- 1Centre for Ecology, Evolution and Environmental Changes and Azorean Biodiversity Group, Faculty of Sciences and Technology, University of the Azores, Ponta Delgada, Portugal
- 2Crop Research Institute, Prague, Czechia
- 3Applied Ecology Research Group, Department of Biology, Anglia Ruskin University, Cambridge, United Kingdom
A list of alien ladybird (Coleoptera: Coccinellidae) species to Europe was prepared and the history and circumstances of their introductions were determined. Currently there are 12 alien ladybird species in Europe, originating from Australian, Oriental, Nearctic, Palearctic and Afrotropical regions. All of the species were intentionally released as biological control agents and most are coccidophagous species. The aphidophagous Harmonia axyridis is the only ladybird regarded as invasive in Europe. The main factors leading to successful establishment were food relationship, living in different habitats in different geographical regions and geographic origin. We found that successful invaders, in their native areas, showed adaptability to a wide range of conditions compared to non-invasive species. Larger aphidophagous alien ladybirds were found to develop as fast as native species. Characteristics that did not differ between native and alien species are thermal requirements for development, fecundity and body size. However, unlike other alien ladybirds, H. axyridis had higher potential dispersal ability.
Introduction
Most species of ladybirds (Coleoptera: Coccinellidae) are carnivorous predators that feed on a variety of phytophagous arthropods including aphids, coccids and mites (Giorgi et al., 2009; Weber and Lundgren, 2009) and therefore render important services to agricultural and forest ecosystems through biological control of those pests. As such, non-native coccinellid species have been introduced around the world. Biological control using coccinellids started in the late 1800s with the successful introduction of Rodolia cardinalis Mulsant from Australia to control populations of the coccid Icerya purchasi Maskell infesting citrus orchards in California. Since then, several other ladybird species have been introduced to new regions for classical biological control (Roy and Migeon, 2010). The objective of the imports and releases was the establishment of self-perpetuating populations in order to exert natural control over specific pests (Michaud, 2012). Besides the benefits, this process also bears risks, including the spread and establishment of ladybirds in new geographic ranges. The monetary costs of managing alien species once established are often very high. The environmental costs (e.g., effects of an alien biological control agent on species other than the pest species) may also be high (van Lenteren et al., 2008). Thus preventing invasion in the first place is highly desirable, and in order to do this, knowledge of the pathways of invasion are needed (Hulme, 2015).
Whilst there have been numerous intentional coccinellid introductions since the end of the nineteenth century (Hokkanen and Sailer, 1985), the negative impacts were only recently acknowledged (van Lenteren et al., 2003, 2006). The need for intensive study of the consequences of alien coccinellids has been realized due to the negative effects of two species: Coccinella septempunctata L. in the Nearctic and Harmonia axyridis Pallas worldwide. However, initially there was little (or no) concern that these species would have negative impacts on “non-target” indigenous species, through species interactions such as competition and/or intraguild predation.
Food Relationship
Apparently, coccidophagous ladybirds are more prone to thrive in novel geographical areas than are aphidophagous ladybirds. One reason underlying this could be related to the availability of food resources in space and time. Generally, aphids are an extremely abundant but time-limited food resource, whereas coccids are scarcer but available for longer periods of time (Evans, 2003; Borges et al., 2006, 2011). It is noteworthy that aphidophagous and coccidophagous ladybirds' paces of life are a reflection of the respective prey's lifestyle (Dixon et al., 1997): in aphidophagous ladybirds the development is faster, the fecunditie are higher and lifetimes shorter in comparison with coccid-feeding species (Dixon, 2000; Borges et al., 2011, 2013). Therefore evolution favored a higher reproductive investment in aphidophagous ladybirds, which are suited to exploring highly crowded and fast developing prey populations that are available for limited periods of time. On the other hand, coccidophagous ladybirds exploit a food resource that forms lots of colonies of small size. In this case, more energy is required by ladybirds to find enough prey colonies sufficient for egg-laying, and it would be adaptative to store more fat in the soma than in the gonads (Borges et al., 2011). This fact could confer to coccidophagous ladybirds an advantage during the spread stage of the invasion process.
Different Habitats in Different Geographical Regions
The physical geography of the continents and specific climatic patterns, in association with typical vegetation, are important drivers of geographic distributions of ladybird prey species (i.e., mainly Coccoidea and Aphididae), which in turn leads to the geographical distribution of the Coccinellidae (Belicek, 1976; Honěk, 2012). According to Iperti (1999), the central and southern part of tropical Africa, South America, India and China (typically infested with Coccoidea) are rich in species from the tribes Chilocorini (e.g., Chilocorus spp.), Scymnini (e.g., Nephus spp., Cryptolaemus spp.), and Noviini (e.g., Rodolia spp.). In the Mediterranean basin, aphids, coccids and mealybugs are common, and coccinellid species—especially Rhyzobius spp. and Novius spp.—coming from temperate and tropical regions, thrive. The predators of whiteflies from the coccinellid genera Clitostethus and Serangium are particularly diverse in the Pacific area. The temperate zones of both Europe and North America are more prone to infestation by Aphididae: while grasslands and crops are inhabited by coccinellids from the tribes Coccinellini (e.g., Coccinella spp., Adalia spp., Harmonia spp., Propylea spp.), Hippodamiini (e.g., Hippodamia spp.), Cheilomenini and Scymnini (e.g., Pullus spp., Scymnus spp.), forests of deciduous and coniferous trees are typically the habitat of other genera of Coccinellini (e.g., Adalia spp., Anatis spp., Myrrha spp., Myzia spp.) and Hippodamiini (e.g., Aphidecta spp., Hippodamia spp.).
Most of the non-native ladybirds released have not spread and become invasive (see Richardson et al., 2000). Indeed, of the ladybirds released in Europe, H. axyridis is the only species that has become invasive. The causes of successful introductions by other ladybirds without apparently any major negative impacts to local fauna remain unknown. One way of clarifying this is to undertake a comparison of the main biological, ecological and behavioral traits supposed to mediate differences in the invasive capacity of species.
To accomplish a successful invasion, alien species must pass through several stages, which include arrival, establishment and dispersal (Davis, 2009). The process depends on several factors: the life-history traits of the non-native (invasiveness); the characteristics of the new habitat that renders the area susceptible to establishment and spread (invasibility); and interactions among natives, non-natives, and the habitat (Marco et al., 2002). The causes that may mediate high invasive capacity (for example in H. axyridis), include high genetic diversity, phenotypic plasticity, adaptation and genetic shift within newly established colonies, the capability to thrive under different physical environments, broad and flexible habitat use and diet, high reproductive performance, large body size, genetically based polymorphism and escape from natural enemies. For instance, the size of the geographic area occupied by a species in its native region can be an important proxy of its tolerance and adaptability to environmental conditions and thus its potential to become an invader. We expect that a species with a large native area, which in turn is a proxy of climatic and habitat diversity conditions, should cope with a broad range of environmental conditions and is more likely to become invasive. However, despite a number of studies, a comparative dataset of geographical distribution and life-history of ladybirds still does not provide a critical insight into the reasons why certain species are more successful invaders than others. Traits databases, as highlighted by Roy et al. (2016), can be very useful as tools to analyze worldwide life-history traits patterns in native and invaded regions. By combining and crossing traits databases at a global scale, intriguing insights are likely to become evident.
Biological Characteristics
Body size and reproductive rate are important determinants of invasiveness (Davis, 2009; Burton et al., 2010). The greater the capacity of a species to produce propagules (which is closely associated with body size), the greater is its capacity to increase its population density and extend its geographic range (Davis, 2009). In ladybird species, body size seems to be a good surrogate of invasiveness once it is positively associated with potential reproductive rate and dispersal ability (Hemptinne et al., 2012). These factors seem to explain why the larger aphidophagous H. axyridis and C. septempunctata extended their geographic ranges in North America by an order of magnitude faster than their close relatives (Hemptinne et al., 2012). In Europe, three aphidophagous species were released extensively for the biological control of aphid populations; Hippodamia convergens Guerin, Harmonia conformis (Boisduval) and H. axyridis (Dixon, 2000; Roy and Migeon, 2010; Gerber and Schaffner, 2016). However, H. axyridis was the only species to become invasive. To what extent can the success of H. axyridis be explained by differences in dispersion rates between these three species? Taxonomy is a fundamental determinant of body size and the differences connected with phylogenetic constraints should be taken into account when evaluating the importance of characteristics determining invasiveness. The invasive coccinellid species have a wide range of sizes. Aphidophagous species (i.e., most Coccinellinae) are larger but also more variable in size than are species eating non-aphid prey. It is therefore appropriate to consider the characteristics of each group separately.
The development times of exotherms depend on temperature and food resources (quantity and quality). Species with lower thermal requirements may potentially complete development earlier and eventually produce more generations per year. In that case, potential invasive ladybirds are expected to be in an advantageous position in comparison with native species due to their lower thermal requirements and the consequent increase in their net reproductive rate and intrinsic rate of population increase.
In this paper we: (i) review the current status of alien ladybird species in Europe, highlighting the circumstances of their introductions, geographic origins and food relationships; (ii) establish some of the reasons why some species succeeded in entering new geographic areas, using the case studies of Europe and the USA; (iii) assess the characteristics that do not differ between native and alien aphidophagous species. With respect to the latter point we test the extent to which, (a) size of the geographic area occupied by aphidophagous ladybird species in their native regions and (b) some biological traits usually referred to as being the bases of successful invasions (body size, fecundity, and developmental time), differ between native and alien species. To do this we test four predictions: (i) native species occupying larger native areas will more likely become invasive when moved to new areas than species occupying smaller native geographic areas; (ii) larger invasive aphidophagous species will exhibit higher reproductive potential compared to smaller species; (iii) successful invasive aphidophagous ladybirds will have faster developmental rates than native species; (iv) the rate of spread of H. axyridis will be higher than that of the other alien aphidophagous species released in Europe.
Methods
The review of non-native ladybird species in Europe to 2008 was provided by Roy and Migeon (2010) and updated to include the data of the period 2009–2017.
The first prediction was tested with aphidophagous species of the subfamily Coccinellinae originating in the Palearctic that spread to the Nearctic. This is because of the availability of information of species distribution in the source area and presence in the recipient area. Seven species that became established in the Nearctic were selected for analysis: C. septempunctata, C. undecimpunctata L., H. axyridis, H. dimidiata (Fabricius), H. quadripunctata (Pontoppidan), Hippodamia variegata (Goeze), and Propylea quatuordecimpunctata (L.) (Hemptinne et al., 2012). The size of native area in the Palearctic was determined using data for Coccinellidae in the Catalog of Palearctic Coleoptera (Kovář, 2007). This catalog divides the Palearctic, mainly according to political boundaries, to 51 districts of Europe and 85 districts of Asia. The size of area occupied by a species was classed as the total number of districts occupied in Europe and Asia. This area size was determined for the above species that invaded the Nearctic and for each of their non-invasive congener species that occur only in the Palearctic. Within each genus the data on species distribution were standardized as SAi = (MAg–Ai)/SDAg where SAi is standardized area of the species i, MAg is mean area of the genus, Ai is area of the species i and SdAg is standard deviation of the mean area of the genus. After standardization, data of all genera were tested for difference between size of area of invasive species (N = 7) and their congeneric non-invasive species (N = 40: Coccinella 27 spp., Harmonia 7 spp., Hippodamia 3 spp., Propylea 3 spp.) using the Mann-Whitney test.
Concerning the second prediction, the relationship between body-length and the number of ovarioles (representing reproductive potential) was tested using morphological and biological data published in Nedvěd and Honěk (2012), using linear regression y = a + bx, where x is body length (mm) and y is ovariole number. The differences in the number of ovarioles were tested using the Mann-Whitney test.
To test the third prediction, we focused on aphidophagous species that invaded from the Palearctic to the Nearctic, and that are widely distributed in the new area (Hemptinne et al., 2012) and for which the relevant thermal characteristics are available. Thermal constants, lower development threshold (LDT) and sum of effective temperatures (SET), were established for a number of coccinellid species. The constants were calculated from published data (Supplementary Material) using linear regression R = a + bT of development rate (R) on temperature (T) as LDT = −a/b and SET = 1/b. Differences in either characteristic were not useful for comparison. This is because LDT and SET are dependent, which means that errors of estimation of LDT are paralleled by errors in estimating SET (high estimates of LDT are compensated by lower estimates of SET and vice versa). Using LDT and SET for eggs, pupae, and total development, we calculated development duration at a range of ecologically relevant temperatures (16, 18, 20, 22, 24, 26 and 28°C) and tested for differences between invasive and non-invasive species. For each stage and temperature the differences between invasive and native species were compared using the Mann-Whitney test.
Regarding the fourth prediction, native and exotic species were characterized in terms of their dry body mass. The power function W = 0.032 L2.62 was used to calculate dry body mass (W; mg) where L is the body length (mm) (Rogers et al., 1976; Sabo et al., 2002); the median body length data for particular species were retrieved from the literature (Dobrzhansky, 1926; Bielawski, 1959; Leeper, 1976, 2015; Gordon, 1985; Raimundo and Alves, 1986; Rathour and Singh, 1991; Stathas et al., 2002). The potential reproductive rate was expressed as half the number of ovarioles, with data retrieved from the literature (e.g., Hemptinne et al., 2012; Nedvěd and Honěk, 2012; and personal observations). Predicted dispersal abilities of native and alien aphidophagous ladybirds were estimated from the values of dry body mass raised to the power of 0.5618, the sum of individual effect of potential reproductive rate (0.3718) and dispersal ability (0.19). We found a significant positive correlation between the logarithm to base e of adult biomass (lnAM) and that of the potential reproductive rate (half the number of ovarioles–lnPRR) and this was expressed as lnPRR = 0.3718 lnAM + 2.6085 (r = 0.50 p = 0.013). The value of the power of dispersal ability takes into account the maximum rate of oxygen consumption, which increases with body mass raised to the power 0.85, and the cost of running, which increases with the exponent 0.67 (Schmidt-Nielsen, 1984) (for further methodological details, see Hemptinne et al., 2012).
Finally, we tested the significance of the values of potential dispersal ability of the aphidophagous alien species (H. axyridis, H. conformis, and H. convergens) against the average potential dispersal ability of native species (one sample t-test; p = 0.05) (SPSS, v. 24).
Results
The Current Status of Alien Ladybird Species in Europe
A total of 24 new alien species-country records were found (i.e., since Roy and Migeon, 2010), taking the total known alien species-country records to 131 (Table 1). Harmonia axyridis is the most widespread ladybird species (Figure 1). From the early 2000s the number of countries where this species was found increased steadily, seemingly reaching a plateau in 2013 (Figure 2). Harmonia axyridis was the species that invaded the most countries, followed by Cryptolaemus montrouzieri Mulsant with only a third of the newly reported records (Table 2). The alien ladybird species present in Europe (12) introduced to control coccids, aphids, psyllids and whitefly, are summarized in Table 3.
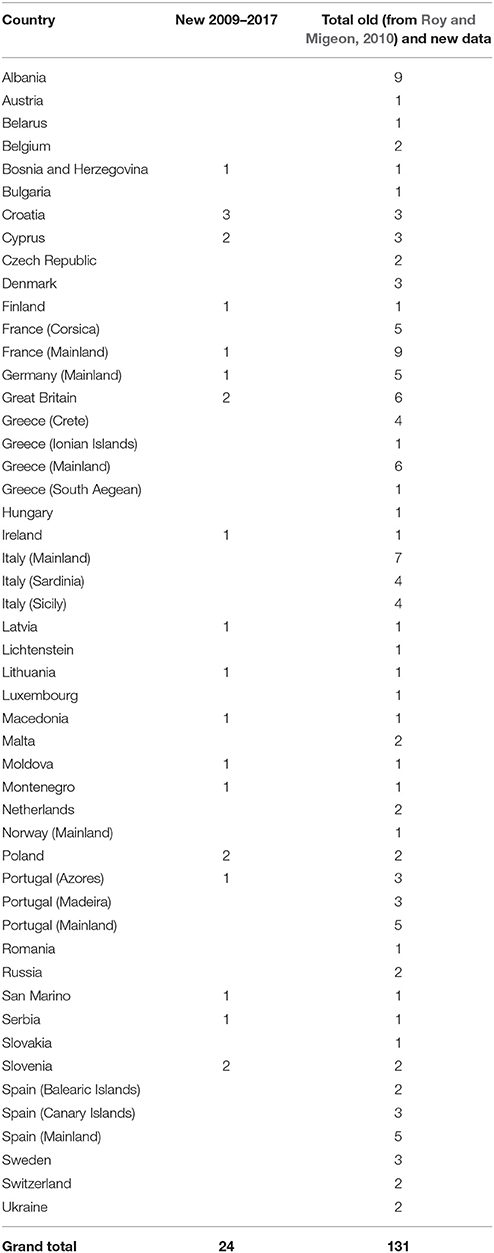
Table 1. Numbers of newly reported (i.e., in literature from 2009 to 2017) and total alien ladybird species in European countries.
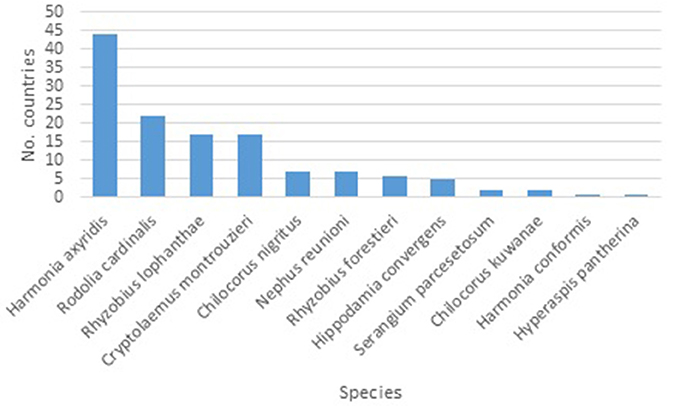
Figure 1. Number of European countries with specified alien ladybird species recorded as present. (Data combined from Roy and Migeon (2010) and 2009–2017 literature searches. Island territories included separately, N = 131).
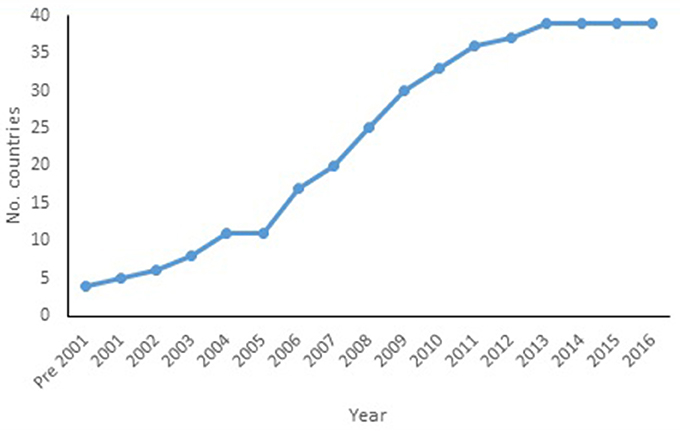
Figure 2. Cumulative number of European countries with H. axyridis recorded as present. (Island territories included with their country, e.g., Corsica is included with France).
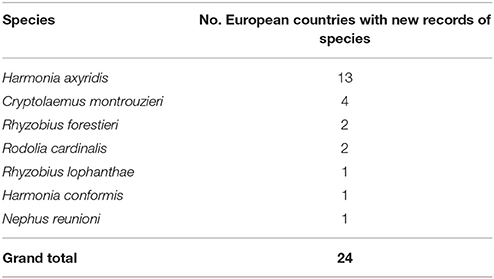
Table 2. Number of European countries with specified alien ladybird species newly reported (i.e., in literature from 2009 to 2017).
The regions of origin of the alien coccinellid species to Europe are as follows: Australian (5 species: C. montrouzieri; H. conformis (Boisduval) R. forestieri, R. lophanthae and R. cardinalis); Oriental (2 species: C. nigritus, and S. parcesetosum); Nearctic (2 species: H. convergens and H. pantherina); Palearctic (2 species: C. kuwanae and H. axyridis) and Afrotropical (1 species: N. reunioni) regions.
Food Relationships of European Alien Species
The majority of the alien coccinellid species in Europe are coccidophagous (7 species: 58%), followed by those which are aphidophagous (4 species: 33%), with a single specialized predator of whiteflies (Hemiptera; Aleyrodidae).
Characteristics of Invasive and Native Species
In their native Palearctic region, invasive species occupied a significantly larger geographic area than the species that did not invade the Nearctic (Mann-Whitney P < 0.001; U = 6.000; Figure 3). In terms of numbers of geographic districts, the average native area of invasive species (N = 7) was 57.7 ± 11.80 districts, while the area of non-invasive species (N = 40) was only 12.2±2.35 districts.
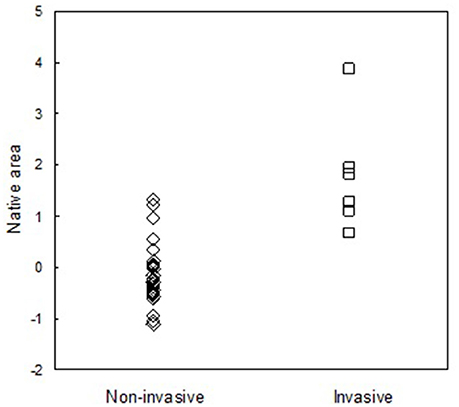
Figure 3. The standardized areas of species of the genera Coccinella, Harmonia, Hippodamia, and Propylea that remained limited to their native areas in the Palearctic (non-invasive species) and seven species of these genera that spread after they invaded the Nearctic (invasive species).
We found that the number of ovarioles increased with body size; both in species feeding on aphids as well as non-aphidophagous species (Figure 4). Larger aphidophagous species had significantly (Mann-Whitney P < 0.001, U = 675.5) more ovarioles (43.6 ± 3.56) than did non-aphidophagous species (15.9 ± 6.48).
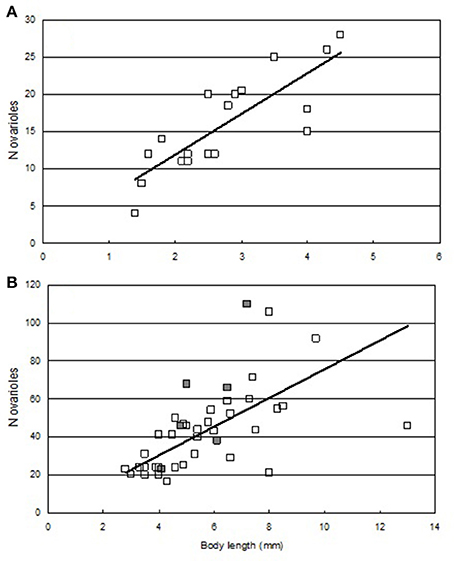
Figure 4. Body-length (mm) and average number of ovarioles in species (A) feeding on non-aphid prey (N = 18, R = 0.821, a = 0.900, b = 5.482, P < 0.001) and species (B) feeding on aphids (N = 40, R = 0.567, a = 8.521, b = 6.203, P < 0.001). Dark symbols: species that invaded the Nearctic from the Palearctic. Data from Nedvěd and Honěk (2012).
The potential dispersal ability of H. axyridis was significantly higher than that of the native species, whilst the dispersal abilities of H. conformis and H. convergens did not significantly differ from native species (Table 4). The rate of spread of H. axyridis in Europe was high, as it was in the USA.
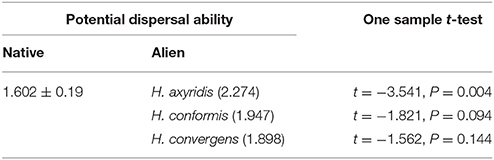
Table 4. Potential dispersal ability (average±SE; N= 13) of native and alien aphidophagous ladybird species in Europe.
In both native and invasive species there were no significant differences in development length at any development stage under any temperature (Mann-Whitney P > 0.05) (Table 5), with similar constants of linear regressions obtained from the development rate (R) and temperature (T) (Table 6) and similar values of lower development threshold (LDT) and sum of effective temperatures (SET) (Table 7).
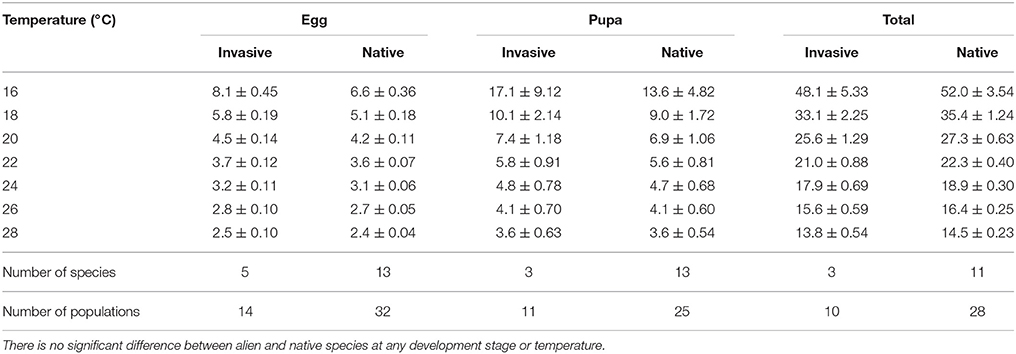
Table 5. The duration of egg, pupa and total development (measured in days) at seven constant temperatures in species invasive to the Nearctic (C. septempunctata, C. undecimpunctata, H. axyridis, H. variegata, P. quatuordecimpunctata), and native to the Nearctic (A. bipunctata, Calvia quatuordecimguttata, Cheilomenes sulphurea, C. californica, C. novemnotata, C. transversoguttata, C. trifasciata, C. maculata, Cycloneda polita, H. parenthesis, H. convergens, H. quinquesignata, H. sinuata, Lemnia biplagiata, O. v-nigrum).
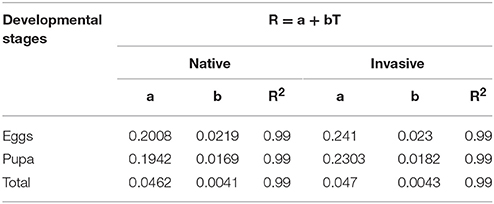
Table 6. Thermal constants at seven constant temperatures in species invasive to the Nearctic (C. septempunctata, C. undecimpunctata, H. axyridis, H. variegata, P. quatuordecimpunctata), and native to the Nearctic (A. bipunctata, C. quatuordeciguttata, C. sulphurea, C. californica, C. novemnotata, C. transversoguttata, C. trifasciata, C. maculata, C. polita, H. parenthesis, H. convergens, H. quinquesignata, H. sinuata, Lemnia biplagiata, O. v-nigrum).
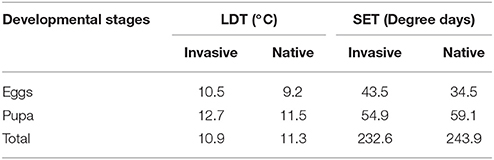
Table 7. Lower development threshold (LDT) and sum of effective temperatures (SET) at seven constant temperatures in species invasive to the Nearctic (C. septempunctata, C. undecimpunctata, H. axyridis, H. variegata, P. quatuordecimpunctata), and native to the Nearctic (A. bipunctata, C. quatuordecimguttata, C. sulphurea, C. californica, C. novemnotata, C. transversoguttata, C. trifasciata, C. maculata, C. polita, H. parenthesis, H. convergens, H. quinquesignata, H. sinuata, L. biplagiata, O. v-nigrum).
Discussion
The 12 alien ladybird species recorded in Europe were introduced to control coccids, aphids, psyllids and whitefly, with the majority of the species being coccidophagous. In 104 species-country records a ladybird species was released in a country as a biological control agent (further called “direct” cases), whereas in 25 species-country records a ladybird species spread from a country in which it was released to a neighboring country (“indirect” cases). In two cases the source of the alien ladybirds is unknown. These figures include species-country records in which the ladybird did not establish, and non-target effects are not evident in many further cases. Hence, only in a relatively small proportion of cases did the alien coccinellid have widespread non-target effects (and these mostly involve H. axyridis). Within the “indirect” biological control cases, the exact mechanisms of spread are often unclear, and for each case of an alien arriving in a particular country, it may do so by various means. For example, in Great Britain some H. axyridis arrived by natural dispersal from mainland Europe, but others are known to have been introduced with products (e.g., imported cut flowers, vegetables and fruits), and yet others arrived with people, transported inadvertently in cars, trains and ships (Roy and Brown, 2015). Additionally, the source populations were not restricted to Europe: some H. axyridis arrived in Great Britain in packages originating from Canada (Majerus et al., 2006). The examples for which the exact pathway is known are rare: in most cases the mechanisms by which a particular insect or group of insects arrived to a particular place is unknown. As such, the best that we can do is to classify all such arrivals under a broad banner such as “introduced as an indirect result of biological control.”
Perhaps as would be expected, food relationship seems to be a good indirect predictor of a successful coccinellid introduction: worldwide, from the total attempts to control aphids (155) and coccids (613) using classical biological control approaches, only one case (0.65%) of aphid control turned out to be satisfactorily successful and complete success has never been achieved (Dixon, 2000). On the contrary, the control of coccids has been considered complete in 23 cases (3.75%) and substantially successful in 30 attempts (4.89%) (Dixon, 2000).
According to our initial prediction, invasive ladybirds tend to be more dispersed geographically in their native areas. An ideal picture of coccinellid distributions would be via high resolution digital maps in which each pixel represents a small area of the Palearctic marked by the presence or absence of the species. However, reaching this goal seems impossible in the near future because of the difficulties associated with recording species presence in most parts of the world. In this study, we have chosen a compromise solution of dividing the Palearctic into political districts and using data on species presence within these districts. This approach has some pitfalls, particularly since the districts are of unequal landscape diversity and size. Small districts with e.g., great altitudinal differences may provide an array of diverse local conditions greater than large districts comprised of flat landscapes. Consequently a species living in a small district with a diversified landscape may be adapted to a wider range of environmental conditions than a species living in an extensive area with uniform landscape conditions. However, the correlation between landscape diversity and range of species adaptation to environmental conditions is not as important as it may seem. Regardless of the range of available options of habitat choice, coccinellid species prefer particular habitats determined by hostplant, microclimate, prey and seasonality (Honěk, 1985; Nedvěd, 1999; Honěk et al., 2015). A consequence of this habitat specialization is a limited choice of utilizable sites, even in areas of high landscape diversity. However, some variation in environment cannot be eliminated even by a strong individual habitat choice: this is the variation associated with climatic differences among districts that fill a large geographic area. Thus a species distributed in a large geographic area, despite its narrow habitat selection, is likely to meet, cope with and be adapted to a greater range of environmental conditions than a species distributed in a small geographic area and this adaptation may be, in our opinion, a basis of its success in colonization of new geographic areas. Our results thus confirm that invasive species are, in their native areas, more prone to adapt to a wide range of conditions compared to non-invasive species in their native ranges.
For generalist predators, flexibility and breadth of habitat use and diet are direct underlying factors of a successful invasion. In general, successful invaders in their new ranges tend to occupy the same habitats as in their native ranges. This is the case with C. septempunctata and H. axyridis, which are most abundant in herbaceous and arboreal habitats, respectively. However, despite occurring in many semi-natural and natural habitats in their invaded ranges, C. septempunctata and H. axyridis, as well as the successful A. bipunctata and P. quatuordecimpunctata, tend to be abundant in agricultural habitats and urban landscapes (e.g., Lucas et al., 2007; Brown et al., 2008; Roy and Migeon, 2010; Evans et al., 2011). In the USA, H. axyridis became a dominant species in agricultural landscapes, thriving in disturbed and man-made habitats such as orchards and urban gardens, feeding on a wide range of aphid species (Koch, 2003; Evans, 2004). In Europe, H. axyridis is more common in northern and central countries, where it can be found in trees, shrubs and herbs (Brown and Roy, 2017; Honěk et al., 2017; Soares et al., 2017). Coccinella septempunctata, H. axyridis and A. bipunctata have a broad and flexible diet range, being quite responsive to new prey species (Evans and Toler, 2007). However, the mechanism by which integration of a new prey species into the diet of a generalist predator is able to drive adaptation to a new geographic range, is unclear. To explore this, it is important to evaluate whether life-history traits of organisms are responsive to selection, and what constraints and trade-offs may limit the overall possible set of traits. However, there are only three studies addressing prey adaptation, two using the generalist H. axyridis as a model (Ueno, 2003; Fukunaga and Akimoto, 2007), and one using A. bipunctata (Rana et al., 2002). Over the course of several generations the aphidophagous species were able to improve their fitness when fed on a single diet of lower prey quality. However, the genetic and epigenetic mechanisms that promote this difference remain to be studied.
The absence of significant differences between developmental rates among aphidophagous ladybirds agrees with previous results from laboratory experiments. For instance, in some studies the overall pre-imaginal development time of H. axyridis and C. maculata were similar (Labrie et al., 2006) with other studies demonstrating a slower developmental time of H. axyridis in comparison to other species (Adalia bipunctata L., H. variegata, Lanzoni et al., 2004; P. quatuordecimpunctata, Mignault et al., 2006; Olla v-nigrum Mulsant, Michaud and Olsen, 2004). An expected consequence of faster developmental rate is the possibility of the invasive species achieving more generations per year. However, this does not occur even with successful ladybirds spreading into novel ranges, partly because of lower average temperatures. For instance, within much of the invaded range, H. axyridis only achieves two generations per year (Roy et al., 2016), whereas in the native range up to eight generations have been observed (Osawa, 2011), this difference being associated with environmental temperatures. In conclusion, our results do not corroborate our initial prediction because the invasive species may not have an advantage against natives in using the thermal environment.
Although fecundity is influenced by abiotic (e.g., temperature) and biotic (e.g., prey quantity and quality) factors, plus factors such as age and life-history (Nedvěd and Honěk, 2012), body size is a primary determinant of the number of ovarioles (Hemptinne et al., 2012). The scatter of data for large aphidophagous species was greater than for small non-aphidophagous species. This may lead to invasive species having higher potential fecundity than non-invasive species. In fact, whilst large invasive species have, in some cases, more ovarioles than predicted by the overall relationship, this was not strongly evident in our results. In the case of H. axyridis fecundity seems to be similar to that of some other aphidophagous species (Soares et al., 2001, 2004, 2008; Bazzochi et al., 2004; Lanzoni et al., 2004; Michaud and Olsen, 2004). For instance, Italian specimens of H. axyridis presented a mean fecundity of 783.8 eggs per female, only slightly higher than that of the indigenous species A. bipunctata (720.2 eggs per female) (Bazzochi et al., 2004). Additionally, fewer eggs were laid by H. axyridis (560.5 eggs per female) than by H. variegata (841.7 eggs per female) in a laboratory study by Lanzoni et al. (2004). Soares et al. (2004) reported an even lower fecundity of 314 eggs per female for the aulica phenotype of H. axyridis. Michaud and Olsen (2004), using psyllids as a food source, showed that H. axyridis laid as many eggs as O. v-nigrum (242.8 vs. 224.5 eggs per female). Despite these similarities in fecundity, the differences in ovariole number do not always mirror clutch size or fecundity. This is because daily and lifetime egg production are influenced by other environmental factors. However, we predicted that H. axyridis has higher dispersal ability than H. conformis and H. variegata. To test this prediction, we compared the potential rate of spread of the three species based on their potential reproductive rates and adult body sizes. In short, invasiveness in aphidophagous ladybirds is associated with their large body size, a good surrogate of reproductive potential and dispersal ability, which determines their efficacy in exploiting aphid outbreaks in extensive forestry and agro-ecosystems (Honěk et al., 2008; Kajita and Evans, 2010; Hemptinne et al., 2012; Soares et al., 2017).
Our data point out trait differences between native/alien and invader/established aphidophagous ladybirds and some traits seem to explain successful establishment. However, data of species that have been introduced from the same region but have not become established in the new region would be important in order to better understand invasion processes.
Author Contributions
All authors listed have made a substantial, direct and intellectual contribution to the work, and approved it for publication.
Conflict of Interest Statement
The authors declare that the research was conducted in the absence of any commercial or financial relationships that could be construed as a potential conflict of interest.
Acknowledgments
This study was financed by Portuguese National Funds, through FCT–Fundação para a Ciência e a Tecnologia, within the project UID/BIA/00329/2013.
IB was funded by a grant from Fundo Regional da Ciência, Regional Government of the Azores. Ref: M3.1.7/F/012/2011.
PB was supported by the Anglia Ruskin University sabbatical fund and by EU COST Action TD1209: Alien Challenge.
AH and ZM were supported by grant 17-06763S of the Czech Science Foundation.
Supplementary Material
The Supplementary Material for this article can be found online at: https://www.frontiersin.org/articles/10.3389/fevo.2018.00057/full#supplementary-material
References
Amaro, P. (1994). “Portugal pioneiro da luta biológica na Europa através do combate à Icéria com Vedália,” in 1° Congresso de Citricultura. Simpósio Protecção Integrada de Citrinos. 20 a 22 de Janeiro de 1993. (Silves) 393–402.
Bazzochi, G. G., Lanzoni, G., Accinelli, G., and Burgio, G. (2004). Overwintering, phenology and fecundity of Harmonia axyridis in comparison with native coccinellid species in Italy. BioControl 49, 245–260. doi: 10.1023/B:BICO.0000025382.07841.b4
Belicek, J. (1976). Coccinellidae of Western Canada and Alaska with analyses of the transmontane zoogeographic relationship between the fauna of British Columbia and Alberta. Questiones Entomol. 12, 283–409.
Bielawski, R. (1959). “Biedronki – Coccinellidae,” in Wróblewski. Klucze do Oznaczania Owadów Polski, Czesc XIX Chrzaszcze – Coleoptera, Zeszyt 7, eds A. Goljan, T. Jaczewski, M. Mroczkowski, J. Nast, J. Noskiewicz, M. Nunberg, J. Pruffer, S. Smreczynski, J. Stach, K. Strawinski, J. Urbanski, and A. Wróblewski (Warszawa: Panstwowe Wydawnictwo Naukowe), 1–92.
Borges, I., Soares, A. O., and Hemptinne, J.-L. (2006). Abundance and spatial distributions of aphids and scales select for different life histories in ladybeetle predators. J. Appl. Entomol. 130, 461–464. doi: 10.1111/j.1439-0418.2006.01071.x
Borges, I., Soares, A. O., and Hemptinne, J.-L. (2013). Contrasting population growth parameters of the aphidophagous Scymnus nubilus and the coccidophagous Nephus reunioni. BioControl 58, 351–357. doi: 10.1007/s10526-012-9490-y
Borges, I., Soares, A. O., Magro, A., and Hemptinne, J.-L. (2011). Prey availability in time and space is a driving force in life history evolution of predatory insects. Evol. Ecol. 25, 1307–1319. doi: 10.1007/s10682-011-9481-y
Brown, P. M. J., and Roy, H. E. (2017). Decline in native ladybird species caused by the invasive harlequin ladybird Harmonia axyridis: evidence from a long term field study. Insect Conserv. Divers. doi: 10.1111/icad.12266. [Epub ahead of print].
Brown, P. M. J., Roy, H. E., Rothery, P., Roy, D. B., Ware, R. L., and Majerus, M. E. N. (2008). Harmonia axyridis in Great Britain: analysis of spread and distribution of a non-native coccinellid. BioControl 53, 55–67. doi: 10.1007/s10526-007-9124-y
Burton, O. J., Phillips, B. L., and Travis, J. M. (2010). Trade-offs and the evolution of life-histories during range expansion. Ecol. Lett. 13, 1210–1220. doi: 10.1111/j.1461-0248.2010.01505.x
Cavalloro, R., and Di Martino, E. (1986). “Integrated pest control in citrus-groves,” Proceedings of the Experts' Meeting, 26–29 March (Acireale), 600.
Dixon, A. F. G. (2000). Insect Predator-Prey Dynamics: Ladybeetles and Biological Control. Cambridge: Cambridge University Press.
Dixon, A. F. G., Hemptinne, J.-L., and Kindlmann, P. (1997). Effectiveness of ladybirds as biological control agents: patterns and processes. Entomophaga 42, 71–83. doi: 10.1007/BF02769882
Dobrzhansky, F. G. (1926). Genital apparatus of ladybirds as individual and group character. Trans. Acad. Sci. USSR 6, Series 20, 1385–1393.
Duverger, C. (1997). Premiére mention de Rhyzobius forestieri (Mulsant) 1853 en France. (Coleoptera Coccinellidae). Bulletin de la Société linnéenne de Bordeaux 25, 81–83.
Evans, E. W. (2003). Searching and reproductive behavior of female aphidophagous ladybirds (Coleoptera: Coccinellidae): a review. Eur. J. Entomol. 100, 1–10. doi: 10.14411/eje.2003.001
Evans, E. W. (2004). Habitat displacement of North American ladybirds by an introduced species. Ecology 85, 637–647. doi: 10.1890/03-0230
Evans, E. W., Soares, A. O., and Yasuda, H. (2011). Invasions by ladybugs, ladybirds, and other predatory beetles. BioControl 56, 597–611. doi: 10.1007/s10526-011-9374-6
Evans, E. W., and Toler, T. (2007). Aggregation of polyphagous predators in response to multiple prey species: ladybirds (Coleoptera:Coccinellidae) foraging in alfalfa. Popul. Ecol. 49, 29–36. doi: 10.1007/s10144-006-0022-4
Fowler, S. V. (2004). Biological control of an exotic scale, Orthezia insignis Browne (Homoptera: Ortheziidae), saves the endemic gumwood tree, Commidendrum robustum (Roxb.) DC. (Asteraceae) on the island of St. Helena. Biol. Control 29, 367–374. doi: 10.1016/j.biocontrol.2003.06.002
Fukunaga, Y., and Akimoto, S. (2007). Toxicity of the aphid Aulacorthum magnoliae to the predator Harmonia axyridis (Coleoptera: Coccinellidae) and genetic variance in the assimilation of the toxic aphids in H. axyridis larvae. Entomol. Sci. 10, 45–53. doi: 10.1111/j.1479-8298.2006.00197.x
Garcia, V. (1986). “Approaches to integrated control of some citrus pests in the Azores and Algarve (Portugal),” in Integrated Pest Control in Citrus Groves. Proceedings of the CEC Experts Meeting, eds R. Cavalloro and E. Di Martino (Acireale), 557–559.
Gerber, E., and Schaffner, U. (2016). “Exotic insect biocontrol agents released in europe,” in Review of Invertebrate Biological Control Agents Introduced into Europe, eds E. Gerber and U. Schaffner (Wallingford, VT: CABI), 9–117.
Giorgi, J. A., Vandenberg, N. J., McHugh, J. V., Forrester, J. A., Slipinski, S. A., Miller, K. B., et al. (2009). The evolution of food preferences in Coccinellidae. Biol. Control 51, 215–231. doi: 10.1016/j.biocontrol.2009.05.019
Gordon, R. D. (1985). The Coccinellidae (Coleoptera) of America North of Mexico. J. New York Entomol. S. 93, 741–743.
Hamid, H. A., and Michelakis, S. (1994). The importance of Cryptolaemus montrouzieri Mulsant (Col., Coccinellidae) in the control of the citrus mealybug Planococcus citri (Hom., Coccoidea) under specific conditions. J. Appl. Entomol. 118, 17–22. doi: 10.1111/j.1439-0418.1994.tb00773.x
Hemptinne, J.-L., Magro, A., Evans, E. W., and Dixon, A. F. G. (2012). Body size and the rate of spread of invasive ladybird beetles in North America. Biol. Invasions 14, 595–605. doi: 10.1007/s10530-011-0101-0
Hokkanen, H. M. T., and Sailer, R. I. (1985). Success in classical biological control. Crit. Rev. Plant Sci. 3, 35–72. doi: 10.1080/07352688509382203
Honěk, A. (1985). Habitat preferences of aphidophagous coccinellids (Coleoptera). Entomophaga 30, 253–264. doi: 10.1007/BF02372226
Honěk, A. (2012). “Distribution and Habitats,” in Ecology and Behaviour of the Ladybird Beetles (Coccinellidae), eds I. Hodek, H. F. van Emden and A. Honěk (Chichester: Wiley-Blackwell), 110–140.
Honěk, A., Dixon, A. F. G., and Martinkova, Z. (2008). Body size, reproductive allocation, and maximum reproductive rate of two species of aphidophagous Coccinellidae exploiting the same resource. Entomol. Exp. Appl. 127, 1–9. doi: 10.1111/j.1570-7458.2007.00663.x
Honěk, A., Dixon, A. F. G., Soares, A. O., Skuhrovec, J., and Martinkova, Z. (2017). Spatial and temporal changes in the abundance and composition of ladybird (Coleoptera: Coccinellidae) communities. Curr. Opin. Insect Sci. 20, 61–67. doi: 10.1016/j.cois.2017.04.001
Honěk, A., Martinkova, Z., and Dixon, A. F. G. (2015). Detecting seasonal variation in composition of adult Coccinellidae communities. Ecol. Entomol. 40, 543–552. doi: 10.1111/een.12225
Hulme, P. E. (2015). Invasion pathways at a crossroad: policy and research challenges for managing alien species introductions. J. Appl. Ecol. 52, 1418–1424. doi: 10.1111/1365-2664.12470
Iperti, G. (1999). Biodiversity of predaceous coccinellidae in relation to bioindication and economic importance. Agr. Ecosyst. Environ. 74, 323–342. doi: 10.1016/S0167-8809(99)00041-9
Kairo, M. T. K., Paraiso, O., Gautam, R. D., and Peterkin, D. D. (2013). Cryptolaemus montrouzieri (Mulsant) (Coccinellidae: Scymninae): a review of biology, ecology, and use in biological control with particular reference to potential impact on non-target organisms. CAB Rev. 8:005. doi: 10.1079/PAVSNNR20138005
Kajita, Y., and Evans, E. W. (2010). Relationships of body size, fecundity, and invasion success among predatory lady beetles (Coleoptera: Coccinellidae) inhabiting alfalfa fields. Ann. Entomol. Soc. Am. 103, 750–756. doi: 10.1603/AN10071
Katsoyannos, P. (1984). The establishment of Rhyzobius forestieri (Col.: Coccinellidae) in Greece and its efficiency as an auxilliary control agent against heavy infestation of Saissetia oleae (Hom.: Coccidae). Entomophaga 29, 387–397. doi: 10.1007/BF02372160
Koch, R. L. (2003). The multicolored Asian lady beetle, Harmonia axyridis: a review of its biology, uses in biological control, and non-target impacts. J. Insect. Sci. 3, 1–16. doi: 10.1673/031.003.3201
Kovář, I. (2007). “Coccinellidae,” in Catalogue of Palaearctic Coleoptera, Vol. 4, eds I. Löbl and A. Smetana (Denmark: Apollo Books), 568–630.
Labrie, G., Lucas, E., and Coderre, D. (2006). Can developmental and behavioral characteristics of the multicolored Asian lady beetle Harmonia axyridis explain its invasive success? Biol. Invasions 8, 743–754. doi: 10.1007/s10530-005-3428-6
Lanzoni, A., Accinelli, G., Bazzocchi, G. G., and Burgio, G. (2004). Biological traits and life table of the exotic Harmonia axyridis compared with Hippodamia variegata and Adalia bipunctata (Col. Coccinellidae). J. Appl. Entomol. 128, 298–306. doi: 10.1111/j.1439-0418.2004.00847.x
Leeper, J. R. (1976). A Review of the Hawaiian Coccinellidae. Proc. Hawaii. Entomol. Soc. XXII, 279–306.
Leeper, J. R. (2015). A Review of Coccinellid (Coleoptera: Coccinellidae) Introductions and Establishments in Hawaii: 1885 to 2015. Manoa: University of Hawaii-eVols series.
Lucas, E., Vincen, C., Labrie, G., Chouinard, G., Fournier, F., Pelletier, F., et al. (2007). The multicolored Asian lady beetle Harmonia axyridis (Coleoptera: Coccinellidae) in Quebec agroecosystems ten years after its arrival. Eur. J. Entomol. 104, 737–743. doi: 10.14411/eje.2007.094
Majerus, M., Strawson, V., and Roy, H. (2006). The potential impacts of the arrival of the harlequin ladybird, Harmonia axyridis (Pallas) (Coleoptera: Coccinellidae) in Britain. Ecol. Entomol. 31, 207–215. doi: 10.1111/j.1365-2311.2006.00734.x
Malausa, J. C., Franco, E., and Brun, P. (1988). Establishment on the Azur coast and in Corsica of Serangium parcesetosum (Col.: Coccinellidae), a predator of the citrus whitefly, Dialeurodes citri (Hom.: Aleyrodidae). Entomophaga 33, 517–519. doi: 10.1007/BF02373187
Malausa, J. C., Rabasse, J. M., and Kreiter, P. (2008). Entomophagous insects of agricultural interest in France since the beginning of the 20th century. OEPP/EPPO Bull. 38, 136–146.
Marco, D. A., Páez, S. A., and Cannas, S. A. (2002). Species invasiveness in biological invasions: a modelling approach. Biol. Invasions 4, 193–205. doi: 10.1023/A:1020518915320
Michaud, J. P. (2012). “Coccinellids in Biological Control,” in Ecology and Behaviour of the Ladybird Beetles (Coccinellidae), eds I. Hodek, H. F. van Emden and A. Honěk (Chichester: Wiley-Blackwell), 488–519.
Michaud, J. P., and Olsen, L. E. (2004). Suitability of Asian citrus psyllid, Diaphorina citri, as prey for ladybeetles. BioControl 49, 417–431. doi: 10.1023/B:BICO.0000034605.53030.db
Mignault, M.-P., Roy, M., and Brodeur, J. (2006). Soybean aphid predators in Québec and the suitability of Aphis glycines as prey for three Coccinellidae. BioControl 51, 89–106. doi: 10.1007/s10526-005-1517-1
Nedvěd, O. (1999). Host complexes of predaceous ladybeetles (Col., Coccinellidae). J Appl. Entomol. 123, 73–76.
Nedvěd, O., and Honěk, A. (2012). “Life history and development,” in Ecology and Behaviour of the Ladybird Beetles (Coccinellidae), eds I. Hodek, H. F. van Emden and A. Honěk (Chichester: Wiley-Blackwell), 54–109.
Osawa, N. (2011). Ecology of Harmonia axyridis in natural habitats within its native range. Biocontrol 56, 613–621. doi: 10.1007/s10526-011-9382-6
Raimundo, A. A. C., and Alves, M. L. L. G. (1986). Revisão dos Coccinelídeos de Portugal. Évora: Universidade de Évora.
Rana, J. S., Dixon, A. F. G., and Jarošík, V. (2002). Costs and benefits of prey specialization in a generalist insect predator. J. Anim. Ecol. 71, 15–22. doi: 10.1046/j.0021-8790.2001.00574.x
Rathour, Y. S., and Singh, T. (1991). Importance of ovariole number in Coccinellidae (Coleoptera). Entomon 16, 35–41.
Richardson, D. M., Pysěk, P., Rejmanek, M., Barbour, M. G., Panetta, F. D., and West, C. J. (2000). Naturalization and invasion of alien plants: concepts and definition. Divers. Distrib. 6, 93–107. doi: 10.1046/j.1472-4642.2000.00083.x
Rogers, R. L., Hinds, W. T., and Buschbom, R. L. (1976). A general weight vs. length relationship for insects. Ann. Entomol. Soc. Am. 69, 387–389. doi: 10.1093/aesa/69.2.387
Roy, H., Brown, P., Frost, R., and Poland, R. (2011). Ladybirds (Coccinellidae) of Britain and Ireland. Wallingford: Natural Environmental Research Centre.
Roy, H. E., and Brown, P. M. J. (2015). Ten years of invasion: Harmonia axyridis (Pallas) (Coleoptera: Coccinellidae) in Britain. Ecol. Entomol. 40, 336–348. doi: 10.1111/een.12203
Roy, H. E., Brown, P. M. J., Adriaens, T., Berkvens, N., Borges, I., Clusella Trullas, S., et al. (2016). The harlequin ladybird, Harmonia axyridis: an inspiration for global collaborations on invasion biology. Biol. Invasions 18, 997–1044. doi: 10.1007/s10530-016-1077-6
Roy, H., and Migeon, A. (2010). Ladybeetles (Coccinellidae). BioRisk 4, 293–313. doi: 10.3897/biorisk.4.49
Sabo, J. L., Bastow, J. L., and Power, M. E. (2002). Length–mass relationships for adult aquatic and terrestrial invertebrates in a California watershed. J. N. Am. Benthol Soc. 21, 336–343. doi: 10.2307/1468420
Schanderl, H., and Almeida, J. M. (1992). Introdução de Harmonia axyridis Pallas (Col., Coccinellidae) na ilha de S. Maria. Açoreana 7, 401–406.
Schanderl, H., Ferran, A., Coderre, D., Ventura, A., Soares, A. O., Almeida, J. M. P., et al. (1991). Capacidade de dispersão de Harmonia axyridis Pallas (Col., Coccinellidae) após uma largada inundativa para controlo de afídeos do Milho Rhopalosiphum padi L. e Sitobion avenae F. (Hom., Aphididae). Relatórios e Comunicações do Departamento de Biologia 20, 59–64.
Schmidt-Nielsen, K. (1984). Scaling. Why Is Animal Size So Important? Cambridge: Cambridge University Press.
Soares, A. O., Borges, I., Borges, P. A. V., Labrie, G., and Lucas, E. (2008). Harmonia axyridis: what will stop the invader? BioControl 53, 127–145. doi: 10.1007/s10526-007-9141-x
Soares, A. O., Coderre, D., and Schanderl, H. (2001). Fitness of two phenotypes of Harmonia axyridis Pallas (Coleoptera: Coccinellidae). Eur. J. Entomol. 98, 287–293. doi: 10.14411/eje.2001.048
Soares, A. O., Coderre, D., and Schanderl, H. (2004). Dietary self-selection behaviour by the adults of the aphidophagous ladybeetle Harmonia axyridis (Coleoptera: Coccinellidae). J. Anim. Ecol. 73, 478–486. doi: 10.1111/j.0021-8790.2004.00822.x
Soares, A. O., Honěk, A., Martinkova, Z., Skuhrovec, J., Cardoso, P., and Borges, I. (2017). Harmonia axyridis did not establish in the Azores: the role of species richness, intraguild interactions resource availability. BioControl 62, 423–434. doi: 10.1007/s10526-017-9794-z
Stathas, G. J., Eliopoulos, P. A., Kontodimas, D. C., and Siamos, D. T. (2002). Adult morphology and life cycle under constant temperatures of the predator Rhyzobius lophanthae Blaisdell (Col., Coccinellidae). Anz. Schadl. J. Pest Sci. 75, 105–109. doi: 10.1034/j.1399-5448.2002.02033.x
Ueno, H. (2003). Genetic variation in larval period and pupal mass in an aphidophagous ladybird beetle (Harmonia axyridis) reared in different environments. Entomol. Exp. Appl. 106, 211–218. doi: 10.1046/j.1570-7458.2003.00027.x
van Lenteren, J. C., Babendreier, D., Bigler, F., Burgio, G., Hokkanen, H. M. T., Kuske, S., et al. (2003). Environmental risk assessment of exotic natural enemies used in inundative biological control. BioControl 48, 3–38. doi: 10.1023/A:1021262931608
van Lenteren, J. C., Bale, J. S., Bigler, F., Hokkanen, H. M. T., and Loomans, A. J. M. (2006). Assessing risks of releasing exotic biological control agents of arthropod pests. Annu. Rev. Entomol. 51, 609–634. doi: 10.1146/annurev.ento.51.110104.151129
van Lenteren, J. C., Loomans, A. J., Babendreier, D., and Bigler, F. (2008). Harmonia axyridis: an environmental risk assessment for Northwest Europe. BioControl 53, 37–54. doi: 10.1007/s10526-007-9120-2
Weber, D. C., and Lundgren, J. G. (2009).Assessing the trophic ecology of the Coccinellidae: their roles as predators and as prey. BioControl. 51, 199–214. doi: 10.1016/j.biocontrol.2009.05.013
Keywords: biological invasion, Europe, fecundity, invasiveness, ladybirds, rate of spread, thermal requirements
Citation: Soares AO, Honěk A, Martinkova Z, Brown PMJ and Borges I (2018) Can Native Geographical Range, Dispersal Ability and Development Rates Predict the Successful Establishment of Alien Ladybird (Coleoptera: Coccinellidae) Species in Europe? Front. Ecol. Evol. 6:57. doi: 10.3389/fevo.2018.00057
Received: 01 October 2017; Accepted: 20 April 2018;
Published: 16 May 2018.
Edited by:
Ann Valerie Hedrick, University of California, Davis, United StatesReviewed by:
Ximena J. Nelson, University of Canterbury, New ZealandAndreas Walzer, Universität für Bodenkultur Wien, Austria
Copyright © 2018 Soares, Honěk, Martinkova, Brown and Borges. This is an open-access article distributed under the terms of the Creative Commons Attribution License (CC BY). The use, distribution or reproduction in other forums is permitted, provided the original author(s) and the copyright owner are credited and that the original publication in this journal is cited, in accordance with accepted academic practice. No use, distribution or reproduction is permitted which does not comply with these terms.
*Correspondence: António O. Soares, YW50b25pby5vYy5zb2FyZXNAdWFjLnB0