- 1Department of Earth and Biological Sciences, Loma Linda University, Loma Linda, CA, United States
- 2Department of Biology, California State University San Bernardino, San Bernardino, CA, United States
Social buffering comprises the reduction of stress an organism experiences when in the presence of a companion and has been well documented in highly social animals, such as birds and mammals. To date, it has not been reported in reptiles. Rattlesnakes are cryptically social, exhibiting kin recognition and forming subtle social networks in certain contexts. We tested for the presence of social buffering against an acute stressor in 25 wild-caught adult southern Pacific rattlesnakes (Crotalus helleri) by measuring heart rate increase during a standardized disturbance when alone, in the presence of a rope (inanimate object control), and in the presence of a same-sex companion. We further tested for a greater proclivity of social buffering in montane snakes, which overwinter communally, than in lowland snakes, which mostly overwinter alone; and for a greater proclivity in females, which often aggregate during gestation and maternally attend their offspring. Results indicated that the presence of a companion significantly reduced emotional tachycardia (change in heart rate) after disturbance compared to the other treatments. We detected no difference in social buffering proclivity between montane and lowland populations, but lowland snakes exhibited a subdued acute stress response across treatments and had a longer heart rate recovery time. No difference in emotional tachycardia existed between the sexes. This novel study can benefit our understanding of social buffering, including its expression, adaptive roles, and practical applications for captive animals.
Introduction
Stress occurs when organisms experience unpredictable or adverse conditions in their environment (Wingfield, 2005). During these events, the hypothalamic-pituitary-adrenocortical (HPA) axis becomes activated, releasing glucocorticoid hormones such as cortisol and/or corticosterone (CORT) into the blood (Wingfield and Romero, 2001). Elevation of these hormones modulates the stress response, resulting in shifts in the function of the autonomic nervous system, neuroendocrine responses, and immune responses, and behavioral shifts that promote antipredator behavior (Silvestre, 2014). The amount of stress an animal is experiencing can be indicated by measuring CORT levels and/or other physiological responses such as elevation in heart rate (HR), or certain behavioral responses (Sapolsky et al., 2000).
Stress responses can comprise two categories: acute and chronic (Dickens and Romero, 2013). Acute stressors are short-term, such as a storm, or an encounter with a predator or aggressive conspecific, resulting in immediate physiological or behavioral responses (Sapolsky et al., 2000; Dickens and Romero, 2013) modulated by the sympathetic nervous system (Silvestre, 2014), which temporarily suspend normal life functions (Wingfield et al., 1998). Chronic stress occurs over a longer duration under unfavorable conditions, leading to extended exposure to high levels of glucocorticosteroids (Wingfield et al., 1998; Dickens and Romero, 2013). During this lengthy period, systems nonessential to long-term survival become suppressed, which can result in increased risk of disease due to reduced immune function and decreased reproductive success (Wingfield et al., 1998; Sapolsky et al., 2000; Bonier et al., 2009).
The presence of a conspecific during an encounter with a stressor can modulate the response of the HPA axis, which comprises a health-promoting effect called social buffering (Hennessy et al., 2009). Social buffering is well documented in highly social animals, such as fishes, birds, mammals, non-human primates, humans, and a single invertebrate group, termites (reviewed by Kikusui et al., 2006; Tian et al., 2017; Culbert et al., 2019). Various factors influence the ability of a partner to buffer stress, including relationship to the partner, group organization within a species, nature and presentation of the stress cue, number of individuals present, and emotional status of the partner (Hennessy et al., 2009). Companions can buffer various stress responses, such as HR, fear-related behaviors, changes in CORT levels, and immunoreactivity (Kikusui et al., 2006). A similar response has not been reported in reptiles, and to our knowledge, no documentation exists of asocial organisms or solitary foragers exhibiting social buffering.
As solitary foragers, snakes are typically considered asocial (Burghardt et al., 2009; Doody et al., 2013). However, mounting evidence suggests that snakes, including pitvipers, exhibit cryptic and sometimes complex behaviors indicative of social interactions (reviewed in rattlesnakes by Schuett et al., 2016; Doody et al., 2021). During the mating season, males engage in male-male combat to establish dominance, with the dominant male gaining priority access to mate with the female (Shine, 1978; Gillingham et al., 1983; Carpenter, 1984). Male western diamondback rattlesnakes (Crotalus atrox) have been observed mate-guarding females (O’Leile et al., 1994; Clark et al., 2014), which likely occurs in other rattlesnake species as well (Bishop et al., 1996; Goode and Wall, 2002; Glaudas and Rodriguez-Robles, 2011). Female pitvipers frequently aggregate during gestation (Graves and Duvall, 1993; Graves and Duvall, 1995; Greene et al., 2002), with at least one species (Crotalus horridus) forming aggregations consisting of relatives (Clark et al., 2012), as pitvipers are capable of kin recognition (Clark, 2004; Hoss et al., 2015). However, female aggregation during gestion appears to be absent in some well-studied rattlesnake populations (Schuett et al., 2016). After birth, female pitvipers frequently maternally attend their young until the neonates shed their skin the first time (reviewed by Greene et al., 2002; Schuett et al., 2016). During maternal attendance, neonates may develop sex-specific affiliative behavior with their kin (Hoss et al., 2015). In cold regions, snakes often aggregate at denning sites for overwintering (Graves and Duvall, 1995; Schuett et al., 2016), and neonate rattlesnakes appear capable of following the scent trails of adults to locate dens (Brown and MacLean, 1983; Reinert and Zappalorti, 1988; Cobb et al., 2005; Hileman et al., 2015; Martin, 2019). Arizona black rattlesnakes (Crotalus cerberus) form social networks when aggregating at these communal denning sites (Amarello, 2012), and the existence of social networks in other snakes (Skinner and Miller, 2020; Skinner and Miller, 2022) highlights their potential importance.
Heart rate has been a standard measure of vertebrate stress and is a sensitive indicator of stress levels in reptiles, particularly for acute stress responses, and could therefore be used as an indicator of social buffering (Silvestre, 2014). Elevation in HR, referred to as emotional tachycardia, has been observed after human handling of turtles, lizards, and alligators (Cabanac and Bernieri, 2000; Cabanac and Cabanac, 2000; Young et al., 2020). These studies concluded that handling elicited a fear/startle response, which caused elevation in HR. Snakes also exhibit an increase in HR when handled (Stinner and Ely, 1993; Hayes et al., 1994) or exposed to a threatening stimulus, such as being tapped on the mid-body or exposed to conspecific musk secretions (a common defensive reaction) followed by human breath (Graves and Duvall, 1988). Defensive behavior can also be used to indicate the level of acute stress and can be readily measured in rattlesnakes, which are known for their elaborate defensive displays that include head-elevated coiling, tail-rattling, striking, and venomous bites (Greene, 1988; Shipman, 2002). Free-ranging cottonmouths (Agkistrodon piscivorus) that had elevated levels of baseline CORT were more likely to strike than to perform a threat display when approached, indicating that baseline stress levels influenced defensive behavior (Herr et al., 2017; but see Claunch et al., 2017). Due to their defensive displays and extensive social behaviors, especially in the vicinity of dens, rattlesnakes are ideally suited to answer many questions regarding social buffering in reptiles, a relatively unstudied group.
In this study, we used HR and defensive behavior to evaluate the ability of southern Pacific rattlesnakes (C. helleri) to use social buffering to alleviate acute stress. Southern Pacific rattlesnakes occur commonly in Southern California and occupy diverse habitats from sea level to high mountain elevations (Klauber, 1972). Proclivities toward communal overwintering at denning sites appear to be greater in C. helleri populations at higher elevations (Klauber, 1972; Dugan et al., 2008; Hayes, pers. obs.; Brian Hinds, pers. comm.), presumably resulting in higher levels of conspecific behavioral interactions during fall ingress and spring egress (Fitch, 1949; Clark et al., 2014) compared to populations at lower elevations that more often overwinter alone. This population difference provides a unique opportunity to examine whether increased interactions resulting from denning behavior has an impact on social buffering.
We experimentally tested three hypotheses. First, because rattlesnakes possess cryptic sociality, we predicted that snakes exhibit social buffering, as inferred by a reduction in defensive behavior along with a decreased change in HR after exposure to an acute stressor when in the presence of a conspecific. Second, if denning behavior influences social buffering proclivities, we predicted that snakes from denning populations (montane) will have a greater social buffering response than those from non-denning populations (lowland). Third, because females tend to aggregate during gestation, we predicted that females have a greater social buffering response than males.
Methods
Experimental animals
The subjects comprised 25 C. helleri from Southern California, including 11 montane (eight males and three females) and 14 lowland (nine males and five females) wild-caught “nuisance” animals donated to the lab by local snake removal services. These snakes had spent 8–201 days in captivity prior to testing. We classified animals as lowland (142–991 m elevation, in areas where snakes generally overwinter individually) or montane (1077–1986 m elevation in the San Bernardino and San Jacinto mountains, in habitats near or similar to known communal hibernaculum sites). The two exceptions were snakes classified as lowland from a high plateau in Anza (1190, 1306 m) where the chaparral habitat resembles that of lower elevations. We housed the snakes individually in 50 × 40 × 18 cm (L × H × W) plastic containers lined with butcher paper and kept at 26–28°C with a 12:12-h light: dark cycle. Snakes were fed rodents once every two weeks, had daily access to water, and were given at least one week to acclimate to captivity before trials began. After feeding, snakes were given at least one week to digest before being tested. We hibernated captive snakes in a cold room (12–13°C) during the winter months (December–March) to duplicate the natural pattern of activity.
Testing procedure
We tested snakes individually in a 19-L plastic bucket by exposing them to three treatments: alone (negative control), with a rope (inanimate object control), and with a same-sex companion. The rope was 4 cm thick and 95 cm long and cleaned by soaking in a 10% bleach solution between trials. We used one male rattlesnake and one female rattlesnake of similar size as the companion animals for all trials to maximize consistency among trials. We painted the rattles of companion animals with non-toxic nail polish to help distinguish the companion animal from the tested animal during trials. We tested each snake three times, once in each of the three treatment conditions, with the order of trials randomized (but balanced in sequence) and a one-day interval between trials to minimize stress. Testing took place during three successive periods: fall (October-November), summer (June-July), and fall again (October-November). We avoided testing during the early spring and late summer/early fall reproductive periods when antagonistic male-male interactions occur (Klauber, 1972; Dugan et al., 2008). We subjected individual snakes to the three treatments twice, once during the summer and once during one of the fall periods, for a total of six trials. One individual died and was only tested during the summer.
To measure HR, one of us removed a snake from its home enclosure and temporarily restrained it in a tube for safety, while a second investigator affixed button electrodes with conductive gel to the snake using medical tape. We positioned two leads near the heart on the right and left side (ca. 30% of SVL from snout), and one lead caudal to the heart on the left side (ca. 50%). We then attached the leads to a heart rate monitor (Heal Force Prince180B Easy ECG Monitor, Heal Force Bio-meditech, Shanghai, China). Once outfitted with electrodes, the snake was then placed in the bucket with a screw-on lid, along with the treatment stimulus (conspecific or rope) if applicable. The lid had a hole in the center to accommodate the electrode wires and a small acrylic window through which we recorded the snake’s behavior using an iPhone 11 Pro (Apple, Inc., Cupertino, California, USA).
We recorded both handling time and temperature to ensure consistency across trials. Handling can induce stress, so we recorded time to set up trials (3.5–9 min; mean ± 1 SD = 4.8 ± 0.8 min) to assess whether snakes in different groups experienced stress over similar periods of time. Temperature can also influence behavioral responses of ectotherms, so we recorded room temperature (24.9–27.0°C; 25.2 ± 0.4°C) and each snake’s external body temperature (24.4–28.0°C; 26 ± 0.7°C) via a thermal gun (Helect Infrared Laser Thermometer, Shenzhen JEWY Tech Co., Shenzhen, China) before and after trials and calculated the average.
We exited the testing room and gave the snakes 20 min to acclimate to the testing setup, with the HR of the last 30 sec averaged to determine a baseline-period HR. We chose to use 20 min based on observations of the snake’s behavior (i.e., termination of rattling and settling into a coil). After the 20-min acclimation we disturbed the bucket by releasing two hinged rubber band-propelled polyvinyl chloride (PVC) pipes (Figure 1), which struck the side of the bucket 3 sec apart with a standardized force to cause a disturbance. During the next 20 min, we recorded the increase in HR from baseline for 30 sec after the disturbance, time until HR returned to baseline levels, and time spent rattling (via videotape review). We cleaned the bucket between trials with a 10% bleach solution and wiped down the electrode cables with 70% alcohol before rinsing them with deionized water. One of us (CEM) analyzed the electrocardiogram record while blinded to treatment, and videos of behavior using JWatcher (v 1.0) while blinded to snake identity (i.e., source location and sex).
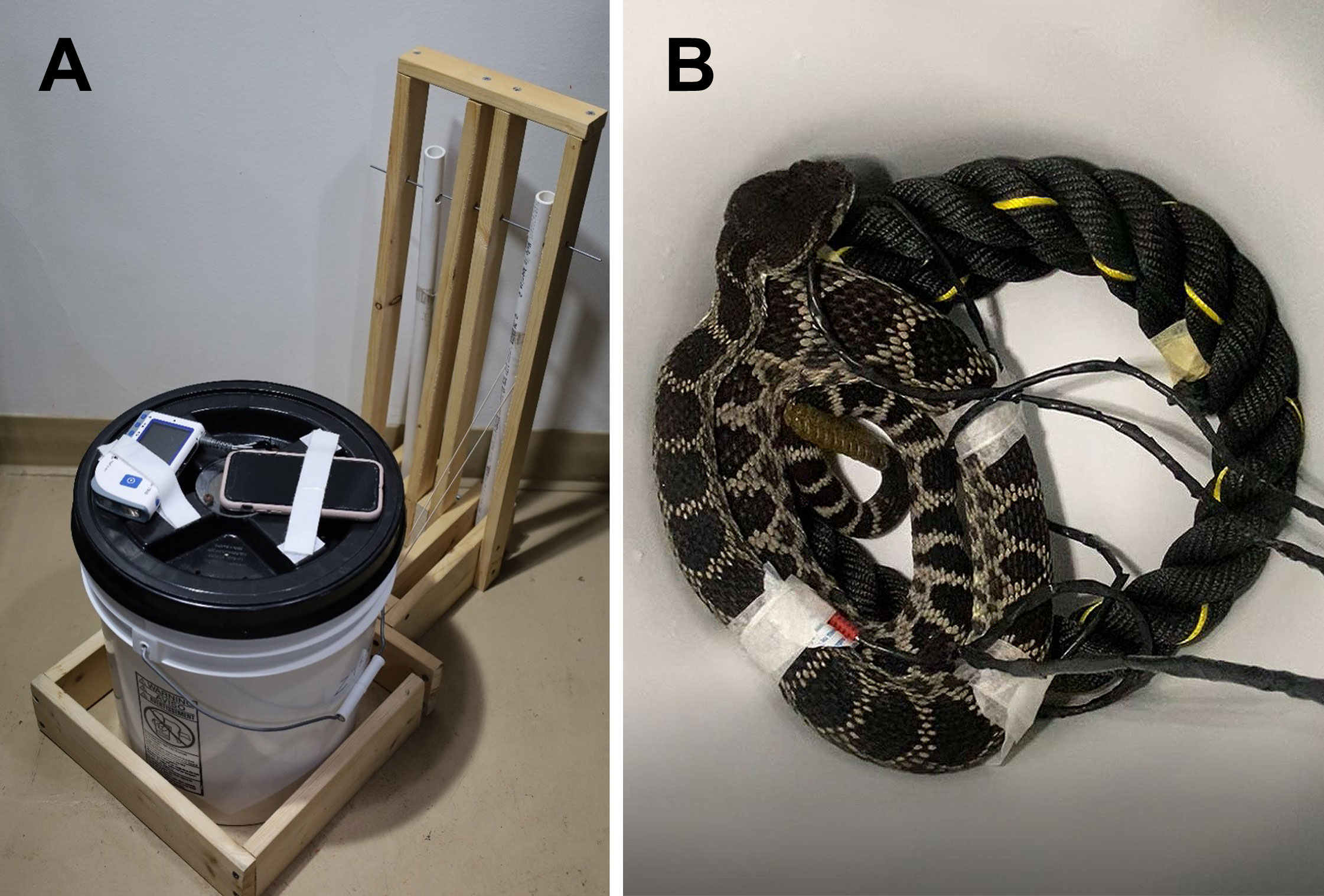
Figure 1 (A) Snakes were individually tested in a 19-L plastic bucket with a screw-on lid outfitted with an acrylic window in the center to view the snake during a trial. An iPhone 11 pro was attached to the lid to record behavior along with an electrocardiography monitor to record heart rate. Wires were fed through a hole in the lid and attached to the snake in the bucket. The bucket was attached to a standardized disturbance apparatus with two hinged rubber band-propelled polyvinyl chloride (PVC) pipes. (B) Southern Pacific rattlesnake (Crotalus helleri) attached to the heart rate monitor via electrodes in the testing bucket with a rope (non-animal control) stimulus.
Statistical analysis
We conducted four tests using R Statistical Software (v4.1.1; R Core Team, 2019), with alpha set to 0.05 and p-values calculated via the ANOVA function in R (car package). Prior to data analysis, we tested variables to ensure that parametric assumptions were met. Percent change of HR and time to baseline HR failed to meet normality assumptions and were therefore square-root-transformed and log-transformed, respectively. Initial analyses revealed that SVL and time in captivity showed no association with any of the dependent variables, and therefore were excluded from all models. We found no difference between the average handling time and temperature of snakes among treatments, so we excluded those variables as well. We further removed non-significant interactions from the final models.
To analyze percent change of HR, we used a linear mixed model (LMM) with the lme4 package (Bates et al., 2015), with treatment (alone, rope, or companion), region (montane or lowland), sex, and baseline HR as fixed factors. We included trial order and snake identity (ID) as random factors to account for repeated measures, and two-way interactions between treatment and baseline HR and between region and baseline HR. Because of a significant interaction between treatment and baseline HR, we conducted three additional post-hoc LMMs to test for pairwise interactions between the treatment combinations and baseline HR and adjusted the p-values for false discovery rate (Benjamini and Hochberg, 1995). We calculated the intraclass correlation coefficient (ICC) for the effect of snake ID (i.e., repeatability of responses within individuals) using the equation ICC = (variance from snake ID)/(variance from snake ID + variance from trial order + residual variance) to estimate how strongly responses varied among individuals. We interpreted ICC values <0.5 to be indicative of low reliability among repeated tests (Koo and Li, 2016).
We used an LMM to examine time to return to baseline HR, with higher values indicative of higher stress. Trial order and snake ID were included as random factors to account for repeated measures, with treatment, region, sex, and percent change in HR included as fixed factors. We also calculated ICC for the effect of snake ID as explained above.
For time spent rattling, we used a generalized LMM with a negative binomial distribution through the glmmTMB package (Brooks et al., 2017) to account for zero inflation from snakes that did not rattle. We included trial order and snake ID as random factors to account for repeated measures, and treatment, region, and sex as fixed factors. Due to a significant main effect of treatment, we conducted a post-hoc analysis and adjusted the p-values for false-discovery rate.
For the companion trials only (49 out of 148), we investigated whether the conspecific partner’s rattling behavior affected the rattling behavior of the focal individual. We analyzed time spent rattling by the focal individual using a generalized LMM with a negative binomial distribution, as above, with trial order and snake ID as random factors to account for repeated measures, and partner time spent rattling included as a fixed factor.
Results
For percent change of HR, baseline HR exerted a significant main effect (F(1,144.79) = 168.89, p < 0.001), with the cardiac response to disturbance becoming weaker at higher baseline HR values. More important, a significant interaction existed between treatment and baseline HR (F(2,124.53) = 4.42, p = 0.014; Figure 2). Post-hoc models examining this interaction with pairwise treatment combinations revealed that snakes at lower baseline HR had a reduced percent change in HR in the companion treatment when compared to the control (p = 0.009) and to the rope (p = 0.004), and no difference occurred between the control and rope treatments (p = 0.650). There was also an interaction between region and baseline HR (F(1,145.32) = 13.60, p < 0.001; Figure 3), with lowland snakes exhibiting a subdued cardiac response to the stressor compared to the montane snakes. There was no significant effect of sex (F(1,24.33) = 0.067, p = 0.797). The ICC showed relatively low repeatability in HR responses within individuals (ICC = 0.34).
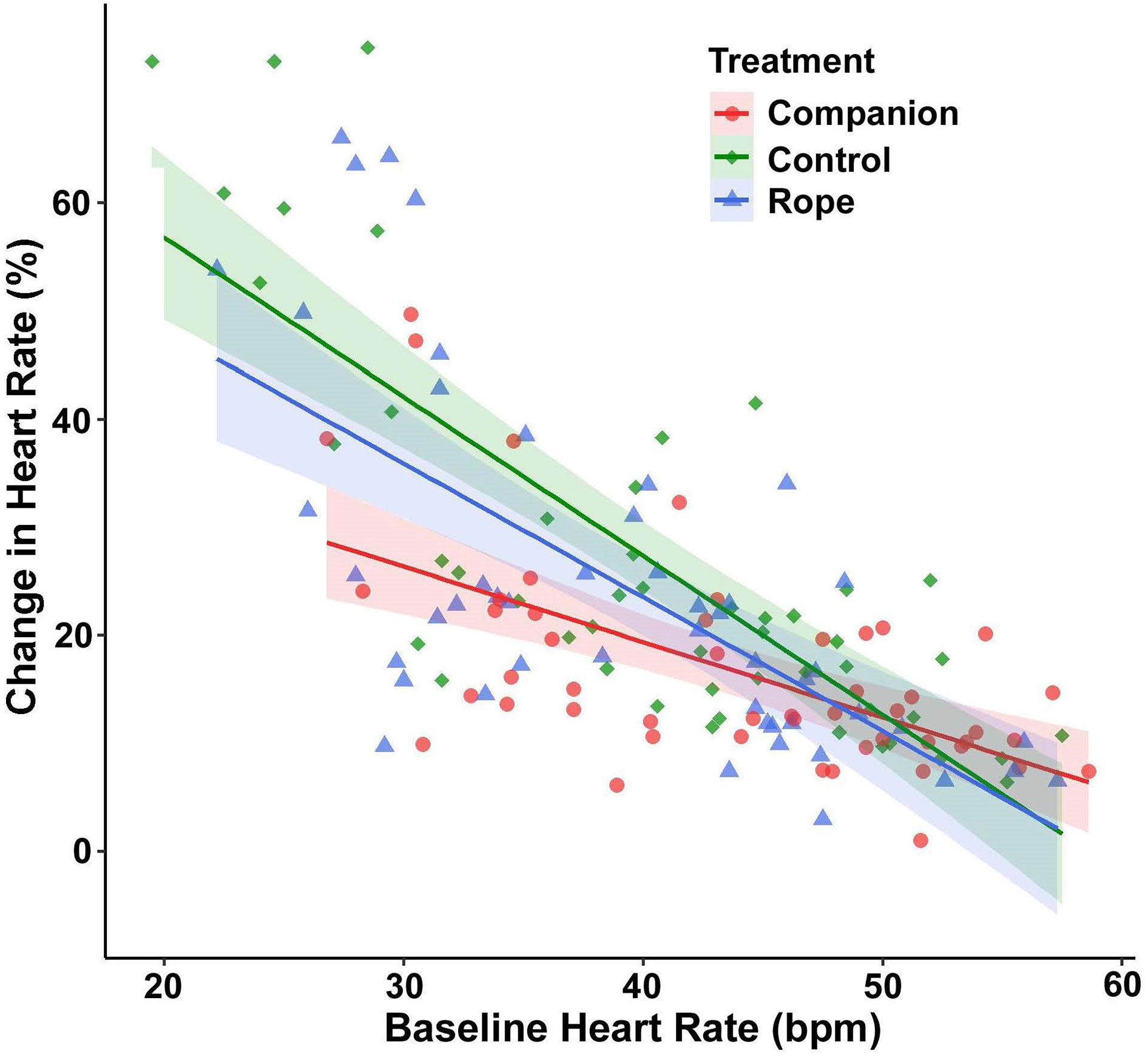
Figure 2 Emotional tachycardia (percent change in heart rate [HR] beats per minute [bpm], with 95% confidence intervals) in adult southern Pacific rattlesnakes (N = 25, each tested three times) following a brief disturbance. An interaction existed between baseline HR and the three treatment conditions for snakes tested alone (negative control), with a rope (inanimate object control), and with a same-sex companion. Snakes at lower baseline HR had a reduced cardiac response in the companion treatment compared to the control and rope treatments, suggesting social buffering.
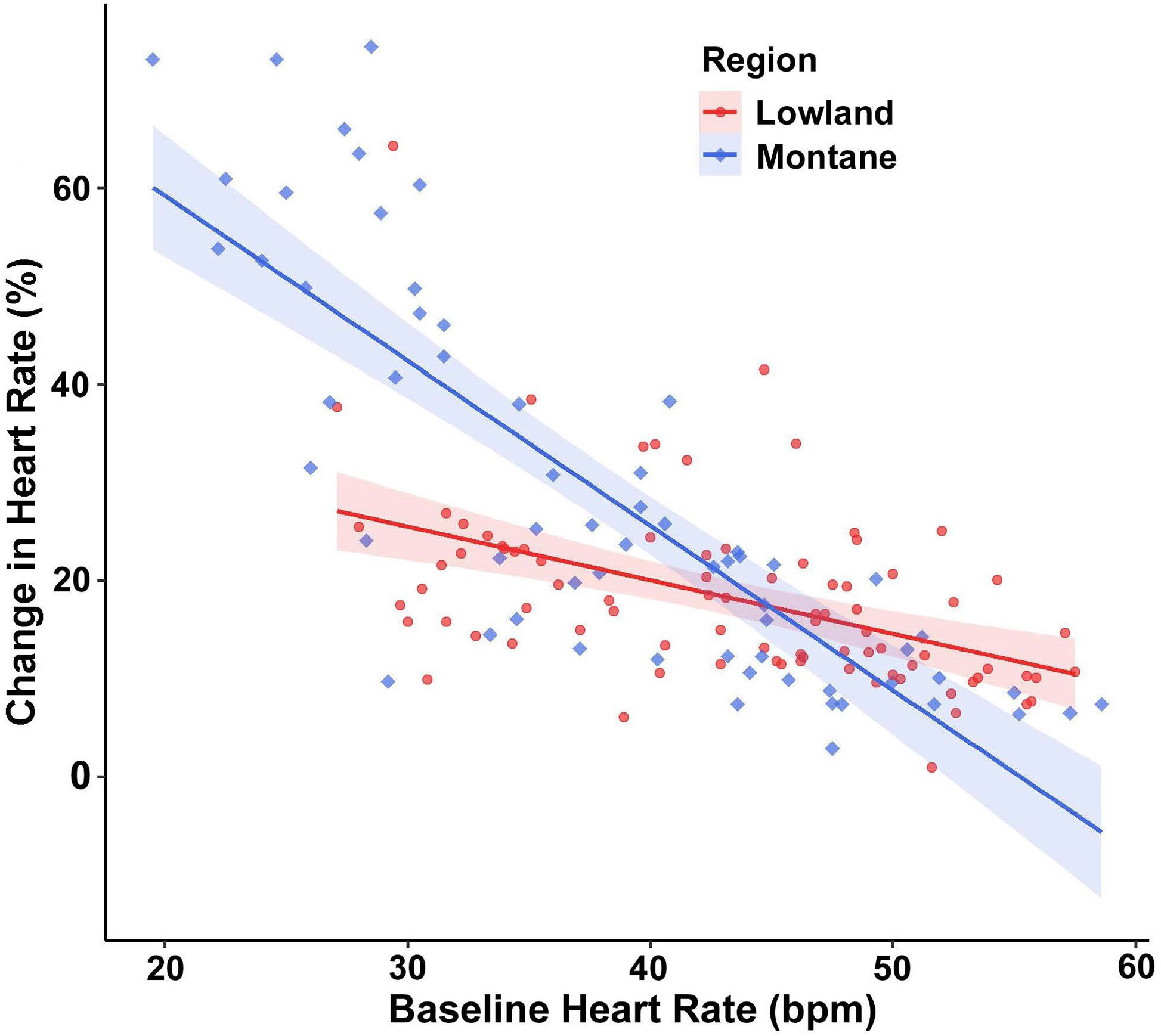
Figure 3 Emotional tachycardia (percent change in heart rate [HR] beats per minute [bpm] with 95% confidence intervals) in adult southern Pacific rattlesnakes (each tested three times) following a brief disturbance. The interaction between region and baseline HR suggests that snakes from lowland populations (N = 14) experienced a more subdued cardiac response than those from montane populations (N = 11).
For time to return to baseline HR, region (F(1,24.465) = 6.55, p = 0.017) and percent change in HR (F(1,133.03) = 48.03, p < 0.001) were significant factors. Montane snakes returned to baseline heart rate more quickly than snakes from lowland populations (Figure 4), and snakes that had a higher percent change in HR took longer to return to baseline than snakes with lower percent change in HR. There was no significant effect of sex (F(1,22.71) = 0.299, p = 0.589). The ICC showed a low amount of repeatability within individuals (ICC = 0.20).
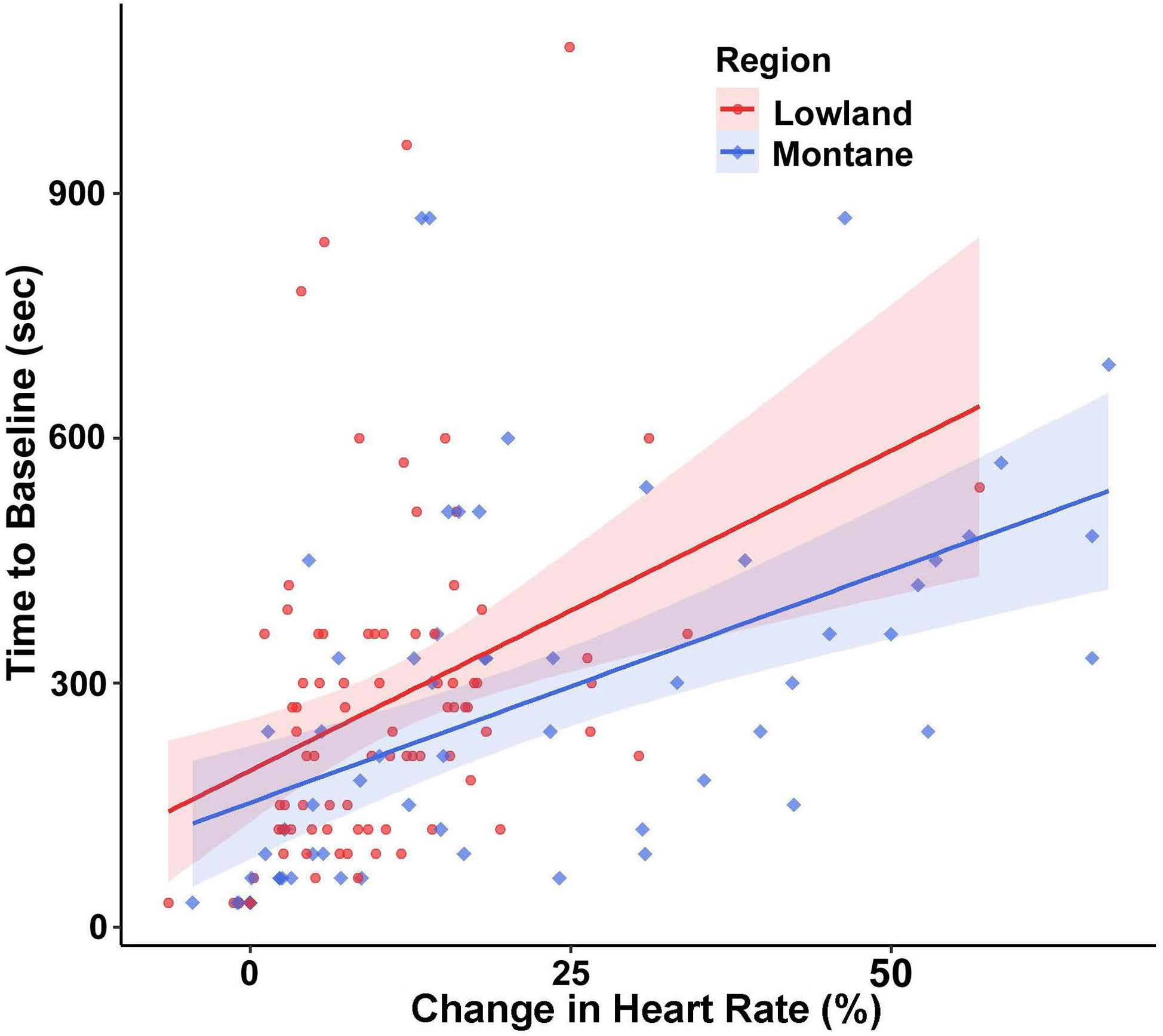
Figure 4 Time for heart rate (HR) to return to baseline (with 95% confidence intervals) of adult southern Pacific rattlesnakes (each tested three times) following a brief disturbance, with snakes from lowland populations (N = 14) taking longer than those from montane populations (N = 11) while controlling for change in HR.
For time spent rattling, treatment was a significant factor (χ2 = 7.12, df = 2, p = 0.028; Figure 5), but post-hoc multiple comparisons indicated that adjusted p-values only approached statistical significance, with snakes rattling less in the presence of the rope and a companion compared to the control (control vs. rope: p = 0.061; control vs. companion: p = 0.056; rope vs. companion: p = 0.80). There was no significant main effect for region (χ2 = 1.32, df = 1, p = 0.250) or sex (χ2 = 1.26, df = 1, p = 0.260).
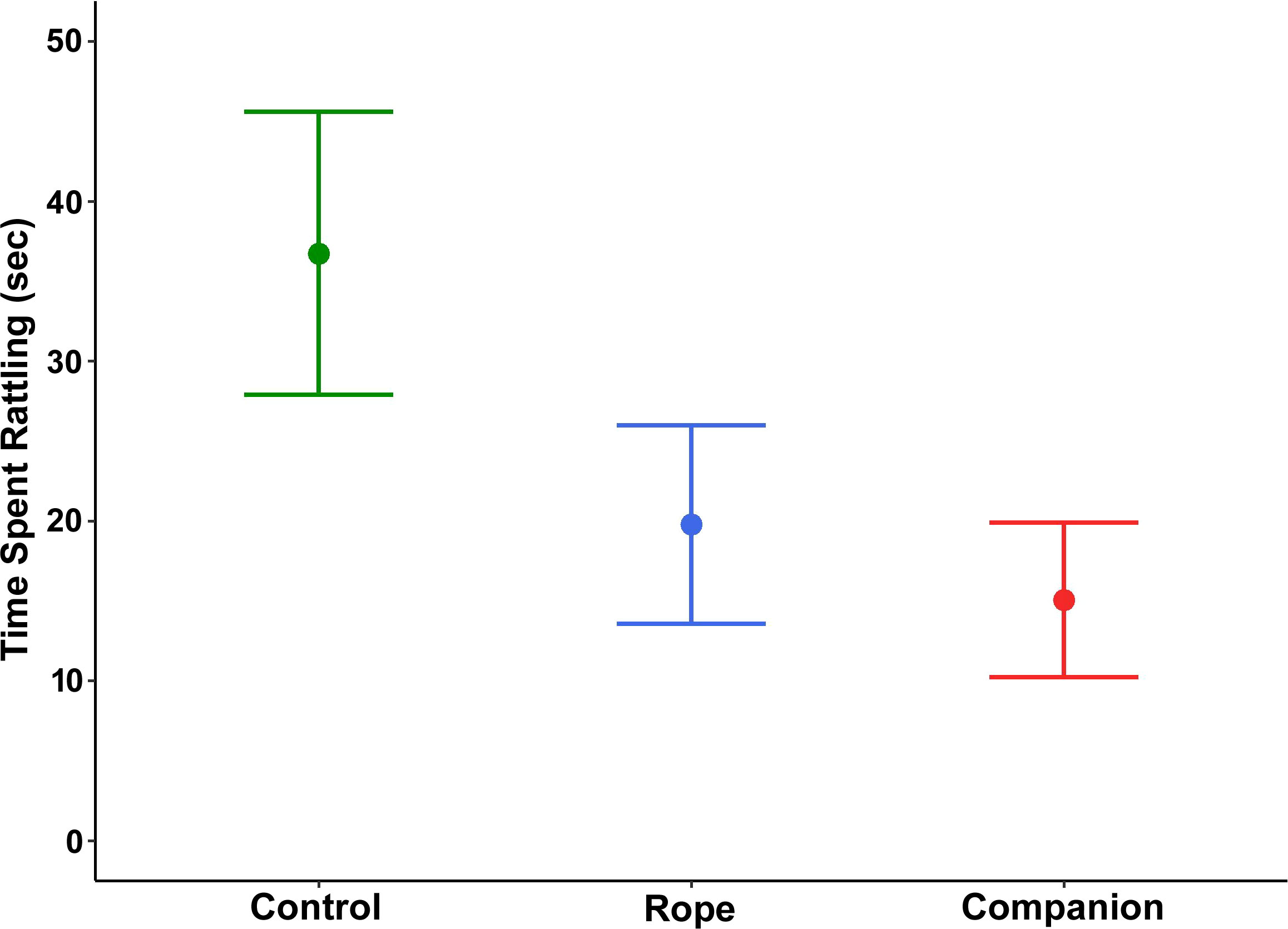
Figure 5 Amount of time spent rattling (mean ± 1 SE) by 25 adult southern Pacific rattlesnakes following a brief disturbance. Snakes tested with a rope or a companion in the bucket rattled less than those tested alone in the bucket.
For the companion trials, partner rattling behavior did not influence time spent rattling by the focal individual (χ2 = 0.001, df =1, p = 0.974).
Discussion
We sought to determine whether a companion’s presence during an acute stressor would buffer the stress response of wild-caught rattlesnakes. Our results supported just one of the three hypotheses tested: emotional tachycardia (change in HR) after exposure to an acute stressor was reduced in the presence of a conspecific, suggesting that social buffering exists in the species. We failed to detect a difference in the social buffering response between montane and lowland populations despite differences in overwintering strategy and social structure, and between the sexes despite the tendency of females to aggregate during pregnancy and remain with newborn offspring.
The subdued cardiac response to an acute stressor when in the presence of a companion supported our first hypothesis that social buffering exists in the species. However, while the effect was significant at lower levels of baseline HR, we could not detect it at higher levels of baseline HR. In retrospect, a longer acclimation period allowing baseline HR to drop further might have strengthened the social buffering effect we observed. We only allowed snakes a 20-min period to return to baseline and this oftentimes was not long enough. Similar cardiac responses to stress have been demonstrated in mammals. A companion, for example, can decrease HR recovery time of horses (Equus caballus) after a startle event (Ricci-Bonot et al., 2021) and can reduce HR variability of pigs (Sus scrofa domesticus) during a restraint test (Reimert et al., 2014). Social buffering may even occur with a different companion species, as the presence of a dog (Canis lupus familiaris) can attenuate HR of humans (Homo sapiens) during a stress test (Polheber and Matchock, 2014). We conducted our study on wild-caught individuals, demonstrating that social buffering in rattlesnakes likely exists in nature, can persist in captivity, and can be detected despite the stresses of testing in captivity. An increased understanding of how social buffering functions under natural conditions will be important in interpreting the consequences of stress in wild populations, especially those impacted by human development.
The rattling component of the defensive response seemed to be reduced by either the presence of a conspecific or an inanimate object (Figure 5). We interpret the tachycardia subsequent to disturbance to be an emotional response, and the rattling to be adoption of a defensive strategy. Thus, we might expect differences in how the treatments affected these two measures. Defensive behaviors of rattlesnakes are often context dependent, and the perception of cover may influence the adoption of an anti-predatory strategy (Duvall et al., 1985; Robillard and Johnson, 2015). The snakes in the bucket might have perceived less vulnerability when in physical contact with the rope or another conspecific (Chiszar et al., 1987), and therefore rattled less.
The results failed to support our second hypothesis, as no difference existed in the social buffering response between montane and lowland snakes. We thought variation might exist because montane snakes in Southern California generally rely on communal hibernacula for overwintering, which presumably promotes formation of social networks (Amarello, 2012; Clark et al., 2014), whereas lowland snakes generally overwinter alone (Klauber, 1972; Dugan et al., 2008; Hayes, pers. obs.; Brian Hinds, pers. comm.). Prior studies suggest that the form of social organization can influence the expression of social buffering (Hennessy et al., 2009). For example, titi monkeys (Callicebus moloch), which form long-term bonded pairs, experienced dampened stress reactivity when in the presence of a bonded heterosexual partner (Hennessy et al., 1995). Squirrel monkeys (Saimiri sciureus), in contrast, are a closely related species that forms large mixed-sex groups, and they exhibited no stress reduction when in the presence of a bonded heterosexual partner (Hennessy et al., 1995). Similar results were found between several closely related rodent species (genus Peromyscus), wherein physical contact with a conspecific positively affected wound healing in a monogamous species but not in a polygamous species (Glasper and DeVries, 2005). Likewise, in cavies (Caviinae), infants had a lower cortisol response in the presence of their mother compared to an unfamiliar female in a monogamous species but not in a harem-forming species (Hennessy et al., 2006).
At present, it remains unknown and difficult to determine whether non-denning populations of rattlesnakes readily interact and if social networks form, thereby facilitating social buffering. Although Arizona black rattlesnakes (C. cerberus) develop social networks at denning sites (Amarello, 2012), the formation of social networks in captive Eastern garter snakes (Thamnophis sirtalis sirtalis; Skinner and Miller, 2020; Skinner and Miller, 2022), suggests they may exist outside the context of denning. Maternal attendance and gene flow among populations may be sufficient to maintain social buffering in non-denning populations despite the potential lack of a social network. Future studies should examine social buffering more broadly in snakes to understand its evolution and characteristics within the group, including populations and species that do not communally den or maternally attend offspring.
Although we detected no difference between populations in social buffering, lowland snakes nevertheless exhibited a subdued acute stress response, as inferred from the lower level of tachycardia, and had a longer heart rate recovery time than montane snakes. In rats (Rattus norvegicus), different stress levels do not influence the efficacy of social buffering (Kiyokawa et al., 2018). However, stress responses, such as elevation in CORT levels, can be influenced by various environmental variables such as levels of food and predation (Clinchy et al., 2004; Berger et al., 2007; Sandfoss et al., 2020), habitat structure (Marra and Holberton, 1998; Busch and Hayward, 2009; Owen et al., 2014), latitude (Wingfield et al., 2008), and climate (including elevation and temperature: Romero et al., 2000; Busch et al., 2010; Gangloff et al., 2017; Fabrício-Neto et al., 2019), even among populations of the same species (Herczeg et al., 2009; Angelier et al., 2011). Stress responses to CORT, however, may vary, as baseline CORT may (Herr et al., 2017) or may not (Claunch et al., 2017) influence the defensive responses of vipers. Lowland populations of C. helleri in Southern California may be chronically stressed compared to montane populations, with the latter occupying habitats with lower species richness and presumably reduced predation pressure (McCain and Grytnes, 2010; Domínguez-Godoy et al., 2020), as well as increased vegetation structure (Schoenherr, 2017) and lower levels of human encroachment and activity. When an organism is chronically stressed, a dampening of the tachycardia response during an acute stressor can occur and could explain the difference in stress response observed between populations (Cyr et al., 2009). Future studies should examine if differences exist in the level of chronic stress between montane and lowland populations of C. helleri, and the extent to which human activity might affect defensive behavior (Atkins et al., 2022) and social buffering.
Our results also failed to support the third hypothesis, as no difference existed in social buffering between males and females. This surprised us given the gregarious nature of female rattlesnakes during gestation and maternal attendance of young (Greene et al., 2002; Schuett et al., 2016), as well as the antagonism (including combat) between males during the breeding season (Shine, 1978; Gillingham et al., 1983), with the latter potentially exacerbating an acute stress response. Reproductive status and seasonality may be important factors for detecting social buffering differences between the sexes, but we are unaware of studies that have examined this possibility. The type of social organization (e.g., mating system and group size), however, can influence both the presence of social buffering and which conspecifics demonstrate the ability to buffer (Hennessy et al., 2009). Many species undergo seasonal shifts in social organization (Rowell and Dixson, 1975; Zhu et al., 2018), but it remains untested whether such a shift also modulates the social buffering response. Mating occurs for C. helleri in February-April and August–October, with females giving birth between August and mid-October (Klauber, 1972; Dugan et al., 2008). We conducted our study in June and in October to early November, just outside of the breeding season and parturition time, when we expected to see sex differences if they exist. Three of the eight females tested were gravid during the summer testing session; however, the stimulus animal was non-reproductive. Our gravid females gave birth in August–September, at least one month before fall trials began. Unfortunately, our sample was too small to evaluate female reproductive status on social buffering. Future studies should examine social buffering during reproductively relevant times of the year and with paired breeding and non-breeding females to better assess whether sex and reproductive status influence social buffering.
As human development expands into natural areas, there will be greater human-wildlife conflict (Frank et al., 2019; Bhatia et al., 2020) and habitat loss (Scolozzi and Geneletti, 2012; Güneralp and Seto, 2013). Gaining a better understanding of how reptiles respond to stress can be important for habitat management and conservation (Tracy et al., 2006; Wikelski and Cooke, 2006; Teixeira et al., 2007). Translocation of venomous snakes to reduce human-snake conflict is one management tool that can be successful in some populations and some locations (Fitzgerald et al., 2018; Bradley et al., 2020; Corbit and Hayes, 2022). Snakes translocated at greater distances are less likely to return to their previous location; however, they are more prone to wander and have increased stress levels (Sullivan et al., 2015; Heiken et al., 2016; Cornelis et al., 2021). Reducing stress before translocation and during transportation via a companion’s presence could be beneficial and increase the likelihood of translocation success (Dickens et al., 2010). Paired housing could also provide more comfortable conditions, reduce stress, and help conserve and more successfully breed endangered snake species. Future work should examine how the presence of a partner influences chronic stress levels during housing by measuring changes in CORT over time.
In conclusion, the reduced tachycardia response to an acute stressor when in the presence of a conspecific suggests that social buffering exists in C. helleri. These findings constitute the first study of reptile social buffering and add additional support to the growing evidence that rattlesnakes are cryptically social. Future studies should examine the presence of social buffering in different reptile taxa and contexts to help gain a broader understanding of the function of social buffering in rattlesnakes.
Data availability statement
The raw data supporting the conclusions of this article will be made available by the authors, without undue reservation.
Ethics statement
The animal study was reviewed and approved by Loma Linda University Institutional Animal Care and Use Committee.
Author contributions
CM and WH conceived the study. CM and GF conducted the research. CM, BP, and WH analyzed the data. CM wrote the first draft and all authors edited subsequent drafts. All authors contributed to the article and approved the submitted version.
Funding
Research was supported by the Loma Linda University Department of Earth and Biological Sciences and approved by the Loma Linda University Institutional Animal Care and Use Committee (protocol 21-206).
Conflict of interest
The authors declare that the research was conducted in the absence of any commercial or financial relationships that could be construed as a potential conflict of interest.
Publisher’s note
All claims expressed in this article are solely those of the authors and do not necessarily represent those of their affiliated organizations, or those of the publisher, the editors and the reviewers. Any product that may be evaluated in this article, or claim that may be made by its manufacturer, is not guaranteed or endorsed by the publisher.
References
Amarello M. (2012). “Social snakes? non-random association patterns detected in a population of Arizona black rattlesnakes (Crotalus cerberus),” in Master's thesis (Tempe, AZ: Arizona State University).
Angelier F., Ballentine B., Holberton R., Marra P. P., Greenberg R. (2011). What drives variation in the corticosterone stress response between subspecies? a common garden experiment of swamp sparrows (Melospiza georgiana). J. Evolutionary Biol. 24 (6), 1274–1283. doi: 10.1111/j.1420-9101.2011.02260.x
Atkins M. C., Howarth C. R., Russello M. A., Tomal J. H., Larsen K. W. (2022). Evidence of intrapopulation differences in rattlesnake defensive behavior across neighboring habitats. Behav. Ecol. Sociobiology 76 (3), 1–11. doi: 10.1007/s00265-021-03100-6
Bates D., Mächler M., Bolker B., Walker S. (2015). Fitting linear mixed-effects models using lme4. J. Stat. Software 67), 1–48. doi: 10.18637/jss.v067.i01
Benjamini Y., Hochberg Y. (1995). Controlling the false discovery rate: a practical and powerful approach to multiple testing. J. R. Stat. Society: Ser. B (Methodological) 57 (1), 289–300. doi: 10.1111/j.2517-6161.1995.tb02031.x
Berger S., Wikelski M., Romero L. M., Kalko E. K., Rödl T. (2007). Behavioral and physiological adjustments to new predators in an endemic island species, the Galápagos marine iguana. Hormones Behav. 52 (5), 653–663. doi: 10.1016/j.yhbeh.2007.08.004
Bhatia S., Redpath S. M., Suryawanshi K., Mishra C. (2020). Beyond conflict: exploring the spectrum of human–wildlife interactions and their underlying mechanisms. Oryx 54 (5), 621–628. doi: 10.1017/S003060531800159X
Bishop L. A., Farrell T. M., May P. G. (1996). Sexual dimorphism in a Florida population of the rattlesnake Sistrurus miliarius. Herpetologica, 52(3):360–364. Available at: http://www.jstor.org/stable/3892655
Bonier F., Moore I. T., Martin P. R., Robertson R. J. (2009). The relationship between fitness and baseline glucocorticoids in a passerine bird. Gen. Comp. Endocrinol. 163 (1-2), 208–213. doi: 10.1016/j.ygcen.2008.12.013
Bradley H. S., Tomlinson S., Craig M. D., Cross A. T., Bateman P. W. (2020). Mitigation translocation as a management tool. Conserv. Biol. 36 (1), e13667. doi: 10.1111/cobi.13667
Brooks M. E., Kristensen K., Van Benthem K. J., Magnusson A., Berg C. W., Nielsen A., et al. (2017). glmmTMB balances speed and flexibility among packages for zero-inflated generalized linear mixed modeling. R J. 9 (2), 378–400. doi: 10.3929/ethz-b-000240890
Brown W. S., MacLean F. M. (1983). Conspecific scent-trailing by newborn timber rattlesnakes, Crotalus horridus. Herpetologica 39 (4), 430–436. Available at: https://www.jstor.org/stable/3892539
Burghardt G. M., Murphy J. B., Chiszar D., Hutchins M. (2009). “Combating ophiophobia: origins, treatment, education, and conservation tools,” in Snakes: ecology and conservation. Eds. Mullin S. J., Seigel R. A. (Ithaca, NY: Cornell University Press), 262–280.
Busch D. S., Addis E. A., Clark A. D., Wingfield J. C. (2010). Disentangling the effects of environment and life-history stage on corticosterone modulation in Costa Rican rufous-collared sparrows, Zonotrichia capensis costaricensis. Physiol. Biochem. Zoology 83 (1), 87–96. doi: 10.1086/648488
Busch D. S., Hayward L. S. (2009). Stress in a conservation context: a discussion of glucocorticoid actions and how levels change with conservation-relevant variables. Biol. Conserv. 142 (12), 2844–2853. doi: 10.1016/j.biocon.2009.08.013
Cabanac M., Bernieri C. (2000). Behavioral rise in body temperature and tachycardia by handling of a turtle (Clemmys insculpta). Behav. Processes 49 (2), 61–68. doi: 10.1016/S0376-6357(00)00067-X
Cabanac A., Cabanac M. (2000). Heart rate response to gentle handling of frog and lizard. Behav. Processes 52 (2-3), 89–95. doi: 10.1016/S0376-6357(00)00108-X
Carpenter C. C. (1984). “Dominance in snakes,” in Vertebrate ecology and systematics: a tribute to Henry S. Fitch. Eds. Seigel R. A., Hunt L.e., Knight J. L., Malaret L., Zuschlag N. L. (Lawrence, KS: University of Kansas Museum of Natural History Special Publications), 195–202.
Chiszar D., Radcliffe C. W., Boyer T., Behler J. L. (1987). Cover-seeking behavior in red spitting cobras (Naja mossambica pallida): effects of tactile cues and darkness. Zoo Biol. 6 (2), 161–167. doi: 10.1002/zoo.1430060206
Clark R. W. (2004). Kin recognition in rattlesnakes. Proc. R. Soc. London. Ser. B: Biol. Sci. 271, S243–S245. doi: 10.1098/rsbl.2004.0162
Clark R. W., Brown W. S., Stechert R., Greene H. W. (2012). Cryptic sociality in rattlesnakes (Crotalus horridus) detected by kinship analysis. Biol. Lett. 8 (4), 523–525. doi: 10.1098/rsbl.2011.1217
Clark R. W., Schuett G. W., Repp R. A., Amarello M., Smith C. F., Herrmann H.-W. (2014). Mating systems, reproductive success, and sexual selection in secretive species: a case study of the western diamond-backed rattlesnake, Crotalus atrox. PloS One 9 (3), e90616. doi: 10.1371/journal.pone.0090616
Claunch N. M., Frazier J. A., Escallón C., Vernasco B. J., Moore I. T., Taylor E. N. (2017). Physiological and behavioral effects of exogenous corticosterone in a free-ranging ectotherm. Gen. Comp. Endocrinol. 248 (1), 87–96. doi: 10.1016/j.ygcen.2017.02.008
Clinchy M., Zanette L., Boonstra R., Wingfield J. C., Smith J. N. (2004). Balancing food and predator pressure induces chronic stress in songbirds. Proc. R. Soc. London. Ser. B: Biol. Sci. 271 (1556), 2473–2479. doi: 10.1098/rspb.2004.2913
Cobb V. A., Green J. J., Worrall T., Pruett J., Glorioso B. (2005). Initial den location behavior in a litter of neonate Crotalus horridus (timber rattlesnakes). Southeastern Nat. 4 (4), 723–730. doi: 10.1656/1528-7092(2005)004[0723:IDLBIA]2.0.CO;2
Corbit A. G., Hayes W. K. (2022). Human–wildlife conflict at a suburban–wildlands interface: effects of short-and long-distance translocations on red diamond rattlesnake (Crotalus ruber) activity and survival. Diversity 14 (2), 130. doi: 10.3390/d14020130
Cornelis J., Parkin T., Bateman P. W. (2021). Killing them softly: a review on snake translocation and an Australian case study. Herpetological J. 31 (3), 118–131. doi: 10.33256/31.3.118131
Culbert B. M., Gilmour K. M., Balshine S. (2019). Social buffering of stress in a group-living fish. Proc. R. Soc. B: Biol. Sci. 286 (1910), 20191626. doi: 10.1098/rspb.2019.1626
Cyr N. E., Dickens M. J., Romero L. M. (2009). Heart rate and heart-rate variability responses to acute and chronic stress in a wild-caught passerine bird. Physiol. Biochem. Zoology 82 (4), 332–344. doi: 10.1086/589839
Dickens M. J., Delehanty D. J., Romero L. M. (2010). Stress: an inevitable component of animal translocation. Biol. Conserv. 143 (6), 1329–1341. doi: 10.1016/j.biocon.2010.02.032
Dickens M. J., Romero L. M. (2013). A consensus endocrine profile for chronically stressed wild animals does not exist. Gen. Comp. Endocrinol. 191, 177–189. doi: 10.1016/j.ygcen.2013.06.014
Domínguez-Godoy M. A., Gómez-Campos J. E., Hudson R., de la Vega A. H. D. (2020). Lower predation with increasing altitude in the mesquite lizard Sceloporus grammicus. Western North Am. Nat. 80 (4), 441–451. doi: 10.3398/064.080.0401
Doody J. S., Burghardt G. M., Dinets V. (2013). Breaking the social–non-social dichotomy: a role for reptiles in vertebrate social behavior research? Ethology 119 (2), 95–103. doi: 10.1111/eth.12047
Doody J. S., Dinets V., Burghardt G. M. (2021). The secret social lives of reptiles (Baltimore, MD: John Hopkins Univeristy Press).
Dugan E., Figueroa A., Hayes W. (2008). “Home range size, movements, and mating phenology of sympatric red diamond (Crotalus ruber) and southern pacific (C. oreganus helleri) rattlesnakes in southern California,” in The biology of rattlesnakes. Eds. Hayes W. K., Beaman K. R., Cardwell M. D., Bush S. P. (Loma Linda, CA: Loma Linda University Press), 353–364.
Duvall D., King M., Gutzwiller K. (1985). Behavioral ecology and ethology of the prairie rattlesnake. Natl. Geographic Res. 1 (1), 80–111.
Fabrício-Neto A., Madelaire C. B., Gomes F. R., Andrade D. V. (2019). Exposure to fluctuating temperatures leads to reduced immunity and to stress response in rattlesnakes. J. Exp. Biol. 222 (22), 1–8. doi: 10.1242/jeb.208645
Fitch H. S. (1949). Study of snake populations in central California. Am. Midland Nat. 41 (3), 513–579. doi: 10.2307/2421774
Fitzgerald L., Walkup D., Chyn K., Buchholtz E., Angeli N., Parker M. (2018). “The future for reptiles: advances and challenges in the anthropocene,” in Encyclopedia of the anthropocene. Eds. Dellasala D. A., Goldstein M. I. (Amsterdam, Netherlands: Elsevier), 163–174.
Frank B., Glikman J. A., Marchini S. (2019). Human–wildlife interactions: turning conflict into coexistence (Cambridge, United Kingdom: Cambridge University Press).
Gangloff E. J., Sparkman A. M., Holden K. G., Corwin C. J., Topf M., Bronikowski A. M. (2017). Geographic variation and within-individual correlations of physiological stress markers in a widespread reptile, the common garter snake (Thamnophis sirtalis). Comp. Biochem. Physiol. Part A: Mol. Integr. Physiol. 205, 68–76. doi: 10.1016/j.cbpa.2016.12.019
Gillingham J. C., Carpenter C. C., Murphy J. B. (1983). Courtship, male combat and dominance in the western diamondback rattlesnake, Crotalus atrox. J. Herpetology 17 (3), 265–270. doi: 10.2307/1563829
Glasper E. R., DeVries A. C. (2005). Social structure influences effects of pair-housing on wound healing. Brain Behavior Immun. 19 (1), 61–68. doi: 10.1016/j.bbi.2004.03.002
Glaudas X., Rodriguez-Robles J. A. (2011). Vagabond males and sedentary females: spatial ecology and mating system of the speckled rattlesnake (Crotalus mitchellii). Biol. J. Linn. Soc. 103 (3), 681–695. doi: 10.1111/j.1095-8312.2011.01677.x
Goode M. J., Wall M. D. (2002). “Tiger rattlesnake ecology and management,” in Unpubl. report. nongame and endangered wildlife program heritage (Phoenix, AZ: Game and Fish Department).
Graves B. M., Duvall D. (1988). Evidence of an alarm pheromone from the cloacal sacs of prairie rattlesnakes. Southwestern Nat. 33 (3), 339–345. doi: 10.2307/3671762
Graves B. M., Duvall D. (1993). Reproduction, rookery use, and thermoregulation in free-ranging, pregnant Crotalus v. viridis. J. Herpetology 27, 33–41. doi: 10.2307/1564902
Graves B. M., Duvall D. (1995). Aggregation of squamate reptiles associated with gestation, oviposition, and parturition. Herpetological Monogr. 9, 102–119. doi: 10.2307/1466999
Greene H. W. (1988). “Antipredator mechanisms in reptiles,” in Biology of the Reptilia. Eds. Gans C., Huey R. B. (New York, NY: Alan R. Liss, Inc), 1–152.
Greene H. W., May P. G., Hardy D. L. Sr., Sciturro J. M., Farrell T. M. (2002). “Parental behavior by vipers,” in Biology of the Vipers. Eds. Schuett G. W., Hoggren M., Douglas M. E., Greene H. W. (Eagle Mountain, UT: Eagle Mountain Publishing), 179–205.
Güneralp B., Seto K. (2013). Futures of global urban expansion: uncertainties and implications for biodiversity conservation. Environ. Res. Lett. 8 (1), 14025. doi: 10.1088/1748-9326/8/1/014025
Hayes W. K., Verde E. A., Hayes F. E. (1994). Cardiac responses during courtship, male-male fighting, and other activities in rattlesnakes. J. Tennessee Acad. Sci. 69 (1), 7–9.
Heiken K. H., Brusch G. A. IV, Gartland S., Escallón C., Moore I. T., Taylor E. N. (2016). Effects of long distance translocation on corticosterone and testosterone levels in male rattlesnakes. Gen. Comp. Endocrinol. 237, 27–33. doi: 10.1016/j.ygcen.2016.07.023
Hennessy M. B., Kaiser S., Sachser N. (2009). Social buffering of the stress response: diversity, mechanisms, and functions. Front. Neuroendocrinol. 30 (4), 470–482. doi: 10.1016/j.yfrne.2009.06.001
Hennessy M. B., Mendoza S. P., Mason W. A., Moberg G. P. (1995). Endocrine sensitivity to novelty in squirrel monkeys and titi monkeys: species differences in characteristic modes of responding to the environment. Physiol. Behav. 57 (2), 331–338. doi: 10.1016/0031-9384(94)00250-9
Hennessy M. B., Neisen G., Bullinger K. L., Kaiser S., Sachser N. (2006). Social organization predicts nature of infant-adult interactions in two species of wild guinea pigs (Cavia aperea and Galea monasteriensis). J. Comp. Psychol. 120 (1), 12–18. doi: 10.1037/0735-7036.120.1.12
Herczeg G., Gonda A., Merilä J. (2009). Predation mediated population divergence in complex behaviour of nine-spined stickleback (Pungitius pungitius). J. Evolutionary Biol. 22 (3), 544–552. doi: 10.1111/j.1420-9101.2008.01674.x
Herr M. W., Graham S. P., Langkilde T. (2017). Stressed snakes strike first: hormone levels and defensive behavior in free ranging cottonmouths (Agkistrodon piscivorus). Gen. Comp. Endocrinol. 243, 89–95. doi: 10.1016/j.ygcen.2016.11.003
Hileman E. T., Bradke D. R., Delaney D. M., King R. B. (2015). Protection by association: implications of scent trailing in neonate eastern massasaugas (Sistrurus catenatus). Herpetological Conserv. Biol. 10 (2), 654–660.
Hoss S. K., Deutschman D. H., Booth W., Clark R. W. (2015). Post-birth separation affects the affiliative behaviour of kin in a pitviper with maternal attendance. Biol. J. Linn. Soc. 116 (3), 637–648. doi: 10.1111/bij.12604
Kikusui T., Winslow J. T., Mori Y. (2006). Social buffering: relief from stress and anxiety. Philos. Trans. R. Soc. B: Biol. Sci. 361 (1476), 2215–2228. doi: 10.1098/rstb.2006.1941
Kiyokawa Y., Kawai K., Takeuchi Y. (2018). The benefits of social buffering are maintained regardless of the stress level of the subject rat and enhanced by more conspecifics. Physiol. Behav. 194, 177–183. doi: 10.1016/j.physbeh.2018.05.027
Klauber L. (1972). Rattlesnakes: their habits, life histories and influence on mankind (Berkeley, CA: University of California Press).
Koo T. K., Li M. Y. (2016). A guideline of selecting and reporting intraclass correlation coefficients for reliability research. J. Chiropractic Med. 15 (2), 155–163. doi: 10.1016/j.jcm.2016.02.012
Marra P. P., Holberton R. L. (1998). Corticosterone levels as indicators of habitat quality: effects of habitat segregation in a migratory bird during the non-breeding season. Oecologia 116, 284–292. doi: 10.1007/s004420050590
Martin C. E. (2019). “Behavioral aspects of chemoreception in juvenile cottonmouths (Agkistrodon piscivorus),” in Master's thesis (Springfield, MO: Missouri State University).
McCain C. M., Grytnes J.-A. (2010). “Elevational gradients in species richness,” in Encyclopedia of life science (Chichester, UK: John Wiley & Sons), 1–10.
O’Leile J., Beaupre S., Duvall D. (1994). A novel form of mate guarding/female defence polygyny in western diamondbacks. Am. Zoologist 34, 62A.
Owen D. A., Carter E. T., Holding M. L., Islam K., Moore I. T. (2014). Roads are associated with a blunted stress response in a north American pit viper. Gen. Comp. Endocrinol. 202, 87–92. doi: 10.1016/j.ygcen.2014.04.020
Polheber J. P., Matchock R. L. (2014). The presence of a dog attenuates cortisol and heart rate in the Trier Social Stress Test compared to human friends. J. Behav. Med. 37 (5), 860–867. doi: 10.1007/s10865-013-9546-1
R Core Team. (2019). R: A language and environment for statistical computing. Vienna, Austria: R Foundation for Statistical Computing. Retrieved from https://www.R-project.org
Reimert I., Bolhuis J. E., Kemp B., Rodenburg T. B. (2014). Social support in pigs with different coping styles. Physiol. Behav. 129, 221–229. doi: 10.1016/j.physbeh.2014.02.059
Reinert H. K., Zappalorti R. T. (1988). Field observation of the association of adult and neonatal timber rattlesnakes, Crotalus horridus, with possible evidence for conspecific trailing. Copeia 1988 (4), 1057–1059. doi: 10.2307/1445732
Ricci-Bonot C., Romero T., Nicol C., Mills D. (2021). Social buffering in horses is influenced by context but not by the familiarity and habituation of a companion. Sci. Rep. 11, 8862. doi: 10.1038/s41598-021-88319-z
Robillard A., Johnson B. (2015). Edge effects on Eastern massasauga rattlesnakes basking in managed habitat. Northeastern Nat. 22 (1), 200–208. Available at: https://www.jstor.org/stable/26453714.
Romero L. M., Reed J. M., Wingfield J. C. (2000). Effects of weather on corticosterone responses in wild free-living passerine birds. Gen. Comp. Endocrinol. 118 (1), 113–122. doi: 10.1006/gcen.1999.7446
Rowell T., Dixson A. (1975). Changes in social organization during the breeding season of wild talapoin monkeys. J. Reprod. Fertility 43 (3), 419–434. doi: 10.1530/jrf.0.0430419
Sandfoss M. R., Claunch N. M., Stacy N. I., Romagosa C. M., Lillywhite H. B. (2020). A tale of two islands: evidence for impaired stress response and altered immune functions in an insular pit viper following ecological disturbance. Conserv. Physiol. 8 (1), 1–20. doi: 10.1093/conphys/coaa031
Sapolsky R. M., Romero L. M., Munck A. U. (2000). How do glucocorticoids influence stress responses? integrating permissive, suppressive, stimulatory, and preparative actions. Endocrine Rev. 21 (1), 55–89. doi: 10.1210/edrv.21.1.0389
Schoenherr A. A. (2017). A natural history of California (Berkeley, CA: University of California Press).
Schuett G. W., Clark R. W., Repp R. A., Amarello M., Smith C. F., Greene H. W. (2016). “Social behavior of rattlesnakes: a shifting paradigm,” in Rattlesnakes of Arizona. Eds. Schuett G. W., Feldner M. J., Reiserer R. S., Smith C. F. (Rodeo, NM: Eco Publishers), 161–244.
Scolozzi R., Geneletti D. (2012). A multi-scale qualitative approach to assess the impact of urbanization on natural habitats and their connectivity. Environ. Impact Assess. Rev. 36, 9–22. doi: 10.1016/j.eiar.2012.03.001
Shine R. (1978). Sexual size dimorphism and male combat in snakes. Oecologia 33 (3), 269–277. Available at: https://www.jstor.org/stable/4215608.
Shipman P. A. (2002). The evolution of antipredator behavior in snakes: ultimate and proximate determinants. Ph.D dissertation (Stillwater, OK: Oklahoma State University).
Silvestre A. M. (2014). How to assess stress in reptiles. J. Exotic Pet Med. 23 (3), 240–243. doi: 10.1053/j.jepm.2014.06.004
Skinner M., Miller N. (2020). Aggregation and social interaction in garter snakes (Thamnophis sirtalis sirtalis). Behav. Ecol. Sociobiology 74 (51), 1–13. doi: 10.1007/s00265-020-2827-0
Skinner M., Miller N. (2022). Stability and change in gartersnake social networks across ontogeny. Ethology 128 (3), 257–267. doi: 10.1007/s00265-020-2827-0
Stinner J., Ely D. (1993). Blood pressure during routine activity, stress, and feeding in black racer snakes (Coluber constrictor). Am. J. Physiol. 264 (1), R79–R84. doi: 10.1152/ajpregu.1993.264.1.R79
Sullivan B. K., Nowak E. M., Kwiatkowski M. A. (2015). Problems with mitigation translocation of herpetofauna. Conserv. Biol. 29 (1), 12–18. doi: 10.1111/cobi.12336
Teixeira C. P., De Azevedo C. S., Mendl M., Cipreste C. F., Young R. J. (2007). Revisiting translocation and reintroduction programmes: the importance of considering stress. Anim. Behav. 73 (1), 1–13. doi: 10.1016/j.anbehav.2006.06.002
Tian L., Preisser E. L., Haynes K. F., Zhou X. (2017). Social buffering in a eusocial invertebrate: termite soldiers reduce the lethal impact of competitor cues on workers. Ecology 98 (4), 952–960. doi: 10.1002/ecy.1746
Tracy C. R., Nussear K., Esque T., Dean-Bradley K., Tracy C., DeFalco L., et al. (2006). The importance of physiological ecology in conservation biology. Integr. Comp. Biol. 46 (6), 1191–1205. doi: 10.1093/icb/icl054
Wikelski M., Cooke S. J. (2006). Conservation physiology. Trends Ecol. Evol. 21 (1), 38–46. doi: 10.1016/j.tree.2005.10.018
Wingfield J. C. (2005). The concept of allostasis: coping with a capricious environment. J. Mammalogy 86 (2), 248–254. doi: 10.1644/BHE-004.1
Wingfield J. C., Maney D. L., Breuner C. W., Jacobs J. D., Lynn S., Ramenofsky M., et al. (1998). Ecological bases of hormone–behavior interactions: the “Emergency life history stage”. Am. Zoologist 38 (1), 191–206. doi: 10.1093/icb/38.1.191
Wingfield J. C., Moore I. T., Vásquez Salfate R., Sabat Kirkwood A. P., Busch S., Clark A., et al. (2008). Modulation of the adrenocortical responses to acute stress in northern and southern populations of Zonotrichia. Ornitologia Neotropical 19, 241–251. Available at: https://repositorio.uchile.cl/handle/2250/119026.
Wingfield J. C., Romero L. M. (2001). Adrenocortical responses to stress and their modulation in free-living vertebrates. in Handbook of physiology, Section 7: The endocrine system, Vol. 4: Coping with the environment: Neural and endocrine mechanisms. Ed: McEwen B. S. (Oxford, NY: Oxford University Press), 211–234. doi: 10.1002/cphy.cp070411
Young B. A., Potter J., Blanchard J., Knoche L., Kondrashova T. (2020). Cardiac response to stimulation and stress in the American alligator (Alligator mississippiensis). Amphibia-Reptilia 1 (aop), 547–551. doi: 10.1163/15685381-bja10013
Keywords: social behavior, reptile, pitvipers, cardiac response, social organization, habitat differences, sex differences, stress response
Citation: Martin CE, Fox GA, Putman BJ and Hayes WK (2023) Social security: can rattlesnakes reduce acute stress through social buffering? Front. Ethol. 2:1181774. doi: 10.3389/fetho.2023.1181774
Received: 07 March 2023; Accepted: 22 May 2023;
Published: 06 July 2023.
Edited by:
Fabio Santos Nascimento, University of São Paulo, Ribeirão Preto, BrazilReviewed by:
Matthew Lovern, Oklahoma State University, United StatesWilfried Klein, University of São Paulo, Brazil
Copyright © 2023 Martin, Fox, Putman and Hayes. This is an open-access article distributed under the terms of the Creative Commons Attribution License (CC BY). The use, distribution or reproduction in other forums is permitted, provided the original author(s) and the copyright owner(s) are credited and that the original publication in this journal is cited, in accordance with accepted academic practice. No use, distribution or reproduction is permitted which does not comply with these terms.
*Correspondence: Chelsea E. Martin, Y2hlbWFydGluOTRAZ21haWwuY29t