- Department of Biological Sciences, University of Denver, Denver, CO, United States
Climate change models predict increased forest fire occurrence and severity in the near future. Forest fire disturbance affects multiple ecological interactions, but there is little evidence for how naturally-occurring fires affect plant quality and herbivore damage, which is important because plants and herbivorous insects comprise most of the diversity in natural ecosystems and are responsible for a variety of ecosystem services. We surveyed three fires in the Rocky Mountains to investigate the effects of fire severity on wax currant (Ribes cereum), an important source of food and cover for wildlife in Colorado. We measured plant quality and herbivore damage; we found that fire severity had a significant negative effect on both measures. Notably, high severity fires decreased herbivore damage by about 50%. Furthermore, we found that the effect of fire on insect herbivore damage is mostly direct, but that indirect effects mediated through changes in plant quality are also significant. Our results have important implications for the effects of climate-driven increases in fire severity on plant-insect interactions, illustrating strong direct and weaker indirect negative effects of fire severity in a forest ecosystem.
Introduction
Forest fires affect ecological interactions, population and community structure, as well as nutrient cycling, and thereby act as an important disturbance that shapes ecosystems (Veblen et al., 1994; Van Langevelde et al., 2003; Kay et al., 2008). Fires can significantly change an organism's habitat including the availability of food, water, and shelter, and these habitat alterations can drastically affect the animal communities in that area (Koltz et al., 2018). It is important to understand how community interactions are affected by fire because fire activity is predicted to increase in the near future due to global climate change (Flannigan et al., 2000). Climate change models project a 25–50% increase in the area burned in the United States through the year 2100, and an increase in fire severity of 10–50% across much of the US by 2060. As annual temperature increases due to climate change, more fires will occur, which will lead to changes in forest assembly (Flannigan et al., 2000; Dale et al., 2001; Clark et al., 2017). For example, lower montane forests are predicted to experience a lower frequency of fires that are more severe (Rocca et al., 2014). Changes in severity and frequency of forest fires may lead to more appreciable changes in an ecosystem. However, few studies so far investigated how fire severity might affect insect-plant interactions, which are a key driver of forest ecosystem dynamics.
Fire affects herbivores including mammalian grazers (Green et al., 2015) and arthropods (Knight and Holt, 2005), but the impacts of fire on arthropod communities are especially important to understand since arthropod herbivores are a key driver of forest ecosystems. For example, insects play important roles in shaping succession and biodiversity (McCullough et al., 1998), nutrient cycling and ecosystem processes (Kay et al., 2008), and even restructuring pollinator networks (Peralta et al., 2017). Fire may reduce arthropod abundance (in spittlebugs, Martin et al., 1999; in caterpillars, Diniz et al., 2011) or have no effect (Christie and York, 2009). But more often, fire can have positive effects on arthropods, increasing abundance and affecting community structure (in spruce beetle, Bebi et al., 2003; in ground beetles, Gandhi et al., 2008; in butterflies, Scandurra et al., 2014; in grasshoppers, Joubert et al., 2016). These effects depend on the arthropods and plants studied, the type of fire (e.g., wildfire, prescribed, surface, crown, etc. McCullough et al., 1998) as well as the frequency and severity of the fire disturbance (Schowalter, 2012). The complexity of these outcomes is further confounded by species interactions; for example, fire alone may not affect insect communities, but fire in conjunction with grazing mammals can reduce arthropod richness and abundance (Bailey and Whitham, 2002; Jonas and Joern, 2007) and can interact with time since fire to reduce density of a pollinator species (Moranz et al., 2014).
Fire may also indirectly affect herbivores by altering the plants they consume (Wan et al., 2014); thus it is critical that we understand how fire affects plants. First, fire directly changes biomass and size structure of the plant community (Higgins et al., 2007). Fire also shapes plant communities; low-severity fires boost plant species abundance and richness while high-severity fires may have the opposite effect (Pourreza et al., 2014). Fire can also affect plant growth, for example causing trees to produce more leaves (Lopes and Vasconcelos, 2011). Important for herbivores, fire also changes host plant quality (e.g., increasing crude protein content in leaves, Greene et al., 2012). While fire does not always change plant chemistry (Christie and York, 2009), fire can affect soil properties like soil carbon and water holding capacity (Kitzberger et al., 2005), which can affect plant nutrient content (Huang and Boerner, 2007). In some cases, fire causes plants to increase chemical defenses against herbivores (Wan et al., 2014).
While fire directly shapes plant communities, insect herbivores are in turn affected by their host plants. Plant community composition affects the herbivore community (Haddad et al., 2001) and herbivore density after fire is positively correlated with plant cover (e.g., Hahn and Orrock, 2015). Host plant quality is perhaps most important for our understanding of how fire, plants, and herbivores interact. Host plant quality directly affects insect herbivore fitness (Awmack and Leather, 2002); a recent meta-analysis showed that insect herbivores have greater fitness on better quality plants (Vidal and Murphy, 2018). Plant quality may also affect immune defense (Klemola et al., 2007), insect life history traits like whether to enter diapause (Hunter and McNeil, 1997), and even the distribution of insect herbivores (Egan and Ott, 2007). Plant quality can influence herbivores through three common measures of quality: toughness, water content and ratio of carbon to nitrogen (C:N) in leaves. For instance, a generalist herbivore was found to have lower pupal mass when reared on tougher leaves (Barnes and Murphy, 2018), and tougher leaves usually have fewer digestible components, which can inhibit larval feeding (Feeny, 1970). Herbivory seems to be positively related to water content (Faeth, 1985), possibly because low water content can make leaves less succulent and attractive to insect herbivores (Feeny, 1970). Finally, insect herbivores are usually limited by the nitrogen content of their host plants, and thus a lower C:N ratio corresponds with higher insect fitness (Mattson, 1980).
As herbivores are directly affected by plant quality traits, the effect of fire on insect herbivores could be direct or indirectly mediated by changes in plant quality (Figure 1). Fire is known to affect host plant quality (Figure 1 path A, e.g., McCullough et al., 1998; Kay et al., 2007, 2008; Greene et al., 2012; Wan et al., 2014; Hood et al., 2015), and host plant quality affects herbivore fitness, abundance, and diversity (Figure 1 path B, e.g., Vidal and Murphy, 2018, and references therein). However, these indirect effects of fire on herbivores via plant quality are not well understood (but see Christie and York, 2009; Kim and Holt, 2012; Hahn and Orrock, 2015). Additionally, fire may directly affect insects, either positively or negatively, through fire or heat mortality or through changes in habitat structure (Figure 1 path C, e.g., Kim and Holt, 2012). All of the studies that have tried to tease apart the direct and indirect effects of fire on insects have studied prescribed fires, not wildfires. For example, Vogel et al. (2010) found that the indirect effect of fire through changes in vegetation composition had a stronger impact on the abundance of prairie butterflies than the direct impact of fire. Kim and Holt (2012) found that fire affected insect communities indirectly through change in habitat structure instead of directly via changes in host plant quality, and Kay et al. (2007) found a strong indirect effect of fire through canopy openness. These studies used time since fire or fire frequency to explore the variable effects of fire, but prescribed fires are generally less severe than wildfires (e.g., Dooley and Treseder, 2012; Stephan et al., 2015). Given that fire severity is projected to increase with climate change (Flannigan et al., 2000; Dale et al., 2001; IPCC, 2014; Rocca et al., 2014; Clark et al., 2017), it is important to understand if severity influences herbivores directly, or indirectly via plant quality or habitat structure.
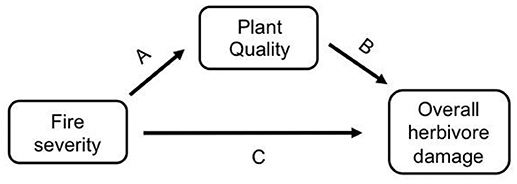
Figure 1. A conceptual model of how herbivore damage may be affected by fire severity either indirectly via changes in host plant quality (i.e., fire severity directly affects host plant quality [path A], which then affects herbivore damage [path B]), or directly via changes to the habitat [path C].
It remains untested if fire severity affects host plant quality and herbivore damage using wildfires, which vary significantly in severity from prescribed fires. We studied three wildfires in the Colorado Rocky Mountains and measured herbivore damage on a dominant understory plant in these forests, wax currant (Ribes cereum), which occurs in unburned, low severity, and high severity burn areas of each fire that we studied. We measured herbivore damage caused by all chewing herbivores as well as by a specific herbivore, the Zephyr Anglewing butterfly (Polygonia gracilis zephyrus; hereafter Polygonia). As Polygonia larvae feed on their host plant, they leave behind a unique stripping pattern that is easily identifiable (Stout, 2008), which allowed us to differentiate their damage from damage by other herbivores. Here we test if fire severity affects herbivore damage directly (Figure 1 path C) or indirectly through change in host plant quality (Figure 1 paths A,B) in order to answer the important question of how fire severity affects insect herbivores.
Methods
Study Location and Site Selection
We surveyed three forest fires within Pike National Forest in the Front Range of Colorado that burned between 1996 and 2002: Buffalo Creek (1996), High Meadows (2000), and Hayman (2002). We surveyed plants for herbivore damage and collected samples to measure plant quality in July 2016, so time since burn varied from 16 to 22 years. Our study sites were located in a subalpine forest ecosystem dominated mainly by Douglas fir and Ponderosa pine trees with mixed vegetation in the low herbaceous layer that included our focal host plant, wax currant (R. cereum). Wax currant plants are usually killed by fires, but seeds in the soil benefit from fire and new plants germinate well, especially after short duration, low severity fires (Marshall, 1995). Wax currants sprout quickly after fires and as they are an alternate host for white pine blister rust (Cronartium ribicola), their recovery from fire is thought to be important to the pathology of this rust and its implications on white pine (Zambino, 2010). Wax currant is a useful indicator species of historical fire regime along the Front Range (Keith et al., 2010).
To assess the impact of fire severity on plant quality and herbivore damage, we identified three regions within each fire that varied in fire severity: unburned, low severity, and high severity. We determined fire severity using data overlays provided by the United States Forest Service (MTBS Data Access: Fire Level Geospatial Data, 2016, April-last revised). Each of the fires was heterogeneous with regard to fire severity (Figure 2) and thus we were able to select sites that were interspersed so that severity types were not clustered together geographically. We visited each site to ensure that the site matched the severity determined by satellite imagery. We characterized high severity sites as completely burned with mostly fallen trees, few standing trees, and little to no canopy cover. We characterized low severity sites as having some standing, scorch-marked trees, and some fully burned fallen trees. We characterized unburned sites as sites with heavy canopy cover and no evidence of burn damage. We selected new sites if, after ground-truthing, the sites did not reflect these characteristics. However, for both the Hayman and High Meadows fires we could find only two unburned sites that had wax currant plants or that did not have active cattle grazing; thus, we had a total of 25 sampled sites (Table 1).
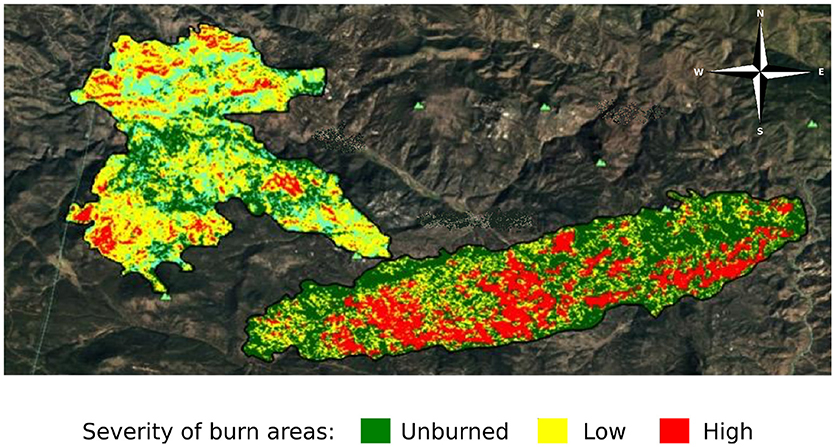
Figure 2. Satellite imagery of the area burned and severity of the High Meadows fire (left) and the Buffalo Creek fire (right) in Colorado; Hayman fire not shown (MTBS Data Access: Fire Level Geospatial Data, 2016, April-last revised). Dark green indicates unburned areas, yellow indicates low severity burn areas, and red indicates high severity burn areas.
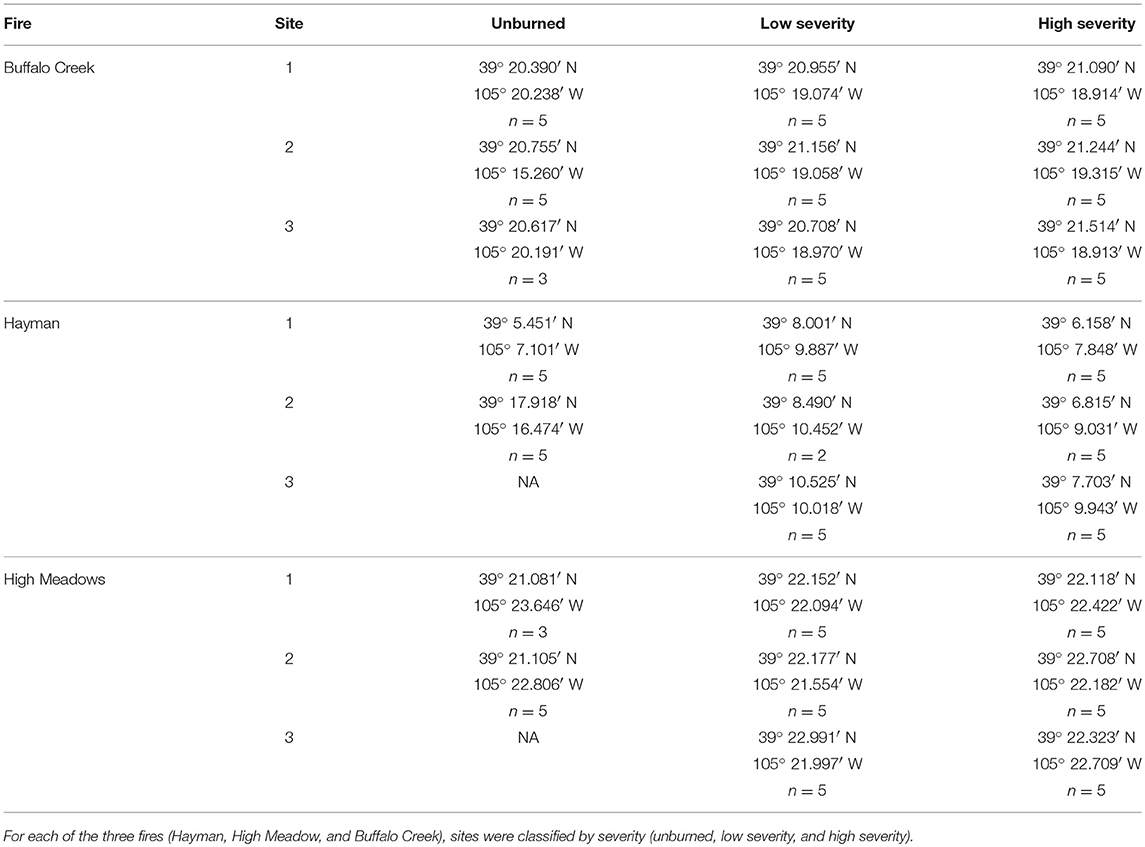
Table 1. GPS coordinates for each of the sampling locations and number of wax currant plants sampled at each site.
Host Plant Density
We surveyed the density of wax currant plants at each site by haphazardly choosing a focal wax currant plant that was located at the approximate center of the site. We then used a transect tape to measure 25 m in each cardinal direction from the central plant, and those points were marked with flags, creating four triangular quadrants (Supplementary Figure 1). To measure density, we counted the number of wax currant plants in each quadrant, calculated a mean number of plants/m2 and then averaged those values across the four quadrants to calculate a mean number of plants/m2 per site.
Host Plant Quality and Herbivore Damage
We sampled wax currant plants to measure plant quality and herbivore damage at each site. We aimed to sample 5 plants per site and used the same 4 transects that we established to measure plant density. The first plant that we sampled at each site was the focal plant at the center of the quadrant. For the other 4 plants, we chose the plant that was the closest to the 10 m mark along each of the 4 transects. Not all sites had 5 wax currant plants and if a site had fewer plants, we sampled all of them at the site (n = 118 plants sampled; Table 1).
To measure herbivore damage, we haphazardly chose two stems from each plant and counted the number of branches coming off of each stem. We then noted the presence or absence of herbivore damage on each branch along the stem. We calculated the percent damage for each plant as the number of branches with herbivore damage divided by the total number of branches per stem. We also noted whether the herbivore damage was due to Polygonia larvae, which leave a unique striping pattern on leaves that is easily identifiable. We also measured the maximum height and width of each plant as well as the number of stems as a measure of plant size.
To assess plant quality, we haphazardly selected two branches from each plant (these branches were not the same as those used to measure herbivore damage), removed the branches from the plant with clippers, and immediately placed them in a cooler filled with ice. We transported samples to the laboratory at the University of Denver and froze them (−20°C) until testing. In the lab, we thawed the plant samples, rinsed them with water to remove dirt, and then let them dry for 15 min. We then randomly selected 10 leaves from each plant by choosing every tenth leaf along both branches. We measured the wet mass of all 10 leaves as a group (to the nearest 0.01 mg) and then haphazardly selected 5 leaves to measure leaf toughness. For each of these 5 leaves, we measured the length of each leaf from the tip of the leaf along the central vein to the base of the stem and measured width across the widest section of the leaf (to the nearest 0.5 mm). We measured toughness using a modified version of the sand-pouring method described by Feeny (1970). We attached a safety pin through the leaf 0.1 cm up from the base of the stem, along the central vein, and then attached the safety pin to a cup by a string. We poured sand into the cup until the safety pin broke all the way through the leaf and weighed the sand. We calculated a mean value per plant using all of the leaf toughness values. After measuring toughness on five leaves, we dried all 10 leaves from each plant for 3 days at 60°C and then weighed them as a group once dry. We calculated percent water by subtracting dry mass from fresh mass and dividing by fresh mass. We performed all water content and leaf toughness mass measurements using a Scout Pro Ohaus Balance (Ohaus Corporation, Pine Brook, NJ USA). For each plant, we ground the dry leaves using a Retsch MM 400 Model mixer mill (Retsch GmbH, Haan, Germany), weighed them using a Mettler-Toledo XP6 microbalance (Mettler-Toledo, Columbus, OH), and rolled them into tin capsules (Elementar Americas). We sent the samples to Cornell University Stable Isotope Laboratory to be analyzed for %N and %C using an elemental analyzer-stable isotope ratio mass spectrometer system (Thermo Delta V Advantage IRMS and Carlo Erba NC2500 EA systems).
Data Analysis
We analyzed our data in two ways, using model selection and mediation analysis. We first used the model selection approach to determine which factors influence plant quality and herbivore damage. In those models, we included all variables that could explain the response variables of interest and using backward selection we could infer which of the variables best explained the changes in plant quality and herbivore damage. We performed 3 independent model selections to analyze: (1) the effect of fire severity and plant characteristics on plant quality (Figure 1, path A), (2) the effect of plant quality on insect herbivore damage (Figure 1, path B), and (3) the effect of plant quality, plant characteristics, and fire severity on herbivore damage (Figure 1, paths B+C). For all our models, site was included as a random factor. To test the effect of fire severity and plant characteristics on plant quality (model selection 1), we had three response variables: toughness, water content, and C:N ratio. For each response variable, we performed a backward model selection starting with all the predictor variables included, which were fire identity, fire severity, the interaction between fire identity and fire severity, plant size, leaf size, and density of Ribes plants at the site. The variables that measure plant size are colinear with each other, as well as the measures of leaf size, which may result in wrong interpretations of statistical significance (Graham, 2003). Therefore, we performed principal component analyses for the variables that measured plant size and leaf size to deal with their collinearity and used the eigenvalues that explained most of the variance in our models (as suggested by Graham, 2003). For leaf size we used the variables leaf width and leaf length to perform the PCA. In our models, we used the first eigenvalue axis that explained 93.4% of the variance in the data. For plant size, we used height, width, and length of each plant measured. In this case, the first axis explained 70.1% and the second explained 18.8% of the variance, and thus we used both in our models. All PCAs were performed using JMP 11®. Plant density was not normally distributed, but the log of density was, which we used in our analysis.
To test if plant quality had an effect on herbivore damage (model selection 2), we had 2 response variables that we tested separately: overall herbivore damage and herbivore damage by Polygonia. Polygonia herbivore damage did not follow a normal distribution, so we used the log transformed data of Polygonia damage + 1. The fixed effects were toughness, C:N ratio, and percent water content. To test the effect of plant quality, plant characteristics, and fire severity on herbivore damage (model selection 3), we had two response variables: overall herbivore damage and herbivore damage by Polygonia. The fixed effects for the starting model were toughness, percent water, C:N ratio, density of plants (log transformed), size of plants (PCA1plantsize and PCA2plantsize), size of leaves (PCA1leafsize), fire severity, fire identity, and the interaction between fire severity and identity. For the backward model selection, we removed step by step the factor that least explained the data, and we compared the reduced model with the previous model using F-statistics. If the reduced model was not significantly different from the extended model, we removed the factor from the analysis and kept the reduced model. The same procedure of backward model selection was performed for groups 1, 2, and 3 of response variables. We further tested if fire severity had an effect on plant density, as a measure of possible change on habitat structure or resource availability. We used log density as the response variable, fire severity as the fixed effect, and fire identity as the random effect.
For the second analysis method, we used mediation analysis with only the variables that were shown to affect the response variables with our model selection. Using this approach, we can directly investigate which factor influenced herbivore damage more: if fire severity had a direct effect on herbivore damage or if the effect of fire severity on herbivore damage was mediated by the effect of fire severity on plant quality (Figure 1). To test that, we used a mediation analysis to parse out the relative contribution of plant quality and fire severity on herbivore damage. For our mediation analysis, we used only the variables that were significant in the model selections for groups 1, 2, and 3 explained above, thus beside giving evidence of the factors that explain herbivore damage and plant quality, the model selection approach provides support for choosing the variables to include in the mediation analysis. For instance, in the mediation analysis for both overall herbivore damage and herbivore damage by Polygonia, we included the effect of fire severity, fire identity, leaf size, and plant size to measure the effect of fire severity on C:N ratio (see results below). However, we were only interested in the effect of fire severity, so we report only the coefficient (or estimate) for the effect of severity on C:N ratio. Then we performed other models to test the effect of C:N ratio alone, fire severity alone, and C:N together with fire severity on herbivore damage. Since our results show that unburned and low severity fires did not differ from each other, and were both different from high severity fire, we combined unburned and low severity into one category. Therefore, our coefficients for the mediation analysis show the difference from low and unburned to high severity fires. All analyses were performed in R environment 3.4.3 (R Development Core Team, 2011) using the package lme4 with lmer function (Bates et al., 2015). To perform pairwise comparisons between the different fire severities in each final model from our model selection done with groups A and C, we used the package multcomp with the glht functions (Hothorn et al., 2008). For the model comparisons, we used package pbkrtest with KRmodcomp function (Halekoh and Hojsgaard, 2014), which gives the p-value for the model comparisons with a Kenward-Roger adjustment. We deposited our data in the Dryad Repository: doi: 10.5061/dryad.t36p0cd (Murphy et al., 2018).
Results
Effect of Fire on Plant Quality
We found that increased fire severity decreased plant quality (Table 2). Leaves were tougher [Figure 3A, F(2, 23.81) = 6.15, P = 0.002], had greater C:N ratio [Figure 3B, F(2, 27) = 10.25, P < 0.001], and lower water content [Figure 3C, F(2, 21.78) = 3.44, P = 0.05] in places with high severity fire compared to areas with low severity fire or unburned areas. Toughness and C:N ratio of Ribes leaves were also influenced by fire identity [toughness: F(2, 20.69) = 3.53, P = 0.023; C:N: F(2, 21.17) = 7.26, P = 0.004] and leaf size [toughness: F(1, 106.07) = 6.12, P = 0.015; C:N: F(1, 107.59) = 4.5, P = 0.036]. Furthermore, C:N ratio was influenced by plant size [PC1plantsize only, F(1, 109.25) = 7.09, P = 0.009]. We found no effect of fire severity on Ribes plant density [F(2, 20.5) = 1.78, P = 0.2].
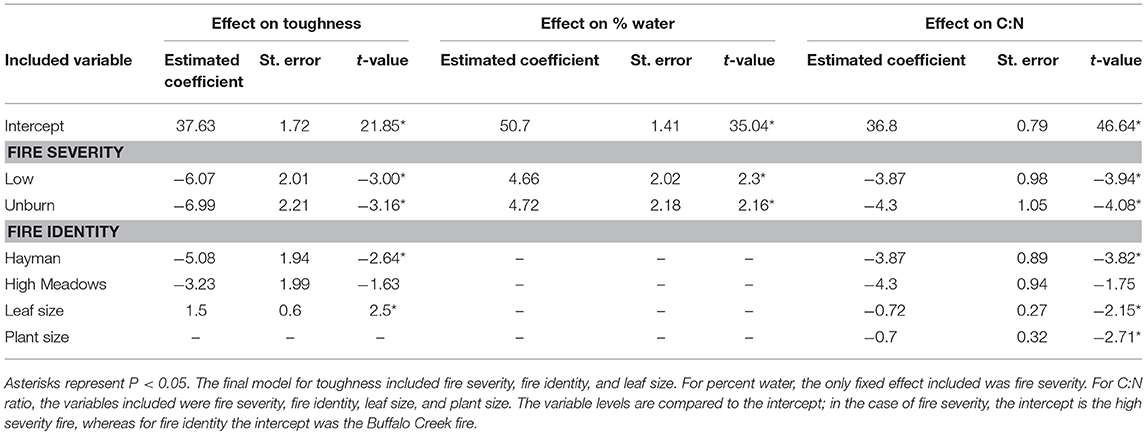
Table 2. Model summary for the effect of each explanatory variable on three measures of host plant quality: leaf toughness, water content, and C:N ratio.
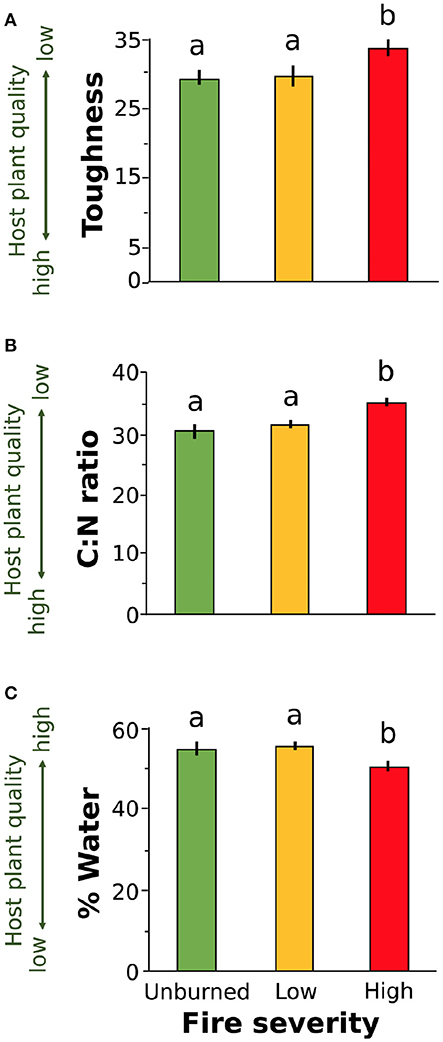
Figure 3. Plant quality measures for leaves collected from wax currant plants growing in unburned, low severity, and high severity fire sites: (A) toughness, (B) carbon to nitrogen ratio, and (C) percent water. Increased leaf toughness and C:N ratio reduce host plant quality for herbivores (A,B) while increased percent water increases host plant quality (C). Means are given ±SE and letters represent pairwise comparisons within host plant quality measure (P < 0.05).
Effect of Plant Quality on Herbivore Damage
Overall herbivore damage and herbivore damage by Polygonia were explained only by the plant quality measure of C:N ratio [overall herbivore damage: F(1, 101.5) = 4.16, P = 0.044; Polygonia: F(1, 108.8) = 6.83, P = 0.01]. Overall herbivore damage and herbivore damage by Polygonia were greater on plants with low C:N ratio [overall herbivore damage: estimate = −0.64 ± 0.31, t = −2.05, P = 0.043; Polygonia: estimate = −0.05 ± 0.02, t = −2.67, P = 0.009].
Effect of Plant Quality and Fire on Herbivore Damage
Overall herbivore damage and damage by Polygonia were both 50% lower in sites with high severity fire than in unburned sites, and were similar between unburned and low severity sites (Figure 4). When we performed our model selection with both plant quality and fire effects on herbivore damage, we found that overall herbivore damage and Polygonia damage were affected only by fire severity [Overall: F(2, 22) = 18.61, P < 0.0001, Polygonia: F(2, 21.82) = 7.21, P = 0.004; Table 3].
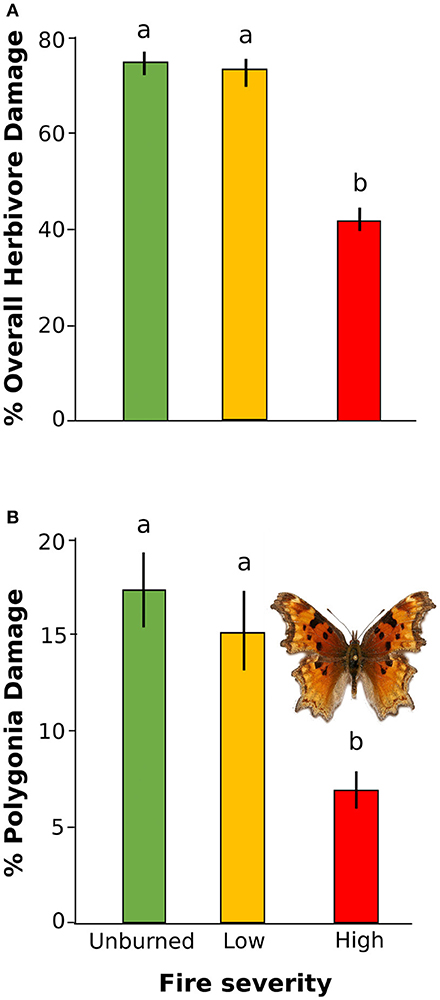
Figure 4. Percent herbivore damage on wax currant plants in unburned, low severity, and high severity fire sites for (A) overall herbivore damage and (B) herbivore damage by Polygonia gracilis zephyrus. Means are given ±SE and letters represent pairwise comparisons within type of herbivore damage (p < 0.05).
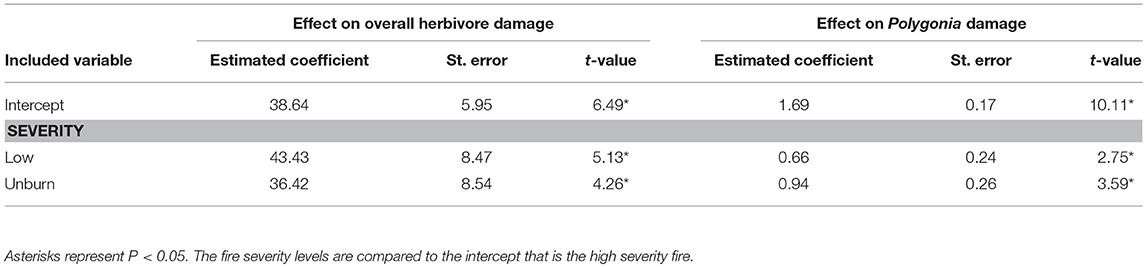
Table 3. Model summary for the effect of fire severity on overall herbivore damage and damage by the butterfly Polygonia gracilis zephyrus to wax currant plants.
From our mediation analysis, both total herbivore damage and damage by Polygonia were mainly affected directly by fire severity (Figure 5). The effect of fire mediated through host plant quality (C:N ratio) was not nearly as strong as the direct effect of fire severity on herbivore damage. When we included C:N in the model with fire severity, the coefficient of fire severity only changed slightly compared with fire severity alone (for overall herbivore damage the coefficient went from 29.08 without C:N to 31.04 with C:N, while for Polygonia herbivore damage the coefficient went from 8.03 to 9.09).
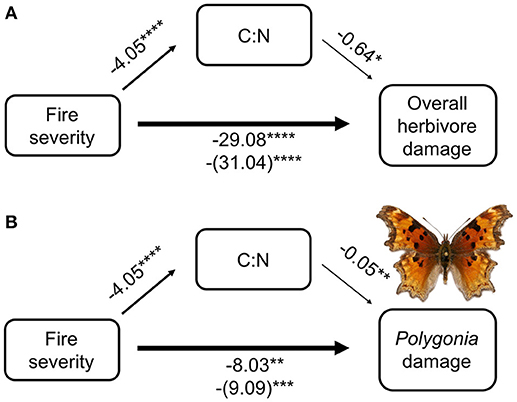
Figure 5. Mediation analysis of fire severity, C:N ratio, and herbivore damage on wax currant plants for (A) overall herbivore damage and (B) herbivore damage by Polygonia gracilis zephyrus. Numbers are model coefficients (coefficients in parentheses are the model that considers both C:N and fire severity) and asterisks indicate significant effects at *p < 0.05, **p < 0.01, ***p < 0.001, ****p < 0.0001.
Discussion
Fire severity directly impacted host plant quality as well as herbivore damage, and the direct effect of fire severity on herbivore damage was stronger than the indirect effect via plant quality. Although plant quality differed across fires (e.g., Buffalo Creek, Hayman, or High Meadows), the effect of fire severity on plant quality was stronger than fire identity, and fire identity did not influence herbivore damage. We found an inverse relationship between fire severity and host plant quality; increasing fire severity decreased plant quality through an increase in C:N ratio and toughness, and a decrease in water content. However, of these quality measures, the only one that predicted herbivore damage by herbivores in general and specifically Polygonia was the C:N ratio. Kim and Holt (2012) studied time since fire rather than fire severity in prescribed burns in the Florida scrub, but similar to our results found that changes in habitat structure from fire had a stronger effect on herbivores than changes in plant quality. Although we did not find an effect of fire severity on plant density, we found that insect herbivores were strongly affected by fire severity through mechanisms other than change in plant quality. Thus, our results demonstrate that severity of forest wildfire influences herbivores through strong direct effects but also indirectly through changes in plant quality.
We focused on severity for this study because models predict that fire severity will be one of the biggest responses to climate change (IPCC, 2014), but previous work tends to focus on time since fire, which is a measure of recovery time of the community (e.g., Kay et al., 2007; Vogel et al., 2010; Kim and Holt, 2012). Notably, the effect of fire severity was greater than that of fire identity suggesting that while our fires happened at three different time points, time since fire does not account for the effects of fire severity; our three fires occurred between 16 and 22 years ago, which may not be a long enough difference to detect a time-since-fire effect. During post-fire succession, wax currant densities can depend on land management (Bock et al., 1978), but plants usually thrive and can remain at high densities for decades post-fire (Coop and Schoettle, 2009). Fire severity has different effects on herbivores than time since fire, because increasing severity significantly affects habitat structure (e.g., increase in canopy openness), soil moisture, and nutrients, as well as which species can survive (New, 2014; Koltz et al., 2018; Pressler et al. in review). These impacts could be responsible for the strong direct effect of severity on herbivore damage that we found in our study, which was stronger than the indirect negative effect mediated through plant quality. Fire severity can influence the survival of insects both above and belowground; for example, belowground insects suffer high mortality in high fire severity that greatly increase the temperature of the soil (New, 2014; Pressler et al. in review). The negative effect of a high severity fire can also affect higher trophic levels that then influence the insect herbivore community (Koltz et al., 2018). Some natural enemies are less mobile (e.g., spiders), or pupate or nest in the soil (e.g., some wasps and ants), and thus may suffer high mortality in severe fire events. The potential negative effect of fire on natural enemies would lead to positive effects on herbivores due to enemy release (Pausas and Parr, 2018). These complex but critical interactions would be missed if we only considered time since fire and not fire severity. Moving forward, it is necessary to consider the different measures of fire disturbance (severity, frequency, time since fire, and time of year of fire) and how they interact with each other when exploring the effect of fire on herbivores.
While less important than the direct effect of fire severity, plant quality also influenced herbivore damage, especially through changes in C:N ratio. The effects of fire on plants (e.g., Lopes and Vasconcelos, 2011; Wan et al., 2014) and the effect of plant quality on herbivore damage (e.g., Greene et al., 2012; Hahn and Orrock, 2015) have been previously documented, but the complex interaction among these processes is critical in order to understand how communities will respond to increase in fire severity. These relationships are even more complex since fire can also influence how herbivores affect plant growth (Conway and Johnstone, 2017), plant chemistry, and decomposition (Kay et al., 2008). While we connected fire severity, plant quality, and herbivore damage, other work has suggested that the soil community should also be considered. For example, fire reduces microbial abundance (Dooley and Treseder, 2012) as well as soil fungal abundance (Holden et al., 2013). Additionally, fire severity affects the reduction in microbial biomass and respiration so that more severe fires have larger negative impacts on the soil community (Holden et al., 2016). This reduction in both fungal and microbial biomass reduces decomposition rates in post-fire soils (Holden et al., 2015), directly affecting the resources available to plants, and thus plant quality, as well as altering the carbon cycle of the ecosystem.
While our work sheds light on the complex interactions among fire, plants, and herbivores, it also inspires questions for future exploration. First, we measured damage to plants holistically as a community level estimate of total damage, but individual specialist herbivore species likely have unique responses to their host plants and to fire. We did, however, examine damage from a common herbivore in our system, Polygonia larvae, and found identical patterns comparing this species' herbivore damage to our community level measure. It is currently unknown if different insect herbivore species will respond similarly to changes in fire severity, though we might expect life history traits like ability to disperse from or avoid fire (e.g., enter diapause), as well as evolutionary history with fire to shape species-specific patterns (Koltz et al., 2018). This is an important question for future work. Additionally, because only one dominant understory shrub was present in all of our sites, we focused on wax currant. Future work should compare our results to herbivores that feed on other plant species, such as grasses or trees. Finally, we might expect some processes, like recolonization rates, to be driven by an interaction between fire severity and burn size, which we did not measure, and future work should explore this interaction.
Global climate change is altering fire regimes worldwide with projected large changes to fire severity (Flannigan et al., 2000; Dale et al., 2001; IPCC, 2014; Rocca et al., 2014; Clark et al., 2017), but how these changes will affect insect communities and species interactions is largely unknown (Koltz et al., 2018). Ours is the first study to investigate how fire severity affects plant-herbivore interactions, as measured by plant quality and herbivore damage, in naturally occurring forest wildfires. Our results demonstrate that severity can have profound impacts on interactions between herbivores and their host plants with severity significantly affecting host plant quality, but with an even larger direct effect on insect herbivores, likely through changes in habitat structure. Thus, our results suggest that increasing severity of wildfires will significantly influence how herbivores interact with their host plants and may impact long-term population sizes and community structure.
Data Accessibility Statement
We deposited our data in the Dryad Repository: doi: 10.5061/dryad.t36p0cd (Murphy et al., 2018).
Author Contributions
LC and SM conceived and designed the experiments; LC, TS, DR, and SM conducted the fieldwork; TS, DR, CH, and SM conducted the labwork; EB, MV, CH, and SM conceptualized the framework for the research; CH and TS managed the data; MV analyzed the data; SM, EB, MV, CH, and TS wrote the paper.
Conflict of Interest Statement
The authors declare that the research was conducted in the absence of any commercial or financial relationships that could be construed as a potential conflict of interest.
Acknowledgments
We thank the University of Denver Undergraduate Research Center for funding this research as well as a NSF GRFP that funded LC. We are grateful to Eva Horna Lowell for help with the fieldwork, Bailey Rojewski and April Vollmer for help with the labwork, and Cathy Durso for help with the statistical analysis. We thank the University of Denver Ecology and Evolution (DUEEBs) group for comments that greatly improved an earlier draft of this manuscript.
Supplementary Material
The Supplementary Material for this article can be found online at: https://www.frontiersin.org/articles/10.3389/fevo.2018.00135/full#supplementary-material
References
Awmack, C. S., and Leather, S. R. (2002). Host plant quality and fecundity in herbivorous insects. Annu. Rev. Entomol. 47, 817–844. doi: 10.1146/annurev.ento.47.091201.145300
Bailey, J. K., and Whitham, T. G. (2002). Interactions among fire, aspen, and elk affect insect diversity: reversal of a community response. Ecology 83, 1701–1712. doi: 10.1890/0012-9658(2002)083[1701:IAFAAE]2.0.CO;2
Barnes, E. E., and Murphy, S. M. (2018). Time-lagged intraspecific competition in temporally separated cohorts of a generalist insect. Oecologia 186, 711–718. doi: 10.1007/s00442-018-4067-9
Bates, D., Machler, M., Bolker, B., and Walker, S. (2015). Fitting linear mixed-effects models using lme4. J. Stat. Softw. 67, 1–48. doi: 10.18637/jss.v067.i01
Bebi, P., Kulakowski, D., and Veblen, T. T. (2003). Interactions between fire and spruce beetles in a subalpine Rocky Mountain forest landscape. Ecology 84, 362–371. doi: 10.1890/0012-9658(2003)084[0362:IBFASB]2.0.CO;2
Bock, J. H., Raphael, M., and Bock, C. E. (1978). A comparison of planting and natural succession after a forest fire in the northern Sierra Nevada. J. Appl. Ecol. 15, 597–602. doi: 10.2307/2402613
Christie, F. J., and York, A. (2009). No detectable impacts of frequent burning on foliar C and N or insect herbivory in an Australian eucalypt forest. Appl. Veg. Sci. 12, 376–384. doi: 10.1111/j.1654-109X.2009.01033.x
Clark, J. A., Loehman, R. A., and Keane, R. E. (2017). Climate changes and wildfire alter vegetation of Yellowstone National Park, but forest cover persists. Ecosphere 8:e01636. doi: 10.1002/ecs2.1636
Conway, A. J., and Johnstone, J. F. (2017). Moose alter the rate but not the trajectory of forest canopy succession after low and high severity fire in Alaska. For. Ecol. Manage. 391, 154–163. doi: 10.1016/j.foreco.2017.02.018
Coop, J. D., and Schoettle, A. W. (2009). Regeneration of Rocky Mountain bristlecone pine (Pinus aristata) and limber pine (Pinus flexilis) three decades after stand-replacing fires. For. Ecol. Manage. 257, 893–903. doi: 10.1016/j.foreco.2008.10.034
Dale, V. H., Joyce, L. A., McNulty, S., Neilson, R. P., Ayres, M. P., Flannigan, M. D., et al. (2001). Climate change and forest disturbances. Bioscience 51, 723–734. doi: 10.1641/0006-3568(2001)051[0723:CCAFD]2.0.CO;2
Diniz, I., Higgins, B., and Morais, H. (2011). How do frequent fires in the Cerrado alter the lepidopteran community? Biodivers. Conserv. 20, 1415–1426. doi: 10.1007/s10531-011-0034-z
Dooley, S. R., and Treseder, K. K. (2012). The effect of fire on microbial biomass: a meta-analysis of field studies. Biogeochemistry 109, 49–61. doi: 10.1007/s10533-011-9633-8
Egan, S. P., and Ott, J. R. (2007). Host plant quality and local adaptation determine the distribution of a gall-forming herbivore. Ecology 88, 2868–2879. doi: 10.1890/06-1303.1
Faeth, S. H. (1985). Quantitative defense theory and patterns of feeding by oak insects. Oecologia 68, 34–40. doi: 10.1007/BF00379470
Feeny, P. (1970). Seasonal changes in oak leaf tannins and nutrients as a cause of spring feeding by winter moth caterpillars. Ecology 51, 565–581. doi: 10.2307/1934037
Flannigan, M. D., Stocks, B. J., and Wotton, B. M. (2000). Climate change and forest fires. Sci. Total Environ. 262, 221–229. doi: 10.1016/S0048-9697(00)00524-6
Gandhi, K. J., Gilmore, D. W., Katovich, S. A., Mattson, W. J., Zasada, J. C., and Seybold, S. J. (2008). Catastrophic windstorm and fuel-reduction treatments alter ground beetle (Coleoptera: Carabidae) assemblages in a North American sub-boreal forest. For. Ecol. Manage. 256, 1104–1123. doi: 10.1016/j.foreco.2008.06.011
Graham, M. H. (2003). Confronting multicollinearity in ecological multiple regression. Ecology 84, 2809–2815. doi: 10.1890/02-3114
Green, D. S., Roloff, G. J., Heath, B. R., and Holekamp, K. E. (2015). Temporal dynamics of the reponses by African mammals to prescribed fire. J. Wildl. Manage. 79, 235–242. doi: 10.1002/jwmg.827
Greene, L., Hebblewhite, M., and Stephenson, T. (2012). Short-term vegetation response to wildfire in the eastern Sierra Nevada: implications for recovering an endangered ungulate. J. Arid Environ. 87, 118–128. doi: 10.1016/j.jaridenv.2012.06.001
Haddad, N. M., Tilman, D., Haarstad, J., Ritchie, M., and Knops, J. M. (2001). Contrasting effects of plant richness and composition on insect communities: a field experiment. Am. Nat. 158, 17–35. doi: 10.1086/320866
Hahn, P. G., and Orrock, J. L. (2015). Land-use legacies and present fire regimes interact to mediate herbivory by altering the neighboring plant community. Oikos 124, 497–506. doi: 10.1111/oik.01445
Halekoh, U., and Hojsgaard, S. (2014). A Kenward-Roger approximation and parametric bootstrap methods for tests in linear mixed models the R package pbkrtest. J. Stat. Softw. 59, 1–32. doi: 10.18637/jss.v059.i09
Higgins, S. I., Bond, W. J., February, E. C., Bronn, A., Euston-Brown, D. I., Enslin, B., et al. (2007). Effects of four decades of fire manipulation on woody vegetation structure in savanna. Ecology 88, 1119–1125. doi: 10.1890/06-1664
Holden, S. R., Berhe, A. A., and Treseder, K. K. (2015). Decreases in soil moisture and organic matter quality suppress microbial decomposition following a boreal forest fire. Soil Biol. Biochem. 87, 1–9. doi: 10.1016/j.soilbio.2015.04.005
Holden, S. R., Gutierrez, A., and Treseder, K. K. (2013). Changes in soil fungal communities, extracellular enzyme activities, and litter decomposition across a fire chronosequence in Alaskan boreal forests. Ecosystems 16, 34–46. doi: 10.1007/s10021-012-9594-3
Holden, S. R., Rogers, B. M., Treseder, K. K., and Randerson, J. T. (2016). Fire severity influences the resopnse of soil microbes to a boreal forest fire. Environ. Res. Lett. 11:035004. doi: 10.1088/1748-9326/11/3/035004
Hood, S., Sala, A., Heyerdahl, E. K., and Boutin, M. (2015). Low-severity fire increases tree defense against bark beetle attacks. Ecology 96, 1846–1855. doi: 10.1890/14-0487.1
Hothorn, T., Bretz, F., and Westfall, P. (2008). Simultaneous inference in general parametric models. Biom. J. 50, 346–363. doi: 10.1002/bimj.200810425
Huang, J., and Boerner, R. E. (2007). Effects of fire alone or combined with thinning on tissue nutrient concentrations and nutrient resorption in Desmodium nudiflorum. Oecologia 153, 233–243. doi: 10.1007/s00442-007-0733-z
Hunter, M. D., and McNeil, J. N. (1997). Host-plant quality influences diapause and voltinism in a polyphagous insect herbivore. Ecology 78, 977–986. doi: 10.1890/0012-9658(1997)078[0977:HPQIDA]2.0.CO;2
IPCC (2014). “Climate change 2014: synthesis report,” in Contribution of Working Groups I, II and III to the Fifth Assessment Report of the Intergovernmental Panel on Climate Change, eds R. K. Pachauri, L. A. Meyer, and Core Writing Team (Geneva: IPCC).
Jonas, J. L., and Joern, A. (2007). Grasshopper (Orthoptera: Acrididae) communities respond to fire, bison grazing and weather in North American tallgrass prairie: a long-term study. Oecologia 153, 699–711. doi: 10.1007/s00442-007-0761-8
Joubert, L., Pryke, J. S., and Samways, M. J. (2016). Positive effects of burning and cattle grazing on grasshopper diversity. Insect Conserv. DiverS. 9, 290–301. doi: 10.1111/icad.12166
Kay, A. D., Mankowski, J., and Hobbie, S. E. (2008). Long-term burning interacts with herbivory to slow decomposition. Ecology 89, 1188–1194. doi: 10.1890/07-1622.1
Kay, A. D., Schade, J. D., Ogdahl, M., Wesserle, E. O., and Hobbie, S. E. (2007). Fire effects on insect herbivores in an oak savanna: the role of light and nutrients. Ecol. Entomol. 32, 754–761. doi: 10.1111/j.1365-2311.2007.00925.x
Keith, R. P., Veblen, T. T., Schoennagel, T. L., and Sherriff, R. L. (2010). Understory vegetation indicates historic fire regimes in ponderosa pine-dominatedecosystems in the Colorado Front Range. J. Veg. Sci. 21, 488–499. doi: 10.1111/j.1654-1103.2009.01156.x
Kim, T. N., and Holt, R. D. (2012). The direct and indirect effects of fire on the assembly of insect herbivore communities: examples from the Florida scrub habitat. Oecologia 168, 997–1012. doi: 10.1007/s00442-011-2130-x
Kitzberger, T., Raffaele, E., Heinemann, K., and Mazzarino, M. J. (2005). Effects of fire severity in a north Patagonian subalpine forest. J. Veg. Sci. 16, 5–12. doi: 10.1111/j.1654-1103.2005.tb02333.x
Klemola, N., Klemola, T., Rantala, M. J., and Ruuhola, T. (2007). Natural host plant quality afects immune defence of an insect herbivore. Entomol. Exp. Appl. 123, 167–176. doi: 10.1111/j.1570-7458.2007.00533.x
Knight, T. M., and Holt, R. D. (2005). Fire generates spatial gradients in herbivory: an example from a Florida sandhill ecosystem. Ecology 86, 587–593. doi: 10.1890/04-1069
Koltz, A. M., Burkle, L. A., Dell, J. E., Pressler, Y., Richards, L. A., Vidal, M. C., et al. (2018). Global change and the importance of fire on the ecology and evolution of insects. Curr. Opin. Insect Sci. 29, 110–116. doi: 10.1016/j.cois.2018.07.015
Lopes, C. T., and Vasconcelos, H. L. (2011). Fire increases insect herbivory in a neotropical savanna. Biotropica 43, 612–618. doi: 10.1111/j.1744-7429.2011.00757.x
Marshall, K. A. (1995). “Ribes cereum,” in Fire Effects Information System, [Online]. U.S. Department of Agriculture; Forest Service; Rocky Mountain Research Station. Available online at: https://www.fs.fed.us/database/feis/plants/shrub/ribvel/all.html
Martin, -R., M., Cox, J. R., Ibarra, -F., F., Alston, D. G., Banner, R. E., and Malecheck, J. C. (1999). Spittlebug and buffelgrass responses to summer fires in Mexico. J. Range Manage. 52, 621–625. doi: 10.2307/4003632
Mattson, W. J. (1980). Herbivory in relation to plant nitrogen content. Annu. Rev. Ecol. Syst. 11, 119–161. doi: 10.1146/annurev.es.11.110180.001003
McCullough, D. G., Werner, R. A., and Neumann, D. (1998). Fire and insects in northern and boreal forest ecosystems of North America. Annu. Rev. Entomol. 43, 107–127. doi: 10.1146/annurev.ento.43.1.107
Moranz, R. A., Fuhlendorf, S. D., and Engle, D. M. (2014). Making sense of a prairie butterfly paradox: the effects of grazing, time since fire, and sampling period on regal fritillary abundance. Biol. Conserv. 173, 32–41. doi: 10.1016/j.biocon,.2014.03.003
MTBS Data Access: Fire Level Geospatial Data (2016). MTBS Project [Online]. USDA Forest Service/U.S. Geological Survey. Available online at: http://mtbs.gov/data/individualfiredata.html
Murphy, S. M., Vidal, M. C., Smith, T. P., Hallagan, C. J., Broder, E. D., Rowland, D., et al. (2018). Data from: forest fire severity affects host plant quality and insect herbivore damage. Dryad Digit. Repository. doi: 10.5061/dryad.t36p0cd. [Epub ahead of print].
Pausas, J. G., and Parr, C. L. (2018). Towards an understanding of the evolutionary role of fire in animals. Evol. Ecol. 32, 113–125. doi: 10.1007/s10682-018-9927-6
Peralta, G., Stevani, E. L., Chacoff, N. P., Dorado, J., and Vázquez, D. P. (2017). Fire influences the structure of plant-bee networks. J. Anim. Ecol. 86, 1372–1379. doi: 10.1111/1365-2656.12731
Pourreza, M., Hosseini, S. M., Sinegani, A. A. S., Matinizadeh, M., and Alavai, S. J. (2014). Herbaceous species diversity in relation to fire severity in Zagros oak forests, Iran. J. Forestry Res. 25, 113–120. doi: 10.1007/s11676-014-0436-3
R Development Core Team (2011). R: A Language and Environment for Statistical Computing. R Foundation for Statistical Computing. Available online at: http://www.r-project.org.
Rocca, M. E., Brown, P. M., MacDonald, L. H., and Carrico, C. M. (2014). Climate change impacts on fire regimes and key ecosystem services in Rocky Mountain forests. For. Ecol. Manage. 327, 290–305. doi: 10.1016/j.foreco.2014.04.005
Scandurra, A., Magliozzi, L., Aria, M., and D'Aniello, B. (2014). Short-term effects of fire on Papilionoidea (Lepidoptera) communities: a pilot study in Mediterranean maquis shrubland. Ital. J. Zool. 81, 599–609. doi: 10.1080/11250003.2014.953218
Schowalter, T. (2012). Insect responses to major landscape-level disturbance. Annu. Rev. Entomol. 57, 1–20. doi: 10.1146/annurev-ento-120710-100610
Stephan, K., Kavanagh, K. L., and Koyama, A. (2015). Comparing the influence of wildfire and prescribed burns on watershet nitrogen biogeochemistry using 15N natural abundance in terrestrial and aquatic ecosystem components. PLoS ONE 10:e0119560. doi: 10.1371/journal.pone.0119560
Van Langevelde, F., Van De Vijver, C. A., Kumar, L., Van De Koppel, J., De Ridder, N., Van Andel, J., et al. (2003). Effects of fire and herbivory on the stability of savanna ecosystems. Ecology 84, 337–350. doi: 10.1890/0012-9658(2003)084[0337:EOFAHO]2.0.CO;2
Veblen, T. T., Hadley, K. S., Nel, E. M., Kitzberger, T., Reid, M., and Villalba, R. (1994). Disturbance regime and disturbance interactions in a Rocky Mountain subalpine forest. J. Ecol. 82, 125–135. doi: 10.2307/2261392
Vidal, M. C., and Murphy, S. M. (2018). Bottom-up vs. top-down effects on terrestrial insect herbivores: a meta-analysis. Ecol. Lett. 21, 138–150. doi: 10.1111/ele.12874
Vogel, J. A., Koford, R. R., and Debinski, D. M. (2010). Direct and indirect responses of tallgrass prairie butterflies to prescribed burning. J. Insect Conserv. 14, 663–677. doi: 10.1007/s10841-010-9295-1
Wan, H. Y., Rhodes, A. C., and St Clair, S. B. (2014). Fire severity alters plant regeneration patterns and defense against herbivores in mixed aspen forests. Oikos 123, 1479–1488. doi: 10.1111/oik.01521
Keywords: fire severity, herbivore damage, Lepidoptera, plant quality, Polygonia gracilis zephyrus, Rocky Mountains, wax currant, Ribes cereum
Citation: Murphy SM, Vidal MC, Smith TP, Hallagan CJ, Broder ED, Rowland D and Cepero LC (2018) Forest Fire Severity Affects Host Plant Quality and Insect Herbivore Damage. Front. Ecol. Evol. 6:135. doi: 10.3389/fevo.2018.00135
Received: 27 June 2018; Accepted: 22 August 2018;
Published: 12 September 2018.
Edited by:
Pavel Kindlmann, Charles University, CzechiaReviewed by:
Karolína Bílá, Global Change Research Institute, Czech Academy of Sciences, CzechiaZdenka Krenova, Global Change Research Institute, Czech Academy of Sciences, Czechia
Copyright © 2018 Murphy, Vidal, Smith, Hallagan, Broder, Rowland and Cepero. This is an open-access article distributed under the terms of the Creative Commons Attribution License (CC BY). The use, distribution or reproduction in other forums is permitted, provided the original author(s) and the copyright owner(s) are credited and that the original publication in this journal is cited, in accordance with accepted academic practice. No use, distribution or reproduction is permitted which does not comply with these terms.
*Correspondence: Shannon M. Murphy, shannon.m.murphy@du.edu
†Present Address: Mayra C. Vidal, Department of Biology, Syracuse University, Syracuse, NY, United States
E. Dale Broder, Department of Biology, St. Ambrose University, Davenport, IA, United States