- Département de Géologie et Paléontologie, Muséum d'Histoire Naturelle, Genève, Switzerland
Since its usage by Darwin (1859), the concept of “living fossil” has undergone multiple definitions and has been much discussed and criticized. Soon after its discovery in 1938, the coelacanth Latimeria was regarded as the iconic example of a “living fossil.” Several morphological studies have shown that the coelacanth lineage (Actinistia) has not displayed critical morphological transformation during its evolutionary history and molecular studies have revealed a low substitution rate for Latimeria, indicating a slow genetic evolution. This statement, however, has been recently questioned by arguing that the low substitution rate was not real, and that the slow morphological evolution of actinistians was not supported by paleontological evidence. The assessment of morphological transformation among three vertebrate lineages during a time interval of circa 400 million years shows that the morphological disparity of coelacanths is much more reduced than the morphological disparity of Actinopterygii and Tetrapoda. These results support the idea that living coelacanths are singular organisms among the living world.
Introduction
The African coelacanth (Latimeria chalumnae) and the Indonesian coelacanth (L. menadoensis) are the only living representatives of the Actinistia, a sarcopterygian clade that appeared in the fossil record in the Early Devonian, circa 400 million years ago. These fishes have been nicknamed “living fossils” because they apparently meet some of the conditions of the original usage of this notion by Charles Darwin. Darwin (1859) regarded some animal taxa as “anomalous forms [that] may almost be called living fossils” because “new forms will have been more slowly formed,” they are “remnants of a once preponderant order” and “they connect to a certain extent orders now widely separated in the natural scale.” Since then, the term “living fossils” has acquired another meaning, i.e., species that were found as fossils before they were found as living forms, a definition also relevant for the coelacanths. We focus here on one aspect of this multiform definition: coelacanths as potential slowly evolving organisms. Several morphological studies have shown that the coelacanth lineage has not displayed critical morphological transformation during its evolutionary history following an early diversification episode in the Devonian (Schaeffer, 1952; Cloutier, 1991; Forey, 1998; Schultze, 2004; Friedman and Coates, 2006) and several molecular studies revealed a low genomic substitution rate for Latimeria for some categories of genes at least (Amemiya et al., 2013 and references therein). In a recent paper, however, Casane and Laurenti (2013) questioned the status of “living fossils” for coelacanths as slowly evolving organisms. They challenged the low substitution rate in Latimeria genome by scanning publications dealing with nuclear gene analyses. A majority of studies (8 among 12 papers) indicates no conclusive evidence for a slow evolution of the coelacanth genome, and those pointing out a slowly evolving genome are mostly based on studies of the HOX gene clusters (2 among 12). At the same time, the full genome sequencing of Latimeria chalumnae was made available (Amemiya et al., 2013), and its study provided ambivalent results with respect to Casane and Laurenti's view by showing that protein-coding genes are significantly more slowly evolving than those of tetrapods, unlike other genomic features.
Casane and Laurenti (2013) also questioned the morphological stability of Actinistia by arguing that no fossils of the genus Latimeria have been found so far, suggesting that morphological differences between extant and extinct coelacanths are important enough to be grouped into distinct genera. They also stated that the morphological stability of coelacanths is not supported by paleontological evidence, and illustrated the actinistian morphological disparity by comparing the body morphology of nine extinct genera ranging in age from the Lower Devonian to the Cretaceous along with the Recent Latimeria. Among anatomical structures taken as examples of characters varying through time, they listed the number of vertebral elements, the ratio between the abdominal and the caudal regions, and pointed out differences in the general body morphology. They briefly compared the anatomy of Latimeria with the Cretaceous Macropoma and noticed differences concerning the orientation of the gape and the shape and relative proportions of some skull bones.
Here we address the issue of coelacanth morphological transformations through deep time. We do not question the statement that morphological differences are observed between extinct actinistian taxa and Latimeria but rather test whether morphological changes occurred in actinistians at the same pace as in other comparable major vertebrate lineages by comparing rate of anatomical novelties acquisition along corresponding evolutionary trajectories. Although computations of morphological acquisitions along the actinistian lineage were performed by several authors (Schaeffer, 1952; Forey, 1988, 1998; Cloutier, 1991; Schultze, 2004), their purpose was to assess fluctuations of morphological changes and not to compare transformations rates with other clades, as proposed here.
Comparing Evolutionary Histories of Vertebrate Lineages
In order to address the issue of morphological transformations through time, we selected three clades of crown-group vertebrates: the Actinistia (coelacanths), the tetrapods (here encompassing Tetrapoda and their closest relatives), and the Actinopterygii (ray-finned fishes) (Figure 1) and propose phylogenetic reconstructions of corresponding extant genera, the coelacanth (Latimeria), a hummingbird (Trochilus) and the perch (Perca) (Figure 2). The Chondrichthyes (chondrichthyans) and the Dipnoi (lungfishes) could not be included in this study because the post-Paleozoic fossil record of these clades lacks sufficient taxa represented by complete and articulated specimens, which prevents homogeneous comparison with the other extant clades considered. The phylogenetic reconstructions presented here are based on a similar model as in the Figure 1 of Casane and Laurenti (2013), but with the notable difference that they are plotted against a time frame (Figure 2A). For each actinistian terminal taxon retained in the original figure of Casane and Laurenti (2013), we looked for approximately coeval tetrapod and ray-finned fish taxa, which have been proposed as close relatives of hummingbird and perch, respectively. Because of the incompleteness of the fossil record, variations in the stratigraphic ranges occur for some of the corresponding genera between the three lineages, and the selected taxa do not represent the closest phylogenetic relatives in all instances, but a genus close to it with a reasonably well-known anatomy (similarly, the actinistian cladogram displays a selection of genera). In this figure, the genera corresponding to the coelacanth Macropoma are Ichthyornis and Hoplopteryx for tetrapods and actinopterygians, respectively, and the genera corresponding to Mawsonia are Gansus and Enchodus, respectively, and so on through deep time. In addition, note that because Miguashaia—the sister group to all other actinistians in Casane and Laurenti's phylogeny—is not the oldest known representative of this clade, the patterns of the three compared cladograms slightly differ at their bases. Moreover, a few choices were made between concurrent phylogenies, such as, for instance, the placement of Dialipina as sister group of all other ray-finned fishes (Zhu et al., 2009).
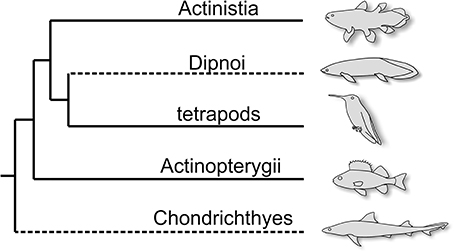
Figure 1. Interrelationships among the five crown-vertebrate clades. The lineages with stippled lines are not included in this study, because their fossil record is mostly composed of fragmentary remains (teeth and tooth plates) for the second half of their evolutionary histories.
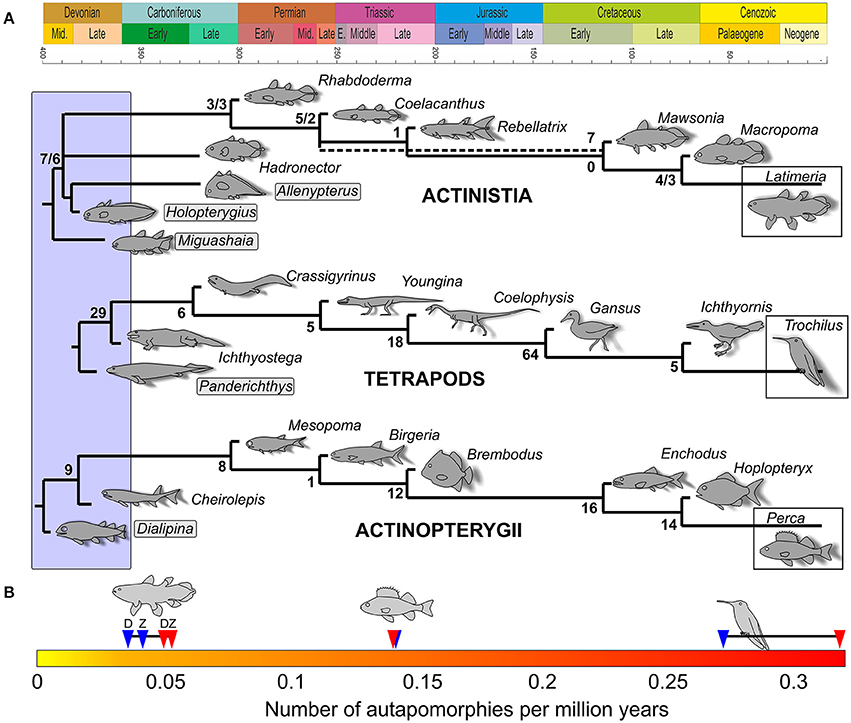
Figure 2. (A) Cladograms of the three crown-vertebrate clades included within a time frame. Numbers indicate uniquely derived apomorphies supporting each node [for actinistians, numbers separated by a slash bar are counted based on data from Dutel et al. (2012) and Zhu et al. (2012), respectively]. (B) Scale indicating the acquisition rate of uniquely derived apomorphies per million years for the three clades. Red arrows indicate rates calculated with the total number of unique apomorphies, blue arrows indicate rates without the basalmost node for each clade (gray frame in the phylogenies above). For actinistians, D and Z indicate calculation with data from Dutel et al. (2012) and Zhu et al. (2012), respectively.
The three calibrated cladograms represent the evolutionary histories of the coelacanth, hummingbird and perch during a similar time interval of almost 400 million years. In a second step, we searched in literature for uniquely derived morphological character states that occurred along the corresponding internodes of the three cladograms. A uniquely derived character is a character that “has evolved only in one direction on a single occasion in the history of the group” (LeQuesne, 1972). Uniquely derived characters, referred to as unique apomorphies below, may or may not include unambiguous character transformations. The rate of unique apomorphies acquisition per million years is calculated for the three lineages by dividing the total number of unique apomorphies by 400 (Figure 2B). Because of the different patterns between the basal part of the three phylogenies, and because of the taxonomic uncertainties mentioned above, the number of unique apomorphies in the three lineages were also computed by excluding the basalmost nodes, i.e., by excluding Miguashaia and the clade Holopterygius + Allenypterus in actinistians, Panderichthys in the tetrapod lineage and Dialipina in actinopterygians. In this case, we consider an average total time interval of 360 Ma for calculating unique apomorphy acquisition per million years.
For actinistians, we used the set of characters from Dutel et al. (2012), which are coded for all genera under consideration, and the set of characters from Zhu et al. (2012), which are coded for all genera except Rebellatrix. Because no phylogenies including all the genera used in the tetrapod and the ray-finned lineages are available, we mapped the characters using several published sources. We selected from each study unique apomorphies that support the six nodes. Unique apomorphies correspond to character changes with a consistency index of 1, although we cannot exclude that for some of them, reversions are possible if the total set of terminal taxa was considered in the phylogenetic analyses. Moreover, we made the assumption that adding taxa would not add unique apomorphies. Because some of the studies used here do not provide lists of character transformations associated with their consistency indexes, we ran the original datamatrices and searched directly for unique apomorphies supporting the nodes (Supplementary Table S1). Table 1 shows the total number of unique apomorphies and the calculated rates of acquisition of unique apomorphies per million years, with and without the basalmost node. Lists of synapomorphies for each of the three lineages are available in Supplementary Material (Supplementary Table S1).
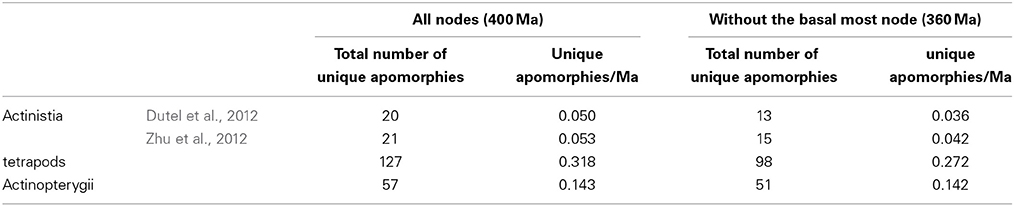
Table 1. Total number of unique apomorphy and corresponding rates of unique apomorphy acquisition per million years for each reconstructed trees.
Morphological Disparity
Superficial comparisons between the calibrated cladograms of tetrapods on one hand, and of the two fish lineages on the other hand clearly indicate that morphological transformations are much more pronounced in the tetrapod evolutionary history (Figure 2).
Looking more precisely into the character changes that occur along the three calibrated cladograms is more informative. The rate of unique apomorphy acquisition in the tetrapod lineage, with and without the basalmost node, is circa twice the rate in the ray-finned lineage and about six times higher than in the actinistian lineage, thus confirming the deeper morphological transformation in the tetrapod lineage than in the fish lineages. The rate is approximately three times higher in the ray-finned lineage than in the actinistian lineage.
Quantifying synapomorphy acquisition is a first approach that should be supplemented by a discussion on the nature of the characters involved in the three clades. In the hummingbird descent, the 127 apomorphies (or 98 without the basalmost node) affect all parts of the skeleton, in particular osteological complexes, or key-innovations, directly related to changes in life environment. In the descent of the perch, the apomorphies also concern all parts of the skeleton (e.g., the skull with mostly buccal and branchial apparatus, vertebral column and fin structure). Other morphological trends occur along this lineage, but are not mapped because they are not precisely located within the general phylogeny. These transformations include changes in histology, such as multilayered ganoine and acrodine tissue that appeared at the base of the cladogram (Schultze and Cumbaa, 2001), the acellular bone present in teleosts (Parenti, 1986), and a general trend of decreasing ossification of the dermal skeleton associated with an increasing ossification of the endoskeleton (Sire et al., 2009). These complex modifications would have been coded through numerous different characters if they were included in a global analysis of the whole actinopterygian clade. They do not appear here because the studies we used are too specific to integrate them. In the descent of Latimeria, the transformations affect mainly details of bones, such as absence/presence of processes or laminae, general shape, fusion/autonomy of ossifications, ornamentation, and pattern of the sensory canal system. The trend toward a near-symmetrical arrangement of radials and finweb distribution around the pectoral lobe is an osteological transformation occurring along the actinistian lineage (Friedman et al., 2007) that is not mapped here because it does not correspond to a discrete character. Exceptions among the coelacanth morphological monotony, however, are the genera Miguashaia, Holopterygius, and Allenypterus, which are basal actinistians with derived characters (Friedman and Coates, 2006). However, their characters are not mapped in Figure 2 because they are not located on the direct lineage of Latimeria.
Although quantifying the amount of morphological transformations is a challenging task tainted with methodological biases, our comparison indicates that morphological changes in the actinistian lineage is markedly lower than in the other two clades, at least after the Late Devonian early morphological diversification of the group (Friedman and Coates, 2006). This difference is likely underestimated in the present study because the calculation for actinistians rests on two data sets that cover the whole sampled taxa, whereas the calculations for actinopterygians and tetrapods are based on several datasets specific to some parts of the phylogenies of these groups. Hence, we assume that if such datamatrices were built for restricted numbers of selected taxa, but encompassing the whole range of the clades as adopted here for actinistians, the number of synapomorphies in both lineages would be much higher and would include, notably, coded characters for the general “trends” mentioned above. The present study, however, is a partly quantitative approach that shows that the actinistian lineage experienced much less morphological transformation than both other compared lineages during the last 360 million years, at least.
It is noteworthy that the choice of the terminal taxa in Figure 1 has an impact on the amount of synapomorphies mapped in the internodes. Choosing for instance the hellbender salamander (Cryptobranchus) and the bichir (Polypterus) as recent representatives of the tetrapods and actinopterygians, respectively, would have probably lowered the amount of osteological synapomorphies in the internodes. Although this should be borne in mind, our aim here is not to search for examples among the compared clades that may have experienced slower morphological evolution. Instead, we aim to emphasize that during the last 400 Ma of their evolutionary history the tetrapod and actinopterygian lineages encompassed representatives that underwent critical morphological transformations whereas such broad morphological modifications are absent in the descent of Latimeria.
“Living Fossils”: A Deep Time Perspective
Casane and Laurenti (2013) supposed that the concept of “living fossil” rests in part on “the remnant misinterpretation of biodiversity as a “ladder of progress.” We do not consider that this Aristotelian notion is the main support of the “living fossil” concept, which rests in our opinion on the genuine fact that paces of morphological evolution vary between vertebrate groups. Casane and Laurenti's rejection of the “living fossil” concept is based on the absence of notions such as deep or geological time in their analysis. Their discussion of cladogram topology lacks any temporal dimension and leads to confusion between synchronous and asynchronous trees (Podani, 2013). Moreover, using Hennig's statement that ancestral species cease to exist as two new sister species form as the basis of the “tree-thinking” point of view (it is actually the way Hennig defined species), they ground their criticism on a strictly “pattern reconstruction” approach (Rieppel, 2010). Such an approach is timeless and excludes evolutionary processes, such as budding speciation (Mayr and Bock, 2002), a process more likely to occur in species with a long stratigraphic range. When included within a time frame, notions such as “basal” or “early branching” make sense for clades diverging early in time along the evolutionary history of a clade (for instance Miguashaia, Dialipina, and Panderichthys are regarded as basal taxa in Figure 2). However, it is noteworthy that including a cladogram within a time-scale does not justify notions of “primitive,” “evolved,” and “progress,” which are subjective concepts suggesting that evolution is oriented.
Conclusions
Although “living fossils” are obviously not non-evolving organisms, they gather an informal group of slowly evolving organisms. We agree with Casane and Laurenti (2013) that the term “living fossil” may affect the understanding of evolution, especially by non-professional biologists. But we insist on the fact that the morphological evolutionary history of Latimeria, together with those of other extant organisms (e.g., lampreys, Australian lungfish, tuatara), shows slow morphological evolution, and we point out that this peculiar condition should not be overlooked. In addition to its slow rate of morphological evolution, Latimeria is located at the tip of an extremely long evolutionary branch (split from its closest extant relative circa 450 million years ago). This feature is also found in the Australian lungfish, Neoceratodus forsteri (split from its closest extant relative circa 250 million years ago) and in that sense, both taxa have a higher evolutionary distinctiveness than, for instance, one specific species of the recently diversified whitefish genus Coregonus (Jacobsen et al., 2012).
Although all these fishes have comparable positions in “pattern reconstructed” trees, the evolutionary distinctiveness caused by slow morphological transformation and by evolutionary isolation make Latimeria and Neoceratodus special cases among the living world, which should impact on their conservation status (Cavin and Kemp, 2011). The sounder conclusion about the interest of these distinct taxa is by Darwin himself: “Species and groups of species, which are called aberrant, and which may fancifully be called living fossils, will aid us in forming a picture of the ancient forms of life” (Origin of Species, conclusion).
Conflict of Interest Statement
The authors declare that the research was conducted in the absence of any commercial or financial relationships that could be construed as a potential conflict of interest.
Acknowledgments
We thank Manuel Ruedi (MHNG) for discussion of this topic and the reviewers, David Marjanović and Gaël Clément, for their relevant and thorough comments during the interactive review process. This paper is a contribution of the project “Fish response to long-term global changes” supported by Swiss National Science Foundation (200021-140827).
Supplementary Material
The Supplementary Material for this article can be found online at: http://www.frontiersin.org/journal/10.3389/fevo.2014.00049/abstract
References
Amemiya, C. T., Alföldi, J., Lee, A. P., Fan, S., Philippe, H., MacCallum, I., et al. (2013). The African coelacanth genome provides insights into tetrapod evolution. Nature 496, 311–316. doi: 10.1038/nature12027
Casane, D., and Laurenti, P. (2013). Why coelacanths are not “living fossils.” Bioessays 35, 332–338. doi: 10.1002/bies.201200145
Cavin, L., and Kemp, A. (2011). The impact of fossils on the evolutionary distinctiveness and conservation status of the Australian lungfish. Biol. Conserv. 144, 3140–3142. doi: 10.1016/j.biocon.2011.08.014
Cloutier, R. (1991). “Patterns, trends, and rates of evolution within the Actinistia,” in The Biology of Latimeria chalumnae and Evolution of Coelacanths, eds J. A. Musick, M. N. Bruton, and E. K. Balon (Netherlands: Springer), 23–58.
Darwin, C. (1859). On the Origin of the Species by Means of Natural Selection, or the Preservation of Favoured Races in the Struggle for Life. London: John Murray.
Dutel, H., Maisey, J. G., Schwimmer, D. R., Janvier, P., Herbin, M., and Clément, G. (2012). The giant Cretaceous coelacanth (Actinistia, Sarcopterygii) Megalocoelacanthus dobiei Schwimmer, Stewart and Williams, 1994, and its bearing on Latimerioidei interrelationships. PLoS ONE 7:e49911. doi: 10.1371/journal.pone.0049911
Forey, P. (1988). Golden Jubilee for the coelacanth Latimeria chalumnae. Nature 336, 727–732. doi: 10.1038/336727a0
Friedman, M., and Coates, M. I. (2006). A newly recognized fossil coelacanth highlights the early morphological diversification of the clade. Proc. Biol. Sci. 273, 245–250. doi: 10.1098/rspb.2005.3316
Friedman, M., Coates, M. I., and Anderson, P. (2007). First discovery of a primitive coelacanth fin fills a major gap in the evolution of lobed fins and limbs. Evol. Dev. 9, 329–337. doi: 10.1111/j.1525-142X.2007.00169.x
Jacobsen, M. W., Hansen, M. M., Orlando, L., Bekkevold, D., Bernatchez, L., Willerslev, E., et al. (2012). Mitogenome sequencing reveals shallow evolutionary histories and recent divergence time between morphologically and ecologically distinct European whitefish (Coregonus spp.). Mol. Ecol. 21, 2727–2742. doi: 10.1111/j.1365-294X.2012.05561.x
LeQuesne, W. J. (1972). Further studies based on the uniquely derived character concept. Syst. Biol. 21, 281–288. doi: 10.1093/sysbio/21.3.281
Mayr, E., and Bock, W. J. (2002). Classifications and other ordering systems. J. Zool. Syst. Evol. Res. 40, 169–194. doi: 10.1046/j.1439-0469.2002.00211.x
Parenti, L. R. (1986). The phylogenetic significance of bone types in euteleost fishes. Zool. J. Linn. Soc. 87, 37–51. doi: 10.1111/j.1096-3642.1986.tb01329.x
Podani, J. (2013). Tree thinking, time and topology: comments on the interpretation of tree diagrams in evolutionary/phylogenetic systematics. Cladistics 29, 315–327. doi: 10.1111/j.1096-0031.2012.00423.x
Rieppel, O. (2010). The series, the network, and the tree: changing metaphors of order in nature. Biol. Philos. 25, 475–496. doi: 10.1007/s10539-010-9216-4
Schaeffer, B. (1952). Rates of evolution in the coelacanth and dipnoan fishes. Evolution 6, 101–111. doi: 10.2307/2405507
Schultze, H.-P. (2004). “Mesozoic sarcopterygians,” in Mesozoic fishes 3—Systematics, Paleoenvironments and Biodiversity, eds G. Arratia and A. Tintori (München: Verlag Dr. Friedrich Pfeil), 463–492.
Schultze, H.-P., and Cumbaa, S. L. (2001). “Dialipina and the characters of basal Actinopterygians,” in Major Events in Early Vertebrate Evolution: Palaeontology, Phylogeny and Development, ed P. Ahlberg (London: Taylor and Francis), 315–332.
Sire, J.-Y., Donoghue, P. C. J., and Vickaryous, M. K. (2009). Origin and evolution of the integumentary skeleton in non-tetrapod vertebrates. J. Anat. 214, 409–440. doi: 10.1111/j.1469-7580.2009.01046.x
Zhu, M., Yu, X., Lu, J., Qiao, T., Zhao, W., and Jia, L. (2012). Earliest known coelacanth skull extends the range of anatomically modern coelacanths to the early Devonian. Nat. Commun. 3, 772. doi: 10.1038/ncomms1764
Keywords: coelacanths, tetrapods, ray-finned fishes, fossil record, morphological disparity
Citation: Cavin L and Guinot G (2014) Coelacanths as “almost living fossils”. Front. Ecol. Evol. 2:49. doi: 10.3389/fevo.2014.00049
Received: 05 May 2014; Accepted: 28 July 2014;
Published online: 13 August 2014.
Edited by:
Zerina Johanson, Natural History Museum, UKReviewed by:
David Marjanović, UMR 7207, AustriaGaël Clément, Museum National d'Histoire Naturelle, France
Copyright © 2014 Cavin and Guinot. This is an open-access article distributed under the terms of the Creative Commons Attribution License (CC BY). The use, distribution or reproduction in other forums is permitted, provided the original author(s) or licensor are credited and that the original publication in this journal is cited, in accordance with accepted academic practice. No use, distribution or reproduction is permitted which does not comply with these terms.
*Correspondence: Lionel Cavin, Département de Géologie et Paléontologie, Muséum d'Histoire Naturelle, 1 rue Malagnou, CP6434, 1211 Genève 6, Switzerland e-mail: lionel.cavin@ville-ge.ch