Erratum: Bergmann's and Allen's Rules in Native European and Mediterranean Phasmatodea
- 1Department of Entomology, National Taiwan University, Taipei, Taiwan
- 2Department of Entomology, Max Planck Institute for Chemical Ecology, Jena, Germany
- 3Department of Ecology—Animal Ecology, Faculty of Biology, Phillips-Universität Marburg, Marburg, Germany
Bergmann's rule states that organisms at higher latitudes should be larger and thicker than those closer to the equator to better conserve heat, and Allen's rule states that they will have shorter and thicker limbs at higher latitudes. Alternative explanations for latitudinal size clines include plant productivity and seasonality. The rules generally hold in endotherms, but in insects different species within the same genus can respond to latitude in unpredictable ways. We present the first biogeographical analysis of these rules in stick insects (order Phasmatodea), using four European species. Their long and thin bauplan makes the Phasmatodea ideal for ecomorphological studies of body length, which could identify the evolutionary drivers of their remarkable size range (including the world's longest insects). Using preserved specimens from collections across Europe; body segment and limb measurements were taken for both genders of the species Bacillus rossius, Clonopsis gallica, Leptynia attenuata, and Pijnackeria hispanica. Lengths and volumetric features were compared to latitude as well as annual mean temperature, net primary productivity, and annual growing degree days, using weighted linear regressions and ANOVA analyses. At lower latitudes/higher temperatures, B. rossius and L. attenuata had longer limbs [Allen clines] and were larger bodied and/or longer [converse-Bergmann clines], while the other species did not show latitudinal clines per se. This matches what was predicted based on closely related insects and the presence of large Phasmatodea in the tropics, but violates the temperature-size rule. Most variation in size could be attributed to temperature, but untested factors could also play a role Whether these ecogeographic rules hold true for tropical Phasmatodea and whether genetics or environment play are more important in determining adult length are topics for future research.
Introduction
The most highly studied ecogeographical rule is Bergmann's (1847) rule. According to the most recent and most thorough critical translation of the original German manuscript (Salewski and Watt, 2017), the rule states that, all else equal, “within species and amongst closely related species of homeothermic animals [those that maintain a constant internal body temperature] a larger size is often achieved in colder climates than in warmer ones, which is linked to the temperature budget of these animals.” Bergmann assumed that an animal's surface area determines its rate of heat dissipation, and its volume determines its heat production, meaning the surface-area-to-volume ratio (SA:V) represents its thermoregulatory ability. Because volume increases faster than surface area due to the square-cube-law, the SA:V of an organism decreases as it gets larger, also implying less heat loss per heat generated, or less heat production needed to maintain body temperatures. Thus, Bergmann's rule can be summarized as saying homeothermic animals will have a lower SA:V at higher latitudes and/or altitudes. A similar rule is Allen's (1877) rule, which states that, within endothermic [generating their own body heat] species, extremity length decreases as latitude increases, for similar thermoregulatory reasons.
Many have debated considerably about the exact definitions and nuances of these rules: Do they apply to ectotherms [animals that predominantly gain heat from the environment]? Can they be tested in intraspecific studies? Must they inherently imply a causative mechanism, and must that always be temperature regulation? (Mayr, 1956; Ray, 1960; Watt et al., 2010; Meiri, 2011; Olalla-Tárraga, 2011) The nuances of Bergmann's rule are particularly highly debated due to the lack of complete English translations of Bergmann's original manuscript (Salewski and Watt, 2017). In addition to thermoregulation, other explanations for Bergmann-type clines include differential dispersal rates, starvation resistances, resource availability, precipitation, primary plant productivity, habitat diversity, seasonality, genetics, and chance (Geist, 1987; Partridge and Coyne, 1997; Blackburn et al., 1999; Meiri et al., 2007). For simplicity, we will use the terms “Bergmann's rule” to cover body size clines and “Allen's rule” to cover extremity size clines over latitude or altitude regardless of taxon-level, thermal regulation, or proposed mechanism driving the cline.
Both rules hold well in mammals and birds and can be considered valid ecological generalizations in these endothermic groups (Meiri and Dayan, 2003; Purvis and Orme, 2005; Salewski and Watt, 2017). In ectotherms, however, heat is not generated but absorbed from the external environment (Ray, 1960). Animals with larger surface areas lose and gain heat faster, and larger volumes mean more heat can be stored but more heat is needed to affect a change in body temperature. Thus, selection for faster heat transfer should lead to larger SA:V ratios, while selection for more stable internal temperatures should lead to smaller SA:V ratios, and either can be preferable for hot or cold climates (Ashton and Feldman, 2003). While some ectotherms try to maintain a constant internal body temperature (homeothermy, such as basking reptiles), others are fully poikilothermic [body temperature varies according to the environment], and many are on a spectrum between the two. These temperature effects will interact with other proposed mechanisms, such as with food availability to affect energy efficiency and demand (Reim et al., 2006). The matter is further complicated by the temperature-size rule, which states that ectotherms will grow slower at cold temperatures but will reach larger adult sizes (van Voorhies, 1996). If this growth rate is standardized across time, then the adult body length may be dependent on season length. Whether the temperature-size rule holds or not is debated (Ray, 1960; Mousseau, 1997), and the mechanisms behind this rule (such as the relative effects of increased cell size or cell number, or of genetics and plasticity) are also contested and unclear (Partridge and Coyne, 1997; Angilletta and Dunham, 2003; Angilletta et al., 2004; Blanckenhorn and Llaurens, 2005; Karl and Fischer, 2008). We stress here that all these “rules” are descriptions of patterns for which multiple exceptions are allowed, and not “laws” in the sense that they are obeyed in all cases.
Among terrestrial ectothermic vertebrates, Bergmann and Allen's rule generally hold for amphibians and turtles (Alho et al., 2011), while snakes and lizards follow the opposite (Ashton and Feldman, 2003), with exceptions in each group. Species showing these “converse-Bergmann” clines are larger in warmer climates, meaning lower latitudes and altitudes. These results held regardless of sample size and other experimental values, suggesting that they are biologically significant for these taxa (Ashton, 2004). By contrast, data on insects shows highly unpredictable variation in size clines, with different species within the same genus often showing contrasting relationships to latitude (Shelomi, 2012). In addition to Bergmann and converse-Bergmann clines, there are non-linear clines: Cases where, for example, organisms are largest at the middle (Vamosi et al., 2007) or the extremes (Johansson, 2003) of their latitudinal range, or where an increasing cline stops or resets periodically [stepwise or sawtooth cline], usually due to changes in voltinism and seasonality (Masaki, 1972; Nylin and Svärd, 1991). Many species showed no clines at all (Nylin and Svärd, 1991; Shelomi, 2012). Experimental design elements such as the body parts measured, inter/intraspecific variability, geographical range size, and contiguousness (whether the samples come from one continuous range or several, unconnected sites) all had effects on the results (Shelomi, 2012). However, some general patterns seemed to appear at the taxonomic level of orders. For example: Coleoptera are more likely to show converse-Bergmann clines, Diptera Bergmann, and Plecoptera no cline (Shelomi, 2012).
Notably absent from the literature is ecogeographical data on the stick and leaf insects (order Phasmatodea). Though this order is generally poorly studied, the lack of morphometrical data is particularly surprising due to the evolutionary importance of size on this order. Phasmatodea range from the small Timematodea of 2–3 cm in length to the world's longest insect, a record presently held by the Bornean Phobaeticus chani Bragg 2008 (Phasmatidae), at 567 mm from foretarsi to hindtarsi, and 357 mm for the body alone (Whitman, 2008). Phasmatodea are often sexually dimorphic, with females being considerably larger than males. They usually follow the reverse of Rensch's (1943) rule, which states that sexual size dimorphism decreases with size in species where females are larger, meaning the dimorphism in phasmids increases with size (Abouheif and Fairbairn, 1997). With their long but thin bauplan, nearly cylindrical in all but the leaf insects (Phylliidae, which have a cylindrical body cavity but broad, leaf-mimicking projections), Phasmatodea are easy to measure for morphometrical assays, and are hence ideally suited for testing Bergmann's and Allen's rules.
Whether Phasmatodea follow these rules is not predictable, but the existence of the record-breakers in the tropics suggests converse-Bergmann clines. Based on taxonomic proximity, converse-Bergmann clines or no clines are predicted because those are the most common results for Bergmann's rule assays in the closely related Orthoptera (Shelomi, 2012). The only intraspecific data on the subject is a comparison of two specimens of Dares murudensis Bragg 1998 (Heteropterygidae), including the holotype, which suggested that this species may be larger at low altitudes [converse-Bergmann cline] (Büscher, 2014). It is also unclear if temperature is the determining force in Phasmatodea size, not only due to the aforementioned alternative mechanisms for size clines, but also because Phasmatodea body dimensions likely influence the effect and success of their mimesis: The ratio of length to thickness and the location of the six limbs can increase or decrease their resemblance to the plants on which they live and feed, and therefore their camouflage ability (Robinson, 1966). Experiments on cultured specimens of the Indian stick insect Carausius morosus Sinety 1901 in Germany suggested that they grow slightly longer at lower temperatures, suggesting Bergmann's and the temperature-size rule hold in this species (Kann, 1937, as cited by Ray, 1960), however these effects could be overshadowed in the field by a number of other processes.
In this study, we performed the first ever test of Bergmann's and Allen's rule in the Phasmatodea, using four species with native habitats across Europe and the southern Mediterranean coast (Brock, 1991). Our hope is to open the field of Phasmatodea morphometrics, whose ultimate end is to answer the question of how and why the order evolved its incredible range of lengths and body sizes.
Materials and Methods
Species and Measurements
The species chosen are four species endemic to Europe: Bacillus rossius Rossi 1788 (Bacillidae), Clonopsis gallica Charpentier 1825 (Bacillidae), Leptynia attenuata Pantel 1890 (Heteronemiidae), and Pijnackeria hispanica Bolívar 1878 (Heteronemiidae). For information on the recent generic revision of the latter two, see Scali (2009). The geographical ranges of these species cover a broad enough latitudinal range to capture possible non-linear clines and seasonality effects, but are not so broad as to be unlikely to show any cline at all, as has been a complication of studies crossing several continents (Shelomi, 2012). When available we included subspecies (ex: B. rossius rossius, B. rossius redtenbacheri), but not other species in the same genus (ex: B. grandii, B. atticus, B. whitei). Data was also collected for C. morosus Sinety 1901 (Phasmatidae), an Indian stick insect known as an invasive species in the UK and Azores as well as South Africa and California (Headrick and Wilen, 2011; Borges et al., 2013), however too few (n = 22) such wild-caught specimens were found for further analysis.
Measurements were done on pinned and dry-mounted specimens in entomological and natural history museum collections (Table S1). Only adult male and female individuals were measured, as determined based on their known minimum lengths (Brock, 1991). Specimens without legible locality data were omitted. Specimens labeled as reared or cultivated were not included in the analysis unless they were captured in adulthood and died shortly thereafter. The geographical localities of these specimens are presented in Figure 1.
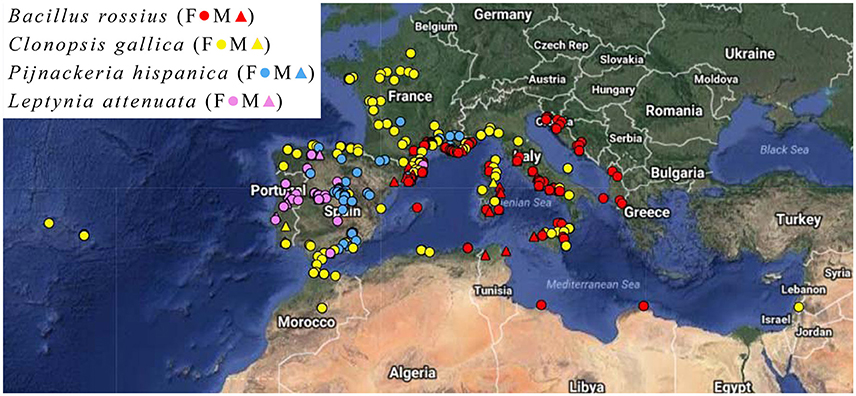
Figure 1. Locality map of the Phasmatodea specimens. Key: Female (circle), male (triangle), Bacillus rossius (red), Clonopsis gallica (yellow), Pijnackeria hispanica (blue), Leptynia attenuata (violet). Map made using HamsterMap.com and satellite imagery from Google Earth.
Measurements were made in mm using Maxwell brand digital calipers with an error of ±0.1 mm, on the following body parts [abbreviations used in data tables] (Figure 2): The length of the head [HL], thorax [TL], abdomen (all 10 segments combined) [AL], forelimb from the distal end of the trochanter to the tip of the arolium [FLL], hind femur [HFL]; and the width of the head [HW], middle thorax (where the middle pair of legs originate) [MT], and the waist (where the hind legs originate) [WT]. Because Phasmatodea can regenerate limbs, and the regenerated limbs will never become as long as the originals (Maginnis, 2008), we ignored limbs noticeably much smaller than the paired limbs on the same individual or other specimens. We generally used the left forelimb and right hind femur, except in cases where said appendage was missing, damaged, or regenerated. For incomplete or damaged specimens, we recorded only what was suitable for measurements.
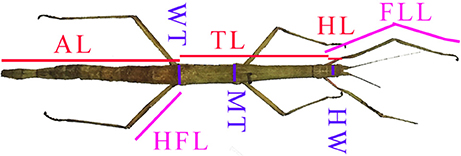
Figure 2. Morphometrical measurements and abbreviations used in this study. Based on a figure of a female Clonopsis gallica from Brock (1991), which is representative of the morphology of all species examined here. AL, abdomen length; FLL, forelimb length; HFL, hind femur length; HL, head length; HW, head width; MT, middle thorax width; TL, thorax length; WT, waist width.
We defined “body length” [BL] as the sum of HL+TL+AL. We also created a metric “fully extended length” [L] as FLL+TL+AL that represents the length of the insect when it holds its forelimbs alongside its head in line with the rest of its body, as they do when attempting to maximize their mimesis toward a branch (Robinson, 1966). This measurement may be a better indicator of selection due to factors affecting camouflage. We estimated surface area (SA, square mm), volume (V, cubic mm), and thoracic circumference (ThC, mm) using BL and the mean of MT and WT with the formulae for these metrics for a cylinder (see also Zeuss et al., 2017). While most Bergmann assays historically only use linear measurements, the use of SA and V allows for the most direct testing of Bergmann's rule via the SA:V ratio's cline over latitude (Salewski and Watt, 2017).
Locality and Independent Variables
Location data and collection date was obtained from specimen labels. From the locations, approximate GPS coordinates were extracted, using center-points of cities or the nearest large park/forests as likely collection areas if no further details were given on the data labels. Specimens with localities that were too generic [Ex: “France”] were omitted. Altitude/Elevation data was also collected, however too few samples (<20%) had this information for further analysis.
From the GPS data we calculated latitude and longitude. The independent, environmental variables for our analyses each test a possible mechanism that could drive a Bergmann-type cline: Annual Mean Temperature [AMT, °C], representing Bergmann's original thermoregulatory hypothesis (Hijmans et al., 2005; http://www.worldclim.org/current); Net Primary Productivity [NPP, grams dry matter per sq. m per year], representing plant resource availability (data from the FAO GeoNetwork, http://www.fao.org/geonetwork/srv/en/metadata.show?id=36915, period 1976–2000); and total annual Growing Degree Days [GDD, a measure in heat units calculated for each day by subtracting a base temperature, in our case 5°C, from the day's mean temperature], representing the length of the year's growing season as the annual amount of thermal energy available for plant and insect growth (New et al., 1999; https://nelson.wisc.edu/sage/data-and-models/atlas/maps.php?datasetid=31&includerelatedlinks=1&dataset=31). In our study area, AMT and GDD were negatively correlated with latitude, while NPP was positively correlated with latitude due to increased aridity around the Mediterranean Sea relative to central Europe (Zeuss et al., 2017).
Statistical Analysis
We analyzed each species and gender combination separately. We did not find enough C. gallica males or P. hispanica males for meaningful statistical analysis (n = 7 and 17 respectively), and so did not analyze them further. Statistics were weighted based on latitude and longitude locality to control for pseudoreplication caused by unequal numbers of specimens per location. We used R to perform weighted single linear regressions for the effects of latitude, longitude, year of collection, and each of the three environmental variables, plus a multiple linear regression for a model of the three environmental variables' effects on each body size measurement, using ANOVA for the significance testing (R Development Core Team, 2008). Because the environmental variables are intercorrelated to some extent, we used the R package hier.part to determine the independent linear contributions of each variable via hierarchical partitioning (Walsh and Mac Nally, 2013) with R-squared as the goodness of fit measure. We also performed canonical correspondence analysis (CCA) using rcorr (Harrell, 2016) on unweighted data. Log transformations of the data did not affect the results of the statistical assays, so we present the results with the untransformed data here.
Results
Basic Statistics
A total of 1,437 individual insects were measured from 18 museums (Table S1) across eight nations, representing localities from 23 European and North African nations under modern borders. Of these individuals, 869 had usable locality data for inclusion in this assay. The locations of each specimen are shown in Figure 1. Further data on the status of the museum collections is available in Shelomi (2016).
The results of the statistical analysis are summarized in Tables 1, 2 and fully provided in Data Sheet S1. The raw data is available in Table S2. Of all the measurements, head length and width were likely the least accurate due to their low ranges and the uneven head shape of Phasmatodea, so these variables may not be trustworthy. All other metrics were reliably measured, with thorax length and hind femur length both the easiest and most accurate to measure. Figure 3 shows the effect of latitude on each species/sex combinations' fully extended length (L), with the linear regression equation given if a significant effect was detected. Figure 4 shows the same for SA:V. Figure 5 shows schematic illustrations of the mean, minimum, and maximum sizes of these insects.
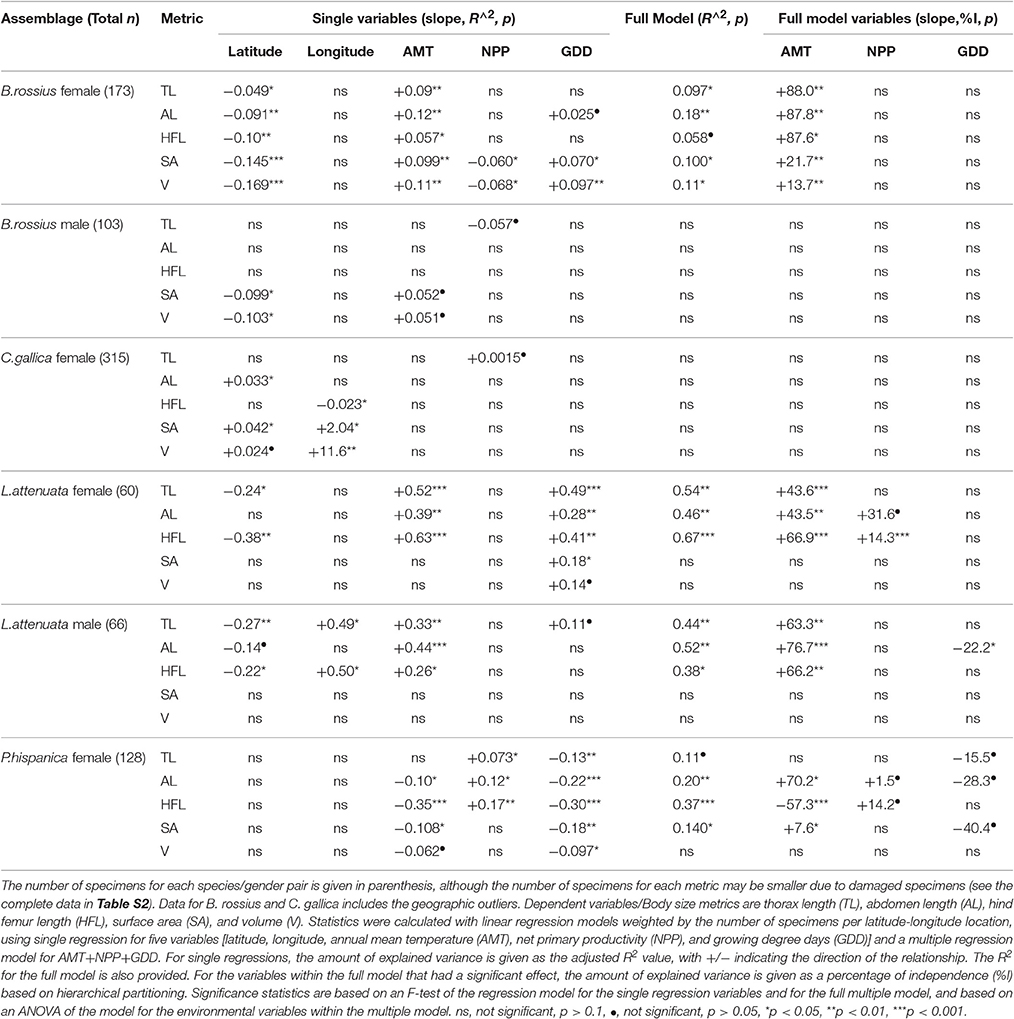
Table 1. Summary of weighted single and multiple linear regression assay and hierarchical partitioning results of ecogeographical body size variation in European Phasmatodea.
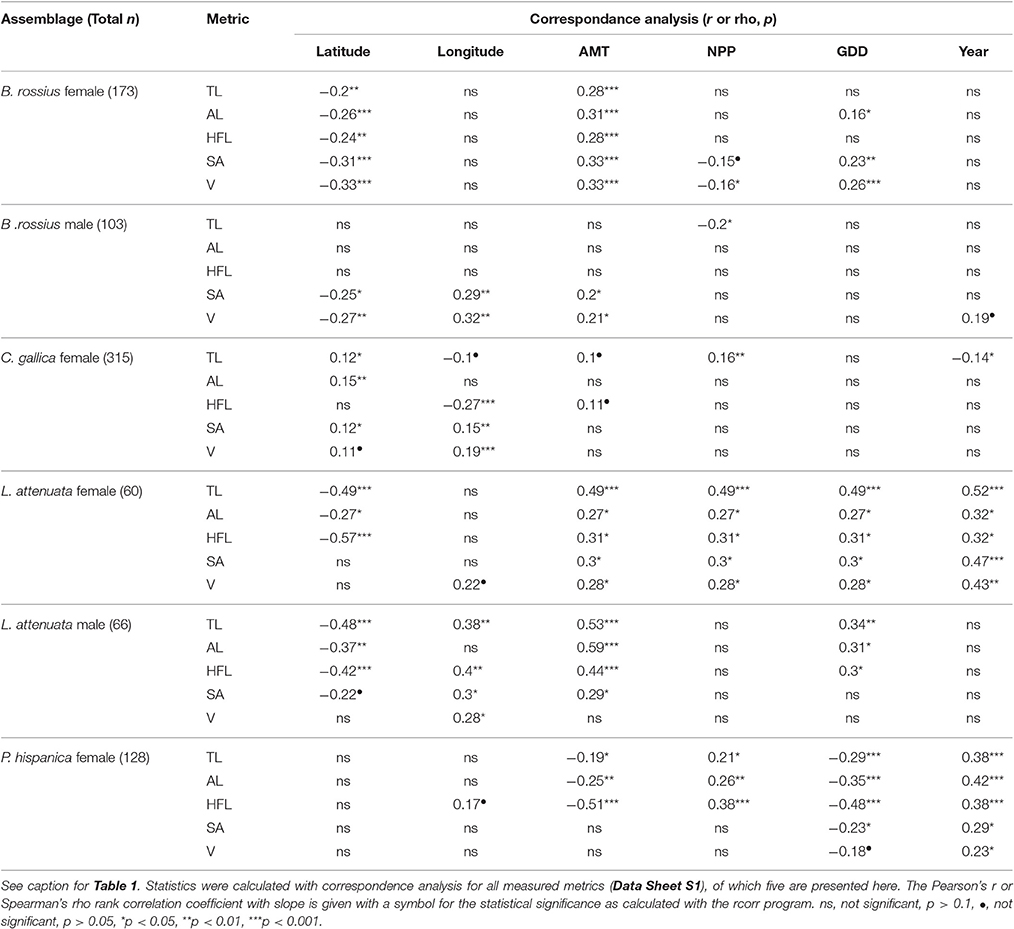
Table 2. Summary of unweighted single correspondence analysis results of ecogeographical body size variation in European Phasmatodea.
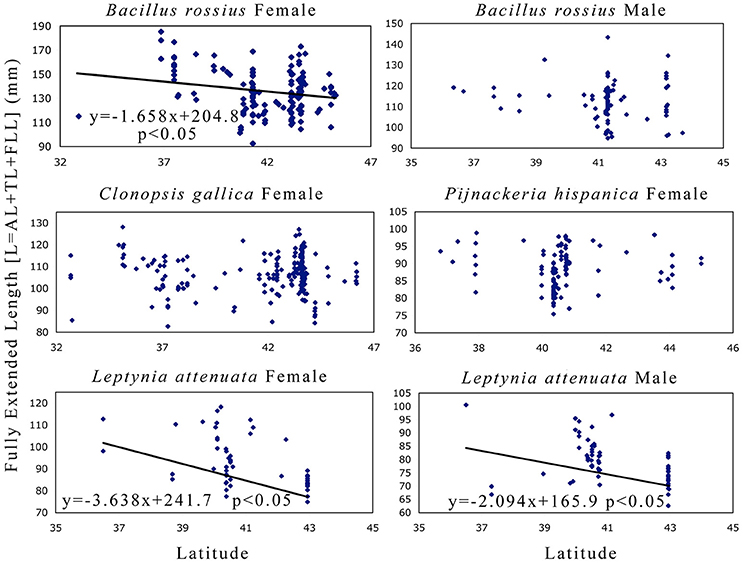
Figure 3. Fully extended length as a function of latitude in Phasmatodea. This variable (L) is the sum of both body [abdomen and thorax] and extremity [forelimb] lengths, so these metrics address Allen's rule [limb length] directly and have historically been used to measure Bergmann's rule [body size]. This metric also has the most direct implication in mimesis, as the extended pose is used by these species to maximize branch-mimicking camouflage. The results suggest that female B. rossius and both gender L. attenuata are shorter at higher latitudes (Allen cline, converse-Bergmann cline). Each point represents an individual specimen. Latitude values are in decimal degrees, L in mm. If the single linear regression weighted by the number of specimens per latitude-longitude location was significant, then the regression line, its equation, and its significance level are provided.
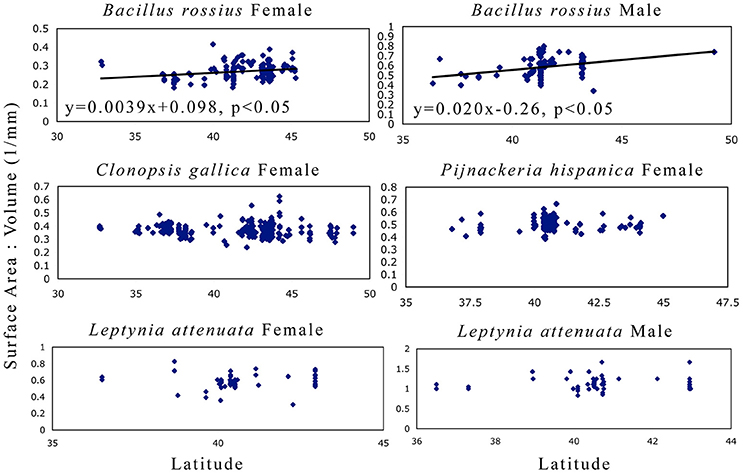
Figure 4. Surface area to volume ratio as a function of latitude in Phasmatodea. These results address Bergmann's rule of whether or not the SA:V ratio correlates with latitude. Larger bodies have a smaller SA:V ratio. Only B. rossius shows a cline, present in both genders, where individuals in higher latitudes are smaller bodied than those in lower latitudes (converse-Bergmann cline). Each point represents an individual specimen. Latitude values are in decimal degrees, SA:V in mm−1. If the single linear regression weighted by the number of specimens per latitude-longitude location was significant, then the regression line, its equation, and its significance level are provided.
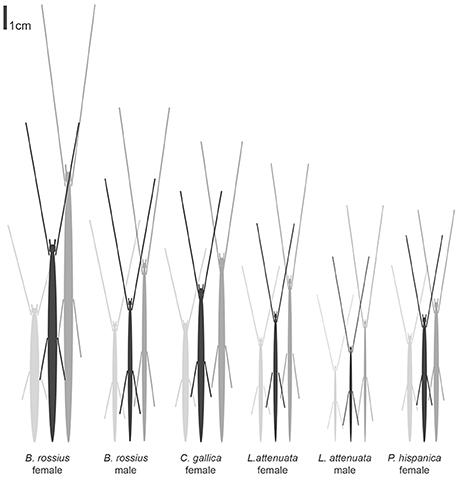
Figure 5. Schematic of the mean, minimum, and maximum sizes of Phasmatodea in this study. Images were made to a scale of 10 pixels per mm first using ellipses with a height of the mean body length [BL] and width of the mean waist thickness [WT], with forelimbs drawn with the length of the mean forelimb length [FLL], and hind femurs drawn with the length of the mean hind femur length [HFL] connected to the insect at the proper location [thorax-abdomen junction] based on the mean abdomen length [AL]. These were scaled up or down according to the largest and smallest recorded insect [L]. Image Credit: Shelomi.
Correlation Analyses: Effects on Insect Size
In the single regressions, decreasing lengths and widths with higher latitude and lower AMT was observed in B. rossius females. Similar effects were noted in the multiple regression assays, with ANOVA suggesting that AMT, which is negatively correlated with latitude, had the strongest and often only significant effect on these species' metrics. Hierarchical partitioning attributed the majority (>50%) of the independent effects of the multiple model on AMT for lengths, but GDD for thickness. Among the tested parameters here, therefore, AMT is the strongest statistical contributor to latitudinal clines in body segment and limb lengths, and GDD to widths and SA:V (Table 1). In the CCA, all non-head size metrics significantly decreased with increasing latitude (p < 0.01) and correspondingly decreasing AMT (p < 0.01), with width-based metrics (widths, surface area, volume, SA:V, and circumfrerence) only increasing with decreasing GDD (p < 0.01), and increasing NPP (p < 0.05). Longitude and year had no effect.
Three apparent outliers in the B. rossius females existed: These specimens were from two locations on the northern coast of Libya (Figure 1), having low latitudes but also small sizes (easily noticed in Figures 3, 4). Excluding these outliers produced stronger single and multiple variable effects, with appearance of an effect of NPP (positive correlation, p < 0.05) in the multiple regression model not present with the outliers (Data Sheet S1).
B. rossius males showed no length clines except in the aforementionedly unreliable head size dimensions. In single regression models, B. rossius males were significantly thinner at larger latitudes (p < 0.05) with an overall increase in SA:V (p < 0.05), but this effect vanished in the multiple model (p > 0.1). The CCA showed negative effects on width based metrics alone for increasing latitude and decreasing AMT, as well as strong (p < 0.01) positive effects of increasing longitude.
In the single regression analyses for C. gallica females, the abdomen was slightly significantly (p < 0.05) larger in higher latitudes, the limbs were shorter (p < 0.05) in higher longitudes [eastward], and no significant effects of environmental variables were noted. C. gallica had a significantly larger surface area (p < 0.05) and, to a lesser extent, volume (p < 0.1) at higher latitudes and longitudes, resulting in a barely insignificant (p < 0.1) decrease in SA:V ratio with latitude alone but a strongly significant decrease for longitude alone (p < 0.001). Hierarchical partitioning suggested NPP affects lengths while AMT and GDD affect width, but the multiple regression models were not significant for this species. CCA suggested increased body segment length with increasing latitude (p < 0.05), plus reduced limb length (p < 0.001) but increased width, and overall size (p < 0.01) with increasing longitude [moving eastward].
Eleven longitudinal outliers in C. gallica existed (Figure 1), 10 from three locations in the Azores [−28.6 to −25.6° longitude] and one from Israel [35.0° longtidue]. Nearly 20° of longitude existed between these specimens groups and their next closest specimens [Iberia and Sicily respectively], meaning they greatly reduced the contiguousness of the dataset and nearly tripled the total longitudinal range. Removing these samples had slight changes in the significance of the observed correlations, with the most noticable effect of removing the latitudinal cline for abdomen length. CCA revealed significantly shorter limbs and larger bodies with increased longitude, with increasing AMT, NPP, and collection year all positively affecting length, but reduced effect of latitude. GDD was never significant in C. gallica CCA.
Single regressions for both genders of L. attenuata reported decreasing lengths with higher latitude and lower AMT, and increased width with higher GDD for females only. In L. attenuata males only, longitude also had a positive effect on limb and thorax lengths. In the mixed model, AMT had the strongest and often only significant effect on these species' metrics. For females, hierarchical partitioning attributed the majority (~65%) of the independent effects of the multiple model on AMT for limb length, but GDD took a larger share of the independent effects for body segment lengths. CCA on females found significant negative effects of increased latitude on body and limb length, but significantly positive and, notably, equal sized effects of AMT, NPP, and GDD (p < 0.05). Year had significant positive effects on all metrics (older specimens were smaller). Note that L. attenuata specimens had the smallest range for all environmental factors and that this was our smallest dataset. For males, hierarchical partitioning suggested AMT is the strongest statistical contributor for most metrics, and CCA found significant negative effects of latitude but positive effects for longitude (p < 0.05), AMT (p < 0.001), and GDD (p < 0.05) only for lengths only. Year had no effect.
In P. hispanica females, single regression suggested a negative effect of GDD (p < 0.01) and AMT (p < 0.05) on limb and body lengths, and a positive effect of NPP on lengths (p < 0.05). CCA suggested the same, as well as a positive effect of year (p < 0.01). This was the only time AMT and GDD were found to have negative effects on specimen size. In the multiple-variable model, limb and body lengths showed different clinal directions: Abdomen length was now positively correlated to AMT (p < 0.05), while limb length was still strongly negatively correlated to AMT (FLL: p < 0.01, HFL: p < 0.001). P. hispanica widths did not differ significantly with any independent variable, and neither did SA:V, although surface area was significantly (p < 0.05) positively correlated with AMT in the single and multiple regression models. Hierarchical partitioning suggested AMT is usually the strongest contributor to among the environmental factors. Overall these idiosyncratic responses produced no latitudinal (Figure 3) or longitudinal clines for lengths in P. hispanica females using any statistical model.
Correlation Analyses: Environment to Geography
Table 3 shows, for each data set, whether the environmental factors correlate to latitude or longitude. We include these data because of samples where the environmental clines and geographic clines do not align. In general, AMT and GDD should decrease and NPP increase with increasing latitude. For AMT this pattern held strongly (p < 0.001) for all except the female L. attenuata (p < 0.01) and P. hispanica (p < 0.05) datasets. The same correlations existed for GDD, very strong (p < 0.001) in all but these two (p < 0.01). NPP correlated positively and strongly (p < 0.001) with latitude for all except the male B. rossius set, where the correlation was weaker (p < 0.05), and the two L. attenuata sets, where it did not correlate at all (p > 0.1). Environmental factors responded to longitude differently, especially NPP, whose correlation to longitude could be positive, negative, or not significant depending on species, sex, and presence or absence of outliers within the dataset.
Discussion
Bergmann's, Allen's, and Temperature-Size Rules in European Phasmatodea
As in all ecogeographical studies, the possibility exists that the sampling did not cover a sufficient range to account for true clines, linear or otherwise. Due to the limitations of museum collection efforts, which are not random in location or timing of collecting (Shelomi, 2016), as well as climate and environmental change over time, we may have missed changes over the decades. Other potential biases are whether the original collectors preferentially kept or noticed larger specimens, whether smaller adults were erroneously discarded as nymphs by the original collectors or in this analysis, or whether smaller adult legs were mistakenly identified as regenerations and excluded. These would all bias the samples toward larger insects. Larger nymphs erroneously labeled as adults would have the opposite effect. While only dry specimens were used to avoid the shrinking effects of ethanol, different preservation methods of the different collectors, such as the substance used to kill the insects in the field and whether the insects dried straight or contorted, would also affect the final specimen dimensions. Adult phasmatodea live for several months, so one cannot tell from the date of collection of an adult whether they spent formative months of their development in colder or warmer seasons. Lastly, the data cannot account for effects of altitude, as most specimen labels did not include altitude data. These factors should all be kept in mind when interpreting the results.
The single-variable regression lines for latitude were better fits to the data than the full model [lower p-values on the F-test of the regression lines] for B. rossius and C. gallica, and were better fits for AMT and GDD in all species, so the frequent absence of an observed effect of GDD in the mixed regression models may be due to autocorrelation from AMTs effect on GDD. Overall and from our three environmental variables, much of the variation in body size of the specimens was attributed to AMT, with GDD also important for body width in particular but not limb length. Little was ever attributed to NPP, which also rarely had an effect in the models. This suggests that abundance of available plant matter has less of a role than the available heat energy in European Phasmatodea size. Alternatively this is because NPP was highly variable over longitude within the datasets, even if it correlated reliably with latitude.
B. rossius females showed unambiguous converse-Bergmann clines, being longer, thicker, and overall larger (smaller SA:V ratios, Figure 4) at lower latitudes and at warmer climates (higher AMTs). These results fit our hypothesis for converse-Bergmann clines in the Phasmatodea based on the presence of giant Phasmatodea species in the tropics and what was observed for the related Orthoptera, but contradict the temperature-size rule that was observed for Carausius. The species also followed Allen's rule (Figure 3), but likely not for thermoregulatory reasons. B. rossius males also showed converse-Bergmann clines in the single regression model (Figure 4) and the CCA (Table 2), but no clines for limb length.
C. gallica showed detectable but statistically insignificant (0.05 < p < 0.1) Bergmann clines for body size [higher surface areas and volumes plus lower SA:V at higher latitudes], due primarily to increased abdominal length. None of the measured environmental factors had significant effects. The results are thus borderline between Bergmann and no cline. One problem may be the species' large longitudinal distribution (Figure 1), as non-contiguousity can obscure the true nature of an ecogeographic cline (Shelomi, 2012), as was strongly the case for B. rossius with the Libyan specimens. Indeed, longitude had significant, positive effects on body size and negative effects on limb length in C. gallica, which were more noticable (p < 0.01) when the longitudinal outliers were removed, however removing these outliers also reduced the impact of latitude on abdomen length (Data Sheet S1). Longitude normally does not figure into ecogeographic clines, however the impact of the Atlantic currents on weather and the large geographical spread of this species provide variables that could covary with longitude in Europe. Limb length was not correlated with latitude or environment, but was strongly negatively affected by increasing longitude. C. gallica also had the greatest total ranges in the environmental factors than the other species, even after removing the outliers, but there is no guarantee that these factors change linearly with latitude and longitude across Europe. We reserve judgment on whether C. gallica truly follows Bergmann's or Allen's rules pending more collecting of this widespread species, especially from the Balkans and Turkey where the species is known to occur (Brock, 1991), but from which region few collected specimens are available for a number of reasons (Shelomi, 2016).
L. attenuata of both genders had significantly longer bodies and limbs in lower latitudes and were possibly thicker at higher GDDs, but did not show trends for SA:V. Males of this species had slightly longer limbs at high longitudes, while older specimens from females were generally larger. Note that this species is the thinnest of the four (width range 1.1–3.3 mm for females, 0.6–1.3 mm for males), which might have affected our ability to accurately measure and detect clines in width or variables that depend on width. That would explain the lack of significant latitudinal clines for SA:V in this species (Figure 4) despite the significant cline in length alone. At the extreme aspect ratio of the Phasmatodea, where length is more than one or even two orders of magnitude longer than diameter, tests of Bergmann's rule for this order, or at least its skinniest species, may thus be best performed with length alone as a proxy for size. Precedent is ample, as a majority of Bergmann's rule assays over decades, most involving far less elongated species than Leptynia, have used length instead of SA:V (Shelomi, 2012). We thus state that L. attenuata shows converse-Bergmann clines as we defined them, follows Allen's rule, and does not obey the temperature-size rule. In addition, the R2 values for the multiple model for lengths were higher for L. attenuata than for other species, suggesting the environmental variables chosen explain a larger percentage of the size variation, although the values for the other species are not unusual for this type of data set (Zeuss et al., 2017). Note also that, almost the opposite of C. gallica, this species covered the smallest geographic and environmental range, in which envrionment and geography were the least well correlated (Table 3), and had the smallest sample size, bordering being too low for sufficient power (α ≥ 0.8) for the CCA.
For P. hispanica females, no latitudinal clines for volumetric measurements were noted, though this species too has a small width (1.3–2.8 mm) that likely limited the reliability of surface area and volume estimates. However, no latitudinal clines for lengths were noted for this species either [except for head length, which we have stated is an unreliable metric]. Unique among the examined Phasmatodea, P. hispanica showed a negative correlation between lengths and AMT and GDD in most models, suggesting the temperature-size rule holds for this species and that longer seasons and/or more time available for growth correlate with smaller sizes. The factors that decrease with latitude (AMT, GDD) had negative effects while the factor that increased with latitude (NPP) had positive effects on size, which suggests a Bergmann cline despite no detectible latitudinal cline. The environmental factors also affected limb length in a way that suggests a converse-Allen cline without an latitudinal correlation. The lack of a direct latitudinal cline despite the strong effects of these environmental clines in the models may be due to the reduced correlation among these variables and latitude over the geographical range of P. hispanica (Table 3).
To summarize: B. rossius shows an unambiguous converse-Bergmann cline (increased SA:V with latitude) and Allen cline (reduced limb length with latitude). L. atenuata shows the same clines for lengths, but not SA:V, so it follows Allen's rule but would not fullfill Salewski and Watt's (2017) interpretation of Bergmann's rule. C. gallica showed weak but not significant Bergmann clines but no response to the environmental factors, while P. hispanica showed no geographic clines but strong enivronmental responses that would otherwise lead to a Bergmann cline.
Significance for Phasmatodea Globally
As in insects in general (Shelomi, 2012), whether Phasmatodea show latitudinal clines depends on species and sex. No generalizations for the order can be concluded from four species alone, two of which showed clear converse-Bergmann and Allen clines while the other two were ambiguously non-clinal tending toward Bergmann. The evidence tentatively suggests converse-Bergmann clines or absence of a cline will be more common for Phasmatodea than Bergmann clines, as was the case with the closely related order Orthoptera (Shelomi, 2012). Size in non-clinal species may be due to reduced climate/latitude correlations in their particular range (Table 3), or the species is affected by something other than climate or has a nonlinear relationship to latitude that was not evident in our analyses.
Among our enivronmental variables, Phasmatodea size changes were best explained with temperature (AMT and, to a lesser extent, GDD), but not plant productivity (NPP). The latter is noteworthy given the dependence of Phasmatodea on plant matter as a diet. We also see converse-Bergmann clines together with Allen clines that would otherwise be contradictory, suggesting that, for an organism as long and thin as the Phasmatodea, the body's allometry is more akin to that of the limbs. If we assume the thermoregulatory hypothesis is true, then the larger size of Phasmatodea evolved to increase heat transfer rates, perhaps to cool faster in the tropics or to better retain heat absorbed in the day for use during the Phasmatodea's nocturnal hours of activity. This could be tested with thermoregulatory experiments, as was done along altitudinal gradients for grasshoppers (Ashby, 1997) and bees (Stone, 1993).
More likely, however, is that Phasmatodea body and limb size do not have a significant thermoregulatory function, but refelect temperature dependent growth rates. Phasmatodea growth rate and/or final size may be positively dependent on heat (GDD) in nymphal or even egg stages. Except possibly P. hispanica, none of our species followed the temperature-size rule's prediction that ectotherms grow faster in warm temperatures but reach smaller sizes. Instead our species were generally larger at warmer climates. Phasmatodea would not be the first organisms to show converse clines to the temperature-size rule, whose generality for ectotherms has already been called into question (Angilletta et al., 2004). Our data does not address the question how fast these insects mature to their final size, which may or may not violate that aspect of the temperature-size rule.
Other ecological values, which may or may not correlate with climate and geographic location, could play a role. Body and limb length affect the insects' crypsis, so the type of plant in a Phasmatodea species' envrionment and its preferred host may affect the evolution of limb and body segment lengths and proportions. Prior laboratory research shows the choice of food plant may or may not affect Phasmatodea size (Hsiung and Panagopoulos, 1998; Boucher and Varady-Szabo, 2005), however many species are polyphagous and are not expected to have co-evolved with a certain plant. Limb size may also have different selective pressures than the body. Phasmatodea lose legs often, both via autotomy to escape predators and, frequently, due to molting complications (Maginnis, 2008). These pressures could select for longer or shorter limbs. The links to latitudes for these hypotheses are possible clines in plant presence (as food or a mimesis target), predator diversity (with longer limbs differently suited to mimicry and/or more likely to be grabbed by a predator rather than the body), and humidity (which may decrease the chance of a molting complication and thus allows for longer legs to evolve). Species-specific importance of these factors could, for example, explain why P. hispanica shows converse-Allen clines while the other species do not.
Extending this analysis to other species is required before drawing conclusions for the entire order. Seeing if such patterns exist in species with stronger sexual dimorphisms, especially those with heavyset, non-flying females and slender, flying males, would be interesting given the different evolutionary constraints on body size for the different sexes in these species, as well as to formally test Rensch's rule. Comparing results in tropical climates to our temperate and semi-arid European and North African dataset could reveal other anomalies. The Libyan specimens suggest that, over a larger latitudinal range, non-linear clines would likely emerge due to negative effects of desert areas between the temperate zones and the equatorial rainforests. These and the C. gallica outliers (the Azores and Israel, Figure 1) show the impact large and non-contiguous samples can have obsfucating any underlying patterns.
One question particularly easily addressed with Phasmatodea is comparing the sizes of those in their native habitat with those reared outside of it: Is body size determined by genetics or by local climate? The large number of Phasmatodea enthusiasts breeding stick insects worldwide provides an ample data-source, especially in Europe where cross-border trade of non-native Phasmatodea is unregulated and private Phasmatodea ownership is not restricted. Tests on the invasive C. morosus comparing wild-caught populations in their native range in India with populations and cultures abroad would be another way to address this question. To that end, we are sharing data on 121 reared and wild-caught C. morosus specimens found in the museums visited for this study (Table S3).
In regard to Bergmann's rule, however, the many confounding variables between the field and the lab as well as founder effects of the likely inbred reared stock would make meaningful analysis difficult. Common-garden experiments are the gold standard method to formally test theories of, for example, temperature effects on size clines. Collections of Carausius specimens or eggs from India and from other regions where it has invaded, and rearing their descendents or hatching the collected eggs respectively in controlled environments in a lab could formally separate the effects of genetics and environmental variables. This can also be done with any other Phasmid species: Taking eggs or individuals from different ends of their size and latitudinal range, rearing groups of each under controlled environments, and seeing whether size responses differ between native and non-native groups.
While we cannot make general conclusions about climate-size relationships in Phasmatodea at this time, we have generated the first dataset in the field of Phasmatodea morphometrics to be used toward this aim. It is our hope that future analyses of Phasmatodea size clines in other parts of the world and common-garden experimental testing of hypothesized mechanims for these clines will eventually explain the existence and evolution of their extreme and record-setting sizes.
Author Contributions
MS conceived of the project, acquired measurement data, contributed to statistical analysis, and drafted and approved of the final manuscript. DZ acquired the environmental data, contributed to statistical analysis, and revised and approved of the final manuscript. All agree to be accountable for the content of the work.
Funding
Travel for this research was supported by the Orthopterists' Society's Theodore J. Cohn research grant (2015).
Conflict of Interest Statement
The authors declare that the research was conducted in the absence of any commercial or financial relationships that could be construed as a potential conflict of interest.
Acknowledgments
Special thanks to Pamm Mihm and Michel Lecoq (Orthopterists' Society). Thanks to the following people, listed in no particular order, for use of their museum collections: Peter J. Schwendinger (Geneva); Fabrizio Rigato (Milan); Maggie Reilly and Jeanne Robinson (Glasgow); Dmitri Logunov (Manchester); Sheila Wright and Andrew King (Nottingham); Amoret Spooner (Oxford); Judith Marshall, Ben Price, and George Beccaloni (London); Jerome Constant (Brussels); Tony Robillard, Simon Poulain, and Emmanuel Delfosse (Paris); Glòria Masó Ros and Berta Caballero (Barcelona); Mercedes París (Madrid); Eva Monteiro and Luis Filipe Lopes (Lisbon); Kai Schütte, Ilonka Rehmann, Thure Dalsgaard (Hamburg); Angelika Weirauch and Stephan Blank (Müncheberg); Michael Ohl (Berlin); Ralph S. Peters and Karin Ulmen (Bonn); Klaus Schönitzer, Bärbel Stock-Dietel, and Franz Schmolke (Munich); Christian Schmidt (Dresden). Thanks also to the following people, in no particular order, who provided information about their museum collections, which were not visited due to lack of appropriate specimens at the time: Volker Lohrmann and Michael Stiller (Übersee-Museum, Bremen), Gellért Puskás and Dávid Murányi (Magyar Természettudományi Múzeum, Budapest), John Cooper (Booth Museum of Natural History, Brighton), Michael Wilson (National Museum of Wales, Cardiff), Elaine Charwat (Linnean Society of London), Hannah Allum (Weston Park Museum, Sheffield), Nigel T. Monaghan (National Museum of Ireland, Dublin), Olivier Gerriet (Muséum d'Histoire naturelle de Nice), Roy Danielsson (Lund University Museum of Zoology), Niklas Apelqvist (Naturhistoriska Riksmuseet, Stockholm), Henrik Enghoff (Natural History Museum of Denmark, Copenhagen), Hans Mejlon (Uppsala University Zoological Museum), Karla Schneider (Zentralmagazin Naturwissenschaftlicher Sammlungen der Martin-Luther-Universität, Halle an der Saale), Maria Dimaki (Goulandris Natural History Museum, Athens), Apostolos Trichas (Natural History Museum of Crete), Luca Bartolozzi (La Specola - Museo di Storia Naturale di Firenze [Florence]), Roberta Salmaso (Museo Civico di Storia Naturale, Verona), Marinella Garzena (Museo Regionale di Scienze Naturali, Turin).
Supplementary Material
The Supplementary Material for this article can be found online at: http://journal.frontiersin.org/article/10.3389/fevo.2017.00025/full#supplementary-material
Data Sheet S1. Full results of the statistical analyses of the ecogeographical clines in European Phasmatodea. Note that Bacillus rossius and Clonopsis gallica female data is presented twice: Once including the outlier specimens and once without. Metrics (dependent variables) are in mm or factors thereof (sq. mm for surface area, etc). The full model is AMT+NPP+GDD. Significance statistics (p-values) are based on an F-test of the regression models for the R2 values (also given for the slopes of the single linear regression), and based on an ANOVA of the results of the multiple linear regression (given with the slopes of the independent variables). For correspondence analysis, the Pearson's r or Spearman's rho rank correlation coefficient with slope is given with a symbol for the statistical significance as calculated with the rcorr program. Key: AL, abdominal length; AMT, annual mean temperature (°C); BL, body length [HL+TL+AL]; FLL, forelimb length; GDD, growing degree days (heat units based on 5°C threshhold); HFL, hind femur length; HL, head length; HW, head width; %I, percentage of variance in the full model explained by each variable; L, fully extended length [FLL+TL+AL]; MT, width of the middle thorax; n, number of specimens used for the analysis [values will differ due to incomplete specimens, such as one missing its rear limbs, etc.]; NPP, net primary productivity (grams dry matter per sq. m per year); SA, surface area; SA:V, surface area to volume ratio; ThC, thoracic circumference; TL, thorax length; V, volume; WT, width of the waist. Blank cells mean no significant effect (p > 0.1). •, not significant, p > 0.05, *p < 0.05, **p < 0.01, ***p < 0.001.
Table S1. Museum collection codes used in supplementary tables.
Table S2. Raw data for endemic European and Mediterranean Phasmatodea in European museum collections. Museum collection codes in Table S1. Localities based on [translations of] the locality labels of each specimen. Date is the date of collection according to the label. Key: AL, abdominal length; BL, body length [calculated as HL+TL+AL]; F, female; FLL, forelimb length; HFL, hind femur length; HL, head length; HW, head width; L, fully extended length [calculated as FLL+TL+AL]; M,male; MT, width of the middle thorax; SA, surface area [calculated as BL*ThC]; SA:V, surface area to volume ratio; ThC, thoracic circumference [calculated as Pi (π) * the mean of Mt and WT]; TL, thorax length; V, volume [calculated as (the mean of MT and WT)∧2* Pi (π) * BL]; WT, width of the waist.
Table S3. Raw data for reared and wild-caught Carausius morosus in European museum collections. Museum collection codes in Table S1. Localities based on [translations of] the locality labels of each specimen. Those with “REARED” under “City, other” were likely raised in captivity. Date is the date of collection according to the label. Key: AL, abdominal length; BL, body length [calculated as HL+TL+AL]; F, female; FLL, forelimb length; HFL, hind femur length; HL, head length; HW, head width; L, fully extended length [calculated as FLL+TL+AL]; MT, width of the middle thorax; SA, surface area [calculated as BL*ThC]; SA:V, surface area to volume ratio; ThC, thoracic circumference [calculated as Pi (π) * the mean of Mt and WT]; TL, thorax length; V, volume [calculated as (the mean of MT and WT)∧2* Pi (π) * BL]; WT, width of the waist.
References
Abouheif, E., and Fairbairn, D. J. (1997). A comparative analysis of allometry for sexual size dimorphism: assessing Rensch's rule. Am. Nat. 149, 540–562. doi: 10.1086/286004
Alho, J. S., Herczeg, G., Laugen, A. T., Räsänen, K., Laurila, A., and Merilä, J. (2011). Allen's rule revisited: quantitative genetics of extremity length in the common frog along a latitudinal gradient J. Evol. Biol. 24, 59–70. doi: 10.1111/j.1420-9101.2010.02141.x
Allen, J. A. (1877). The influence of physical conditions in the genesis of species. Radic. Rev. 1, 108–140.
Angilletta, M. J. Jr., and Dunham, A. E. (2003). The temperature-size rule in ectotherms: simple evolutionary explanations may not be general. Am. Nat. 162, 332–342. doi: 10.1086/377187
Angilletta, M. J. Jr., Steury, T. D., and Sears, M. W. (2004). Temperature, growth rate, and body size in ectotherms: fitting pieces of a life-history puzzle. Integr. Comp. Biol. 44, 498–509. doi: 10.1093/icb/44.6.498
Ashby, P. D. (1997). Conservation of mass-specific metabolic rate among high-and low-elevation populations of the acridid grasshopper Xanthippus corallipes. Physiol. Zool. 70, 701–711. doi: 10.1086/515877
Ashton, K. G. (2004). Sensitivity of intraspecific latitudinal clines of body size for tetrapods to sampling, latitude and body size. Integr. Comp. Biol. 44, 403–412. doi: 10.1093/icb/44.6.403
Ashton, K. G., and Feldman, C. R. (2003). Bergmann's rule in nonavian reptiles: turtles follow it, lizards and snakes reverse it. Evolution 57, 1151–1163. doi: 10.1111/j.0014-3820.2003.tb00324.x
Bergmann, K. (1847). Ueber die verhältnisse der wärmeökonomie der thiere zu ihrer grösse. Gottinger Stud. 3, 595–708.
Blackburn, T. M., Gaston, K. J., and Loder, N. (1999). Geographic gradients in body size: a clarification of Bergmann's rule. Divers. Distribut. 5, 165–174.
Blanckenhorn, W. U., and Llaurens, V. (2005). Effects of temperature on cell size and number in the yellow dung fly Scathophaga stercoraria. J. Therm. Biol. 30, 213–219. doi: 10.1016/j.jtherbio.2004.11.004
Borges, P. A., Reut, M., Ponte, N. B., Quartau, J. A., Fletcher, M., Sousa, A. B., et al. (2013). New records of exotic spiders and insects to the Azores, and new data on recently introduced species. Arquipélago. Life Mar. Sci. 30, 57–70.
Boucher, S., and Varady-Szabo, H. (2005). Effects of different diets on the survival, longevity and growth rate of the Annam stick insect, Medauroidea extradentata (Phasmatodea: Phasmatidae). J. Orthoptera Res. 14, 115–118. doi: 10.1665/1082-6467(2005)14[115:EODDOT]2.0.CO;2
Brock, P. (1991). Stick Insects of Britain, Europe, and the Mediterranean. London: Fitzgerald Publishing.
Büscher, T. (2014). Identification of PSG 332 (Dares sp. “Crocker Range”). Phasmid Study Group Newslett. 133, 1–3. doi: 10.13140/RG.2.1.2325.8647
Harrell, Jr. F. E. (2016). Hmisc: Harrell Miscellaneous. R Package Version 4.0-2. Available online at: https://cran.r-project.org/package=Hmisc
Hijmans, R. J., Cameron, S. E., Parra, J. L., Jones, P. G., and Jarvis, A. (2005). Very high resolution interpolated climate surfaces for global land areas. Int. J. Climatol. 25, 1965–1978. doi: 10.1002/joc.1276
Hsiung, C.-C., and Panagopoulos, D. (1998). Preliminary observations on the effects of food plant on the stick insect Eurycantha calcarata Lucas (Cheleutoptera: Phasmatidae). J. Orthoptera Res. 7, 93–98. doi: 10.2307/3503500
Johansson, F. (2003). Latitudinal shifts in body size of Enallagma cyathigerum (Odonata). J. Biogeogr. 30, 29–34. doi: 10.1046/j.1365-2699.2003.00796.x
Kann, F. (1937). Temperatureinflüsse auf die Wachstumsstadien von Dixippus (Carausius) morosus. Biol. Gen. 13, 25–66.
Karl, I., and Fischer, K. (2008). Why get big in the cold? Towards a solution of a life-history puzzle. Oecologia 155, 215–225. doi: 10.1007/s00442-007-0902-0
Maginnis, T. L. (2008). Autotomy in a stick insect (Insecta: Phasmida): predation versus molting. Florida Entomol. 91, 126–127. doi: 10.1653/0015-4040(2008)091[0126:AIASII]2.0.CO;2
Masaki, S. (1972). Climatic adaptation and photoperiodic response in the band-legged ground cricket. Evolution 26, 587–600. doi: 10.2307/2407055
Meiri, S. (2011). Bergmann's Rule – what's in a name? Glob. Ecol. Biogeogr. 20, 203–207. doi: 10.1111/j.1466-8238.2010.00577.x
Meiri, S., and Dayan, T. (2003). On the validity of Bergmann's rule. J. Biogeogr. 30, 331–351. doi: 10.1046/j.1365-2699.2003.00837.x
Meiri, S., Yom-Tov, Y., and Geffen, E. (2007). What determines conformity to Bergmann's rule? Glob. Ecol. Biogeogr. 16, 788–794. doi: 10.1111/j.1466-8238.2007.00330.x
Mousseau, T. A. (1997). Ectotherms follow the converse to Bergmann's Rule. Evolution 51, 630–632. doi: 10.2307/2411138
New, M., Hulme, M., and Jones, P. (1999). Representing twentieth-century space-time climate variability. Part I: development of a 1961-90 mean monthly terrestrial climatology. J. Clim. 12, 829–856. doi: 10.1175/1520-0442(1999)012<0829:RTCSTC>2.0.CO;2
Nylin, S., and Svärd, L. (1991). Latitudinal patterns in the size of European butterflies. Holarct. Ecol. 14, 192–202. doi: 10.1111/j.1600-0587.1991.tb00652.x
Olalla-Tárraga, M. Á. (2011). “Nullius in Bergmann” or the pluralistic approach to ecogeographical rules: a reply to Watt et al. (2010). Oikos 120, 1441–1444. doi: 10.1111/j.1600-0706.2011.19319.x
Partridge, L., and Coyne, J. A. (1997). Bergmann's Rule in ectotherms: is it adaptive? Evolution 51, 632–635. doi: 10.2307/2411139
Purvis, A., and Orme, C. D. L. (2005). “Evolutionary Trends in Body Size,” in Deciphering Growth, eds J. C. Carel, P. A. Kelly, and Y. Christen (Berlin: Springer-Verlag), 1–18.
R Development Core Team (2008). R: A Language and Environment for Statistical Computing. Vienna: R Foundation for Statistical Computing. Available online at: http://www.R-project.org
Ray, C. (1960). The application of Bergmann's and Allen's rules to the poikilotherms. J. Morphol. 106, 85–108. doi: 10.1002/jmor.1051060104
Reim, C., Teuschl, Y., and Blanckenhorn, W. U. (2006). Size-dependent effects of temperature and food stress on energy reserves and starvation resistance in yellow dung flies. Evol. Ecol. Res. 8, 1215–1234.
Rensch, B. (1943). Studien über Korrelation und klimatische Parallelität der rassenmerkmale von Carabus-Formen. Zool. Jahrb. 76, 103–170.
Robinson, M. H. (1966). Anti-Predator Adaptations in Stick- and Leaf-Mimicking Insects. Dissertation, University of Oxford.
Salewski, V., and Watt, C. (2017). Bergmann's rule: a biophysiological rule examined in birds. Oikos 126. doi: 10.1111/oik.03698
Scali, V. (2009). Revision of the Iberian stick insect genus Leptynia Pantel and description of the new genus Pijnackeria. Ital. J. Zool. 76, 381–391. doi: 10.1080/11250000802702062
Shelomi, M. (2012). Where are we now? Bergmann's rule sensu lato in insects. Am. Nat. 180, 511–519. doi: 10.1086/667595
Shelomi, M. (2016). Status of Europe's native & invasive phasmatodea collections. Metaleptea 36, 22–25.
Stone, G. N. (1993). Thermoregulation in four species of tropical solitary bees: the roles of size, sex and altitude. J. Comp. Physiol. B 163, 317–326. doi: 10.1007/BF00347782
Vamosi, S. M., Naydani, C. J., and Vamosi, J. C. (2007). Body size and species richness along geographical gradients in Albertan diving beetle (Coleoptera: Dytiscidae) communities. Can. J. Zool. 85, 443–449. doi: 10.1139/Z07-021
van Voorhies, W. A. (1996). Bergmann size clines: a simple explanation for their occurrence in ectotherms. Evolution 50, 1259–1264.
Walsh, C., and Mac Nally, R. (2013). hier.part: Hierarchical Partitioning. R package version 1.0-4. Available online at: http://CRAN.R-project.org/package=hier.part
Watt, C., Mitchell, S., and Salewski, V. (2010). Bergmann's rule; a concept cluster? Oikos 119, 89–100. doi: 10.1111/j.1600-0706.2009.17959.x
Whitman, D. W. (2008). The significance of body size in the Orthoptera: a review. J. Orthoptera Res. 17, 117–134. doi: 10.1665/1082-6467-17.2.117
Keywords: phasmatodea, Bergmann's rule, Allen's rule, latitudinal cline, insect ecology, morphometrics
Citation: Shelomi M and Zeuss D (2017) Bergmann's and Allen's Rules in Native European and Mediterranean Phasmatodea. Front. Ecol. Evol. 5:25. doi: 10.3389/fevo.2017.00025
Received: 28 November 2016; Accepted: 21 March 2017;
Published: 05 April 2017.
Edited by:
Thomas Buckley, Landcare Research, New ZealandReviewed by:
Alberto G. Sáez, University of Zurich, SwitzerlandDmitry Yurievich Sherbakov, Limnological Institute (RAS), Russia
Marco Gottardo, University of Siena, Italy
Copyright © 2017 Shelomi and Zeuss. This is an open-access article distributed under the terms of the Creative Commons Attribution License (CC BY). The use, distribution or reproduction in other forums is permitted, provided the original author(s) or licensor are credited and that the original publication in this journal is cited, in accordance with accepted academic practice. No use, distribution or reproduction is permitted which does not comply with these terms.
*Correspondence: Matan Shelomi, bXNoZWxvbWlAbnR1LmVkdS50dw==