.frontiersin.org/articles/10.3389/fevo.2018.00177/full#supplementary-material
References
Agnarsson, I., Avilés, L., and Maddison, W. P. (2013). Loss of genetic variability in social spiders: genetic and phylogenetic consequences of population subdivision and inbreeding. J. Evol. Biol. 26, 27–37. doi: 10.1111/jeb.12022
Akimoto, S. (2006). Inbreeding depression, increased phenotypic variance, and a trade-off between gonads and appendages in selfed progeny of the aphid Prociphilus oriens. Evolution 60, 77–86. doi: 10.1111/j.0014-3820.2006.tb01083.x
Ala-Honkola, O., Laine, L., Pekkala, N., Kotiaho, J. S., Honkola, T., and Puurtinen, M. (2015). Males benefit from mating with outbred females in Drosophila littoralis: male choice for female genetic quality? Ethology 121, 577–585. doi: 10.1111/eth.12369
Ala-Honkola, O., Uddström, A., Pauli, B. D., and Lindström, K. (2009). Strong inbreeding depression in male mating behaviour in a poeciliid fish. J. Evol. Biol. 22, 1396–1406. doi: 10.1111/j.1420-9101.2009.01765.x
Aspi, J. (2000). Inbreeding and outbreeding depression in male courtship song characters in Drosophila montana. Heredity 84(Pt. 3), 273–282. doi: 10.1046/j.1365-2540.2000.00655.x
Bailey, N. W., Marie-Orleach, L., and Moore, A. J. (2017). Indirect genetic effects in behavioral ecology: does behavior play a special role in evolution? Behav. Ecol. 29, 1–11. doi: 10.1093/beheco/arx127
Bates, D., Maechler, M., and Bolker B Walker, S. (2014). Linear Mixed-Effects Models Using Eigen and S4. R package version 1.1.12. Available online at: http://cran.R-project.org/package=lme4.
Berglund, A., and Rosenqvist, G. (2001). Male pipefish prefer dominant over attractive females. Behav. Ecol.1 12, 402–406. doi: 10.1093/beheco/12.4.402
Charlesworth, D., and Charlesworth, B. (1987). Inbreeding depression and its evolutionary consequences. Annu. Rev. Ecol. Syst. 18, 237–268. doi: 10.1146/annurev.es.18.110187.001321
Charlesworth, D., and Willis, J. H. (2009). The genetics of inbreeding depression. Nat. Rev. Genet. 10, 783–796. doi: 10.1038/nrg2664
Dall, S. R. X., Houston, A. I., and McNamara, J. M. (2004). The behavioural ecology of personality: consistent individual differences from an adaptive perspective. Ecol. Lett. 7, 734–739. doi: 10.1111/j.1461-0248.2004.00618.x
de la Paz Fernández, M., Chan, Y.-B., Yew, J. Y., Billeter, J.-C., Dreisewerd, K., Levine, J. D., et al. (2010). Pheromonal and behavioral cues trigger male-to-female aggression in Drosophila. PLoS Biol. 8:e1000541. doi: 10.1371/annotation/1c19d040-9f9f-4b9f-b678-70f1fe387192
Dembeck, L. M., Böröczky, K., Huang, W., Schal, C., Anholt, R. R., and Mackay, T. F. (2015). Genetic architecture of natural variation in cuticular hydrocarbon composition in Drosophila melanogaster. Elife 4:e09861. doi: 10.7554/eLife.09861
DeRose, M. A., and Roff, D. A. (1999). A comparison of inbreeding depression in life-history and morphological traits in animals. Evolution 53, 1288–1292. doi: 10.1111/j.1558-5646.1999.tb04541.x
Dolphin, K., and Carter, A. (2016). Inbreeding decreases promiscuity in Drosophila melanogaster females. Ethol. Ecol. Evol. 28, 202–210. doi: 10.1080/03949370.2015.1039466
Doutrelant, C., and McGregor, P. K. (2000). Eavesdropping and mate choice in female fighting fish. Behaviour 137, 1655–1668. doi: 10.1163/156853900502763
Drayton, J., Hunt, J., Brooks, R., and Jennions, M. (2007). Sounds different: inbreeding depression in sexually selected traits in the cricket Teleogryllus commodus. J. Evol. Biol. 20, 1138–1147. doi: 10.1111/j.1420-9101.2006.01286.x
Drayton, J. M., Milner, R. N., Hunt, J., and Jennions, M. D. (2010). Inbreeding and advertisement calling in the cricket Teleogryllus commodus: laboratory and field experiments. Evolution 64, 3069–3083. doi: 10.1111/j.1558-5646.2010.01053.x
Dronnet, S., Lohou, C., Christides, J.-P., and Bagnères, A.-G. (2006). Cuticular hydrocarbon composition reflects genetic relationship among colonies of the introduced termite Reticulitermes santonensis Feytaud. J. Chem. Ecol. 32, 1027–1042. doi: 10.1007/s10886-006-9043-x
Eklund, A. (1996). The effects of inbreeding on aggression in wild male house mice (Mus domesticus). Behaviour 133, 883–901. doi: 10.1163/156853996X00297
Fisher, D. O., and Cockburn, A. (2005). The large-male advantage in brown antechinuses: female choice, male dominance, and delayed male death. Behav. Ecol. 17, 164–171. doi: 10.1093/beheco/arj012
Foley, B., Chenoweth, S. F., Nuzhdin, S. V., and Blows, M. W. (2007). Natural genetic variation in cuticular hydrocarbon expression in male and female Drosophila melanogaster. Genetics 175, 1465–1477. doi: 10.1534/genetics.106.065771
Geiselhardt, S., Otte, T., and Hilker, M. (2009). The role of cuticular hydrocarbons in male mating behavior of the mustard leaf beetle, Phaedon cochleariae (F.). J. Chem. Ecol. 35, 1162–1171. doi: 10.1007/s10886-009-9704-7
Geiselhardt, S., Otte, T., and Hilker, M. (2012). Looking for a similar partner: host plants shape mating preferences of herbivorous insects by altering their contact pheromones. Ecol. Lett. 15, 971–977. doi: 10.1111/j.1461-0248.2012.01816.x
Genung, M. A., Bailey, J. K., and Schweitzer, J. A. (2013a). The afterlife of interspecific indirect genetic effects: genotype interactions alter litter quality with consequences for decomposition and nutrient dynamics. PLoS ONE 8:e53718. doi: 10.1371/journal.pone.0053718
Genung, M. A., Bailey, J. K., and Schweitzer, J. A. (2013b). Belowground interactions shift the relative importance of direct and indirect genetic effects. Ecol. Evol. 3, 1692–1701. doi: 10.1002/ece3.582
Griggio, M., Biard, C., Penn, D. J., and Hoi, H. (2011). Female house sparrows “count on” male genes: experimental evidence for MHC-dependent mate preference in birds. BMC Evol. Biol. 11:44. doi: 10.1186/1471-2148-11-44
Hedrick, P. W., and Kalinowski, S. T. (2000). Inbreeding depression in conservation biology. Ann. Rev. Ecol. Syst. 31, 139–162.
Howard, R. W., and Blomquist, G. J. (2005). Ecological, behavioral, and biochemical aspects of insect hydrocarbons. Ann. Rev. Entomol. 50, 371–393. doi: 10.1146/annurev.ento.50.071803.130359
Hunt, J., Breuker, C. J., Sadowski, J. A., and Moore, A. J. (2009). Male–male competition, female mate choice and their interaction: determining total sexual selection. J. Evol. Biol. 22, 13–26. doi: 10.1111/j.1420-9101.2008.01633.x
Ilmonen, P., Stundner, G., Thoß, M., and Penn, D. J. (2009). Females prefer the scent of outbred males: good-genes-as-heterozygosity? BMC Evol. Biol. 9:104. doi: 10.1186/1471-2148-9-104
Keller, L. F., and Waller, D. M. (2002). Inbreeding effects in wild populations. Trends Ecol. Evol. 17, 230–241. doi: 10.1016/S0169-5347(02)02489-8
Keppner, E. M., Prang, M., Engel, K. C., Ayasse, M., Stökl, J., and Steiger, S. (2017). Beyond cuticular hydrocarbons: chemically mediated mate recognition in the subsocial burying beetle Nicrophorus vespilloides. J. Chem. Ecol. 43, 84–93. doi: 10.1007/s10886-016-0806-8
Khudr, M. S., Oldekop, J. A., Shuker, D. M., and Preziosi, R. F. (2013). Parasitoid wasps influence where aphids die via an interspecific indirect genetic effect. Biol. Lett. 9:20121151. doi: 10.1098/rsbl.2012.1151
Kokko, H., and Ots, I. (2006). When not to avoid inbreeding. Evolution 60, 467–475. doi: 10.1111/j.0014-3820.2006.tb01128.x
Kováts, E. (1958). Gas-chromatographische Charakterisierung organischer Verbindungen.1. Retentionsindices aliphatischer Halogenide, Alkohole, Aldehyde und Ketone. Helv. Chim. Acta 41, 1915–1932.
Kuriwada, T., Kumano, N., Shiromoto, K., and Haraguchi, D. (2011). The effect of inbreeding on mating behaviour of west indian sweet potato weevil Euscepes postfasciatus. Ethology 117, 822–828. doi: 10.1111/j.1439-0310.2011.01937.x
Lenth, R. V. (2016). Least square means: the R package lsmeans. J. Stat. Softw. 69, 1–33. doi: 10.18637/jss.v069.i01
Lihoreau, M., and Rivault, C. (2009). Kin recognition via cuticular hydrocarbons shapes cockroach social life. Behav. Ecol. 20, 46–53. doi: 10.1093/beheco/arn113
Lockey, K. H. (1988). Lipids of insect cuticle–origin, composition and function. Comp. Biochem. Physiol. B Biochem. Mol. Biol. 89, 595–645. doi: 10.1016/0305-0491(88)90305-7
Manenti, T., Pertoldi, C., Nasiri, N., Schou, M. F., Kjærsgaard, A., Cavicchi, S., et al. (2015). Inbreeding affects locomotor activity in Drosophila melanogaster at different ages. Behav. Genet. 45, 127–134. doi: 10.1007/s10519-014-9683-5
Mariette, M., Kelley, J. L., Brooks, R., and Evans, J. P. (2006). The effects of inbreeding on male courtship behaviour and coloration in guppies. Ethology 112, 807–814. doi: 10.1111/j.1439-0310.2006.01236.x
Menzel, F., Radke, R., and Foitzik, S. (2016). Odor diversity decreases with inbreeding in the ant Hypoponera opacior. Evolution 70, 2573–2582. doi: 10.1111/evo.13068
Meunier, J., and Kölliker, M. (2013). Inbreeding depression in an insect with maternal care: influences of family interactions, life stage and offspring sex. J. Evol. Biol. 26, 2209–2220. doi: 10.1111/jeb.12217
Michalczyk, L., Millard, A. L., Martin, O. Y., Lumley, A. J., Emerson, B. C., Chapman, T., et al. (2011). Inbreeding promotes female promiscuity. Science 333, 1739–1742. doi: 10.1126/science.1207314
Müller, T., and Juškauskas, A. (2018). Inbreeding affects personality and fitness of a leaf beetle. Anim. Behav. 138, 29–37. doi: 10.1016/j.anbehav.2018.02.002
Müller, T., Lamprecht, T. D., and Schrieber, K. (2018). Lifetime inbreeding depression in a leaf beetle. Ecol. Evol. 8, 6889–6898. doi: 10.1002/ece3.4205
Müller, T., and Müller, C. (2016). Consequences of mating with siblings and nonsiblings on the reproductive success in a leaf beetle. Ecol. Evol. 6, 3185–3197. doi: 10.1002/ece3.2103
Mutis, A., Parra, L., Palma, R., Pardo, F., Perich, F., and Quiroz, A. (2009). Evidence of contact pheromone use in mating behavior of the raspberry weevil (Coleoptera: Curculionidae). Environ. Entomol. 38, 192–197. doi: 10.1603/022.038.0124
Nilsson, S. Ö., and Nilsson, G. E. (2000). Free choice by female sticklebacks: lack of preference for male dominance traits. Can. J. Zool. 78, 1251–1258. doi: 10.1139/z00-051
Oksanen, J., Blanchet, F. G., Friendly, M., Kindt, R., Legendre, P., McGlinn, D., et al. (2017). Vegan: Community Ecology Package. R-package version 2.4.-3. Available online at: http://CRAN.R-project.org/package=vegan.
Otte, T., Hilker, M., and Geiselhardt, S. (2018). Phenotypic plasticity of cuticular hydrocarbon profiles in insects. J. Chem. Ecol. 44, 235–247. doi: 10.1007/s10886-018-0934-4
Pedrini, N., Ortiz-Urquiza, A., Huarte-Bonnet, C., Zhang, S., and Keyhani, N. O. (2013). Targeting of insect epicuticular lipids by the entomopathogenic fungus Beauveria bassiana: hydrocarbon oxidation within the context of a host-pathogen interaction. Front. Microbiol. 4:24. doi: 10.3389/fmicb.2013.00024
Peng, L., Zou, M., Ren, N., Xie, M., Vasseur, L., Yang, Y., et al. (2015). Generation-based life table analysis reveals manifold effects of inbreeding on the population fitness in Plutella xylostella. Sci. Rep. 5:12749. doi: 10.1038/srep12749
Penn, J. P., and Frommen, J. G. (2010). “Kin recognition: an overview of conceptual issues, mechanisms and evolutionary theory,” in Animal Behaviour: Evolution and Mechanisms, ed P. Kappeler (Heidelberg: Springer), 55–85.
Pilakouta, N., Halford, C., Rácz, R., and Smiseth, P. T. (2016). Effects of prior contest experience and contest outcome on female reproductive decisions and offspring fitness. Am. Nat. 188, 319–328. doi: 10.1086/687392
Pilakouta, N., and Smiseth, P. T. (2017). Female mating preferences for outbred versus inbred males are conditional upon the female's own inbreeding status. Anim. Behav. 123, 369–374. doi: 10.1016/j.anbehav.2016.11.023
Pölkki, M., Krams, I., Kangassalo, K., and Rantala, M. J. (2012). Inbreeding affects sexual signalling in males but not females of Tenebrio molitor. Biol. Lett. 8, 423–425. doi: 10.1098/rsbl.2011.1135
Pusey, A., and Wolf, M. (1996). Inbreeding avoidance in animals. Trends Ecol. Evol. 11, 201–206. doi: 10.1016/0169-5347(96)10028-8
R Development Core Team (2015). R: A Language and Environment for Statistical Computing. Version 3.2.3. Available online at: http://cran.R-project.org/.
Rebar, D., and Rodríguez, R. L. (2014). Trees to treehoppers: genetic variation in host plants contributes to variation in the mating signals of a plant-feeding insect. Ecol. Lett. 17, 203–210. doi: 10.1111/ele.12220
Richardson, J., and Smiseth, P. T. (2017). Intraspecific competition and inbreeding depression: increased competitive effort by inbred males is costly to outbred opponents. Am. Nat. 189, 539–548. doi: 10.1086/691328
Savarit, F., and Ferveur, J.-F. (2002). Genetic study of the production of sexually dimorphic cuticular hydrocarbons in relation with the sex-determination gene transformer in Drosophila melanogaster. Genet. Res. 79, 23–40. doi: 10.1017/S0016672301005481
Schneider, J., Atallah, J., and Levine, J. D. (2017). Social structure and indirect genetic effects: genetics of social behaviour. Biol. Rev. 92, 1027–1038. doi: 10.1111/brv.12267
Shackleton, M. A., Jennions, M. D., and Hunt, J. (2005). Fighting success and attractiveness as predictors of male mating success in the black field cricket, Teleogryllus commodus: the effectiveness of no-choice tests. Behav. Ecol. Sociobiol. 58, 1–8. doi: 10.1007/s00265-004-0907-1
Shannon, C., and Weaver, W. (1949). The Mathmatical Theory of Information. Urbana, IL: University of Illinois Press.
Singer, T. L. (1998). Roles of hydrocarbons in the recognition systems of insects. Am. Zool. 38, 394–405. doi: 10.1093/icb/38.2.394
Stamps, J., and Groothuis, T. G. G. (2010). The development of animal personality: relevance, concepts and perspectives. Biol. Rev. 85, 301–325. doi: 10.1111/j.1469-185X.2009.00103.x
Steiger, S., and Stökl, J. (2014). The role of sexual selection in the evolution of chemical signals in insects. Insects 5, 423–438. doi: 10.3390/insects5020423
Szulkin, M., Stopher, K. V., Pemberton, J. M., and Reid, J. M. (2013). Inbreeding avoidance, tolerance, or preference in animals? Trends Ecol. Evol. 28, 205–211. doi: 10.1016/j.tree.2012.10.016
Thom, M. D., Stockley, P., Jury, F., Ollier, W. E., Beynon, R. J., and Hurst, J. L. (2008). The direct assessment of genetic heterozygosity through scent in the mouse. Curr. Biol. 18, 619–623. doi: 10.1016/j.cub.2008.03.056
Valtonen, T. M., Roff, D. A., and Rantala, M. J. (2014). The deleterious effects of high inbreeding on male Drosophila melanogaster attractiveness are observed under competitive but not under non-competitive conditions. Behav. Genet. 44, 144–154. doi: 10.1007/s10519-013-9639-1
van Bergen, E., Brakefield, P. M., Heuskin, S., Zwaan, B. J., and Nieberding, C. M. (2013). The scent of inbreeding: a male sex pheromone betrays inbred males. Proc. R. Soc. B 280:20130102. doi: 10.1098/rspb.2013.0102
Veltsos, P., Fang, Y., Cossins, A. R., Snook, R. R., and Ritchie, M. G. (2017). Mating system manipulation and the evolution of sex-biased gene expression in Drosophila. Nat. Commun. 8:2072. doi: 10.1038/s41467-017-02232-6
Venables, W. N., and Ripley, B. D. (2000). Modern Applied Statistics with S, 4th Edn. R-package version 7.3.45. New York, NY: Springer. Available online at: http://CRAN.R-project.org/package=MASS.
Weddle, C. B., Mitchell, C., Bay, S., Sakaluk, S. K., and Hunt, J. (2012). Sex-specific genotype-by-environment interactions for cuticular hydrocarbon expression in decorated crickets, Gryllodes sigillatus: implications for the evolution of signal reliability. J. Evol. Biol. 25, 2112–2125. doi: 10.1111/j.1420-9101.2012.02593.x
Keywords: aggression, cuticular hydrocarbon profile, inbreeding depression, indirect genetic effects, Phaedon cochleariae, chemical signaling traits, terminal investment, wild population
Citation: Müller T, Lachenicht L and Müller C (2018) Inbreeding Alters the Chemical Phenotype and Mating Behavior of a Beetle. Front. Ecol. Evol. 6:177. doi: 10.3389/fevo.2018.00177
Received: 06 June 2018; Accepted: 15 October 2018;
Published: 09 November 2018.
Edited by:
Astrid T. Groot, University of Amsterdam, NetherlandsReviewed by:
Johannes Stökl, Justus Liebig Universität Gießen, GermanyAhmed M. Saveer, North Carolina State University, United States
Tamara Pokorny, University of Regensburg, Germany
Copyright © 2018 Müller, Lachenicht and Müller. This is an open-access article distributed under the terms of the Creative Commons Attribution License (CC BY). The use, distribution or reproduction in other forums is permitted, provided the original author(s) and the copyright owner(s) are credited and that the original publication in this journal is cited, in accordance with accepted academic practice. No use, distribution or reproduction is permitted which does not comply with these terms.
*Correspondence: Thorben Müller, dGhvcmJlbi5tdWVsbGVyQHVuaS1iaWVsZWZlbGQuZGU=