- 1Muséum National d’Histoire Naturelle, Institut de Systématique, Évolution, Biodiversité, UMR 7205 Muséum National d’Histoire Naturelle, CNRS, Sorbonne Université, EPHE, Université des Antilles, Paris, France
- 2CNRS, Station d’Ecologie Théorique et Expérimentale, UAR 2029, Moulis, France
- 3Earth and Life Institute, UCLouvain, Louvain-la-Neuve, Belgium
Shorebirds are iconic examples of food resource partitioning through use of contrasted morphological structures to acquire food. Differences in beak lengths and shapes allow species catching their food at various sediment depths. Contrasted leg lengths allow species foraging at distinct water depths. Despite these morphological differences, shorebirds use a small number of stereotyped behaviors for food acquisition. We classify these behaviors by analyzing video sequences of ca. two dozen species of Western Palearctic shorebirds, during migration or wintering. We suggest disassembling food acquisition in three successive stages: foraging, feeding, and swallowing. The foraging stage regroups the locomotion behaviors associated to food detection, and the behaviors used during food capture. The feeding stage encompasses the handling behaviors used to kill or stun the prey and to extract its edible parts, and the behaviors used to transport the prey from the distal part of the beak to the bird’s pharynx. In the swallowing stage, the edible parts of the prey enter the pharynx. We show that three of these behaviors (locomotion, capture and transport) are made up of stereotypical, mutually exclusive components, and can be considered as performances. Each of our study species use one or maximum two components of these three performances. Overall, our study provides insights on interspecific variation in shorebird food acquisition behaviors that we put in a phylogenetic perspective. We confirm the long-standing hypothesis that pecking is the plesiomorphic behavior of food capture, and we show that those locomotion and transport behaviors associated with pecking differ from those associated with derived capture behaviors, leading to a syndrome of food acquisition behaviors in shorebirds.
1 Introduction
Shorebirds are iconic examples of the partitioning of food resources through use of contrasted morphological structures (e.g., bills, legs) to capture prey. Shorebirds forage mainly on the land-water borders of fresh, brackish, or marine waters where they feed mostly on invertebrates (Colwell, 2010), but vegetal material are also ingested by many species, and can constitute a predominant part of their diet during certain periods of the year (e.g., in Calidris pugnax, Cramp and Simmons, 1983). Differences in bill lengths provide shorebird species with the opportunity to catch food particles at various sediment depths. Similarly, contrasted leg lengths allow species to forage at distinct water depths. Despite these morphological differences, shorebirds appear to use a small number of stereotyped food acquisition behaviors (e.g., Burton, 1974; Hamilton, 1975). A seminal interspecific comparative study introduced a classification of these foraging-feeding species-specific stereotyped behaviors (Barbosa, 1993; Barbosa and Moreno, 1999). This classification was subsequently used in numerous studies investigating the diet or the food acquisition behaviors of shorebirds (e.g., Nebel et al., 2005; Thomas et al., 2006; Jing et al., 2007; Piersma and van Gils, 2011; Nol et al., 2014; Novcic, 2016; Mathot et al., 2019; Novcic, 2019; Angarita-Baez and Carlos, 2023).
Here we propose to revisit this classification, by defining a functional and integrative analysis of behaviors associated with food acquisition in shorebirds. More precisely, based on our filmed observations, food acquisition can be divided in three successive stages: foraging, feeding, and swallowing. The foraging stage concerns the different behaviors associated to food detection and capture: locomotion behaviors (how do birds move in suitable foraging habitats) and capture behaviors (how do birds locate and catch food particles. The feeding stage encompasses the behaviors used to possibly stun or kill the food particle: handling behaviors (how do birds manipulate the food particle to extract edible parts) and transport behaviors (how do birds bring it to the pharynx). In the swallowing stage, the edible part of the food particle enters the digestive tractus. By doing this deconstruction of food acquisition, we assume that the evolution of morphological structures associated with the partitioning of food resources in shorebirds may covariate with the selection of behaviors specific to each of these three stages, as predicted by the concept of neuroethological morphology (Bels et al., 2022). This concept integrates ethology (including behavioral ecology and neuroethology already classically associated), with functional morphology (including biomechanics and physics). This interdisciplinary concept can help understand the characteristics of all behaviors of an individual that are capable of initiating, modulating or integrating modal action patterns (Barlow, 1977) to respond to the environment and communicate.
This association is rather obvious for capture behaviors: the pecking behavior of a golden plover (Pluvialis apricaria) that hunts prey by sight on the surface of the substrate is very different from the probing behavior of a long-billed snipe (Gallinago gallinago) that locates its prey by touch deep into the sediments (Burton, 1974). But our hypothesis is that the two following stages of food acquisition can also be affected by the structure of the beak: the opening of the valves of shellfish by oystercatchers (Haematopus ostralegus) and the transport of their flesh out of the shell towards their buccal cavities all along their long, robust beaks (Hulscher, 1996) engenders constraints that are very different from those faced by red phalaropes (Phaloropus fulicarius) handling very small preys that birds enclose in drops of water to move them along their narrow beaks to their buccal cavities (Rubega, 1997; Prakash et al., 2008). The solidly bony structure of the decurved bills of curlews obliterates the lumen for much of its length and brings about great reduction in relative size of the tongue (Burton, 1974; Burton, 1986). The bird is obliged to remove a worm from the mud before it can transport it back to the mouth and swallow it; this is achieved by head jerking, coordinated with opening and shutting of the jaws (Burton, 1974; Burton, 1986). By contrast, snipes (Gallinago gallinago) and woodcocks (Scolopax rusticola), which have the lumen open and long tongues, are able to transport a worm along the bill while it is still inserted in the substrate, by a combination of a rapid series of retractions of the rhynchokinetic upper jaw tip and tongue action (Burton, 1974; Burton, 1986). Godwits (Limosa spp.), although showing some bill reinforcement and tongue reduction, are often able to do the same (Burton, 1974; Burton, 1986).
Here we approach in a more detailed way the stereotyped behaviors involved in each of the three stages of food acquisition, by analyzing video sequences of ca. two dozen species of Western Palearctic shorebirds, during migration or wintering. This procedure makes it possible to standardize observations of stereotyped behaviors, and to summarize them in a comprehensive repertoire that is as complete as possible. In addition to introducing three stages in the study of shorebird food acquisition, we will revisit the existing state of the art. Descriptions of several stereotyped behaviors involved in the foraging stage in shorebirds do exist in the literature (e.g., Burton, 1974; Hamilton, 1975), and Barbosa and Moreno (1999) provided the outline of a first classification recently rearranged by Angarita-Baez and Carlos (2023). Whereas we do not doubt the heuristic importance of these descriptions and classification, here we want to reassess and standardize the foraging stage by using our video analysis method. Besides, we introduce descriptions of the feeding and the swallowing stages that are not explicitly addressed in the literature (but see Rubega and Obst, 1993; Rubega, 1997; Estrella et al., 2007; Prakash et al., 2008; Angarita-Baez and Carlos, 2023 and especially Bels et al., 2023 for behaviors associated to food transport from the beak to the pharynx).
Overall, our study provides insights on the interspecific variations and on the evolution of food acquisition behaviors in shorebirds. We look for stereotyped behaviors for each species during each of the three stages of food acquisition and investigate the relationships between these behaviors. We also provide a glimpse on the development of these behaviors, both by putting these relationships into a phylogenetic context using the dated phylogeny of Cerny and Natale (2022) and by exploring the ontogeny of these behaviors.
2 Methods
2.1 Behavioral data collection
Shorebird food acquisition behaviors were opportunistically recorded during field trips in a variety of locations in Western and Southern Europe between 2021 and 2023. Data were collected as follows: observers avoiding bird disturbance were positioned at distances ranging from 5 m (in cars or hides) to 60 m, and filmed using two cameras: either an Olympus OMD-EM1x with a M.Zuiko Digital ED 150-400 mm F4.5 lens, which provides magnification up to 40 times, or a Nikon RX 100 with a Tamron 600 150-500mm F/5.6-6.3 lens, with a magnification up to 10 times. Focal individuals (Altmann, 1974) were selected (among flocks when necessary) and filmed during at least 1 minute at speed of 25fps, 60fps or 120fps. Species identity, recording location, and number of individuals are shown in Table 1. Altogether, we gathered data on 26 species belonging to two of the three suborders of Charadriiformes: 18 Scolopaci and 8 Charadri species according to Cerny and Natale (2022), which represents approx. 17% and 8% of the total number of species in each suborder, respectively (e.g. Billerman et al., 2022).
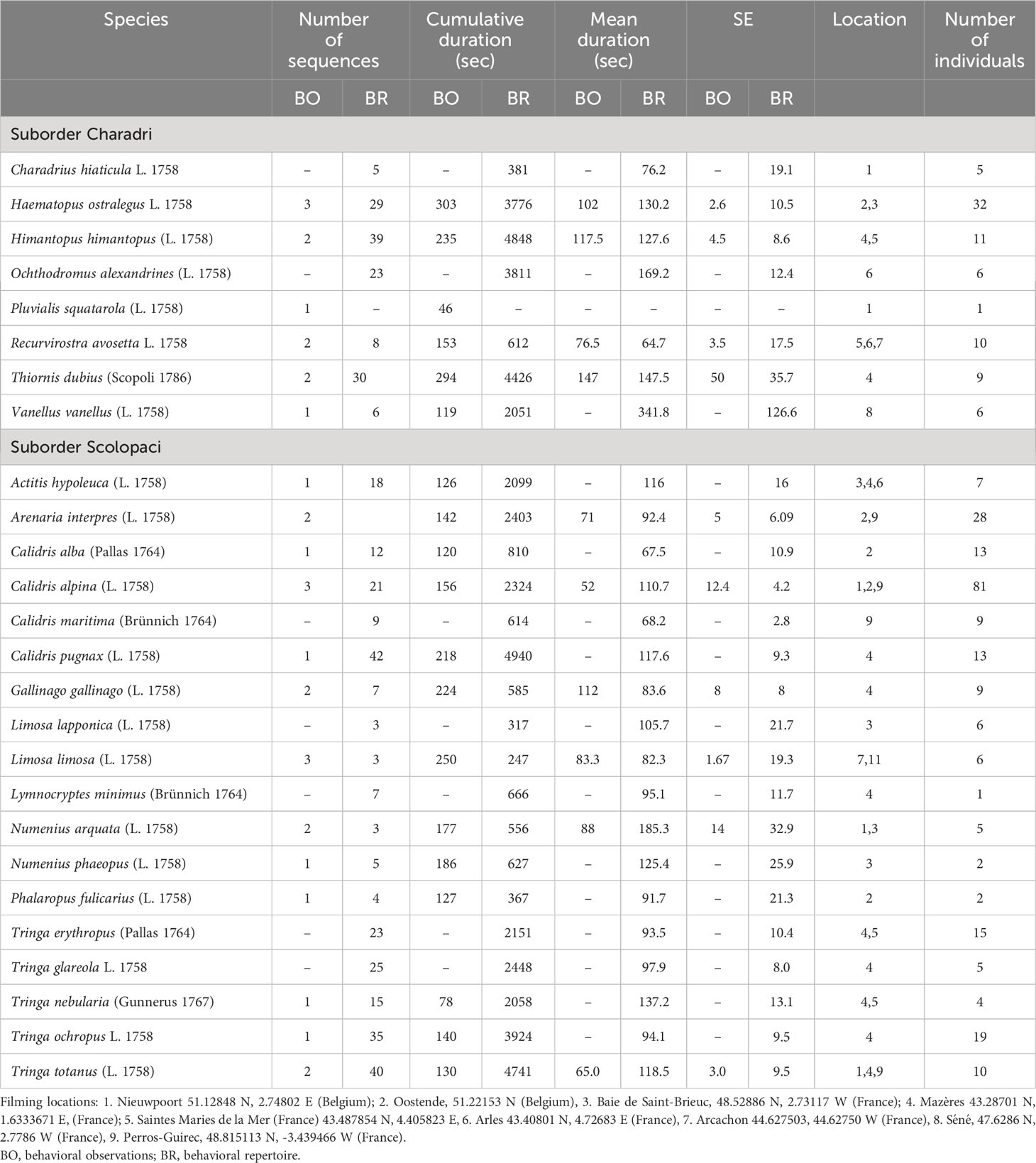
Table 1 Descriptive statistics of the video records on which the behavioral repertoire is built, and on which the overview of food acquisition behaviors of 26 Western Palearctic shorebird species is based.
2.2 Phylogenetic tree
The phylogenetic relationships between the 26 shorebird species of our data set were drawn from the consensus tree of Cerny and Natale (2022). We privilege this phylogenetic hypothesis among others because (1) it is the most comprehensive non-supertree phylogeny of shorebirds to date, (2) it is based on a total-evidence approach, and (3) its dataset comprises 90% of all extant or recently extinct species. Regarding total evidence, this phylogeny is inferred from 27 genes and 69 morphological characters, and time-scaled using 14 vetted fossil calibrations. The time-calibrated consensus tree of Cerny and Natale (2022) was pruned to our 26 study species using iTOL (Letunic and Bork, 2021).
2.3 Behavioral data analyses
In a first step, we watched five video sequences of at least one minute on every species of our sample. We selected five video sequences that were recorded at different places and over different time periods, to cover as far as possible the potential species-specific diversity in food acquisition behaviors. These criteria were relaxed for species that were under-represented in our data set because of their rarity in the study area, or their lack of occurrence during our field trips. This first step allowed us to establish that it was possible to divide food acquisition behaviors into three stages: foraging, feeding, and swallowing as indicated above. Then we looked for stereotyped behaviors associated to each of these stages that were repeated from one video to another, on the same individual, from one conspecific individual to another, and between individuals of different species. The work of Barbosa and Moreno (1999) served as a general inspiration for defining those stereotypical behaviors, but we developed our own determination criteria detailed below. We looked for stereotyped behaviors by accepting some variations on the same theme, particularly during interspecific comparisons. Then we used BORIS (Behavioral Observation Research Interactive Software, Friard and Gamba, 2016) on a subset of our data set (19 species, Table 1). This subset of 19 species was chosen because there covered the full range of stereotyped behaviors we observed. The BORIS software was used to annotate our records, i.e., to assign video bouts to those particular behaviors that are associated to the foraging and feeding stages. We thus ended with a behavioral repertoire of food acquisition in our subset of shorebird species, in which each behavior was well characterized by reliable diagnostic criteria. This repertoire was built by two of us (L.H. and M.B.), with frequent cross-validation to guarantee inter-observer reliability of behavioral coding following the recommendation of Burghardt et al. (2012). The verbal description of this repertoire we provide here below is illustrated by excerpts drawn from our videos, in which we provide representative sequences of each behavior.
In a second step, we viewed all the video sequences of every species on which we collected data. For each species the same observers (L.H. and M.B.) recorded behaviors associated with food acquisition according to our behavioral repertoire, with cross-validation when needed. The final product of this step is a species-specific list of behaviors for all the 26 shorebird species of our data set.
3 Results
Table 1 shows the number, the total duration, and the mean duration with its standard error of the videos used to build the behavioral repertoire of food acquisition behaviors for 26 species of Western Palearctic shorebirds during migration or overwintering. Here we begin by an attempt to organize this extensive material in a logical, hierarchical manner (Figure 1), which would constitute a behavioral repertoire of food acquisition that could be generalized to all shorebirds, with the possible addition of behaviors from species that we have not studied. Then, for each of these 26 species, we propose an overview of their species-specific behavioral repertoire as drawn from our filmed observation.
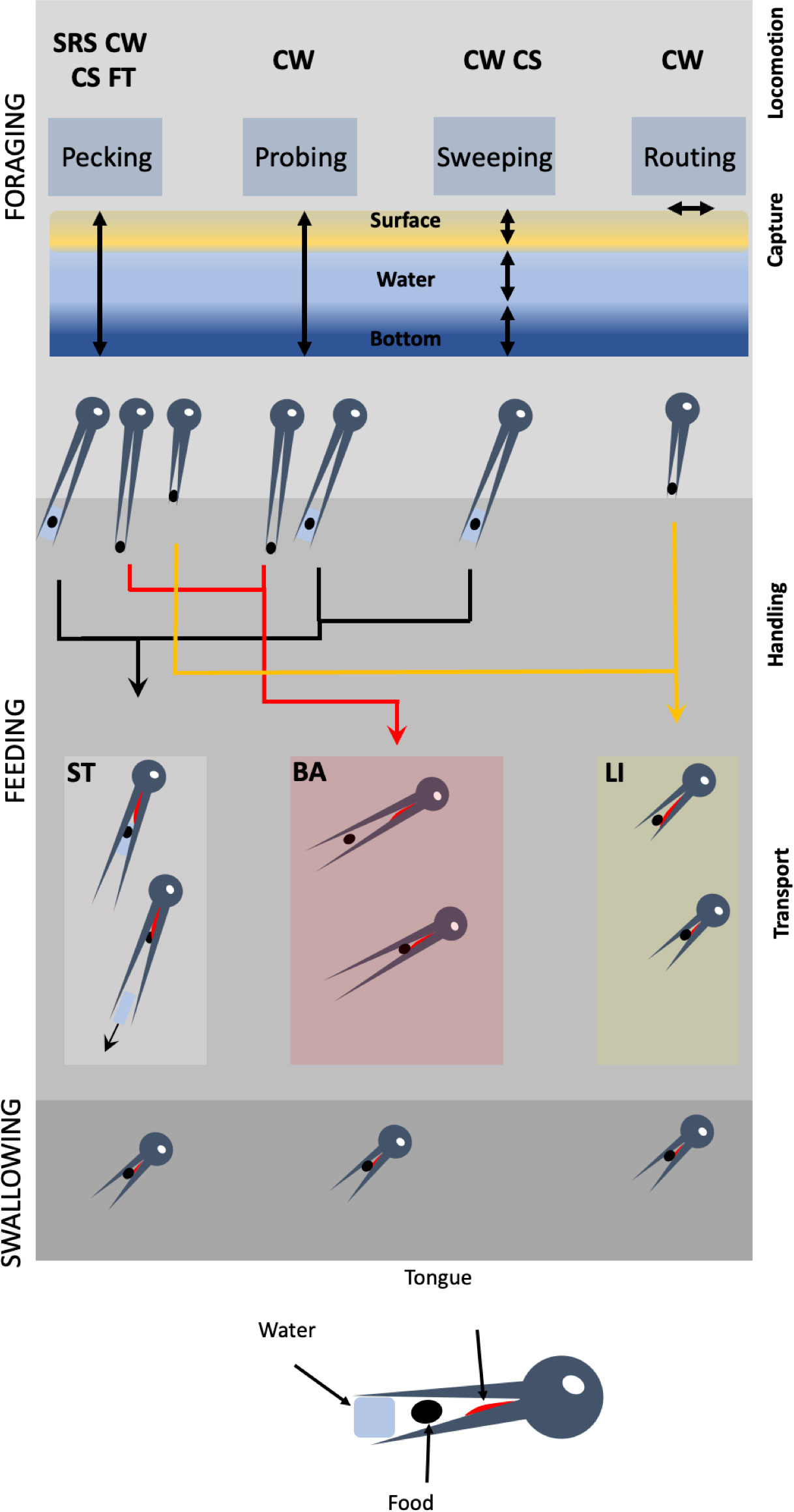
Figure 1 Hierarchical organization of the involvement of the cranial system during the three stages of food acquisition in shorebirds (foraging, feeding, swallowing). Foraging is divided into locomotion and capture. During locomotion, birds move their legs looking for foods using four stereotyped behaviors: SRS, stop-run-stop; CW, continuous walking; CS, continuous swimming; FT, foot trembling. The four capture behaviors (pecking, probing, sweeping, routing) are used at various water heights. Feeding begins by food handling in which the food particle is neutralized and fragmented if necessary, and oriented to be transported into the pharynx. Three modes of transport exist, each associated with more than one capture behavior: ST, surface tension; BA, ballistic and LI, lingual.
3.1 Repertoire of behaviors associated to food acquisition in shorebirds
As previously mentioned, we propose to deconstruct food acquisition in three successive stages, each composed of one or more behavioral sequences, which follow one another in time (Figure 1). Each sequence involves the use of stereotyped or non-stereotyped behaviors, as it will be explicitly mentioned. The first stage is foraging, in which birds move in suitable habitats looking for food and catch food with their beak. Foraging encompasses thus two different behavioral sequences, locomotion and capture. The locomotion sequence primarily involves the appendicular postcranial musculo-skeletal system and the sensory organs, while capture involves the cranial and axial postcranial musculo-skeletal systems, and still the sensory organs. The capture of a food particle in the beak ends the foraging stage and begins the second stage of food acquisition, which we call the feeding stage. Feeding also encompasses two different behavioral sequences, food handling and food transport. In food handling, the bird manipulates the food particle so that it can be in a state and in a position that can allow its transport into the pharynx. This sequence of course involves the sense organs, as well as the cranial and postcranial axial musculoskeletal systems. During this food handling sequence, the birds sometimes start moving again to look for locations suitable for manipulation (hard or wet substrate), which then also involves the locomotory system. Note that this food handling sequence is facultative: when the food particle is small, directly usable, and defenseless, it can be transported directly into the beak without handling. The transport sequence involves movements of the head, beak, and tongue to bring the food particle into the pharynx, and therefore recruits the cranial and axial post-cranial musculoskeletal systems, and always the sense organs. The entry of the food particle into the pharynx marks the end of the feeding stage and the beginning of the third stage, which we call swallowing. During swallowing, the food particle is swallowed and begins its digestion process. This stage involves the hyo-lingual musculoskeletal system and the sense organs. The salient point is that behavioral sequences and behaviors associated to foraging and feeding can be considered as performances sensu Irshick and Higham (2016), i.e., quantifiable measure of how well an organism performs an ecologically relevant task that is crucial for its fitness. The measurability of these performances ensures the possibility of evaluating their inter- and intra-specific variations, and thus to better understand their evolvability.
3.1.1 Foraging
The two behavioral sequences involved in foraging each have variations corresponding to stereotyped behaviors that can be found in different species. Locomotion takes four different forms, in which birds move their legs to find food. The bird can walk continuously and regularly in the habitat to locate food particles. We call this locomotion behavior “continuously walking” and notice that it corresponds to the widely foraging strategy as described by Pianka (1984). It should be noted that shorebirds using this behavior most often stop while capturing, transporting, and swallowing food particles, and only then resume walking. In a variant of this sequence, swimming shorebirds forage continuously in deep water, so we call this behavior “continuously swimming”. In the third strategy, motionless birds suddenly head towards a prey that they possibly catch. This “stop, run, stop” behavior is like the sit and wait strategy of Pianka (1984). Some shorebirds as the lapwing (Vanellus vanellus) or the little ringed plover (Thiornis dubius) using this stop, run, stop behavior sometimes wiggle one of their feet while standing still. By doing this, the birds entice prey buried in the substrate to come out of their holes, allowing to capture them. This foot trembling behavior is reputed to be practiced when preys are not very active (Simmons British Birds, Cramp and Simmons, 1983). Foot trembling entails a strong coordination between the appendicular postcranial musculo-skeletal system, the sensory organs, and the cranial and axial postcranial musculo-skeletal systems. Capture is carried out using four different, very stereotypical behaviors. “Pecking” consists in capturing a food particle either at the surface of the substrate, either on plants, bare soil, mud, water or at the bottom of the water. Pecking is accomplished by a rapid spring of the head and the neck, directed towards the food particle (Figure 2). “Probing” is realized by the insertion of the beak into soft sediments (silt or mud). The depth to which the beak is sunk in the sediments is very variable, from the culmen to just its tip. Probing is usually done by repeated vertical pounding movements of the head and neck, which are interrupted when a food particle is detected (Figure 3). Variants of probing exist in which the beak is repeatedly pushed obliquely forward into the substrate (Figure 4), which corresponds to the “ploughing” of oystercatchers (Haematopus ostralegus) (Hulscher, 1996). The beak can also be or inserted rather vertically, but in a series of side-swiping movements (Figure 5). Depending on how deep the beak is inserted and the nature of the substrate, probing can occur with the head fully emerged or fully submerged. In this last case, in most species the eyes are closed during the whole probing process and open when the bird moves the head and the prey out of water. The only exceptions in our data set are the oystercatcher (Haematopus ostralegus), which always keeps the eyes open, and the snipes (Gallingo gallinago and Lymnocryptes minimus) that only close their eyes when they are fully submersed. The opening of the beak during probing is variable. “Sweeping” corresponds to capture of food particles by rapidly turning the neck and head from right to left and vice versa with the beak held in the water (Figure 6). During these scything movements, the food particles are retained in the space between the upper mandible and the lower mandible while most of the water is allowed to run off freely. The head can be fully emerged or fully submersed, with all intermediaries possible. In our view and in contrast to other works (Angarita-Baez and Carlos, 2023), sweeping differs from lateral probing by the fact that the beak of the sweeping bird searches in open water or on the surface of the substrate, and does not sink into the substrate. In avocets (Recuvirostra avosetta), eyes are closed if the head is submersed during sweeping. “Routing” is a behavior in which birds moving continuously from side to side suddenly stop to search for food by turning with their beak stones, shells, or kelps littering on an emerged substrate. Routing behavior involves rapid and powerful movements of both rotation and elongation - retraction of the neck and head, as well as beak lifts and tractions. Food particles unearthed in this way are then captured by pecking (Figure 7).
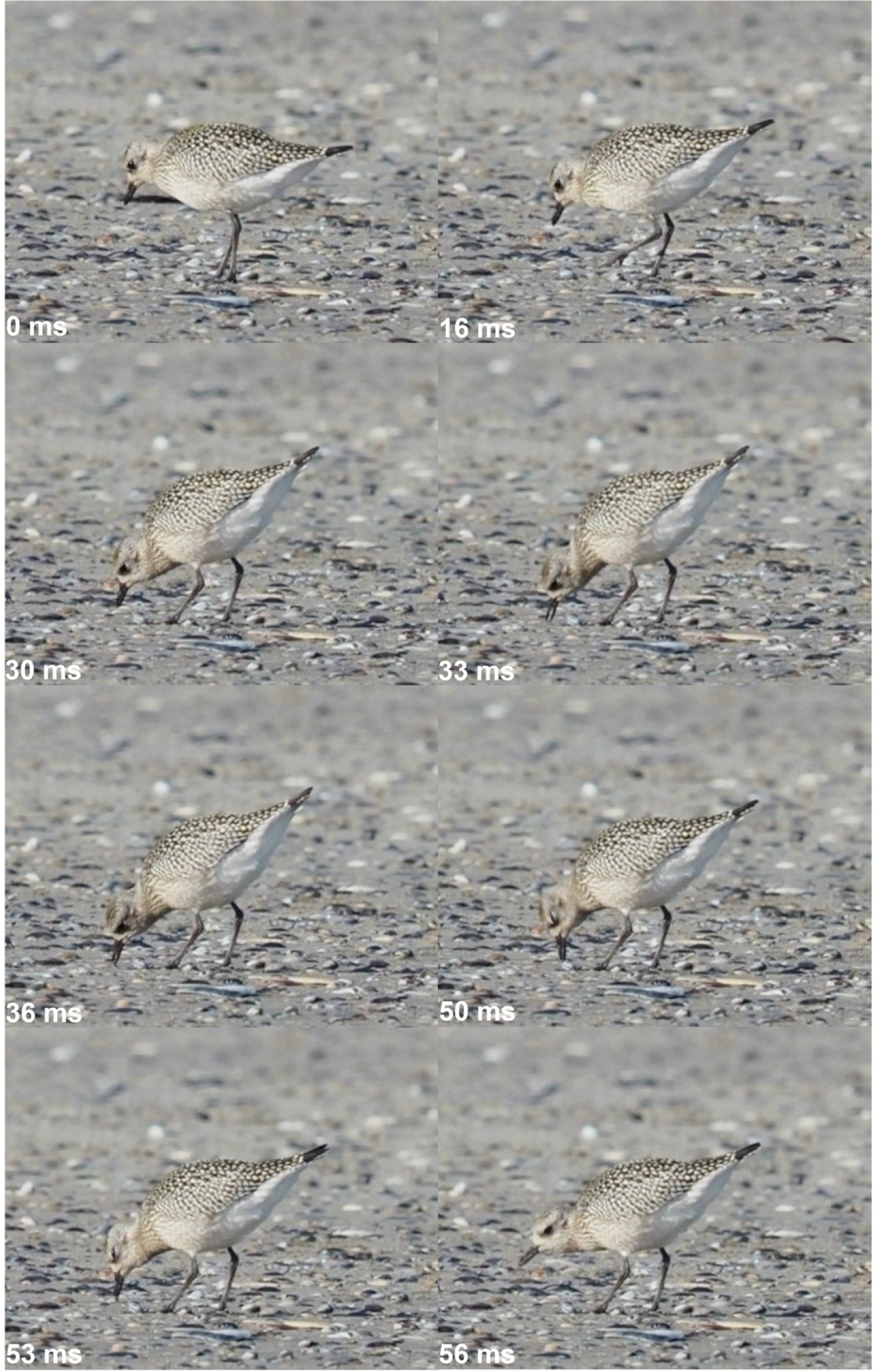
Figure 2 Foraging and pecking behavior of a grey plover (Pluvialis squatarola), beach of Nieuwpoort (B), September 22nd, 2022. At time 0 ms, the bird locates the prey, and then heads to it (16 ms). The capture behavior begins at time 30 ms, with a rapid spring of the head and the neck, di-rected towards the prey. The prey is caught on the surface of the ground by opening and closing movements of the beak (33-50 ms), and the capture ends when the prey is at the tip of the beak and the bird raises its head (53-56 ms). Pictures Michel Baguette.
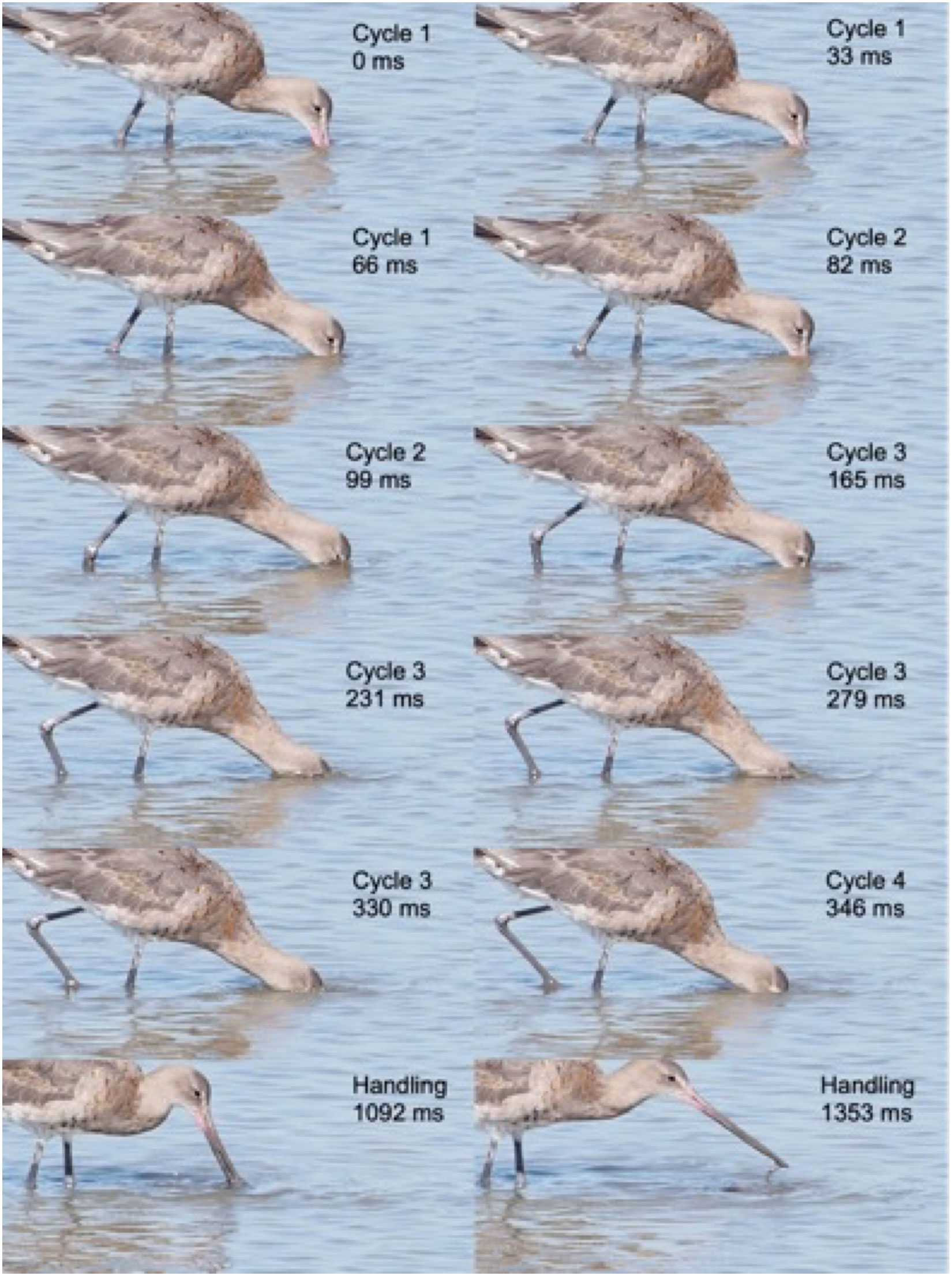
Figure 3 Foraging, almost vertical probing behavior and prey handling by a black tailed godwit (Limosa limosa), Pont de Gau, Saintes Maries de la Mer (F-13), July 7th, 2023. The bird explores the sediment by using various number of head vertical movements that are organized in cycles according to their vertical back and forth. For instance, cycle 1 ends when the bird lifted its head and began to plunge it back. Cycle 1-4: successive probing beak cycles 1 to 4. Probing can occur either when the bird stops during beak movements, or when the bird moves continuously. Eyes are closed during probing under water. Handling: worm handling after capture, using rynchokinesis (opening of the distal part of the beak while the proximal part remains closed) at 1092 ms. Pictures Michel Baguette.
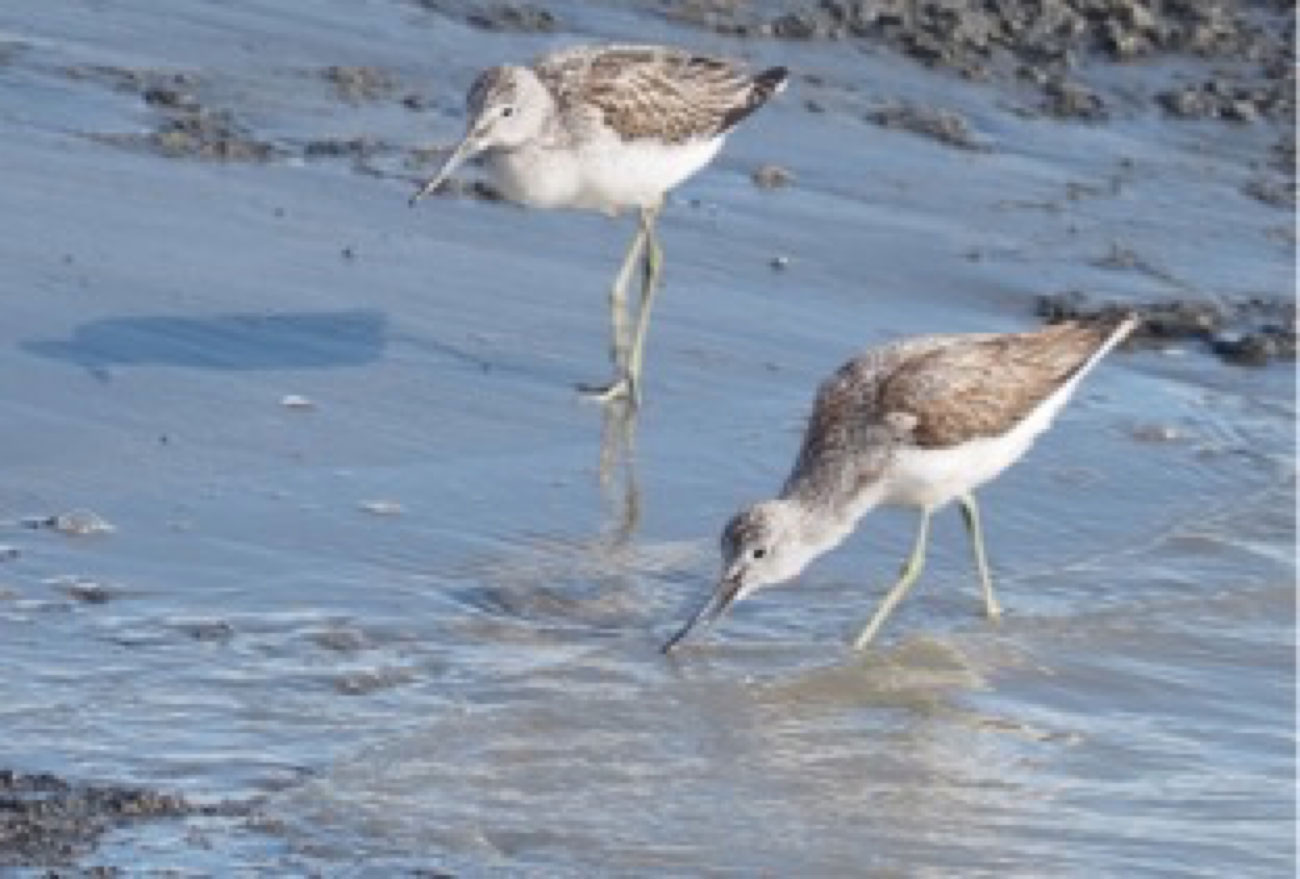
Figure 4 Oblique probing behavior of a greenshank (Tringa nebularia), Ijzermondig, Nieuwpoort (B), September 20th, 2022. The bird advances pushing its ajar beak in front of it, which scrapes away the sediment and excavates food particles. Picture Michel Baguette.
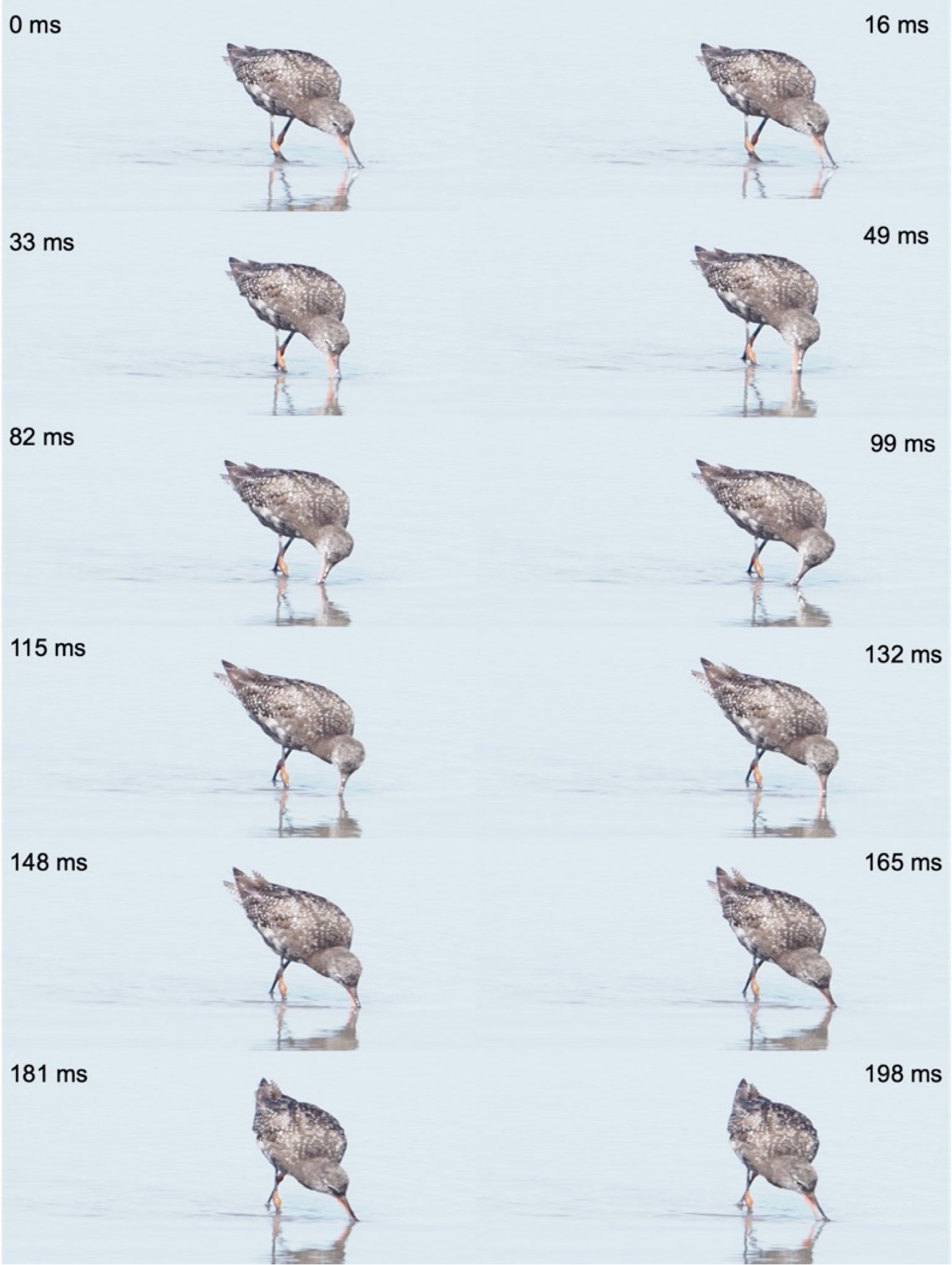
Figure 5 Lateral probing behavior of a spotted redshank (Tringa erythropus), Pont de Gau, Sainte Marie de la Mer (F-13), July 7th, 2023. The bird advances forwards, balancing its ajar beak from side to side, which scrapes away the sediment and excavates food particles. Pictures Michel Baguette.
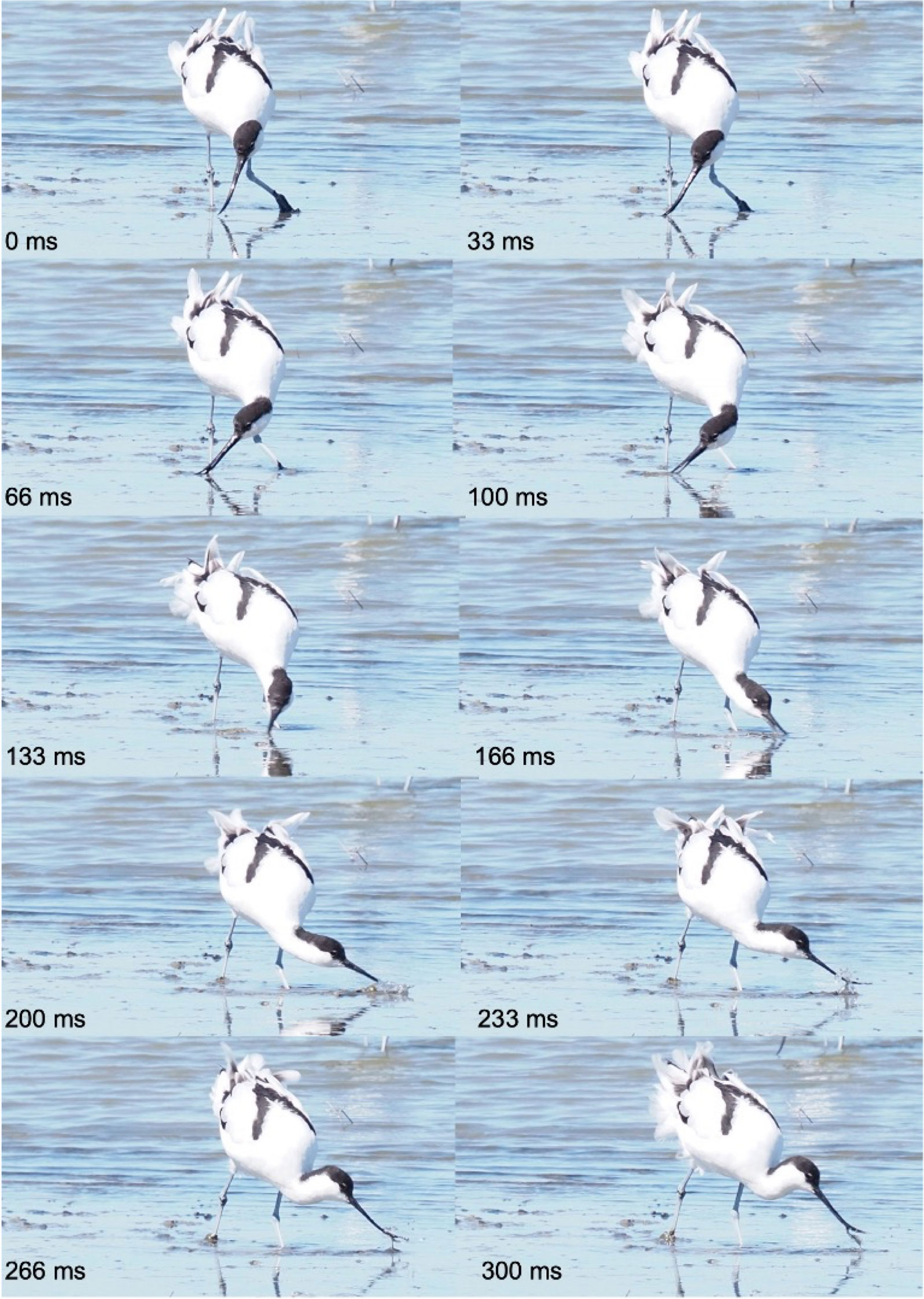
Figure 6 Foraging and sweeping behavior of an avocet (Recuvirostra avosetta), Réserve onithologique du Teich, bassin d’Arcachon (F-33), April 9th, 2022. The bird walking continuously moves its head from side to side at various water depths and stops for food transport. Small preys are captured at the distal tip of the slightly ajar beak during the wide lateral movements of the head, which give this capture behavior its name sweeping. Pictures Michel Baguette.
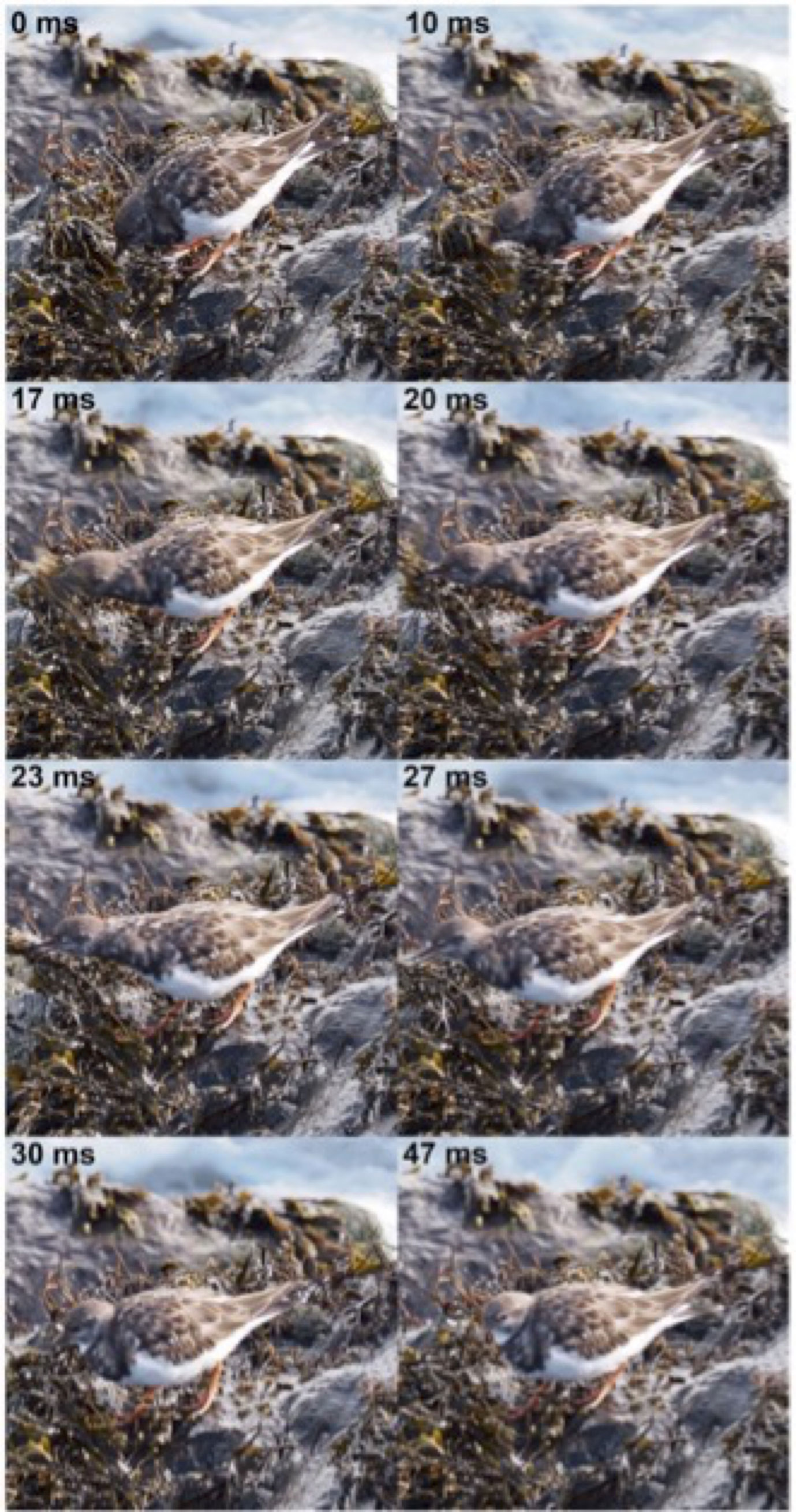
Figure 7 Routing behavior of a ruddy turnstone (Arenaria interpres), pier of Oostende (B), September 20th, 2022. The bird lifts kelp by using various powerful and rapid head and beak movements. Eyes are closed and beak is open when the bird is in close contact with kelp. The prey will finally be caught by pecking. Pictures Michel Baguette.
3.1.2 Feeding
According to the detailed investigations of Hulscher (1999) on oystercatchers, and to our own observations on other species, handling, the first behavioral sequence involved in the feeding stage, consists in behaviors that are generally less stereotyped, probably because they are pretty dependent on the context (i.e., size, shape, and possibly defense of food particles) and on the condition of the birds, particularly their experience. The final stage of this handling stage is to have a food particle that can be transported into the beak to the pharynx. Birds must therefore find idiosyncratic solutions to the various situations they face, ranging from killing and shredding bulky prey, eliminating potentially injurious parts, or removing indigestible extraneous bodies. Oystercatchers (Haematopus spp.) are rather exceptions: they have two main stereotypical handling behaviors to open the bivalves they preferentially feed on, associated with differences in beak morphology (Hulscher, 1996). In oystercatchers, “Stabbing” involves the rapid section of shell retractor muscles when the bird finds a slightly open bivalve (Figure 8), whereas “Hammering” is a behavior in which birds pound bivalves with their beak on a hard substrate to destroy the shell and extract the flesh. Oystercatchers, and some other species sometimes move the prey they have caught several meters or more. It is then a question of finding a substrate which is suitable for handling, hard to be able to effectively hammer a bivalve, wet to moisten food particles, or on the contrary dry to effectively shred preys. It may also involve leaving an immediate socially threatening environment (theft of food by conspecific or heterospecific individuals). Transport (the transfer of food particles from the tip of the beak to the pharynx) can be achieved in three different ways. In “ballistic transport”, a backward linear movement of the head or a backward rotation of the head launches the food particle from the tip of the bill towards its distal extremity and induce a straight-draw transport, or a parabolic trajectory, respectively. These movements can be repeated until the food particle encounters the tongue, and from this time onwards lingual transport leads the prey to the pharynx (Figure 9). In “surface tension transport”, small food particles are enclosed within a drop of water that progressively advances along the beak. The movement of the drop is induced by successive openings and closings of the beak, until the drop encounters the tongue (Figure 10). The tongue then controls the transport of the food particle, while the water is expelled in the form of either a drop or several droplets at the tip of the beak. Larger food particles glide along the lumen of the beak, aided by the lubricating properties of the water along the smooth walls of this tube, until the food particle encounters the tongue, which here again takes the control of the end of the transport. In snipes probing with the beak vertically in the sediments, the progression of the prey along the long beak is facilitated by the adoption of a posture during which the bird takes its beak out of the water and places it at an angle approximately 45° relative to the surface. “Lingual transport” is always the final stage of ballistic or surface tension transports, but it can also serve as a sole means of transport. The tongue then directly contacts the prey at the tip of the beak and brings it in the pharynx (Figure 11).
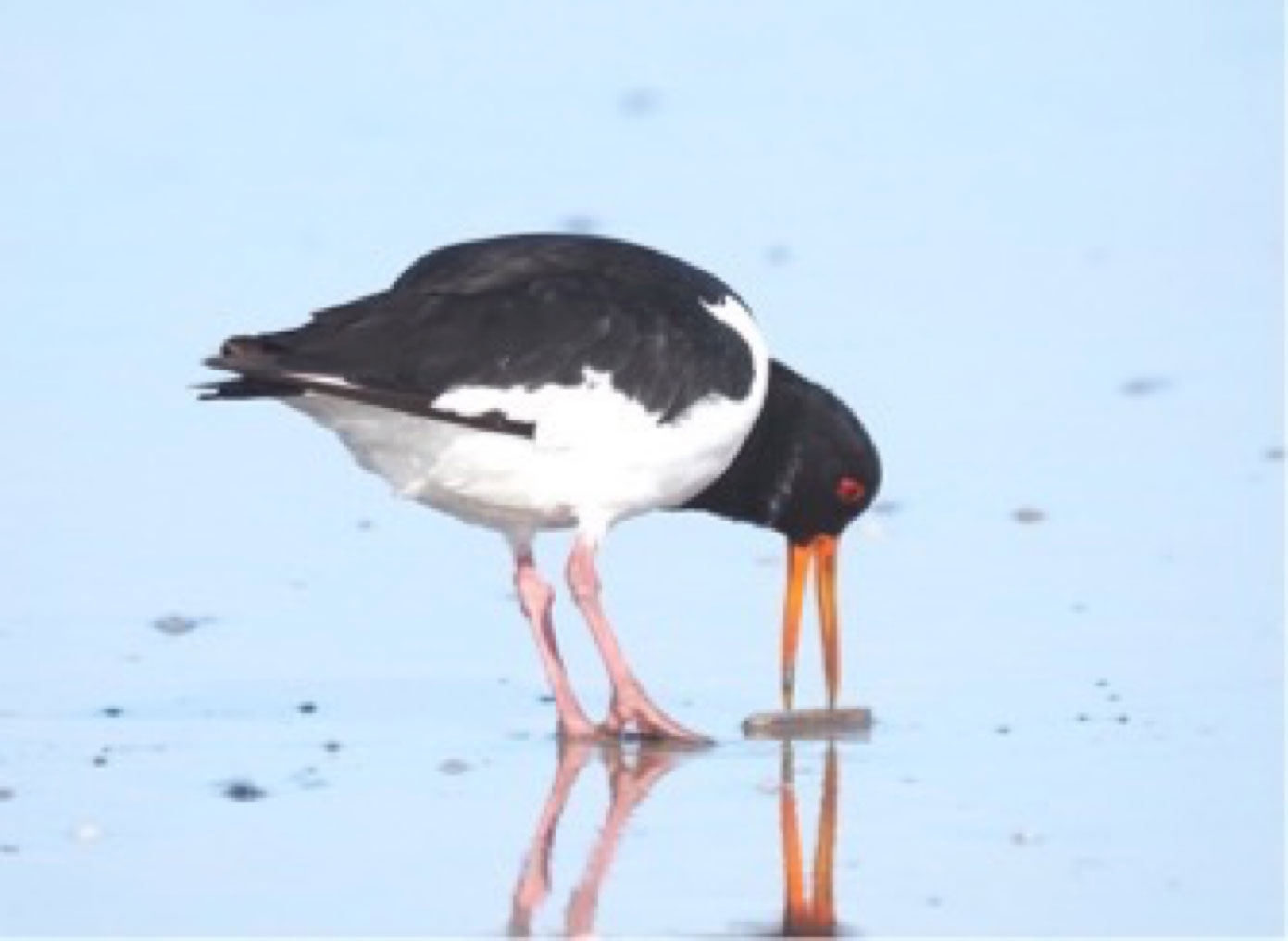
Figure 8 Stabbing by an oystercatcher (Haematopus ostralegus), beach of Oostende (B), September 20th, 2022. The bird inserts its beak between the valves of a slightly open razor clam, to dissect the shell retractor muscles. Picture Michel Baguette.
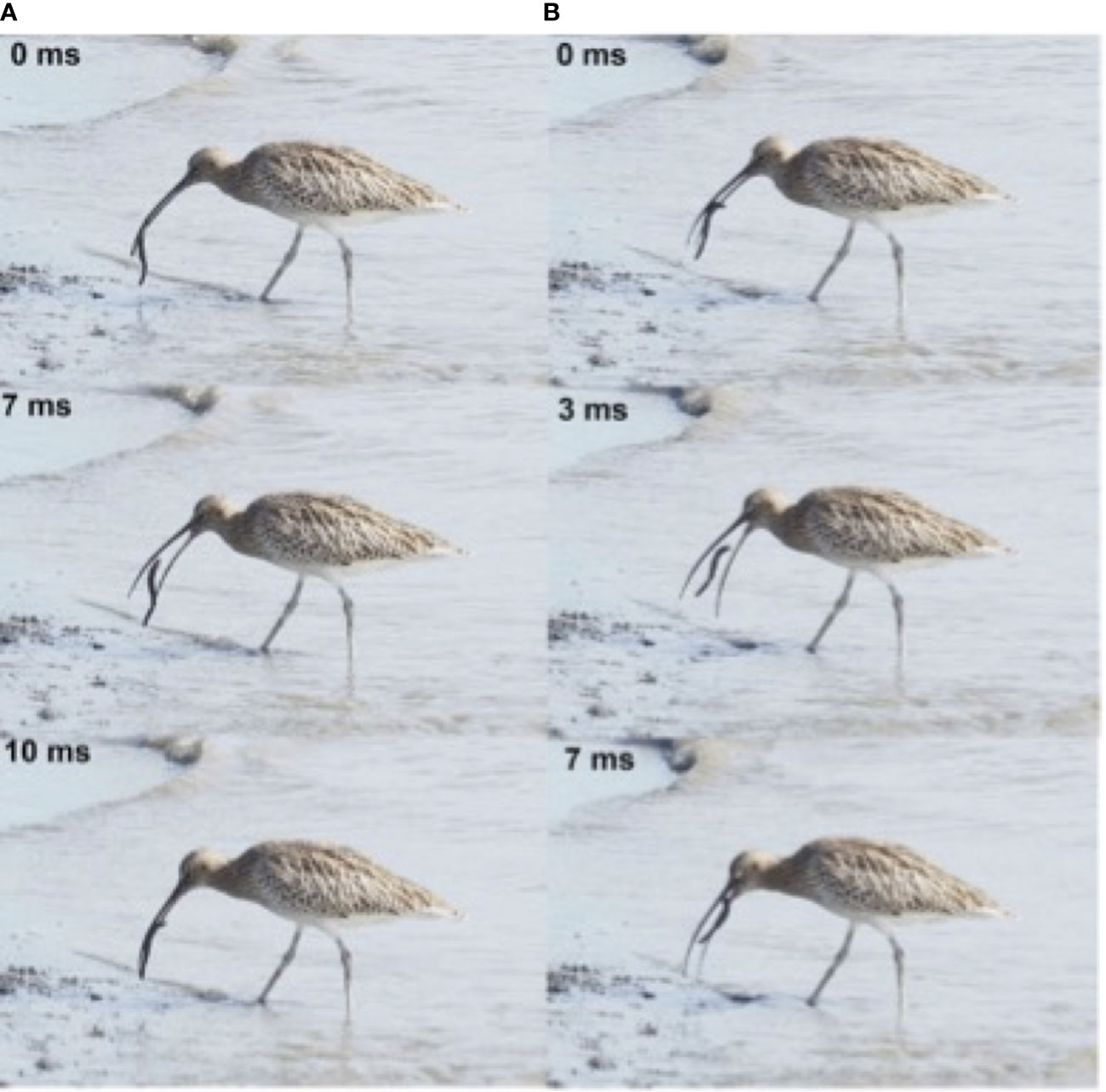
Figure 9 Four cycles of ballistic transport of a large worm by a Eurasian curlew (Numenius arquata), Ijzermondig, Nieuwpoort (B), September 20th, 2022. (A, B), two successive ballistic transports showing the prey moving from the tip of the beak to the tongue. The prey is freed from the beak, which then suddenly moves forwards and recaught the prey so fast that the prey “levitates” during this brief interval. These successive cycles show how this bird with a long thin beak transports any prey without using water (surface tension). Lingual transport only occurs as soon as the tongue contacts the prey and can act on its posterior displacement toward the pharynx. Pictures Michel Baguette.
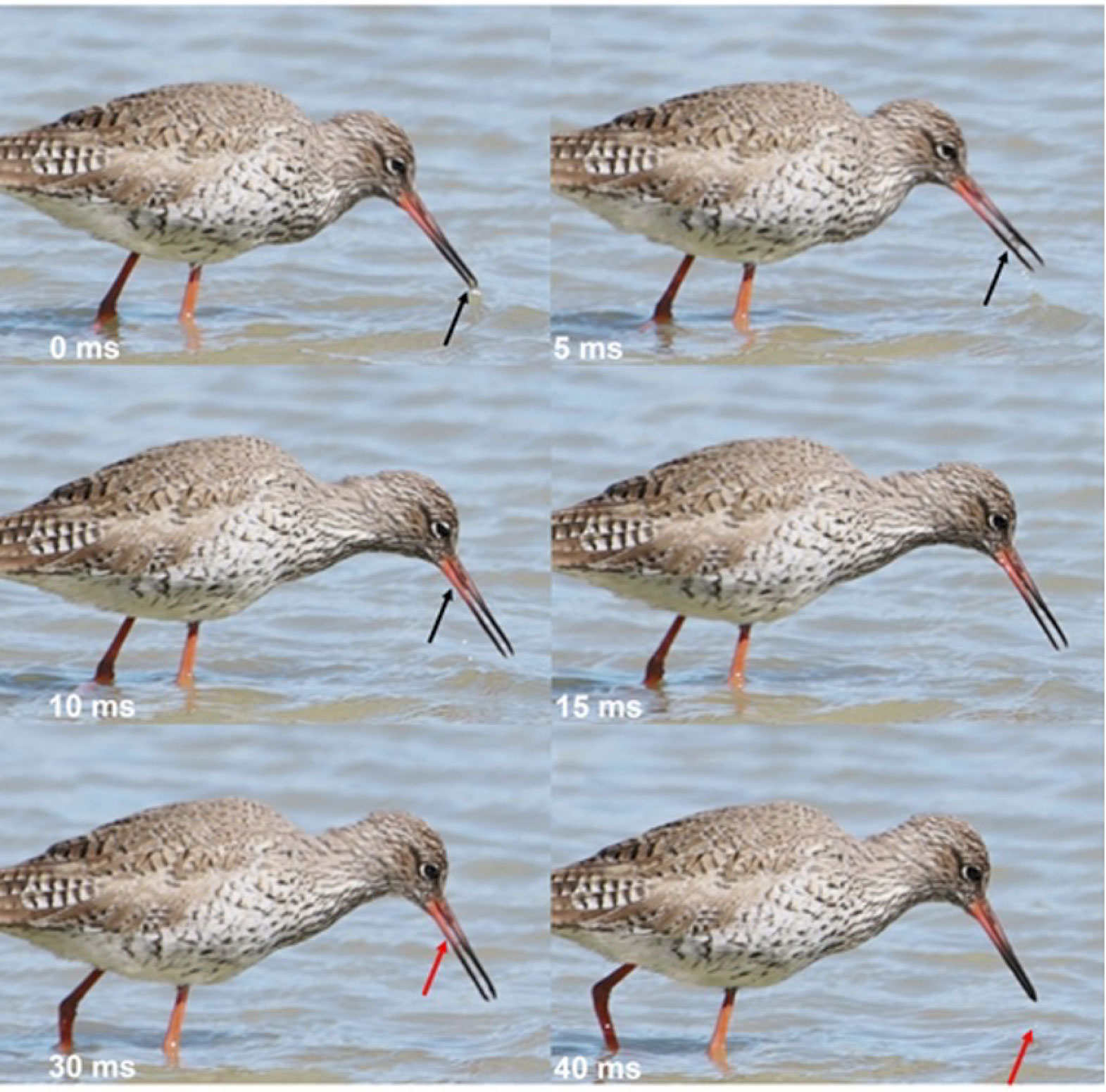
Figure 10 Surface tension transport by a redshank (Tringa totanus), Domaine des Oiseaux, Mazère (F-09), March 28th, 2023. The food particle caught by the beak tips is moved along the beak by integrated head movements and surface tension mechanism due to the food inclusion in a water droplet. The black arrow shows the food position along the head cycles. From time 0 ms to 5 ms, bird suddenly moves the head backwards to give energy to the food included in the droplet and opens the beak. The food glides by surface tension along the beak to reach a posterior position at time 10 ms. The bird moves the head forward from time 10 ms to time 15 ms for helping movement of the food in the droplet. Between time 15 ms and 30 ms, the bird does not move the head, and it is the tongue that transports the food to the pharynx. At the same time, the water droplet glides to the beak tip due to the gravity and is expulsed from the beak cavity when the beak closes at time 40 ms. Black arrows: food particle included in water droplet. Red arrow: water droplet expulsed from the beak of the bird. Pictures Michel Baguette.
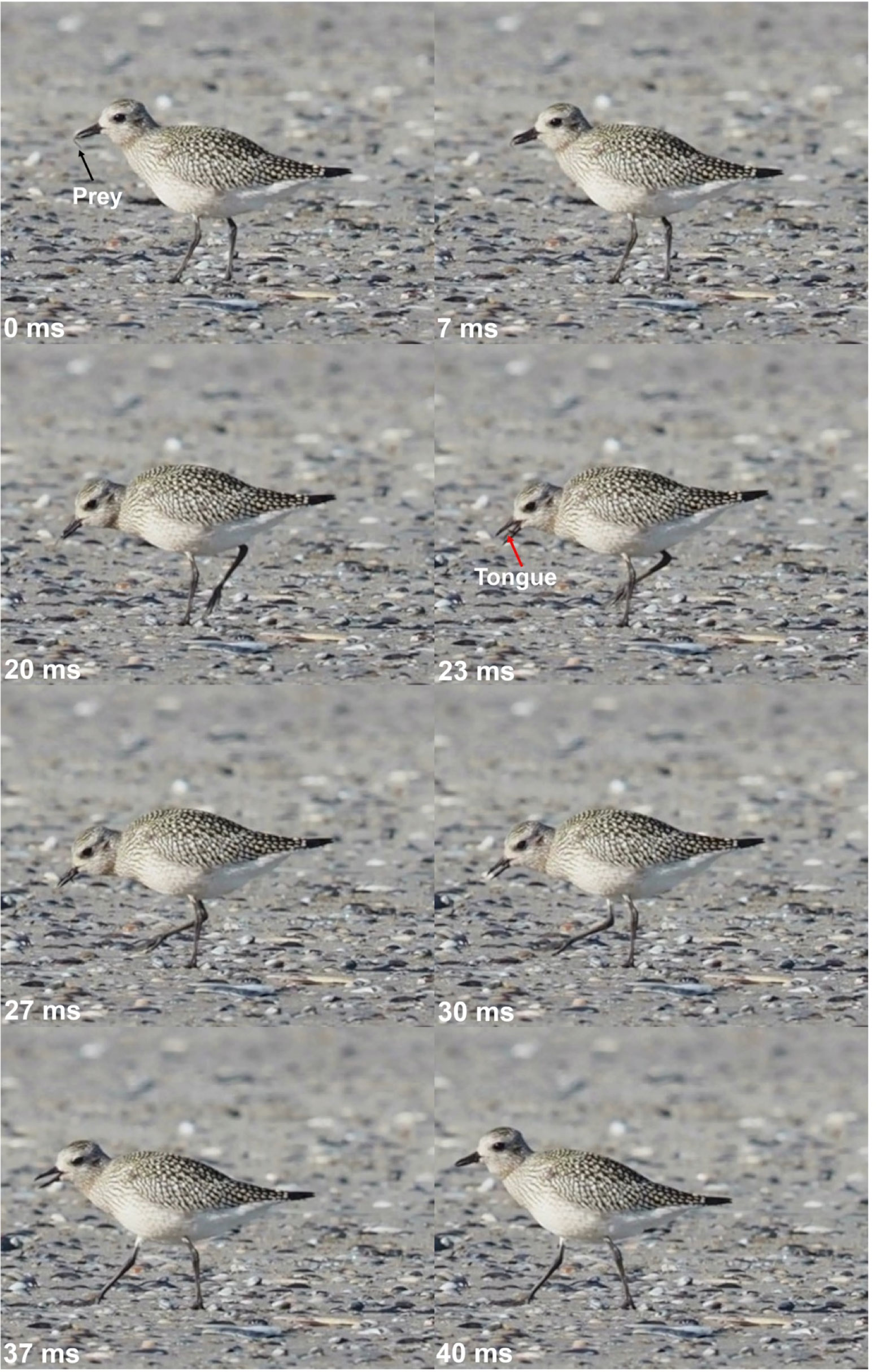
Figure 11 Lingual transport in a grey plover (Pluvialis squatarola), beach of Nieuwpoort (B), September 22nd, 2022. The bird uses one jaw-tongue coordinated cycle to move to prey from the beak tips toward the pharynx. The amplitude of the gape cycle is small, and the tongue elongation corresponds to the lower beak length. In plovers, food transport occurs during locomotion, showing an independent motor organization for the cranial and post cranial systems. Black arrow: prey. Red arrow: tongue. Pictures Michel Baguette.
3.1.3 Swallowing
The swallowing stage is the end point of the food acquisition process that is characterized by the entrance of the food particle within the pharynx. Whereas the beginning of this stage is usually well defined, the detail of processing of food within the pharynx is difficult to study in our observations. This is the reason why we do not consider here swallowing as a performance, i.e., a quantifiable measure of how well an organism performs an ecologically relevant task that is crucial for its fitness (Irshick and Higham, 2016).
3.2 Overview of the behavioral repertoire of 26 Western Palearctic shorebird species
The stereotyped behaviors of food acquisition observed in our set of 26 shorebirds species are summarized in Table 2, and their associations in Figure 12. Each species uses usually one, rarely two locomotion behaviors. Stop-run-stop is used by Charadri only, but not by all Charadri species, and the foot trembling behavior is always associated with it, but only in two species of our dataset (the little ringed plover Charadrius hiaticula and the lapwing Vanellus vanellus), as already noticed by Simmons (1961) and Cramp and Simmons (1983). Continuous walking is the only locomotion behavior for species that use it, except for the avocet (Recuvirostra avosetta), which can also do continuous swimming. In our dataset, the red phalarope (Pahaloropus fulicarius) uses continuous swimming as the only locomotion behavior (Figure 13). These birds grasp their preys while swimming in shallow water, without their legs touching the ground. This mode of locomotion provides them with great maneuverability and allows them to feed at sea in the area where the waves are beating, where the ebb and flow causes their prey to move up and down in the water column. Pecking is the most frequently used capture behavior: it is recorded in 22 out of 26 species. In 13 species out of these 22, it is associated with another capture behavior (probing in 11 species, sweeping in 2 species, routing in 1 species). Probing is used by 15 species, as unique capture behavior in 4 species and in association with pecking in 11 species. Sweeping is only used by the avocet (Recuvirostra avosetta) and the black-winged stilt (Himantopus himantopus. The turnstone (Arenaria interpres) is the only species to use routing as a capture behavior. Ballistic transport is the most used transport behavior, by 18 out of 26 species. It is associated with the surface tension transport behavior in 14 species. Only two species use surface tension as their sole transport behavior, i.e., the common snipe (Gallinago gallinago) and the jacksnipe (Lymnocryptes minimus). Altogether, these results indicate species-specific preferences in the use of behaviors associated with the three performances mobilized by food acquisition in shorebirds.
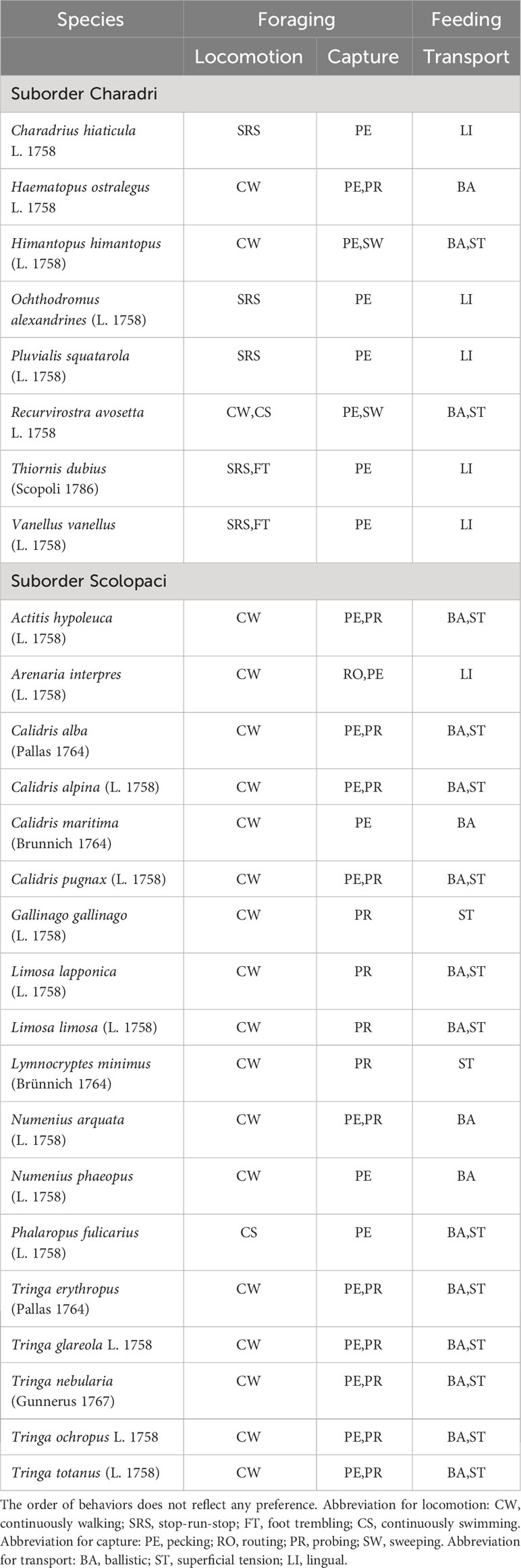
Table 2 Locomotion, capture, and transport behaviors observed in our subset of 26 Western Palearctic shorebird species.
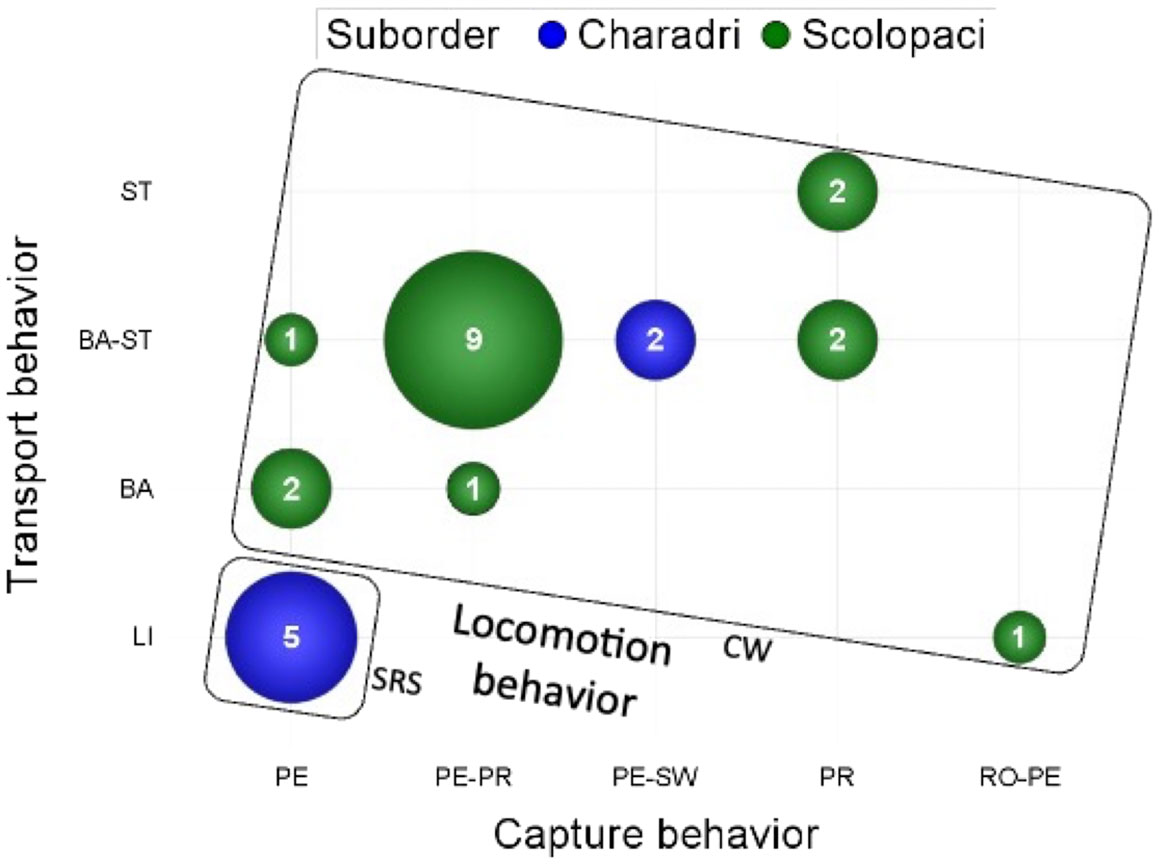
Figure 12 The frequency and association of the stereotyped behaviors in the 26 studied species, represented as the numbers of species using pairs of transport-capture behaviors. SRS locomotion behavior is systematically associated with LI transport and PE capture behaviors, and present only in the basal clade of Charadri.
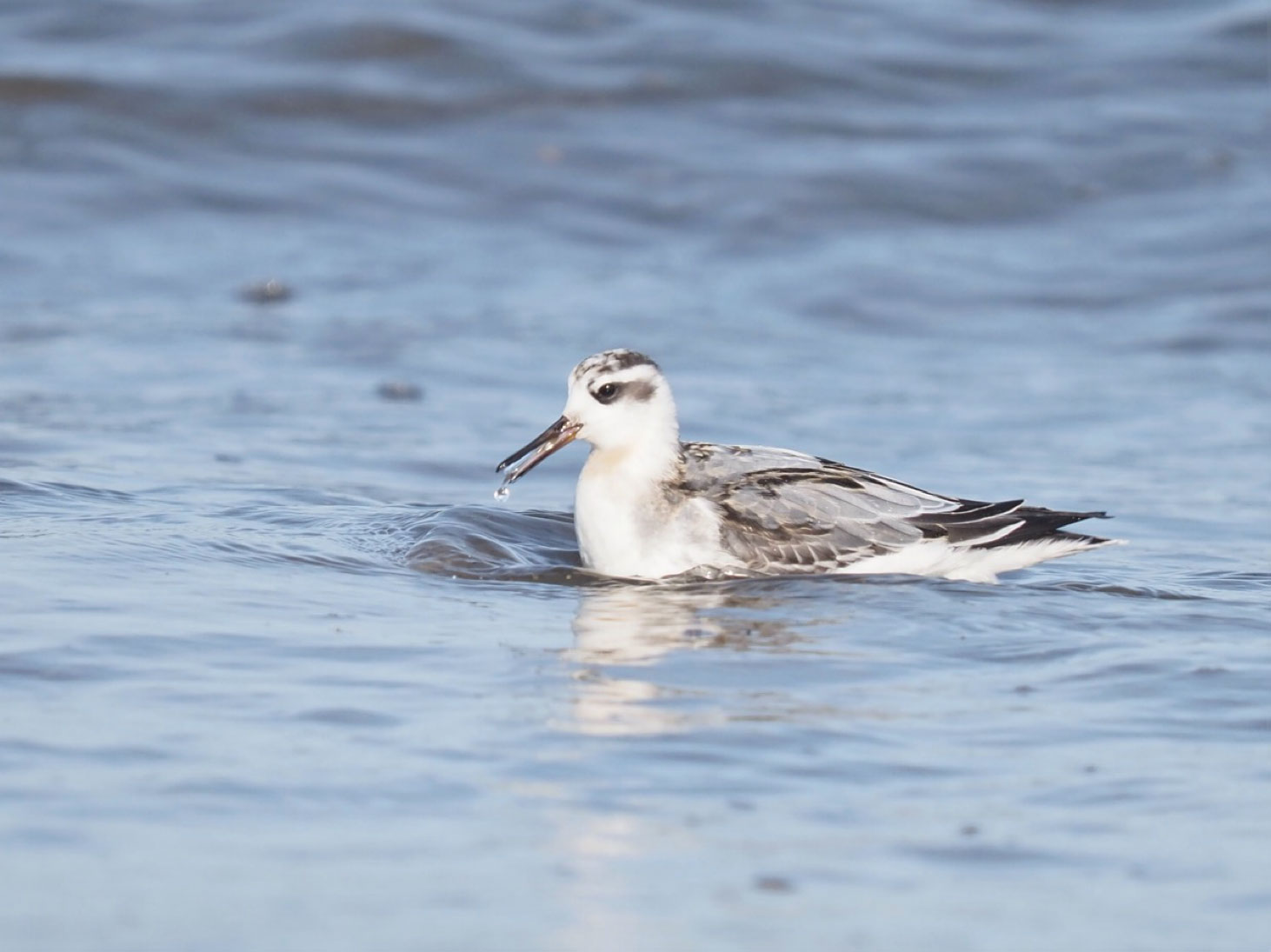
Figure 13 Swimming red phalarope (Pahaloropus fulicarius) using tension surface transport to move a prey along its beak. Oostende September 22nd, 2022. Picture Michel Baguette.
4 Discussion
Our data set is restricted to shorebird species living in Western Palearctic. We sampled behaviors of 26 species, which represent the majority of shorebirds that breed, migrate and/or overwinter in this area. Figure 14 shows the hypothetical phylogenetic relationships among our study 26 species, as inferred from the dated consensus tree of Cerny and Natale (2022). Our dataset includes representatives of the two Charadriiforme suborders that make up the shorebird group. Oystercatcher, avocet and stilt, plover and lapwing are representative of the three families of the suborder Charadri, namely Haematopoidae, Recuvirostridae and Charadriidae. The suborder Scolopaci is only represented by members of the Scolopacidae, the other family of this suborder (Jacanidae) having no representative in the western Palearctic. The diversity of the Scolopacidae of the Western Palearctic is however well sampled, with sandpipers, shanks, snipes, godwits and curlews.
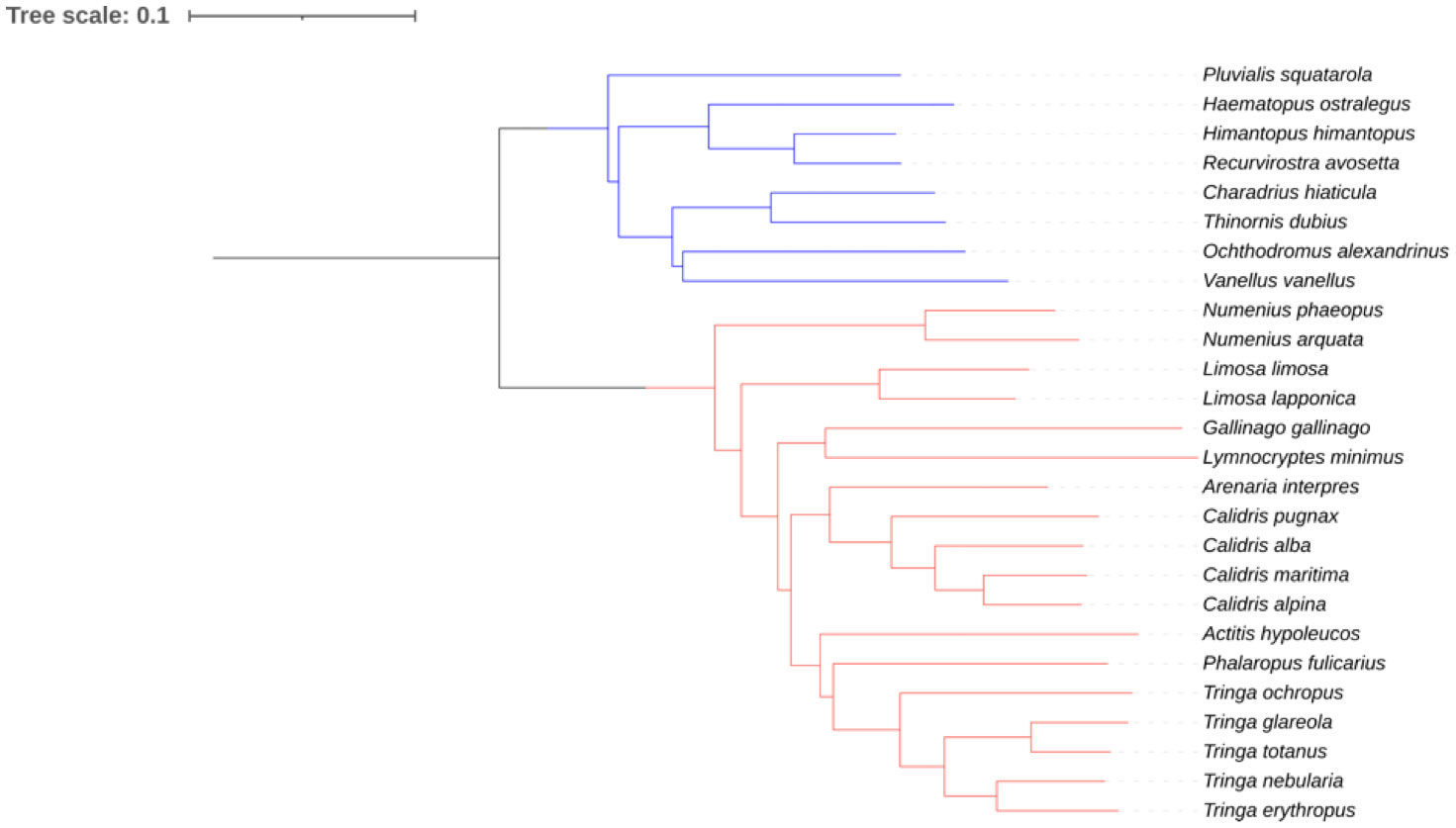
Figure 14 Phylogenetic relationships between the 26 shorebird species of our data set drawn from the consensus tree of Cerny and Natale [22], pruned to our 26 study species using iTOL [23]. Blue and red bars correspond to species belonging to Charadri and Scolopaci, respectively.
Given the methodology used, including repeated observations in space and time, we have a good coverage of food acquisition behaviors for the studied species, although the attribution of one or the other behavior of our repertoire to a given species may be missing. In this sense, our repertoire is a first step towards a more complete final product. Besides, it is possible that our subset of shorebirds uses other behaviors than those that we present here. Literature data indeed indicate that some Calidris species graze on biofilm, i.e., a surface layer (~0.01–2 mm) of organic detritus, unicellulars, benthic invertebrates, and sediments bound together by extracellular polymeric substances secreted by diatoms and bacteria (Elner et al., 2005; Kuwae et al., 2008). We did not have the opportunity to observe this behavior, although the dunlin (Calidris alpina), which is recorded in our dataset was observed practicing it in North America. This might be due to the fact that Calidris alpina is a polytypic species, with 10 subspecies described (Gill et al., 2023), and the individuals on which biofilm grazing behavior was observed belong to a subspecies (C. a. pacifica) (Elner et al., 2005) that differs from the subspecies which overwinters in the Western Palearctic. Finally, we do not pretend that we have recorded all possible behaviors used by shorebirds. Beside biofilm grazing, we can expect that species with extravagant bills like the aptly named spoon-billed sandpiper (Calidris pygmaea), which has a dorso-ventrally flattened beak, or the wrybill (Anarhynchus frontalis) with its thin elongate (for a plover) and especially right bended beak would use unusual behaviors to acquire food. Moreover, the Tuamotu sandpiper (Prosobonia parvirostris), which is the only living representative of an almost extinct clade of South Pacific sandpipers, is reputed to be nectarivorous. The surprising diet specialization of this insular bird suggests the use of particular behaviors of food acquisition.
4.1 Postcranial system and locomotor behavior
In all vertebrates (Bels and Whishaw, 2019), any foraging and feeding behaviors is the result of integrative motor actions of various skeletal and muscular systems [e.g., fixed-action-pattern sensu Tinbergen and Lorenz in the 1930th (Schleidt, 1974; Beer, 2020; Tinbergen, 2020) or modal-action-pattern (Barlow, 1977)]. During the last decade, the functional integration between the cranial and postcranial muscular and skeletal systems for determining the characteristics of the foraging and feeding behaviors has been largely emphasized in a large diversity of vertebrates including aquatic birds (Montuelle and Kane, 2019; Heiss et al., 2023; Pallandre et al., 2023). But such integration has not yet been studied in Aves as shorebirds living at the limit of both aquatic and terrestrial environments, although food resources with highly different ecological, physiological, morphological, and behavioral traits are available in various habitats (e.g., prey living at the water surface, on sediments, and within sediments at various depths). Heiss et al. (2023) did not discussed the shorebirds in their exhaustive comparative analysis of Sauropsida because they primarily considered foraging and feeding behaviors targeting freely moving aquatic preys (e.g., filtration, skimming, suction, and ram feeding). Our data demonstrate that the foraging stage of food acquisition in all studied shorebirds relies on the combination of two motor patterns integrated with various sensory controls: locomotion and capture. The salient point is that during evolution successful foraging strategies involve either coupled or decoupled postcranial and cranial motor patterns to achieve food capture. In our integrative approach to determine the foraging behaviors, we thus propose a major alternative method to the classification of Barbosa and Moreno (1999) in which both patterns are mixed. Indeed, a strong difference was recorded in shorebirds that use three major locomotor behaviors: (i) stop-run-stop, (ii) continuous walking, and (iii) swimming. The stop-run-stop behavior is always associated with a visual detection of the prey and a pecking capture behavior (Figure 1). Birds using the continuous walking or swimming locomotion behavior can also detect their preys by sight, but they most often do not interrupt their walk or swim: the prey is picked up by the beak at the surface of the sediment or of the water while the bird remains in motion. Locomotion is thus interrupted in the first case, whereas it is a continuous process in the other cases. This is a key finding because it implies that birds using the stop-run-stop locomotion behavior decouple the appendicular postcranial musculoskeletal system involved in locomotion from the cranial and axial postcranial musculoskeletal systems involved in capture. This decoupling occurs in the ancient clades of the sub-order Charadri (plovers, lapwing) in our data set that are considered as the most ancient shorebirds according to the dated phylogeny of Cerny and Natale (2022). On the contrary, in birds that use continuous locomotion behaviors (walking or swimming), there is no decoupling between the different musculoskeletal systems. It is interesting to notice that this absence of decoupling concerns all Scolopaci, but also those recent Charadri in our dataset (avocet, stilt, and oystercatcher) according to the dated phylogeny of Cerny and Natale (2022) that use continuous walking or swimming locomotion behaviors.
4.2 Cranial system and food capture
Pecking is considered as the ancestral capture behavior in birds, from which probing and sweeping are derived by progressive modification of the beak length, shape, and internal structure according to the seminal works of Zweers and colleague (Zweers, 1991; Zweers et al., 1994; Zweers and Gerritsen, 1997; Zweers and Vanden Berge, 1997). However, it is worth noticing that the deductive method used by Zweers and collaborators to infer that pecking is plesiomorphic compared to probing and sweeping should be happily complemented by a rigorous cladistic analysis. All the five species of our data set that are in the ancient clades of the sub-order Charadri (plovers, lapwing) according to the dated phylogeny of Cerny and Natale (2022) indeed use pecking as the only capture behavior. The three species of Scolopaci using only pecking have each a particular feeding habitat or diet. Purple sandpipers (Calidris maritima) feed on preys that they peck from hard substrates on rocky coasts and breakwaters. Red phalaropes (Phalaropus fulicarius) capture invertebrates that they peck on the surface or in shallow water. Eurasian whimbrels (Numenius phaeopus) feed on crabs that they capture on sandy soils. These three species have in common that they only capture their prey on sight, just like the five species belonging to the basal clades of Charadri. Another argument indicating that pecking behavior is indeed an ancestral character is provided by the ontogeny of foraging. Preliminary observations from our own indicate that pulli of sandpipers, shanks, stilts and avocets use pecking exclusively to capture their prey, whereas adults mix pecking and either probing or sweeping capture behaviors. In oystercatchers and snipes, adults capture small preys and present them to pulli, which peck the prey at the tip of the adult’s beak.
As mentioned by Zweers and colleagues, the transition from pecking to other capture behaviors involves the use of touch sense. Mechanoreceptors located in the bill skin that convert pressures and vibrations into electric signals allow the location of prey by direct touch and even by remote touching (Piersma et al., 1998; van der Kam et al., 2004). In shorebirds, increased sensitivity is obtained by enlarging the dermal area available for mechanoreceptor positioning. Extra space is gained by a dermis invading the bone, in the form of depressions of the premaxilla and mandible (Zweers et al., 1994). The number of these depressions, also called sensory pits has been used by Barbosa and Moreno (1999) to try to assess the more or less prober character of different species of shorebirds. But this approach seems unreliable, because (1) there seems to be a very large variation, at least geographically in the number of sensory pits for a given species, (2) there are currently no data on the density of mechanoreceptors in these depressions, and (3) it seems that in at least one case the depressions are present on the beak, but the mechanoreceptors are no longer active. In their classification of food acquisition behaviors, Barbosa and Moreno (1999) used the work of Hoerschelmann (1972) to infer the number of sensory pits located in the distal portions of maxillae and mandibles of several shorebird species. However, the number of sensory pits reported by Hoerschelmann (1972) on shorebirds probably collected in Germany differs considerably from those figured in Bolze (1968) or more recently published by Lin (2022) on shorebirds from Australia and New Zealand. Lin’s Master’s thesis presents the number of sensory pits of 14 shorebird species corroborated by photographs of beak skeleton with the sensory pits located there, for the ruddy turnstone (Arenaria interpres), the common greenshank (Tringa nebularia) and the bar tailed godwit (Limosa lapponica). In all cases the number of pits is much higher than those mentioned by Hoerschelmann (1972) for the same species. At this stage it is not possible to determine whether this difference comes from a different geographical origin of the birds, from a possible temporal variation, or from differences in the definition of sensory pits. We therefore think that it is better to establish a standardized measurement methodology before using the number of sensory pits as indicators of a bird’s tendency to efficiently practice probing behavior.
To be rigorous, however, it will be necessary to investigate which types of mechanoreceptors are present in the pits, and what are their respective densities. Four distinct morphological types of mechanoreceptors are ordered according to size and complexity: free nerve endings, Merkel cells (often arranged into Merkel corpuscles), Grandry corpuscles, and Herbst corpuscles. All are involved with different facets of mechanoreception: the majority is rapidly adapting, while Merkel cells detect amplitude, Grandry corpuscles velocity and Herbst corpuscles acceleration components in mechanical stimuli (Zweers et al., 1994; Ziolkowski et al., 2022). Accordingly, the identification and the quantification of the mechanoreceptors in the pits are keys to assess the species-specific efficiency of probing behavior. The presence of numerous sensory pits on the beak of the ruddy turnstone (Arenaria interpres) is intriguing as birds belonging to this species do not use probing as a capture behavior. This particularity encourages du Toit (2021) to consider these sensory pits as a plesiomorphic character in this species. Turnstones would have lost the use of touch but kept the corresponding beak structure. This potential loss of function of the touch organ would have occurred relatively recently (Cerny and Natale, 2022), and further investigations of A. interpres could be carried out to check if these birds lack the hypertrophy in the regions of the brain associated with the processing of tactile information from the beak.
Many species (11) in our data set use a mixed pecking-probing strategy, which means shifting from sight to touch organ for prey detection. Eye closure seems a good indicator of the preeminent use of touch sense over visual stimuli. All species using probing behaviors close their eyes even before eye submersion, with the exception of snipes (Gallinago gallinago and Lymnocryptes minimus) that close their eyes at submersion, and the oystercatcher (Haematopus ostralegus) that keeps its eyes open underwater. Careful examination of the transport stage indicates that in species using ballistic transport, individuals close their eyes when the prey encounters the tongue at the end of the transport process. We suggest that at this time the touch stimuli become super important so that the prey is oriented in a good way to enter the pharynx. At this point, all of the bird’s attention would be focused on the stimuli coming from the mechanoreceptors, causing the eyes to be “turned off”.
The use of visual vs. touch stimuli during capture in shorebirds can look somewhat controversial. It is clear that many probing individuals do not randomly probe the substrate, but rather use visual stimuli to guide their research during foraging in such a way that their locomotion behavior is oriented (e.g., van der Kam et al., 2004; Ersoy et al., 2022). Many visual cues are left by buried preys on the surface of the substrate e.g., on mudflats (see van der Kam et al., 2004 for excellent illustrations), and oystercatchers (Haematopus ostralegus) for instance are experts in their use and will probe by digging with their beak around these cues (Hulscher, 1996). However, locomotion and capture are clearly two distinct facets of foraging, so here we consider that the sensory organ that guides the prey capture is the very last one used by the bird before its beak closes on the prey. The recent work of Ersoy et al. (2022) on a wintering population of red knots (Calidris canutus) very convincingly demonstrates that there is intraspecific variation in a personality trait, namely a bimodal distribution of individuals’ ability to explore their environment. The exploration abilities of a sample of individuals were scored in an experimental setting and both their food acquisition behavior and their diet were monitored by video recording and stable isotope analyses of droppings, respectively. Scores of slow and fast explorer individuals were shown to remain consistent over time. Results show that slow explorers feed mainly on deeply buried prey that they detect by random probing, whereas fast explorers feed on preys caught near the substrate surface that they detect by probing near visible cues of prey presence. There is thus a difference in the probing behavior, either random or guided by sight. The examination of the capture phase on the videos published by Ersoy et al. (2022) indeed confirms that both slow and fast explorers caught their preys by probing.
4.3 Food handling and transport
Prey handling and transport follow prey capture. As previously mentioned, handling is an extremely plastic behavior that depends both on the environmental and social context, and on the condition of each individual (e.g., age, personality, sex). Accordingly, we believe that this behavior is not only very difficult to categorize, but it remains difficult to assess, which therefore means that it cannot be divided in standardized performances (sensu Irshick and Higham (2016). However, we think that it is perhaps possible to find invariants as to the maximum size of piece of prey that constitutes a mouthful for an individual, or as to the maximum handling time that it will invest in attempts to cut pieces (e.g., appendices of crabs) or to access soft consumable flesh (e.g., mollusks). Finding these invariants requires accumulating more precise data than we currently have. It would of course be necessary here to rely on the many very interesting studies which demonstrate the usefulness of Optimal Foraging Theory in understanding the choices made by individuals depending on the environmental context (energy intake and density of prey, presence of potential competitors) and their condition (sex, age, physical condition) (see e.g., van der Kam et al., 2004 for a clever introduction to this topic).
Immediately after prey capture, a successful individual may encounter attempts at kleptoparasitism, both by conspecifics and heterospecifics. We did not measure the frequency of occurrence of these behaviors, which seem rather infrequent. However, it would be interesting to measure their success rates, and to see to what extent they generate an intra- and interspecific hierarchy. During kleptoparasitism attempts, individuals move with their prey in their beaks to escape their competitors. Such movements are also observed when birds search for a particular substrate. It can be a hard substrate for shredding the prey, like oystercatchers looking for “anvils”, i.e., hard rocks to hammer mussels (Hulscher, 1996). It may also be a search for water, perhaps to wash the prey as is often indicated in the literature, but more likely to take advantage of a lubricant that facilitates the transport of the prey from the tip of the beak to the pharynx (Burton, 1974) by surface tension (Rubega and Obst, 1993; Rubega, 1997; Estrella et al., 2007; Prakash et al., 2008). This use of water as lubricant during transport seems however not restricted to small preys enclosed in a drop of water but may also occur with larger preys – this point deserves further investigation. Another black box at this point is the use of the tongue during transport.
Here we demonstrated that shorebirds use three major prey transport: (i) lingual feeding, (ii) surface tension, and (iii) ballistic transport. This is the first time that this last transport mechanism is recorded in shorebirds although a lot of pictures in the literature clearly demonstrate this feeding pattern primarily determined in birds exploiting fruits and large invertebrate and vertebrate prey (Baussart et al., 2009; Baussart and Bels, 2011). The prey is freely moved between the upper and lower beaks by one or several transport cycles regardless their size, volume and mass. The prey is generally brought to the tongue, and as soon as contacts with the tongue occurs it is moved toward the pharynx by lingual cycles. Despite a large diversity of shapes (Burton, 1974), the tongue plays a key role in food transport either being the only one involved during lingual transport, or at the final phase of ballistic transport and also surface tension (Bels et al., 2023). Surface tension and capillarity have been determined a one specialized mechanism relatively widespread in a lot of shorebirds exploiting small prey at the surface of the water and captured in water and humid sediments (Rubega and Obst, 1993; Rubega, 1997; Estrella et al., 2007; Prakash et al., 2008). Alternatively, some species (e.g. Phalaropus sp.) are able to use ballistic transport for large food items (see Supplementary Material in Bels et al., 2023). For those species that use ballistics or surface tension, it should be noted that the length of their tongue is less or even much less than the size of the beak (Burton, 1974). The elongation of the beak has probably occurred in a decoupled manner independent of tongue size and shape in relationship with foraging behavior associated with prey selection. Beak elongation can be associated with bill curvature for probing and capturing infaunal prey hidden in the sediments (e.g. Numenius spp.) or for capturing prey at the water surface (e.g. Recuvirostra avocetta). If food acquisition is optimized by beak elongation, these birds have to use a « novel » transport behaviors as (i) ballistic transport and (ii) surface tension, although the question of the evolution of ballistic transport in birds remains to be clarified (Bels et al., 2023). In anyway, both transport behaviors in shorebirds permit to move the prey from the tip of the beak toward the pharyngeal cavity and swallowing can occur by lingual action as in all birds (Rico-Guevara et al., 2019). This suggests an adaptive functional response (use of water or not) in the food transport in these shorebirds. However, two major properties limit the use of surface tension and capillarity: (i) size/volume of the prey, and (ii) the need to evacuate the water used as lubricant, in the form of drops or droplets that are ejected as soon as the lingual prey transport occurs. Water ejection seems based on gravitational mechanism, and either falls on the substrate or is mixed with water as soon as the bird enters again the beak in water for the next pecking/probing event. For those species using surface tension transport, and especially snipes, to what extent the tongue could act as a piston generating a pump effect remains to be determined.
4.4 A food acquisition syndrome
Careful examination of Table 1 and Figure 12 indicates the existence of a behavioral syndrome of food acquisition in shorebirds. There are indeed covariations between the three behaviors that can be associated with performances sensu Irshick and Higham (2016), i.e., locomotion, capture and transport, and there is support for some phylogenetic origin for these syndromes. All the species using a stop-run-stop locomotion behavior (5) use pecking as capture behavior and use lingual transport. These species are all belonging to the Charadri suborder, and more specifically to the Charadriidae, which is an ancient clade according to the time-dated phylogeny of Cerny and Natale (2022). The three remaining Charadri species of our data set use continuous walking as locomotion behavior. The two of them that are the only ones in our dataset to use sweeping as capture behavior are the two representatives of the Recuvirostridae, which have the most recent origin according to the time-dated phylogeny of Cerny and Natale (2022). All species members of the Scolopaci suborder using a mixed capture strategy combining pecking and probing (10) move using continuous walking as locomotion behavior, and all but one (Numenius arquata) use a mixed transport strategy combining ballistic and surface tension transport behaviors. The snipes that are the two species using exclusively probing as capture behavior are also the two species using exclusively surface tension as transport behavior.
Documenting such patterns of covariation among behaviors belonging to the different stages of food acquisition offers a key framework to understand the evolution of shorebirds. The huge variation in morphological structures associated with food acquisition among shorebird species, especially beak length and shape does not translate into a huge variety of behaviors. Besides, this variation in morphological structures (beak and legs) occur similarly in recent Charadri and in Scolopaci that share locomotion, capture and transport behaviors. The existence of behavioral syndromes involving a small number of behaviors we highlight here provides evidence of differences between the evolution speed of morphological structures and behaviors involved in food acquisition. It is possible that rapid evolution of the beak and leg morphology became key innovations of shorebird adaptive radiation for optimizing their food acquisition behavior. Such rapid evolution could be driven either by phenotypic plasticity or by genetic differentiation as shown either in some invasive birds for prey selection (Le Gros et al., 2016) or under environmental constrains (Lamichhaney et al., 2015). However, food acquisition seems to explain only ca. 10% of beak shape variation when all birds are considered (Navalón et al., 2019; Van Wassenbergh and Baeckens, 2019). This point might be elucidated by (1) collecting morphological and behavioral data on a larger sample of species and (2) performing in-depth quantitative comparisons of morphological structures in a phylogenetic context.
4.5 Conclusions
Our paper is mainly descriptive, but we are persuaded that it will serve as building blocks to quantitative analyses, like those on our research agenda, i.e., the investigation of relationships between functional morphology and behaviors in a phylogenetic framework. or the evolution of proto sociality in multi-specific groups of shorebirds that should be favored by the partage of food resources. Accordingly, we wanted to provide here a classification of the behaviors involved in food acquisition using what was called ethograms for dozens of years. Doing that, we follow the sound recommendation of Burghardt (2020): thorough descriptions of study systems rooted in natural history are essential prerequisites to any quantitative analyses of ecological and evolutionary processes.
Data availability statement
The raw data supporting the conclusions of this article will be made available by the authors, without undue reservation.
Ethics statement
Ethical approval was not required for the study involving animals in accordance with the local legislation and institutional requirements because Ethical approval for bird observations not required.
Author contributions
MB: Conceptualization, Data curation, Formal analysis, Funding acquisition, Investigation, Methodology, Project administration, Resources, Supervision, Writing – original draft, Writing – review & editing. GL: Data curation, Formal analysis, Investigation, Methodology, Writing – review & editing. LH: Data curation, Formal analysis, Investigation, Methodology, Writing – review & editing, Writing – original draft. FK: Data curation, Investigation, Methodology, Visualization, Writing – review & editing. NS: Data curation, Formal analysis, Methodology, Visualization, Writing – review & editing. VS: Conceptualization, Formal analysis, Investigation, Methodology, Visualization, Writing – review & editing. VB: Conceptualization, Data curation, Formal analysis, Funding acquisition, Methodology, Project administration, Visualization, Writing – original draft, Writing – review & editing.
Funding
The author(s) declare financial support was received for the research, authorship, and/or publication of this article. This research was funded by the Agence National de la Recherche, grant number ANR-10-LABX-41, France, to MB and VS and by the ISYEB UMR 7205 via internal grants to MB and VB.
Acknowledgments
MB and VS are members of the Labex TULIP and acknowledge support from the CHANGE Team of the CNRS UAR 2029.
Conflict of interest
The authors declare that the research was conducted in the absence of any commercial or financial relationships that could be construed as a potential conflict of interest.
The author(s) declared that they were an editorial board member of Frontiers, at the time of submission. This had no impact on the peer review process and the final decision.
Publisher’s note
All claims expressed in this article are solely those of the authors and do not necessarily represent those of their affiliated organizations, or those of the publisher, the editors and the reviewers. Any product that may be evaluated in this article, or claim that may be made by its manufacturer, is not guaranteed or endorsed by the publisher.
References
Altmann J. (1974). Observational study of behaviour: Sampling methods. Behaviour 49, 227–226. doi: 10.1163/156853974X00534
Angarita-Baez J., Carlos C. (2023). Foraging behavior of migratory shorebirds during non-breeding periods in the Americas: a review. Ornithology Res. 31, 141–151. doi: 10.1007/s43388-023-00125-5
Barbosa A. (1994). Estudio ecomorfologico de las aves limicolas (Avec : Charadri) (Facultad de ciencias biologicas: Modificaciones adaptivas relacionadas con la busqueda de alimento. Universidad complutense de Madrid), 166.
Barbosa A., Moreno E. (1999). Evolution of foraging strategies in shorebirds: an ecomorphological approach. Auk 116, 712–725. doi: 10.2307/4089332
Barlow G. W. (1977). “Modal action patterns,” in How animals communicate. Ed. Sebeok T. A. (Bloomington: Indiana University Press), 98–134.
Baussart S., Bels V. (2011). Tropical hornbills (Aceros cassidix, Aceros undulatus, and Buceros hydrocorax) use ballistic transport to feed with their large beaks. J. Exp. Zoology Part A: Ecol. Genet. Physiol. 315, 72–83. doi: 10.1002/jez.590
Baussart S., Korsoun L., Libourel P. A., Bels V. (2009). Ballistic food transport in toucans. J. Exp. Zoology Part A: Ecol. Genet. Physiol. 311, 465–474. doi: 10.1002/jez.542
Beer C. (2020). Niko Tinbergen and questions of instinct. Anim. Behav. 164, 261–265. doi: 10.1016/j.anbehav.2019.08.005
Bels V., Lefloch G., Kirchoff F., Davenport J., Baguette M. (2023). Food transport in Reptilia: a comparative viewpoint. Philos. Trans. R. Soc. B 378, 20220542. doi: 10.1098/rstb.2022.0542
Bels V., Whishaw I. Q. (2019). Feeding in vertebrates: evolution, morphology, behavior, biomechanics (Cham, Switzerland: Springer International Publishing), 883.
Billerman S. M., Keeney B. K., Rodewald P. G., Schulenberg T. S. (Eds.) (2022). Birds of the world (Ithaca, NY, USA: Cornell Laboratory of Ornithology). Available at: https://birdsoftheworld-org.mnhn.idm.oclc.org/bow/home.
Bels V. L., Pallandre J.-P., Pelle E., Kirchhoff F. (2022). Studies of the behavioral sequences: The neuroethological morphology concept crossing ethology and functional morphology. Animals 12, 1336. doi: 10.3390/ani12111336
Bolze G. (1968). Anordnung und Bau der Herbstschen Körperchen in Limicolenschnäbeln im Zusammenhang mit der Nahrungsfindung. Zoologischer Anzeiger 181, 313–355.
Burghardt G. M. (2020). Insights found in century-old writings on animal behaviour and some cautions for today. Anim. Behav. 164, 241–249. doi: 10.1016/j.anbehav.2020.02.010
Burghardt G. M., Bartmess-LeVasseur J. N., Browning S. A., Morrison K. E., Stec C. L., Zachau C. E., et al. (2012). Minimizing observer bias in behavioral studies: A review and recommendations. Ethology 118, 511e517. doi: 10.1111/j.1439-0310.2012.02040.x
Burton P. J. K. (1974). Feeding and feeding apparatus in waders (London: British Museum of Natural History Publication No. 719), 150.
Burton P. J. K. (1986). Curlews' Numenius bills: some anatomical notes. Bird Study 33, 70. doi: 10.1080/00063658609476897
Cerny D., Natale R. (2022). Comprehensive taxon sampling and vetted fossils help clarify the time tree of shorebirds (Aves, Charadriiformes). Mol. Phylogenet. Evol. 177, 107620. doi: 10.1016/j.ympev.2022.107620
Colwell M. A. (2010). Shorebird ecology, conservation, and management (Berkeley: University of California Press), 344.
Cramp S., Simmons K. E. L. (1983). “Handbook of the birds of europe, the middle east and North Africa,” in Waders to gulls, vol. III. (Oxford: Oxford University Press).
du Toit C. (2021). Mechanosensory structures in the beaks of probe-foraging birds in relation to their foraging ecology (Cape Town: FitzPatrick Institute of African Ornithology, University of Cape Town), 277. PhD thesis.
Elner R. W., Beninger P. G., Jackson D. L., Potter T. M. (2005). Evidence of a new feeding mode in western sandpiper (Calidris mauri) and dunlin (Calidris alpina) based on bill and tongue morphology and ultrastructure. Mar. Biol. 146, 1223–1234. doi: 10.1007/s00227-004-1521-5
Ersoy S., Beardsworth C., Dekinga A., van der Meer M. T. J., Piersma T., Groothuis T. G. G., et al. (2022). Exploration speed in captivity predicts foraging tactics and diet in free-living red knots. J. Anim. Ecol. 91, 356–366. doi: 10.1111/1365-2656.13632
Estrella S. M., Masero J. A., Pérez-Hurtado A. (2007). Small-prey profitability: Field analysis of shorebirds’ use of surface tension of water to transport prey. Auk 124, 1244–1253. doi: 10.1093/auk/124.4.1244
Friard O., Gamba M. (2016). BORIS: a free, versatile open-source event-logging software for video/audio coding and live observations. Methods Ecol. Evol. 7, 1325–1330. doi: 10.1111/2041-210X.12584
Gill F., Donsker D., Rasmussen P. (2023). “Sandpipers, snipes, coursers,” in IOC World Bird List Version 13.2 (International Ornithologists' Union). Available at: https://www.worldbirdnames.org/new/ioc-lists/master-list-2/.
Hamilton R. B. (1975). Comparative behavior of the American avocet and the black-necked stilt (Re- curvirostridae) (Lawrence,Kansas: Allen Press, Ornithological Monographs No. 17).
Heiss E., Gignac P. M., Porro L. B., Lemell P. (2023). “Convergence of aquatic feeding modes in the Sauropsida (Crocodiles, Birds, Lizards, Snakes and Turtles),” in Convergent Evolution: Animal Form and Function. Eds. Bels V. L., Russell A. P. (Cham: Springer International Publishing), 141–181.
Hoerschelmann H. (1972). Strukturen der Schnabelkammer bei Schnepfenvögeln (Charadriidae und Scolopacidae). Z. für wissenschaftliche Zoologie 185, 105–121.
Hulscher J. B. (1996). “Food and feeding behaviour,” in The Oystercatcher. From individuals to populations. Ed. Gross-Custard J. D. (Oxford: Oxford University Press), 7–29.
Irshick D. J., Higham T. E. (2016). Animal Athletes: an ecological and evolutionary approach (Oxford: Oxford University Press), 255.
Jing K., Ma Z., Li B., Li. J., Chen J. (2007). Foraging strategies involved in habitat use of shorebirds at the intertidal area of Chongming Dongtan, China. Ecol. Res. 22, 559–570. doi: 10.1007/s11284-006-0302-7
Kuwae T., Beninger P. G., Decottignies P., Mathot K. J., Lund D. R., Elner R. W. (2008). Biofilm grazing in a higher vertebrate: the Western sandpiper, Calidris mauri. Ecology 89, 599–606. doi: 10.1890/07-1442.1
Lamichhaney S., Berglund J., Almén M. S., Maqbool K., Grabherr M., Martinez-Barrio A., et al. (2015). Evolution of Darwin’s finches and their beaks revealed by genome sequencing. Nature 518, 371–375. doi: 10.1038/nature14181
Le Gros A., Samadi S., Zuccon D., Cornette R., Braun M. P., Senar J. C., et al. (2016). Rapid morphological changes, admixture and invasive success in populations of Ring-necked parakeets (Psittacula krameri) established in Europe. Biol. Invasions 18, 1581–1598. doi: 10.1007/s10530-016-1103-8
Letunic I., Bork P. (2021). Interactive tree of life (iTOL) v5: an online tool for phylogenetic tree display and annotation. Nucleic Acids Res. 49, W293–W296. doi: 10.1093/nar/gkab301
Lin C.-T. (2022). Morphology of the feeding apparatus in shorebirds (Charadriiformes): a comparative analysis (Palmerston North: Massey University), 113. MSc thesis.
Mathot K. J., Piersma T., Elner R. (2019). “Shorebirds as integrators and indicators of mudflat ecology,” in Mudflat Ecology. Aquatic Ecology Series, vol. 7 . Ed. Beninger P. G. (Cham: Springer International Publishing), 309–338.
Montuelle S. J., Kane E. A. (2019). “Food capture in vertebrates: a complex integrative performance of the cranial and postcranial systems,” in Feeding in vertebrates: evolution, morphology, behavior, biomechanics. Eds. Bels V. L., Wishaw I. (Cham: Springer International Publishing), 71–137.
Navalón G., Bright J. A., Marugán-Lobón J., Rayfield E. J. (2019). The evolutionary relationship among beak shape, mechanical advantage, and feeding ecology in modern birds. Evolution 73, 422–435. doi: 10.1111/evo.13655
Nebel S., Jackson D. L., Elner R. W. (2005). Functional association of bill morphology and foraging behaviour in calidrid sandpipers. Anim. Biol. 55, 235–243. doi: 10.1163/1570756054472818
Nol E., MacCulloch K., Pollock L., McKinnon L. (2014). Foraging ecology and time budgets of non-breeding shorebirds in coastal Cuba. J. Trop. Ecol. 30, 347–357. doi: 10.1017/S0266467414000182
Novcic I. (2016). Niche dynamics of shorebirds in Delaware Bay: foraging behavior, habitat choice and migration timing. Acta Oecologica 75, 68–76. doi: 10.1016/j.actao.2016.07.002
Novcic I. (2019). Weak niche partitioning by migrating shorebirds in a single-food-type environment. Avian Biol. Res. 12, 109–117. doi: 10.1177/1758155919841279
Pallandre J. P., Lavenne F., Pellé E., Ortiz K., Bels V. L. (2023). “The sacro-iliac joint of the Felidae and Canidae and their large ungulate prey: An Example of Divergence and Convergence,” in Convergent Evolution: Animal Form and Function. Eds. Bels V. L., Russell A. P. (Cham: Springer International Publishing), 79–114.
Piersma T., van Aelst R., Kurk K., Berkhoudt H., Maas L. R. M. (1998). A new pressure sensory mechanism for prey detection in birds: the use of principles of seabed dynamics? Proc. R. Soc. B 265, 1377–1383. doi: 10.1098/rspb.1998.0445
Piersma T., van Gils J. A. (2011). The flexible phenotype: a body-centred integration of ecology, physiology, and behaviour (Oxford: Oxford University Press), 238.
Prakash M., Quéré D., Bush J. W. (2008). Surface tension transport of prey by feeding shorebirds: the capillary ratchet. Science 320, 931–934. doi: 10.1126/science.1156023
Rico-Guevara A., Sustaita D., Gussekloo S., Olsen A., Bright J., Corbin C., et al. (2019). “Feeding in birds: thriving in terrestrial, aquatic, and aerial niches,” in Feeding in vertebrates: Evolution, morphology, behavior, biomechanics. Eds. Bels V. L., Russell A. P. (Cham: Springer International Publishing), 643–693.
Rubega M. A. (1997). Surface tension prey transport in shorebirds: how widespread is it? Ibis 139, 488–493. doi: 10.1111/j.1474-919X.1997.tb04663.x
Rubega M. A., Obst B. S. (1993). Surface-tension feeding in phalaropes: discovery of a novel feeding mechanism. Auk 110, 169–178.
Schleidt W. M. (1974). How “fixed” is the fixed action pattern? Z. für Tierpsychologie 36, 184–211. doi: 10.1111/j.1439-0310.1974.tb02131.x
Thomas T., Székely R. F., Cuthill I. C. (2006). Eye size, foraging methods and the timing of foraging in shorebirds. Funct. Ecol. 20, 157–165. doi: 10.1111/j.1365-2435.2006.01073.x
Tinbergen N. (2020). “The study of instinct,” (Cambridge: Pygmalion Press, an imprint of Plunkett Lake Press), 388.
van der Kam J., Ens B., Piersma T., Zwarts L. (2004). Shorebirds. An illustrated behavioural ecology (Utrecht: KNNV Publishers).
Van Wassenbergh S., Baeckens S. (2019). Digest: Evolution of shape and leverage of bird beaks reflects feeding ecology, but not as strongly as expected. Evolution 73, 621–622. doi: 10.1111/evo.13686
Ziolkowski L. H., Gracheva E. O., Bagriantsev S. N. (2022). Tactile sensation in birds: physiological insights from avian mechanoreceptors. Curr. Opin. Neurobiol. 74, 102548. doi: 10.1016/j.conb.2022.102548
Zweers G. A. (1991). Transformation of avian feeding mechanisms. A deductive method. Acta Biotheoretica 39, 15–36. doi: 10.1007/BF00046405
Zweers G. A., Berkhoudt H., Vanden Berge J. E. (1994). “Behavioral mechanisms of avian feeding,” in Biomechanics of Feeding in Vertebrates Advances in Comparative and Environmental Physiology, vol. 18 . Eds. Bels V. L., Chardon M., Vandewalle P. (Berlin, Heidelberg: Springer), 241–279.
Zweers G. A., Gerritsen A. F. C. (1997). Transitions from pecking to probing mechanisms in waders. Netherlands J. Zoology 47, 167–207.
Keywords: interspecific competition, character displacements, behavioral syndrome, foraging, pecking, probing, sweeping, transport
Citation: Baguette M, Le Floch G, Hannier L, Kirchhoff F, Schtickzelle N, Stevens VM and Bels V (2024) Repertoire of food acquisition behaviors in Western Palearctic shorebirds (Aves, Charadriiformes). Front. Ethol. 3:1351994. doi: 10.3389/fetho.2024.1351994
Received: 07 December 2023; Accepted: 25 January 2024;
Published: 12 February 2024.
Edited by:
Rulon Clark, San Diego State University, United StatesReviewed by:
Caio J. Carlos, Federal University of Rio Grande do Sul, BrazilRonald Ydenberg, Simon Fraser University, Canada
Copyright © 2024 Baguette, Le Floch, Hannier, Kirchhoff, Schtickzelle, Stevens and Bels. This is an open-access article distributed under the terms of the Creative Commons Attribution License (CC BY). The use, distribution or reproduction in other forums is permitted, provided the original author(s) and the copyright owner(s) are credited and that the original publication in this journal is cited, in accordance with accepted academic practice. No use, distribution or reproduction is permitted which does not comply with these terms.
*Correspondence: Michel Baguette, bWljaGVsLmJhZ3VldHRlQG1uaG4uZnI=