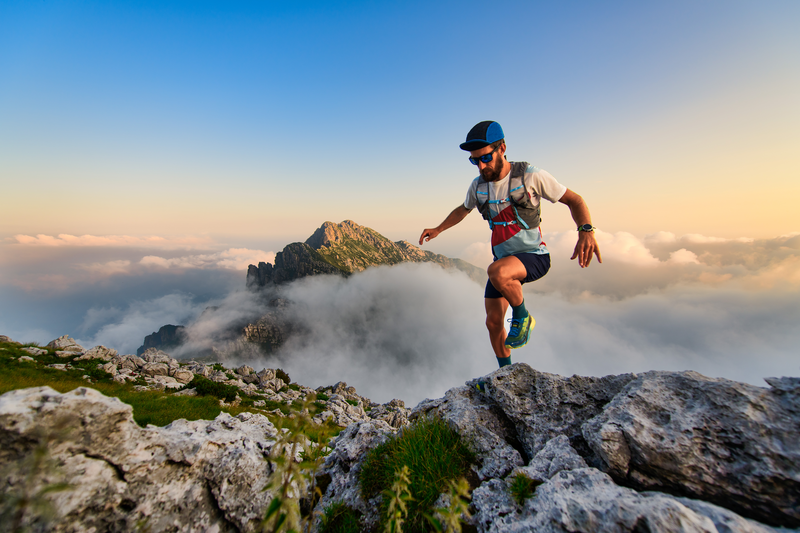
95% of researchers rate our articles as excellent or good
Learn more about the work of our research integrity team to safeguard the quality of each article we publish.
Find out more
ORIGINAL RESEARCH article
Front. Ecol. Evol. , 07 September 2018
Sec. Agroecology
Volume 6 - 2018 | https://doi.org/10.3389/fevo.2018.00133
This article is part of the Research Topic Ecosystem Services and Disservices Provided by Plant-Feeding Predatory Arthropods View all 12 articles
Many plant-dwelling mites including phytophagous spider mites and predacious phytoseiid mites suffer lethal deleterious effects from solar ultraviolet-B (UVB; 280–315 nm wavelength) radiation. Phytoseiid species also often feed on pollen as an alternative food source. As pollen is frequently exposed to solar radiation, it is likely to contain compounds that protect germ cells from UVB radiation and radiant heat. If phytoseiid mites can obtain these protective compounds, pollen feeding may play a role in their adaptation to UVB. In this study, we compared the potential protective effects of tea pollen, peach pollen, and Tetranychus urticae mites as food items in Neoseiulus californicus. Egg hatchability and adult female survival after UVB irradiation were higher in pollen-fed than mite-fed N. californicus. The major protective effects of peach pollen and tea pollen were UVB shielding effects and antioxidant capacity, respectively, and these were derived from distinctive antioxidant components. The major antioxidant in peach pollen was tri-p-coumaroylspermidine, although its antioxidant capacity was relatively low; instead, it effectively absorbed UVB radiation. By contrast, the major antioxidants of tea pollen were catechin and epicatechin 3-gallate, which had high antioxidant capacities. Our results indicate that the protective antioxidant components in pollen improved UVB resistance in N. californicus, contributing to their adaptation to solar radiation.
Phytophagous spider mites and predacious phytoseiid mites suffer deleterious effects from solar ultraviolet-B (UVB, 280–315 nm wavelength) radiation (Ohtsuka and Osakabe, 2009; Onzo et al., 2010; Sakai and Osakabe, 2010; Sakai et al., 2012; Tachi and Osakabe, 2012, 2014; Koveos et al., 2017). UVB radiation generates DNA lesions such as cyclobutane pyrimidine dimers (CPD) and (6–4) photoproducts (6–4PP; Wang et al., 1974; Sinha and Häder, 2002; Murata and Osakabe, 2017), as well as reactive oxygen species (ROS), such as singlet oxygen, which cause oxidative damage to DNA (Kielbassa et al., 1997; Zhang et al., 1997; Cadet et al., 2015) and lipid peroxidation (Girotti, 1998; Miyamoto et al., 2014; Morita et al., 2016; Atarashi et al., 2017). Therefore, many mites avoid UV damage by residing on the lower side of leaves (Sudo and Osakabe, 2011). Leaves contain compounds that shield against UV radiation to protect their inner organs (Lavola et al., 1998; Rousseaux et al., 2004; Tegelberg et al., 2004; Izaguirre et al., 2007); therefore, mites below leaves are sheltered from UV (Ohtsuka and Osakabe, 2009; Sakai and Osakabe, 2010).
Spider mite species of the Genus Panonychus such as the citrus red mite Panonychus citri (McGregor), constitutively produce astaxanthin, which accumulates mainly as esters (Metcalf and Newell, 1962; Atarashi et al., 2017; Bryon et al., 2017). Astaxanthin is the most powerful antioxidant of the carotenoids (Miki, 1991; Camera et al., 2009; Hama et al., 2012). It reduces lipid peroxidation via its antioxidant effects (Atarashi et al., 2017), and thus the eggs of P. citri are more tolerant to UVB radiation than the eggs of the two-spotted spider mite Tetranychus urticae Koch (Fukaya et al., 2013). Moreover, wild-type P. citri females exhibit higher survival rates than albino P. citri females, which lack the capacity to produce astaxanthin, under thermal oxidative stress (Atarashi et al., 2017; Bryon et al., 2017), which suggests that intrinsic astaxanthin confers tolerance to UV radiation. Consequently, Panonychus species can use upper leaf surfaces (Foott, 1963; Jones and Parrella, 1984; Morimoto et al., 2006; Osakabe et al., 2006; Fukaya et al., 2013).
Upper leaf surfaces may also be predator-free spaces, because predacious phytoseiid mites are more vulnerable to UVB radiation than spider mites (Onzo et al., 2010; Tachi and Osakabe, 2012; Koveos et al., 2017). Nakai et al. (2018) reported that the egg hatchability and survival rate of hatched larvae of the phytoseiid mite Neoseiulus californicus (McGregor) after exposure to UVB radiation is higher when parent females feed on P. citri than when they feed on T. urticae. The eggs likely inherit protective compounds that their mother ingests, and non-enzymatic antioxidants may help phytoseiid mites survive exposure to UVB radiation. Spider mites are potentially reactivated from UV damage through photoenzymatic repair of DNA lesions by CPD photolyase using energy from UVA (315–400 nm wavelength) and visible lights (photoreactivation; Murata and Osakabe, 2014, 2017; Suzuki et al., 2014) though no genes of (6–4) photolyase that repairs 6–4PP are found in T. urticae genome (Grbić et al., 2011). Nakai et al. (2018) reported a marked photoreactivation in N. californicus (but see Koveos et al., 2017). The enzymes associated with photoreactivation might be protected from oxidative stresses by non-enzymatic antioxidants.
Pollen is a preferred alternative food for many phytoseiid mites, in their natural habitat and in artificial propagation for the production of biological control agents (Castagnoli and Simoni, 1999). Pollen provides nutrients for many phytoseiid mites to develop and reproduce (McMurtry and Croft, 1997; Croft et al., 1998); thus pollen availability (wind-borne pollen in many cases) affects the abundance of phytoseiid mites in the field (Addison et al., 2000; Duso et al., 2004; Villanueva and Childers, 2004). On the other hand, pollen is frequently exposed to solar radiation. Therefore, it likely contains protective compounds that protect germ cells from UVB damage caused by solar radiation (Feng et al., 2000; Koti et al., 2005; Wang et al., 2010; Zhang C. et al., 2014; Žilić et al., 2014). If phytoseiid mites obtain these protective compounds (Stewart et al., 1996), pollen feeding may contribute to their adaptation to solar UVB radiation. Moreover, because spider mites are economically important horticultural pests that have developed serious acaricide resistance, physical control of spider mites using UVB is now under development (Tanaka et al., 2016). Improving UVB resistance in phytoseiid mites is advantageous for the concurrent use of UVB and biological control in greenhouses.
In this study, we first tested a hypothesis that pollen feeding improves UVB resistance of N. californicus. For this purpose, we designed experiments to test questions whether pollen diet increased adult survivorship and egg hatchability after UVB irradiation. We also considered the protective effects on photoenzymatic repair system of DNA lesion and the vulnerable age specific effects in eggs. In these experiments, we compared the effects of pollen with those of a prey mite, T. urticae. Finally, absorbance spectra and antioxidant capacities of pollen and prey mite extracts were compared and antioxidants in pollen were identified. Consequently, we provide evidence of the importance of pollen as antioxidant source in solar adaptation.
Neoseiulus californicus is a widespread Type II phytoseiid mite (McMurtry and Croft, 1997; Luh and Croft, 2001) used for spider mite control in horticultural crops and orchards worldwide. This mite prefers to feed on both Tetranychus spider mites and pollen (Castagnoli and Simoni, 1999). Therefore, it can be used to compare the effects of pollen and prey mites (T. urticae) on UVB resistance. The N. californicus population used in this study was originally established from field population collected from Matsukawa, Nagano Prefecture, Japan (35°36′ N, 137°55′ E; Toyoshima and Hinomoto, 2004) in September 2000. T. urticae is a pest of horticultural crops and orchards. Because it has developed resistance to most acaricides, chemical control has been difficult, and alternative management systems are sought. The T. urticae population used in this study is a laboratory population cultured on potted kidney bean plants at 25–28°C for more than 9 years. These mites were reared on kidney bean leaves placed on water-soaked cotton in Petri dishes in a chamber at 25°C with a photoperiod of 16:8 (L:D) h (fluorescent lights turned on at 07:00 h and off at 23:00 h). T. urticae and pollen were provided as food for N. californicus.
We used tea (Camellia sinensis [L.] Kuntze) and peach (Amygdalus persica L.) pollen. Buds were collected from tea trees at the Kitashirakawa Experimental Field of Kyoto University, Kyoto, Japan (35°2′ N, 135°47′ E) and from peach trees of the variety “Akatsuki” from the experimental field of the Nagano Fruit Tree Experiment Station, Suzaka, Japan (36°39′ N, 138°19′ E). Anthers were detached from the buds. After anther dehiscence, tea pollen was separated from the anthers using sieves, whereas peach pollen was used together with the anthers owing to its adherence to anthers. The pollen was stored in a freezer at −20°C until use in bioassays. Pollen from both tea and peach trees was sieved, although peach pollen was adherent, and immediately used for compound extraction.
UVB irradiation of adult females was performed at 25°C in a laboratory illuminated with fluorescent lamps. Females on leaf disks in Petri dishes were placed on a shelf located at a distance of 0.45 m from an overhead UVB (UVB intensity: 1.43 W m−2, peak weavelength: 312 nm, full width at half maximum: 28) lamp (20 W; YGRFX21701GH; Panasonic Co., Osaka, Japan; Figure S1A) affixed to the top of a steel rack (1.9 m high × 0.6 m wide × 0.6 m deep). Petri dishes for untreated controls were placed under UV-opaque film (HB3 polyester film, 25 μm thick; Teijin DuPont Films, Tokyo, Japan), which filtered out >90% of <380 nm wavelength and >99% of <363 nm wavelength (Sakai and Osakabe, 2010).
For experiments on eggs, we used a UVB lamp (6 W; Panasonic Co., Osaka, Japan) affixed to a shelf 0.67 m overhead (UVB intensity: 0.16 W m−2; Figure S1B) in a dark growth chamber at 25°C. Two halogen lamps (130 W; JDR110V-85WHM/K7-H; Ushio Lighting Co. Ltd., Tokyo, Japan; Figure S1C) affixed to a shelf 67 cm overhead and set at a distance of 22.5 cm from the UVB lamp were used to induce photoreactivation (visible light intensity: 67.7 W m−2).
Five gravid adult females of N. californicus within 4 days after their last molt were introduced to 24 kidney bean leaf squares (3.5 × 3.5 cm) on water-soaked cotton in Petri dishes (9 cm in diameter; a leaf square per dish). We prepared three types of food source for phytoseiid mite; 8 of the 24 leaf squares contained T. urticae eggs (produced by 10 adult females for 24 h), tea pollen, or peach pollen. The phytoseiid mites had developed on the same food as that prepared on the leaf squares. Four of the 8 leaf squares assigned for a given food type were irradiated with UVB at intensity of 1.43 W m−2 (UVB+), while the remaining four leaf squares were covered with UV-opaque film on the same shelf during the UVB-irradiation (UVB-unirradiated control; UVB–). UVB irradiation was performed for 90 min (cumulative irradiance = 7.72 kJ m−2) or for 180 min (15.44 kJ m−2); UVB dose of the former was equivalent to the daily cumulative UVB irradiance in February and early November, and the latter was the similar to that in April and September in Kyoto City, Japan.
After irradiation, phytoseiid mites were individually moved to new leaf squares (1.5 × 1.5 cm), which contained assigned food source, on water-soaked cotton in a rectangular plastic case (10 × 19 × 2 cm). Then the N. californicus females were reared in the laboratory (day 0), and survival and behavior were assessed every day until day 5. Damage was determined by stimulating the females with a fine small brush and categorized as four levels: (a) walked normally, (b) walked, but sluggish, (c) did not walk, and (d) died. The prey mites and pollen were properly added during the rearing experiments. This experiment was replicated four times.
We preliminarily confirmed that the individuals that did not walk (c) never recovered and died later; thus, individuals categorized into groups (a) and (b) were grouped as survivors while those in (c) and (d) were considered dead. Prior to statistical analyses, the datasets (numbers of individuals) were combined over the 4 replications. We excluded the data for the phytoseiid mites that escaped from leaf squares from the following data analysis. Consequently, for treatments at 7.72 kJ m−2 irradiance, a total of 78, 69, and 78 females were evaluated in the T. urticae, tea pollen, and peach pollen treatments, respectively, and 79, 75, and 76 females were evaluated in the untreated control group. For treatments at 15.44 kJ m−2 irradiance, the numbers were 78, 77, and 77, and 77, 79, and 74, respectively.
To determine an appropriate UVB dose for evaluating dietary effects, we tentatively tested the effects of doses of 0.174, 0.192, 0.288, and 0.408 kJ m−2 on the hatchability of eggs produced by mite- and tea pollen-fed N. californicus females. More than 90% of the eggs irradiated at 0.174 kJ m−2 hatched, and in contrast, almost all the eggs irradiated at 0.408 kJ m−2 died. Eventually, we chose a UVB dose of 0.192 kJ m−2 for subsequent experiments because the dietary effects were most clear at this level (Figure S2).
Four leaf squares (2 × 2 cm) were placed on water-soaked cotton in 6 Petri dishes. Leaf squares in 2 of the 6 Petri dishes contained T. urticae eggs (produced by 10 females for 24 h), tea pollen, or peach pollen. Five gravid adult N. californicus females were introduced to each leaf square and reared in the laboratory for egg production. The phytoseiid mites had developed on the same prey or foods as that on the leaf square. Females were removed from Petri dishes after 24 h, 1 of the 2 Petri dishes containing the same food and N. californicus eggs was assigned to the UVB irradiation treatment and the other was assigned to the untreated control group. The Petri dish assigned to the UVB irradiation treatment (4 leaf squares) was irradiated with UVB at 0.16 W m−2 for 20 min (0.192 kJ m−2) in a dark growth chamber. The Petri dish for untreated controls (4 leaf squares) was put in a cardboard box and placed in a growth chamber during the UVB irradiation treatments. After UVB irradiation, the Petri dish irradiated with UVB was also put in a cardboard box to keep them in the dark and placed in the laboratory (day 0). The number of eggs hatched was counted each day until day 4. The Petri dishes were moved into a transparent plastic box on day 2 to minimize photoreactivation efficiency by the 4 h time lag between UVB irradiation and visible light irradiation of T. urticae (Murata and Osakabe, 2014). This experiment was replicated three times. Prior to statistical analyses, the datasets were combined over replications. Consequently, the number of eggs produced by females of T. urticae, tea pollen, and peach pollen diets were 100, 89, and 67, respectively, in the UVB irradiation treatment and 109, 89, and 73, respectively, in the untreated control.
We designed three treatments for N. californicus eggs produced by mite-feeding females, tea pollen-feeding females, and peach pollen-feeding females: the eggs were (1) irradiated with UVB but not irradiated with visible light (UVB+/VIS–), (2) irradiated with UVB and then irradiated with visible light (UVB+/VIS+), or (3) never irradiated with either UVB or visible light (UVB–/VIS–). Three Petri dishes containing four leaf squares (2 × 2 cm) were prepared for each treatment. The leaf squares contained the same food as that females had fed on. Five gravid N. californicus females were introduced to each leaf square and maintained in the laboratory for 24 h. After females were removed from leaf squares, the Petri dishes assigned to (1) and (2) were irradiated with UVB at 0.16 W m−2 for 40 min (0.384 kJ m−2) in a dark growth chamber. The Petri dishes assigned to (3) were kept in the dark in a cardboard box and placed in the growth chamber. Immediately after UVB irradiation, the Petri dishes for (1) were moved inside the cardboard box, and only the Petri dishes for (2) were irradiated with visible light for 90 min (365.58 kJ m−2). Then the Petri dishes for (2) were also put into the cardboard box and placed in the laboratory (day 0). Egg hatchability was observed in the same manner as described in section Egg Hatchability. This experiment was replicated three times. Prior to statistical analyses, the datasets were combined over replications. The number of eggs produced by females of T. urticae, tea pollen, and peach pollen diets were 149, 134, and 96, respectively, in (1); 142, 130, and 109, respectively, in (2); and 145, 121, and 87, respectively, in (3).
Four Petri dishes containing four leaf squares (2 × 2 cm) were prepared: two Petri dishes were assigned for each type (mite-feeding and tea pollen-feeding) of females, and prey eggs and tea pollen on the leaf squares were prepared as in section Egg Hatchability, but 20 adult T. urticae females were introduced to each leaf square for prey egg preparation. One of the two Petri dishes for each type was assigned to the UVB irradiation treatment and the other was the untreated control. Ten adult N. californicus females that developed on the same prey or pollen as on the leaf square were introduced to each square. We prepared another four Petri dishes containing six leaf squares (1 × 1 cm) without prey or pollen. Then we moved all N. californicus eggs laid on the 4 leaf squares in a Petri dish to one of the six squares in the new Petri dishes every 8 h until 48 h later. After 24 h, to avoid shortages of prey and pollen, the adult N. californicus females were moved to newly prepared leaf squares. Immediately after all operations were completed, the eggs in the Petri dishes for UVB irradiation treatment were exposed to UVB radiation at 0.16 W m−2 for 20 min (0.192 kJ m−2) in a dark growth chamber. The Petri dishes of untreated controls were put in a cardboard box and placed in the growth chamber during UVB irradiation. Then the UVB-irradiated Petri dishes were also put in the cardboard box and placed in the laboratory (day 0). Using this method, we exposed N. californicus eggs categorized into six different age classes to UVB radiation at the same time: 0–8 h, 8–16 h, 16–24 h, 24–32 h, 32–40 h, and 40–48 h. Egg hatchability was observed in the same manner as described in section Egg Hatchability. This experiment was replicated three times. The average number of eggs used for each replication was 91.5 ± 8.0 (SD) and 88.3 ± 6.1 in T. urticae-fed UVB irradiated and untreated mites, respectively, and 81.2 ± 9.8 and 80.0 ± 12.4 in tea pollen-fed irradiated and untreated mites, respectively. To evaluate the effects of irradiation time and diet, and the interaction between the two, the hatchability in each replication was transformed as the empirical logit. To evaluate the effects of diet at each irradiation exposure duration, the datasets were combined over replications. The numbers of eggs used for 0–8 h, 8–16 h, 16–24 h, 24–32 h, 32–40 h, and 40–48 h were 81, 95, 89, 91, 105, and 88, respectively, in T. urticae-diet and 89, 88, 81, 82, 96, and 94, respectively, in tea pollen-diet.
Tea pollen (370 mg) and peach pollen (140 mg) from 511 and 315 buds, respectively, were collected and soaked in 20 and 15 mL methanol, respectively, for 3 days under dark conditions in the laboratory at room temperature. The resulting methanol solutions were filtrated using qualitative filter paper (No. 2; Advantec, Co., Ltd., Tokyo, Japan), and concentrated at 45°C in vacuo to yield 111 and 51 mg extracts from tea and peach pollen, respectively. Adult T. urticae females (1,600 ♀♀, 25 mg) were soaked in 8 mL methanol for 1 day. The methanol solution was centrifuged at 6,000 rpm for 15 min, and the supernatant was concentrated at 45°C in vacuo to yield 7.4 mg extract.
The UVB absorbing capacity was evaluated using two methods. First, the UV spectra (280–315 nm wavelength) of the methanol solutions (10 μg extract mL−1) were measured using a spectrophotometer (UV-1800; Shimadzu Co., Ltd., Kyoto, Japan), and the area under the curve (AUC) was calculated. Second, we analyzed the peak area detected at a wavelength of 300 nm by high-performance liquid chromatography (HPLC; L-7420 UV-Vis detector; Hitachi High-Tech Science Co., Tokyo, Japan) using a reverse-phase column (6 × 100 mm, 5 μm; YMC-Pack ODS-AQ311; YMC Co., Ltd., Kyoto, Japan). UV at 300 nm and shorter wavelengths kill N. californicus eggs, whereas UV at 310 nm and longer wavelengths do not (Tachi and Osakabe, 2014). The mobile phase was eluted with a gradient from 0.1% acetic acid/15% methanol to 0.1% acetic acid/70% methanol between 0–40 min, and isocratic 0.1% acetic acid/99.9% methanol between 40–50 min. We calculated the total peak integral values (TPV). Extracts (4 μg) from T. urticae, peach pollen, and tea pollen were analyzed with HPLC under the above conditions. The total areas of the peaks detected by an integrator were calculated, and the areas per 4 μg were converted into those per 1 mg fresh weight (FW).
We evaluated the antioxidant activities of the extracts using 1,1-diphenyl-2-picrylhydrazyl (DPPH) radical scavenging activity. Extracts were resolved with methanol, and 50 μL extract solution and 100 μL DPPH methanol solution (200 μM) were mixed in a well of a 96-well microplate. The extracts were diluted to the concentration appropriate to measure dose-response. The microplate was incubated in the dark at room temperature for 20 min. Then the absorbance of the mixture at 520 nm was measured with a microplate absorbance reader (iMark; Bio-Rad Co., Ltd., Hercules, CA, USA). Radical scavenging activities (RSAs) were calculated using the following formula:
where Acontrol, Asample, and Ablank represent the absorbance at 520 nm of the control that excluded the extract in the reaction mixture, the sample, and the blank (methanol), respectively. Antioxidant activity was evaluated in terms of IC50 values (mg FW mL−1) and EC50 values (μM).
The methanol extract (434 mg) of peach pollen was dissolved in 60 mL water and partitioned three times with 30 mL ethyl acetate. The aqueous layer was partitioned three times with 30 mL n-butanol. The ethyl acetate and n-butanol layers were combined and concentrated to yield viscous compounds (189 mg). These compounds were applied to a silica gel (11 g) column and eluted with mixtures of chloroform and methanol. Compounds eluted with 15% and 20% methanol were combined and concentrated to yield viscous compounds (67 mg). The compounds were applied to an ODS (18 g) column and eluted with mixtures of water and methanol. Compounds eluted with 30% methanol solution were concentrated to yield compounds (22 mg). The compounds were subjected to a preparative HPLC (column: YMC-Pack AQ-311 ODS 6 mm i.d. × 100 mm, eluent: 0.1% acetic acid/23% methanol, flow rate: 1.0 mL/min, detection: 254 nm). Compounds eluted at tR 10.4 and 11.4 min were collected and concentrated to yield compound 2 (1 mg) and compound 3 (0.3 mg), respectively. Concentration of the fraction eluted with 30% methanol from the silica gel column yielded a yellow crystalline compound (compound 1, 3 mg).
The concentrated methanol extract (33 mg) was dissolved in 30 mL water and partitioned with 15 mL ethyl acetate. The organic layer was concentrated to yield viscous compounds (8 mg). The compounds were subjected to a preparative HPLC (column: YMC-Pack AQ-311 ODS 6 mm i.d. × 100 mm, eluent: 50% methanol aqueous solution containing 0.1 % acetic acid, flow rate: 1.0 mL min−1, detection: 254 nm). The compounds eluted at tR 7.5, 9.5, 12.3, and 17.6 min were separately collected under darkness, and concentrated to yield compounds 4, 5, 6, and 7, respectively. For spectral analyses of the substances, we used Nuclear magnetic resonance (NMR; Bruker Avance III 400 [400 M Hz], CD3OD; Bruker, Billerica, MA, USA) and liquid chromatography coupled with mass spectrometry (LC-MS; Waters Hclass/Xevo G2-S QTof; column: Acquity UPLC BoEH C18; Waters Co., Milford, MA, USA).
The methanol extract (58 mg) from tea pollen was dissolved in 40 mL water and partitioned three times with 15 mL ethyl acetate. Ethyl acetate-soluble compounds (8 mg) were applied to an ODS gel column (4 g, YMC-GEL-ODS-AQ, 12 nm, S-50 μm, YMC, Kyoto, Japan), and eluted with 30 mL each of 0, 10, 20, 30, 50, 70, and 100% methanol in water. Compounds (1 mg) eluted with 30% methanol were purified with an ODS HPLC column (YMC-Pack AQ-311 ODS 6 mm i.d. × 100 mm) by elution with 0.1% acetic acid/40% methanol at 1.0 mL min−1, with the eluate monitored at 254 nm. A compound eluted at tR 1.7 min was collected and concentrated to yield a trace amount of compound 10 [(+)-catechin]. Compounds (<1 mg) eluted with 50% methanol were purified with an ODS HPLC column under the above conditions. A compound eluted at tR 2.9 min was collected and concentrated to yield a trace amount of compound 8. Compounds (1 mg) eluted with 70% methanol were concentrated to yield a mixture (0.8 mg) of compounds 6, 7, and 9. NMR and LC-MS were used for spectral analyses of the substances.
Tea pollen (73, 210, and 136 mg were used for replications) and peach pollen (37, 41, and 62 mg; replications) were newly collected and soaked in 5 mL methanol for 3 days in the dark at room temperature. Consequently, we obtained tea pollen extracts of 15, 51, and 31 mg and peach pollen extracts of 13, 16, and 22 mg. Compounds 7–10 in tea pollen extracts and compounds 1–7 in peach pollen extracts were quantified using HPLC (column: YMC-Pack AQ-311 ODS 6 mm i.d. x 100 mm, flow rate: 1.0 mL min−1). The mobile phases were 0.1% acetic acid/50% methanol (detection at 254 nm) for compounds 7 and 9, 0.1% acetic acid/35% methanol (detection at 254 nm) for compound 8, and 0.1% acetic acid/25% methanol (detection at 254 nm) for compound 10. The mobile phases were 0.1% acetic acid/40% methanol (detection at 272 nm) for compound 1, 0.1% acetic acid/23% methanol (detection at 254 nm) for compounds 2 and 3, and 0.1% acetic acid/50% methanol (detection at 254 nm) for compounds 4–7. Quantification of each compound was performed by comparing integral values of the peak area of the samples to that of the standard compound.
Differences in the survival of adult females, egg hatchability, and photoreactivation efficiency of eggs were evaluated by pairwise comparisons of proportions using Fisher's exact test with less conservative corrections (Benjamini and Hochberg, 1995; [BH]) using “fmsb” package (Nakazawa, 2017) in “R” software (R Core Team, 2014).
The effects of UVB irradiation timing (egg age; 2.4.4) and the diets of parent females on egg hatchability were evaluated using two-way analysis of variance (ANOVA) using the “aov” module followed by Tukey's honestly significant difference (HSD) post hoc test (“TukeyHSD” function in R) after conducting a Bartlett's test for homogeneity of variances (“bartlett.test” function in R). Prior to ANOVA, an empirical logit transformation was applied to the dataset (Stevens et al., 2016; Donnelly and Verkuilen, 2017). Data from 40–48 h from the age specific vulnerability in eggs were excluded from the two-way ANOVA and post-hoc analyses to ensure equal variance.
AUC and TPV were standardized by subtracting an average value from each raw data point and then dividing the remainder by the standard deviation prior to statistical analyses. The effects of diet and measuring methods on the UVB absorbing capacity were evaluated using a generalized linear regression model (GLM) with the “glm” module followed by post hoc analyses using the Tukey method with the “glht” module of the “multcomp” package in R software.
The RSAs of methanol extracts of the diets were evaluated in the exact same way. Linear regression analyses between dose and RSA in each diet (methanol extract) were performed using “lm” module with the intercept assumed to be equal to 0.
Symptom (b) (walked, but sluggish) appeared soon after irradiation with UVB in N. californicus females fed T. urticae (Figures S3, S4). Consequently, the survival rates were 45.1 and 11.8% at 7.72 and 15.44 kJ m−2, respectively, after 5 days (Figure 1). A greater number of N. californicus females survived when fed pollen than when fed T. urticae for 5 days after UVB irradiation at both 7.72 and 15.44 kJ m−2 (Figure 1). The number of damaged individuals was higher in females fed tea pollen than in those fed peach pollen at 7.72 kJ m−2 (Figure S3), and thus the survival rate by day 5 was higher in the latter than in the former, although no statistically significant difference was detected (Fisher's exact test [BH], P > 0.05; Figure 1A). By contrast, the survival rate of females fed tea pollen was higher than that of those fed peach pollen at 15.44 kJ m−2 (P < 0.05; Figure 1B). Many of the surviving pollen-fed females remained at symptom of (b) (Figure S4). Most females (>90%; Figure 1) fed pollen survived for 5 days in the control (Figures S3, S4), whereas the survival rate of females fed T. urticae was lower in both experiments (P < 0.05; Figures 1A,B).
Figure 1. Comparison of the survival rates of adult females fed mites or pollen for 5 days after UVB irradiation. (A) 7.72 kJ m−2, (B) 15.44 kJ m−2. UV+, irradiated with UVB; UV–, untreated control. Prey and food sources of parental females were T. urticae (Tu), peach pollen (Pe), and tea pollen (Te). The vertical lines on each bar show 95% CI. Different letters indicate statistically significant differences based on pairwise comparisons of proportions (Fisher's exact test, P < 0.05).
More than 98% of untreated eggs hatched within 4 days, whereas irradiated eggs exhibited decreased hatchability (Figure 2). The eggs produced by T. urticae-fed females showed the greatest decrease in hatchability after UVB irradiation at 0.192 kJ m−2 (50%), whereas the degradation of the hatchability was minimal in eggs produced by tea pollen-fed females (71.9%; Fisher's exact test [BH], P < 0.05). The degree of degradation in hatchability was intermediate in the eggs produced by peach pollen-fed females (61.2%).
Figure 2. Comparison of the hatchability of N. californicus eggs produced by females fed mites or pollen and irradiated with UVB at 0.192 kJ m−2. UV+, irradiated with UVB; UV–, untreated control. Prey and food sources of parental females were T. urticae (Tu), peach pollen (Pe), and tea pollen (Te). Vertical lines on bars show 95% CI. Different letters in each bar denote statistically significant differences (Fisher's exact test, P < 0.05).
Egg hatchability after UVB irradiation at 0.38 kJ m−2 without photoreactivation (UV+/VIS–; Figure 3) was highest in eggs from peach pollen-fed females and lowest in those from T. urticae-fed females (Fisher's exact test [BH], P < 0.05). The hatchability was markedly increased by photoreactivation induced by visible light irradiation after UVB irradiation (UV+/VIS+; Figure 3), and was highest in eggs from tea pollen-fed females and lowest in those from T. urticae-fed females (P < 0.05). When the eggs were not irradiated with UVB, hatchability was greater than 98% (UV–/VIS–; Figure 3).
Figure 3. Comparison of the hatchability of N. californicus eggs produced by females fed mites or pollen with and without photoreactivation after irradiation with UVB at 0.38 kJ m−2. UV+, irradiated with UVB; UV–, untreated control. Prey and food sources of parental females were T. urticae (Tu), peach pollen (Pe), and tea pollen (Te). Vertical lines on bars show 95% CI. Different letters in each bar denote statistically significant differences (Fisher's exact test, P < 0.05).
The vulnerability of eggs to UVB radiation varied by both their age [two-way ANOVA, F(4, 20) = 40.083, P = 2.78 × 10−9] and the diet of parent females [F(1, 20) = 7.434, P = 0.013], whereas the interaction between those factors was not significant [F(4, 20) = 0.585, P = 0.677; Figure 4]. Eggs irradiated within 16 h after oviposition (0–8 h and 8–16 h) were more vulnerable to UVB than those treated more than 16 h after oviposition (Tukey HSD, P < 0.05). The effects of female diet on egg vulnerability were obvious in the early vulnerable stages at 0–8 h and 8–16 h.
Figure 4. Comparison of age specific vulnerability of N. californicus eggs produced by females fed mites or pollen after irradiation with UVB at 0.192 kJ m−2. Prey and food sources of parental females were T. urticae (Tu), peach pollen (Pe), and tea pollen (Te). Each plot shows hatchability calculated from combined data over three replications. Vertical lines on bars show 95% CI. Different letters above the plot area indicate statistically significant differences between the timing of UVB irradiation using the Tukey-Kramer method (P < 0.05).
The AUCs of tea pollen, peach pollen, and T. urticae were 2.54, 2.13, and 1.23 per 10 μg extract, respectively (Figure S5). The TPVs of tea pollen, peach pollen and T. urticae were 1.0 × 106, 1.7 × 106, and 1.1 × 106 per 4 μg extract, respectively (Figure S6). Consequently, the UVB-absorbing capacity per fresh weight (FW) was highest in peach pollen extract (AUC: 739 [mg FW]−1, TPV: 15 × 107 [mg FW]−1), second highest in tea pollen (AUC: 618 [mg FW]−1, TPV: 6.1 × 107 [mg FW]−1), and lowest in T. urticae (AUC: 371 [mg FW]−1, TPV: 2.6 × 107 [mg FW]−1) (Tukey method, P < 0.01; Figure 5). Pollen diets potentially have greater shielding effects against UVB radiation than mite diets.
Figure 5. Standardized UVB-absorbing capacity of methanol extracts from T. urticae (Tu), peach pollen (Pe), and tea pollen (Te). UVB-absorbing capacity measured as the area under the curve (AUC) of UV spectra at 280–315 nm wavelength by a spectrophotometer and total peak integral values (TPV) by high-pressure liquid chromatography (HPLC) equivalent to fresh weights. Different letters at the right of the plots represent statistically significant differences (Tukey method, P < 0.01).
The RSA of the methanol extracts was highest in tea pollen, followed by peach pollen and T. urticae (Tukey method, P < 0.001; Figure 6). The IC50 values per FW were 16, 7, and 1 mg FW mL−1 for T. urticae, peach pollen, and tea pollen, respectively.
Figure 6. Radical scavenging activities (RSA) of methanol extracts from T. urticae (Tu), peach pollen (Pe), and tea pollen (Te). Different letters on the regression line indicate statistical significance (Tukey method, P < 0.001). Regression line formulas are Tu: y = 10.5x [SE for slope = 0.2, r2 = 0.999, F(1, 2) = 2,488, P = 0.0004]; Pe: y = 18.9x [SE for slope = 1.2, r2 = 0.989, F(1, 2) = 267.8, P = 0.0037]; and Te: y = 213.1x [SE for slope = 12.2, r2 = 0.984, F(1, 4) = 303, P = 6.39 × 10−5].
Using HPLC and RSA, compounds 1–7 with antioxidant capacity were obtained from the methanol extract of peach pollen. Compounds 1–3 were identified as 8-methoxykaempferol 3-O-sophoroside (yellow pigment), 1-O-feruloyl β-D-glucose, and 1-O-(Z)-feruloyl β-D-glucose, respectively, by comparing their spectral data (Figure 7, Table S1) with literature data (Harborne and Corner, 1961; Stošić et al., 1988). Compounds 4–7 were identified as N1,N5,N10-tri-p-(Z,Z,Z)-coumaroylspermidine, a mixture of N1,N5,N10-tri-p-(E,Z,Z)-coumaroylspermidine and N1,N5,N10-tri-p-(Z,Z,E)-coumaroylspermidine, N1,N5,N10-(E,Z,E)-tri-p-coumaroylspermidine, and N1,N5,N10-tri-p-(E,E,E)-coumaroylspermidine, respectively, by comparison of their spectral data (Strack et al., 1990; Figure 7, Table S1). The purified compounds 4–7 were easily isomerized, and gave an equilibrium mixture of 4, 5, 6, and 7 at a ratio of 33:45:20:2. However, the methanol extracts from peach pollen frozen for 2 months contained compound 7 and traces of compounds 4–6, which indicates that compound 7, with a maximum absorption wavelength of 310 nm (Figure S7), is a major isomer in peach pollen.
The RSAs of compounds 1–3 and 7 (containing 4–6) were high (EC50 >700 μM; Table 1). We tentatively compared the antioxidant activity among compounds 4–7 based on RSA only at 400 μM (n = 3) because the content of each isomer was insufficient to determine EC50 values. The RSA (%) increased as the Z isomer of the coumaroyl groups increased: 20.7 ± 1.6 (SE), 15.1 ± 2.7, 9.2 ± 2.3, and 9.2 ± 2.4 in compounds 4, 5, 6, and 7, respectively. Although its antioxidant activity was low, tri-p-coumaroylspermidine was often present in peach pollen, thus its contribution to the total RSA in peach pollen was highest (38%) among the constituents (7% each by 1 and 1-O-feruloylglucose [2, 3]; Table 1).
Table 1. Contents, activities and maximum absorption wavelength of antioxidants in peach and tea pollens.
Compound 8, a mixture of compounds 6, 7, and 9, and compound 10 were obtained from the methanol extract of tea pollen as antioxidants, based on their RSAs. Compound 8 was identified as epicatechin 3-gallate by LC-MS and NMR (Yang et al., 2012; Figure 7, Table S1). Compounds 6 and 7 were identified as tri-p-coumaroylspermidine isomers, similar to the components from peach pollen, and 9 was identified as N1,N10-di-p-(E,E)-coumaroyl-N5-(E)-caffeoylspermidine (Bokern et al., 1995; Yang et al., 2012; Figure 7, Table S1) by NMR and LC-MS analyses. Compound 10 (tR 5.5 min) was identified as catechin (Figure 7, Table S1), which would be (+)-catechin, frequently found in tea leaves and flowers (Nanjo et al., 1996; Sano et al., 2001; Yang et al., 2009).
Of the comprehensive antioxidant activity in methanol extract from tea pollen, 12, 12, and 10% of the antioxidant capacity was due to compound 8, a mixture of compound 10, and a mixture of compounds 6, 7, and 9, respectively (Table 1). In complex spermidines, compound 9 and di-p-coumaroyl-feruloylspermidine showed higher antioxidant capacities than compound 7 (Table 1). We tentatively confirmed the higher antioxidant capacities of caffeic acid (RSA EC50 = 12 μM) and ferulic acid (20 μM) compared to p-coumaric acid (>1,500 μM), thus the antioxidant capacities of compound 9 and di-p-coumaroyl-feruloylspermidine were likely due to caffeoyl- and feruloyl-base, respectively. Catechins 8 and 10 exhibited higher antioxidant capacity and equal to or greater content than complex spermidines (Table 1). Therefore, catechins were the major antioxidants, rather than complex spermidines.
Interactions between plants and predators have been addressed in evolutionary scenarios of prey–predator relationships between herbivores and predators, such as sequestration of plant-derived toxins by herbivores (Koller et al., 2007; Opitz and Müller, 2009; Suzuki et al., 2011), and exploitation of herbivore-induced plant volatiles by predators (Price et al., 1980; Dicke and Baldwin, 2010; Aartsma et al., 2017). However, little attention has been paid to plant–herbivore–predator tri-trophic interactions or direct plant–predator interactions via protective compounds produced by plants in environmental adaptations of herbivores and predators that affect community development.
Solar UVB radiation has adverse effects on plants (Greenberg et al., 1989; Bothwell et al., 1994), inducing them to accumulate protective compounds in their epidermis that possibly shield against UVB penetration to mesophyll cells (Izaguirre et al., 2007). UVB-absorbing compounds that accumulate in plant leaves include flavonoids and its polymers (tannins), sinapinic acid and its esters, salicylic acid, chlorogenic acid, mycosporine-like amino acids (algae), and others (Dunlap and Shick, 1998; Clé et al., 2008; Dean et al., 2014; Khan et al., 2015; Nascimento et al., 2015; Vidović et al., 2015). Such compounds are frequently induced by UV irradiation (Logemann et al., 2000) and protect plants from oxidative stress not only through their UVB-absorbing capacity but also their antioxidant capacity (Jansen et al., 1998). As pollen is frequently exposed to solar UVB radiation, it is also protected by UVB-absorbing compounds. The content of UVB-absorbing compounds in flower organs such as ovaries is not affected by UVB irradiance, whereas the content of these compounds in pollen is increased by UVB irradiation (Day and Demchik, 1996; Santos et al., 1998; Rozema et al., 2001; Fraser et al., 2014). Moreover, pollen frequently fluoresces under ultraviolet light, whereas the petals and filaments of stamens do not (Berger, 1934; Asbeck, 1955; Pöhlker et al., 2013). Through autofluorescence, pollen may escape part of the energy of UV.
Both UVB-absorbing capacity and DPPH radical-scavenging capacity were higher in methanol extracts from pollen than from spider mites, and the extracts of peach and tea pollen showed distinctive features from each other. Peach pollen had greater UVB absorbance, whereas tea pollen had higher antioxidant capacity. Although no significant differences were detected among UVB-absorbing capacities equivalent to the weight of extracts from diets, the capacities equivalent to the FW of diets was highest in peach pollen, followed by tea pollen and then T. urticae, which suggests that the concentration of UVB-absorbing compounds was higher in peach pollen than in tea pollen. In peach pollen, compound 7 was the major antioxidant and presented as an EEE isomer, which had the lowest antioxidant capacity among its isomers. However, the absorption wavelength spectrum of compound 7 (peak at 299–310 nm) was consistent with the solar radiation wavelengths causing the largest biological impact (Coohill and Sagripanti, 2009). Peach pollen also contained substances that can shield against UVB: fluorescent substances (2, 3) and a flavonoid glycoside (1). Tea pollen also possessed tri-p-coumaroylspermidine, but its content was not large compared to that in peach pollen. We found that tea pollen frequently contained catechins (8, 10), as found in tea leaves and flowers (Matsuzaki and Hara, 1985; Sano et al., 2001).
Therefore, the major protective effects of peach pollen and tea pollen were UVB shielding and antioxidant capacity, respectively, and the differences in protective functions were derived from their distinctive antioxidant components. The fact that the protective function of tea pollen was more effective than that of peach pollen suggests the significance of antioxidant capacity for phytoseiid mites. Moreover, the improved hatchability after UVB irradiation of eggs from females fed pollen diets indicates that the females delivered antioxidants to their eggs. Maternal inheritance of an antioxidant essential to diapause induction, β-carotene, has been shown in the eggs of Tetranychus spider mites (Veerman and Helle, 1978; Veerman, 1980). In a previous study, egg hatchability and survival rate of hatched larvae after UVB radiation were higher for N. californicus females fed P. citri than for females fed T. urticae (Nakai et al., 2018). P. citri possess the strongest antioxidant among carotenoids, astaxanthin (Metcalf and Newell, 1962; Atarashi et al., 2017). Therefore, it is likely that female phytoseiid mites normally (or selectively) deliver antioxidants from food components to their eggs.
Neoseiulus californicus eggs were vulnerable to UVB radiation during the first third of egg development and resistant to UVB at later stages. In a previous study, the same pattern of vulnerability was observed in T. urticae: eggs 24–48 h after oviposition were most vulnerable to UVB radiation (Murata and Osakabe, 2014), the vulnerable periods corresponded with periods of embryonic development (Dearden et al., 2002). This suggests that N. californicus eggs were commonly vulnerable to UVB radiation at their embryonic development periods, and our findings in this study show that maternal pollen diet mitigated UVB damage during the vulnerable periods of eggs. We also found efficient photoreactivation in N. californicus, line with Nakai et al. (2018) (but see Koveos et al., 2017). Interestingly, maternal diet source also affected the photoreactivation efficiency of eggs. When no photoreactivation occurred after UVB irradiation, the peach pollen diet increased egg hatchability. By contrast, when photoreactivation occurred, the tea pollen diet increased hatchability, which suggests an improvement of photoreactivation function with ROS scavenging by antioxidants. The tea pollen diet also significantly increased egg hatchability in the absence of photoreactivation, and the survival of adult females under lower UVB irradiance. Therefore, the ROS scavenging function may be more effective than the shielding function for protecting phytoseiid mites from UVB damage.
Zhang G. H. et al. (2014) showed that antioxidant enzymes of Neoseiulus cucumeris (Oudemans) responded to oxidative thermal stress, but the effects were not always sufficient to suppress lipid peroxidation. Other studies have confirmed the responses of antioxidant enzyme systems against oxidative stress, thermal stress and UVB radiation in P. citri, but the effects on lipid peroxidation were not clear (Yang et al., 2010; Feng et al., 2015). P. citri are frequently exposed to solar UVB radiation and radiant heat owing to their characteristic distribution to the upper surfaces of host plant leaves (Jones and Parrella, 1984; Fukaya et al., 2013). Atarashi et al. (2017) demonstrated that astaxanthin and its esters suppress lipid peroxide accumulation caused by high temperatures and UVB radiation. Spider mites possess carotenoid biosynthetic genes (Altincicek et al., 2012; Bryon et al., 2013, 2017), as they take in plant compounds including carotenoids and transfer them to eggs (Bosse and Veerman, 1996; Kawaguchi et al., 2016). The protective effects of antioxidant compounds from pollen as well as astaxanthin metabolized from β-carotene in spider mites on phytoseiid mites (Veerman, 1970, 1974) provide evidence for a close relationship between the solar adaptations of the predacious mites and the host plants.
The vulnerability of N. californicus eggs to UVB radiation evaluated after a single acute UVB irradiation was higher than that of spider mite eggs (Tachi and Osakabe, 2012). Under intermittent UVB irradiation used for spider mite control in strawberry greenhouses (Tanaka et al., 2016), the egg hatchability of N. californicus is higher than that of T. urticae, whereas the residual effects of UVB irradiation decrease the survival of hatched larvae (Nakai et al., 2018). Our findings show that providing antioxidants improves the robustness of phytoseiid mites for use as spider mite controls, contributing to the development of integrated pest management.
Spider mites are protected from UVB radiation through the shielding effects of UVB-absorbing compounds in plant leaves and antioxidants such as carotenoids that are self-produced or obtained from plants (Sakai and Osakabe, 2010; Atarashi et al., 2017). Phytoseiid mites obtain antioxidants via prey mites (Nakai et al., 2018) and pollen diets. Therefore, the antioxidant network contributes to solar adaptation and survival strategies in plant-dwelling mite communities.
MO and NH planned the study described herein and wrote manuscript. MO performed the statistical analyses. NS performed bioassay and MK identified components and performed chemical and physical assays. NS and MK wrote the first partial drafts of manuscript. All authors read and approved the final draft for submission.
This study was partially supported by JSPS KAKENHI (a Grant-in-Aid for Scientific Research from the Ministry of Education, Culture, Sports, Science and Technology of Japan) Grant Number 26292029 and by Council for Science, Technology and Innovation (CSTI), Cross-ministerial Strategic Innovation Promotion Program (SIP), Technologies for creating next-generation agriculture, forestry and fisheries (funding agency: Bio-oriented Technology Research Advancement Institution, NARO) to MO.
The authors declare that the research was conducted in the absence of any commercial or financial relationships that could be construed as a potential conflict of interest.
We thank Mr. Terunori Sasawaki and Mr. Masao Kaneko (Nagano Fruit Tree Experiment Station) for their help to collect peach buds for the preparation of pollens used in this study. We also appreciate participants in Panasonic Co. for providing and setting UVB lamp.
The Supplementary Material for this article can be found online at: https://www.frontiersin.org/articles/10.3389/fevo.2018.00133/full#supplementary-material
Aartsma, Y., Bianchi, F. J. J. A., van der Werf, W., Poelman, E. H., and Dicke, M. (2017). Herbivore-induced plant volatiles and tritrophic interactions across spatial scales. New Phytol. 216, 1054–1063. doi: 10.1111/nph.14475
Addison, J. A., Hardman, J. M., and Walde, S. J. (2000). Pollen availability for predaceous mites on apple: spatial and temporal heterogeneity. Exp. Appl. Acarol. 24, 1–18. doi: 10.1023/A:1006329819059
Altincicek, B., Kovacs, J. L., and Geratdo, N. M. (2012). Horizontally transferred fungal carotenoid genes in the two-spotted spider mite Tetranychus urticae. Biol. Lett. 8, 253–257. doi: 10.1098/rsbl.2011.0704
Asbeck, F. (1955). Fluoreszierender blütenstaub. Naturwissenschaften 42, 632–632. doi: 10.1007/BF00621726
Atarashi, M., Manabe, Y., Kishimoto, H., Sugawara, T., and Osakabe, M. (2017). Antioxidant protection by astaxanthin in the citrus red mite (Acari: Tetranychidae). Environ. Entomol. 46, 1143–1150. doi: 10.1093/ee/nvx121
Benjamini, Y., and Hochberg, Y. (1995). Controlling the false discovery rate: a practical and powerful approach to multiple testing. J. R. Statist. Soc. B 57, 289–300.
Berger, F. (1934). Das verhalten der heufieber-erregenden pollen in filtrien ultravioleten licht. Beitr. Biol. Pflanzen. 22, 1–12.
Bokern, M., Witte, L., Wray, V., Nimitz, M., and Meurer-Grimes, B. (1995). Trisubstituted hydroxycinnamic acid spermidines from Quercus dentata pollen. Phytochemistry 39, 1371–1375. doi: 10.1016/0031-9422(95)00151-V
Bosse, T. C., and Veerman, A. (1996). Involvement of vitamin A in the photoperiodic induction of diapause in the mite Tetranychus urticae is demonstrated by rearing an albino mutant on a semi-synthetic diet with and without β-carotene or vitamin A. Physiol. Entomol. 21, 188–192. doi: 10.1111/j.1365-3032.1996.tb00854.x
Bothwell, M. L., Sherbot, D. M., and Pollock, C. M. (1994). Ecosystem response to solar ultraviolet-B radiation: influence of trophic-level interactions. Science 265, 97–100. doi: 10.1126/science.265.5168.97
Bryon, A., Kurlovs, A. H., Dermauw, W., Greenhalgh, R., Riga, M., Grbić, M., et al. (2017). Disruption of a horizontally transferred phytoene desaturase abolishes carotenoid accumulation and diapause in Tetranychus urticae. Proc. Natl. Acad. Sci. U.S.A. 114, E5871–E5880. doi: 10.1073/pnas.1706865114
Bryon, A., Wybouw, N., Dermauw, W., Tirry, L., and Van Leeuwen, T. (2013). Genome wide gene-expression analysis of facultative reproductive diapause in the two-spotted spider mite Tetranychus uritcae. BMC Genomics 14:815. doi: 10.1186/1471-2164-14-815
Cadet, J., Douki, T., and Ravanat, J.-L. (2015). Oxidatively generated damage to cellular DNA by UVB and UVA radiation. Photochem. Photobiol. 91, 140–155. doi: 10.1111/php.12368
Camera, E., Mastrofrancesco, A., Fabbri, C., Daubrawa, F., Picardo, M., Sies, H., et al. (2009). Astaxanthin, canthaxanthin and β-carotene differently affect UVA-induced oxidative damage and expression of oxidative stress-responsive enzymes. Exp. Dermatol. 18, 222–231. doi: 10.1111/j.1600-0625.2008.00790.x
Castagnoli, M., and Simoni, S. (1999). Effect of long-term feeding history on functional and numerical response of Neoseiulus californicus (Acari: Phytoseiidae). Exp. Appl. Acarol. 23, 217–234. doi: 10.1023/A:1006066930638
Clé, C., Hill, L. M., Niggeweg, R., Martin, C. R., Guisez, Y., Prinsen, E., et al. (2008). Modulation of chlorogenic acid biosynthesis in Solanum lycopersicum; consequences for phenolic accumulation and UV-tolerance. Phytochemistry 69, 2149–2156. doi: 10.1016/j.phytochem.2008.04.024
Coohill, T. P., and Sagripanti, J.-L. (2009). Bacterial inactivation by solar ultraviolet radiation compared with sensitivity to 254 nm radiation. Photochem. Photobiol. 85, 1043–1052. doi: 10.1111/j.1751-1097.2009.00586.x
Croft, B. A., Monetti, L. N., and Pratt, P. D. (1998). Comparative life histories and predation types: are Neoseiulus californicus and N. fallacis (Acari: Phytoseiidae) similar Type II selective predators of spider mites? Environ. Entomol. 27, 531–538. doi: 10.1093/ee/27.3.531
Day, T. A., and Demchik, S. M. (1996). Influence of enhanced UV-B radiation on biomass allocation and pigment concentrations in leaves and reproductive structures of greenhouse-grown Brassica rapa. Vegetatio 127, 109–116. doi: 10.1007/BF00044635
Dean, J. C., Kusaka, R., Walsh, P. S., Allais, F., and Zwier, T. S. (2014). Plant sunscreens in the UV-B: ultraviolet spectroscopy of jet-cooled sinapoyl malate, sinapic acid, and sinapate ester derivatives. J. Am. Chem. Soc. 136, 14780–14795. doi: 10.1021/ja5059026
Dearden, P. K., Donly, C., and Grbić, M. (2002). Expression of pair-rule gene homologues in a chelicerate: early patterning of two-spotted spider mite Tetranychus urticae. Development 129, 5461–5472. doi: 10.1242/dev.00099
Dicke, M., and Baldwin, I. T. (2010). The evolutionary context for herbivore-induced plant volatiles: beyond the ‘cry for help.’ Trends Plant Sci. 15, 167–175. doi: 10.1016/j.tplants.2009.12.002
Donnelly, S., and Verkuilen, J. (2017). Empirical logit analysis is not logistic regression. J. Mem. Lang. 94, 28–42. doi: 10.1016/j.jml.2016.10.005
Dunlap, W. C., and Shick, J. M. (1998) Ultraviolet radiation-absorbing mycosporine-like amino acids in coral reef organisms: a biochemical environmental respective. J. Phycol. 34, 418–430. doi: 10.1046/j.1529-8817.1998.340418.x
Duso, C., Malagnini, V., Paganelli, A., Aldegheri, L., Bottini, M., and Otto, S. (2004). Pollen availability and abundance of predatory phytoseiid mites on natural and secondary hedgerows. BioControl 49, 397–415. doi: 10.1023/B:BICO.0000034601.95956.89
Feng, H., An, L., Tan, L., Hou, Z., and Wang, X. (2000). Effect of enhanced ultraviolet-B radiation on pollen germination and tube growth of 19 taxa in vitro. Environ. Exp. Bot. 43, 45–53. doi: 10.1016/S0098-8472(99)00042-8
Feng, Y. C., Liao, C. Y., Xia, W. K., Jiang, X. Z., Shang, F., Yuan, G. R., et al. (2015). Regulation of three isoforms of SOD gene by environmental stresses in citrus red mite, Panonychus citri. Exp. Appl. Acarol. 67, 49–63. doi: 10.1007/s10493-015-9930-3
Foott, W. H. (1963). Competition between two species of mites. II. Factors influencing intensity. Can. Entomol. 95, 45–57.
Fraser, W. T., Lomax, B. H., Jardine, P. E., Gosling, W. D., and Sephton, M. A. (2014). Pollen and spores as a passive monitor of ultraviolet radiation. Front. Ecol. Evol. 2:12. doi: 10.3389/fevo.2014.00012
Fukaya, M., Uesugi, R., Ohashi, H., Sakai, Y., Sudo, M., Kasai, A., et al. (2013). Tolerance to solar ultraviolet-B radiation in the citrus red mite, and upper surface user of host plant leaves. Photochem. Photobiol. 89, 424–431. doi: 10.1111/php.12001
Girotti, A. W. (1998). Lipid hydroperoxide generation, turnover, and effector action in biological systems. J. Lipid Res. 39, 1529–1542.
Grbić, M., Van Leeuwen, T., Clark, R. M., Rombauts, S., Rouzé, P., Grbić, V., et al. (2011). The genome of Tetranychus urticae reveals herbivorous pest adaptations. Nature 479, 487–492. doi: 10.1038/nature10640
Greenberg, B. M., Gaba, V., Canaani, O., Malkin, S., Mattoo, A. K., and Edelman, M. (1989). Separate photosensitizers mediate degradation of the 32-kDa photosystem II reaction center protein in the visible and UV spectral regions. Proc. Natl Acad. Sci. U.S.A. 86, 6617–6620. doi: 10.1073/pnas.86.17.6617
Hama, S., Uenishi, S., Yamada, A., Ohgita, T., Tsuchiya, H., Yamashita, E., et al. (2012). Scavenging of hydroxyl radiacals in aqueous solution by astaxanthin encapsulated in liposomes. Biol. Pharm. Bull. 35, 2238–2242. doi: 10.1248/bpb.b12-00715
Harborne, J. B., and Corner, J. J. (1961). Plant polyphenols. 4. Hydroxycinnamic acid-sugar derivatives. Biochem. J. 81, 242–250.
Izaguirre, M. M., Mazza, C. A., Svatoš, A., Baldwin, I. T., and Ballaré, C. L. (2007). Solar ultraviolet-B radiation and insect herbivory trigger partially overlapping phenolic responses in Nicotiana attenuata and Nicotiana longiflora. Ann. Bot. 99, 103–109. doi: 10.1093/aob/mcl226
Jansen, M. A. K., Gaba, V., and Greenberg, B. M. (1998). Higher plants and UV-B radiation: balancing damage, repair and acclimation. Trends Plant Sci. 3, 131–135. doi: 10.1016/S1360-1385(98)01215-1
Jones, V. P., and Parrella, M. P. (1984). Intratree regression sampling plans for the citrus red mite (Acari: Tetranychidae) on lemons in southern California. J. Econ. Entomol. 77, 810–813. doi: 10.1093/jee/77.3.810
Kawaguchi, S., Manabe, Y., Sugawara, T., and Osakabe, M. (2016). Imaginal feeding for progression of diapause phenotype in the two-spotted spider mite (Acari: Tetranychidae). Environ. Entomol. 45, 1568–1573. doi: 10.1093/ee/nvw127
Khan, M. I., Fatma, M., Per, T. S., Anjum, N. A., and Khan, N. A. (2015). Salicylic acid-induced abiotic stress tolerance and underlying mechanisms in plants. Front. Plant. Sci. 6:462. doi: 10.3389/fpls.2015.00462
Kielbassa, C., Roza, L., and Epe, B. (1997). Wavelength dependence of oxidative DNA damage induced by UV and visible light. Carcinogenesis 18, 811–816. doi: 10.1093/carcin/18.4.811
Koller, M., Knapp, M., and Schausberger, P. (2007). Direct and indirect adverse effects of tomato on the predatory mite Neoseiulus californicus feeding on the spider mite Tetranychus evansi. Entomol. Exp. Appl. 125, 297–305. doi: 10.1111/j.1570-7458.2007.00625.x
Koti, S., Reddy, K. R., Reddy, V. R., Kakani, V. G., and Zhao, D. (2005). Interactive effects of carbon dioxide, temperature, and ultraviolet-B radiation on soybean (Glycine max L.) flower and pollen morphology, pollen production, germination, and tube lengths. J. Exp. Bot. 56, 725–736. doi: 10.1093/jxb/eri044
Koveos, D. S., Suzuki, T., Terzidou, A., Kokkari, A., Floros, G., Damos, P., et al. (2017). Egg hatching response to a range of ultraviolet-B (UV-B) radiation doses for four predatory mites and the herbivorous spider mite Tetranychus urticae. Exp. Appl. Acarol. 71, 35–46. doi: 10.1007/s10493-016-0102-x
Lavola, A., Julkunen-Tiitto, R., Roininen, H., and Aphalo, P. (1998). Host-plant preference of an insect herbivore mediated by UV-B and CO2 in relation to plant secondary metabolites. Biochem. Syst. Ecol. 26, 1–12.
Logemann, E., Tavernaro, A., Schulz, W., Somssich, I. E., and Hahlbrock, K. (2000). UV light selectively coinduces supply pathways from primary metabolism and flavonoid secondary product formation in parsley. Proc. Natl. Acad. Sci. U.S.A. 97, 1903–1907. doi: 10.1073/pnas.97.4.1903
Luh, H. K., and Croft, B. A. (2001). Quantitative classification of life-style types in predaceous phytoseiid mites. Exp. Appl. Acarol. 25, 403–424. doi: 10.1023/A:1017988925742
Matsuzaki, T., and Hara, Y. (1985). Antioxidative activity of tea leaf catechins. Nippon Nog. Kag. Kaish. 59, 129–134. doi: 10.1271/nogeikagaku1924.59.129
McMurtry, J. A., and Croft, B. A. (1997). Life-styles of phytoseiid mites and their roles in biological control. Annu. Rev. Entomol. 42, 291–321. doi: 10.1146/annurev.ento.42.1.291
Metcalf, R. L., and Newell, I. M. (1962). Investigation of the biochronies of mites. Ann. Entomol. Soc. Am. 55, 350–353.
Miki, W. (1991). Biological functions and activities of animal carotenoids. Pure Appl. Chem. 63, 141–146.
Miyamoto, S., Martinez, G. R., Medeiros, M. H., and Mascio, P. (2014). Singlet molecular oxygen generated by biological hydroperoxides. J. Photochem. Photobiol. B Biol. 139, 24–33. doi: 10.1016/j.jphotobiol.2014.03.028
Morimoto, K., Furuichi, H., Yano, S., and Osakabe, M. (2006). Web mediated interspecific competition among spider mites. J. Econ. Entomol. 99, 678–684. doi: 10.1603/0022-0493-99.3.678
Morita, M., Naito, Y., Yoshikawa, T., and Niki, E. (2016). Rapid assessment of singlet oxygen-induced plasma lipid oxidation and its inhibition by antioxidants with diphenyl-1-pyrenylphosphine (DPPP). Anal. Bioanal. Chem. 408, 265–270. doi: 10.1007/s00216-015-9102-7
Murata, Y., and Osakabe, M. (2014). Factors affecting photoreactivation in UVB-irradiated herbivorous spider mite (Tetranychus urticae). Exp. Appl. Acarol. 63, 253–265. doi: 10.1007/s10493-014-9773-3
Murata, Y., and Osakabe, M. (2017). Photo-enzymatic repair of UVB-induced DNA damage in the two-spotted spider mite Tetranychus urticae. Exp. Appl. Acarol. 71, 15–34. doi: 10.1007/s10493-016-0100-z
Nakai, K., Murata, Y., and Osakabe, M. (2018). Effects of low temperature on spider mite control by intermittent ultraviolet-B irradiation for practical use in greenhouse straw berries. Environ. Entomol. 47, 140–147. doi: 10.1093/ee/nvx179
Nakazawa, M. (2017). Package ‘fmsb’ ver. 0.6.1. Functions for Medical Statistics Book with some Demographic Data. Available online at: https://cran.r-project.org/web/packages/fmsb/index.html.
Nanjo, F., Goto, K., Seto, R., Suzuki, M., Sakai, M., and Hara, Y. (1996). Scavenging effects of tea catechins and their derivatives on 1,1-diphenyl-2-picrylhydrazyl radical. Free Radic. Biol. Med. 21, 895–902.
Nascimento, L. B. D. S., Leal-Costa, M. V., Menezes, E. A., Lopes, V. R., Muzitano, M. F., and Tavares, E. S. (2015). Ultraviolet-B radiation effects on phenolic profile and flavonoid content of Kalanchoe pinnata. J. Photochem. Photobiol. B Biol. 148, 73–81. doi: 10.1016/j.jphotobiol.2015.03.011
Ohtsuka, K., and Osakabe, M. (2009). Deleterious effects of UV-B radiation on herbivorous spider mites: they can avoid it by remaining on lower leaf surfaces. Environ. Entomol. 38, 920–929. doi: 10.1603/022.038.0346
Onzo, A., Sabelis, M. W., and Hanna, R. (2010). Effects of ultraviolet radiation on predatory mites and the role of refuges in plant structures. Environ. Entomol. 39, 695–701. doi: 10.1603/EN09206
Opitz, S. E. W., and Müller, C. (2009) Plant chemistry and insect sequestration. Chemoecology 19, 117–154. doi: 10.1007/s00049-009-0018-6
Osakabe, M., Hongo, K., Funayama, K., and Osumi, S. (2006). Amensalism via webs causes unidirectional shifts of dominance in spider mite communities. Oecologia 150, 496–505. doi: 10.1007/s00442-006-0560-7
Pöhlker, C., Huffman, J. A., Förster, J.-D., and Pöschl, U. (2013). Autofluorescence of atmospheric bioaerosols: spectral fingerprints and taxonomic trends of pollen. Atmos. Meas. Tech. 6, 3369–3392. doi: 10.5194/amt-6-3369-2013
Price, P. W., Bouton, C. E., Gross, P., McPheron, B. A., Thompson, J. N., and Weis, A. E. (1980). Interactions among three trophic levels: influence of plants on interactions between insect herbivores and natural enemies. Annu. Rev. Ecol. Syst. 11, 41–65. doi: 10.1146/annurev.es.11.110180.000353
R Core Team (2014). R: A Language and Environment for Statistical Computing. R Foundation for Statistical Computing, Vienna. Available online at: http://www.R-project.org/.
Rousseaux, M. C., Julkunen-Tiitto, R., Searles, P. S., Scopel, A. L., Aphalo, P. J., and Ballaré, C. L. (2004). Solar UV-B radiation affects leaf quality and insect herbivory in the southern beech tree Nothofagus antarctica. Oecologia 138, 505–512. doi: 10.1007/s00442-003-1471-5
Rozema, J., Broekman, R. A., Blokker, P., Meijkamp, B. B., de Bakker, N., van de Staaij, J., et al. (2001). UV-B absorbance and UV-B absorbing compounds (para-coumaric acid) in pollen and sporopollenin: the perspective to track historic UV-B levels. J. Photochem. Photobiol. B. 62, 108–117. doi: 10.1016/S1011-1344(01)00155-5
Sakai, Y., and Osakabe, M. (2010). Spectrum-specific damage and solar ultraviolet radiation avoidance in the two-spotted spider mite. Photochem. Photobiol. 86, 925–932. doi: 10.1111/j.1751-1097.2010.00739.x
Sakai, Y., Sudo, M., and Osakabe, M. (2012). Seasonal changes in the deleterious effects of solar ultraviolet-B radiation on eggs of the twospotted spider mite, Tetranychus urticae (Acari: Tetranychidae). Appl. Entomol. Zool. 47, 67–73. doi: 10.1007/s13355-011-0090-6
Sano, M., Tabata, M., Suzuki, M., Degawa, M., Miyase, T., and Maeda-Yamamoto, M. (2001). Simultaneous determination of twelve tea catechins by high-performance liquid chromatography with electrochemical detection. Analyst 126, 816–820. doi: 10.1039/b102541b
Santos, A., Almeida, J. M., Santos, I., and Salema, R. (1998). Biochemical and ultrastructural changes in pollen of Zea mays L. grown under enhanced UV-B radiation. Ann. Bot. 82, 641–645. doi: 10.1006/anbo.1998.0724
Sinha, R. P., and Häder, D. P. (2002). UV-induced DNA damage and repair: a review. Photochem. Photobiol. Sci. 1, 225–236. doi: 10.1039/B201230H
Stevens, S., Valderas, J. M., Doran, T., Perera, R., and Kontopantelis, E. (2016). Analysing indicators of performance, satisfaction, or safety using empirical logit transformation. BMJ 352:i1114. doi: 10.1136/bmj.i1114
Stewart, M. S., Cameron, G. S., and Pence, B. C. (1996). Antioxidant nutrients protect against UVB-induced oxidative damage to DNA of mouse keratinocytes in culture. J. Invest. Dermatol. 106, 1086–1089.
Stošić, D., Gorunović, M., Skaltsounis, A.-L., Tillequin, F., and Koch, M. (1988). New flavonoid glycosides from Paeonia tenuifolia L. Helv. Chim. Acta 71, 348–353.
Strack, D., Eilert, U., Wray, V., Wolff, J., and Jaggy, H. (1990). Tricoumaroylspermidine in flowers of rosaceae. Phytochemistry 29, 2893–2896. doi: 10.1016/0031-9422(90)87099-G
Sudo, M., and Osakabe, M. (2011) Do plant mites commonly prefer the underside of leaves? Exp. Appl. Acarol. 55, 25–38. doi: 10.1007/s10493-011-9454-4
Suzuki, H., Yasuda, K., Ohashi, K., Takahashi, H., Fukaya, M., Yano, S., et al. (2011). Kanzawa spider mites acquire enemy-free space on a detrimental host plant, oleander. Entomol. Exp. Appl. 138, 212–222. doi: 10.1111/j.1570-7458.2010.01092.x
Suzuki, T., Yoshioka, Y., Tsarsitalidou, O., Ntalia, V., Ohno, S., Ohyama, K., et al. (2014). A LED-based UV-B irradiation system for tiny organisms: system description and demonstration experiment to determine the hatchability of eggs from four Tetranychus spider mite species from Okinawa. J. Insect Physiol. 62, 1–10. doi: 10.1016/j.jinsphys.2014.01.005
Tachi, F., and Osakabe, M. (2012). Vulnerability and behavioral response to ultraviolet radiation in the components of a foliar mite prey-predator system. Naturwissenschaften 99, 1031–1038. doi: 10.1007/s00114-012-0984-3
Tachi, F., and Osakabe, M. (2014). Spectrum-specific UV egg damage and dispersal responses in the phytoseiid predatory mite, Neoseiulus californicus (Acari: Phytoseiidae). Environ. Entomol. 43, 787–794. doi: 10.1603/EN13336
Tanaka, M., Yase, J., Aoki, S., Sakurai, T., Kanto, T., and Osakabe, M. (2016). Physical control of spider mites using ultraviolet-B with light reflection sheets in greenhouse strawberries. J. Econ. Entomol. 109, 1758–1765. doi: 10.1093/jee/tow096
Tegelberg, R., Julkunen-Tiitto, R., and Aphalo, P. J. (2004). Red: far-red light ratio and UV-B radiation: their effects on leaf phenolics and growth of silver birch seedlings. Plant Cell Environ. 27, 1005–1013. doi: 10.1111/j.1365-3040.2004.01205.x
Toyoshima, S., and Hinomoto, N. (2004). Intraspecific variation of reproductive characteristics of Amblyseius californicus (McGregor) (Acari: Phytoseiidae). Appl. Entomol. Zool. 39, 351–355. doi: 10.1303/aez.2004.351
Veerman, A. (1970). The pigments of Tetranychus cinnabarinus Boisd (Acari: Tetranychidae). Comp. Biochem. Physiol. 36, 749–763.
Veerman, A. (1974). Carotenoid metabolism in Tetranychus urticae Koch (Acari: Tetranychidae). Comp. Biochem. Physiol. B Biochem. Mol. Biol. 47, 101–116.
Veerman, A. (1980). Functional involvement of carotenoids in photoperiodic induction of diapause in the spider mite, Tetranychus urticae. Physiol. Entomol. 5, 291–300.
Veerman, A., and Helle, W. (1978). Evidence for the functional involvement of carotenoids in the photoperiodic reaction of spider mite. Nature 275:234. doi: 10.1038/275234a0
Vidović, M., Morina, F., Milić, S., Zechmann, B., Albert, A., Winkler, J. B., et al. (2015). Ultraviolet-B component of sunlight stimulates photosynthesis and flavonoid accumulation in variegated Plectranthus coleoides leaves depending on background light. Plant Cell Environ. 38, 968–979. doi: 10.1111/pce.12471
Villanueva, R. T., and Childers, C. C. (2004). Phytoseiidae increase with pollen deposition on citrus leaves. Fla. Entomol. 87, 609–611. doi: 10.1653/0015-4040(2004)087[0609:PIWPDO]2.0.CO;2
Wang, R. J., Stoien, J. D., and Landa, F. (1974). Lethal effect of near-ultraviolet irradiation on mammalian cells in culture. Nature 247, 43–45. doi: 10.1038/247043a0
Wang, S., Xie, B., Yin, L., Duan, L., Li, Z., Eneji, A. E., et al. (2010) Increased UV-B radiation affects the viability, reactive oxygen species accumulation antioxidant enzyme activities in maize (Zea mays L.) pollen. Photochem. Photobiol. 86, 110–116. doi: 10.1111/j.1751-1097.2009.00635.x
Yang, L.-H., Huang, H., and Wang, J.-J. (2010). Antioxidant responses of citrus red mite, Panonychus citri (McGregor) (Acari: Tetranychidae), exposed to thermal stress. J. Insect Physiol. 56, 1871–1876. doi: 10.1016/j.jinsphys.2010.08.006
Yang, Z., Dong, F., Baldermann, S., Murata, A., Tu, Y., Asai, T., et al. (2012). Isolation and identification of spermidine derivatives in tea (Camellia sinensis) flowers and their distribution in floral organs. J. Sci. Food Agric. 92, 2128–2132. doi: 10.1002/jsfa.5596
Yang, Z., Tu, Y., Baldermann, S., Dong, F., Xuc, Y., and Watanabe, N. (2009). Isolation and identification of compounds from the ethanolic extract of flowers of the tea (Camellia sinensis) plant and their contribution to the antioxidant capacity. LWT-Food Sci. Technol. 42, 1439–1443. doi: 10.1016/j.lwt.2009.03.017
Zamble, A., Sahpaz, S., Hennebelle, T., Carato, P., and Bailleul, F. (2006) N1,N5,N10-Tris(4-hydroxycinnamoyl)spermidines from Microdesmis keayana roots. Chem. Biodivers. 3, 982–989. doi: 10.1002/cbdv.200690107
Zhang, C., Yang, Y. P., and Duan, Y. W. (2014). Pollen sensitivity to ultraviolet-B (UV-B) suggests floral structure evolution in alpine plants. Sci. Rep. 4:4520 doi: 10.1038/srep04520
Zhang, G. H., Liu, H., Wang, J.-J., and Wang, Z.-Y. (2014). Effects of thermal stress on lipid peroxidation and antioxidant enzyme activities of the predatory mite, Neoseiulus cucumeris (Acari: Phytoseiidae). Exp. Appl. Acarol. 64, 73–85. doi: 10.1007/s10493-014-9806-y
Zhang, X., Rosenstein, B. S., Wang, Y., Lebwohl, M., and Wei, H. (1997). Identification of possible reactive oxygen species involved in ultraviolet radiation-induced oxidative DNA damage. Free Radic. Biol. Med. 23, 980–985.
Keywords: UV damage, UVB resistance, catechins, tea, peach, Neoseiulus californicus, Tetranychus urticae, physical control
Citation: Sugioka N, Kawakami M, Hirai N and Osakabe M (2018) A Pollen Diet Confers Ultraviolet-B Resistance in Phytoseiid Mites by Providing Antioxidants. Front. Ecol. Evol. 6:133. doi: 10.3389/fevo.2018.00133
Received: 28 May 2018; Accepted: 17 August 2018;
Published: 07 September 2018.
Edited by:
Carlo Duso, Università degli Studi di Padova, ItalyReviewed by:
Menelaos Stavrinides, Cyprus University of Technology, CyprusCopyright © 2018 Sugioka, Kawakami, Hirai and Osakabe. This is an open-access article distributed under the terms of the Creative Commons Attribution License (CC BY). The use, distribution or reproduction in other forums is permitted, provided the original author(s) and the copyright owner(s) are credited and that the original publication in this journal is cited, in accordance with accepted academic practice. No use, distribution or reproduction is permitted which does not comply with these terms.
*Correspondence: Masahiro Osakabe, bWhvc2FrYUBrYWlzLmt5b3RvLXUuYWMuanA=
Disclaimer: All claims expressed in this article are solely those of the authors and do not necessarily represent those of their affiliated organizations, or those of the publisher, the editors and the reviewers. Any product that may be evaluated in this article or claim that may be made by its manufacturer is not guaranteed or endorsed by the publisher.
Research integrity at Frontiers
Learn more about the work of our research integrity team to safeguard the quality of each article we publish.