- 1Department of Ecology and Evolutionary Biology, Cornell University, Ithaca, NY, United States
- 2Cornell Lab of Ornithology, Ithaca, NY, United States
Glucocorticoid hormones are important regulators of metabolic processes, and of the behavioral and physiological responses to stressors. Within-population variation in circulating glucocorticoids has been linked with both reproductive success and survival, but the presence and direction of relationships vary. Although conceptual models suggest the potential for interactions between glucocorticoid secretion under acute stress and non-acute stress contexts to influence phenotype and fitness, very little is known about the presence or implications of such interactions. Here we use a large data set from breeding tree swallows (Tachycineta bicolor; n = 215 adults) to test the predictors of reproductive success and annual survival probability in females and males over a four-year period. Across years and life history stages, glucocorticoids predicted fitness in female tree swallows. Under challenging conditions, females that maintained low baseline corticosterone levels during incubation had higher reproductive success. During the nestling provisioning period, interactions between baseline glucocorticoids and the stress response suggest that females may face trade-offs in the regulation of glucocorticoids across contexts. Reproductive success was highest among females that maintained low baseline glucocorticoids coupled with a strong acute stress response, and among females with high baseline glucocorticoids and a weak acute stress response. Females with low or high glucocorticoid levels across contexts (baseline and acute stress) fledged fewer young. Glucocorticoid levels did not predict fledging success in males. None of the models of annual survival probability in females received strong support; model comparisons suggested weak negative effects of stress responsiveness during nestling provisioning, and positive effects of body condition and age, on survival. Male survival probability was not predicted by breeding phenotype. Within and across years the glucocorticoid stress response was individually repeatable; across-year repeatability was particularly high during the nestling provisioning period. Both measures of the acute stress response—circulating stress-induced corticosterone levels and their stress-induced increase—showed similar repeatability, but baseline corticosterone was not repeatable within or across years. Overall, our results suggest that taking into account the potential for individual differences in glucocorticoid trait expression in one context to influence optimal endocrine expression in other contexts could be important for understanding the evolution of endocrine systems.
Introduction
Hormones are central regulators of phenotypic flexibility. Glucocorticoid hormones play key roles in helping organisms to prepare for and respond to challenges and changing contexts by regulating metabolism, mobilizing energy, and mediating the behavioral and physiological stress response (Sapolsky et al., 2000). Because of the diversity of important traits that they mediate, it has been widely predicted that individual differences in glucocorticoid responses could influence the ability to survive and reproduce (Wingfield et al., 1998; Angelier and Wingfield, 2013). Empirical data have, however, provided mixed support for these relationships (Breuner et al., 2008; Bonier et al., 2009a; Crespi et al., 2013). While a number of studies in free-living populations have found that variation in baseline glucocorticoid levels, or in the magnitude of the glucocorticoid stress response, predict components of fitness (Blas et al., 2007; Cabezas et al., 2007; Rivers et al., 2012; Patterson et al., 2014; Vitousek et al., 2014), both the presence and direction of these links varies widely (Angelier et al., 2009b; Bonier et al., 2009b; Riechert et al., 2014). These inconsistent patterns have raised questions about the extent to which variation in glucocorticoid regulation influences fitness.
Studies of hormone-fitness relationships typically examine the relationships between natural variation in glucocorticoid levels in free-living organisms and their fitness correlates. Hormones are, however, highly labile traits that respond rapidly to changes in environmental, social, and internal conditions (Lema and Kitano, 2013; Hau and Goymann, 2015). Circulating hormone concentrations at any point in time are therefore likely to be influenced by multiple within-individual reaction norms (Hau et al., 2016). Recent studies have indicated that individuals can differ consistently in the slope of their glucocorticoid reaction norm across specific environmental gradients (Lendvai et al., 2014), and in response to specific stimuli or changes in context (Martin and Liebl, 2014; Lendvai et al., 2015). It is not known, however, whether individual differences in the glucocorticoid response to a specific stimulus or change in context influence optimal glucocorticoid expression in other contexts. If the glucocorticoid response to one context alters optimal endocrine expression in other contexts, these trade-offs could obscure or amplify the links between endocrine traits and fitness (Taff and Vitousek, 2016). Analyses that assess the potential for interactions among glucocorticoid responses to different contexts to influence glucocorticoid-fitness relationships could help to elucidate the potential for these central mediators of phenotype to be shaped by selection.
Both theoretical predictions and empirical data suggest that single time-point measurements of baseline glucocorticoids in free-living populations may be positively or negatively associated with fitness (Bonier et al., 2009a). Because hormone levels typically rise in organisms facing major stressors, baseline glucocorticoids are classically predicted to be higher in individuals with lower fitness prospects (“cort-fitness hypothesis:” Bonier et al., 2009b; Angelier et al., 2010; Strasser and Heath, 2013). However, at relatively low concentrations glucocorticoids promote foraging behavior, and energy mobilization and use (Landys et al., 2006; Crossin et al., 2012; Ouyang et al., 2013a). If slight to moderate elevations in glucocorticoids help to sustain individuals during demanding life history stages, then glucocorticoid levels could be positively associated with fitness (“cort-adaptation hypothesis:” Bonier et al., 2009a; Rivers et al., 2012; Patterson et al., 2014).
During an acute stress response the substantial (up to 200-fold) increase in circulating glucocorticoids produces very different phenotypic effects than those produced by low glucocorticoid concentrations. At low levels, glucocorticoids bind predominantly at intracellular mineralocorticoid receptors (MR), which have a high affinity for glucocorticoids. At higher concentrations, when most MR are occupied, glucocorticoids bind predominantly at glucocorticoid receptors (GR) (Breuner and Orchinik, 2001; Romero, 2004; Lattin et al., 2012). These receptor types appear to differ in their rapid effects—at least in mammals—as well as in the ways that they change gene expression (Tasker et al., 2006; Dorey et al., 2011; Chantong et al., 2012). The effects of glucocorticoid receptor binding are believed to promote the response to and recovery from acute stressors (Sapolsky et al., 2000). Thus, individuals that mount a greater glucocorticoid response when facing major stressors are predicted to have higher survival than those that mount a weaker response (Wingfield et al., 1998; Angelier et al., 2009b; Patterson et al., 2014). However, this response can also be costly: at very high concentrations glucocorticoids can suppress reproduction (Wingfield and Sapolsky, 2003), and, during a sustained response, can impose widespread phenotypic damage (McEwen, 2012). For organisms engaged in particularly valuable reproductive attempts, or those facing chronic inescapable stressors, mounting a weaker stress response may therefore be adaptive (Cabezas et al., 2007; Bókony et al., 2009).
To our knowledge, no previous studies have investigated whether interactions between baseline glucocorticoids and their stress-induced increase predict fitness. However, the potential for context-dependent trade-offs in optimal glucocorticoid expression is consistent with some conceptual models. For example, the reactive scope model proposes a threshold over which glucocorticoids begin to cause phenotypic damage (Romero et al., 2009). Under this scenario, an acute stress response of the same magnitude may be more likely to exceed the threshold if baseline glucocorticoids are also high. Additionally, this model proposes that chronically elevated baseline hormone levels may lower the threshold over which glucocorticoids induce phenotypic damage; if so, mounting a strong stress response may be even more costly for individuals with higher baseline hormone levels than for those with lower baseline levels.
Here we use a four-year data set from breeding tree swallows (Tachycineta bicolor) to test whether individual variation in glucocorticoid levels predicts reproductive success and survival across life history stages in both males and females. To assess potential trade-offs in glucocorticoid secretion across contexts, we determined whether the baseline hormone levels associated with the highest reproductive success and survival differ depending on an individual's acute stress response. Because it is not clear whether total circulating glucocorticoid levels following acute stress (stress-induced levels) or their stress-induced increase better predict the transcriptomic, phenotypic, and fitness effects of stress we constructed separate candidate model sets that included each of these measures of the stress response. Finally, we explored the potential for selection to operate on glucocorticoid levels by estimating individual repeatability in baseline glucocorticoids, and in both measures of the glucocorticoid stress response, within and across years.
Materials and Methods
Field Data Collection
Data on the physiology, morphology, and reproductive success of female and male tree swallows were collected from 2013 to 2015 in Tompkins County, New York (~42° 29′N, 76° 27′W, 340 m elevation). This long-term study population, monitored since 1986, breeds in nest boxes provided by researchers (Winkler, 1992). Nest boxes are arranged in grids or rows, separated by ~10 m. Both occupancy rates and the total population size varies over time (Shutler et al., 2012). During the period of study, occupancy rates were ~60%, and between 272 and 343 nesting attempts were recorded during each year (only a portion of these were used for this study). Survival was assessed using capture records from 2014 to 2016. All birds were captured from inside the nest box, either by hand (blocking the entrance hole) or using an internal wig-wag trap (in which a small plastic sheet secured inside the box and attached to a length of fishing line is manually pulled across the entrance hole when the bird is inside). Traps were attended continuously during the time they were set. Birds were captured and sampled on specific days, and during a specified time window (0700–1000 h), to minimize variation in circulating hormone levels due to daily rhythms and changes across life history stages. Female tree swallows (>97% of those with active nests at the study site) were first captured during the middle of the incubation period (day 6–7). Females that remained reproductively active were again captured and sampled during the mid-nestling provisioning period (day 3–7). Males at all nests were targeted for capture during the nestling provisioning period (day 3–7); capture attempts were successful at ~72% of nests.
At each capture, blood samples were taken from the alar vein within 2.5 min (mean: 97.3 ± 1.2 s, range: 38–149 s, n = 374) of disturbance (initial approach of the bird) to measure baseline circulating corticosterone levels (the primary avian glucocorticoid), and again 30 min after initial disturbance, to measure stress-induced corticosterone. In between blood sampling events birds were maintained in a cloth bag except during the time in which they were measured. Individuals were weighed and measured using standard field protocols (Ardia, 2005). Where possible, age was determined using nestling banding records from previous years, or based on when females were recorded breeding in second-year plumage (indicating that they were first-year breeders). For females that had not previously been recorded as breeding in second-year plumage, and had not fledged from the site, minimum age was considered to be the number of years since the first breeding record, plus two. For males first recorded as adults, minimum age was estimated as the number of years since the first breeding record plus one. The status of each nest was monitored every 1–2 days from the initiation of activity at the site until nestlings were sampled and banded 12 days after hatching. To avoid inducing premature fledging, nests were not checked when nestlings were 13–21 days of age. Fledge checks were conducted 22 days after hatching to determine the fate of all nestlings alive at banding. All capture, sampling, and monitoring protocols were approved by Cornell University's Animal Care and Use Committee (IACUC protocol #2001-0051).
Corticosterone Assays
Blood samples were kept on ice until centrifugation, and plasma samples were frozen at −30°C until analysis. In 2013 and 2014, all plasma samples were assayed using a miniaturized double antibody radioimmunoassay (RIA) kit (ImmuChem Corticosterone I-RIA, MP Biomedicals: 07-120103; Palacios et al., 2007). Intra-assay variation for RIAs was 4.3%, and inter-assay variation was 8.5% (detection limits: 0.6 ng/mL). In 2015, samples were analyzed using a combination of RIA (n = 106) and enzyme immunoassay (EIA) (n = 86). In this protocol, samples were extracted using a triple ethyl acetate extraction and assayed using a corticosterone EIA kit (DetectX Corticosterone, Arbor Assays: K014-H5). Extraction efficiency, which was determined by including plasma aliquots spiked with a known amount of steroid standard prior to extraction on each plate (as described in the Arbor Assays DetectX Steroid Extraction Protocol), averaged 85.4%. Intra-assay variation was 5.4%, and inter-assay variation was 5.7% (detection limits: 0.8 ng/mL). A comparison of samples analyzed using both of these methods indicated that measured concentrations were highly correlated [(Stedman et al., 2017): r2 = 0.93, F(60, 1) = 809.3, n = 62, P < 0.0001], but total measured concentrations differed between RIA and EIA. To correct for this difference, the concentration of samples measured with EIAs were adjusted to RIA-equivalent concentrations using the equation described in Stedman et al. (2017) (CORTRIA = 1.43*CORTEIA – 0.889). To confirm that assay method did not affect model outcome we ran models of reproductive success with EIA-measured data excluded; in all cases the same best-fit model was identified in this restricted analysis, as was identified using the full data set. Assays were run annually (2013: seven RIAs, 2014: eight RIAs, 2015: five RIAs, eight EIAs). Within years, all samples from the same individual were run in the same assay. When all samples were considered together, sampling latency within the 2.5 min sampling window was positively associated with baseline corticosterone; however, the amount of the variation in baseline corticosterone levels explained by sampling latency was low [r2 = 0.035, F(1, 372) = 13.7, p < 0.001, n = 374].
Data Analyses
Data analyses were conducted using R v. 3.2.1 (R Development Core Team, 2015). Incomplete records (those missing data on any of the fixed or random effects included in any of the candidate models) were excluded from analyses of reproductive success and fitness, as were records from attempts in which either parent was involved in major manipulative studies during the year of data collection. The final data set used for fitness analyses included records from: females during incubation (n = 164 breeding records from 142 individuals), females provisioning nestlings (n = 138 breeding records from 122 individuals), and males provisioning nestlings (n = 86 breeding records from 73 individuals). We did not attempt to identify extra-pair offspring of study males; male reproductive success refers to the number of offspring fledged from the social nest only.
Predictors of clutch size and reproductive success (the number of offspring that fledged from the nest) were tested using generalized linear mixed models with a Poisson distribution and a log link function using the “glmer” function in the “lme4” package (Bates et al., 2015). Best-fit models were identified by comparing the Corrected Akaike Information Criterion (AICc) scores of all candidate models. Separate models were constructed for males and females that included ID as a random effect, and combinations of the fixed effects (continuous except where specified): year (categorical), body condition (scaled body mass index: Peig and Green, 2009), age (categorical), clutch initiation date, daily mean temperature (during incubation or nestling provisioning), baseline corticosterone, and one of the two measures of the stress response: total stress-induced hormone levels (circulating stress-induced) or their increase over baseline (stress-induced increase). It is not clear whether individual differences in the hormonal response to acute stress are better assessed by measuring the increase in glucocorticoids attributable to acute stress or total circulating concentrations under acute stress; thus, we constructed separate sets of models that included each of these metrics. Temperature variables included daily mean temperature during the entire incubation or nestling provisioning period, as recorded by Northeast Regional Climate Center stations in Tompkins County (located < 7 km from the nest boxes used in this study). These measures were included because periods of low ambient temperature can have deleterious effects on tree swallow reproductive success (Winkler et al., 2013; Ouyang et al., 2015). Candidate model sets additionally included a null model that contained the random effect only.
Because conceptual models of glucocorticoids suggest the possibility of trade-offs between elevating baseline corticosterone and maintaining a robust stress response (e.g., Romero et al., 2009), we also included candidate models that contained an interaction between baseline corticosterone and the stress response. In this data set, baseline corticosterone levels were positively correlated with stress-induced corticosterone levels [incubation: r2 = 0.04, F(1, 167) = 6.49, p = 0.012; provisioning: r2 = 0.04, F(1, 230) = 9.13, p = 0.003], but not with the stress-induced increase in corticosterone [incubation: r2 = 0.00, F(1, 167) = 0.55, p = 0.46; provisioning: r2 = 0.00, F(1, 230) = 0.08, p = 0.77]. To test whether the predictors of fitness differed across contexts, we also tested candidate models that included interactions between corticosterone and daily mean temperature, or between corticosterone and year.
Models of the predictors of clutch size, and of reproductive success that used incubation period data, included all females with active nests on incubation day 6–7. Models of the predictors of reproductive success from the mid-nestling provisioning period included data from females (or males) that still had active nests during the mid-nestling provisioning capture period only (day 3–7 after hatching). Among the females that were sampled twice, we also used the same modeling approach to test whether reproductive success was predicted by within-individual changes in corticosterone from the incubation to provisioning periods. Birds in this population are typically single-brooded; few successfully rear a second brood following nest failure. However, two females in our data set experienced nest failure during the nestling period but subsequently initiated new nests at which they successfully fledged young. The exclusion of these two individuals from analyses does not affect model outcomes.
Annual survival probabilities were estimated in Program MARK 8.1 using Cormack-Jolly-Seber (CJS) models (White and Burnham, 1999). This approach enables the estimation of both apparent survival (φ; the probability that an individual captured at time t survives and returns to the study area at time t+1), and recapture rate (p; the probability that individuals alive and present are detected). In this data set, we saw no evidence of missed detections in females (years in which birds known to have survived were not detected); thus, we constrained p = 1.0 for all analyses involving females. All other model parameters were estimated using maximum likelihood, and the relative support for candidate models evaluated using an information theoretic approach. Candidate models included the same combinations of factors used in analyses of reproductive success: year, scaled body mass index, age, clutch initiation date, daily mean temperature, baseline corticosterone, and one of the two measures of the glucocorticoid stress response. Individual covariates included in our candidate models represented values measured during an individual's first breeding record. Within each set of candidate models, the best-fit model was considered to be the model with the lowest quasi Akaike Information Criterion (QAICc) score. In females, separate sets of candidate models were constructed using data collected during incubation and provisioning, and based on the seasonal change in phenotypic traits (the change in glucocorticoid levels and body mass from incubation to provisioning period). Male survival probability was modeled using provisioning period data only.
The repeatability of baseline and stress-induced corticosterone (Cockrem, 2013), and of the stress-induced increase in corticosterone, were assessed within and across years. Analyses were restricted to females, because most males in our data set were only sampled once. Linear mixed-effects models using the restricted maximum-likelihood method (REML), with identity as a random factor, were constructed using “rptR” (Nakagawa and Schielzeth, 2010) in R 3.2.1 (R Development Core Team, 2015). Confidence intervals were estimated with parametric bootstrapping (1,000 simulation iterations). Within-year repeatability was assessed in multiply-sampled females only, as males were only captured once per season. As described above, samples were not included in this data set if they were collected following major experimental manipulations. An overall repeatability estimate was determined using all available data; a similar analysis was restricted to samples collected within the same year. To assess whether hormone repeatability across years differed between life history stages, we also generated separate estimates of across-year repeatability during incubation and nestling provisioning.
Results
Annual Differences in Temperature and Reproductive Success
Ambient temperatures differed among years. Mean daily temperatures were lowest in 2013, both during incubation (mean daily ambient temp during incubation ± SE 2013: 17.4 ± 0.2, 2014: 18.7 ± 0.2, 2015: 20.1 ± 0.2°C; Kruskal–Wallis test: χ2 = 113.7, df = 2, p < 0.0001) and during the nestling provisioning period (mean daily ambient temp ± SE 2013: 18.5 ± 0.1, 2014: 20.4 ± 0.1, 2015: 20.9 ± 0.2 °F; Kruskal–Wallis test: χ2 = 104.2, df = 2, p < 0.0001). Annual reproductive success was also lower in 2013 than in 2014 or 2015 [mean offspring fledged ± SE, 2013: 1.9 ± 0.2, 2014: 3.2 ± 0.3, 2015: 3.2 ± 0.3; One-way ANOVA: F(2, 194) = 8.3, p = 0.0003].
Clutch Size
Neither glucocorticoids nor any of the other phenotypic traits measured during incubation, or environmental factors, were significantly associated with clutch size in female tree swallows. In both candidate model sets, the best-fit model of clutch size contained clutch initiation date alone; females that laid earlier in the season had larger clutches [Intercept (Est. ± S.E.): 2.90 ± 0.71, z-value: 4.06, p < 0.0001, date: −0.009 ± 0.005, z-value = −1.67, p = 0.095; full model comparisons in Table S1].
Reproductive Success
The top two models of fledging success that used incubation period measurements had very similar AICc scores and model weight (Table 1). Both of these models contained baseline corticosterone, year, and their interaction. In 2013 only, baseline corticosterone during incubation negatively predicted reproductive success (Figure 1). One of the two best-fit models also contained the stress response and its interaction with baseline corticosterone (Tables 1, 2). In this model the highest reproductive success was seen in birds with low baseline glucocorticoids and stress responses, but this interaction was weak. Candidate model sets that included total circulating stress-induced corticosterone as a measure of the stress response identified the same best-fit models as those that instead included the stress-induced increase in corticosterone (Table 1, Tables S2, S3).
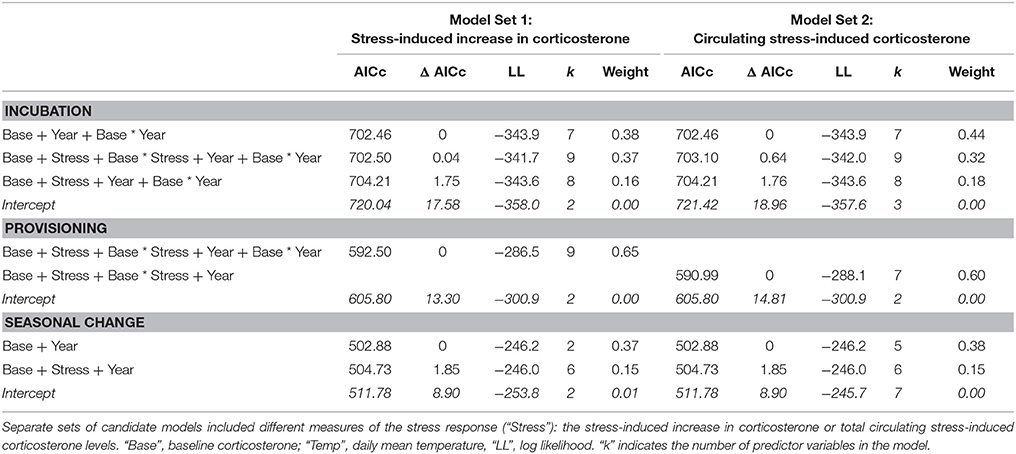
Table 1. Model comparisons of the predictors of reproductive success in female tree swallows, showing all candidate models within 2 Δ AICc of the best-fit model, and the null model.
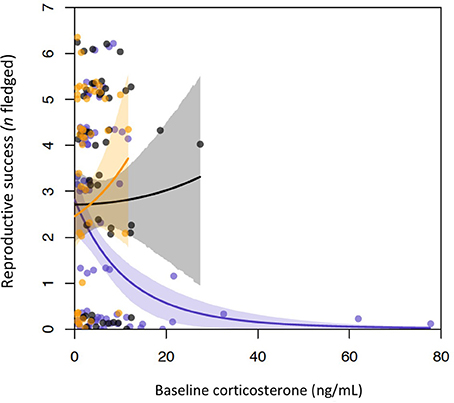
Figure 1. Baseline glucocorticoids and reproductive success by year in incubating female tree swallows. Baseline levels were negatively associated with reproductive success in 2013 (purple points and line), but not in 2014 (gray), or 2015 (orange). Lines show the relationships between baseline corticosterone and reproductive success during each year, as predicted by generalized linear mixed models.
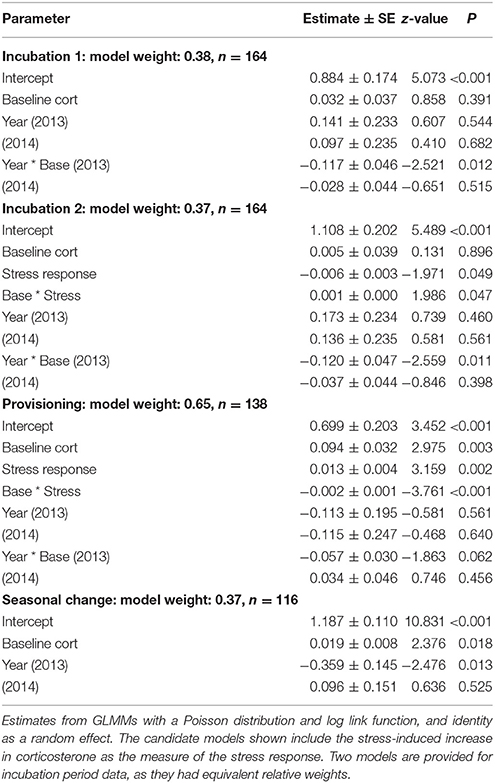
Table 2. Best-fit models of reproductive success (number of nestlings fledged) in female tree swallows.
During the nestling provisioning period, the best-fit models of reproductive success in females contained baseline corticosterone, the stress response, and their interaction (Tables 1, 2). However, an examination of the interaction revealed a different pattern than that observed during incubation (Figure 2, Figure S1). During the nestling provisioning period, females with a robust glucocorticoid stress response fledged more offspring if they maintained low baseline glucocorticoid levels. In contrast, among females with a weak acute stress response, those with higher baseline corticosterone fledged more offspring. Among the candidate models that included the stress-induced increase in corticosterone, but not among those that included total stress-induced corticosterone levels, the best-fit model also contained an interaction between baseline corticosterone and year (Table 1; Table S3). In these models, baseline corticosterone during provisioning was positively associated with reproductive success in the relatively mild years of 2014 and 2015, but not in 2013.
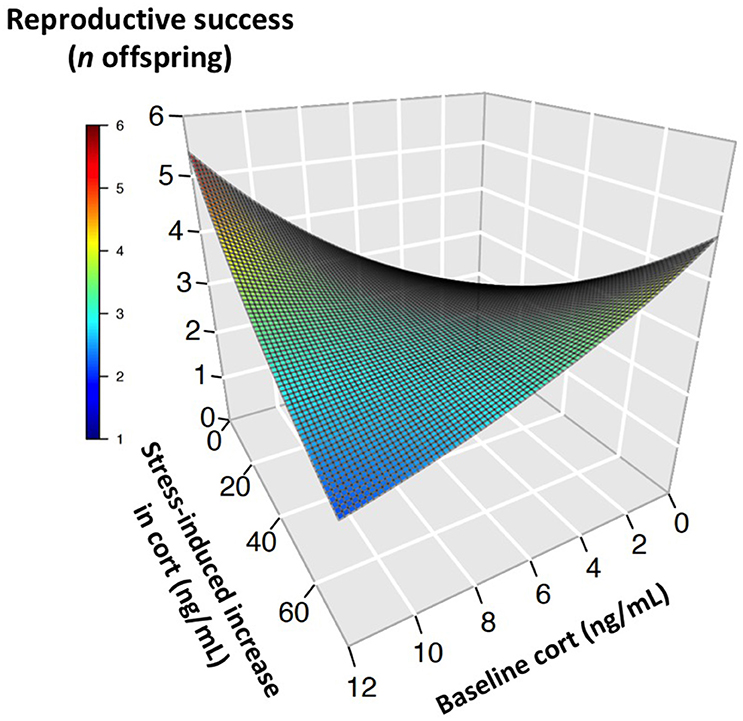
Figure 2. Glucocorticoids and reproductive success in provisioning female tree swallows. Three-dimensional surface plot illustrates the relationship between baseline corticosterone, the stress-induced increase in glucocorticoids, and the number of offspring reared to independence, as predicted by generalized linear mixed models. The highest reproductive success was seen in individuals with low baseline corticosterone coupled with a high stress-induced increase (right side of surface), or with high baseline corticosterone coupled with a low stress-induced increase (left side of surface).
Among candidate models that included the seasonal change in glucocorticoids, the best-fit model of female reproductive success included the change in baseline corticosterone, and year (Tables 1, 2). Females that increased corticosterone levels between incubation and provisioning fledged more young.
In males, the best-fit models of the number of offspring fledged from the social nest contained year alone (Table 3; Year: 2013: z-value = −2.737, p = 0.006, 2014: z-value = −0.48, p = 0.633; Intercept: 1.45 ± 0.13, z-value = 11.61, p < 0.001). The relative weight of this model was fairly low; other candidate models that received some support included those with measures of the stress response (the increase in corticosterone from acute stress or circulating stress-induced level), date, and body condition (Table 3, Table S2, Figure S2).
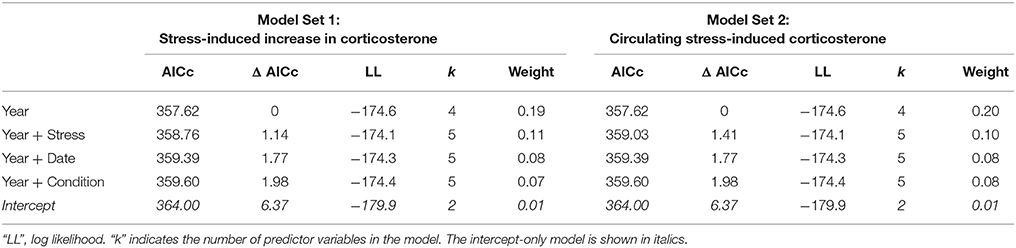
Table 3. Model comparisons of the predictors of reproductive success in male tree swallows, showing all candidate models within 2 Δ AICc of the best-fit model.
Survival
Model comparison failed to identify a best-fit model of survival probability based on female phenotypic data during provisioning (Table 4; Tables S4). Candidate models that received some support included stress responsiveness, body condition, and age, alone or in combination. The relative weight of these models was very similar, suggesting that they explained similar variation in annual survival probability. But their effect on survival probability, if any, was weak. The intercept-only model had only a slightly higher QAIC score than the top five models; however, its deviance was higher, suggesting a modest improvement in the fit of models that included stress responsiveness, body condition, and/or age over the intercept-only model. Within the set of best-fit survival models there was evidence of a weak negative relationship with stress responsiveness, and a weak positive relationship with body condition and age (Table S5).
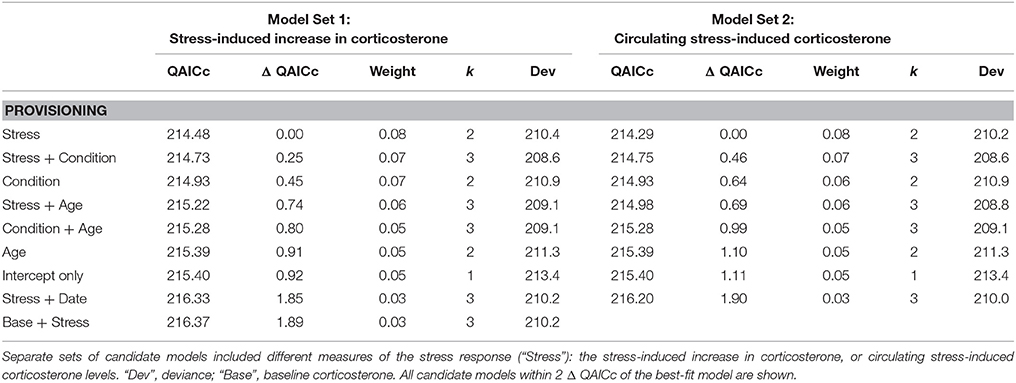
Table 4. Model comparisons of annual survival probability in female tree swallows, based on data collected during the nestling provisioning period.
Annual survival probability was not predicted by female phenotypic traits or ambient temperatures measured during incubation, or by the seasonal change in female phenotype. Similarly, in males, survival probability was not predicted by phenotypic or environmental measures during the nestling provisioning period. In all these cases, the null model was the best fit to the data (Table S4).
Glucocorticoid Repeatability
Overall estimates of repeatability, using samples collected across years, indicate that baseline corticosterone was not repeatable, but both measures of the stress response were significantly repeatable (Table 5; Figure 3). Restricting analyses to samples collected in the same year only yielded similar estimates (baseline: R = 0.04 [95%CI = 0–0.20], p = 0.25, n = 251; corticosterone increase to acute stress: R = 0.21 [0.03–0.38], p = 0.019, n = 238; circulating stress-induced corticosterone: R = 0.29 [0.13–0.44], p = 0.003, n = 260). Across-year repeatability in the stress response differed by life history stage; glucocorticoid repeatability was higher during nestling provisioning than during incubation (Table 5).
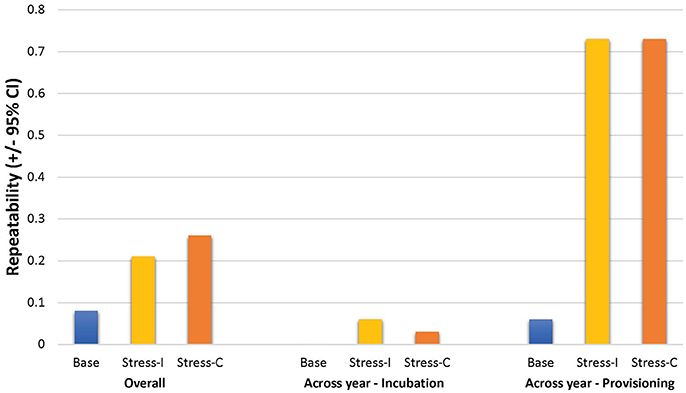
Figure 3. Estimates of glucocorticoid repeatability in female tree swallows. Bar chart shows overall repeatability (all available samples), and across-year repeatability during the incubation and provisioning periods for baseline corticosterone (“Base”), the stress-induced increase in corticosterone (“Stress-I”) and circulating stress-induced levels (“Stress-C”).
Discussion
Our results indicate that individual variation in glucocorticoids significantly predicts some components of fitness in tree swallows, but in a complex and context-dependent manner. Glucocorticoid levels associated with the highest fitness differed across life history stages, and between males and females. Most notably, we find that in females, the baseline glucocorticoid levels associated with highest reproductive success differed according to stress responsiveness. The glucocorticoid stress response was repeatable within and across years, but baseline glucocorticoid levels were not repeatable.
Reproductive Success
Across the reproductive period, individual variation in glucocorticoid levels predicted reproductive success in female tree swallows. This pattern did not result from differences in clutch size—models of clutch size found no significant relationship with glucocorticoid levels. Instead, as has been demonstrated previously, female tree swallows that began nesting earlier in the season produced larger clutches (Stutchbury and Robertson, 1988; Winkler and Allen, 1996). Lay date was not, however, retained in the top models of reproductive success.
The nature of the relationship between female glucocorticoids and reproductive success differed across life history stages. During incubation, reproductive success was predicted by an interaction between baseline corticosterone and year. In 2013, when several strong cold snaps reduced food availability and fledging success, baseline corticosterone levels were higher overall (Figure 1). During this year, females with elevated baseline corticosterone during incubation fledged fewer young. This relationship was not apparent during the other, more moderate years, when baseline corticosterone levels were lower. The interaction between baseline corticosterone and year was retained in both of the two top models in this set, which had equivalent relative weights; one also contained the stress response, and an interaction between baseline corticosterone and the stress response. In this model, females with low stress responses and low baseline corticosterone levels during incubation had the highest reproductive success; however, this interaction was weak. A negative association between incubation period glucocorticoid levels and reproductive success could result from glucocorticoids causally affecting parental investment and offspring phenotype. For example, acute or chronic elevations in glucocorticoids can impair parental care (Angelier et al., 2009a; Vitousek et al., 2014), and influence yolk compound deposition (Bowers et al., 2016). Direct, deleterious effects of glucocorticoids on reproduction may be greater during poor environmental conditions, such as in 2013, when baseline glucocorticoid levels of tree swallows at our study site were higher. Alternatively, glucocorticoid-fitness relationships may not result from glucocorticoid-mediated effects on performance and fitness. For example, baseline glucocorticoids could be elevated in individuals facing more severe challenges (Bonier and Martin, 2016), or in individuals with a reduced capacity to cope with these challenges.
During the nestling provisioning period, the relationship between baseline glucocorticoids and reproductive success differed depending on how a female responded to acute stress. Stronger stress responders fledged more young if they also had low baseline corticosterone levels; weaker stress responders fared better if they had high baseline corticosterone. This pattern could be explained in several ways. First, if much of the variation in baseline corticosterone is driven by context or condition, then this relationship could indicate that during provisioning, a weaker stress response is favored under challenging conditions (when baseline corticosterone is high), whereas a stronger stress response is favored under better conditions (when baseline corticosterone is low). Because of the negative effects of high glucocorticoids on reproduction, mounting a strong stress response is widely predicted to negatively impact reproductive success (Wingfield and Sapolsky, 2003; Lendvai et al., 2007; Breuner et al., 2008; Bókony et al., 2009). Within populations, negative relationships between early season glucocorticoid responses and subsequent reproductive success have been found in several songbirds (Patterson et al., 2014; Vitousek et al., 2014). The costs of mounting a strong glucocorticoid response are, however, predicted to be higher when baseline glucocorticoids, and other mediators of stress, are elevated (Romero et al., 2009). Under less challenging conditions, the benefit of mounting a stronger glucocorticoid stress response could outweigh the costs—for example, if stronger responders are better able to defend their nests from predators, or respond more effectively to fluctuations in environmental conditions (Angelier and Wingfield, 2013).
A second explanation for the interaction between baseline glucocorticoids and the acute stress response lies in the role of baseline glucocorticoids in promoting parental care. Several studies in birds have found that moderate elevations in baseline glucocorticoids can promote parental care (Bonier et al., 2009b; Crossin et al., 2012; Ouyang et al., 2013a; Patterson et al., 2014). In this study, a positive relationship between baseline corticosterone and reproductive success was seen during mild years. Thus, the observed variation in baseline glucocorticoids could also reflect differing levels of parental investment or nestling demand. In this case, the significant interaction could indicate a trade-off between glucocorticoid-mediated reproductive strategies: reproduction could benefit from either an up-regulation of baseline corticosterone (promoting nestling feeding) or the maintenance of a strong acute stress response—which could, for example, facilitate nest defense or the response to environmental challenges. Under this scenario, it may not be possible to effectively maximize both strategies, resulting in a trade-off between glucocorticoid-mediated reproductive strategies across contexts.
Several recent studies have suggested that within-individual changes in hormone levels may also be important predictors of reproductive performance and reproductive success (Bonier et al., 2009b; Ouyang et al., 2013b). In this study, females whose baseline corticosterone levels increased between incubation and provisioning fledged more young. This pattern, which has also been found in another population of tree swallows (Bonier et al., 2009b), further supports the hypothesis that increases in baseline corticosterone—when coupled with a low acute stress response—may support the energetic demands of provisioning nestlings.
While we focus here on identifying glucocorticoid levels associated with the highest fitness, individuals may differ in their optimal level of trait expression due to differences in their genetic makeup, environmental or social context, or constraints imposed by the expression of other traits (Angelier and Wingfield, 2013; Hau et al., 2016; Taff and Vitousek, 2016). Thus, individuals that are not displaying glucocorticoid levels associated with the highest fitness could still be regulating glucocorticoid expression in a way that maximizes their own individual fitness (Bonier and Martin, 2016). Future studies that address the potential for variable relationships between hormones and fitness, and the complexities of interacting reaction norms and shifting environmental contexts, are needed in order to clarify the complex and multidimensional relationships between glucocorticoids and fitness.
Male tree swallows do contribute to parental care, but their contribution is less than that provided by females. Incubation is done by females alone; males are active participants in offspring provisioning, but provision at lower rates than do females (Ardia, 2007). In contrast to the significant relationships between corticosterone and reproductive success in females, male corticosterone levels were unrelated to within-pair fledging success. The best-fit models of male fledging success contained year alone. Birds that reproduced during the cold and highly variable year of 2013 fledged significantly fewer young from their social nests than those that reproduced during other years. Thus, for males, the number of offspring fledged from the social nest appears to be more strongly influenced by external conditions than by internal physiological or endocrine state. This is consistent with other studies in tree swallows that have found that for males, within-pair reproductive investment and success appear to be particularly sensitive to external factors, including weather and the condition of their social mate (Lessard et al., 2014; Ouyang et al., 2015). It is important to note, however, that tree swallows have high rates of extra-pair paternity (~50% in this population over the years of study: Hallinger et al., in review). Thus, the number of offspring fledged from a male's social nest is likely to be a relatively poor predictor of the total number of his genetic offspring that survive to fledging. Future analyses that address the genetic reproductive success of males (Lessard et al., 2014) will be necessary to determine the relative roles of glucocorticoids, and other male traits, in predicting fitness.
For all analyses, year and interactions between year and glucocorticoids were more strongly associated with reproductive success than ambient temperatures during a given reproductive attempt. Previous analyses in this population indicate that across years, ambient temperature strongly predicts both insect availability and annual reproductive success (Winkler et al., 2013). However, it is possible that the relevant timescale over which ambient temperature affects reproductive performance is longer than what was measured here. It is also possible that other unmeasured environmental factors influence reproductive success, alone or in combination with temperature (e.g., wind speed or precipitation: Ouyang et al., 2015; Irons et al., 2017). Finally, year effects could better predict reproductive success because, unlike ambient temperature, they incorporate other longer-term sources of variation, including the social environment, predator pressure, carry-over effects of conditions experienced during migration, or even global weather patterns that affect swallows on their non-breeding grounds.
Survival
In females, survival probability was not predicted by incubation period phenotype or environmental conditions, or by seasonal change in phenotype. During the nestling provisioning period, there was only very weak support for relationships between phenotype and survival probability. Models that included stress responsiveness, body condition, or age, alone or in combination, received slightly more support than the null model, but the relative weight of each of the six models that performed slightly better than the null model were very low.
The top ranked model of annual survival probability (which had a Δ QAICc of 0.41 from the second-ranked model) included a negative relationship with female stress responsiveness during nestling provisioning. Although mounting a strong stress response is generally predicted to increase the likelihood of survival (Wingfield et al., 1998; Breuner et al., 2008; Hau et al., 2010), the cost of mounting a glucocorticoid response to stimuli that do not pose an immediate survival threat (e.g., many social stressors: Sapolsky, 2010) or to inescapable threats (e.g., widespread food shortage: Romero and Wikelski, 2010), may outweigh the benefits. A negative relationship between the stress response and survival is seen in European white stork (Ciconia ciconia), which have high population densities, engage in frequent social interactions, and experience a relatively low risk of predation (Blas et al., 2007). During their short breeding season tree swallows often nest close together, engaging in frequent social interactions. Swallows experience much higher population densities throughout the non-breeding season, as they over-winter in large, mobile roosts along the Gulf Coast that contain up to a million individuals (Winkler, 2006). Under these conditions, mounting a strong stress response—which is unlikely to help individuals respond effectively to the social interactions that can trigger it (Gesquiere et al., 2011; Deviche et al., 2014)—could be disadvantageous. In contrast, populations at lower density, and those that face a higher risk of predation, more often show a positive relationship between the glucocorticoid stress response and survival (Cabezas et al., 2007; Angelier et al., 2009b; Patterson et al., 2014, but see Rivers et al., 2012).
In the other models that received slightly more support than the null model, survival probability was positively associated with both body condition and age. Body condition is often predictive of survival probability in birds (Blas et al., 2007; Rivers et al., 2012). A positive relationship between survival and age could be driven by individual differences in quality or life history strategy that result in some individuals exhibiting markedly longer lifespans than others. Note, however, that there was very weak support for these relationships. Models that included baseline corticosterone were a poorer fit to survival data than the null model—this pattern is consistent with findings of a weak or nonexistent effect of baseline corticosterone on return rate in other tree swallow populations (Bonier et al., 2009b; Madliger and Love, 2016; Ouyang et al., 2016).
In males, there was no support for a relationship between phenotype and survival probability. The null model was the best fit to the data. Sex differences in the predictors of survival could be driven by differences in life history. Male tree swallows over-winter in the same roosts as females, and thus are expected to face similar selective pressures during the non-breeding season. However, they return to the breeding site in the spring up to several weeks before females return. During this period, they are frequently exposed to severe and unpredictable weather; male mortality is quite common during the pre-breeding period in our study population. If mounting a more robust stress response helps males to cope with unpredictable weather events during the pre-breeding period, then it is conceivable that this benefit could counteract the cost of such a response on the wintering grounds, resulting in no detectable relationship between the stress response and inter-annual survival. A future study that addresses whether male glucocorticoid responses influence the likelihood of surviving inclement weather—or other defined challenges—could help shed light on this question.
It is important to note that although our analyses accounted for missed detections of returning birds, we were unable to distinguish between mortality and dispersal in non-returning birds. Tree swallows have high breeding site philopatry: on average, 96% of surviving males and 86% of females breed at the same location in the following year (Winkler et al., 2004). Thus, the large majority of non-returning birds are likely to have died. Nevertheless, some of the non-returning birds in this study likely dispersed to other locations in subsequent years. It is not known whether glucocorticoid levels are associated with dispersal probability in tree swallows, although this relationship has been found in some other taxa (Belthoff and Dufty, 1998; Vercken et al., 2007). Future studies that experimentally address the links between glucocorticoids and natal or breeding dispersal could help to elucidate the mediators of dispersal, and clarify glucocorticoid-fitness relationships.
Repeatability
Repeatability analyses indicated very different patterns in baseline glucocorticoids and the acute stress response. The glucocorticoid stress response was significantly repeatable within and across years; across-year repeatability of both measures of the stress response was higher during nestling provisioning than during incubation. These results, together with the significant heritability of glucocorticoid stress responses in this population (Stedman et al., 2017), suggest that selection could be operating on the acute stress response in tree swallows. In contrast, baseline glucocorticoids were not repeatable within or across years. Although some of the variation in this trait appears to be heritable in tree swallows (Stedman et al., 2017), this highly plastic trait is also known to respond rapidly to changes in internal and external context. Thus, while the acute stress response appears to be a relatively consistent aspect of phenotype, variation in baseline glucocorticoids may better reflect the conditions or context of an individual.
These results are consistent with the higher repeatability of stress-induced glucocorticoids than baseline glucocorticoids across taxa (Taff et al., 2018). This pattern could indicate true biological differences in the stability of these traits within individuals, or may simply reflect differences in stimulus control between sample types: baseline measurements in free-living populations include little to no control over the stimuli experienced prior to capture, whereas the primary stimulus influencing stress-induced glucocorticoid concentrations (restraint) is relatively standardized (Taff et al., 2018).
Measuring the Stress Response
This study compared two measures of the stress response: the corticosterone increase induced by acute stress, and circulating stress-induced corticosterone levels. Both measures of change (e.g., Addis et al., 2011; Rensel and Schoech, 2011; Patterson et al., 2014) and total circulating levels (Angelier et al., 2009a; Patterson et al., 2014; Vitousek et al., 2014) are widely reported measures of the stress response. At present there is no clear consensus as to which type of measurement better characterizes variation in the hormonal stress response, although arguments have been made in both directions (Romero, 2004; Cockrem et al., 2009; Patterson et al., 2014; Hau et al., 2016). The influence of glucocorticoids on gene expression will depend on whether they bind to MR or GR, and where those receptors are located. The binding of available receptors is determined by circulating glucocorticoid concentrations (e.g., Breuner and Orchinik, 2001; Lattin et al., 2012); thus, circulating stress-induced levels may better indicate the downstream effects of glucocorticoids than their stress-induced increase over baseline. However, if receptor expression or distribution is functionally linked with baseline levels (or their stress-induced increase), then the change in glucocorticoids in response to acute stress could better predict downstream impacts on gene expression. Variation in receptor expression has been linked with both baseline and stress-induced glucocorticoid levels in mammals (e.g., Harris et al., 2013), but few studies have addressed these relationships in birds. Among those that have, baseline glucocorticoid levels have not predicted MR or GR expression in the brain (Liebl et al., 2013; Krause et al., 2015; Cornelius et al., 2018). To our knowledge, the only study to date to have looked at the response to restraint stress in birds found that variation in the stress-induced increase in glucocorticoids is predicted by MR expression in the hippocampus in house sparrows (Liebl et al., 2013). This study did not, however, contrast this measure with circulating stress-induced levels. It is also important to note that GR and MR expression can differ substantially across tissues within individuals (Lattin et al., 2015); thus, the same hormone concentration may have very different impacts on gene expression in different target tissues.
Because of this uncertainty, we analyzed relationships between both measures of the stress response and components of fitness here. A previous study in this population of tree swallows found that the increase in corticosterone triggered by acute stress had higher heritability than circulating stress-induced levels, and was less strongly impacted by developmental environment (Stedman et al., 2017). However, in this analysis both measures of the glucocorticoid stress response showed equivalent repeatability, and were similarly associated with both reproductive success and survival. Future work that probes the relationships between individual variation in hormone levels and receptor densities and distributions could shed light on this question, as will studies that address whether absolute circulating levels or their stress-induced increases better predict the transcriptomic, phenotypic, and fitness effects of stress.
Summary and Conclusions
In female tree swallows, glucocorticoids predicted reproductive success, and possibly survival, but these relationships were complex and context-dependent. Particularly during nestling provisioning, females may face trade-offs between elevating baseline glucocorticoid levels, and mounting a robust acute stress response. The presence or implications of such trade-offs have received very little attention, but could have major implications for how glucocorticoids systems—and the multitude of traits that they mediate—evolve. Determining the potential for interactions between different components of HPA activity to influence performance and fitness is important for revealing how constraints operate on phenotypic flexibility, and how endocrine traits are shaped by selection. While these analyses identify the glucocorticoid phenotypes associated with the highest fitness across a population, individuals may differ in circulating hormone levels, as well as in their own optima, due to a variety of intrinsic and extrinsic factors. Because of the complexity of the environments inhabited by free-living organisms such questions will not be easy to address, but will require carefully designed studies that probe how the context-dependent expression of endocrine traits influences performance and fitness across different environments. As a first step toward this goal, researchers can begin to assess interactions among baseline glucocorticoids, the response to acute stress, and the efficacy of negative feedback, as these traits are widely measured in free-living populations. However, it is important to remember that each of these components of HPA activity can show reaction norms of expression in response to environmental gradients or contexts (reviewed in Taff and Vitousek, 2016). Gaining a true understanding of how endocrine traits evolve in multidimensional environments will therefore require assessing the interacting effects of components of glucocorticoid responses across a diversity of contexts and environmental gradients.
Author Contributions
MV: conceived of and designed the study; MV, KH, CT, and CZ: collected data; CT, CZ, and MV: conducted hormone assays; CT, MV, and KH: analyzed the data; DW: provided important logistical support, and aided in the interpretation of data; MV: wrote the paper. All authors contributed to manuscript revision, and approved the final version of the manuscript for submission.
Funding
This work was funded by NSF IOS 1457251 and Cornell University (to MV) and by NSF LTREB DEB-0717021 (to DW).
Conflict of Interest Statement
The authors declare that the research was conducted in the absence of any commercial or financial relationships that could be construed as a potential conflict of interest.
Acknowledgments
We thank Jocelyn Stedman, David Scheck, Austin Huffmann, Teresa Pegan, Alyssa Rodriguez, and the many members of the tree swallow research team for field and laboratory work, Laura Schoenle and the Vitousek Lab for helpful discussions, and Fran Bonier for feedback on a previous draft. We are also grateful to Ned Place, Betty Hansen, and Steve Lamb at Cornell's Diagnostic Endocrinology Laboratory for conducting radioimmunoassays.
Supplementary Material
The Supplementary Material for this article can be found online at: https://www.frontiersin.org/articles/10.3389/fevo.2018.00042/full#supplementary-material
References
Addis, E. A., Davis, J. E., Miner, B. E., and Wingfield, J. C. (2011). Variation in circulating corticosterone levels is associated with altitudinal range expansion in a passerine bird. Oecologia 167, 369–378. doi: 10.1007/s00442-011-2001-5
Angelier, F., Clément-Chastel, C., Welcker, J., Gabrielsen, G. W., and Chastel, O. (2009a). How does corticosterone affect parental behaviour and reproductive success? A study of prolactin in black-legged kittiwakes. Funct. Ecol. 23, 784–793. doi: 10.1111/j.1365-2435.2009.01545.x
Angelier, F., Holberton, R. L., and Marra, P. P. (2009b). Does stress response predict return rate in a migratory bird species? A study of American redstarts and their non-breeding habitat. Proc. R. Soc. B Biol. Sci. 276, 3545–3551. doi: 10.1098/rspb.2009.0868
Angelier, F., and Wingfield, J. C. (2013). Importance of the glucocorticoid stress response in a changing world: theory, hypotheses and perspectives. Gen. Comp. Endocrinol. 190, 118–128. doi: 10.1016/j.ygcen.2013.05.022
Angelier, F., Wingfield, J. C., Weimerskirch, H., and Chastel, O. (2010). Hormonal correlates of individual quality in a long-lived bird: a test of the “corticosterone-fitness hypothesis.” Biol. Lett. 6, 846–849. doi: 10.1098/rsbl.2010.0376
Ardia, D. R. (2005). Individual quality mediates trade-offs between reproductive effort and immune function in tree swallows: individual quality and immune function. J. Anim. Ecol. 74, 517–524. doi: 10.1111/j.1365-2656.2005.00950.x
Ardia, D. R. (2007). Site- and sex-level differences in adult feeding behavior and its consequences to offspring quality in tree swallows (Tachycineta bicolor) following brood-size manipulation. Can. J. Zool. 85, 847–854. doi: 10.1139/Z07-070
Bates, D., Maechler, M., Bolker, B., and Walker, S. (2015). Fitting linear mixed-effects models using lme4. J. Stat. Softw. 67, 1–48. doi: 10.18637/jss.v067.i01
Belthoff, J. R., and Dufty, A. M. (1998). Corticosterone, body condition, and locomotor activity: a model for dispersal in screech-owls. Anim. Behav. 55, 405–415. doi: 10.1006/anbe.1997.0625
Blas, J., Bortolotti, G. R., Tella, J. L., Baos, R., and Marchant, T. A. (2007). Stress response during development predicts fitness in a wild, long lived vertebrate. Proc. Natl. Acad. Sci. U.S.A. 104, 8880–8884. doi: 10.1073/pnas.0700232104
Bókony, V., Lendvai, Á. Z., Liker, A., Angelier, F., Wingfield, J. C., and Chastel, O. (2009). Stress response and the value of reproduction: are birds prudent parents? Am. Nat. 173, 589–598. doi: 10.1086/597610
Bonier, F., and Martin, P. R. (2016). How can we estimate natural selection on endocrine traits? Lessons from evolutionary biology. Proc. R. Soc. B Biol. Sci. 283:20161887. doi: 10.1098/rspb.2016.1887
Bonier, F., Martin, P. R., Moore, I. T., and Wingfield, J. C. (2009a). Do baseline glucocorticoids predict fitness? Trends Ecol. Evol. 24, 634–642. doi: 10.1016/j.tree.2009.04.013
Bonier, F., Moore, I. T., Martin, P. R., and Robertson, R. J. (2009b). The relationship between fitness and baseline glucocorticoids in a passerine bird. Gen. Comp. Endocrinol. 163, 208–213. doi: 10.1016/j.ygcen.2008.12.013
Bowers, E. K., Bowden, R. M., Thompson, C. F., and Sakaluk, S. K. (2016). Elevated corticosterone during egg production elicits increased maternal investment and promotes nestling growth in a wild songbird. Horm. Behav. 83, 6–13. doi: 10.1016/j.yhbeh.2016.05.010
Breuner, C. W., and Orchinik, M. (2001). Seasonal regulation of membrane and intracellular corticosteroid receptors in the house sparrow brain. J. Neuroendocrinol. 13, 412–420. doi: 10.1046/j.1365-2826.2001.00646.x
Breuner, C. W., Patterson, S. H., and Hahn, T. P. (2008). In search of relationships between the acute adrenocortical response and fitness. Gen. Comp. Endocrinol. 157, 288–295. doi: 10.1016/j.ygcen.2008.05.017
Cabezas, S., Blas, J., Marchant, T. A., and Moreno, S. (2007). Physiological stress levels predict survival probabilities in wild rabbits. Horm. Behav. 51, 313–320. doi: 10.1016/j.yhbeh.2006.11.004
Chantong, B., Kratschmar, D. V., Nashev, L. G., Balazs, Z., and Odermatt, A. (2012). Mineralocorticoid and glucocorticoid receptors differentially regulate NF-kappaB activity and pro-inflammatory cytokine production in murine BV-2 microglial cells. J. Neuroinflammation 9:1. doi: 10.1186/1742-2094-9-260
Cockrem, J. F. (2013). Corticosterone responses and personality in birds: individual variation and the ability to cope with environmental changes due to climate change. Gen. Comp. Endocrinol. 190, 156–163. doi: 10.1016/j.ygcen.2013.02.021
Cockrem, J. F., Barrett, D. P., Candy, E. J., and Potter, M. A. (2009). Corticosterone responses in birds: individual variation and repeatability in Adelie penguins (Pygoscelis adeliae) and other species, and the use of power analysis to determine sample sizes. Gen. Comp. Endocrinol. 163, 158–168. doi: 10.1016/j.ygcen.2009.03.029
Cornelius, J. M., Perreau, G., Bishop, V. R., Krause, J. S., Smith, R., Hahn, T. P., et al. (2018). Social information changes stress hormone receptor expression in the songbird brain. Horm. Behav. 97, 31–38. doi: 10.1016/j.yhbeh.2017.10.002
Crespi, E. J., Williams, T. D., Jessop, T. S., and Delehanty, B. (2013). Life history and the ecology of stress: how do glucocorticoid hormones influence life-history variation in animals? Funct. Ecol. 27, 93–106. doi: 10.1111/1365-2435.12009
Crossin, G. T., Trathan, P. N., Phillips, R. A., Gorman, K. B., Dawson, A., Sakamoto, K. Q., et al. (2012). Corticosterone predicts foraging behavior and parental care in Macaroni Penguins. Am. Nat. 180, E31–E41. doi: 10.1086/666001
Deviche, P., Beouche-Helias, B., Davies, S., Gao, S., Lane, S., and Valle, S. (2014). Regulation of plasma testosterone, corticosterone, and metabolites in response to stress, reproductive stage, and social challenges in a desert male songbird. Gen. Comp. Endocrinol. 203, 120–131. doi: 10.1016/j.ygcen.2014.01.010
Dorey, R., Piérard, C., Shinkaruk, S., Tronche, C., Chauveau, F., Baudonnat, M., et al. (2011). Membrane mineralocorticoid but not glucocorticoid receptors of the dorsal hippocampus mediate the rapid effects of corticosterone on memory retrieval. Neuropsychopharmacology 36, 2639–2649. doi: 10.1038/npp.2011.152
Gesquiere, L. R., Learn, N. H., Simao, M. C. M., Onyango, P. O., Alberts, S. C., and Altmann, J. (2011). Life at the top: rank and stress in wild male baboons. Science 333, 357–360. doi: 10.1126/science.1207120
Harris, A. P., Holmes, M. C., de Kloet, E. R., Chapman, K. E., and Seckl, J. R. (2013). Mineralocorticoid and glucocorticoid receptor balance in control of HPA axis and behavior. Psychoneuroendocrinol 38, 648–658. doi: 10.1016/j.psyneuen.2012.08.007
Hau, M., Casagrande, S., Ouyang, J. Q., and Baugh, A. T. (2016). Glucocorticoid-mediated phenotypes in vertebrates: multilevel variation and evolution. Adv. Study Behav. 48, 1–75. doi: 10.1016/bs.asb.2016.01.002
Hau, M., and Goymann, W. (2015). Endocrine mechanisms, behavioral phenotypes and plasticity: known relationships and open questions. Front. Zool. 12:1. doi: 10.1186/1742-9994-12-S1-S7
Hau, M., Ricklefs, R. E., Wikelski, M., Lee, K. A., and Brawn, J. D. (2010). Corticosterone, testosterone and life-history strategies of birds. Proc. R. Soc. B Biol. Sci. 277, 3203–3212. doi: 10.1098/rspb.2010.0673
Irons, R. D., Harding Scurr, A., Rose, A. P., Hagelin, J. C., Blake, T., and Doak, D. F. (2017). Wind and rain are the primary climate factors driving changing phenology of an aerial insectivore. Proc. R. Soc. B Biol. Sci. 284:20170412. doi: 10.1098/rspb.2017.0412
Krause, J. S., McGuigan, M. A., Bishop, V. R., Wingfield, J. C., and Meddle, S. L. (2015). Decreases in mineralocorticoid but not glucocorticoid receptor mRNA expression during the short arctic breeding season in free-living Gambel's white-crowned sparrow (Zonotrichia leucophrys gambelii). J. Neuroendocrinol. 27, 66–75. doi: 10.1111/jne.12237
Landys, M. M., Ramenofsky, M., and Wingfield, J. C. (2006). Actions of glucocorticoids at a seasonal baseline as compared to stress-related levels in the regulation of periodic life processes. Gen. Comp. Endocrinol. 148, 132–149. doi: 10.1016/j.ygcen.2006.02.013
Lattin, C. R., Bauer, C. M., de Bruijn, R., and Romero, L. M. (2012). Hypothalamus-pituitary-adrenal axis activity and the subsequent response to chronic stress differ depending upon life history stage. Gen. Comp. Endocrino. 178, 494–501. doi: 10.1016/j.ygcen.2012.07.013
Lattin, C. R., Keniston, D. E., Reed, J. M., and Romero, L. M. (2015). Are receptor concentrations correlated across tisuses within individuals? A case study examining glucocorticoid and mineralocorticoid receptor binding. Endocrinol 156, 1354–1361. doi: 10.1210/en.2014-1811
Lema, S. C., and Kitano, J. (2013). Hormones and phenotypic plasticity: implications for the evolution of integrated adaptive phenotypes. Curr. Zool. 59, 506–525. doi: 10.1093/czoolo/59.4.506
Lendvai, Á. Z., Giraudeau, M., Bókony, V., Angelier, F., and Chastel, O. (2015). Within-individual plasticity explains age-related decrease in stress response in a short-lived bird. Biol. Lett. 11:20150272. doi: 10.1098/rsbl.2015.0272
Lendvai, A. Z., Giraudeau, M., and Chastel, O. (2007). Reproduction and modulation of the stress response: an experimental test in the house sparrow. Proc. R. Soc. B Biol. Sci. 274, 391–397. doi: 10.1098/rspb.2006.3735
Lendvai, Á. Z., Ouyang, J. Q., Schoenle, L. A., Fasanello, V., Haussmann, M. F., Bonier, F., et al. (2014). Experimental food restriction reveals individual differences in corticosterone reaction norms with no oxidative costs. PLoS ONE 9:e110564. doi: 10.1371/journal.pone.0110564
Lessard, A., Bourret, A., Bélisle, M., Pelletier, F., and Garant, D. (2014). Individual and environmental determinants of reproductive success in male tree swallow (Tachycineta bicolor). Behav. Ecol. Sociobiol. 68, 733–742. doi: 10.1007/s00265-014-1686-y
Liebl, A. L., Shimizu, T., and Martin, L. B. (2013). Covariation among glucocorticoid regulatory elements varies seasonally in house sparrows. Gen. Comp. Endocrinol. 183, 32–37. doi: 10.1016/j.ygcen.2012.11.021
Madliger, C. L., and Love, O. P. (2016). Do baseline glucocorticoids simultaneously represent fitness and environmental quality in a declining aerial insectivore? Oikos 125, 1824–1837. doi: 10.1111/oik.03354
Martin, L. B., and Liebl, A. L. (2014). Physiological flexibility in an avian range expansion. Gen. Comp. Endocrinol. 206, 227–234. doi: 10.1016/j.ygcen.2014.07.016
McEwen, B. S. (2012). Brain on stress: how the social environment gets under the skin. Proc. Natl. Acad. Sci. U.S.A. 109, 17180–17185. doi: 10.1073/pnas.1121254109
Nakagawa, S., and Schielzeth, H. (2010). Repeatability for Gaussian and non-Gaussian data: a practical guide for biologists. Biol. Rev. 85, 935–956. doi: 10.1111/j.1469-185X.2010.00141.x
Ouyang, J., Lendvaiá, Z., Dakin, R., Domalik, A., Fasanello, V., Vassallo, B., et al. (2015). Weathering the storm: parental effort and experimental manipulation of stress hormones predict brood survival. BMC Evol. Biol. 15:219. doi: 10.1186/s12862-015-0497-8
Ouyang, J. Q., Lendvai, A. Z., Moore, I. T., Bonier, F., and Haussmann, M. F. (2016). Do hormones, telomere lengths, and oxidative stress form an integrated phenotype? A case study in free-living tree swallows. Integr. Comp. Biol. 56, 138–145. doi: 10.1093/icb/icw044
Ouyang, J. Q., Muturi, M., Quetting, M., and Hau, M. (2013a). Small increases in corticosterone before the breeding season increase parental investment but not fitness in a wild passerine bird. Horm. Behav. 63, 776–781. doi: 10.1016/j.yhbeh.2013.03.002
Ouyang, J. Q., Sharp, P., Quetting, M., and Hau, M. (2013b). Endocrine phenotype, reproductive success and survival in the great tit, Parus major. J. Evol. Biol. 26, 1988–1998. doi: 10.1111/jeb.12202
Palacios, M. G., Cunnick, J. E., Winkler, D. W., and Vleck, C. M. (2007). Immunosenescence in some but not all immune components in a free-living vertebrate, the tree swallow. Proc. R. Soc. B Biol. Sci. 274, 951–957. doi: 10.1098/rspb.2006.0192
Patterson, S. H., Hahn, T. P., Cornelius, J. M., and Breuner, C. W. (2014). Natural selection and glucocorticoid physiology. J. Evol. Biol. 27, 259–274. doi: 10.1111/jeb.12286
Peig, J., and Green, A. J. (2009). New perspectives for estimating body condition from mass/length data: the scaled mass index as an alternative method. Oikos 118, 1883–1891. doi: 10.1111/j.1600-0706.2009.17643.x
R Development Core Team (2015). R: A Language and Environment for Statistical Computing. Vienna: R Foundation for Statistical Computing.
Rensel, M. A., and Schoech, S. J. (2011). Repeatability of baseline and stress-induced corticosterone levels across early life stages in the Florida scrub-jay (Aphelocoma coerulescens). Horm. Behav. 59, 497–502. doi: 10.1016/j.yhbeh.2011.01.010
Riechert, J., Becker, P. H., and Chastel, O. (2014). Predicting reproductive success from hormone concentrations in the common tern (Sterna hirundo) while considering food abundance. Oecologia 176, 715–727. doi: 10.1007/s00442-014-3040-5
Rivers, J. W., Liebl, A. L., Owen, J. C., Martin, L. B., and Betts, M. G. (2012). Baseline corticosterone is positively related to juvenile survival in a migrant passerine bird. Funct. Ecol. 26, 1127–1134. doi: 10.1111/j.1365-2435.2012.02025.x
Romero, L. M. (2004). Physiological stress in ecology: lessons from biomedical research. Trends Ecol. Evol. 19, 249–255. doi: 10.1016/j.tree.2004.03.008
Romero, L. M., Dickens, M. J., and Cyr, N. E. (2009). The reactive scope model — A new model integrating homeostasis, allostasis, and stress. Horm. Behav. 55, 375–389. doi: 10.1016/j.yhbeh.2008.12.009
Romero, L. M., and Wikelski, M. (2010). Stress physiology as a predictor of survival in Galapagos marine iguanas. Proc. R. Soc. B Biol. Sci. 277, 3157–3162. doi: 10.1098/rspb.2010.0678
Sapolsky, R. M. (2010). “Stress, health and social behavior,” in Encyclopedia of Animal Behavior, ed M. D. Breed and J. Moore (London: Elsevier), 350–357.
Sapolsky, R. M., Romero, L. M., and Munck, A. U. (2000). How do glucocorticoids influence stress responses? Integrating permissive, suppressive, stimulatory, and preparative actions. Endocr. Rev. 21, 55–89. doi: 10.1210/er.21.1.55
Shutler, D., Hussell, D. J. T., Norris, D. R., Winkler, D. W., Robertson, R. J., Bonier, F., et al. (2012). Spatiotemporal patterns in nest box occupancy by tree swallows across North America. Avian Cons. Ecol. 7:3. doi: 10.5751/ACE-00517-070103
Stedman, J. M., Hallinger, K. K., Winkler, D. W., and Vitousek, M. N. (2017). Heritable variation in circulating glucocorticoids and endocrine flexibility in a free-living songbird. J. Evol. Biol. 30, 1724–1735. doi: 10.1111/jeb.13135
Strasser, E. H., and Heath, J. A. (2013). Reproductive failure of a human-tolerant species, the American kestrel, is associated with stress and human disturbance. J. Appl. Ecol. 50, 912–919. doi: 10.1111/1365-2664.12103
Stutchbury, B. J., and Robertson, R. J. (1988). Within-season and age-related patterns of reproductive performance in female tree swallows (Tachycineta bicolor). Can. J. Zool. 66, 827–834. doi: 10.1139/z88-122
Taff, C. C., Schoenle, L. A., and Vitousek, M. N. (2018). The repeatability of glucocorticoids: a review and meta-analysis. Gen. Comp. Endocrinol. 260, 136–145. doi: 10.1016/j.ygcen.2018.01.011
Taff, C. C., and Vitousek, M. N. (2016). Endocrine flexibility: optimizing phenotypes in a dynamic world? Trends Ecol. Evol. 31, 476–488. doi: 10.1016/j.tree.2016.03.005
Tasker, J. G., Di, S., and Malcher-Lopes, R. (2006). Rapid glucocorticoid signaling via membrane-associated receptors. Endocrinology 147, 5549–5556. doi: 10.1210/en.2006-0981
Vercken, E., de Fraipont, M., Dufty, A. M., and Clobert, J. (2007). Mother's timing and duration of corticosterone exposure modulate offspring size and natal dispersal in the common lizard (Lacerta vivipara). Horm. Behav. 51, 379–386. doi: 10.1016/j.yhbeh.2006.12.006
Vitousek, M. N., Jenkins, B. R., and Safran, R. J. (2014). Stress and success: individual differences in the glucocorticoid stress response predict behavior and reproductive success under high predation risk. Horm. Behav. 66, 812–819. doi: 10.1016/j.yhbeh.2014.11.004
White, G. C., and Burnham, K. P. (1999). Program MARK: survival estimation from populations of marked animals. Bird Study 46, 120–139. doi: 10.1080/00063659909477239
Wingfield, J. C., Maney, D. L., Breuner, C. W., Jacobs, J. D., Lynn, S., Ramenofsky, M., et al. (1998). Ecological bases of hormone—behavior interactions: the “emergency life history stage.” Am. Zool. 38, 191–206.
Wingfield, J. C., and Sapolsky, R. M. (2003). Reproduction and resistance to stress: when and how. J. Neuroendocrinol. 15, 711–724. doi: 10.1046/j.1365-2826.2003.01033.x
Winkler, D. W. (1992). Causes and consequences of variation in parental defense behavior by tree swallows. Condor 94, 502–520. doi: 10.2307/1369222
Winkler, D. W., and Allen, P. E. (1996). The seasonal decline in tree swallow clutch size: physiological constraint or strategic adjustment? Ecology 77, 922–932. doi: 10.2307/2265512
Winkler, D. W., Luo, M. K., and Rakhimberdiev, E. (2013). Temperature effects on food supply and chick mortality in tree swallows (Tachycineta bicolor). Oecologia 173, 129–138. doi: 10.1007/s00442-013-2605-z
Keywords: corticosterone, endocrine flexibility, evolutionary endocrinology, fitness, glucocorticoids, reproductive success, stress response, survival
Citation: Vitousek MN, Taff CC, Hallinger KK, Zimmer C and Winkler DW (2018) Hormones and Fitness: Evidence for Trade-Offs in Glucocorticoid Regulation Across Contexts. Front. Ecol. Evol. 6:42. doi: 10.3389/fevo.2018.00042
Received: 15 August 2017; Accepted: 27 March 2018;
Published: 13 April 2018.
Edited by:
Carlos Alonso Alvarez, Consejo Superior de Investigaciones Científicas (CSIC), SpainReviewed by:
Pierre Bize, University of Aberdeen, United KingdomPierre J. Deviche, Arizona State University, United States
Christine Lattin, Yale University, United States
Copyright © 2018 Vitousek, Taff, Hallinger, Zimmer and Winkler. This is an open-access article distributed under the terms of the Creative Commons Attribution License (CC BY). The use, distribution or reproduction in other forums is permitted, provided the original author(s) and the copyright owner are credited and that the original publication in this journal is cited, in accordance with accepted academic practice. No use, distribution or reproduction is permitted which does not comply with these terms.
*Correspondence: Maren N. Vitousek, bW52NkBjb3JuZWxsLmVkdQ==