- 1Plants and Cropping Systems in Horticulture (INRA), Avignon, France
- 2Dipartimento di Fisica, Università di Parma, Parma, Italy
- 3Genetics and Improvement of Fruit and Vegetables (INRA), Avignon, France
- 4CAPES Foundation, Ministry of Education of Brazil, Brasília, Brazil
Brown rot, triggered by Monilinia spp., causes significant economic losses in fruit crop and it is mainly controlled by chemicals with inherent environmental costs. Controlling brown rot spreading by diminishing fruit susceptibility to the disease, via sustainable cultural practices, is a promising approach. In a 2 years experiment (2014–2015) on a peach (Prunus persica) orchard, we controlled fruit growth rates by varying the fruit load. Fruit thinning practices enhanced the fruit growth and laboratory analyses showed that, in both 2014 and 2015 samples, fast growing fruits were more susceptible to infection when in contact with conidia suspension of Monilinia laxa. In the field, brown rot infection took place in 2014 and not in 2015. In 2014, trees subject to moderate thinning intensities had the highest brown rot incidence. We argue that this is due to the fact that, for null thinning, slow growing fruits are less susceptible to the infection while, for intense thinning, even if faster growing fruits are more susceptible to infection, the lower fruits density reduces per-contact probability of infection. We compared meteorological data of 2014 and 2015 and we argue that brown rot did not spread in 2015 due to an absence of favorable conditions, summarized as the number of rainy days with mean temperature between 22 and 26°C, in the period of fruit susceptibility.
Introduction
Brown rot caused by Monilinia spp. is one of the main diseases in stone fruits. It affects peach (Prunus persica) trees all around the world (Oliveira et al., 2016) and it is responsible of fruit rotting, and consequent crop losses, during the pre and post-harvest period (Lichou et al., 2003; Larena et al., 2005; Bussi et al., 2015). The control of brown rot is fundamental to preserve peach production and it is usually based on the use of chemicals with negative consequences on the environment and possibly on the human health (Larena et al., 2005). However, there is increasing evidence that brown rot incidence can be reduced also by sustainable agricultural practices in substitution or addition to the use of chemicals (Mercier et al., 2008; Gibert et al., 2009; Bussi et al., 2015).
Monilinia spp. overwinter in twig cankers or rotten fruits over the tree or the orchard ground. Conidial spores, which germinate from the mummies in spring when favorable environmental conditions are met, represent the primary inoculum for the infection. Primary inoculum spores are disseminated within the tree crown and in the orchard by rain and air currents (Byrde and Willetts, 1977; Van Leeuwen et al., 2000). The spores that successfully infect a fruit reproduce and generate secondary inoculum (Biggs and Northover, 1985) that will cause new infections (Corbin, 1963; Byrde and Willetts, 1977). Infection occurs when the fungus succeeds in passing through the fruit cuticular surface via stomata, lenticels, wounds or cuticle cracks (Byrde and Willetts, 1977; Xu and Robinson, 2000; Gibert et al., 2009) and reaches the fruit pulp. Cuticle cracks can represent more than 10% of the fruit surface area at ripeness (Gibert et al., 2007) and provide straightforward ways for fungal infection (Nguyen-the, 1991; Fourie and Holz, 2003).
In the present work we use field and laboratory data to test if (i) fruits that experienced different growth rates show different susceptibility to brown rot infection in laboratory conditions and if (ii) possible differences in fruit susceptibility are associated with different brown rot incidence in the field. Eventually, since in the experimental orchard brown rot infestation took place in 2014 but not in 2015, we perform an exploratory data analysis to investigate if the absence of infestation in 2015 were due to internal (e.g., a different susceptibility of fruits) or external (e.g., meteorological conditions) differences.
Materials and Methods
Experimental Orchard and Sampling Design
We performed a 2-year (2014–2015) experiment on a peach orchard (cultivar Magic, grafted onto GF677 rootstock) planted in 2006 with 4 m spacing between rows and trees. The orchard was located at the INRA Centre of Avignon (southeastern France: 43°9′N, 4°8′E) and it consisted of 5 rows of 10 trees each. Yet, in 2014 only 43 trees were left. From 2013, trees were not treated with fungicide after bud burst and they were subject to different thinning intensities (TI) in order to alter the fruit/leaf ratio and obtain different fruit growth rates (Fishman and Genard, 1998). We thinned the trees at Julian day (JD) 136 in the year 2014 and at JD 153 in 2015. With the term Julian Day of a given year, we refer to the number of days passed from the 1st of January of the considered year. In thinned trees, we left 3 and 6 fruits per meter of fruit bearing shoot (FBS), respectively in the case of intense and moderate thinning intensity. In the case of null thinning, a FBS bore on average 25 fruits per meter. In 2014 we applied three levels of TI (null, moderate and intense) while in 2015 we applied only two levels of TI (null and intense). Apart from TI and absence of fungicide applications, we managed the trees according to normal commercial practices with respect to winter pruning, fertilization, irrigation and insecticide application.
We chose 18 trees in the orchard, excluding those on the border sides and those which displayed low vigor at the beginning of the vegetative season. We weekly monitored the status (i.e., symptomless or infected) and the size of 3,310 fruits (770 in 2014 and 2,540 in 2015) born on selected 1 year-old FBS (5 FBS per tree in 2014 and 10 FBS per tree in 2015) from JD 100 to JD 196 in 2014, and from JD 124 to 198 in 2015. Trees flowered on JD 58 and JD 74, and the harvest occurred on the JD 196 and JD 209, respectively in 2014 and 2015. In Tables A1, A2 (Supplementary Materials), we report details of the monitored trees (thinning intensity, length of the monitored shoots, initial number of fruits, etc.). In the Supporting Information A3 we depict the spatial plan of the orchard (See Figures A6, A7 in Supplementary Materials).
Laboratory Analyses
We used the strain Ml3 of Monilinia laxa to test in the laboratory fruit susceptibility to infection. We took the strain from an apricot fruit mummy in March 2011 at the INRA station of Gotheron (southeastern France: 43°9′N, 4°8′E). We isolated a mono-spore and stored it at−20°C. We kept the fungus in a glycerol solution in aliquots of 45 μl and we multiplied it by transplanting aliquots on a petri dish containing V8 agar, medium based on vegetable juice, 2 weeks before conducting the infection experiment that required a spore suspension. We counted the number of spores of a dilution 1/10 of the mother solution on a Malassez cell under a microscope to estimate the spore concentration of the suspension. We verified the spore viability for each suspension on a PDA culture medium.
We tested the fruit susceptibility to brown rot infection on 633 and 917 fruits, respectively in 2014 and 2015. We measured cheek fruit diameter (FD), we disinfected fruits in a water bath at 55°C for 40 s. We put the fruits in acrylic plastic boxes placed in a growth chamber (8 h of darkness at 18°C and 16 h of light at 24°C) and we deposed a 10 μl drop of a suspension of M. laxa at 105 spores ml−1 on each fruit. We guaranteed high humidity (relative humidity of 100%) in the boxes by adding cups of water and closing them. We recorded the infection status (healthy or infected) of each fruit after 6 days.
Statistical Analysis
We estimated the average growth rate of fruits, subject to different thinning treatments, via a linear regression of observed FD vs. fruit age. We tested for differences in fruits growth rates among treatments by analyzing the differences in the slope coefficient of the linear regressions using a randomization test procedure (Hesterberg et al., 2003). See Supporting Information A1.1 for details. In addition, to test the difference in fruits growth rate among treatments, we tested for slope coefficients difference of the linear regressions FD vs. fruit age using the correct statistical test (CST) for the equality of regression coefficients (Paternoster et al., 1998). To be sure that possible differences in fruit growth rates were due to thinning treatments, we tested for differences in fruit sizes, between thinning treatments, at different sampling dates using the non-parametric Wilcoxon-Mann Whitney test for non-paired samples (Bauer, 1972) and the parametric two samples t-Student test for non-paired samples (Rice, 2006).
We assumed the probability P of a fruit of being susceptible to brown rot infection to be a logistic function of the FD with maximum value equal to one:
where a is a shape parameter and b is a semi-saturation constant. We assessed these two parameters in 2014 and 2015 samples by minimizing the sum of squared errors SSE between the probability of being susceptible predicted by the model (Equation 1) and those observed in the laboratory.
In the 2014 season, when the infection took place in the orchard, we tested for difference in the brown rot incidence among thinning treatments, at different times, using a randomization test procedure. See Supporting Information A1.2 for details.
We assumed that brown rot can spread if favorable meteorological conditions are met in the period of fruit susceptibility. We assumed a fruit to be susceptible to infection from the time ti at which P(FD) = 0.1, with FD assumed to be a linear function of time, until the time of fruit harvest tH. We assumed as favorable those days when precipitations occurred and mean temperature was comprehended between 22 and 26°C in accordance to previous studies indicating that conidia reproduction preferably occurs in wet conditions (Tamm and Flückiger, 1993; Xu and Robinson, 2000; Gell et al., 2008; Holb, 2008) and that it is impaired at “extreme” temperatures that are likely to be met in those days with mean daily temperature < 22 or >26°C (Tamm and Flückiger, 1993; Holb, 2008). Eventually we computed how many favorable days for brown rot spreading occurred in 2014 and 2015, (i.e., number of rainy days with mean temperature between 22 and 26°C in the time period ti-th).
Results
Thinning Practices and Fruit Growth
Temporal variation of FD is well described by a linear model (Figure 1), for all thinning treatments (r2 = 0.92, 0.93, 0.94, respectively for null, moderate and intense TI in 2014; and r2 = 0.81 and 0.83 for null and intense TI in 2015). Growth rates of FD varied between (4.76–5.67) × 10−2 cm day−1 in 2014 and (5.13–6.24) × 10−2 cm day−1 in 2015. In 2014, the randomization test indicate that fruits on thinned trees (both moderate and intense TI) grew faster than on non-thinned ones (p < 0.01) while there was no significant difference between moderate and intense TI (p = 0.21). Also the correct CST for the equality of regression coefficients confirmed a difference in the fruit growth rates: thinned trees (both moderate and intense TI) grew faster than non-thinned ones (P < 0.01) and intensively thinned ones grew faster than moderately thinned ones (P < 0.01). Similarly, in 2015, fruits on thinned trees grew faster than non-thinned ones (P < 0.01 for both randomization and CST). Differences in the average FD between thinned and non-thinned trees became evident 2 weeks after the thinning date (JD 136 in 2014 and JD 153 in 2015, see Figures A1, A2 in Supplementary Materials) (P < 0.01 for both wilcox and t-test) (See Figures A3, A4 in Supplementary Materials). We found no significant difference between moderate and intense TI in 2014 (See Figure A3 in Supplementary Materials).
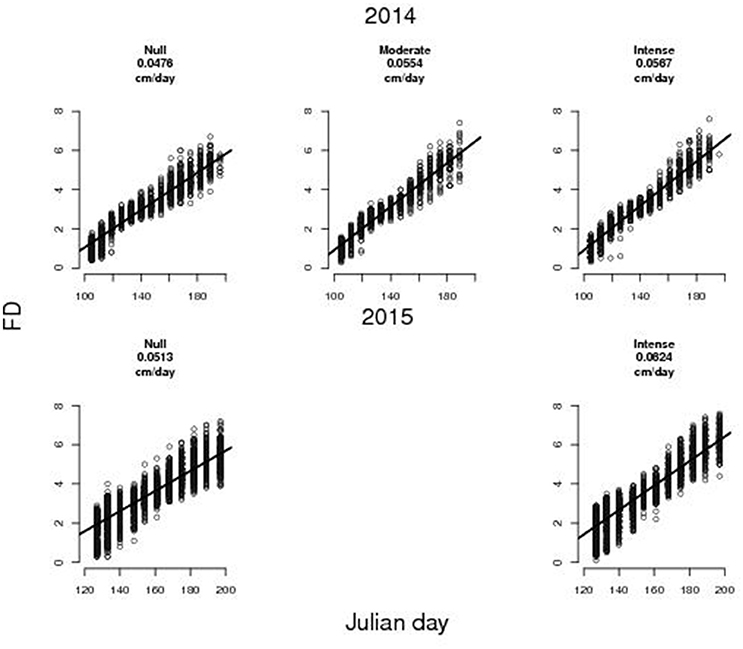
Figure 1. Observed fruit diameters (FD) at different sampling dates relevant to trees subject to different thinning treatments (i.e., null, moderate, and intense thinning intensity, that correspond to leaving almost 25, 6, and 3 fruits per meter of fruit bearing shoot). The black line represents the linear regression of the FD (cm) vs. Julian Day. Estimated average fruit growth rate in diameter is reported in each panel.
Fruit Size and Susceptibility to Brown Rot Infection in the Laboratory
The logistic function (parameters a = −1.3, b = 6.1 for the 2014 and a = −1.5, b = 5.95 for the 2015) well explained the variability in the fraction of susceptible fruits as a function of FD with a probability of being susceptible >10% for FD equal to 4.4 and 4.8 cm in the 2014 and 2015. According to the calibrated growth curves, such critical sizes had been attained at ti = 175 and ti = 185, respectively in 2014 and 2015. Accordingly, resistant fruits had significantly lower FD (non-paired Wilcoxon-Mann Whitney test, p < 0.001 in both 2014 and 2015) than susceptible ones (3.83 vs. 5.02 cm in 2014 and 3.28 vs. 4.7 cm in 2015). Frequency density distributions of resistant and susceptible fruits as a function of FD are reported in Figure 2.
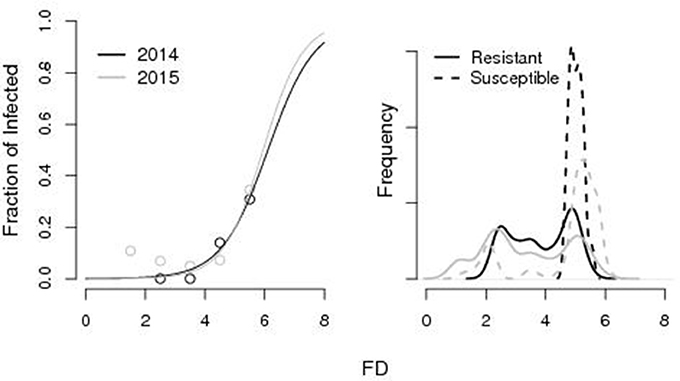
Figure 2. Results from laboratory infections. Left: Fraction of susceptible fruits as a function of the fruit diameter (FD). Right: Frequency density distribution of resistant and susceptible fruits as a function of the FD (cm). The pattern is well described by the logistic function [1 + ea(b−FD)]−1 with parameters a = −1.3, b = 6.1 for the 2014 and a = −1.5, b = 5.95 for the 2015.
Brown Rot Incidence in the Field
Temporal variations of the brown rot incidence in fruits subject to different TI are reported in Figure 3 (See Figure A5 in Supplementary Materials). First infected fruits were observed at JD 170, 19th June, in both years, but the disease spread in 2014 while it did not in 2015. In 2014 almost any fruit was infected at the harvest time but, during the growth season, temporal dynamics of brown rot incidence differed between thinning treatments with higher prevalence in trees subject to moderate TI (P < 0.01). No difference was found between the null and the intense TI (p = 0.2) and difference was not highly significant between the moderate and intense thinning (p = 0.08). In 2015 brown rot did not spread and its incidence was lower than 8%, all over the season, whatever the TI.
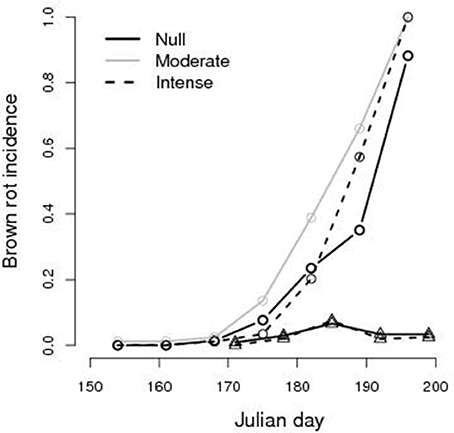
Figure 3. Brown rot incidence (fraction of infected fruits) on monitored fruits of peach Prunus persica trees, during the vegetative season after the date of thinning (e.g., JD 136 for 2014 and JD 153 for 2015), in an orchard non-treated with fungicide. Circles: 2014 data; triangles: 2015 data.
According to our criteria for brown rot spreading, we identified 9 favorable days in the period 173-196 JD in 2014 and none in the period 185–209 in 2015 according to the meteorological records reported in Figure 4.
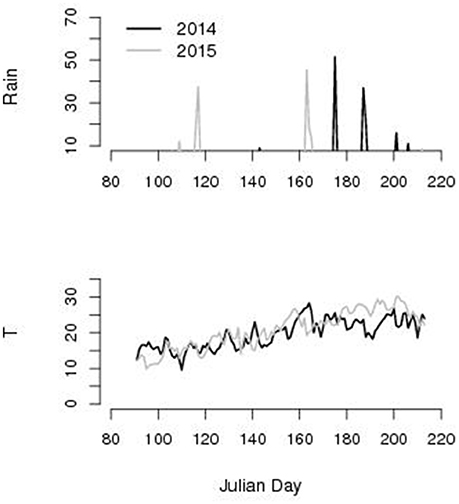
Figure 4. Rain (mm) and average daily temperature T (°C) along Julian day left column. We found 9 favorable days in 2014 and 0 in 2015 for brown rot spreading (rainy and 22 < T < 26°C) for the two seasons experiment during the window of fruits susceptibility (JD 173–198 in 2014 and 185–209 in 2015).
Discussion
Our results confirm the efficacy of fruit load control practices in affecting fruit growth rates. This is not surprising and in accordance with previous findings, not only on peaches, showing that a lower crop load releases fruit competition for resources and induces higher growth rates in the remaining fruits (Burge et al., 1987; DeJong and Grossman, 1995; Berman and DeJong, 1997; Miras-Avalos et al., 2013).
The analysis of laboratory data indicated that fast growing and bigger fruits are more susceptible to brown rot infection. Our results are in agreement with those obtained by Gibert et al. (2009) who found a relationship between the fruit size and its susceptibility to brown rot infection. In the case of high growth rates, it is likely that the pulp expansion rate exceeds the cuticle expansion rate (Gibert et al., 2005) and results in cuticular cracking which makes fruits more susceptible to the brown rot infection. A similar pattern seems to hold for different fruit tree species (Fourie and Holz, 2003) as pre-harvest cuticle cracks, due to rapid cuticular cells expansion, have been suggested to facilitate the infection by Monilinia spp. also in the sweet cherry (Børve and Sekse, 2000); and a positive relationship between the crack surface and the susceptibility to fungal disease has been documented in prune (Michailides and Morgan, 1997) and litchis (Underhill and Simons, 1993).
However, the analysis of field data indicated that brown rot incidence during the growing season is maximum in moderately thinned trees and not in the intensively thinned ones, which display the highest growth rates. Such an apparently counterintuitive result can be explained by the epidemiological theory (see Madden et al., 2007 for an overview on plant disease epidemics). In fact, the probability of a fruit to become infected, and infectious, is a joint probability of being susceptible to the infection, which depends on the fruit growth rate, and to be exposed to the pathogen, which depend on the abundance and proximity of infectious fruits. On the one side, increasing TI generates fruits with higher cuticle crack surface, but, on the other side, it decreases fruit density and augment average distance among fruits hence decreasing the probability of a fruit to be exposed to secondary inoculum. Such a conceptual scheme is graphically scratched in Figure 5. However, the consequences of a given value of TI on the effective yield should be observed in experimental orchards with all the trees subject to the same TI as simulated in Bevacqua et al. (2017) Since the laboratory data showed that fruits were susceptible to infection also in the year 2015 (see Figure 2) and the thinning treatments were similar to those of the previous year, we argue that the brown rot did not spread in 2015 due to the absence of favorable environmental conditions for spore germination and reproduction. The indicator of favorable conditions for brown rot spreading that we proposed gives a value of 9 in 2014 and 0 in 2015 hence providing a rough quantification of the difference in the disease risk in the 2 years. Similar indicators are very useful as they can help in reducing fungicide treatments by concentrating them in the most critical periods or they can be used to foresee unexpected consequences of possible climate changes (Launay et al., 2014). In order to test the validity of such an indicator one would need several years of records of temperature, precipitations and brown rot prevalence in orchards not treated with fungicide. In present work we simply searched for a possible reason explaining the absence of infection in 2015. Although the definition of a novel indicator of brown rot risk was out of the aim of present work, we are confident that our result can inspire further research.
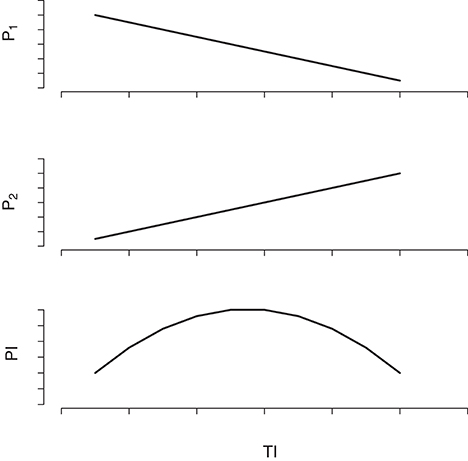
Figure 5. Qualitative scratch of the effect of the thinning intensity (TI) on the probability of infection (PI) of the host fruit. The probability P1 that an airborne spore produced by an infectious fruit is deposited on a susceptible fruit decreases with TI that decreases fruit density; the probability P2 that a fruit in contact with Monilinia spp. spores develops infection increases with TI since lower fruit density determines higher fruit growth rates and cuticle cracking; the probability of an healthy fruit to become infectious (PI) results from the product P1 × P2 that is a concave function of TI.
Author Contributions
MB: analyzed data, conceived and wrote the paper; BQ-T: performed lab experiment and wrote the paper; DB: performed experiment, analyzed data, conceived, and wrote the paper; LO: lab analyses.
Funding
This research was supported by the CLIF ACCAF and ECOVERGER projects.
Conflict of Interest Statement
The authors declare that the research was conducted in the absence of any commercial or financial relationships that could be construed as a potential conflict of interest.
Acknowledgments
This work has been partly carried out as part of the INRA Metaprogramme ACCAF (project 429 CLIF) and the ECOVERGER project funded by ONEMA within the call APR “resistance et 430 pesticides” managed by MEDDE French Ministry. This research was supported by the CLIF ACCAF project. We thank Signoret V., Leon I., and Tran A. for field work. We are grateful to the IEUMR1114 team for taking care of the experimental orchard.
Supplementary Material
The Supplementary Material for this article can be found online at: https://www.frontiersin.org/articles/10.3389/fevo.2017.00170/full#supplementary-material
References
Bauer, D. F. (1972). Constructing confidence rank sets using statistics. J. Am. Stat. Assoc. 67, 687–690. doi: 10.1080/01621459.1972.10481279
Berman, M. E., and DeJong, T. M. (1997). Crop load and water stress effects on daily stem growth in peach (Prunus persica). Tree Physiol. 17, 467–472. doi: 10.1093/treephys/17.7.467
Bevacqua, D., Quilot-Turion, B., and Bolzoni, L. (2017). A model for temporal dynamics of brown rot spreading in fruit orchard. Phytopatology doi: 10.1094/PHYTO-07-17-0250-R. [Epub ahead of print].
Biggs, A. R., and Northover, J. (1985). Inoculum sources for Monilinia fructicola in Ontario peach orchards. Can. J. Plant Pathol. 7, 302–307. doi: 10.1080/07060668509501695
Børve, J., and Sekse, L. (2000). Cuticular fractures promote postharvest fruit rot in sweet cherries. Plant Dis. 84, 1180–1184. doi: 10.1094/PDIS.2000.84.11.1180
Burge, G. K., Spence, C. B., and Marshall, R. R. (1987). Kiwifruit: effects of thinning on fruit size, vegetative growth, and return bloom. New Zeal. J. Exp. Agric. 15, 317–324. doi: 10.1080/03015521.1987.10425577
Bussi, C., Plenet, D., Merlin, F., Guillermin, A., and Mercier, V. (2015). Limiting brown rot incidence in peach with tree training and pruning. Fruits 70, 303–309. doi: 10.1051/fruits/2015030
Byrde, R. J. W., and Willetts, H. J. (1977). Brown Rot Fungi of Fruit: Their Biology and Control. Oxford: Pergamon Press.
Corbin, J. (1963). Factors determining the length of the incubation period of Monilinia fructicola (wint.) honey in fruits of Prunus spp. Aust. J. Agric. Res. 14, 51–60. doi: 10.1071/AR9630051
DeJong, T. M., and Grossman, Y. L. (1995). Quantifying sink and source limitations on dry matter partitioning to fruit growth in peach trees. Physiol. Plant. 95, 437–443. doi: 10.1111/j.1399-3054.1995.tb00860.x
Fishman, S., and Genard, M. (1998). A biophysical model of fruit growth: simulation of seasonal and diurnal dynamics of mass. Plant Cell Environ. 21, 739–752. doi: 10.1046/j.1365-3040.1998.00322.x
Fourie, R. H., and Holz, G. (2003). Germination of dry, airborne conidia of Monilinia laxa and disease expression on plum fruit. Australas. Plant Pathol. 32, 19–25. doi: 10.1071/AP02066
Gell, I., De Cal, A., Torres, R., Usall, J., and Melgarejo, P. (2008). Relationship between the incidence of latent infections caused by Monilinia spp. and the incidence of brown rot of peach fruit: factors affecting latent infection. Eur. J. Plant Pathol. 121, 487–498. doi: 10.1007/s10658-008-9268-3
Gibert, C., Chadœuf, J., Nicot, P., Vercambre, G., Genard, M., and Lescourret, F. (2009). Modelling the effect of cuticular crack surface area and inoculum density on the probability of nectarine fruit infection by Monilinia laxa. Plant Pathol. 58, 1021–1031. doi: 10.1111/j.1365-3059.2009.02121.x
Gibert, C., Chadœuf, J., Vercambre, G., Génard, M., and Lescourret, F. (2007). Cuticular cracking on nectarine fruit surface: spatial distribution and development in relation to irrigation and thinning. J. Am. Soc. Hortic. Sci. 132, 583–591.
Gibert, C., Lescourret, F., Génard, M., Vercambre, G., and Pérez Pastor, A. (2005). Modelling the effect of fruit growth on surface conductance to water vapour diffusion. Ann. Bot. 95, 673–683. doi: 10.1093/aob/mci067
Hesterberg, T., Moore, D. S., Monaghan, S., Clipson, A., and Epstein, R. (2003). Bootstrap Methods and Permutation Tests. New York, NY: W. H. Freeman.
Holb, I. J. (2008). Monitoring conidial density of Monilinia fructigena in the air in relation to brown rot development in integrated and organic apple orchards. Eur. J. Plant Pathol. 120, 397–408. doi: 10.1007/s10658-007-9233-6
Larena, I., Torres, R., De Cal, A., Liñán, M., Melgarejo, P., Domenichini, P., et al. (2005). Biological control of postharvest brown rot (Monilinia spp.) of peaches by field applications of Epicoccum nigrum. Biol. Control 32, 305–310. doi: 10.1016/j.biocontrol.2004.10.010
Launay, M., Caubel, J., Bourgeois, G., Huard, F., Cortazar-Atauria, I. G., de Bancal, M.-O., et al. (2014). Climatic indicators for crop infection risk: application to climate change impacts on five major foliar fungal diseases in Northern France. Agric. Ecosyst. Environ. 197, 147–158. doi: 10.1016/j.agee.2014.07.020
Lichou, J., Mandrin, J. F., Bréniaux, D., Mercier, V., Giauque, P., Desbrus, D., et al. (2003). Une nouvelle moniliose. Monilia fructicola s'attaque aux arbres fruitiers à noyaux. Phytoma Défense Végétaux 547, 22–25.
Madden, L. V., Hughes, G., and van den Bosch, F. (2007). The Study of Plant Disease Epidemics. St. Paul, MN.
Mercier, V., Bussi, C., Plenet, D., and Lescourret, F. (2008). Effects of limiting irrigation and of manual pruning on brown rot incidence in peach. Crop Prot. 27, 678–688. doi: 10.1016/j.cropro.2007.09.013
Michailides, T. J., and Morgan, D. P. (1997). Influence of fruit-to-fruit contact on the susceptibility of French prune to infection by Monilinia fructicola. Plant Dis. 81, 1416–1424.
Miras-Avalos, J., Alcobendas, R., Alarcón, J., Pedrero, F., Valsesia, P., Lescourret, F., et al. (2013). Combined effects of water stress and fruit thinning on fruit and vegetative growth of a very early-maturing peach cultivar: assessment by means of a fruit tree model, QualiTree. Irrig. Sci. 31, 1039–1051. doi: 10.1007/s00271-012-0385-6
Nguyen-the, C. (1991). Structure of epidermis wall, cuticle and cuticular microcracks in nectarine fruit. Agronomie 11, 909–920. doi: 10.1051/agro:19911008
Oliveira Lino, L, Pacheco, I., Mercier, V., and Quilot-Turion, B. (2016). Brown rot strikes Prunus fruit: an ancient fight almost always lost. J. Agric. Food Chem. 64, 4029–4047. doi: 10.1021/acs.jafc.6b00104
Paternoster, R., Brame, R., Mazerolle, R., and Piquero, A. (1998). Using the correct statistical test for equality of regression coefficients. Criminology 36, 859–866. doi: 10.1111/j.1745-9125.1998.tb01268.x
Tamm, L., and Flückiger, W. (1993). Influence of temperature and moisture on growth, spore production, and conidial germination of Monilinia laxa. Phytopathology 83, 1321–1326. doi: 10.1094/Phyto-83-1321
Underhill, S., and Simons, D. (1993). Lychee (Litchi chinensis Sonn.) pericarp dessication and the importance of postharvest micro-cracking. Sci. Hortic. 54, 287–294. doi: 10.1016/0304-4238(93)90107-2
Van Leeuwen, G. C. M., Stein, A., Holb, I., and Jeger, M. J. (2000). Yield loss in apple caused by Monilinia fructigena (Aderh. and Ruhl.) honey, and spatio-temporal dynamics of disease development. Eur. J. Plant Pathol. 106, 519–528. doi: 10.1023/A:1008701315200
Keywords: Monilinia spp., agricultural ecosystems, fungi disease, Prunus persica, peach orchard, brown rot incidence, fruit thinning, sustainable agriculture
Citation: Bellingeri M, Quilot-Turion B, Oliveira Lino L and Bevacqua D (2018) The Crop Load Affects Brown Rot Progression in Fruit Orchards: High Fruit Densities Facilitate Fruit Exposure to Spores but Reduce the Infection Rate by Decreasing Fruit Growth and Cuticle Cracking. Front. Ecol. Evol. 5:170. doi: 10.3389/fevo.2017.00170
Received: 30 August 2017; Accepted: 13 December 2017;
Published: 05 January 2018.
Edited by:
Su Wang, Beijing Academy of Agricultural and Forestry Sciences, ChinaReviewed by:
Eugene B. Postnikov, Kursk State University, RussiaFreerk Molleman, Indian Institute of Science Education and Research, Thiruvananthapuram, India
Copyright © 2018 Bellingeri, Quilot-Turion, Oliveira Lino and Bevacqua. This is an open-access article distributed under the terms of the Creative Commons Attribution License (CC BY). The use, distribution or reproduction in other forums is permitted, provided the original author(s) or licensor are credited and that the original publication in this journal is cited, in accordance with accepted academic practice. No use, distribution or reproduction is permitted which does not comply with these terms.
*Correspondence: Michele Bellingeri, bWljaGVsZS5iZWxsaW5nZXJpQG5lbW8udW5pcHIuaXQ=