- 1Université de Rennes 1, Ethologie Animale et Humaine (UMR 6552)—Centre National de la Recherche Scientifique, Paimpont, France
- 2Centre d'Ecologie Fonctionnelle et Evolutive (UMR 5175), Centre National de la Recherche Scientifique—Université de Montpellier—Université Paul-Valéry Montpellier—EPHE, Montpellier, France
- 3Developmental Ethology and Cognitive Psychology Group, Centre des Sciences du Goût (UMR 6265), Centre National de la Recherche Scientifique-Université de Bourgogne-Franche-Comté, Dijon, France
- 4Department of Arts and Sciences, Université de Nîmes, Nîmes, France
- 5Centre National de la Recherche Scientifique, Ethologie Animale et Humaine (UMR 6552)—Université de Rennes 1, Rennes, France
- 6Département Scientifique et Pédagogique, Planète Sauvage, Port-Saint-Père, France
Chemosensory perception in cetaceans remains an intriguing issue as morphological, neuroanatomical and genetic studies draw unclear conclusions, while behavioral data suggest that dolphins may use it for food selection or socio-sexual interactions. Experimental approaches have been scarce due to the practical difficulties of testing chemoreception in wild dolphins. Go/no-go tasks are one elegant way to investigate discrimination abilities; however, they require to train the animals, thus preventing spontaneous responses and hence the expression of preferences. Here, we aimed at testing potential spontaneous responses to chemical stimuli and developed novel procedures. First, we conducted a study to test whether captive dolphins respond to a biologically relevant smell. Therefore, we placed dead fish within an opaque barrel at the border of the pool and counted the number of respirations at proximity as an indicator of investigation. The same dead fishes were presented several times during experiments lasting three consecutive days. From the second day on (i.e., when the odor composition changed), dolphins breathed more often close to the fish-smelling barrel than close to the visually identical but empty control barrel. Second, we conducted a study to test whether dolphins are able to discriminate food flavors. Captive dolphins are commonly provided with ice cubes as a source of enrichment. We took this opportunity to provide ice cubes with different flavors and to compare the reaction to these different flavors as a measure of discrimination. Hence, we used the latency of return to the ice cube begging spot as a measure of discrimination from the previous ice cube flavor. Thus, our method used a non-invasive and easily replicable technique based on the spontaneous begging responses of dolphins toward more or less attractive items bearing biological relevance. The procedures used enabled us to show that dolphins may discriminate odors and flavors respectively.
Introduction
Although chemoreception plays an important role not only for terrestrial species, but also for animals with an entire or semi-aquatic lifestyle (Nevitt et al., 1995; Catania, 2006; Davis et al., 2006; Hara, 2006; Endres and Lohmann, 2012), it has drawn little attention in research on cetaceans. In dolphins, few studies have been performed compared to other sensory modalities (see Kremers et al., 2016), and their results are contradictory.
On the one hand, several authors posit that some cetacean species have lost their nasal (Kishida et al., 2007) and oral chemoreception (Jiang et al., 2013) in the course of evolution, as airborne odorants may be considered irrelevant due to their aquatic lifestyle (Thewissen et al., 2011). Firstly, corresponding anatomical structures are rudimentary or absent, at least in adult animals. In the nasal cavity of toothed whales, the cribriform plate of the ethmoid bone and ethmoturbinals are absent (Pihlström, 2008). In their oral cavity, no taste buds were found on the tongue or other body areas of various odontocete species (Kuznetzov, 1990). However, the number and/or age of individuals investigated is usually unknown or very limited. Secondly, central structures devoted to olfaction are rudimentary or absent. The olfactory nerve [cranial nerve (CN) I] seems to vanish during early ontogenesis (Oelschläger and Buhl, 1985). The main and accessory olfactory tracts are completely absent in toothed whales, and absent or considerably reduced in baleen whales (Oelschläger, 2008; Pihlström, 2008). Thirdly, olfactory receptor (OR) and taste receptor genes are mostly pseudogenised or entirely absent in Odontoceti (Kishida et al., 2007; Jiang et al., 2013).
By contrast, numerous studies argue in favor of functional chemoreception in cetaceans. Firstly, chemoreceptive cells were found in the frontal and vestibular sac (close to the blowhole) of harbor porpoises (Behrmann, 1989), perhaps enabling some kind of chemical sensation in this species. Moreover, taste buds were found in younger individuals of the same species that were previously described as not having them when investigating adult individuals (Yamasaki et al., 1978; Behrmann, 1988; Kuznetzov, 1990). Other studies did not describe taste buds, but found marginal and vallate papillae on the tongues of dolphins, known to be potential locations of taste buds (Kastelein and Dubbeldam, 1990; Werth, 2007). Secondly, Odontoceti were found to possess a well-developed olfactory tubercle (Oelschläger and Oelschläger, 2009) and bottlenose dolphins possess prominent olfactory lobes possibly activated by the trigeminal nerve (CN V; Jacobs et al., 1971). This nerve is very well developed in dolphins (Oelschläger, 2008) and necessary for odor location in humans (Kleemann et al., 2009). It was proposed that in dolphins CN V might provide a pathway to transmit impulses from the oral cavity to the brain (Oelschläger and Oelschläger, 2009), called trigeminal chemoreception (Kuznetzov, 1990). Unlike other mammals, where cranial nerve VII innervates the taste buds of the tongue (Purves et al., 2001), this nerve does not seem to be involved in dolphin chemoreception but rather in acoustic signal production (Oelschläger, 2008). However, cranial nerve V is, just as cranial nerve VII, able to excite the gustatory neurons in the nucleus of the solitary tract in the medulla (Purves et al., 2001; Boucher et al., 2003). Thirdly, although OR genes are reported to be functionally reduced by pseudogenization in Odontoceti (Kishida et al., 2007), bottlenose dolphins in particular possess 23 G protein-coupled OR genes that are not pseudogenized, thus potentially functional (SEVENS database of G-protein coupled receptor genes; available at: http://sevens.cbrc.jp/search.php?db=ttru&level=4). Similarly, taste receptor genes were found to be mostly pseudogenized in Odontoceti: in bottlenose dolphins sweet, umami, bitter and sour taste receptor genes were found to be inactivated, whereas salty taste receptor genes are intact and potentially functional (Jiang et al., 2013; Feng et al., 2014; Kishida et al., 2015). Finally, go/no-go behavioral tests with trained bottlenose dolphins showed that they can perceive sour, bitter and salty tastes nearly as well as humans (Nachtigall and Hall, 1984; Friedl et al., 1990; Kuznetzov, 1990). The authors of these behavioral studies noticed that dolphins were able to perceive orally what other mammals perceive by smell wherefore they called this perception “quasi-olfaction” (Kuznetzov, 1990) or “water-borne sense of smell” (Nachtigall, 1986). Taken together, this second set of studies suggests that cetaceans might have, to some extent, access to chemosensory information through the olfactory (Thewissen et al., 2011) and/or taste systems (Watkins and Wartzok, 1985; Pihlström, 2008). As anatomical, neuroanatomical, and molecular evidence draw unclear conclusions, behavioral studies are needed.
Obviously cetaceans are difficult to study, especially in their natural habitat where they are difficult to find and to follow, and controlled experiments are often hardly feasible. Therefore, we present two promising experimental approaches for initial behavioral studies on olfaction and gustation in captive dolphins. Given the complex but sometimes subtle behaviors displayed by dolphins in response to internal or external stimuli, go/no-go tasks are one elegant way to investigate chemoperceptual abilities; however, they require to train the animals, thus preventing spontaneous responses. Therefore, the go/no-go paradigm is not optimal to investigate preferences because it imposes time-consuming training of animals and minimizes the measurement of spontaneously-expressed preferences. As we aimed at testing potential spontaneous responses to chemical stimuli, we developed and tried novel methods. First, we conducted a pioneer experiment to test for odor perception. We assumed that biologically relevant odors should be intriguing for the dolphins, especially when food-related. Therefore, we predicted that, if dolphins were capable of perceiving odors, they would express some “sniffing”-like behavior (i.e., taking more breaths) within the range of the odor source. Second, we investigated the flavor discrimination abilities of dolphins, predicting that, if dolphins were capable of perceiving flavors, they would behave discriminatively in response to control vs. flavored ice-cubes, and that they would discriminate different flavors along either sensory features involving their source (i.e., fish vs. non-fish) or along previous exposure to the stimuli (familiar vs. unfamiliar).
Study I: Olfaction
Materials and Methods
Study Subjects and Housing Conditions
In May and June 2013, we studied six captive-born bottlenose dolphins (Tursiops truncatus, Montagu, 1821) in the delphinarium of Planète Sauvage (Port-Saint-Père, France): four males (aged 5, 8, 14, and 29 years) and two females (aged 5 and 12 years). The three oldest dolphins had been housed together for more than four years when the study took place and participated also in the study on gustation; the three youngest dolphins arrived in the facility one year before the study took place.
Overall, this outdoor facility consists of four pools, covering 2000 m2 water surface and containing 7.5 million liters salt water cleaned with ozone (without any chlorine). The diet of the dolphins was composed of frozen-stored fresh herring, capelin, sprat, mackerel, blue whiting and squid. The species composition of the diet changed on a daily basis, but contained at least three different fish species each day. A daily ration of 5–10 kg per individual (depending on its weight) was given throughout the day during eight feeding sessions (approx. 15 min lasting), the first at 9:00 a.m. and the last at 5:00 p.m. These feedings were conducted by the dolphin trainers, using the food as primary reinforcement for medical training (e.g., acceptance of inspection and palpation of all parts of the body or being touched by medical equipment) as well as training for public shows (e.g., jump on command). The trainers gave the food directly into the mouth of the dolphin. During the experiment, dolphins were together as a group and free to move.
Stimuli
One kilogram of mixed fish (herring, capelin, sprat, mackerel, and whiting) and squids (hereafter referred to as “fish”) that were defrosted during the night preceding the first day of each one of the three experiments were used as odor source and were actually those destined to feed the dolphins. Mixing species was done to avoid responses biased by potential individual preferences. The fish was placed in a familiar opaque plastic barrel (height: 26 cm; diameter: 17 cm), perforated all around with 40 small holes (diameter: 3 mm), that was familiar to the dolphins (Figure 1). The inside surface of the barrel was covered with a black plastic bag to avoid visual cues and any leak into the pool. The barrel was placed uncovered at the edge of the pool, simultaneously with a second, identical but empty control barrel. The two barrels were 8 m away from each other (linear distance) and the position for fish/control barrel was randomly changed between sessions that lasted 10 min. The dolphins never had physical contact with the barrels and their top opening was too high for them to look inside even when raising their heads out of the water. Thus, vision and touch were excluded as conveyors of cues to differentiate both barrels.
Data Collection
The same fish were presented to the group of dolphins on three consecutive days (thus producing an increasingly intense odor of rotten fish) with two sessions per day with the largest possible time interval between experimental and feeding session. Three days made up for an experimental section. Three sections were done in total with a new mixture of fish each time, leading to 18 sessions in total over 9 days (Table 1). During a section, the fish was stored at room temperature to facilitate the change in odor composition. This change was also detectable for humans who could discriminate between the fish and the control barrel based on their smell only. During the experimental sessions, that lasted 10 min, each barrel was video-recorded with a Sony HDR-XR155 video camera and neither the experimenter nor another person was close to the pool. An observer blind to the content of the barrels then analyzed the videos.
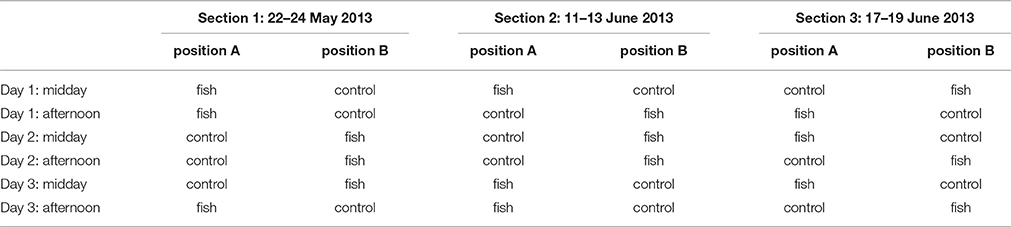
Table 1. Chronological sequence of stimuli presentation (position for fish/control changed randomly between sessions).
As no previous studies on olfaction in dolphins were available, we had no information on how a possible reaction of a dolphin toward an odorous stimulus might look like. As the perception of odorants is affected by breathing patterns in other mammals (e.g., Saslow, 2002), we chose to count the number of respirations for each dolphin within a range of 2.5 m around either barrel. Respiration was defined as a visible and audible opening of the blowhole above the water surface. Individuals could be reliably identified based on physical differences (e.g., shape of the dorsal fin).
Data analysis
Statistical analyses were run using R software (version 2.15.0, R Development Core Team, www.r-project.org). We calculated a Respiration Bias Index (RBI) using the following formula: , resulting in RBI values ranging from +1 to −1. Thus, positive RBI values indicate a bias in respiration activity toward the fish barrel (i.e., dolphins breathe more often in the area around the barrel containing fish compared to the control barrel). Accordingly, negative RBI values indicate a bias in respiration activity toward the control barrel (i.e., dolphins breathe more often in the area around the control barrel compared to the barrel containing fish). This kind of index is common for example in primate laterality studies (Hopkins, 1999). As odor composition changed on a daily basis due to fish decomposition, we tested whether there was a direct relationship between RBI and day with a Wald test on a Linear Mixed Model (ANOVA for repeated measurements; n = 8, α = 0.05; R-package: lme4). Sections were considered as replicates and therefore treated as random factor. Identity of the dolphins was taken into account by treating individual as random factor in the model.
To further investigate this relationship, we compared the number of respirations between fish and control for each day separately with two-tailed Wilcoxon signed rank tests (n = 6, α = 0.05). To ensure that dolphins did not differ in respiration activity between the 3 days of the experimental sections, we additionally compared the total number of respirations (i.e., no matter which odor) between all days with two-tailed Wilcoxon signed rank tests (n = 12, α = 0.05). For the tests, we summed up each individual's values obtained during the sessions of the first, the second, and the third days, respectively, of the three experimental sections. Respiration values in the text and figure are given as mean ± standard error and refer to the session's duration of 10 min.
Results
There was a linear relationship between the Respiration Bias Index (RBI) and the day of the experiment (mixed LM: χ2 = 3.877, P = 0.0489). The Figure 2 shows, that the bias of dolphin respiration activity toward the fish barrel increased over the course of the experiment.
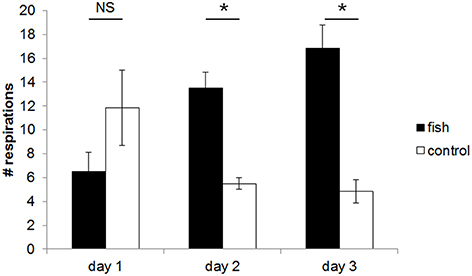
Figure 2. Mean (±SE) number of respirations per 10 min of the dolphins in the area within a radius of 2.5 m around the barrel containing fish or the empty control barrel. The dolphins breathed more often close to the barrel containing fish compared to the control barrel both at day 2 and 3. Statistical difference is indicated by asterisks (*P ≤ 0.05; NS, not significant; two-tailed Wilcoxon signed rank tests using the sums of each individual's values obtained during the sessions of the 3 days of the three experimental sections).
On the first day, the number of respirations toward the barrel containing fish vs. the control barrel did not differ significantly (fish: 6.5 ± 1.6; control: 11.8 ± 3.1; P = 0.063, V = 20). However, the dolphins breathed significantly more often in the area around the fish-smelling than in the control situation both at the second day (fish: 13.5 ± 1.3; control: 5.5 ± 0.5; P = 0.036, V = 0) and at the third day (fish: 16.8 ± 1.9; control: 4.8 ± 1.0; P = 0.031, V = 0; Figure 2). Overall, the total number of respirations (regardless the odor source close by) did not differ between the 3 days (day 1 vs. 2: P = 0.824, V = 30; day 2 vs. 3: P = 0.348, V = 22; day 1 vs. day 3: P = 0.783, V = 35).
Conclusion
The response of six captive bottlenose dolphins to visually identical but differently smelling devices suggests that this species is capable of perceiving chemosensory stimuli in air (odors). This is, to our knowledge, the first study on spontaneous behavioral responses of dolphins toward a potentially biologically relevant odor. That the dolphins did not discriminate between the fish-smelling and the control device at the first day might be caused by the odor composition. Thus, dolphins may respond to certain molecules whose concentrations increase progressively in the course of decomposition, such as putrescine (Sil et al., 2008). Alternatively, dolphins may be sensitive to some molecules dominant at the second/third day of the experiment due to another interest than foraging. Maybe the dolphins simply responded because of their curiosity to the unfamiliar stimulus, as curiosity seems to be a common personality trait in dolphins (Highfill and Kuczaj, 2007; Kuczaj et al., 2012). For example, the natural curiosity of dolphins and their attraction to novelty may lead them to explore preferentially unknown odors.
Study II: Gustation
Materials and Methods
Study Subjects
In January and February 2012, we studied four captive-born bottlenose dolphins in the delphinarium of Planète Sauvage: three males (aged 8, 12, and 27 years) and one female (aged 10 years). The dolphins had been housed together for more than three years when the study took place. For information on housing conditions see above.
Stimuli
In the current study, we aimed at testing potential spontaneous preferences for food flavors in a “naturalistic” setting, meaning in conditions where dolphins may express their preferences without going through conditioning procedures. Therefore, we developed and tried an approach enabling to measure spontaneous responses of dolphins to different flavors. It is usual to provide captive dolphins with ice cubes as a source of enrichment (Warne-Reese, 1997). We took this opportunity to provide ice cubes with different flavors and to compare the reaction of the dolphins to these different flavors as a measure of discrimination. Hence, we used the latency of return to the begging spot for ice cubes as a measure of discrimination in the previous ice cube flavor. Informal observations of dolphins' behavior after receiving an ice cube indicated that they hold it in mouth and assess it orally. Thus, our method used a non-invasive and easily replicable technique based on the spontaneous begging responses of dolphins toward more or less attractive items bearing biological relevance.
Ice cubes were equally familiar to all tested dolphins as they were commonly used as part of environmental enrichment in the dolphinarium (1–2 times per week); therefore, all dolphins were accustomed to receive, sense and ingest odor- and tasteless pure water ice cubes. For the present experiment, we produced ice cubes with herring, salmon and shrimp flavors (Table 2), originally used for human cooking (salmon/shrimp) or for baiting fish (herring). Herring was familiar to all dolphins through food exposure, whereas salmon and shrimp were not. Semispherical ice cubes (basis diameter: 4 cm; height: 2.5 cm) of 20 mL each were produced with every flavor diluted in plain mineral water (to ensure constant basic composition; Danone “Volvic,” Paris, France). Flavorless yellow or purple food colorants (Brauns-Heitmann Ltd. “Crazy Colors,” Warburg, Germany) were added to homogenize the visual appearance of the ice cubes for the dolphins and to increase their visibility in the pool for the experimenter. To prevent any flavor-color association by the dolphins, the colors were randomly distributed over ice cubes carrying different flavors. Thus, the ice cubes differed only in terms of flavor, but were visually- and tactually-similar. Ice cube were frozen at −21.5°C.
Data Collection
Experimental sessions were performed 1–5 times per day between the feeding sessions (with the largest possible time interval between experimental and feeding session) and lasted on average 8 ± 2 min. Two experimental sessions were separated by at least 60 min. During one experimental session one single flavor was tested. The four stimuli were tested consecutively, meaning we completed all sessions for a given flavor before testing a new flavor: first herring, then salmon, followed by shrimp and last control (the order of the four stimuli was chosen randomly).
All dolphins were together in the pool and when they saw the experimenter coming, they immediately and spontaneously approached her standing at the side of the pool. The dolphins were free to participate, meaning that they received ice cubes only when begging (i.e., when clearly opening their rostrum with the head and eyes over the water surface while being oriented to, and less than 1 m away from, the experimenter; Figure 3). This behavior was displayed only in this context and was obviously identifiable. The experimental session started when the experimenter took up her position at the pool (no other person was around the pool) where she was standing with the ice cubes next to her. The experimenter, who was familiar with all dolphins and could reliably identify each individual based on physical differences, never interacted with the dolphins beside of responding to their begging by giving an ice cube. After the display of the begging behavior, the experimenter let the ice cube fall in the open mouth of the dolphin. Begging latency was timed (with a stopwatch) from the moment a given dolphin received an ice cube (contact with the tongue) to the moment it begged for a new one. Begging latency was the only measurable parameter, as other behaviors that occurred between the receipt of an ice cube and the begging of a new one (e.g., playing with the ice cube) were not clearly visible from surface as the dolphins swam around in the pool.
Data Analysis
Statistic calculations were run using R software (version 2.15.0, R Development Core Team, www.r-project.org). As we predicted that all dolphins would react differently to fish (salmon/herring) vs. non-fish (shrimp/control) items or to familiar (herring/control) vs. unfamiliar (salmon/shrimp) food, we compared begging latencies between different flavors by using a Wald test on a Linear Mixed Model, considering the individual as random factor (R-package: lme4). Data have been log-transformed prior to analyses in order to homogenize the variances. Pairwise comparisons were performed with the contrasts method (correction for multiple testing: false discovery rate; R-package: doBy). As dolphins were unrestrained in this experiment, number of ice cubes received differed between individuals and between different tastes. However, this was taken into account by treating individual as random factor in the statistical analysis. Additionally, in order to control that the varied number of received ice cubes per dolphin did not bias the results, the same statistical tests were done with a subset of the data, using only the first eight latencies measured per individual and per taste (as n = 8 was the smallest total number of ice cubes delivered; see Table 3).
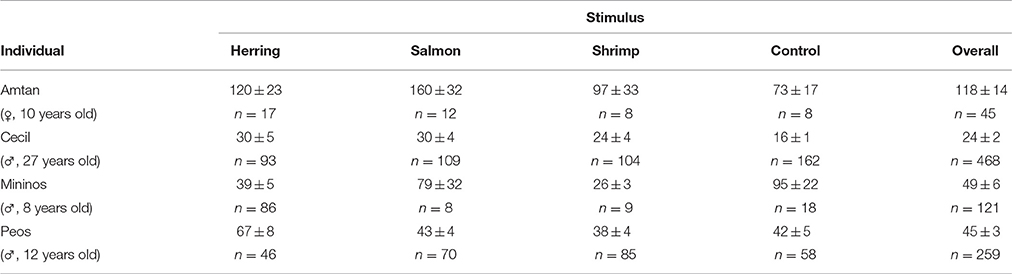
Table 3. Average latency to beg for another ice cube (in seconds) and number of given ice cubes for the differently flavored stimuli and for each individual dolphin (mean ± SE; n).
Results
The average latency of the four dolphins to beg for another ice cube differed significantly between the distinctly flavored ice cubes (mixed LM: χ2 = 19.16, P = 0.0003). Post-hoc tests indicated that all dolphins took more time to come back after receiving herring- or salmon-flavored ice cubes than after receiving shrimp-flavored or control ice cubes (all dyadic comparisons: 5.04 ≤ χ2 = 13.84, 0.001 ≤ P ≤ 0.037; Figure 4). Both fish-flavored ice cubes triggered similar latencies in the dolphins (χ2 = 0.54, P = 0.553); the same was true for the non-fish tasting ice cubes and the control ice cubes (χ2 = 0.33, P = 0.564). The two familiar flavors (herring and control) elicited different latencies (χ2 = 8.64, P = 0.007); the same was true for the two non-familiar flavors (salmon and shrimp: χ2 = 9.19, P = 0.007).
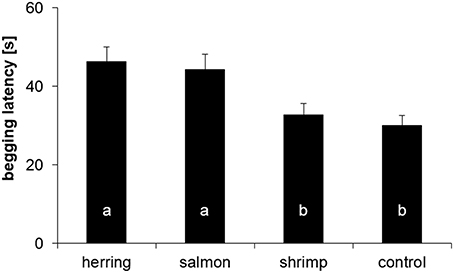
Figure 4. Mean (±SE) latency [s] of the dolphins to come back and beg for a new ice cube after receiving a herring, salmon, shrimp, or control ice cube. Same letters indicate absence of statistical difference (α = 0.05).
Even when using a homogeneous subset of the data (i.e., the first 8 latencies), there was still an effect of taste on the begging latency (mixed LM: χ2 = 10.72, P = 0.0134). Post-hoc tests indicated, similarly to the analysis with all data, that dolphins came back after significantly shorter latencies after receiving shrimp-flavored ice cubes compared to the other tastes (6.34 ≤ χ2 ≤ 8.34, 0.023 ≤ P ≤ 0.024), whereas herring, salmon, and control ice cubes triggered similar latencies (0.00 ≤ χ2 ≤ 0.14, 0.883 ≤ P ≤ 0.973).
Conclusion
Using an original method to test spontaneous preferences of dolphins for food flavors, it was possible to show that four captive bottlenose dolphins discriminated visually similar stimuli that differed only by their flavor. Dolphins took more time to beg for a new ice cube after receiving herring-/salmon-flavored ice cubes compared to shrimp-flavored/control ice cubes, indicating that they discriminated between fish and non-fish flavors. Whether stimuli were familiar (herring/control) or unfamiliar (salmon/shrimp) did not seem to influence their response. The prolonged latency after receiving fish-flavored ice cubes can be interpreted differently. One hypothesis is that the dolphins are not interested in fish-flavored ice cubes, wherefore they do not come back fast to beg for a new one. Alternatively, the dolphins might be very well interested in those fish-flavored ice cubes, wherefore they spend a longer time orally assessing these ice cubes. Thus, a prolonged begging latency might reflect a longer time spent “exploring” the flavor by the dolphin. We assume that this second hypothesis is probable because the dolphins could indeed be sporadically observed (when they were close enough to the experimenter) playing with the ice cube in their mouth, however, more experiments are needed to conclude further.
Discussion
The here presented approaches allow the conclusion that dolphins may be able to discriminate odors and flavors respectively. Other behavioral studies on dolphin chemoreception are rare, but show for example that bottlenose dolphins can detect the four basic flavors nearly as well as humans (Friedl et al., 1990). These chemoreceptive abilities might be useful in the context of predation. Odor perception might be used for prey location, as fish, the main prey of dolphins (Spitz et al., 2006), do indeed emit odors (Hirvonen et al., 2000). Although most studies on aquatic species focus on the fact that these odors are used by prey species to detect their respective predator fish, it seems possible that olfactory cues may play an important role as well in the reverse case (i.e., the detection of prey by its predator). There are also reports that some dolphins occasionally feed on already dead prey, precisely they take fish baits, sometimes minutes after baiting (Sumpton et al., 2010). One can therefore wonder whether olfactory cues may contribute to fast localization. Similarly, it has been suggested that some Mysticeti may detect prey by using odors in air although they are produced underwater (Thewissen et al., 2011).
Moreover, chemoreception might play a role in prey evaluation. Free-ranging dolphins do indeed display a feeding selectivity as they preferentially select high-energy density fish species even though they are less abundant than low-energy density fish species (Spitz et al., 2010). One possibility is that dolphins make food choices based on visual or texture differences. Another possibility relates to choices based on taste, odor, or flavor differences. In line with this, salmon and herring, the flavors eliciting longer latencies in our study, are about 1.5–2.5 times more energetic than shrimps (National Nutrient Database for Standard Reference, US National Agricultural Library; available at: http://ndb.nal.usda.gov/). Learned flavor preference may also underlie the choice of dolphins for some foods over others. In the wild, the diet of bottlenose dolphins is primarily composed of fish (94.2% of stomach contents in stranded dolphins), whereas crustaceans are only occasional prey (2.0%; Spitz et al., 2006). This might be caused, aside from differences in pelagic vs. benthic preys, by a spontaneous or learned preference for the flavor of fish.
Finally, chemoreceptive abilities may be useful during socio-sexual interactions. Individual recognition or mate detection (e.g., female receptiveness) could be chemically mediated as in many other species. It has been suggested that dolphins may utilize chemosensory cues to gain information about another dolphin's physiological state, for example in reproduction contexts (Norris, 1991; Muraco and Kuczaj, 2015) and they seem to be able to detect the urine and feces of conspecifics (Kuznetzov, 1990). However, further studies are needed to investigate whether and to which extend dolphins actually use chemical cues in different contexts. Proposition on this issue can be found in Kremers et al. (2016).
How dolphins perceive chemical cues remains unclear. Waterborne odors can be carried in water and air what makes them perceptible via different perception pathways. Therefore, further investigations are required to shed light on this topic.
In conclusion, our behavioral studies provide results on perception of odors and flavors in dolphins, thus opening new lines of research on cetacean chemoreception. Although the here presented methods are non-invasive, do not require previous training (although these animals were familiar with the barrel and used to receive water ice-cubes) and are easily replicable, we must acknowledge some limitations. First, social facilitation was not controlled in our setting, as dolphins were not tested individually. Second, we used artificial flavors in the gustation experiment and the experimenter was not blind to the stimuli (although we made sure to control the behavior of the experimenter best possible in order to prevent any clue to the dolphins). Moreover, the measured parameter (begging latency) and its interpretation (social influence of collectively assessed animals, habituation) also raise issues wherefore the use of the ice cubes method remains to be validated (e.g., by blindly testing of shortly isolated individuals). Future studies, which should consider these factors, are needed to conclude further.
Ethical Statement
The experiments described in this paper were carried out in accordance with the current laws of the country in which they were performed. They complied with the current French laws (Centre National de la Recherche Scientifique) related to animal experimentation and were in accordance with the European directive 86/609/CEE. The research was approved by the “Direction Départementale des Services Vétérinaires” committee of Loire-Atlantique prefecture. No further permit was needed as only behavioral observations were performed. Animal husbandry and veterinary care were under management of Planète Sauvage, as this study involved animals from a private animal park (no laboratory animals) with whom informed consent has been granted.
Author Contributions
All co-authors designed the work. AC, AL, MH, and SC equally coordinated the study on olfaction, including contribution to analysis and interpretation of data. AL, BS, MH, and MT equally coordinated the study on gustation, including contribution to analysis and interpretation of data. DK performed the experiments, supported by MB, on both olfaction and gustation, and analyzed and interpreted the data. DK, AL, and MH prepared the main manuscript text. All co-authors contributed to the manuscript preparation in the form of discussion and critical comments, and reviewed the manuscript.
Funding
This work was supported by the Centre National de la Recherche Scientifique (Groupement de Recherche 2822), by the Agence Nationale de la Recherche (grant ORILANG to AL) and the Institut Universitaire de France. Further, it was funded by the Association Nationale de la Recherche et de la Technologie (CIFRE grant #2010/0471 to DK). No funding source played a role study design, data collection and analysis, decision to publish, or preparation of the manuscript.
Conflict of Interest Statement
The authors declare that the research was conducted in the absence of any commercial or financial relationships that could be construed as a potential conflict of interest.
Acknowledgments
We thank the management of Planète Sauvage and the trainer staff of the Cité Marine for their cooperation, as well as Julie Galia, Juliana López for their contribution and Françoise Joubaud for logistic support. Thank you to Maxime Hervé for assistance with statistical analysis.
References
Behrmann, G. (1988). The peripheral nerve ends in the tongue of the harbour porpoise Phocoena phocoena (Linne, 1758). Aquat. Mammals 14, 107–112.
Behrmann, G. (1989). The olfactory regions in the nose of the harbour porpoise Phocoena phocoena (Linne, 1758). Aquat. Mammals 15, 130–133.
Boucher, Y., Simons, C. T., Faurion, A., Azérad, J., and Carstens, E. (2003). Trigeminal modulation of gustatory neurons in the nucleus of the solitary tract. Brain Res. 973, 265–274. doi: 10.1016/S0006-8993(03)02526-5
Catania, K. C. (2006). Underwater ‘sniffing’ by semi-aquatic mammals. Nature 444, 1024–1025. doi: 10.1038/4441024a
Davis, M. W., Spencer, M. L., and Ottmar, M. L. (2006). Behavioral responses to food odor in juvenile marine fish: acuity varies with species and fish length. J. Exp. Marine Biol. Ecol. 328, 1–9. doi: 10.1016/j.jembe.2005.04.029
Endres, C. S., and Lohmann, K. J. (2012). Perception of dimethyl sulfide (DMS) by loggerhead sea turtles: a possible mechanism for locating high-productivity oceanic regions for foraging. J. Exp. Biol. 215, 3535–3538. doi: 10.1242/jeb.073221
Feng, P., Zheng, J., Rossiter, S. J., Wang, D., and Zhao, H. (2014). Massive losses of taste receptor genes in toothed and baleen whales. Genome Biol. Evol. 6, 1254–1265. doi: 10.1093/gbe/evu095
Friedl, W. A., Nachtigall, P. E., Moore, P. W. B., Chun, N. K. W., Haun, J. E., Hall, R. W., et al. (1990). “Taste reception in the Pacific bottlenose dolphin (Tursiops truncatus gilli) and the California sea lion (Zalophus californianus),” in Sensory Abilities of Cetaceans, eds J. A. Thomas and R. A. Kastelein (New York, NY: Plenum Press), 447–454.
Hara, T. J. (2006). Feeding behaviour in some teleosts is triggered by single amino acids primarily through olfaction. J. Fish Biol. 68, 810–825. doi: 10.1111/j.0022-1112.2006.00967.x
Highfill, L. E., and Kuczaj, S. A. (2007). Do bottlenose dolphins (Tursiops truncatus) have distinct and stable personalities?. Aquat. Mammals 33, 380–389. doi: 10.1578/AM.33.3.2007.380
Hirvonen, H., Ranta, E., Piironen, J., Laurila, A., and Peuhkuri, N. (2000). Behavioral response of naïve Artic charr young to chemical cues from salmonid and non-salmonid fish. Oikos 88, 191–199. doi: 10.1034/j.1600-0706.2000.880121.x
Hopkins, W. D. (1999). On the other hand: statistical issues in the assessment and interpretation of hand preference data in nonhuman primates. Int. J. Primatol. 20, 851–866.
Jacobs, M. S., Morgane, P. J., and McFarland, W. L. (1971). The anatomy of the brain of the bottlenosed dolphin. Rhinic lobe (rhinencephalon). I. The paleocortex. J. Comp. Neurol. 141, 205–272.
Jiang, P., Josue, J., Li, X., Glaser, D., Li, W., Brand, J. G., et al. (2013). Major taste loss in carnivorous mammals. Proc. Natl. Acad. Sci. U.S.A. 109, 4956–4961. doi: 10.5167/uzh-62705
Kastelein, R., and Dubbeldam, J. L. (1990). Marginal papillae on the tongue of the harbour porpoise (Phocoena phocoena), bottlenose dolphin (Tursiops truncatus) and Commerson's dolphin (Cephalorhynchus commersonii). Aquat. Mammals 15, 158–170.
Kishida, T., Kubota, S., Shirayama, Y., and Fukami, H. (2007). The olfactory receptor gene repertoire in secondary-adapted marine vertebrates: evidence for reduction of the functional proportion in cetaceans. Biol. Lett. 3, 428–430. doi: 10.1098/rsbl.2007.0191
Kishida, T., Thewissen, J. G. M., Hayakawa, T., Imai, H., and Agata, K. (2015). Aquatic adaptation and the evolution of smell and taste in whales. Zool. Lett. 1, 9. doi: 10.1186/s40851-014-0002-z
Kleemann, A. M., Albrecht, J., Schöpf, V., Haegler, K., Kopietz, R., Hempel, J. M., et al. (2009). Trigeminal perception is necessary to localize odors. Physiol. Behav. 94, 401–405. doi: 10.1016/j.physbeh.2009.03.013
Kremers, D., Célérier, A., Schaal, B., Campagna, S., Trabalon, M., Böye, M., et al. (2016). Sensory perception in cetaceans: part I – current knowledge about dolphin senses as a representative species. Front. Ecol. Evol. 4:49. doi: 10.3389/fevo.2016.00049
Kuczaj, S. A., Highfill, L. E., and Byerly, H. (2012). The importance of considering context in the assessment of personality characteristics: evidence from ratings of dolphin personality. Int. J. Comp. Psychol. 25, 309–329.
Kuznetzov, V. B. (1990). “Chemical sense of dolphins: quasi-olfaction,” in Sensory Abilities of Cetaceans, eds J. A. Thomas and R. A. Kastelein (New York, NY: Plenum Press), 481–503.
Montagu, G. (1821). “Description of a species of Delphinus, which appears to be new,” in Memoirs of the Wernerian Natural History Society, Vol. 3 (Black), 75–82.
Muraco, H., and Kuczaj, S. A. I. I. (2015). Conceptive estrus behavior in three bottlenose dolphins (Tursiops truncatus). Anim. Behav. Cogn. 2, 30–48. doi: 10.12966/abc.02.03.2015
Nachtigall, P. E. (1986). “Vision, audition, and chemoreception in dolphins and other marine mammals,” in Dolphin Cognition and Behavior: A Comparative Approach, eds R. J. Schustermann, J. A. Thomas, and F. G. Wood (Hillsdale, NJ: Lawrence Erlbaum Associates), 79–113.
Nachtigall, P. E., and Hall, R. W. (1984). Taste reception in the bottlenose dolphin. Acta Zool. Fennica 172, 147–148.
Nevitt, G. A., Veit, R. R., and Kareiva, P. (1995). Dimethyl sulphide as a foraging cue for Antarctic procellariiform seabirds. Nature 376, 680–682.
Norris, K. S. (1991). Dolphin Days: The Life and Times of the Spinner Dolphin. New York, NY: W. W. Norton.
Oelschläger, H. H. A. (2008). The dolphin brain – a challenge for synthetic neurobiology. Brain Res. Bull. 75, 450–459. doi: 10.1016/j.brainresbull.2007.10.051
Oelschläger, H. H. A., and Buhl, E. H. (1985). Development and rudimentation of the peripheral olfactory system in the harbor porpoise, Phocoena phocoena (Mammalia: Cetacea). J. Morphol. 184, 351–360.
Oelschläger, H. H. A., and Oelschläger, J. S. (2009). “Brain,” in Encyclopedia of Marine Mammals, 2nd Edn, eds W. F. Perrin, B. Würsig, and J. G. M. Thewissen (Burlington, MA: Academic Press), 134–149.
Pihlström, H. (2008). “Comparative anatomy and physiology of chemical senses in aquatic mammals,” in Sensory Evolution on the Threshold: Adaption in Secondarily Aquatic Mammals, eds J. G. M. Thewissen and S. Nummela (Berkeley and Los Angeles, CA: University of California Press), 95–109.
Purves, D., Augustine, G. J., Fitzpatrick, D., Katz, L. C., LaMantia, S., McNamara, J. O., et al. (2001). Neuroscience, 2nd Edn. Sunderland: Sinauer Ass.
Saslow, C. A. (2002). Understanding the perceptual world of horses. Appl. Anim. Behav. Sci. 78, 209–224. doi: 10.1016/S0168-1591(02)00092-8
Sil, S., Joseph, J., and Kumar, K. A. (2008). Changes in biogenic amines during iced and ambient temperature storage of tilapia. J. Sci. Food Agr. 88, 2208–2212. doi: 10.1002/jsfa.3058
Spitz, J., Mourocq, E., Leauté, J.-P., Quéro, J.-C., and Ridoux, V. (2010). Prey selection by the common dolphin: fulfilling high energy requirements with high quality food. J. Exp. Marine Biol. Ecol. 390, 73–77. doi: 10.1016/j.jembe.2010.05.010
Spitz, J., Rousseau, Y., and Ridoux, V. (2006). Diet overlap between harbour porpoise and bottlenose dolphin: an argument in favour of interference competition for food?. Estuarine Coast. Shelf Sci. 70, 259–270. doi: 10.1016/j.ecss.2006.04.020
Sumpton, W. D., Lane, B., and Ham, T. (2010). Gear modifications and alternative baits that reduce bait scavenging and minimize by-catch on baited drum-lines used in the Queensland Shark Control Program. Proc. R. Soc. Qld. 116, 23–35.
Thewissen, J. G. M., George, J., Rosa, C., and Kishida, T. (2011). Olfaction and brain size in the bowhead whale (Balaena mysticetus). Mar. Mam. Sci. 27, 282–294. doi: 10.1111/j.1748-7692.2010.00406.x
Watkins, W. A., and Wartzok, D. (1985). Sensory biophysics of marine mammals. Mar. Mam. Sci. 1, 219–260.
Werth, A. J. (2007). Adaptions of the cetacean hyolingual apparatus for aquatic feeding and thermoregulation. Anat. Rec. 290, 546–568. doi: 10.1002/ar.20538
Keywords: cetaceans, Tursiops truncatus, olfaction, gustation, flavor discrimination
Citation: Kremers D, Célérier A, Schaal B, Campagna S, Trabalon M, Böye M, Hausberger M and Lemasson A (2016) Sensory Perception in Cetaceans: Part II—Promising Experimental Approaches to Study Chemoreception in Dolphins. Front. Ecol. Evol. 4:50. doi: 10.3389/fevo.2016.00050
Received: 24 February 2016; Accepted: 24 April 2016;
Published: 10 May 2016.
Edited by:
Wayne Iwan Lee Davies, University of Western Australia, AustraliaReviewed by:
Thierry Bernard, Centre national de la Recherche Scientifique, FranceJason Neal Bruck, University of St. Andrews, Scotland
Copyright © 2016 Kremers, Célérier, Schaal, Campagna, Trabalon, Böye, Hausberger and Lemasson. This is an open-access article distributed under the terms of the Creative Commons Attribution License (CC BY). The use, distribution or reproduction in other forums is permitted, provided the original author(s) or licensor are credited and that the original publication in this journal is cited, in accordance with accepted academic practice. No use, distribution or reproduction is permitted which does not comply with these terms.
*Correspondence: Alban Lemasson, YWxiYW4ubGVtYXNzb25AdW5pdi1yZW5uZXMxLmZy