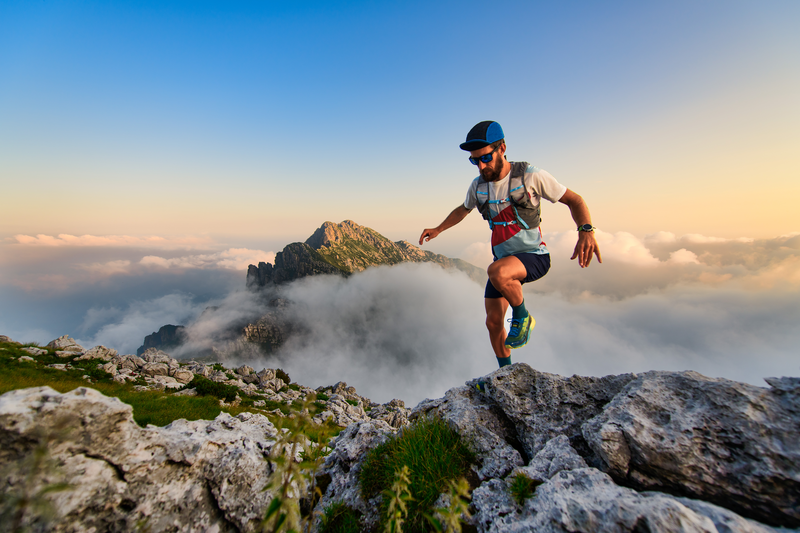
94% of researchers rate our articles as excellent or good
Learn more about the work of our research integrity team to safeguard the quality of each article we publish.
Find out more
ORIGINAL RESEARCH article
Front. Ecol. Evol. , 17 November 2016
Sec. Chemical Ecology
Volume 4 - 2016 | https://doi.org/10.3389/fevo.2016.00130
Olfactory systems evolved to detect and identify volatile chemical cues, in many cases across great distances. However, the precision of copulatory and oviposition behaviors suggest that they may be guided by olfactory cues detected by sensory systems located on or near the ovipositor. Here we present evidence of a small number of functional olfactory sensilla on the ovipositor of the hawkmoth Manduca sexta. Gene expression analysis of isolated ovipositor tissue indicated active transcription of gustatory and both classes of olfactory receptor genes. Expression of the olfactory co-receptor ORCo and the antennal ionotropic co-receptors IR8a and IR25a suggests that functional olfactory proteins may be present in the sensory structures located on the ovipositor. Scanning electron microscopy identified five to nine porous sensilla on each of the anal papillae of the ovipositor. Furthermore, HRP immunostaining indicated that these sensilla are innervated by the dendrite-like structures from multiple neurons. Finally, we functionally characterized neural responses in these sensilla using single sensillum recordings. Stimulation with a panel of 142 monomolecular odorants revealed that these sensilla indeed house functional olfactory sensory neurons (OSNs). While it remains to be determined what role these chemosensory sensilla play in odor and gustatory guided behaviors, our data clearly demonstrate an olfactory function for neurons present in M. sexta ovipositor sensilla.
Among the most important sensory abilities of winged insects is the ability to detect, identify and locate the sources of relevant volatile chemical cues. Indeed the chemical senses are involved in the guidance of a large number of evolutionarily critical behaviors (Hansson and Stensmyr, 2011). Olfactory neuronal networks allow insects to assess the chemical composition of their environment in order to locate, for example food sources, available mating partners, or suitable oviposition sites (Reisenman and Riffell, 2015; Hansson and Wicher, 2016). The role of olfactory function in oviposition and the accuracy with which many insects commit this behavior is exceptional and suggests a far more involved role for olfaction in determining precise positioning of the egg on specific plant tissue.
Odorants are primarily detected by olfactory sensory neurons (OSNs) situated on the antennae and, to a lesser extend, the maxillary palps (Wicher, 2015). The dendrites of these OSNs stretch into porous hair-like structures, the olfactory sensilla. Finally, it is the chemically-sensitive receptor in the dendritic membrane of these sensory cells that mediate the neural response to odorants (Wicher, 2015). The receptor types expressed in each OSN determine its detection spectrum. Insect receptor types known to respond to volatiles mostly belong to two receptor families, the olfactory receptors (ORs; Clyne et al., 1999; Gao and Chess, 1999; Vosshall et al., 1999) and the antennal ionotropic receptors (IRs; Benton et al., 2009). ORs are seven-transmembrane receptors related to gustatory receptors (GRs) (Robertson et al., 2003) that form a heteromultimeric complex composed of a variable OR-X protein and the co-receptor ORCo (Neuhaus et al., 2005). While OR subunits determine ligand-specificity ORCo is required for localization and maintenance of ORs in ciliary membranes of OSNs (Hallem et al., 2004; Larsson et al., 2004; Benton et al., 2006; Vosshall and Hansson, 2011). The OR/ORCo complex acts as an ion channel (Sato et al., 2008; Wicher et al., 2008), and potentially also employs metabotropic signaling (Wicher et al., 2008; Stengl, 2010; Stengl and Funk, 2013). The unrelated antennal IRs were more recently identified as odorant detecting (Benton et al., 2009); they likely form heteromeric complexes with either of two IR co-receptors, IR8a or IR25a and variable IR partners (Abuin et al., 2011; Rytz et al., 2013). A third co-receptor, IR76b, has been suggested as well (Abuin et al., 2011). However, such IRs are also involved in the detection of other sensory stimuli, like tastants and sound (Senthilan et al., 2012; Zhang et al., 2013; Koh et al., 2014).
In recent years, several studies have reported expression of genes encoding olfactory receptors not only on the antennae and maxillary palps but also in ovipositors of a variety of lepidopteran species, yet their function remains largely unknown. For example, in the noctuid Sesamia nonagrioides, Glaser et al. reported expression of both IRs and ORs, as well as ORCo (Glaser et al., 2013); in pheromone glands and ovipositor of the grass moth Chilo suppressalis, expression of two ORs but no ORCo has been described (Xia et al., 2015). Finally, in Heliothis virescens two pheromone receptors are expressed in the ovipositor as well, where they might be involved in feedback regulation of the pheromone release (Widmayer et al., 2009), but again their function has not been confirmed. Additionally, morphological studies of the ovipositors of Monopis crocicapitella and Homoeosoma nebulella reported sensilla with a multiporous surface (Faucheux, 1988, 1991), a feature generally distinguishing olfactory from gustatory sensilla, which usually have one terminal pore (Altner and Prillinger, 1980).
While olfactory function has not been demonstrated yet, several studies on a large variety of lepidopteran species have shown that the ovipositor is indeed equipped with sensory neurons that detect both mechanical and contact chemosensory (i.e., gustatory) stimuli (Yamaoka et al., 1971; Waladde, 1983; Klijnstra and Roessingh, 1986; Qiu et al., 1998; Banga et al., 2003; Maher and Thiery, 2004; Maher et al., 2006). A recent study by Seada et al. demonstrated that four gustatory neuron types housed in sensilla on the ovipositor of Spodoptera littoralis detect salt, caffeine, sugar and water, and therefore all major taste modalities (Seada et al., 2016). However, the presence of putative olfactory sensilla as well as expression of olfactory receptor genes in a variety of species raises the question: Is there an olfactory function for any of the sensilla located on the ovipositor?
One problem in answering this question is that presently information on gene expression and morphology are not correlated in a single species; a second is the absence of functional studies using volatile stimuli. Finally, since olfactory function in the ovipositor would likely play a role in either mating or oviposition, behaviors that are generally connected to mating status, an analysis of differences between mated and virgin females would be useful. We specifically looked for morphological and physiological indicators of olfactory function in the sensilla of the ovipositor of both mated and virgin females of a single lepidopteran species, the hawkmoth Manduca sexta. The species is well described with regards to olfactory structure, function and odor guided behavioral performance within an ecological context (Hansson, 1995; Shields and Hildebrand, 2001; Riffell et al., 2009; Reisenman et al., 2010, 2013; Stengl, 2010; Allmann et al., 2013; Späthe et al., 2013b; Ghaninia et al., 2014). Furthermore, the chemosensory receptor families have been identified in this model (Patch et al., 2009; Große-Wilde et al., 2010; Grosse-Wilde et al., 2011; Howlett et al., 2012; Koenig et al., 2015; Kanost et al., 2016). Here, we provide new information on gene expression, sensillar morphology, and studies confirming olfactory function in response to a large panel of volatile organic compounds for a subset of sensilla located on the ovipositor.
Animals were taken from established M. sexta colonies at either the Max Planck Institute for Chemical Ecology, Jena, Germany or the University of Kassel, Germany. Larvae used in the experiments were reared on artificial diet (Bell and Joachim, 1976), under either 16:8 h light: dark photoperiod with a relative humidity of 75% at 26°C (MPI for Chemical Ecology) or 17:7 h light: dark photoperiod with a relative humidity of 40–55% at 26°C (University of Kassel). For acquisition of adult virgin female animals, pupae were transferred individually to paper bags and kept separate until needed. For mated samples, females were kept with males in a flight cage for at least 24 h.
For each sample ovipositors, which contain abdominal segments 8–11, three M. sexta females were dissected. These segments were chosen since the pressure that needed to be exerted on the abdomen of the animal to make the area in question accessible prevented contamination-free dissection of a smaller sample. Afterwards, samples were transferred to Tri Reagent (Sigma-Aldrich, USA). Samples were homogenized for 5 min at 50 Hz with two 3 mm steel beads (Qiagen, Germany) using a TissueLyser (Qiagen, Germany). Samples were stored at −20°C. RNA isolation was performed according to manufacturer's instruction.
Library construction and sequencing was performed by the Max Planck Genome Centre Cologne, Germany (http://mpgc.mpipz.mpg.de/home/). One microgram of total RNA from each sample was poly-A enriched and then used for TruSeq RNA library preparation. The library was sequenced on an Illumina HiSeq 2500 sequencer. We obtained 13,054,453 single reads of 100 bp for the virgin sample, and 11,771,158 for the mated sample. The RNAseq data is available at the European Nucleotide Archive (http://www.ebi.ac.uk/ena/data/view/PRJEB11891, accession PRJEB11891).
For mapping we used the official gene set 2 (OGS 2) of M. sexta, which can be accessed from https://i5k.nal.usda.gov/Manduca_sexta or ftp://ftp.bioinformatics.ksu.edu/pub/Manduca/OGS2/. The OGS 2 was updated for OR, IR, and GR sequences as reported in Koenig et al. (2015). The reads were mapped to the reference and the expression values were calculated as RPKM (Reads per kilobase per million mapped reads) using CLC Genomics Workbench v8.5 (http://www.clcbio.com) with default settings.
Total RNA was isolated from single ovipositors of virgin or mated females as described for RNAseq. Mated animals were allowed to lay eggs on Nicotiana attenuata before dissection. The mating status of the animal was confirmed if larvae hatched from the eggs. RNA samples were treated with TurboDNAse (Ambion, USA). Three biological replicates were obtained for each mating status. The RNA samples served as template for cDNA synthesis using the SuperScript III Reverse Transcriptase (Invitrogen, USA) and oligo-dT Primers according to the manufacturer's instructions. PCRs were performed using Advantage 2 Polymerase Mix (Clontech, USA) and gene specific primers (Supplementary Table S1) under the following conditions: 5 min 94°C, 35 cycles [30 s 94°C, 30 s (temperature see Ta in Supplementary Table S1), 90 s 68°C], 3 min 68°C.
Samples were loaded onto a 1.5% agarose gel. Resulting bands were excised, extracted using the EZNA Gel Extraction Kit (Omega, USA) according to the manual, and sequenced on a 3730XL DNA Analyzer (Applied Biosystems, USA).
For antennae, ovipositors and central brains the respective tissues of five virgin or mated females were pooled, for forleg muscle samples tissues of two male M. sexta. Tissues pools were homogenized in radioimmunoprecipitation assay (RIPA) buffer [50 mM Tris-HCl, 30 mM NaCl, 1% (v/v) IGEPAL CA-360, 1% (w/v) sodium cholate hydrate, 0.1% (w/v) SDS; 30 μl/antenna, 10 μl/ovipositor and central brain, 120 μl/pooled muscle tissue] containing protease inhibitors (Roche cOmplete ULTRA, Roche Applied Science, Germany), and incubated for 30 min at 60°C. After centrifugation (2 × 5 min, 17,000 g), supernatants were diluted 1:2 with sample buffer and incubated for 10 min at 90°C. Volumes of 10 μl/lane were then run on a 10% polyacrylamide gel and blotted for 1 h at 100 V to polyvinylidenfluorid membrane. The membrane was blocked in Tris-buffered saline (TBS) containing 5% (w/v) milk-powder and 0.1% (v/v) Tween 20 for 2 h at room temperature. The anti-ORCo antibody, a gift by Jürgen Krieger (University of Halle), was raised in rabbit (Charles River Laboratories, Germany) against the peptide NH2-NQSNSHPLFTESDARYH-COOH (Squarix Biotechnology, Germany). It was used at a concentration of 1.74 ng/μl in TBS containing 0.1% (v/v) Tween 20 and 1% (w/v) milk-powder at 4°C over night. After primary antibody incubation, the membrane was washed 3 × 1, 1 × 5, 1 × 10, and 1 × 30 min with TBS containing 0.05% (v/v) Tween 20, 1% (v/v) Triton X-100, 0.1% (w/v) SDS. The HRP-coupled goat anti-rabbit secondary antibody (Dianova, Germany) was applied for 1 h diluted 1:10,000 in TBS containing 0.1% (v/v) Tween 20 and the membrane then washed as before with two additional steps of 5 min each. A LI-COR Odyssey Fc system (LI-COR Biosciences, USA) was used to visualize the chemiluminescent signal.
By applying gentle pressure to the abdomen, virgin females were incited to extend their ovipositor. The extended female ovipositors were cut between the seventh and eighth abdominal segment. The ovipositors were fixed in 4% glutaraldehyde (Sigma-Aldrich, USA) at 4°C overnight, dehydrated in an ascending ethanol series (70%, 80%, 90%, 96%, and 3 × 100% ethanol) and finally critical point dried (BAL-TEC CPD 030, Bal-Tec Union Ltd., Liechtenstein). The samples were mounted on a metal holder covered with adhesive tape and sputter coated with gold prior to examination with a scanning electron microscope (LEO 1530, Zeiss, Germany), which was set to 8 kV and an 8–11 mm working distance.
The extended ovipositors of anesthetized of both virgin and mated M. sexta females were cut between the seventh and eighth abdominal segment and fixed overnight in 4% paraformaldehyde (Carl Roth, Germany) in 1 M NaHCO3 (Sigma Aldrich, USA, pH 9.5) and 1% TritonX-100 (Sigma Aldrich, USA). All following incubation and washing steps were performed on a shaker at 4°C. After fixation the ovipositors were washed several times with 0.1 M phosphate-buffered saline (pH 7.4) containing 0.3% TritonX-100 (PBST) for at least 2 h. For sectioning with a vibratome (MICROM HM 650 V, Germany) ovipositors were embedded in 5% agarose (Carl Roth, Germany). Horizontal and sagittal sections of 100 μm were made, followed by additional washes in PBST. Pre-incubation was performed in a blocking solution containing PBST and 2% normal goat serum (Sigma-Aldrich, USA) for 6 h at 4°C. Afterwards the sections were incubated in the blocking solution containing an antibody directed against ORCo (anti-ORCo) at 1:500, and/or Cy3-conjugated Affine Pure goat anti-HRP (Jackson ImmunoResearch, USA, 1:100) and Hoechst 33342 nuclear staining (Life Technologies, Germany, 1:1500) for 2 days. Anti-HRP has been shown to bind to insect neuronal membranes (Jan and Jan, 1982; Loesel et al., 2006) and was therefore used as a neuronal marker. After incubation ovipositor sections were washed several times with PBST for at least 4 h. Preparations previously treated with anti-ORCo were then incubated with a secondary goat anti-mouse Alexa488 antibody (Thermo Fisher Scientific, Germany) at conditions as for the first antibody, again by several washes with PBST for at least 4 h. Then preparations were mounted in MOWIOL (Calbiochem, USA). Overview images were taken with an AxioZoom.V16 (Zeiss, Germany). For detailed analysis a confocal laser scanning microscope (LSM 880, Zeiss, Germany) in multitrack mode and a 40x objective (C-Apochromat 40x/1.20 W Korr M27) was used. Nuclear staining was visualized using a 405 nm laser diode, neuronal staining using a 543 nm Helium Neon or a 488 nm Argon laser. Additionally, cuticular autofluorescence was detected using a 488 nm Argon laser, which was also used to generate transmission light images.
Both virgin and mated females were used for electrophysiological measurements in this study. Two day-old virgin females were allowed to mate for 1 day and then used. Typically, mated females oviposited already in the mating cage. Virgin females were used 2–3 days after eclosion.
A 5 ml pipette tip (Biozym, Germany) was adjusted to a length of 3 cm, with a widened opening at the tapered end with a diameter of 1 cm. M. sexta females were anesthetized, and wings, legs and antennae removed. The animal was gently pushed into the prepared pipette tip, with the abdomen protruding. The ovipositor was extended by gently pressing onto the abdomen, then fixed with dental wax onto glass slides and wrapped tightly with Parafilm® (Pechiney Plastic Packaging, USA; Supplementary Figure S1).
Single sensillum recordings were made using electrolytically sharpened tungsten electrodes (Harvard Apparatus Ltd., United Kingdom). Recording electrodes were inserted into either the shaft or the base of individual sensilla using piezoelectric micromanipulators PM-10 (Märzhäuser Wetzlar GmbH, Germany) under visual guidance with an upright microscope (Olympus BX51WI, Japan). A silver wire reference electrode was placed into the eye of the moth. The signal was amplified (10x) by Syntech Universal AC/DC Probe, sampled (10,667.0. samples/s), filtered (100 Hz–3 kHz with 50/60 Hz suppression) and digitized through an IDAC-4 -USB-interface (Syntech, Netherlands), then saved to computer disk. Recordings were visualized and analyzed using Autospike v3.7 (Syntech, Netherlands).
Responses of individual neurons were quantified by subtracting the number of spikes 3 s prior to the onset of the 500 ms stimulus from the number of spikes during the subsequent 3 s. We measured the system intrinsic delay by photoionization detection (Fast Response Miniature Photo-Ionization Detector, 200A, Aurora Scientific, USA). The PID sensor was placed at the same position as the ovipositor preparation in electrophysiological recordings. Use of 2-heptanone revealed a system intrinsic delay of 273 ± 7 ms (mean ± STD, n = 22). Due to different physicochemical properties of the used chemicals and for simplification purposes, the onset of odor stimulation for purposes of spike count analysis was corrected by 250 ms across all recordings. Baseline spiking activity was quantified by counting the spikes in a 3 s window prior to the onset of the stimulus, reporting average spikes per second. Neurons were distinguished by spike amplitude, and a neuron was counted as responsive to odors if after odor stimulation with at least one compound the neuron exhibited an increase in spiking activity of at least 50% and 5 spikes/3 s.
Odor responses were normalized for each neuron and clustered using Wards agglomerative hierarchical clustering method (XLSTAT, Addinsoft, USA). Statistical tests, heatmap, and bar-diagrams were created with PAST (Palaeontological Statistics, http://folk.uio.no/ohammer/past/). The Mann–Whitney U-test was used to compare responses of OSNs of virgin and mated animals for responsive odors. Figures were assembled in Adobe Illustrator CS6 (Adobe Systems, USA).
The monomolecular odorants presented during single sensillum recordings are listed in Supplementary Table S2. All odorants were diluted to a final concentration of 10 μg/μl (1% w/v), according to their vapor pressure either in hexane (<1.5 mm Hg at 25°C) or mineral oil (>1.5 mm Hg at 25°C). Ten microliters of the diluted odors were pipetted onto a small piece of filter paper and placed inside a glass Pasteur pipette. A constant charcoal-filtered/humidified air-flow was delivered through a aluminum-tube with a length of 11 cm; its outlet was positioned 5–10 mm from the ovipositor. Approximately 8 cm apart from the metal tubes outlet was a 2 mm injection port, where odor cartridges were inserted prior to stimulation. A stimulus controller delivered the content of each cartridge by replacing half of the total air-flow. Air was then delivered over the ovipositor.
Initially we analyzed the ovipositor of M. sexta for expression of chemosensory receptor genes (ORs, GRs, IRs). We chose to perform RNAseq, using mRNA from ovipositors of virgin and mated females, three animals each, to test for expression of chemosensory receptors, and investigate potential differences due to mating status. The reads were trimmed for both adaptor sequences and quality, and mapped against a reference that was based on the available official gene set (OGS2.0, Kanost et al., 2016), with replaced chemosensory receptor gene models as described in Koenig et al. (2015). Using the resultant RPKM values as proxy for gene expression we detected candidates of all three chemosensory receptor families (Table 1). For the GRs we found the putative CO2-receptors (MsexGR2 and MsexGR3, Howlett et al., 2012; Koenig et al., 2015) in the datasets. Several IR transcripts were present (MsexIR1, MsexIR4, MsexIR7d, MsexIR68a, MsexIR75d, MsexIR75p.2, and MsexIR76b), as well as two OR transcripts (MsexOR9 and MsexOR26). Most crucially, the co-receptors of both the IR and OR receptor families (MsexIR8a, MsexIR25a, MsexORCo) exhibited expression as well, indicating potential olfactory function. However, we need to note that expression of several genes in one or both samples was at or below the detection limit of RNAseq (<RPKM of 1). Therefore, we opted to verify both expression of the identified candidate genes in general, and qualitative differences between virgin/mated animals with RT-PCR (Figure 1A). Using this approach, we were able to confirm expression of MsexGR2, MsexGR3, MsexIR7d, MsexIR8a, MsexIR25a, MsexIR75d, MsexIR76b, MsexOR9, MsexOR26, and MsexORCo across multiple replicates (≥3; Figure 1A). However, we were not able to verify that expression of any of these genes was restricted to animals of either mating status, although quantitative differences could not be excluded.
Figure 1. (A) Representative RT-PCR amplifications of RL31 (house keeping gene, encoding a ubiquitous ribosomal protein) and several chemosensory receptor transcripts using female cDNA of virgin and mated M. sexta ovipositor tissue. Bands of the predicted size revealed expression of MsexGR2, MsexGR3, MsexIR7d, MsexIR8a, MsexIR25a, MsexIR75d, MsexIR76b, MsexOR9, MsexOR26, and MsexORCo in both virgin and mated M. sexta ovipositors. M, marker; ant, female antenna; mat, mated; ovi, ovipositor; vir, virgin; w, water; RL31, ribosomal protein L31. (B) Western blot of different M. sexta tissues including ovipositor tissue. A signal near the predicted molecular weight of MsexORCo (53.7 kDa) indicates that the antibody recognizes MsexORCo in mated female antennae (ant) and ovipositor tissues of virgin and mated animals (ovi 1 and ovi 2 represent two independent samples). Central brain (cb) and male muscle (mm) tissues lack a signal at the approximate size of MsexORCo.
In contrast to IRs, non-olfactory functions have not been reported for ORs/ORCo. Accordingly, the detection of ORCo transcripts was the best indication for presence of OSNs in the ovipositor. To acquire additional evidence we used an antibody directed against an epitope common in ORCo of Lepidoptera (Nolte et al., under revision) with tissue-specific western blots. Each blot included protein preparations of antennal, central brain, and muscle tissue for control, as well as two independent ovipositor protein preparations for each mating status (virgin and mated). After hybridization with the ORCo-antibody, a secondary antibody and subsequent development, a band matching the predicted molecular weight of MsexORCo (53.7 kDa) could be detected for ovipositor preparations of animals of either mating status (Figure 1B). As expected, a band of the same size was also present for the antennae, but not for central brain or muscle tissue (Figure 1B). Control experiments omitting the ORCo-antibody did not yield this band (Supplementary Figure S2).
After we found expression of chemosensory receptor genes, as well as the presence of ORCo protein in ovipositor protein preparations of M. sexta, we searched for putative chemosensory sensilla. The ovipositor of M. sexta consists of a pair of anal papillae surrounding the ovipore, and the papillae are described as covered with short, pointed microtrichia and tubercles, bearing one larger sensillum each (Eaton, 1986). We examined the surface of the anal papillae using scanning electron micrography, and found many sensilla with a poreless surface that ended in bent, fine-pointed tips (Figure 2A, arrowheads). These sensilla were inserted into raised sockets, indicating a potential function in mechanosensation (Figure 2B, arrowhead). Additionally, on each of the anal papilla we were able to identify four to nine blunt-tipped sensilla (n = 3; Figure 2A, arrows; Figure 2B, asterisks). In contrast to the fine-pointed sensilla, the surface of the blunt-tipped sensilla was porous including a terminal pore, suggesting a function in chemosensation, and possibly olfaction (Figure 2C).
Figure 2. Scanning electron micrographs (SEM) of the ovipositor of M. sexta and its associated sensilla. (A) Ventral overview of the extended terminal segment of the M. sexta ovipositor, which contains two anal papillae surrounding the ovipore (o). Most abundant are filiform sensilla (arrowheads), which vary in length. Nine putative chemosensory sensilla occur on each papilla (arrows). White box and letter in this picture presents the place where the following picture originates. (B) The non-porous, filiform sensilla have raised sockets (arrowheads). Asterisks indicate putative chemosensory sensilla with sockets showing flexible areas (arrows). The papillae are covered with short-pointed microtrichia (m) and tubercles (t; indicated by dashed circle). (C) Detailed view of one putative olfactory sensillum. The shaft is pierced by numerous small pores and one large terminal pore (arrowheads). Scale bars: (A) 100 μm; (B) 20 μm; (C) 1 μm.
Next, we looked for OSNs in the ovipositor. Immunohistochemistry was performed using the antibody directed against ORCo. However, we were not able to identify any resulting signals in the ovipositor, even though we did detect signals in other chemosensory tissues using the same antibody (data not shown). To test for presence of neurons we employed an antibody directed against horseradish peroxidase (HRP), which is commonly used as neuronal marker (Jan and Jan, 1982; Loesel et al., 2006; Figures 3A–H). Experiments were performed using both virgin and mated females. Using this approach, we were able to identify four to five cell-shaped signals close to the porous sensilla (Figures 3B–D). The size of the stained cell bodies was not uniform; in each case, one larger soma was present; across 15 sensilla, the larger soma averaged 12.9 μm diameter (STD = 2.9 μm), the smaller somata had an average diameter of 5.9 μm (STD = 1.1 μm) (Figures 3C,D). Additionally, we found labeling of dendrite-like structures within the sensillum shaft, with the labeling terminating near the sensillum tip (Figures 3E,F). In no case were the signals directly connected to the sensillum socket, contradictory to a possible mechanosensory function. All labeled cells also possessed axon-like protrusions (Figures 3B–D, arrows). Based on their morphological characteristics we conclude that the labeled cells are neurons innervating the porous sensilla. In comparison, for the more copiously present non-porous sensilla we found one associated neuron each (Figures 3B,G,H). Here, the dendritic tip was attached to the base of the sensillum, consistent with a previously suggested function of these sensilla in mechanosenation. These fine-tipped, non-porous sensilla were named sensilla trichodea by Eaton (1986); based on their morphology, we suggest renaming them as sensilla chaetica according to the terminology by Altner and Prillinger (1980). Classification of the nine putative chemosensory sensilla we newly identified remains ambiguous; they exhibit similarities to antennal trichoid sensilla, especially the porous surface, but also to the scattered blunt sensilla on tibia and tarsus, with which they share general shape (Kent and Griffin, 1990). We did not see any differences between mated and virgin females.
Figure 3. Laser scanning micrographs of a 100 μm thick horizontal vibratome section of the ovipositor of M. sexta. Neurons were labeled using Cy3-conjugated anti-HRP (red), nuclei using Hoechst 33342 (blue), and the cuticle (green) was visualized using the cuticular autofluorescence. (A) Picture of a 100 μm thick horizontal vibratome section through a M. sexta ovipositor visualized using a fluorescent stereomicroscope. Cuticular anal papillae, which bears the tubercles with the sensilla have a higher autofluorescence (green) and are on the left and right side of the slide. The ovipore is in the upper part of the image between the two anal papillae. The excerpt shown in (B) is indicated by the white box. (B) Total projection of a confocal laser scanning z-stack, imaging autofluorescence (green), cell nuclei (blue), anti-HRP-immunoreactivity (red), and transmitted light. It shows a part of an anal papillus bearing sensilla chaetica (Sch) and a putative chemosensory sensillum (asterisk). Anti-HRP-immunoreactive cells have dendritic- (arrowheads) and axon-like extensions (arrows); resembling the morphology of neurons. White boxes and letters in this picture present the place where the following pictures originate. (C) Single confocal plane, focusing on multiple anti-HRP-immunoreactive cells (red) below a putative chemosensory sensillum. Indicated are dendritic- (arrowheads) and axon-like (arrows) extensions of those cells; nuclei were stained with Hoechst (blue). Other stained nuclei belong to support or epidermal cells. (D) A second confocal plane of the same section, showing two additional neurons. Numbers in (C,D) label, the different neurons, which are five for this putative chemosensory sensillum. Indicated are dendritic-(arrowheads) and axon-like (arrows) extensions. (E) Single confocal plane showing some dendritic extensions, which can be followed up into the sensillum shaft (arrowhead), however they cannot be assigned to specific neurons due to the very bright HRP signals in the transition zone between inner and outer dendritic segments. (F) In another confocal plane, the dendrites end close to the sensillum tip (arrowhead). (G) Confocal plane, through a single HRP- immunoreactive cell beneath a Sensillum chaeticum (Sch). The prospective dendrite terminates at the base of the sensillum (arrowhead), not extending into the shaft, which indicates a mechanosensory function of this sensillum. (H) Projection of 30 optical sections, showing a second Sensillum chaeticum (Sch). Also here a single anti-HRP-immunoreactive cell was found below, with its dendrite-like extension ending (arrowhead) at the sensillum base. Scale bars: (A) 200 μm; (B) 50 μm; (C–E,G,H) 10 μm; (F) 5 μm.
Finally, we determined whether these identified putative chemosensory sensilla served an olfactory function using single sensillum recordings. Although, our initial results did not indicate a clear difference between virgin and mated animals, both were chosen for the experiments, and mating status recorded. When electrophysiological measurements were made we noted four to nine (n = 36) putative chemosensory sensilla for each anal papilla on ovipositor preparations. For stimulation we used a panel of odorants that are either known to be detected by the antennae of M. sexta, and/or reported as being relevant for specific behaviors, as well as additional volatiles to reasonably cover chemical space. Accordingly, the panel contained 142 odorants that could be roughly classified into acids, alcohols, aldehydes, esters, amines, aromatics, ketones, lactones, quinones, and terpenes (Supplementary Table S2). Single sensillum recordings clearly showed functionally distinct neuronal units within each sensillum, with baseline spiking activity between 0 and more than 15 spikes/s. Based on our results from immunohistochemistry we expected responses from one to five neurons. However, when differentiating neurons by spike amplitude we consistently found only one or two neurons per sensillum exhibiting baseline spiking activity (Figures 4, 6). The neurons with baseline spiking activity will be called OSNs in the following text. We were not able to record spiking activity from the additional cells that were present based on the findings of the immunohistochemistry, both before and after stimulation with odorants.
Figure 4. Representative single sensillum recording (SSR) traces of a putative chemosensory M. sexta ovipositor sensilla. (A) The recorded ovipositor sensillum housed two olfactory sensory neurons (OSNs), A (larger spike amplitude) and B (smaller spike amplitude). Stimulation with γ-hexalactone elicits strong and sustained responses in the A-neuron whereas the B-neuron exhibits very weak spiking activity. (B) Excitatory, sustained response of the B-neuron (small spike amplitude) to 4-methyl-1-pentanol whereas the A-neuron did not respond to the stimulus. (C) None of the two OSNs responded to the solvent hexane alone. All odorants were diluted to a final concentration of 10 μg/μl either in hexane or mineral oil. The stimulus bar (500 ms) was corrected by the system intrinsic delay of 250 ms. All responses shown are from the same recording.
In total, 30 out of 49 tested sensilla exhibited responses from a single OSN. The remaining 19 sensilla showed two distinct baseline spiking amplitudes, likely originating from two neurons. Out of these 19 sensilla, only in nine sensilla both neurons responded to stimulation with odorants. The other 10 sensilla contained one responding neuron, while the other neuron showed baseline activity but did not respond to odorants from our panel. Responses for all 58 OSNs that reacted to at least one of the tested odorants are summarized in Figure 5.
Figure 5. Odorant response profiles of 58 individual OSNs (x-axis) in response to 142 individually presented odorants (y-axis) from 49 sensilla from the ovipositor of female M. sexta moths. Classification dendrogram was generated using wards agglomerative hierarchical clustering method. The dotted line represents the automatic truncation, leading to four functional neuron classes (I–IV). Responses are normalized and color coded for each neuron: red indicates the maximum response by the given OSN across all odorants; yellow, medium excitation (50%); light green, weak excitation (25%); light blue, no response and dark blue indicates that spontaneous spiking activity was reduced compared to baseline. All odorants were diluted to a final concentration of 10 μg/μl either in hexane or mineral oil. Asterisks symbolize mated females. The colored dots refer to Figure 6 where SSR traces of these OSNs are shown to represent each of the four functional OSN types.
Classification using Wards agglomerative hierarchical clustering (Figure 5) identified four distinct functional OSN types. The first type responded to odorants from several chemical classes, but the strongest responses were elicited by alcohols, especially 4-methyl-1-pentanol (Figures 4B, 6A). The second functional OSN type displayed a more narrow response profile, showing strong responses only to stimulation with p-toluquinone, and weaker responses to p-benzoquinone and pyrrolidine (Figure 6B). The third functional type responded mainly to γ-hexalactone, and to a lesser degree to (S)-verbenol and γ-valerolactone (Figure 6C). The last type responded best to γ-hexalactone and (S)-verbenol, but also exhibited strong to medium responses to γ-heptalactone, γ-valerolactone and p-toluquinone (Figure 6D).
Figure 6. Odor response spectra of the four functional OSN types with representative single sensillum recording (SSR) traces. Depicted single OSNs are marked in Figure 5 with colored dots. Reproduced are 20 representative odors eliciting responses in at least one of the four functional types of OSNs. (A) The first type responds to odorants of several chemical classes. The recorded ovipositor sensillum housed two OSNs, A (larger spike amplitude) and B (smaller spike amplitude). Only B-neurons with small spike amplitude clustered into this type. Stimulation with 4-methyl-1-pentanol elicits strong and sustained responses in the B-neuron whereas the A-neuron did not respond to the stimulus. The B-neurons (small spike amplitude) responded to the GLV cis-3-hexenol whereas the A-neuron did not exhibit spiking activity. First and third trace of this type also appeared in Figure 4. (B) The second OSN type showed a more narrow response profile. In the depicted traces only a single neuron (A-neuron) exhibited stronger responses to stimulation with p-toluquinone, and weaker to pyrrolidine. (C) The depicted third type OSN exhibited strong and weak excitatory response of an A-neuron (large spike) to γ-hexalactone and γ-valerolactone. The B-neuron did not respond to any of the odors tested, but exhibited generally high spontaneous activity. (D) The last type responded best to γ-hexalactone and (S)-verbenol. Representative traces from a sensillum potentially housing a single OSN (A-neuron). In all cases, none of the OSNs responded to the solvent hexane alone. Bold, red letters indicate if either OSN A (larger spike amplitude) or OSN B (smaller spike amplitude) corresponds to the respective functional type. The pairing of OSN types was not stereotypical. All odorants were diluted to a final concentration of 10 μg/μl either in hexane or mineral oil. The stimulus bar (500 ms) was corrected by the system intrinsic delay of 250 ms in all traces. Error bars represent standard deviation.
Not a single cluster contained responses only from mated or virgin animals, thus we did not find any qualitative differences based on mating status. Furthermore, we looked for differences between virgin and mated animals using the Mann–Whitney U-test for cluster (III) and (IV), applied for each odorant. No significant differences were found (p in all cases ≥ 0.28).
In general, spiking frequencies of OSNs in the ovipositor were low compared to antennal OSNs. The maximal spiking activity observed after stimulation was ~41 spikes/s (type I, mean ~24 spikes/s) in the first type of OSN after application of 4-methyl-1-pentanol (Figure 6A). The other types showed lower maximal responses, with the lowest occurring in the second type, where stimulation with p-toluquinone elicited ~12 spikes/s (type II, mean ~7 spikes/s; Figure 6B).
Our results demonstrate that a small number of sensory sensilla located on the ovipositor of the tobacco hornworm M. sexta possess cells that function as OSNs. Both expression of chemosensory receptors genes and western blot analyses of OR co-receptor ORCo support presence of olfactory function. We also discovered that porous, putative chemosensory sensilla housing dendrites of multiple neurons exist on the anal papillae. Finally, electrophysiological measurements from the identified putative chemosensory sensilla demonstrated the presence of neuronal spiking activity, with the observed neurons responding to distinct sets of odorants with an increase in firing rate. We were not able to confirm any differences between virgin and mated individuals in general as well as in gene expression analysis. Nevertheless, one might expect a broad diversity of expression of receptor types since the primary behaviors involving the ovipositor are clearly of primary importance for mating and/or oviposition in females. For example, participation of the chemosensory function of the ovipositor in mating behaviors likely plays a role in proper mate selection. It is conceivable that, male-emitted secretions released by hairpencils in M. sexta might be detected with the ovipositor to facilitate mating behavior (Grant and Eaton, 1973; Birch et al., 1990). In M. sexta, composition of the secretion partially remains elusive, but para- and meta-cresol have been identified as components (Reisenman et al., 2009). Furthermore, mated females need to carefully scrutinize potential oviposition sites, an obvious candidate behavior for the involvement of olfaction in the ovipositor. There are several results that are noteworthy in this context. Single sensillum recordings revealed neurons that did not respond to stimulation with any odorant tested (Figure 6C, B-neurons). We assume that these neurons might be chemosensory, but they may detect compounds not included in our panel. Accordingly, such neurons could be narrowly tuned, a feature common for OSNs detecting odorants of crucial importance to specific behaviors. Good examples would be pheromones in moths (Roelofs et al., 1974; Kaissling et al., 1978, 1989; Tumlinson et al., 1989; Kalinová et al., 2001; Stengl, 2010) or markers for important toxins in flies (Stensmyr et al., 2012). Obviously, neurons with such narrow tuning would make prime candidates for the detection of compounds connected to mating status specific behaviors. Alternatively, the non-responsive neurons could be non-olfactory.
This later interpretation is consistent with the observation that there were more immuno-labeled cells within individual sensilla than spike types observed in the sensillar recordings. Thus, based on their morphology we suggest that the additional neurons observed in these sensilla could also be involved in the detection of other modalities. In addition to a possible mechanosensory function, the non-active neurons may be sensitive to CO2, gustatory cues, or possibly humidity. The expression of the two putative CO2-receptor MsexGR2 and MsexGR3 further suggests that these sensory functions likely exist in the ovipositor. Also expressed is MsexIR76b, which in Drosophila melanogaster serves in the detection of polyamines (Hussain et al., 2016) and salts (Zhang et al., 2013); chemosensory sensilla detecting salts have been found on the ovipositors of Chilo partellus, Eldana saccharina, and Pieris brassicae (Waladde, 1983; Klijnstra and Roessingh, 1986). Finally, mechano- and hygroreceptive functions in ovipositors have both been reported for other species, and suggested for M. sexta (Waladde, 1983; Qiu et al., 1998; Banga et al., 2003; Seada et al., 2016).
Generally, involvement of GRs and IRs seems likely; responses to amines and hexanoic acid, for example, might be explained by IRs, such as IR75d which in D. melanogaster responds to pyrrolidine (Rytz et al., 2013) (Figure 6B). However, the involvement of ORs/ORCo in the measured responses is unclear. While present in the abdominal segments 8–11 based on gene expression and western blots, we were unable to directly associate them with the identified chemosensory sensilla. Given that we were able to detect MsexORCo in other tissues, this might indicate a function not in association with the sensilla studied. However, responses to several tested compounds suggest otherwise. For example, lactones elicited responses in several measurements, and so far, only ORs have been associated with the detection of lactones in any insect species (Hallem and Carlson, 2006; Pask et al., 2013).
Based on the response profiles of measured OSNs we can speculate on connected behaviors. Especially interesting is the first type, for which 4-methyl-1-pentanol elicited maximal responses, but also detects several other green leaf volatiles (GLVs), like cis-3-hexenol and trans-2-hexenol (Figure 6A). Many GLVs are known semiochemicals of special importance for several insect species with some GLVs acting as a plant defense (Scala et al., 2013). For example, N. attenuata (a host plant of M. sexta) will emit GLVs such as methyl jasmonate when damaged by M. sexta larvae, these semiochemical cues then attract predators of M. sexta (Halitschke et al., 2001; Kessler and Baldwin, 2001; Allmann and Baldwin, 2010). Furthermore, female M. sexta can identify herbivore-damaged plants by their volatile emissions, and will avoid ovipositing on N. attenuata and Solanum lycopersicum (tomato) when the plant is damaged, but not on Datura wrightii (Reisenman et al., 2013; Späthe et al., 2013b). Using olfactory cues, M. sexta females preferentially choose D. wrightii over N. attenuata headspaces (Späthe et al., 2013a). Additionally D. wrightii flowers use odor to attract females of M. sexta for pollination; females also use these cues in oviposition behavior (Reisenman et al., 2010). While GLVs are detected by the adult antennae (Shields and Hildebrand, 2001; Ghaninia et al., 2014) it is conceivable that the detection in the ovipositor can serve as a last warning at close proximity to a prospective oviposition site. In this context one specific, potentially ovipositor-connected, olfactory-driven behavior that has been reported previously could be important. In wind tunnel experiments M. sexta females respond to attractive host plant odors not only with upwind flight, but also abdominal curling on approach; the abdominal curling occurs even when the animals are prevented from contacting the host plant (Mechaber et al., 2002). Curling of the abdomen after the extension of the last two abdominal segments is a precursor of egg deposition (Eaton, 1986), but has also been described as a typical acceptance posture in female butterflies (Nishida et al., 1996) and a posture toward the courting male in H. virescens (Hillier and Vickers, 2004). Since contact to the plant in the experiments by Mechaber et al. was not necessary to trigger this behavior in M. sexta, it is likely elicited by volatile cues. It seems possible that the ovipositor is able to detect such olfactory cues, and probably serves as an element in the chain for acceptance and rejection for oviposition site choice. If this is true, the observed in-flight abdominal curling might well represent sampling of volatiles (Mechaber et al., 2002), triggered as a consequence of other behaviors, likely mediated by antennal olfaction. In other moth species, antennae are clearly involved in mating and male acceptance (Hillier and Vickers, 2004; Roscoe et al., 2016), but it remains to be investigated whether ovipositor OSNs are involved as well. However, host choice experiments with antennectomized females could help determining the contribution of ovipositor olfactory sensory structures in mediating oviposition and/or mating acceptance.
In summary, we have demonstrated that female M. sexta can detect odorants with sensilla located on their ovipositor; the nature of the tissue and the detected odorants suggests a potential role in behaviors connected to reproduction. However, the exact nature of this involvement and the related behaviors are still elusive.
Conceived and designed experiments: CFK, EG, and BH; performed scanning electron micrography (SEM): CM, CFK; performed Immunohistochemistry: CM; performed RNASeq: CK; performed RT-PCR: CK, CFK; performed western blots: AW; performed single sensillum recordings (SSR): CFK; data analysis/analysis tools: CFK, SB, KD, EG, and BH. Drafted/corrected the paper: CFK, EG, BH, MS, KD, CK, CM, SB, and AW. All authors gave final approval for publication.
This work was supported by the Max-Planck-Society.
The authors declare that the research was conducted in the absence of any commercial or financial relationships that could be construed as a potential conflict of interest.
The reviewer NKH declared a past collaboration with several of the authors EG, KD to the handling Editor, who ensured that the process met the standards of a fair and objective review.
The authors thank Sandor Nietzsche from the Electron Microscopy Center Jena, Sascha Bucks and Johanna Meyer for assistance with molecular biology work. Furthermore we thank Jaime Martinez-Harms for support and helpful advice, Veit Grabe for assistance in figure preparation, Sylke Dietel-Gläßer for insect rearing, and Daniel Veit for technical support. We also thank Michael Thoma and Hany Dweck for support and helpful advice in electrophysiology. We are grateful to Dr. T. Gerke (Henkel AG & Co. KGaA, Düsseldorf, Germany) for providing the Henkel 100 mixture.
The Supplementary Material for this article can be found online at: http://journal.frontiersin.org/article/10.3389/fevo.2016.00130/full#supplementary-material
Abuin, L., Bargeton, B., Ulbrich, M. H., Isacoff, E. Y., Kellenberger, S., and Benton, R. (2011). Functional architecture of olfactory ionotropic glutamate receptors. Neuron 69, 44–60. doi: 10.1016/j.neuron.2010.11.042
Allmann, S., and Baldwin, I. T. (2010). Insects betray themselves in nature to predators by rapid isomerization of green leaf volatiles. Science 329, 1075–1078. doi: 10.1126/science.1191634
Allmann, S., Späthe, A., Bisch-Knaden, S., Kallenbach, M., Reinecke, A., Sachse, S., et al. (2013). Feeding-induced rearrangement of green leaf volatiles reduces moth oviposition. Elife 2013:e00421. doi: 10.7554/eLife.00421
Altner, H., and Prillinger, L. (1980). Ultrastructure of invertebrate chemo-, thermo-, and hygroreceptors and its functional significance. Int. Rev. Cytol. 67, 69–139. doi: 10.1016/S0074-7696(08)62427-4
Banga, N., Albert, P. J., Kapoor, N. N., and McNeil, J. N. (2003). Structure, distribution, and innervation of sensilla on the ovipositor of the spruce budworm, Choristoneura fumiferana, and evidence of a gustatory function for type II sensilla. Can. J. Zool. 81, 2032–2037. doi: 10.1139/z03-175
Bell, R. A., and Joachim, F. G. (1976). Techniques for rearing laboratory colonies of tobacco hornworms and pink bollworms. Ann. Entomol. Soc. Am. 69, 365–373. doi: 10.1093/aesa/69.2.365
Benton, R., Sachse, S., Michnick, S. W., and Vosshall, L. B. (2006). Atypical membrane topology and heteromeric function of Drosophila odorant receptors in vivo. PLoS Biol. 4:e20. doi: 10.1371/journal.pbio.0040020
Benton, R., Vannice, K. S., Gomez-Diaz, C., and Vosshall, L. B. (2009). Variant ionotropic glutamate receptors as chemosensory receptors in Drosophila. Cell 136, 149–162. doi: 10.1016/j.cell.2008.12.001
Birch, M. C., Poppy, G. M., and Baker, T. C. (1990). Structures of male moths. Annu. Rev. Entomol. 35, 25–58. doi: 10.1146/annurev.en.35.010190.000325
Clyne, P. J., Warr, C. G., Freeman, M. R., Lessing, D., Kim, J., and Carlson, J. R. (1999). A novel family of divergent seven-transmembrane proteins: candidate odorant receptors in Drosophila. Neuron 22, 327–338. doi: 10.1016/S0896-6273(00)81093-4
Eaton, J. L. (1986). Morphology of abdominal segments eight and nine of the female tobacco hornworm, Manduca sexta (Lepidoptera: Sphingidae). Ann. Entomol. Soc. Am. 79, 629–635. doi: 10.1093/aesa/79.4.629
Faucheux, M. J. (1988). Multiporous sensilla on the ovipositor of Monopis crocicapitella Clem (Lepidoptera: Tineidae). Int. J. Insect Morphol. Embryol. 17, 473–475. doi: 10.1016/0020-7322(88)90026-8
Faucheux, M. J. (1991). Morphology and distribution of sensilla on the cephalic appendages, tarsi and ovipositor of the European Sunflower Moth, Homoeosoma nebulella Den. & Schiff. (Lepidoptera: Pyralidae). Int. J. Insect Morphol. Embryol. 20, 291–307. doi: 10.1016/0020-7322(91)90018-5
Gao, Q., and Chess, A. (1999). Identification of candidate Drosophila olfactory receptors from genomic DNA sequence. Genomics 60, 31–39. doi: 10.1006/geno.1999.5894
Ghaninia, M., Olsson, S. B., and Hansson, B. S. (2014). Physiological organization and topographic mapping of the antennal olfactory sensory neurons in female hawkmoths, Manduca sexta. Chem. Senses 39, 655–671. doi: 10.1093/chemse/bju037
Glaser, N., Gallot, A., Legeai, F., Montagné, N., Poivet, E., Harry, M., et al. (2013). Candidate chemosensory genes in the Stemborer Sesamia nonagrioides. Int. J. Biol. Sci. 9, 481–495. doi: 10.7150/ijbs.6109
Grant, G. G., and Eaton, J. L. (1973). Scent brushes of the male tobacco hornworm Manduca sexta (Lepidoptera: Sphingidae). Ann. Entomol. Soc. Am. 66, 901–904. doi: 10.1093/aesa/66.4.901
Grosse-Wilde, E., Kuebler, L. S., Bucks, S., Vogel, H., Wicher, D., and Hansson, B. S. (2011). Antennal transcriptome of Manduca sexta. Proc. Natl. Acad. Sci. U.S.A. 108, 7449–7454. doi: 10.1073/pnas.1017963108
Große-Wilde, E., Stieber, R., Forstner, M., Krieger, J., Wicher, D., Hansson, B. S., et al. (2010). Sex-specific odorant receptors of the tobacco hornworm Manduca sexta. Front. Cell. Neurosci. 4:22. doi: 10.3389/fncel.2010.00022
Halitschke, R., Schittko, U., Pohnert, G., Boland, W., and Baldwin, I. T. (2001). Molecular interactions between the specialist herbivore Manduca sexta (Lepidoptera, Sphingidae) and its natural host Nicotiana attenuata. III. Fatty acid-amino acid conjugates in herbivore oral secretions are necessary and sufficient for herbivore-specific plant responses. Plant Physiol. 125, 711–717. doi: 10.1104/pp.125.2.711
Hallem, E. A., and Carlson, J. R. (2006). Coding of odors by a receptor repertoire. Cell 125, 143–160. doi: 10.1016/j.cell.2006.01.050
Hallem, E. A., Ho, M. G., and Carlson, J. R. (2004). The molecular basis of odor coding in the Drosophila antenna. Cell 117, 965–979. doi: 10.1016/j.cell.2004.05.012
Hansson, B. S., and Stensmyr, M. C. (2011). Evolution of insect olfaction. Neuron 72, 698–711. doi: 10.1016/j.neuron.2011.11.003
Hansson, B. S., and Wicher, D. (2016). “Chemical ecology in insects,” in Chemosensory Transduction, eds F. Zufall and S. D. Munger (Academic Press), 29–45. doi: 10.1016/B978-0-12-801694-7.00002-0
Hillier, N. K., and Vickers, N. J. (2004). The role of heliothine hairpencil compounds in female Heliothis virescens (Lepidoptera: Noctuidae) behavior and mate acceptance. Chem. Senses 29, 499–511. doi: 10.1093/chemse/bjh052
Howlett, N., Dauber, K. L., Shukla, A., Morton, B., Glendinning, J. I., Brent, E., et al. (2012). Identification of chemosensory receptor genes in Manduca sexta and knockdown by RNA interference. BMC Genomics 13:211. doi: 10.1186/1471-2164-13-211
Hussain, A., Zhang, M., Üçpunar, H. K., Svensson, T., Quillery, E., Gompel, N., et al. (2016). Ionotropic chemosensory receptors mediate the taste and smell of polyamines. PLoS Biol. 14:e1002454. doi: 10.1371/journal.pbio.1002454
Jan, L. Y., and Jan, Y. N. (1982). Antibodies to horseradish peroxidase as specific neuronal markers in Drosophila and in grasshopper embryos. Proc. Natl. Acad. Sci. U.S.A. 79, 2700–2704. doi: 10.1073/pnas.79.8.2700
Kaissling, K.-E., Hildebrand, J. G., and Tumlinson, J. H. (1989). Pheromone receptor cells in the male moth Manduca sexta. Cell 279, 273–279.
Kaissling, K. E., Kasang, G., Bestmann, H. J., Stransky, W., and Vostrowsky, O. (1978). A new pheromone of the silkworm moth Bombyx mori. Naturwissenschaften 65, 382–384. doi: 10.1007/BF00439702
Kalinová, B., Hoskovec, M., Liblikas, I., Unelius, C. R., and Hansson, B. S. (2001). Detection of sex pheromone components in Manduca sexta (L.). Chem. Senses 26, 1175–1186. doi: 10.1093/chemse/26.9.1175
Kanost, M. R., Arrese, E. L., Cao, X., Chen, Y. R., Chellapilla, S., Goldsmith, M. R., et al. (2016). Multifaceted biological insights from a draft genome sequence of the tobacco hornworm moth, Manduca sexta. Insect Biochem. Mol. Biol. 76, 118–147. doi: 10.1016/j.ibmb.2016.07.005
Kent, K. S., and Griffin, L. M. (1990). Sensory organs of the thoracic legs of the moth Manduca sexta. Cell Tissue Res. 259, 209–223. doi: 10.1007/BF00318442
Kessler, A., and Baldwin, I. T. (2001). Defensive function of herbivore-induced plant volatile emissions in nature. Science 291, 2141–2144. doi: 10.1126/science.291.5511.2141
Klijnstra, J. W., and Roessingh, P. (1986). Perception of the oviposition deterring pheromone by tarsal and abdominal contact chemoreceptors in Pieris brassicae. Entomol. Exp. Appl. 40, 71–79. doi: 10.1111/j.1570-7458.1986.tb02157.x
Koenig, C., Hirsh, A., Bucks, S., Klinner, C., Vogel, H., Shukla, A., et al. (2015). A reference gene set for chemosensory receptor genes of Manduca sexta. Insect Biochem. Mol. Biol. 66, 51–63. doi: 10.1016/j.ibmb.2015.09.007
Koh, T. W., He, Z., Gorur-Shandilya, S., Menuz, K., Larter, N. K., Stewart, S., et al. (2014). The Drosophila IR20a clade of ionotropic receptors are candidate taste and pheromone receptors. Neuron 83, 850–865. doi: 10.1016/j.neuron.2014.07.012
Larsson, M. C., Domingos, A. I., Jones, W. D., Chiappe, M. E., Amrein, H., and Vosshall, L. B. (2004). Or83b encodes a broadly expressed odorant receptor essential for Drosophila olfaction. Neuron 43, 703–714. doi: 10.1016/j.neuron.2004.08.019
Loesel, R., Weigel, S., and Bräunig, P. (2006). A simple fluorescent double staining method for distinguishing neuronal from non-neuronal cells in the insect central nervous system. J. Neurosci. Methods 155, 202–206. doi: 10.1016/j.jneumeth.2006.01.006
Maher, N., and Thiery, D. (2004). Distribution of chemo- and mechanoreceptors on the tarsi and ovipositor of female European grapevine moth, Lobesia botrana. Entomol. Exp. Appl. 110, 135–143. doi: 10.1111/j.0013-8703.2004.00131.x
Maher, N., Thiery, D., and Städler, E. (2006). Oviposition by Lobesia botrana is stimulated by sugars detected by contact chemoreceptors. Physiol. Entomol. 31, 14–22. doi: 10.1111/j.1365-3032.2005.00476.x
Mechaber, W. L., Capaldo, C. T., and Hildebrand, J. G. (2002). Behavioral responses of adult female tobacco hornworms, Manduca sexta, to hostplant volatiles change with age and mating status. J. Insect Sci. 2, 1–8. doi: 10.1673/031.002.0501
Neuhaus, E. M., Gisselmann, G., Zhang, W., Dooley, R., Störtkuhl, K., and Hatt, H. (2005). Odorant receptor heterodimerization in the olfactory system of Drosophila melanogaster. Nat. Neurosci. 8, 15–17. doi: 10.1038/nn1371
Nishida, R., Schulz, S., Kim, C. S., Fukami, H., Kuwahara, Y., Honda, K., et al. (1996). Male sex pheromone of a giant danaine butterfly, Idea leuconoe. J. Chem. Ecol. 22, 949–972. doi: 10.1007/BF02029947
Pask, G. M., Romaine, I. M., and Zwiebel, L. J. (2013). The Molecular Receptive Range of a Lactone Receptor in Anopheles gambiae. Chem. Senses 38, 19–25. doi: 10.1093/chemse/bjs074
Patch, H. M., Velarde, R. A., Walden, K. K. O., and Robertson, H. M. (2009). A candidate pheromone receptor and two odorant receptors of the hawkmoth Manduca sexta. Chem. Senses 34, 305–316. doi: 10.1093/chemse/bjp002
Qiu, Y., van Loon, J. J. A., and Roessingh, P. (1998). Chemoreception of oviposition inhibiting terpenoids in the diamondback moth Plutella xylostella. Entomol. Exp. Appl. 87, 143–155. doi: 10.1046/j.1570-7458.1998.00316.x
Reisenman, C. E., and Riffell, J. A. (2015). The neural bases of host plant selection in a Neuroecology framework. Front. Physiol. 6:229. doi: 10.3389/fphys.2015.00229
Reisenman, C. E., Riffell, J. A., Bernays, E. A., and Hildebrand, J. G. (2010). Antagonistic effects of floral scent in an insect-plant interaction. Proc. R. Soc. B Biol. Sci. 277, 2371–2379. doi: 10.1098/rspb.2010.0163
Reisenman, C. E., Riffell, J. A., Duffy, K., Pesque, A., Mikles, D., and Goodwin, B. (2013). Species-specific effects of herbivory on the oviposition behavior of the moth Manduca sexta. J. Chem. Ecol. 39, 76–89. doi: 10.1007/s10886-012-0228-1
Reisenman, C. E., Riffell, J. A., and Hildebrand, J. G. (2009). Neuroethology of oviposition behavior in the moth Manduca sexta. Ann. N.Y. Acad. Sci. 1170, 462–467. doi: 10.1111/j.1749-6632.2009.03875.x
Riffell, J. A., Lei, H., and Hildebrand, J. G. (2009). Neural correlates of behavior in the moth Manduca sexta in response to complex odors. Proc. Natl. Acad. Sci. U.S.A. 106, 19219–19226. doi: 10.1073/pnas.0910592106
Robertson, H. M., Warr, C. G., and Carlson, J. R. (2003). Molecular evolution of the insect chemoreceptor gene superfamily in Drosophila melanogaster. Proc. Natl. Acad. Sci. U.S.A. 100(Suppl.), 14537–14542. doi: 10.1073/pnas.2335847100
Roelofs, W. L., Hill, A. S., Carde, R. T., and Baker, T. C. (1974). Two sex pheromone components of the tobacco budworm moth, Heliothis virescens. Life Sci. 14, 1555–1562. doi: 10.1016/0024-3205(74)90166-0
Roscoe, L. E., Silk, P., and Eveleigh, E. S. (2016). Evidence of male hair pencil pheromone in Choristoneura fumiferana (Lepidoptera: Tortricidae). J. Insect Sci. 16:27. doi: 10.1093/jisesa/iew010
Rytz, R., Croset, V., and Benton, R. (2013). Ionotropic receptors (IRs): chemosensory ionotropic glutamate receptors in Drosophila and beyond. Insect Biochem. Mol. Biol. 43, 888–897. doi: 10.1016/j.ibmb.2013.02.007
Sato, K., Pellegrino, M., Nakagawa, T., Nakagawa, T., Vosshall, L. B., and Touhara, K. (2008). Insect olfactory receptors are heteromeric ligand-gated ion channels. Nature 452, 1002–1006. doi: 10.1038/nature06850
Scala, A., Allmann, S., Mirabella, R., Haring, M. A., and Schuurink, R. C. (2013). Green leaf volatiles: a plant's multifunctional weapon against herbivores and pathogens. Int. J. Mol. Sci. 14, 17781–17811. doi: 10.3390/ijms140917781
Seada, M. A., Ignell, R., and Anderson, P. (2016). Morphology and distribution of ovipositor sensilla of female cotton leaf worm Spodoptera littoralis (Lepidoptera: Noctuidae), and evidence for gustatory function. Entomol. Sci. 19, 9–19. doi: 10.1111/ens.12160
Senthilan, P. R., Piepenbrock, D., Ovezmyradov, G., Nadrowski, B., Bechstedt, S., Pauls, S., et al. (2012). Drosophila auditory organ genes and genetic hearing defects. Cell 150, 1042–1054. doi: 10.1016/j.cell.2012.06.043
Shields, V. D. C., and Hildebrand, J. G. (2001). Responses of a population of antennal olfactory receptor cells in the female moth Manduca sexta to plant-associated volatile organic compounds. J. Comp. Physiol. A 186, 1135–1151. doi: 10.1007/s003590000165
Späthe, A., Reinecke, A., Haverkamp, A., Hansson, B. S., and Knaden, M. (2013a). Host plant odors represent immiscible information entities - blend composition and concentration matter in hawkmoths. PLoS ONE 8:e77135. doi: 10.1371/journal.pone.0077135
Späthe, A., Reinecke, A., Olsson, S. B., Kesavan, S., Knaden, M., and Hansson, B. S. (2013b). Plant species- and status-specific odorant blends guide oviposition choice in the moth Manduca sexta. Chem. Senses 38, 147–159. doi: 10.1093/chemse/bjs089
Stengl, M. (2010). Pheromone transduction in moths. Front. Cell. Neurosci. 4:133. doi: 10.3389/fncel.2010.00133
Stengl, M., and Funk, N. W. (2013). The role of the coreceptor Orco in insect olfactory transduction. J. Comp. Physiol. A Neuroethol. Sens. Neural Behav. Physiol. 199, 897–909. doi: 10.1007/s00359-013-0837-3
Stensmyr, M. C., Dweck, H. K. M., Farhan, A., Ibba, I., Strutz, A., Mukunda, L., et al. (2012). A conserved dedicated olfactory circuit for detecting harmful microbes in Drosophila. Cell 151, 1345–1357. doi: 10.1016/j.cell.2012.09.046
Tumlinson, J. H., Brennan, M. M., Doolittle, R. E., Mitchell, E. R., Brabham, A., Mazomenos, B. E., et al. (1989). Identification of a pheromone blend attractive to Manduca sexta (L.) males in a wind tunnel. Arch. Insect Biochem. Physiol. 10, 255–271. doi: 10.1002/arch.940100402
Vosshall, L. B., Amrein, H., Morozov, P. S., Rzhetsky, A., and Axel, R. (1999). A spatial map of olfactory receptor expression in the Drosophila antenna. Cell 96, 725–736. doi: 10.1016/S0092-8674(00)80582-6
Vosshall, L. B., and Hansson, B. S. (2011). A unified nomenclature system for the insect olfactory coreceptor. Chem. Senses 36, 497–498. doi: 10.1093/chemse/bjr022
Waladde, S. M. (1983). Chemoreception of adult stem-borers: tarsal and ovipositor sensilla on Chilo partellus and Eldana saccharina. Int. J. Trop. Insect Sci. 4, 159–165. doi: 10.1017/S1742758400004173
Wicher, D. (2015). “Olfactory signaling in insects,” in Progress in Molecular Biology and Translational Science, ed R. Glatz (Burlington: Academic Press), 37–54.
Wicher, D., Schäfer, R., Bauernfeind, R., Stensmyr, M. C., Heller, R., Heinemann, S. H., et al. (2008). Drosophila odorant receptors are both ligand-gated and cyclic-nucleotide-activated cation channels. Nature 452, 1007–1011. doi: 10.1038/nature06861
Widmayer, P., Heifetz, Y., and Breer, H. (2009). Expression of a pheromone receptor in ovipositor sensilla of the female moth (Heliothis virescens). Insect Mol. Biol. 18, 541–547. doi: 10.1111/j.1365-2583.2009.00894.x
Xia, Y. H., Zhang, Y. N., Hou, X. Q., Li, F., and Dong, S. L. (2015). Large number of putative chemoreception and pheromone biosynthesis genes revealed by analyzing transcriptome from ovipositor-pheromone glands of Chilo suppressalis. Sci. Rep. 5:7888. doi: 10.1038/srep07888
Yamaoka, K., Hoshino, M., and Hirao, T. (1971). Role of sensory hairs on the anal papillae in oviposition behaviour of Bombyx mori. J. Insect Physiol. 17, 897–911. doi: 10.1016/0022-1910(71)90106-5
Keywords: Manduca sexta, olfactory sensilla, ovipositor, olfaction, physiology
Citation: Klinner CF, König C, Missbach C, Werckenthin A, Daly KC, Bisch-Knaden S, Stengl M, Hansson BS and Große-Wilde E (2016) Functional Olfactory Sensory Neurons Housed in Olfactory Sensilla on the Ovipositor of the Hawkmoth Manduca sexta. Front. Ecol. Evol. 4:130. doi: 10.3389/fevo.2016.00130
Received: 30 August 2016; Accepted: 28 October 2016;
Published: 17 November 2016.
Edited by:
Emmanuelle Jacquin-Joly, French National Institute for Agricultural Research, FranceReviewed by:
Neil Kirk Hillier, Acadia University, CanadaCopyright © 2016 Klinner, König, Missbach, Werckenthin, Daly, Bisch-Knaden, Stengl, Hansson and Große-Wilde. This is an open-access article distributed under the terms of the Creative Commons Attribution License (CC BY). The use, distribution or reproduction in other forums is permitted, provided the original author(s) or licensor are credited and that the original publication in this journal is cited, in accordance with accepted academic practice. No use, distribution or reproduction is permitted which does not comply with these terms.
*Correspondence: Ewald Große-Wilde, Z3Jvc3NlLXdpbGRlQGljZS5tcGcuZGU=
†These authors have contributed equally to this work.
Disclaimer: All claims expressed in this article are solely those of the authors and do not necessarily represent those of their affiliated organizations, or those of the publisher, the editors and the reviewers. Any product that may be evaluated in this article or claim that may be made by its manufacturer is not guaranteed or endorsed by the publisher.
Research integrity at Frontiers
Learn more about the work of our research integrity team to safeguard the quality of each article we publish.