- 1Department of Population Health Sciences, Section Animals in Science and Society, Faculty of Veterinary Medicine, Utrecht University, Utrecht, Netherlands
- 2Department of Pediatrics, Wilhelmina Children’s Hospital, University Medical Centre Utrecht, Utrecht University, Utrecht, Netherlands
- 3Department of Pediatric Pulmonology, Wilhelmina Children’s Hospital, University Medical Center Utrecht, Utrecht University, Utrecht, Netherlands
Introduction: Social play behaviour facilitates the development of social, emotional and cognitive capacities, including resilience. Deprivation of social play in rats leads to alterations in anxiety, stress and social behaviour. The aim of this study was to elucidate the effects of social play deprivation, specifically on the responsivity to social and non-social challenges. We hypothesised that play deprivation leads to impairments in stress resilience later in life, particularly in social contexts.
Methods: To test this, play-deprived rats were compared with undeprived control rats for their responsivity to (1) a novel environment, (2) facing an aggressive male rat in its territory (resident-intruder test) and (3) an encounter with two unfamiliar conspecifics in a neutral, familiar environment (stranger encounter test).
Results: Corticosterone concentrations in response to these different stressors were comparable between play-deprived and control rats. Behavioural analysis revealed that play-deprived rats responded similar to undeprived controls when confronted with an aggressive rat in its territory. In the stranger encounter test, the unfamiliar strangers directed more play behaviour towards play-deprived animals than towards undeprived control animals.
Discussion: Together our results indicate that, except for subtle differences in play behaviour in a social challenging condition, play deprivation did not result in altered corticosterone responses to the different stressors. Our data add to the existing knowledge about the impact of social play for the development of resilient and social behaviour later in life.
Introduction
Social play is a highly rewarding activity and is abundantly displayed in young mammals. including humans, as well as in certain birds and reptiles (Graham and Burghardt, 2010; Ginsburg et al., 2007; Pellis and Pellis, 2009; Vanderschuren and Trezza, 2014). It is well accepted that play is important for a healthy development of brain and behaviour (Ginsburg et al., 2007; Pellis and Pellis, 2009; Achterberg and Vanderschuren, 2023). Play allows for experimentation with one’s behavioural repertoire. Therefore, it is assumed that play facilitates the development of social competence, emotional and motor capacities, resilience, creativity and problem-solving skills (Pellis and Pellis, 2009; Vanderschuren and Trezza, 2014; Špinka et al., 2001; Lillard, 2017; Nijhof et al., 2018).
Rodent studies have been instrumental in understanding the impact of social play on the development of social behaviour, but also on (emotional) resilience. For example, rats that were deprived of social play have been shown to develop social and cognitive dysfunctions. Several studies have revealed anomalies in anxiety, stress and social behaviour in rats that were deprived of social play behaviour (Hol et al., 1999; Van den Berg et al., 1999; Von Frijtag et al., 2002; Lukkes et al., 2009; Baarendse et al., 2013; Bijlsma et al., 2022). In line with these findings, human case studies suggest that play in a natural environment can increase children’s problem-solving skills and resilience to stress (Harrison and Harrison, 2013), while reduced risky and outdoor play is associated with lower self-esteem and academic achievements (Tremblay et al., 2011, 2015). An important group in this respect are children with a neurodevelopmental disability or with a chronic disease (Nijhof et al., 2018). Limited opportunities to participate successfully in (social) play may contribute to the risk for developmental delays in the social, emotional, physical, and cognitive domain that characterises these children (e.g. Pinquart, 2017; Maurice-Stam et al., 2019; Martinez et al., 2011; Schulte and Barrera, 2010; Munambah et al., 2020).
The hypothalamus-pituitary-adrenal (HPA) axis has been implicated in the regulation of the stress response and play behaviour in rats. For example, repeated treatment with corticosterone during early life (postnatal day (PND) 1-4) reduced social play behaviour during adolescence (Meaney et al., 1982), while daily injections with corticosterone from PND26-40 did not affect social play behaviour (Meaney and Stewart, 1983). Glucocorticoid treatment between PND 28 and 42 caused more aggressive play and blunted the corticosterone response to novel environment stress in another study (Veenit et al., 2013). The regulation of the HPA axis changes from pre-weaning through adolescence (McCormick et al., 2008), paralleling the marked neuronal development and reorganisation during adolescence (Willing and Juraska, 2015).
The aim of this study was to further elucidate the effects of social play deprivation, with a particular focus on the responsivity to social and non-social stressors. We hypothesised that a lack of social play experience during early life leads to atypical tuning of the hypothalamic-pituitary-adrenal axis and thereby to impairments in stress resilience later in life, particularly in social contexts. To test this, rats deprived of social play (SPD) were compared with rats that were group housed (i.e. they could play freely) during early adolescence for their responsivity to situations both humans and rats encounter in their natural environment. As a non-social stressor, a novel environment test (NET) was performed. As social stressors, we exposed the rats either to a modified resident-intruder test (RIT) or to two strangers in a familiar environment (stranger encounter test, SET).
Methods
Animals
Male Lister Hooded rats (N = 84; Charles River, Sulzfeld, Germany) arrived at the animal facility with nursing mothers at PND 14. They were housed in Macrolon cages (L40 x W26 x H20 cm) under controlled conditions (20-21°C temperature, 60-65% relative humidity) at a 12-h light/dark circle (lights off at 9 pm). Food and water were available ad libitum. In addition, male Wistar rats (N = 8, >400g), from an in-house breeding colony, were used as resident for the resident/intruder test. These rats were solitarily housed in another room under otherwise identical housing conditions and selected for their territorial behaviour. Experimental procedures were carried out in the dark phase. Experiments were approved by the Animal Ethics Committee of Utrecht University and in accordance with the Dutch and European regulations (Wet op dierproeven 2014 and Guideline 86/609/EEC; Directive 2010/63/EU).
Social play deprivation
Social play behaviour in rats is most abundant between PND 21-42 (Panksepp, 1981). Play deprivation was induced as previously described (Bijlsma et al., 2022, 2024, Marquardt et al., 2023; Peartree et al., 2012). Immediately after weaning on PND 21, the rats were divided into either the control or SPD group in weight matched pairs, both from the same mother. A transparent plexiglass barrier separated the rats in the cages of the social SPD pairs (see Figure 1). The barrier contained small holes allowing the rats to see, smell and hear each other, but preventing them to engage in social (play) and other behaviour involving physical contact. From PND 21 to 42, the rats were only handled for their weekly health check and cage change. After the social play deprivation period on PND 42, SPD rats were re-socialised by removing the barrier. Both groups stayed housed in the same pairs until the experiments commenced in adulthood from PND 75 onwards. After re-socialisation, the rats were frequently handled and habituated to the tail incision method used for blood sampling (Figure 1).
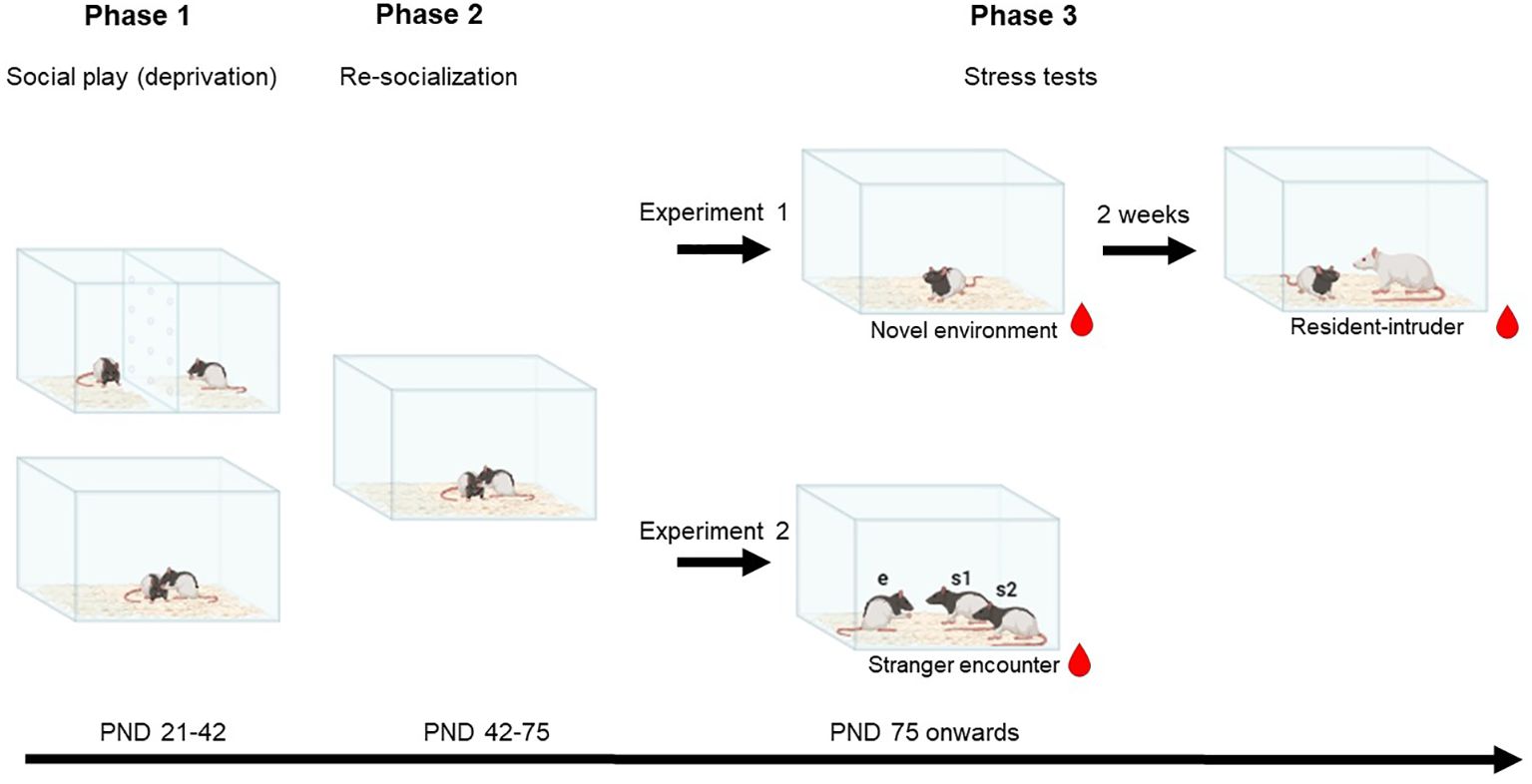
Figure 1. Schematic of the experimental design. On postnatal day (PND) 21, rat pairs were divided into the control or social play deprivation (SPD) group. A transparent, perforated barrier separated the rats of the SPD group, making them unable to engage in social play behaviour. After 3 weeks, on PND 42, the barriers were removed. In adulthood, between PND 74 and 112, in experiment 1 rats were subjected to the novel environment and resident-intruder stress tests. In experiment 2, rats were subjected to a stranger encounter paradigm. Plasma corticosterone was measured at different time points before, during, and after the tests. Social behaviour was scored in the resident-intruder and stranger encounter test. This image was created using BioRender.com.
Stress tests
Different tests were chosen to represent challenging situations that both humans and male rats encounter in their natural environment as adults. It is plausible for adult humans to come across novel environments, meet multiple strangers in a familiar environment (e.g. in a pub) or encounter aggressive individuals. Male rats in the wild may leave their natal areas (Greenwood, 1980; Gardner-Santana et al., 2009; Gatto-Almeida et al., 2022). During that transition, rats may encounter novel environments that need to be explored and aggressive conspecifics in their respective territories. It is also possible for rats to come across strangers when they themselves establish a new territory. These challenges can be tested in a laboratory setting using the NET and RIT. In addition, we designed the SET to capture behaviour displayed when confronted with two unfamiliar animals in a familiar environment. Specifics of these tests are described below.
Novel environment test
The NET was used to examine corticosterone concentrations in response to a non-social mild stressor (Brown et al., 1974; File and Peet, 1980; Marques et al., 2008). Rats were placed alone in a novel environment, i.e. a clean Macrolon cage (L40 x W26 x H20 cm) for 180 minutes with food and water access. Blood samples were taken right before the NET (baseline) and 15, 30, 45, 60, 90, 120 and 180 minutes into the NET.
Modified resident-intruder test
The RIT, as described by Koolhaas et al. (2013) is a standardised test to measure aggression and social stress. We decided to modify the paradigm to a milder social stress paradigm. For that purpose, the resident was not housed with a female companion before the test. We theorised that this would make the resident less aggressive and elicit a subtle form of social stress rather than only physical stress due to fighting. The behaviour of the intruder (focal rat) directed towards the resident (unfamiliar conspecific) and vice versa was analysed.
This test was performed two weeks after the novel environment test. Prior to the modified resident-intruder test, the resident rats (unfamiliar conspecific) were placed into the test room in their own homecage. One by one, the focal rats were placed into one of the residents’ cages for 10 minutes. Over two weeks, every resident was used once a week and had 2 interactions on its designated test-day. The interaction was recorded and stored for offline behavioural analysis. Blood samples were taken from the focal rats 60 minutes before (baseline) and 15, 30, 45, 60, 90, 120 and 180 minutes after the end of the RI test, during which the focal rats were housed individually. After the last blood sample was taken, the focal rats returned to their homecages.
Stranger encounter test
During the period between social play deprivation and testing in adulthood (PND 75-80), the focal animals were habituated to the tail incision procedures and the test cages (social interaction cage: L40 x W40 x H60 cm). The stimulus rats (strangers) were habituated to encountering an unfamiliar animal in the test cages. In this habituation phase, the unfamiliar animals were “stranger” rats from a different homecage.
Both the focal and the stranger rats were socially isolated 24 hours prior to the experiment to control the level of and enhance the motivation for social interaction. This test lasted for 15 minutes, during which time the focal rat (control or SPD) was introduced in the test cage to the pair of stranger rats. After the test, the animals returned to their respective homecages. This test was repeated 3 times for each focal animal, with at least one day in between and every time the focal animals were unfamiliar to the stranger pair of rats. Around the first test, blood samples were collected 60 min prior to (baseline) and 15, 45 and 90 minutes after the start of the SET.
Blood sampling and corticosterone analysis
Blood sampling
During the intermediate period between SPD and testing in adulthood, the experimental animals were habituated to the tail incision procedures, a procedure with minimal consequences for corticosterone concentrations (Fluttert et al., 2000). These habituation procedures consisted of several steps. Repetitions of procedures were performed on different days. Step 1: Rats could explore the towel for 5 minutes; this was repeated 3 times. Step 2: While exploring the towel, part of the towel is placed on top of the rat. This was repeated 3 times. Step 3: While exploring the towel, part of the towel is placed on top of the rat and the rat is gently restrained in one place while the tail is gently held. This is repeated 5 times. Step 4: Similar to step 3 but a covered razorblade is pressed against the tail while the tail is being held.
Radioimmunoassay
To examine the corticosterone concentration in blood plasma at different time points (NET and RIT), blood samples (< 100 µL) were collected with Microvettes (CB 300 K2E, Sarstedt, Nümbrecht, Germany) from a small incision in one of the lateral tail veins and stored on ice (Fluttert et al., 2000). The samples were centrifuged the same day (10 min, 2400 rpm, -4°C. Microlite RF, Thermo Refrigerated Centrifuge) and plasma was stored at -20°C until further processing. Plasma corticosterone concentrations were determined in duplicate using ImmuChem™ Double Antibody Corticosterone 125I radioimmunoassay kits for rats and mice (MP Biomedicals LLC, Orangeburg. USA). The samples were diluted 1:200 and processed according to the manufacturer’s protocol. The covariance coefficient between the duplicate samples was always <10%, indicating reliable duplications. The average corticosterone concentration between duplicates was used for the final analyses.
Enzyme-linked immunosorbent assay for plasma corticosterone analysis
For the SET, plasma concentrations of corticosterone were measured using a commercially available rat corticosterone (CORT) high sensitivity ELISA kit (AC-15F1, Immunodiagnostic Systems) according to the manufacturer’s instructions. Blood was collected in heparin-coated test tubes and was centrifuged at 4°C for 30 min at 3000 rpm. The supernatant (plasma) was collected and stored at -20°C until analysed. Samples were tested in duplicate and were processed in a 1:10 dilution according to the manufacturer’s instructions. GraphPad Prism was used to interpolate corticosterone concentrations from a four-parametric logarithmic standard curve. Intra-assay variability was between 5.99 and 9.62% CV (coefficient of variation) and inter-assay variability was 5.20% CV.
Behavioural analysis
Behaviour in the modified RIT and SET was recorded (Logitec C922 Pro Stream webcam) and stored for off-line analysis. All behavioural data were analysed using Observer XT 15/16 (Noldus Information Technology B.V., Wageningen, the Netherlands).
For the modified RIT, the behaviour of both the resident and intruder rats was assessed. The behaviours that we observed were based on previous detailed descriptions of the behavioural repertoire of residents and intruders (Koolhaas et al., 2013). For the resident, the following behaviours were scored (duration and frequency); inactivity, non-social behaviour, moving towards, social exploration, ano-genital sniffing, lateral threat, upright position, clinch attack and keep down. For the intruder, non-social behaviour, moving towards, social exploration, freezing, upright position, clinch attack (initiated by resident) and submission were assessed (see Table 1 for the ethogram).
For the SET, the focal animals were the experimental rats. The analysis was aimed at understanding their interaction with the stranger rats, based on the paradigms described in Pellis et al. (2022). The behaviour of the stranger rats directed to one another is not discussed in this paper. The frequency and duration of the following behaviours were scored: pouncing, pinning, social exploration and non-social behaviour (see Table 2 for the ethogram).
Statistical analyses
The data were analysed with GraphPad Prism 9.3.0 for Windows (GraphPad Software, San Diego, USA).
Novel environment test
Two-way repeated measures ANOVA was used to analyse the corticosterone response in the NET (control, N = 16 and SPD, N = 16), with group as the between-subjects factor and time as the within-subjects factor.
Modified resident-intruder test
After the RIT, it became clear that in some cases the residents fought with their intruder, thereby possibly provoking more social stress than intended with this test and compared to the majority of the experimental animals. Therefore, we decided to exclude the corticosterone and behavioural data from the intruders that were engaged in fights (N = 4 and 3 from control and SPD, respectively) from the RIT analyses. The corticosterone response data for these animals were analysed separately (see Supplementary Data S5). Because of one missing data point, the multiple imputation method (5 imputations) was used to enter this missing data point after which a repeated measures ANOVA was used to analyse the RIT data (control, N = 12 and SPD, N = 13), again with group as the between-subjects factor and time as the within-subjects factor. To determine differences in the intruders’ behavioural response during the RIT, we compared the total duration, frequencies, and latencies to start the behaviour between groups using an unpaired Students t-test.
Stranger encounter test
The behavioural data were analysed using a repeated measures ANOVA with test-day as within-subjects factor and treatment as between-subjects factor.
Corticosterone concentrations
The corticosterone concentrations for the three tests (novel environment, resident intruder and stranger encounter) were analysed using repeated measures ANOVAs with time as within-subject factor and group as between-subjects factor.
To test whether variances of the differences between treatments were equal, the Geisser and Greenhouse epsilon hat method was used. If the assumption of sphericity was violated, the degrees of freedom were corrected to more conservative values. Corrected degrees of freedom are presented rounded to the nearest integer. Post hoc pairwise comparisons were performed with Tukey’s tests, when applicable. For all statistical tests, alpha was set to 0.05.
Results
Plasma corticosterone response in the NET
Analysis of the plasma corticosterone concentrations in the NET revealed a significant overall time-dependent corticosterone response (Ftime(3.4, 104.5) = 19.27, p<0.0001). The SPD rats reached peak corticosterone concentrations at 30 min (279 ± 32.77 ng/ml) and corticosterone concentrations peaked at 90 min for the control group (244.96 ± 50.42 ng/ml). However, SPD did not significantly affect the plasma corticosterone response in the NET, when compared to control rats (Ftreatment*time (7,210) = 1.74, p=0.10), nor was there an overall group effect (Ftreatment(1,30) = 0.094, p=0.76; Figure 2A). At 180 min, plasma corticosterone returned to concentrations comparable or lower to before exposure to the novel environment (t = 0). Peak values or area under the curve (AUC) did not differ between control and SPD rats (data not shown). For the numerical data, see Supplementary Table S1.
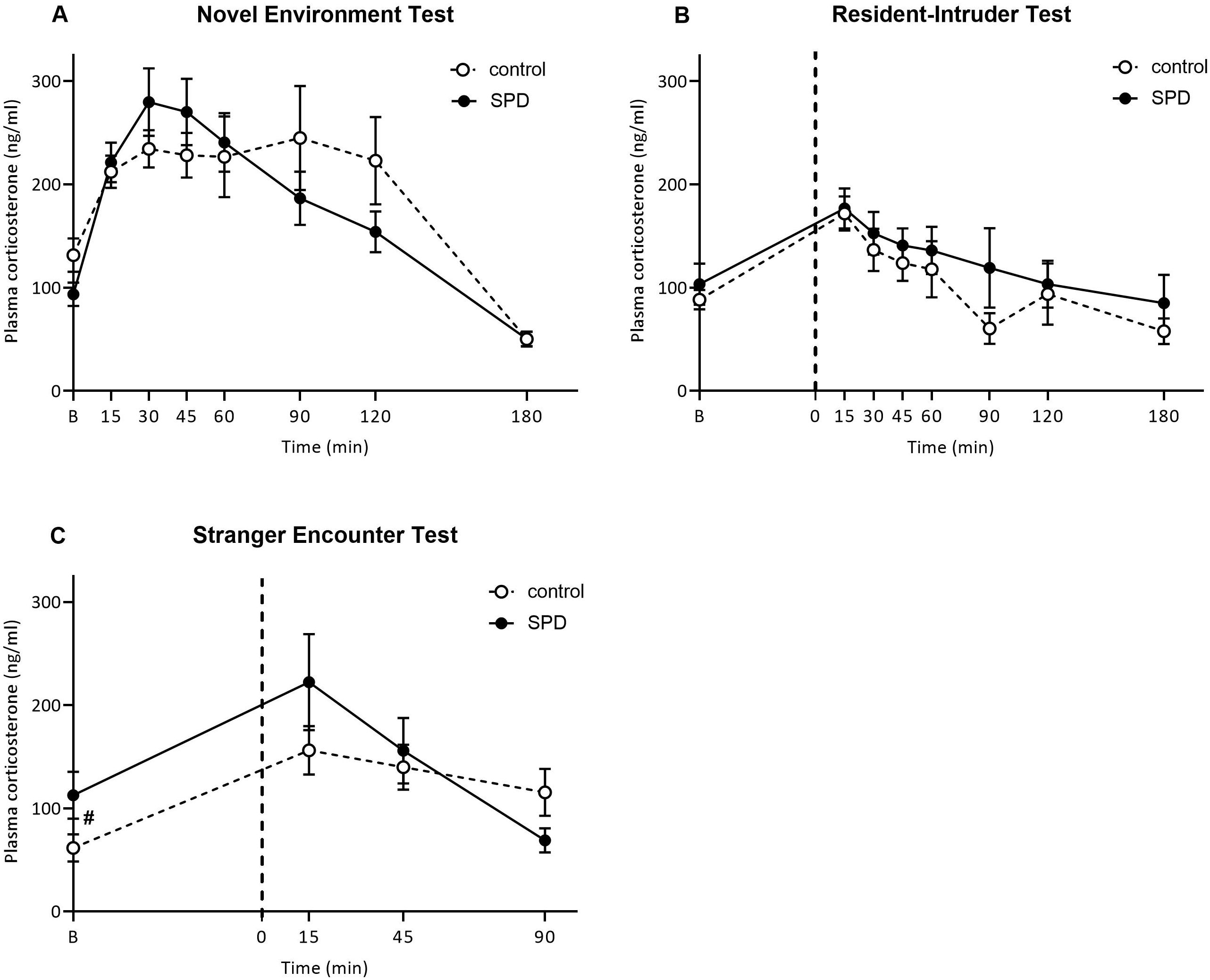
Figure 2. Plasma corticosterone concentrations (ng/ml) in response to the novel environment test (NET), resident-intruder test (RIT) and stranger encounter test (SET). (A) Plasma corticosterone at different time points relative to the novel environment stress test (NET) for control (°, N = 16) and social play deprived (SPD, deprived in the peak play period from post-natal day 21-42) (•, N = 16). Except for effects of time, no difference between control and SPD rats were found. (B) Plasma corticosterone concentrations at time points relative to the 10-minute RIT (ends on t=0) for control (°, N = 12) and SPD (•, N = 13). Except for effects of time, no differences between socially housed and SPD rats were found. (C) Plasma corticosterone concentrations at time points relative to a 15-minute SET. A trend for an interaction effect of treatment and time was found (p=0.07). SPD rats tended (p=0.06) to have higher initial corticosterone concentrations compared to control rats (°, N = 16) and SPD (•, N = 16). The dotted line indicates the start of the test. Data are presented as mean ± SEM.
Plasma corticosterone response in the modified RIT
There was an overall effect of exposure to the RIT test on plasma corticosterone concentrations over time (Ftime(7,161) = 4.57, p = 0.005; Figure 2B). The peak corticosterone concentrations for both groups were observed at 15 min after the RI (control: 171.8 ± 17.75, SPD: 176.7 ± 17.15 ng/ml). However, there was no difference between control and SPD rats in their plasma corticosterone response to the RIT (Ftreatment*time(7,161) = 0.05, p=0.99), nor was there an overall treatment effect (Ftreatment(1,23) = 0.73, p=0.40) (Figure 2B). Peak values or AUC did not differ between control and SPD rats (data not shown). For the numerical data, see Supplementary Table S2.
Plasma corticosterone response in the SET
When comparing SPD and control rats. a trend for an interaction effect emerged (Ftime*treatment(3,90) = 2.47, p = 0.07, Figure 2C) but there was no overall effect of SPD on the corticosterone response to the SET (Ftreatment(1,30) = 0.74, p = 0.40). Additionally, the corticosterone response changed over time (Ftime(3,90) = 9.30, p < 0.001). Post hoc analysis showed that SPD rats tended to have higher initial corticosterone concentrations compared to control animals (SPD vs control 60 minutes before test: p = 0.06). Both groups reached their peak corticosterone concentrations 15 minutes after the test (control: 156.19 ± 23.56, SPD: 222.28 ± 46.59 ng/ml). There were no significant differences between treatment groups on any of the timepoints after exposure to the SET (SPD vs control 15 minutes after test: p = 0.22; SPD vs control 45 minutes after test: p = 0.68; SPD vs control 90 minutes after test: p = 0.08).
Separate analyses of the two groups revealed that the corticosterone response significantly changed over time for both the control and SPD rats (Fcontrol,time(3,45) = 4.96, p = 0.005; FSPD,time(3,45) = 6.37, p = 0.001). In the control group, corticosterone concentrations increased 15 minutes after the test whereas the SPD group showed only a trend towards increased corticosterone concentrations compared to baseline (see Supplementary Table S3). Subsequently, corticosterone concentrations remained elevated in the control rats whereas they declined in the SPD rats (see Supplementary Table S3). Peak values or AUC did not differ between control and SPD rats (data not shown).
Behaviour in the modified RIT
Inherent to the resident-intruder test is the potential variation in the degree of aggressive behaviour of the resident and the degree of social behaviour displayed by the resident rat. Together these determine the degree of stress the intruder experienced during the RI test. Behaviour of the resident towards an experimental rat or the behaviour of the experimental rat towards the resident did not differ between control or SPD rats (see Supplementary Table S4 for a summary and the statistics).
Behaviour in the SET
Frequency of play behaviour
Pounces initiated by the focal rats
Comparison of initiated pouncing behaviour between the SPD and control group revealed no significant effects (Fday*treatment(2,60) = 0.20, p = 0.82; Fday(2,60) = 1.00, p = 0.38; Ftreatment(1,30) = 0.51, p = 0.48; Figure 3A).
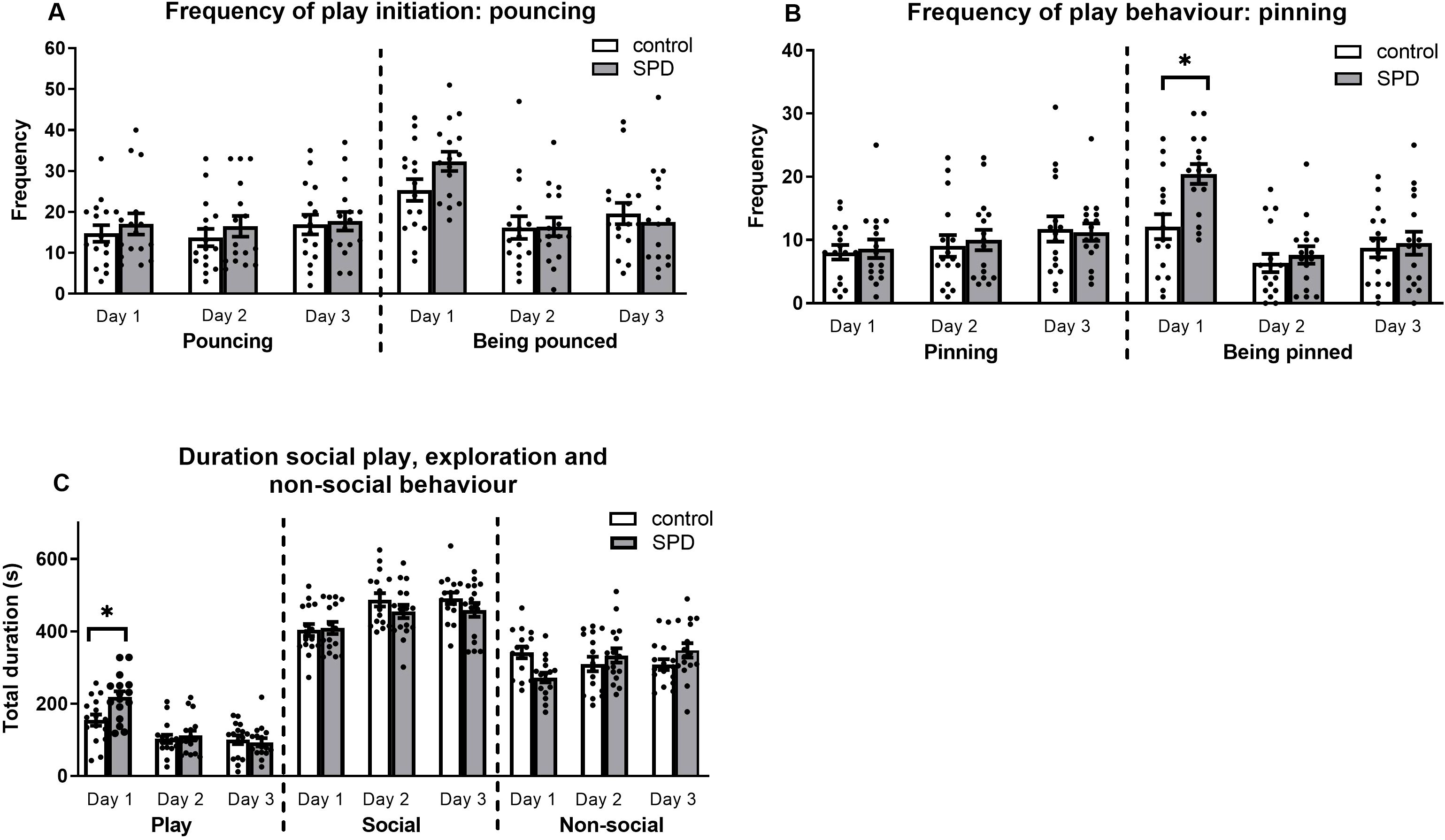
Figure 3. Frequency and duration of (social play) behaviour in the stranger encounter test (SET). (A) Frequency of play invitations initiated (pouncing) and received (being pounced) for the focal rats during the 15-minute test, with white bars representing control rats (unrestricted play behaviour during adolescence) and grey bars representing SPD rats (deprived of social play behaviour during post-natal day 21-42). No significant effects were observed for pouncing initiated by the experimental rats. For the frequency of received pounces, there was a main effect for day. The total number of received pounces was significantly higher on day 1 compared to both days 2 and 3, with no significant difference between days 2 and 3. (B) Frequency of pins performed and received (being pinned) by the focal rat. On the third test day, the experimental rats tended to pin more compared to day 1, irrespective of treatment. Additionally, SPD animals were being pinned more than controls on day 1. Both SPD and control animals received more pins on day 1 compared to day 2 whereas only SPD animals received more pins on day 1 compared to day 3. (C) Duration of social play, social and non-social exploration in seconds. SPD rats played longer than control animals on day 1 which disappeared on days 2 and 3. Regardless of treatment, less time was spent on social exploration on day 1 compared to days 2 and 3. SPD animals spent less time exploring the cage on day 1 compared to control animals but not on days 2 and 3. In addition, SPD animals spent less time exploring the cage on day 1 compared to days 2 and 3. Individual animals are represented as dots. Data are represented as mean ± SEM. *p < 0.05. **p < 0.01. Control: n = 16; SPD: n = 16.
Pounces received by the focal rats
The results did not show an interaction effect, but there was a main effect for the day of testing (Fday*treatment(2,60) = 2.11, p = 0.13; Fday(2,60) = 18.73, p < 0.001; Ftreatment(1,30) = 0.50, p = 0.48). Regardless of treatment, the total number of pounces the focal animal received was higher on day 1 compared to both days 2 and 3 (day 1 vs day 2: p < 0.001; day 1 vs day 3: p < 0.001). The frequency of received pounces did not differ between days 2 and 3 (p = 0.82).
Pins initiated by the focal rats
The data did not reveal a significant interaction between the treatment and day of testing (Fday*treatment(2,60) = 0.20, p = 0.82; Fday(2,60) = 3.65. p = 0.03; Ftreatment(1,30) = 0.04, p = 0.85; Figure 3B). However, a significant day effect emerged, indicating a trend for an increased number of pins initiated by the focal animals on day 3 compared to day 1, regardless of treatment (day 1 vs day 3: p = 0.06). No significant differences were observed between the other days (day 1 vs day 2: p = 0.92; day 2 vs day 3: p = 0.26).
Pins received by the focal rats
The data showed that, particularly on day 1, SPD rats were pinned more by the stimulus rats compared to control rats (Fday*treatment(2,60) = 5.27. p = 0.01; Fday(2,60) = 27.87, p < 0.001; Ftreatment(1,30) = 3.88, p = 0.06) (SPD day 1 vs control day 1: p = 0.002). No treatment effects were found on days 2 and 3 (SPD day 2 vs control day 2: p = 0.54; SPD day 3 vs control day 3: p = 0.75). Further post hoc analysis showed that in both treatment groups, the rats received a significantly higher number of pins on day 1 compared to day 2 (FSPD, day(2,30) = 27.84, p < 0.001; Fcontrol, day(2.30) = 4.99, p = 0.01) (SPD day 1 vs SPD day 2: p < 0.001; control day 1 vs control day 2: p = 0.02). SPD animals were pinned more frequently on day 1 compared to day 3 (SPD day 1 vs SPD day 3: p < 0.001) but this effect was not observed in the control group (control day 1 vs control day 3: p = 0.30). Results of day 2 did not differ from those of day 3 for either play-deprived or control group (SPD day 2 vs SPD day 3: p = 0.99; control day 2 vs control day 3: p = 0.56).
Duration of play behaviour, social exploration and non-social behaviour
Duration of play behaviour
The total amount of time spent playing between SPD and control rats over the three test days revealed an interaction effect, i.e. the animals spent different amounts of time playing over days depending on their housing condition during adolescence (Fday*treatment(2,60) = 5.42, p = 0.007; Fday(2,60) = 37.93, p < 0.001; Ftreatment(130) = 2.50, p = 0.13) (Figure 3C). Post hoc tests demonstrated that the time both SPD and control rats engaged in play varied across days (FSPD,day(2,30) = 33.73, p < 0.001; Fcontrol,day(2,30) = 7.94, p = 0.002). Specifically, both SPD and control rats played longer on day 1 compared to days 2 and 3 (SPD day 1 vs SPD day 2: p < 0.001; SPD day 1 vs SPD day 3: p < 0.001; control day 1 vs control day 2: p = 0.008; control day 1 vs control day 3 p < 0.02). However, no statistical differences were observed between the results for days 2 and 3 for both treatment groups (SPD day 2 vs SPD day 3: p = 0.74; control day 2 vs control day 3: p = 1.00). In addition, on day 1, SPD animals played longer compared to control animals (SPD day 1 vs control day 1: p = 0.008). However, SPD did not influence the duration of total play behaviour on days 2 and 3 (SPD day 2 vs control day 2: p = 0.62; SPD day 3 vs control day 3: p = 0.67).
Duration of social exploration
Concerning the time spent on social exploration, no interaction was observed between the day of testing and the housing condition during adolescence (Fday*treatment(2,60) = 1.23, p = 0.30; Fday(2,60) = 15.11. p < 0.001; Ftreatment(1,30) = 1.07, p = 0.31). However, there was a main effect of the day of testing. Post hoc analysis revealed that the duration of social exploration on day 1 was shorter compared to day 2 and 3 (day 1 vs day 2: p < 0.001; day 1 vs day 3: p < 0.001). No significant differences were observed between the amount of time spent on social exploration on day 2 compared to day 3 (day 2 vs day 3: p = 1.00).
Duration of non-social behaviour
The amount of time spent on non-social behaviour revealed an interaction effect for day and treatment group (Fday*treatment(2,60) = 7.95, p < 0.001; Fday(2,60) = 1.06, p = 0.35; Ftreatment(1,30) = 0.02, p = 0.90). SPD rats spent less time exploring the cage, walking around or interacting with bedding compared to control rats on day 1 (SPD day 1 vs control day 1: p = 0.002). On days 2 and 3, control and SPD rats spent equal amounts of time exploring the cage (SPD day 2 vs control day 2: p = 0.41; SPD day 3 vs control day 3: p = 0.13). Further post hoc testing showed that the interaction effect was caused by the behaviour of the SPD rats, not the control rats (FSPD,day(2,30) = 10.17, p < 0.001; Fcontrol,day(2,30) = 1.29, p = 0.29). Specifically on day 1, SPD rats spent less time on non-social behaviours compared to days 2 and 3 (SPD day 1 vs SPD day 2: p = 0.009; SPD day 1 vs SPD day 3: p < 0.001). However, no difference was found when comparing days 2 and 3 (SPD day 2 vs SPD day 3: p = 1.00).
Discussion
Emerging evidence supports the notion that a lack of social play in early life can lead to impairments in stress resilience and social interaction later in life. In this study, we hypothesised that an atypical development of the HPA axis contributes to these impairments in adulthood. To test this hypothesis, we examined the effects of SPD in juvenile rats on plasma corticosterone concentrations in adulthood when subjected to three potentially stressful tests; NET, modified RIT and SET. These tests reflect aspects of challenges that male rats may encounter in the wild, i.e. entering a novel environment when leaving the home territory, being confronted with territorial aggression from an unfamiliar conspecific and encountering strangers in a newly established territory. Plasma corticosterone concentrations were not significantly different between SPD and control rats in response to a NET or the modified RIT, nor were there behavioural differences between SPD and control rats in the RIT. Prior to the SET, SPD rats tended to have higher initial corticosterone concentrations compared to control rats, yet the corticosterone concentrations in response to the SET were comparable between the groups. Interestingly, in the SET, we found a difference in the degree SPD rats are played with by unfamiliar conspecifics, especially in the first encounter.
Corticosterone concentrations in response to stressors are not markedly altered by play deprivation during the play peak
The SPD and control rats did not differ in their plasma corticosterone curves in response to a NET or the modified RIT whereas SPD animals showed a trend approaching significance towards an elevated baseline level of corticosterone in the SET. Peak concentrations and AUC did not differ between control and SPD animals in any test. The lack of overall differences in responsivity to these environmental (social) challenges between control and SPD rats is in line with previous findings. For example, SPD rats showed a similar plasma corticosterone response to restraint stress when compared to undeprived rats (Lukkes et al., 2009). However, in the study by Van den Berg et al. (1999), SPD rats showed prolonged higher corticosterone concentrations in response to a RIT compared to undeprived rats.
The residents in the current experiment were not housed with a female and therefore showed a milder behavioural repertoire towards the intruder. Indeed, no differences in any of the behavioural measures were observed between SPD and control rats in this test. A limitation of the current study is that the residents did not always respond similarly towards intruders. Indeed, no fights occurred for 3 out of 16 SPD rats and 4 out 16 control rats. These results were not included in the corticosterone analysis and analysed separately because it provides a different experience for the experimental animals (see Supplementary Data S5). Indeed, as shown in Supplementary Data S5, non-fighting rats showed lower overall corticosterone concentrations compared to the rats that did encounter aggression (Figure 1B) with no difference between control and SPD rats.
For the current study, relatively milder stressors were used when compared to previously published reports on the impact of SPD on stress responsivity. This relatively milder stress approach was chosen to investigate the impact of SPD on the responsivity to more conflicting (social) environmental challenges compared to more severe stressful conditions (Lukkes et al., 2009; Van den Berg et al., 1999). The current data suggest that the stress response is comparable for SPD and control rats in these environmental challenges.
Taken together, these findings suggest that SPD has little impact on the stress response in conflicting (social) environmental challenges, in contrast to previously reported altered responses to severely stressful experiences. However, the discrepancy between the current study and previous findings can be attributed to the methodology to induce SPD. Whereas Lukkes et al. (2009) and Van den Berg et al. (1999) used isolation housing, we placed a divider in the home cage that prevented the rats from engaging in physical play but allowed them to see, hear and smell each other. It is likely that the total lack of social contact during early development may impact stress responsivity whereas ‘merely’ limiting social play whilst maintaining other means of social contact does not affect the corticosterone response to stress to a similar degree.
Subtle effects in the behavioural response of SPD animals in the SET
In this study, we introduced a novel social stress situation (the SET) that could be intimidating but not necessarily aversive. For that purpose, rats were confronted in a familiar environment, with two unfamiliar rats that were socially housed and cage-mates. This was done to simulate a context where rats establish a new territory in which they encounter unfamiliar individuals. This social context induced an increase in corticosterone concentrations, suggesting that this test may indeed reflect a social stressor.
Although there was no difference between SPD and control rats in the corticosterone concentrations after exposure to the SET, there was more play directed towards SPD rats than to the non-deprived control rats in this context. This effect was only present on the first day of testing and specifically for the number of pins received by the SPD animals. Interestingly, there was no difference in play initiation by the SPD or control rats. It remains to be investigated why the stranger rats played more with the SPD rats compared to the control rats. A possible explanation is that SPD rats emit (ultrasonic) vocal or behavioural signals that promote play. In addition, because these rats lack three weeks of play experience and are housed with similar treated animals after the SPD period, these animals may respond differently than the stranger rats. Indeed, while juvenile male rats respond to a pounce with a complete rotation (a pin), from puberty onwards they respond also with a partial rotation (Pellis and Pellis, 1990; Pellis et al., 1992). It could be that in the first test SPD rats respond with a more juvenile approach by rolling into the pin position, whereas in the subsequent tests they alter their response to a pounce. To gain insight into what is underlying the differences in play behaviour on test-day 1 compared to test-day 2 and 3, a microstructural analysis of the different responses to a pounce (Stark, 2021; Pellis et al., 2022) could be compared between control- and SPD rats. In addition, the behaviour of the individual stranger rats towards the experimental rat as well as towards one another could be further analysed. Important in this respect is the limitation that these observations represent male rats only. Female rats do not change their pattern of play behaviour with age to the same degree (Pellis and Pellis, 1990), which could provide an additional clue for the behavioural differences observed. However, this is beyond the scope of the current study.
Conclusion
SPD rats exposed to relatively mild stressors did not respond differently in terms of corticosterone concentrations. Behaviourally, the SPD rats responded similarly to the undeprived rats in a modified RIT. More play behaviour was directed towards them upon a first exposure to two unfamiliar stranger rats in a SET. These results highlight the importance of play behaviour for social behaviour in specific situations in adulthood, independent of corticosterone concentrations.
Data availability statement
The raw data supporting the conclusions of this article will be made available by the authors upon request.
Ethics statement
The animal study was approved by Animal Ethics Committee of Utrecht University. The study was conducted in accordance with the local legislation and institutional requirements.
Author contributions
EA: Conceptualization, Formal analysis, Investigation, Methodology, Supervision, Writing – original draft, Writing – review & editing. JK: Methodology, Supervision, Writing – review & editing. TP: Formal analysis, Investigation, Methodology, Writing – original draft. AA: Investigation, Methodology, Writing – review & editing. JH: Formal analysis, Methodology, Validation, Writing – review & editing. SN: Conceptualization, Funding acquisition, Supervision, Writing – original draft, Writing – review & editing. CE: Conceptualization, Funding acquisition, Supervision, Writing – original draft, Writing – review & editing. HL: Conceptualization, Formal analysis, Funding acquisition, Investigation, Methodology, Project administration, Resources, Supervision, Visualization, Writing – original draft, Writing – review & editing.
Funding
The author(s) declare financial support was received for the research, authorship, and/or publication of this article. This study was funded by Dynamics of Youth UU.
Acknowledgments
We would like to thank Susanne Kirchhoff for technical assistance with the RIA and ELISA.
Conflict of interest
The authors declare that the research was conducted in the absence of any commercial or financial relationships that could be construed as a potential conflict of interest.
Publisher’s note
All claims expressed in this article are solely those of the authors and do not necessarily represent those of their affiliated organizations, or those of the publisher, the editors and the reviewers. Any product that may be evaluated in this article, or claim that may be made by its manufacturer, is not guaranteed or endorsed by the publisher.
Supplementary material
The Supplementary Material for this article can be found online at: https://www.frontiersin.org/articles/10.3389/fetho.2024.1458136/full#supplementary-material
References
Achterberg E. J. M., Trezza V., Siviy S. M., Schrama L., Schoffelmeer A. N. M., Vanderschuren L. J. M. J. (2014). Amphetamine and cocaine suppress social play behavior in rats through distinct mechanisms. Psychopharmacology 231, 1503–1515. doi: 10.1007/s00213-013-3272-9
Achterberg E. J. M., Vanderschuren L. J. M. J. (2023). The neurobiology of social play behaviour: Past, present and future. Neurosci. Biobehav. Rev. 152, 105319. doi: 10.1016/j.neubiorev.2023.105319
Baarendse P. J., Counotte D. S., O’Donnell P., Vanderschuren L. J. M. J. (2013). Early social experience is critical for the development of cognitive control and dopamine modulation of prefrontal cortex function. Neuropsychopharmacology 38, 1485–1494. doi: 10.1038/npp.2013.47
Bijlsma A., Birza E. E., Pimentel T. C., Maranus J. P., van Gaans M. J., Lozeman-van t Klooster J. G., et al. (2024). Opportunities for risk-taking during play alters cognitive performance and prefrontal inhibitory signalling in rats of both sexes. Eur. J. Neurosci. 59 (10), 2748–2765. doi: 10.1111/ejn.16313
Bijlsma A., Omrani A., Spoelder M., Verharen J. P. H., Bauer L., Cornelis C., et al. (2022). Social play behavior is critical for the development of prefrontal inhibitory synapses and cognitive flexibility in rats. J. Neurosci. 42, 8716–8728. doi: 10.1523/JNEUROSCI.0524-22.2022
Brown G. M., Uhlir I. V., Seggie J., Schally A. V., Kastin A. J. (1974). Effect of septal lesions on plasma levels of MSH, corticosterone, GH and prolactin before and after exposure to novel environment: Role of MSH in the septal syndrome. Endocrinology 94, 593–597. doi: 10.1210/endo-94-2-583
File S. E., Peet L. A. (1980). The sensitivity of the rat corticosterone response to environmental manipulations and to chronic chlordiazepoxide treatment. Physiol. Behav. 25, 753–758. doi: 10.1016/0031-9384(80)90379-0
Fluttert M., Dalm S., Oitzl M. S. (2000). A refined method for sequential blood sampling by tail incision in rats. Lab. Anim. 34, 372–378. doi: 10.1258/002367700780387714
Gardner-Santana L. C., Norris D. E., Fornadel C. M., Hinson E. R., Klein S. L., Glass G. E. (2009). Commensal ecology, urban landscapes, and their influence on the genetic characteristics of city-dwelling Norway rats (Rattus norvegicus). Mol. Ecol. 18, 2766–2778. doi: 10.1111/j.1365-294X.2009.04232.x
Gatto-Almeida F., de Araújo Soares A., Degrandi T. M., Tiepolo L. M., Pichmueller F., Hass I. (2022). Assessment of dispersal and population structure of Norway rats (Rattus norvegicus) in a seaport setting. Urban Ecosyst. 25, 535–544. doi: 10.1007/s11252-021-01171-x
Ginsburg K. R., American Academy of Pediatrics Committee on, C, American Academy of Pediatrics Committee on Psychosocial Aspects of, C. & Family, H (2007). The importance of play in promoting healthy child development and maintaining strong parent-child bonds. Pediatrics 119, 182–191. doi: 10.1542/peds.2006-2697
Graham K. L., Burghardt G. M. (2010). Current perspectives on the biological study of play signs of progress. Q. Rev. Biol. 85, 393–418. doi: 10.1086/656903
Greenwood P. J. (1980). Mating systems, philopatry and dispersal in birds and mammals. Anim. Behav. 28, 1140–1162. doi: 10.1016/S0003-3472(80)80103-5
Harrison T., Harrison D. (2013). Does a nurturing approach that uses an outdoor play environment build resilience in children from a challenging background? J. Adventure Educ. Outdoor Learn. 13, 238–254. doi: 10.1080/14729679.2013.776862
Hol T., Van den Berg C. L., Van Ree J. M., Spruijt B. M. (1999). Isolation during the play period in infancy decreases adult social interactions in rats. Behav. Brain Res. 100, 91–97. doi: 10.1016/S0166-4328(98)00116-8
Koolhaas J. M., Coppens C. M., de Boer S. F., Buwalda B., Meerlo P., Timmermans P. J. A. (2013). The resident-intruder paradigm: a standardized test for aggression, violence and social stress. J. visualrd experiments: JoVE (77), e4367. doi: 10.3791/4367
Koolhaas J. M., Schuurman T., Wiepkema P. R. (1980). The organization of intraspecific agonistic behaviour in the rat. Prog. Neurobiol. 15, 247–268. doi: 10.1016/0301-0082(80)90024-6
Lillard A. S. (2017). Why do the children (Pretend) play? Trends Cogn. Sci. 21 (11), 826–834. doi: 10.1016/j.tics.2017.08.001
Lukkes J. L., Mokin M. V., Scholl J. L., Forster G. L. (2009). Adult rats exposed to early-life social isolation exhibit increased anxiety and conditioned fear behavior, and altered hormonal stress responses. Hormones Behav. 55, 248–256. doi: 10.1016/j.yhbeh.2008.10.014
Marquardt A. E., VanRyzin J. W., Fuquen R. W., McCarthy M. M. (2023). Social play experience in juvenile rats is indispensable for appropriate socio-sexual behavior in adulthood in males but not females. Front. Behav. Neurosci. 16. doi: 10.3389/fnbeh.2022.1076765
Marques J. M., Olsson A. S., Ogren S. O., Dahlborn K. (2008). Evaluation of exploration and risk assessment in pre-weaning mice using the novel cage test. Physiol. Behav. 93, 139–147. doi: 10.1016/j.physbeh.2007.08.006
Martinez W., Carter J. S., Legato L. J. (2011). Social competence in children with chronic illness: a meta-analytic review. J. Pediatr. Psychol. 36, 878–890. doi: 10.1093/jpepsy/jsr035
Maurice-Stam H., Nijhof S. L., Monninkhof A. S., Heymans H. S. A., Grootenhuis M. A. (2019). Review about the impact of growing up with a chronic disease showed delays achieving psychosocial milestones. Acta Paediatr. 108, 2157–2169. doi: 10.1111/apa.14918
McCormick C. M., Smith C., Mathews I. Z. (2008). Effects of chronic social stress in adolescence on anxiety and neuroendocrine response to mild stress in male and female rats. Behav. Brain Res. 187, 228–238. doi: 10.1016/j.bbr.2007.09.005
Meaney M. J., Stewart J. (1983). The influence of exogenous testosterone and corticosterone on the social behavior of prepubertal male rats. Bull. Psycho. Soc. 21, 232–234. doi: 10.3758/BF03334695
Meaney M. J., Stewart J., Beatty W. W. (1982). The influence of glucocorticoids during the neonatal period on the development of play-fighting in Norway rat pups. Horm. Behav. 16, 475–491. doi: 10.1016/0018-506x(82)90054-x
Munambah N., Cordier R., Speyer R., Toto S., Ramugondo E. L. A. (2020). Systematic review comparing the play profiles of children with special health care needs with typically developing children. BioMed. Res. Int., 9582795. doi: 10.1155/2020/9582795
Nijhof S. L., Vinkers C. H., van Geelen S. M., Duijff S. N., Achterberg E. J. M., van der Net J., et al. (2018). Healthy play, better coping: The importance of play for the development of children in health and disease. Neurosci. Biobehav. Rev. 95, 421–429. doi: 10.1016/j.neubiorev.2018.09.024
Panksepp J. (1981). The ontogeny of play in rats. Dev. Psychobiol. 14, 327–332. doi: 10.1002/dev.420140405
Panksepp J., Beatty W. W. (1980). Social deprivation and play in rats. Behav. Neural Biol. 30, 197–206. doi: 10.1016/S0163-1047(80)91077-8
Peartree N. A., Hood L. E., Thiel K. J., Sanabria F., Pentkowski N. S., Chandler K. N., et al. (2012). Limited physical contact through a mesh barrier is sufficient for social reward-conditioned place preference in adolescent male rats. Physiol. Behav. 105, 749–756. doi: 10.1016/j.physbeh.2011.10.001
Pellis S. M., Pellis V. C. (1990). Differential rates of attack, defense and counterattack during the developmental decrease in play fighting by male and female rats. Dev. Psychobiol. 23, 215–231. doi: 10.1002/dev.420230303
Pellis S. M., Pellis V. C. (2009). The playful brain: venturing to the limits of neuroscience (Oxford: Oneworld).
Pellis S. M., Pellis V. C., Burke C. J., Stark R. A., Ham J. R., Euston D. R., et al. (2022). Measuring play fighting in rats: A multilayered approach. Curr. Protoc. 2, e337. doi: 10.1002/cpz1.337
Pellis S. M., Pellis V. C., Whishaw I. Q. (1992). The role of the cortex in play fighting by rats: Developmental and evolutionary implications. Brain Behav. Evol. 39, 270–284. doi: 10.1159/000114124
Pinquart M. (2017). Systematic review: bullying involvement of children with and without chronic physical illness and/or physical/sensory disability-a meta-analytic comparison with healthy/nondisabled peers. J. Pediatr. Psychol. 42, 245–259. doi: 10.1093/jpepsy/jsw081
Schulte F., Barrera M. (2010). Social competence in childhood brain tumor survivors: a comprehensive review. Support Care Cancer 18, 1499–1513. doi: 10.1007/s00520-010-0963-1
Špinka M., Newberry R. C., Bekoff M. (2001). Mammalian play: training for the unexpected. Q Rev. Biol. 76, 141–168. doi: 10.1086/393866
Stark R. A. (2021). The role of play-derived experiences on the development of the medial prefrontal cortex and adult social behavior (Order No. 28768877). Canada: Dissertations & Theses @ University of Lethbridge; ProQuest Dissertations & Theses A&I. 2587788441.
Tremblay M. S., LeBlanc A. G., Kho M. E., Saunders T. J., Larouche R., Colley R. C., et al. (2011). Systematic review of sedentary behaviour and health indicators in school-aged children and youth. Int. J. Behav. Nutr. Phys. activity 8, 98. doi: 10.1186/1479-5868-8-98
Tremblay M. S., Gray C., Babcock S., Barnes J., Bradsheet C. C., Carr D., et al. (2015). Position statement on active outdoor play. Int. J. Environ. Res. Public Health 12, 6475. doi: 10.3390/ijerph120606475
Van den Berg C. L., Hol T., van Ree J. M., Spruijt B. M., Everts H., Koolhaas J. M. (1999). Play is indispensable for an adequate development of coping with social challenges in the rat. Dev. psychobiol. 34, 129–138. doi: 10.1002/(SICI)1098-2302(199903)34:2<129::AID-DEV6>3.0.CO;2-L
Vanderschuren L. J. M. J., Trezza V. (2014). What the laboratory rat has taught us about social play behavior: role in behavioral development and neural mechanisms. Curr. topics Behav. Neurosci. 16, 189–212. doi: 10.1007/7854_2013_268
Veenit V., Cordero M. I., Tzanoulinou S., Sandi C. (2013). Increased corticosterone in peripubertal rats leads to long-lasting alterations in social exploration and aggression. Front. Behav. Neurosci. 7. doi: 10.3389/fnbeh.2013.00026
Von Frijtag J. C., Schot M., van den Bos R., Spruijt B. M. (2002). Individual housing during the play period results in changed responses to and consequences of a psychosocial stress situation in rats. Dev. psychobiol. 41, 58–69. doi: 10.1002/dev.10057
Keywords: social play, deprivation, stress response, resilience, social behaviour
Citation: Achterberg EJM, Lozeman-van ‘t Klooster JG, Pimentel TC, Aleksić A, Hendriks JCMJ, Nijhof S, van der Ent CK and Lesscher HMB (2024) Effect of social play deprivation on stress sensitivity in response to social and non-social challenges. Front. Ethol. 3:1458136. doi: 10.3389/fetho.2024.1458136
Received: 01 July 2024; Accepted: 18 November 2024;
Published: 13 December 2024.
Edited by:
Christine Drea, Duke University, United StatesReviewed by:
Scott Nunes, University of San Francisco, United StatesWojciech Pisula, Polish Academy of Sciences, Poland
Copyright © 2024 Achterberg, Lozeman-van ‘t Klooster, Pimentel, Aleksić, Hendriks, Nijhof, van der Ent and Lesscher. This is an open-access article distributed under the terms of the Creative Commons Attribution License (CC BY). The use, distribution or reproduction in other forums is permitted, provided the original author(s) and the copyright owner(s) are credited and that the original publication in this journal is cited, in accordance with accepted academic practice. No use, distribution or reproduction is permitted which does not comply with these terms.
*Correspondence: Heidi M. B. Lesscher, SC5NLkIuTGVzc2NoZXJAdXUubmw=