- Centre for Ecological Sciences, Indian Institute of Science, Bengaluru, India
The rapid rate of urbanization worldwide and its consequences for affected species and ecosystems urgently warrants research and action. Whether animals are effectively coping from urbanization is hotly debated, especially since interpretations are based on different measures of animal responses. Here, we take a physiology-centric view of animal coping strategies, and set up predictions for distinguishing urban adaptors and exploiters using multiple markers of health. Our study compares rural and urban populations of a tropical lizard species, the Indian rock agama (Psammophilus dorsalis), that lives in a rapidly changing and highly threatened semi-arid ecosystem in Southern India. Contrary to expectations, we did not find any significant differences in body condition, number of ectoparasites, and degree of cell-mediated immune response between lizards from urban and rural areas, despite previously documented differences in aspects of their behavior. However, urban lizards had lower heterophil to lymphocyte ratios, as well as lower testosterone levels compared to rural lizards, which suggest active physiological coping responses. Notably, we find no correlation between the different physiological measures within individuals; strongly reflecting the flexible and independent axes of health responsiveness. Overall, the considerable overlap in responses by urban and rural lizards highlights the importance of using multiple measures of immunity to evaluate health status. These data collectively indicate that P. dorsalis is an urban adaptor, and that the tropical urban environment is not associated with harmful consequences for their health.
Introduction
Urban human populations and urban land expansion are increasing at an alarming rate worldwide (Seto et al., 2012; UNDESA, 2014). Rapid urbanization causes modifications to natural ecosystems and introduces unique challenges for its resident flora and fauna. Despite the variation in rate and form around the world, urbanization is characterized by the replacement of natural, often contiguous, habitats by anthropogenic structures, such as roads and buildings (Sol et al., 2013). Presence of these structures causes the loss, fragmentation, and alteration of natural habitats, in addition to introducing pollution and toxicity in the environment (Kylin et al., 2011; Sol et al., 2013). Moreover, urbanization also impacts the biotic components of the ecosystem, which include changes in the composition and intensity of trophic interactions, such as altered predator communities and predation intensities, as well as the composition, distribution and availability of food resources (Sih et al., 2011; Sol et al., 2013). Finally, the altered configuration of suitable habitats in urban areas can affect local population density and competition dynamics of resident wildlife and exposes them to novel disturbances, including humans, domestic animals, and other commensals (Sorace, 2002; Fischer et al., 2012; Sol et al., 2013). All of these abiotic and biotic changes may be perceived as additional stressors for resident wildlife. Consequently, urbanization is expected to negatively impact wildlife, which is why it is not surprising that there is abundant evidence for loss of species richness and diversity in urban areas (Blair, 1996; Blair and Launer, 1997; Germaine and Wakeling, 2001).
Since urbanization is associated with the introduction of novel stressors, animals that manage to live in urban areas are expected to adjust their physiology in response. Numerous studies have therefore tested the prediction that urban animals experience greater stress, and hence have higher circulating glucocorticoid levels, compared to their counterparts in rural areas. Till date there has been mixed evidence for the urban stress hypothesis, with studies reporting increased, decreased, as well as similar glucocorticoid responses in different taxa in urban areas compared to rural areas (French et al., 2008; Bonier, 2012; Scheun et al., 2015; Polich, 2016; Gabor et al., 2018). Hence there is no evidence for a general pattern of how stress physiology of animals is affected by urbanization. The knowledge gap is even greater for physiological processes other than glucocorticoid responses. For instance, the induction of the glucocorticoid stress response can lead to changes in other steroid hormone levels or immune responsiveness (Sapolsky et al., 2000; Davis et al., 2008). Dealing with urban stressors also requires mobilization and reallocation of nutrients to processes involving inflammation or oxidative stress (Isaksson et al., 2017). Thus, acquiring data on multiple physiological markers beyond the glucocorticoid response is imperative. Such information, which can be assessed with minimally-invasive techniques, is critical to determine how animals are perceiving and responding to the potential stress of anthropogenically-altered landscapes.
The impact of urbanization on wildlife is highly variable and many animals persist in urban areas despite the environmental challenges and potential stressors (Ditchkoff et al., 2006; Sol et al., 2013). Urban residents have been defined as either opportunistic “exploiters” that thrive and depend on anthropogenic resources, or as “adapters” that utilize urban environments with relatively similar effectiveness as undisturbed areas (McKinney, 2002; Shochat et al., 2006). Differences in the behavioral ecology of exploiters and adaptors should also correspond to differences in physiological stress and immune responsiveness. For example, in birds, behaviorally-defined adaptor and exploiter species differ in biomarkers of health and nutrition (Isaksson et al., 2017). We extend this distinction between urban exploiter and adaptor species and propose that physiological biomarkers can, in fact, be used to distinguish between these two types of urban residents. Since exploiters are actually benefiting from the anthropogenic conditions, we expect them to have lower stress levels and better health indices in urban areas compared to in undisturbed or rural areas. Alternatively, adaptors should show at least some signatures of physiological coping strategies in urban environments, such as higher stress levels or reduced immunocompetence. At best, given enough time to habituate, adaptor species could show health indices and immune responses that are comparable to those under natural undisturbed conditions. Hence, by comparing physiological indices of urban and undisturbed populations, it is possible to differentiate between adaptor and exploiter strategies. Information on such physiological responses, coupled with knowledge about behavioral changes, can therefore provide a better understanding of whether and how animals are coping with the challenge of urbanization.
Several studies in birds and some in herpetofauna have investigated the health of animals in urban areas using one or two immune parameters (French et al., 2008; Audet et al., 2016; Lazic et al., 2016; Iglesias-Carrasco et al., 2017; Isaksson et al., 2017; but see French et al., 2017). The immune system in vertebrates is a composite system encompassing innate, humoral, and cell-mediated immunity that collectively maintain the health of an organism (Cheng and Lamont, 1988). Measure of a single aspect of the immune system assumes an interconnected response by every component. However, environmental challenges such as urbanization can result in resource limitation, which can force organisms to strategically invest in different physiological and behavioral processes (Norris and Evans, 2000). Trade-offs in resource allocation will therefore arise for example, when investment in one immune component limits investment in other immune components (Norris and Evans, 2000; Matson et al., 2006). Because of differential investment, different components of animal physiology will respond differently to the impact of urbanization; a powerful approach to evaluate the health of an animal would be to assess a combination of immunity measures (Norris and Evans, 2000; Faivre et al., 2003; Adamo, 2004). These measures should include monitoring techniques, which provide information about the health of the individual at the time of sampling, as well as challenge techniques, which measure the response of the immune system after exposure to a novel antigen (Norris and Evans, 2000). Such a combination of parameters would more effectively assess the health status of animals that survive in a potentially challenging urban environment.
India is a rapidly developing nation with one of the highest projected urban land expansion growth rates (Seto et al., 2011). India is also home to an incredible diversity of herpetofauna, with several species found in modified urban environments. In this study, we examined the impact of urbanization on the health of the Indian rock agama Psammophilus dorsalis, an agamid lizard species that is found in semi-arid areas of southern peninsular India. This species is also found in human-inhabited areas, such as the city of Bengaluru (Karnataka, India). Here, P. dorsalis are restricted to small pockets of suitable areas surrounded by uninhabitable anthropogenic development. Compared to the rural population, lizards from the urban population are habituated to human activity, and they show antipredator behaviors that reflect overall lower reactivity, such as allowing closer approaches before flight (Batabyal et al., 2017). Reduction in antipredator response by urban lizards is not a measure of overall apathy, as these urban lizards are quicker to learn the location of safe refuges than rural lizards (Batabyal, 2017). These behavioral shifts seem to suggest that this species is an urban adaptor. Here we examined whether P. dorsalis are urban adaptors based on signatures of physiological coping to the novel environment. By measuring several health parameters in lizards from in and around the city of Bengaluru (Karnataka, India), we explicitly tested the prediction that urban lizards have some biomarkers of compromised health. For both males and females of this species, we employed monitoring techniques, such as the measurement of body condition, ectoparasite infestation, and H:L ratio, as well as a challenge technique to measure cell-mediated immunity (PHA assay). Body condition is a widely accepted index of general health status and reflects lifetime health conditions (Peig and Green, 2009), whereas phytohaemagglutinin (PHA)-induced swelling response is an effective standardized challenge to measure immunocompetence (Kennedy and Nager, 2006). Ectoparasites are known to negatively impact host fitness by decreasing hematocrit, whereas H:L ratio, calculated using leukocyte profiles, is a measure of innate immunity known to be affected by glucocorticoid levels (Dunlap and Mathies, 1993; Davis et al., 2008; Davis and Maney, 2018). Because the hormone testosterone can be immunosuppressive in many vertebrates (Roberts et al., 2004), we also compared plasma testosterone levels in males from both urban and rural areas. These measures from each individual provide us with a multi-variate assay of immunity and health. If P. dorsalis is indeed an urban adaptor, we predict that the lizards from urban areas will have at least one of these physiological differences: lower body condition, lower cell-mediated immunity, a greater number of ectoparasites, higher H:L ratio, and lower testosterone levels compared to lizards from rural areas. These physiological responses in a persisting urban population would reflect active measures to cope with the challenging urban environment.
Methods
Study Areas
The study was conducted during the breeding season for P. dorsalis (May-September in 2015 and 2016), since most reptiles exhibit peak stress levels during the breeding period (Romero, 2002). We caught adult male and non-gravid female lizards by noosing from urban and rural areas (N = 30 males and 11 females from urban sites, N = 28 males and 14 females from rural sites). The urban study area was located within the city of Bengaluru (centroid 13.0422° N, 77.5940° E) while the rural study area was located on the outskirts of Bengaluru, near the Anthargange forest range in Kolar district of Karnataka, India (centroid 13.1243° N, 78.0346° E). These study areas are ~60 km apart and differ structurally. The urban area consists of semi-developed residential plots, gardens, and construction sites where the lizards are found in small, high-density pockets in the midst of human habitation. Here, they are typically found basking on man-made structures such as cement walls and house ledges. In contrast, the rural area consists of relatively undisturbed boulder fields and rocky outcrops (see Batabyal et al., 2017 for detailed description of the study sites).
Within 4 h of capture, lizards were transported to the laboratory in cloth bags and housed in individual terraria. These terraria (60 × 30 × 25 cm) were equipped with basking lights and rock refuges and were lined with paper towels as substratum. The lizard housing facility allowed for ambient temperature and humidity conditions and natural day/night cycles. Previous research has shown that P. dorsalis engage in normal social interactions, such as courtship and aggressive behavior with context specific displays and color changes, in captivity for up to 9 days (Batabyal and Thaker, 2017). All measurements described below were completed within 3 days, during which lizards were consuming live crickets and grasshoppers provided daily (with ad libitum water) and showed no signs of distress. All lizards were returned to their site of capture after measurements. Capture and experimental protocols have been approved by the Institutional Animal Ethics Committee at the Indian Institute of Science (CAF/Ethics/394/2014).
Body Condition
All lizards were weighed and their snout-vent length (SVL) was measured in order to calculate body condition, using the scaled mass index (SMI) method:
Where Mi and Li are the mass and the SVL of the individual i respectively, Lo is the mean value of SVL for the sample population and b is the slope of the standardized major axis regression of log transformed mass on log transformed SVL (Peig and Green, 2009). The SMI was calculated separately for urban and rural males and females, respectively.
Number of Ectoparasites
Number of ectoparasites present on each lizard was counted manually. Ectoparasites were morphologically distinguishable as either orange-red colored chigger mites (family Trombiculidae) or dark red colored mites, Pterygosoma foliosetis Jack (B. Mallik personal communication; Jack, 1961). The chigger mites were typically found on the neck of the lizards while Pterygosoma mites were found on the folds of the limbs under the scales.
Cell-Mediated Immunity
Cell-mediated immunity of each lizard was measured using the phytohaemagglutinin (PHA) assay. PHA is a lectin protein which, when injected sub-dermally, causes a complex reaction involving local inflammation and proliferation of lymphocytes, as well as mobilization of heterophils and macrophages (Kennedy and Nager, 2006). The resulting swelling from the PHA injection is therefore a response that involves components of both innate and adaptive immunity, wherein the size of swelling is an indication of the strength of the immune response (Kennedy and Nager, 2006). For the assay, the thickness of the palm on the left and right front limbs of lizards was first measured using digital calipers. Following this, one of the palms was injected with 50 mg of PHA (Sigma, L-8754) dissolved in 10 ml phosphate buffer saline (PBS) such that the total volume injected was 3 μl of this solution per gram of lizard mass (Belliure et al., 2004). The other limb was injected with same volume of PBS. The position of PHA injection in the left or right palm was randomized. Lizards were released in their individual terraria after injections and the thickness of swelling was measured 18 h later. This time-line was determined after a pilot study where maximum swelling in response to PHA injection was found to be at 18 h post injection (N = 5 lizards tested; Supplementary Figure S1). Response to PHA was calculated as the change in thickness of the PHA-injected hand (difference between thickness 18 h after injection and thickness before injection) minus the change in thickness of PBS-injected hand.
H:L Ratio
After measuring the PHA-induced response, a small blood sample (30–60 μl) was obtained from each lizard through its retro-orbital sinus using a heparinized microcapillary tube. Immediately upon collection, a single drop of blood was used to prepare blood smears on microscopic slides. These slides were fixed for 3 min in absolute methanol and then stained with Giemsa stain. Slides were examined under a compound microscope under oil immersion at 100X to determine the heterophil to lymphocyte (H:L) ratio. To achieve this, white blood cells (WBCs) were counted until a total of 100 WBCs were reached. These were segregated into different cell types (basophils, eosinophils, monocytes, heterophils etc.) based on morphological characteristics, and only the number of heterophils and lymphocytes was used to calculate the H:L ratio (Fokidis et al., 2008; Stacy et al., 2011; Campbell, 2015). Each slide was counted twice in random order under blind conditions by the same person (AK) and the mean value of H:L ratio was used.
Hormone Assay
The rest of the blood sample was centrifuged and the plasma was stored at −20°C until hormone assays were conducted. Plasma levels of testosterone for males only were measured using Enzyme-Immuno Assay kits from Arbor Assays (K032-H1), optimized for the species. Because females are smaller than males, we obtained a lower volume of blood for females compared to males and thus did not analyse testosterone levels for females. Each sample was run in duplicate with a plasma volume of 4 μl and a dilution ratio of 1:140. Some of the samples were subsequently re-run at dilution ratios 1:100 and 1:50 in order to detect the lower levels of testosterone that were not detectable at 1:140 dilution ratio. The intra-assay coefficient of variation was 0.06–8.7% and the inter-assay coefficient of variation was 13% (n = 4 kits), based on a single lab standard of testosterone placed in each kit.
Statistical Analysis
Assuming independent axes of responses, we first fitted separate linear models to test whether body condition, cell-mediated immune response, and H:L ratio differed in lizards as a function of site (urban or rural) and sex (male or female). Each of the models had site as the explanatory variable, with sex and an interaction between site and sex as covariates. Since the cell-mediated immune response was found to be highly correlated with body length (SVL), we divided that value by SVL of each individual and used this size-dependent transformed variable for the general linear model. We similarly SVL-corrected the number of ectoparasites per individual and square root transformed that value to meet assumptions of normality. The H:L ratio was log-transformed to meet assumptions of normality. For both H:L ratio and number of ectoparasites, we graphically show the non-transformed values for ease of interpretation. All the models were checked for normality of residuals. Testosterone values of males from the two study areas were compared with a Wilcox test. Testosterone data from one rural male was excluded from this analysis as it was considerably higher (876 ng/ml) than the typical range observed in this species (this study; Batabyal and Thaker, in review), and thus could have been due to measurement error.
We also tested for correlations between each of the health measures within individuals, i.e., body condition, cell-mediated immune response, number of ectoparasites, H:L ratio, and plasma testosterone (for males only). Finally, we performed a principal component analysis (PCA) using three variables (SVL-corrected cell-mediated immune response, SVL-corrected number of Pterygosoma mites, and H:L ratio) to examine overall health differences between sites and sexes. All the data used in this study are provided in Data Sheet 1 (Supplementary Material). All the analysis was performed in R version 3.2.3 (R Development Core Team, 2016).
To ensure that the results of the statistical tests above were not being driven by a few outliers in the data, we also re-ran the statistical analyses after removing outliers in H:L ratio (N = 2) and number of Pterygosoma mites (N = 4), which were two standard deviations from the mean. Results from the conservative data set remained qualitatively unchanged on the exclusion of these data, and therefore we present results from the complete dataset.
Results
We found no significant interaction between site and sex for any of the health parameters, and thus report only the main effect comparisons below.
Body Condition
Consistent with reported sexual dimorphism, males (SVL range 101–150 mm, body mass range 40–100 g) of this species are larger than females (SVL range 77–111 mm, body mass range 16–36 g). We found that males also had significantly greater body condition than females [F(1,79) = 319.11, p < 0.0001, N = 58 males, 25 females, Figure 1A], with no significant difference between urban and rural lizards [F(1,79) = 1.75, p = 0.18, N = 42 rural, 41 urban, Figure 1A].
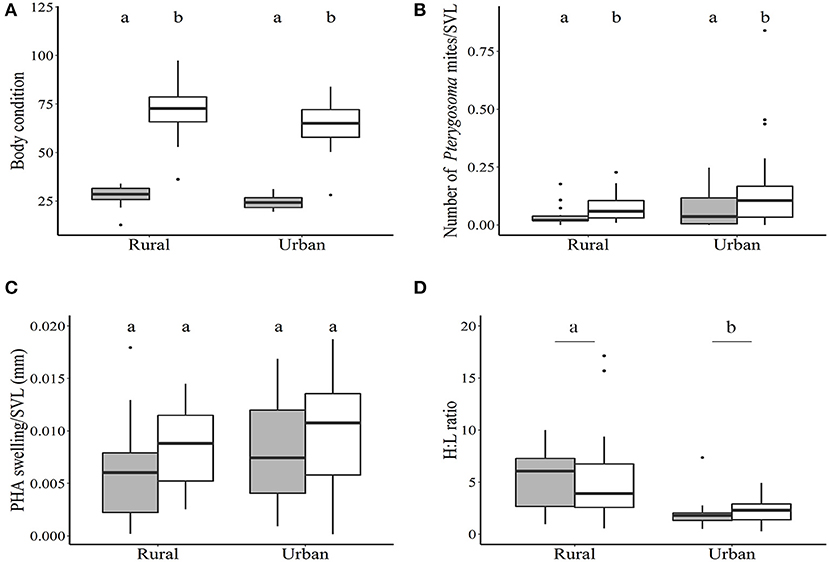
Figure 1. Health parameters of lizards captured from urban and rural areas. (A) body condition, (B) SVL-corrected number of Pterygosoma mites, (C) SVL-corrected PHA swelling (in mm), and (D) Heterophil:Lymphocyte ratio. Box plots display median, quartiles, 5th and 95th percentile and extreme values. White and gray bars represent males and females, respectively. Significant differences at p < 0.05 are denoted by lower case letters above the box plots.
Ectoparasites
Rural lizards were found to have both chigger mites as well as the Pterygosoma mites, while only the Pterygosoma mites were present on the skin of urban lizards (exception: 1 urban male and 1 urban female had both types of mites). Therefore, we used only the number of Pterygosoma mites in our linear model to determine differences between sites and sexes. The number of Pterygosoma mites on a lizard was positively correlated with its SVL (Spearman's rank correlation rho 0.36, p < 0.001). After correcting for SVL, we found that males had significantly higher number of Pterygosoma mites compared to females [F(1,79) = 6.79, p = 0.01, N = 58 males, 25 females, Figure 1B]. There was no significant difference in the number of Pterygosoma mites between urban and rural lizards [F(1,79) = 3.85, p = 0.05, N = 42 rural lizards, 41 urban lizards, Figure 1B]. The number of red chigger mites present on rural lizards was not significantly different between males and females (Wilcoxon rank sum test W = 228, p = 0.39, mean ± 1 se = 136.8 ±22.19, N = 28 males, 14 females).
Cell-Mediated Immunity
Cell-mediated immune response of lizards was positively correlated with SVL (Pearson's correlation coefficient 0.409, p < 0.001), and after correcting for SVL, we found no significant difference between males and females [F(1,79) = 3.607, p = 0.06, N = 58 males, 25 females, Figure 1C], or between lizards from urban and rural sites [F(1,79) = 2.082, p = 0.15, N = 42 rural lizards, 41 urban lizards, Figure 1C].
H:L Ratio
We found that urban lizards had lower H:L ratio compared to rural lizards [F(1,64) = 14.41, p < 0.001, N = 37 urban lizards, 31 rural lizards, Figure 1D]. There was no significant difference in the H:L ratio between males and females [F(1,64) = 0.0001, p = 0.99, N = 48 males, 20 females, Figure 1D].
Testosterone
Urban males had significantly lower testosterone levels (63.39 ± 21.20 ng/ml) compared to rural males (89.58 ± 21.57 ng/ml, Wilcoxon rank sum test W = 384, p = 0.009, N = 25 rural males, 22 urban males, Figure 2).
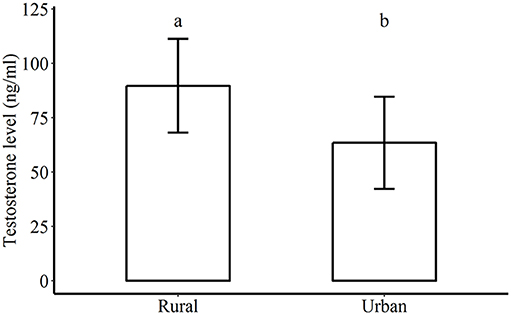
Figure 2. Testosterone levels (mean ± se) of male lizards measured from urban and rural sites. Letters above the bars denote significant difference at p < 0.05.
Correlations and PCA of Health Parameters
Overall, only body condition and SVL-corrected cell-mediated immune response was correlated, such that lizards with high body condition had a greater swelling in response to PHA (Pearson's correlation coefficient 0.273, p = 0.012, Figure 3).
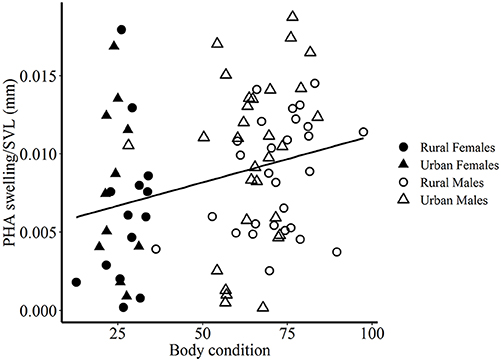
Figure 3. Positive relationship between body condition and size-corrected immune response to PHA (in mm). Circles denote rural lizards and triangles denote urban lizards. Filled and open shapes indicate female and male lizards respectively.
A PCA using three health parameters (H:L ratio, SVL-corrected number of Pterygosoma mites, and SVL-corrected response to PHA) generated three principal components. The first principal component (PC1) accounted for 37.27% of the variation, while the second principal component (PC2) accounted for an additional 34.22% of the variation. Response to PHA (positive loading of 0.742) was explained by PC1 while number of ectoparasites (negative loading of −0.734) and H:L ratio (positive loading of 0.678) loaded on the PC2 axis. When the PC scores were plotted against each other, urban females (N = 10) and rural females (N = 10) showed almost complete overlap, while urban males (N = 27) and rural males (N = 21) showed greater variation in their immune responses (Figure 4).
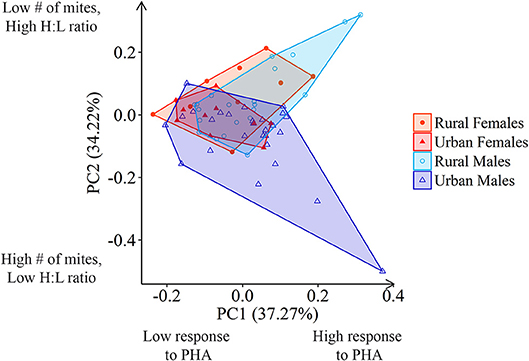
Figure 4. Principle Component Analysis shows almost complete overlap of health parameters (SVL-corrected response to PHA and number of Pterygosoma mites, and H:L ratio) between males and females from urban and rural areas.
Discussion
Urbanization can affect the physiological processes of animals. Both increases as well as decreases in various immune functions have been reported for many taxa living in urban areas, but we still lack sufficient information for lizards (see review in Bradley and Altizer, 2007). Most of our knowledge about the impact of urbanization on physiology has also been generated from studies in temperate regions of the world, with considerable focus on birds, which are highly mobile organisms (e.g., Bonier et al., 2007; Fokidis et al., 2008; French et al., 2008, 2018; Partecke et al., 2010; Giraudeau and McGraw, 2014; Meillère et al., 2015; Audet et al., 2016; Lazic et al., 2016; Iglesias-Carrasco et al., 2017; Isaksson et al., 2017). However, organisms such as herpetofauna are less mobile and by virtue of being range restricted, are likely to be strongly affected by urbanization. In addition, tropics are at the global center of rapid urban expansion while simultaneously being rich in endemic herpetofauna. To our knowledge, this study is the first to examine the impact of urbanization on biomarkers of health in a tropical agamid lizard species. Of the measures of health and physiological responses in this study, we found that H:L ratio and testosterone were significantly lower for lizards in urban areas compared to those from rural areas. In addition, except for a positive association between body condition and cell-mediated immune response, none of the other health parameters were correlated with each other. Taken together, these results emphasize the importance of considering several measures of immunity and suggest that urbanization has altered the innate physiology of the Indian rock agama such that they are effectively coping as urban adaptors.
Urban and rural lizards differed in some, but not all physiological parameters. Urban lizards had a lower H:L ratio compared to rural lizards, a result in agreement from another study in tree lizards (Urosaurus ornatus) in USA (French et al., 2008). Evidence from other experiments with P. dorsalis support the urban stress hypothesis, as urban males have higher corticosterone levels than rural males under various control and social conditions (Batabyal and Thaker, in review). Stress is known to affect leukocyte profiles such that increased glucocorticoid levels lead to an increase in circulating heterophils and a decrease in circulating lymphocytes, thereby increasing the overall H:L ratio (Davis et al., 2008). Another factor that can affect leukocyte numbers is the presence of infection but we have detected no infection by typical blood endoparasites in our study system (Jalal, Thaker and Ishtiaq, unpublished data). We are reluctant to conclude that lower H:L ratio in urban lizards compared to rural lizards indicates lower levels of overall stress in urban areas compared to rural areas (Davis and Maney, 2018). We do think that lower H:L ratio in urban lizards is a strong indication that lizards in the city are actively coping with urban stressors.
Male lizards from urban areas also had lower testosterone levels compared to those from rural areas. These levels are comparable to testosterone levels exhibited by males under control conditions in captivity, in the absence of any social interactions (Batabyal and Thaker, in review). Hence, they reflect baseline levels of the hormone required by these lizards to perform activities such as courting, fighting or maintenance of territories during the breeding season. In urban sites, P. dorsalis reside in small environmentally suitable pockets in high density and frequently encounter mates as well as competitors (Amdekar and Thaker, unpublished data). The “challenge hypothesis” postulates that males present in high density populations should exhibit higher levels of plasma testosterone compared to males in lower density populations because the former are expected to engage in more encounters with females as well as competitive interactions with males (Ball and Wingfield, 1987). Although support for this hypothesis has been found in many species (Hirschenhauser and Oliveira, 2006), we find the opposite pattern in P. dorsalis. Lower levels of testosterone found in males from urban areas may reflect a strategy to avoid engaging in frequent social interactions, such as fighting with other males throughout the breeding season, since such frequent agonistic interactions can have high physiological and physical costs (Wingfield et al., 2001). Sexual signals (color and social behavior) in males of this species are also less elaborate in urban areas compared to rural areas (Batabyal and Thaker, 2017; Batabyal and Thaker, in review). Many studies in lizards have found that sexual signaling is strongly mediated by testosterone (Salvador et al., 1996; Cox et al., 2008) which also supports the pattern we find here; urban lizards have lower testosterone and lower sexual signaling.
Contrary to our expectations, we found no effect of urbanization on PHA-induced immune responses and number of ectoparasites on lizards. Studies with other lizards have found mixed results, with some species showing no difference in cell-mediated immune response between urban and natural habitats, while others reporting reductions in immunocompetence in response to human disturbance (Amo et al., 2006; Iglesias-Carrasco et al., 2016; French et al., 2017). Poor body condition in lizards from disturbed areas compared to those from natural areas has been documented for Anolis antonii and Psammodroumus algirus, but we did not find differences in body condition between urban and rural lizards in our study (Amo et al., 2007; Gallego-Carmona et al., 2016). Species that are able to exploit food resources, despite the altered conditions in urban areas, would match or even fare better than their rural counterparts. Although we currently do not have information about prey resource distribution across the urban-rural gradient, we have found that dietary overlap in P. dorsalis from urban and rural areas is high, thereby showing no restriction to acquiring resources (Balakrishna et al., 2016).
We did not find sex-based differences in any of the health parameters except for the expected difference in body condition due to sexual dimorphism and the number of Pterygosoma mites. In general in vertebrates, males are predicted to suffer from reduced immunocompetence and higher parasite infection during the peak breeding season because of the immunosuppressive effects of the hormone testosterone, as well as through their aggressive and territorial behavior (Zuk and McKean, 1996). Accordingly, studies in many different taxa have reported lower immune response in males as compared to females (Saad, 1989; Moreno et al., 2001; Tschirren et al., 2003). We found no evidence for immunosuppression in males, and the lack of sex differences for most of the health parameters measured in our study may be due to similar exposure and susceptibility of both the sexes to immune challenges in urban and rural environments.
Our results show lack of either positive or negative correlations between the different health parameters at the individual level. This suggests that there is neither a trade-off nor a correlated response between the multiple health parameters, and that these parameters act as independent axes of health. Evidence for trade-offs between different immune components has been found in some birds in other environmental contexts (Ardia, 2007). For example, in house sparrows (Passer domesticus), individuals that have a greater humoral immune response also have a lower cell-mediated immune response (Buchanan et al., 2003). Other studies in birds, but not lizards, have found varying degrees of correlation between other sets of physiological measures such as immune function and basal metabolic rate, or antioxidative measures (e.g., Cohen and McGraw, 2009; Buehler et al., 2012). We also did not find any correlation between testosterone levels in males and any of the immune parameters measured, a result inconsistent with the proposed immunosuppressive nature of testosterone (Roberts et al., 2004). Lack of trade-offs between physiological components is possible if environments have sufficient resources, such that individuals are not forced to trade-off across multiple processes. In P. dorsalis, dietary overlap between urban and rural lizards is high (Balakrishna et al., 2016), suggesting sufficient resources in these environments. Independent health axes would also enable these lizards to independently adjust each of these components in response to a challenge, especially if the environment is such that different selection pressures affect different parts of physiology. Further experiments in free-living vertebrates that manipulate one immune component and examine the impact on other components, or directly manipulate access to resources will shed more light on the trade-offs possible between immune components. Such trade-offs are more likely between immune components that are innate vs. induced, as they signify investment in current health state and the ability to combat future infections.
Urbanization creates rapid and dramatic changes in both abiotic and biotic components of the environment. Often, these changes are thought to have immediate negative consequences for animals that utilize these heavily altered landscapes. However, animals differ in their degree of physiological flexibility depending on their ecology and life-history characteristics, as well as the features of the altered environment. We show here that physiological markers can be used to differentiate between adaptor and exploiter species. They are also an effective tool to assess the health status of animals in disturbed areas, a phenomenon that has gained momentum in informing conservation decisions (Wikelski and Cooke, 2006; Busch and Hayward, 2009). Identifying species as adaptors or exploiters can be the first step toward devising better plans for the management of species in disturbed areas. As opposed to blanket strategies for the conservation of urban residents, information about how animals are coping would allow for more targeted approaches, especially for adaptor species that are exhibiting altered strategies. The natural habitats of P. dorsalis are mostly semi-arid savannas of southern India, which are rapidly being converted into agricultural land or urban areas. Protection of P. dorsalis would therefore have to include the maintenance of populations in urban areas. The multiple physiological markers of health and the recorded shifts in social and antipredator behaviors of P. dorsalis lead us to conclude that this tropical agamid is actively coping in the modified environment. We stress, however, that a single measure of physiological response would have been misleading, as these signatures of coping would have been undetected. Use of multiple physiological tools is essential to generate a more integrative view of whether and how urbanization affects the coping strategies of resident animals.
Author Contributions
MA and MT conceived the study; MA and AK performed the experiments; MA carried out statistical analysis; MA and MT wrote the manuscript. All authors have read and approved the final version of the manuscript.
Funding
This work was supported by funding from DBT-IISc partnership program, DST-FIST, and the MOEF-CC to MT, as well as a British Herpetological Society Student Grant to MA. AK was supported by the Summer Research Fellowship programme of the Jawaharlal Nehru Centre for Advanced Scientific Research. MHRD supported a research fellowship to MA.
Conflict of Interest Statement
The authors declare that the research was conducted in the absence of any commercial or financial relationships that could be construed as a potential conflict of interest.
Acknowledgments
We thank Mihir Joshi and Anuradha Batabyal for help in field and Anuradha Batabyal for advice on statistical analysis.
Supplementary Material
The Supplementary Material for this article can be found online at: https://www.frontiersin.org/articles/10.3389/fevo.2018.00128/full#supplementary-material
References
Adamo, S. A. (2004). How should behavioural ecologists interpret measurements of immunity? Anim. Behav. 68, 1443–1449. doi: 10.1016/j.anbehav.2004.05.005
Amo, L., López, P., and Martín, J. (2006). Nature-based tourism as a form of predation risk affects body condition and health state of Podarcis muralis lizards. Biol. Conserv. 131, 402–409. doi: 10.1016/j.biocon.2006.02.015
Amo, L., López, P., and Martín, J. (2007). Habitat deterioration affects antipredatory behavior, body condition, and parasite load of female Psammodromus algirus lizards. Can. J. Zool. 85, 743–751. doi: 10.1139/Z07-052
Ardia, D. R. (2007). The ability to mount multiple immune responses simultaneously varies across the range of the tree swallow. Ecography 30, 23–30. doi: 10.1111/j.2006.0906-7590.04939.x
Audet, J. N., Ducatez, S., and Lefebvre, L. (2016). The town bird and the country bird: problem solving and immunocompetence vary with urbanization. Behav. Ecol. 27, 637–644. doi: 10.1093/beheco/arv201
Balakrishna, S., Batabyal, A., and Thaker, M. (2016). Dining in the city: dietary shifts in Indian rock agamas across an urban–rural landscape. J. Herpetol. 50, 423–428. doi: 10.1670/14-073
Ball, G. F., and Wingfield, J. C. (1987). Changes in plasma levels of luteinizing hormone and sex steroid hormones in relation to multiple-broodedness and nest-site density in male starlings. Physiol. Zool. 60, 191–199.
Batabyal, A. (2017). Urbanisation and Shifting Phenotypes: Behavioural, Physiological and Cognitive Strategies of the Indian Rock Agama Psammophilus dorsalis. dissertation thesis. Indian Institute of Science, Bengaluru
Batabyal, A., Balakrishna, S., and Thaker, M. (2017). A multivariate approach to understanding shifts in escape strategies of urban lizards. Behav. Ecol. Sociobiol. 71:83. doi: 10.1007/s00265-017-2307-3
Batabyal, A., and Thaker, M. (2017). Signalling with physiological colours: high contrast for courtship but speed for competition. Anim. Behav. 129, 229–236. doi: 10.1016/j.anbehav.2017.05.018
Belliure, J., Smith, L., and Sorci, G. (2004). Effect of testosterone on T cell-mediated immunity in two species of mediterranean lacertid lizards. J. Exp. Zool. 301A, 411–418. doi: 10.1002/jez.a.20068
Blair, R. B. (1996). Land use and avian species diversity along an urban gradient. Ecol. Appl. 6, 506–519. doi: 10.2307/2269387
Blair, R. B., and Launer, A. E. (1997). Butterfly diversity and human land use: species assemblages along an urban gradient. Biol. Conserv. 80, 113–125. doi: 10.1016/S0006-3207(96)00056-0
Bonier, F. (2012). Hormones in the city: endocrine ecology of urban birds. Horm. Behav. 61, 763–772. doi: 10.1016/J.YHBEH.2012.03.016
Bonier, F., Martin, P. R., Sheldon, K. S., Jensen, J. P., Foltz, S. L., and Wingfield, J. C. (2007). Sex-specific consequences of life in the city. Behav. Ecol. 18, 121–129. doi: 10.1093/beheco/arl050
Bradley, C. A., and Altizer, S. (2007). Urbanization and the ecology of wildlife diseases. Trends Ecol. Evol. 22, 95–102. doi: 10.1016/j.tree.2006.11.001
Buchanan, K. L., Evans, M. R., and Goldsmith, A. R. (2003). Testosterone, dominance signalling and immunosuppression in the house sparrow, Passer domesticus. Behav. Ecol. Sociobiol. 55, 50–59. doi: 10.1007/s00265-003-0682-4
Buehler, D. M., Vézina, F., Goymann, W., Schwabl, I., Versteegh, M., Tieleman, B. I., et al. (2012). Independence among physiological traits suggests flexibility in the face of ecological demands on phenotypes. J. Evol. Biol. 25, 1600–1613. doi: 10.1111/j.1420-9101.2012.02543.x
Busch, D. S., and Hayward, L. S. (2009). Stress in a conservation context: a discussion of glucocorticoid actions and how levels change with conservation-relevant variables. Biol. Conserv. 142, 2844–2853. doi: 10.1016/J.BIOCON.2009.08.013
Cheng, S., and Lamont, S. J. (1988). Genetic analysis of immunocomptence measures in a white leghorn chicken line. Poutry Sci. 67, 989–995.
Cohen, A. A., and McGraw, K. J. (2009). No simple measures for antioxidant status in birds: complexity in inter- and intraspecific correlations among circulating antioxidant types. Funct. Ecol. 23, 310–320. doi: 10.1111/j.1365-2435.2009.01540.x
Cox, R. M., Zilberman, V., and John-Alder, H. B. (2008). Testosterone stimulates the expression of a social color signal in Yarrow's spiny lizard, Sceloporus jarrovii. J. Exp. Zool. Part A Ecol. Genet. Physiol. 309, 505–514. doi: 10.1002/jez.481
Davis, A. K., and Maney, D. L. (2018). The use of glucocorticoid hormones or leucocyte profiles to measure stress in vertebrates: what's the difference? Methods Ecol Evol. 9, 1556–1568. doi: 10.1111/2041-210X.13020
Davis, A. K., Maney, D. L., and Maerz, J. C. (2008). The use of leukocyte profiles to measure stress in vertebrates: a review for ecologists. Funct. Ecol. 22, 760–772. doi: 10.1111/j.1365-2435.2008.01467.x
Ditchkoff, S. S., Saalfeld, S. T., and Gibson, C. J. (2006). Animal behavior in urban ecosystems: modifications due to human-induced stress. Urban Ecosyst. 9, 5–12. doi: 10.1007/s11252-006-3262-3
Dunlap, K. D., and Mathies, T. (1993). Effects of nymphal ticks and their interaction with malaria on the physiology of male fence lizards. Copeia 1993, 1045–1048.
Faivre, B., Préault, M., Salvadori, F., Théry, M., Gaillard, M., and Cézilly, F. (2003). Bill colour and immunocompetence in the European blackbird. Anim. Behav. 65, 1–7. doi: 10.1006/anbe.2003.2142
Fischer, J. D., Cleeton, S. H., Lyons, T. P., and Miller, J. R. (2012). Urbanization and the predation paradox: the role of trophic dynamics in structuring vertebrate communities. Bioscience 62, 809–818. doi: 10.1525/bio.2012.62.9.6
Fokidis, H. B., Greiner, E. C., and Deviche, P. (2008). Interspecific variation in avian blood parasites and haematology associated with urbanization in a desert habitat. J. Avian Biol. 39, 300–310. doi: 10.1111/j.2008.0908-8857.04248.x
French, S. S., Fokidis, H. B., and Moore, M. C. (2008). Variation in stress and innate immunity in the tree lizard (Urosaurus ornatus) across an urban–rural gradient. J. Comp. Physiol. B 178, 997–1005. doi: 10.1007/s00360-008-0290-8
French, S. S., Neuman-Lee, L. A., Terletzky, P. A., Kiriazis, N. M., Taylor, E. N., and DeNardo, D. F. (2017). Too much of a good thing? Human disturbance linked to ecotourism has a “dose-dependent” impact on innate immunity and oxidative stress in marine iguanas, Amblyrhynchus cristatus. Biol. Conserv. 210, 37–47. doi: 10.1016/j.biocon.2017.04.006
French, S. S., Webb, A. C., Hudson, S. B., and Virgin, E. E. (2018). Town and country reptiles: a review of reptilian responses to urbanization. Integr. Comp. Biol. 1–19. doi: 10.1093/icb/icy052/5032882
Gabor, C. R., Davis, D. R., Kim, D. S., Zabierek, K. C., and Bendik, N. F. (2018). Urbanization is associated with elevated corticosterone in Jollyville Plateau salamanders. Ecol. Indic. 85, 229–235. doi: 10.1016/J.ECOLIND.2017.10.047
Gallego-Carmona, C. A., Castro-Arango, J. A., and Bernal-Bautista, M. H. (2016). Effect of habitat disturbance on the body condition index of the Colombian endemic lizard Anolis antonii (Squamata: Dactyloidae). South Am. J. Herpetol. 11, 183–187. doi: 10.2994/SAJH-D-16-00020.1
Germaine, S. S., and Wakeling, B. F. (2001). Lizard species distributions and habitat occupation along an urban gradient in Tucson, Arizona, USA. Biol. Conserv. 97, 229–237. doi: 10.1016/S0006-3207(00)00115-4
Giraudeau, M., and McGraw, K. J. (2014). Physiological correlates of urbanization in a desert songbird. Integr. Comp. Biol. 54, 622–632. doi: 10.1093/icb/icu024
Hirschenhauser, K., and Oliveira, R. F. (2006). Social modulation of androgens in male vertebrates: meta-analyses of the challenge hypothesis. Anim. Behav. 71, 265–277. doi: 10.1016/j.anbehav.2005.04.014
Iglesias-Carrasco, M., Head, M. L., and Cabido, C. (2016). Habitat dependent effects of experimental immune challenge on lizard anti-predator responses. Behav. Ecol. Sociobiol. 70, 1931–1939. doi: 10.1007/s00265-016-2199-7
Iglesias-Carrasco, M., Martín, J., and Cabido, C. (2017). Urban habitats can affect body size and body condition but not immune response in amphibians. Urban Ecosyst. 20, 1331–1338. doi: 10.1007/s11252-017-0685-y
Isaksson, C., Andersson, M. N., Nord, A., von Post, M., and Wang, H.-L. (2017). Species-dependent effects of the urban environment on fatty acid composition and oxidative stress in birds. Front. Ecol. Evol. 5:44. doi: 10.3389/fevo.2017.00044
Jack, K. M. (1961). New species of Near Eastern agamid scale-mites (Acarina, Pterygosomidae) with notes on the developmental stages of Geckobia hemidactyli Law 1936. Parasitology 51:241. doi: 10.1017/S0031182000068633
Kennedy, M. W., and Nager, R. G. (2006). The perils and prospects of using phytohaemagglutinin in evolutionary ecology. Trends Ecol. Evol. 21, 653–655. doi: 10.1016/j.tree.2006.09.017
Kylin, H., Bouwman, H., and Evans, S. W. (2011). Evaluating threats to an endangered species by proxy: air pollution as threat to the blue swallow (Hirundo atrocaerulea) in South Africa. Environ. Sci. Pollut. Res. 18, 282–290. doi: 10.1007/s11356-010-0369-0
Lazic, M. M., Carretero, M. A., Zivkovic, U., and Crnobrnja-Isailović, J. (2016). City life has fitness costs: lower body condition and increased parasite intensity in urban common wall lizards Podarcis muralis. Salamandra 53, 10–17.
Matson, K. D., Cohen, A. A., Klasing, K. C., Ricklefs, R. E., and Scheuerlein, A. (2006). No simple answers for ecological immunology: relationships among immune indices at the individual level break down at the species level in waterfowl. Proc. R. Soc. B Biol. Sci. 273, 815–822. doi: 10.1098/rspb.2005.3376
McKinney, M. L. (2002). Urbanization, biodiversity, and conservation: The impacts of urbanization on native species are poorly studied, but educating a highly urbanized human population about these impacts can greatly improve species conservation in all ecosystems. Bioscience 52, 883–890. doi: 10.1641/0006-3568(2002)052[0883:UBAC]2.0.CO;2
Meillère, A., Brischoux, F., Parenteau, C., and Angelier, F. (2015). Influence of urbanization on body size, condition, and physiology in an urban exploiter: a multi-component approach. PLoS ONE 10:e135685. doi: 10.1371/journal.pone.0135685
Moreno, J., Potti, J., Yorio, P., and Borboroglu, P. G. (2001). Sex differences in cell-mediated immunity in the Magellanic penguin Spheniscus magellanicus. Ann. Zool. Fennici 38, 111–116. doi: 10.2307/23735756
Norris, K., and Evans, M. R. (2000). Ecological immunology : life history trade-offs and immune defense in birds. Behav. Ecol. 11, 19–26. doi: 10.1093/beheco/11.1.19
Partecke, J., Schwabl, I., and Gwinner, E. (2010). Stress and the city : urbanization and its effects on the stress physiology in European blackbirds. Ecology 87, 1945–1952. doi: 10.1890/0012-9658(2006)87[1945:SATCUA]2.0.CO;2
Peig, J., and Green, A. J. (2009). New perspectives for estimating body condition from mass/length data: the scaled mass index as an alternative method. Oikos 118, 1883–1891. doi: 10.1111/j.1600-0706.2009.17643.x
Polich, R. L. (2016). Stress hormone levels in a freshwater turtle from sites differing in human activity. Conserv. Physiol. 4:cow016. doi: 10.1093/conphys/cow016
R Development Core Team (2016). R: A Language and Environment for Statistical Computing. Vienna: R Foundation for Statistical Computing.
Roberts, M. L., Buchanan, K. L., and Evans, M. R. (2004). Testing the immunocompetence handicap hypothesis: a review of the evidence. Anim. Behav. 68, 227–239. doi: 10.1016/j.anbehav.2004.05.001
Romero, M.L. (2002). Seasonal changes in plasma glucocorticoid concentrations in free-living vertebrates. Gen. Comp. Endocrinol. 128, 1–24. doi: 10.1016/S0016-6480(02)00064-3
Saad, A. H. (1989). Sex-associated differences in the mitogenic responsiveness of snake blood lymphocytes. Dev. Comp. Immunol. 13, 225–229. doi: 10.1016/0145-305X(89)90003-7
Salvador, A., Veiga, J. P., Martin, J., Lopez, P., Abelenda, M., and Puerta, M. (1996). The cost of producing a sexual signal: testosterone increases the susceptibility of male lizards to ectoparasitic infestation. Behav. Ecol. 7, 145–150. doi: 10.1093/beheco/7.2.145
Sapolsky, R. M., Romero, L. M., and Munck, A. U. (2000). How do glucocorticoids influence stress responses ? Integrating permissive, suppressive, stimulatory and preparative actions. Endocr. Rev. 21, 55–89. doi: 10.1210/er.21.1.55
Scheun, J., Bennett, N. C., Ganswindt, A., and Nowack, J. (2015). The hustle and bustle of city life: monitoring the effects of urbanisation in the African lesser bushbaby. Sci. Nat. 102:57. doi: 10.1007/s00114-015-1305-4
Seto, K. C., Fragkias, M., Güneralp, B., and Reilly, M. K. (2011). A meta-analysis of global urban land expansion. PLoS ONE 6:e23777. doi: 10.1371/journal.pone.0023777
Seto, K. C., Güneralp, B., and Hutyra, L. R. (2012). Global forecasts of urban expansion to 2030 and direct impacts on biodiversity and carbon pools. Proc. Natl. Acad. Sci. U.S.A. 109, 16083–16088. doi: 10.1073/pnas.1211658109
Shochat, E., Warren, P. S., Faeth, S. H., McIntyre, N. E., and Hope, D. (2006). From patterns to emerging processes in mechanistic urban ecology. Trends Ecol. Evol. 21, 186–191. doi: 10.1016/J.TREE.2005.11.019
Sih, A., Ferrari, M. C. O., and Harris, D. J. (2011). Evolution and behavioural responses to human-induced rapid environmental change. Evol. Appl. 4, 367–387. doi: 10.1111/j.1752-4571.2010.00166.x
Sol, D., Lapiedra, O., and González-Lagos, C. (2013). Behavioural adjustments for a life in the city. Anim. Behav. 85, 1101–1112. doi: 10.1016/j.anbehav.2013.01.023
Sorace, A. (2002). High density of bird and pest species in urban habitats and the role of predator abundance. Ornis Fenn. 79, 60–71.
Stacy, N. I., Alleman, A. R., and Sayler, K. A. (2011). Diagnostic hematology of reptiles. Clin. Lab. Med. 31, 87–108. doi: 10.1016/j.cll.2010.10.006
Tschirren, B., Fitze, P. S., and Richner, H. (2003). Sexual dimorphism in susceptibility to parasites and cell-mediated immunity in great tit nestlings. J. Anim. Ecol. 72, 839–845. doi: 10.1046/j.1365-2656.2003.00755.x
Wikelski, M., and Cooke, S. J. (2006). Conservation physiology. Trends Ecol. Evol. 21, 38–46. doi: 10.1016/J.TREE.2005.10.018
Wingfield, J. C., Lynn, S. E., and Soma, K. K (2001). Avoiding the “costs” of testosterone: ecological bases of hormone-behavior interactions. Brain Behav. Evol. 57, 239–251. doi: 10.1159/000047243
Keywords: urban, rural, physiology, immune, heterophil, testosterone, Psammophilus, urban adaptor
Citation: Amdekar MS, Kakkar A and Thaker M (2018) Measures of Health Provide Insights Into the Coping Strategies of Urban Lizards. Front. Ecol. Evol. 6:128. doi: 10.3389/fevo.2018.00128
Received: 09 May 2018; Accepted: 09 August 2018;
Published: 07 September 2018.
Edited by:
Caitlin R. Gabor, Texas State University System, United StatesReviewed by:
Inger Suzanne Prange, Ohio University, United StatesRichard Anthony Peters, La Trobe University, Australia
Copyright © 2018 Amdekar, Kakkar and Thaker. This is an open-access article distributed under the terms of the Creative Commons Attribution License (CC BY). The use, distribution or reproduction in other forums is permitted, provided the original author(s) and the copyright owner(s) are credited and that the original publication in this journal is cited, in accordance with accepted academic practice. No use, distribution or reproduction is permitted which does not comply with these terms.
*Correspondence: Maria Thaker, mthaker@iisc.ac.in
†Present Address: Aishwaarya Kakkar, Department of Biotechnology, Goa University, Goa, India