- 1Department of General Practice, University Hospital of Liège, Liège, Belgium
- 2Department of Animal Pathology, Fundamental and Applied Research for Animals & Health, University of Liège, Liège, Belgium
- 3Department of Clinical Microbiology, University Hospital of Liège, Liège, Belgium
- 4Department of Infectious Diseases and General Internal Medicine, University Hospital of Liège, Liège, Belgium
Arthropod-borne viral diseases are likely to be affected by the consequences of climate change with an increase in their distribution and intensity. Among these infectious diseases, chikungunya and dengue viruses are two (re)emergent arboviruses transmitted by Aedes species mosquitoes and which have recently demonstrated their capacity for rapid expansion. They most often cause mild diseases, but they can both be associated with complications and severe forms. In Europe, following the establishment of invasive Aedes spp, the first outbreaks of autochtonous dengue and chikungunya have already occurred. Northern Europe is currently relatively spared, but climatic projections show that the conditions are permissive for the establishment of Aedes albopictus (also known as the tiger mosquito) in the coming decades. It is therefore essential to question and improve the means of surveillance in northern Europe, at the dawn of inevitable future epidemics.
1 Introduction
It is now an established fact that the climate is changing as a result of human activities, and that this trend will accelerate in the coming decades, unless we change drastically the way we use energy (1). Consequences on human health are multiple and of extreme importance, and justify a One Health approach that takes global changes into account in public health policies (2, 3).
Among human health domains that are likely to be impacted by climate change, now or in the future, infectious diseases have recently attracted renewed interest. Recent decades have showed a rapid increase of emerging diseases, mainly zoonoses and vector-transmitted diseases (4). Arbovirosis—a group of viral diseases transmitted by arthropod—appear likely to show particular sensitivity to the main abiotic consequences of climate change, namely rising temperature and changing precipitation patterns (5).
Arthropods are affected by external temperature, on which their survival, feeding and reproduction depend (6–8). The alternation of rain and drought, as well as extreme events, favours the development of stagnant water points, and therefore the reproduction of mosquitoes (9, 10). Viral replication within the arthropod vector also depends on the external temperature. Ultimately, the interaction between the vector and the virus results in a bell-shaped temperature response with an optimum, a lower threshold and a higher threshold (10–13).
Evidence of the climate change impact on the distribution of vectors, the endemic area, and the occurrence of outbreaks already exist for many arboviruses, including dengue (14), chikungunya and zika (15), tick-borne encephalitis (16, 17), Crimean-Congo haemorrhagic fever (18) and West Nile virus (5, 19). Current researches are largely focused on the development of predictive distribution models of these diseases, particularly in Europe (20, 21). These models predict an expansion of the endemic areas of arboviruses studied towards higher latitudes and altitudes (22–27). The expected impact is particularly significant in Europe (23, 24, 28). The spread of these pathogens in this immunologically naive population could lead to severe cases and outbreaks (24, 29).
We will focus this review on two emerging Aedes-borne arboviruses which are already a threat in some European countries that have to some extent, an animal reservoir and have, according to current models, a predicted expansion in next decades toward northern Europe: chikungunya virus (CHIKV) and dengue virus (DENV).
2 Invasive Aedes mosquitoes
Aedes (Ae.) spp mosquitoes, and in particular Aedes aegypti and Aedes albopictus, are major vectors of arboviruses, among which the yellow fever, dengue, chikungunya and zika viruses (30, 31). Due to their invasion potential and their health importance, they are the subject of entomological surveillance programs at nationals and European level (32, 33).
2.1 Aedes albopictus
Aedes albopictus, or tiger mosquito, was originally to be found in Asia, but has now spread to all continents (34). Currently, Ae. albopictus is introduced in Belgium, and considered established in France and Germany, as well as in large parts of Europe (35, 36). The success of Ae. albopictus in our latitudes can be explained by its robust physiology and adaptability. Indeed, this species is capable of surviving and reproducing above the average annual temperature threshold of 10° or 11°, with optimum activity between 25 and 30 degrees, and provided that a minimum of 500 mm of precipitation occurs on an annual basis (37, 38). Even more surprising, European strains of the mosquito show a capacity for hibernation, in the form of eggs which enter diapause in response to the reduction in the photoperiod (37, 39). This diapause allows European strains to withstand temperatures down to −10°C during a short period and increases the hatching rate at the end of winter (39). This species also proliferates in a much more urban environment than its original environment, can feed on a wide range of vertebrates, modify its periods of activity and lay eggs in artificial water points (38, 40). Furthermore, it is a daytime-biter and shows resistance to common insecticides, two characteristics that pose problems in terms of vector control (41).
When comparing the theoretical climatic thresholds of Ae. albopictus to current climate data, the fundamental niche of Ae. albopictus does not yet include northern parts of Europe, mainly due to insufficient summer temperatures (42). However, more recent studies based on climatic data from areas already colonized by this vector conclude that the fundamental niche is much broader (23, 43). In all cases, the climatic conditions in northern Europe should not represent an obstacle to the establishment (and role of vector) of Ae. albopictus by 2050, even in the most favourable climatic scenarios (23, 24, 44).
2.2 Aedes aegypti
Aedes aegypti was previously established in southern Europe, around the Mediterranean basin, from which it disappeared in the early 1900s (37). In recent years, it has reconquered a small part of European territory, in particular Madeira (45). The relatively high thermal requirements of Ae. Aegypti currently prevent its expansion to northern Europe (37). It has an optimum around 29°C and remains capable of proliferating up to 36°C, while the population decreases below the threshold of 15°C (46, 47). This threshold temperature of 15 degrees is also approximately the one below which the adult ceases to be active (48). Aedes aegypti is rather anthropophilic, although it can feed on other mammals (37).
The fundamental niche of Ae. aegypti does not currently cover northern Europe, unlike some southern regions of France, Italy and Spain (23, 43). Aedes aegypti will see its global distribution area increase significantly in the coming decades in every climate change scenario (23, 24, 43). Under the “worst case scenario” hypothesis it could even cease to be a vector in Africa and make a shift towards a northern and more seasonal transmission (24). However, it is not expected for this mosquito to become endemic in northern Europe by the end of the century (23, 43, 49, 50).
2.3 Current situation of Aedes aegypti and Ae. albopictus in Europe
Monitoring programs for tropical mosquitoes (in particular Aedes spp) aim to see the evolution of their population in Europe. The ECDC distinguishes three phases of colonization of invasive species: the absence, introduction, and establishment of the invasive species. Ae. albopictus is already established in most of the France (with the exception of some northern regions), and occasionally leads to autochthonous transmission of arboviruses (51, 52). In Andalusia (Spain), a permanent population of Ae. aegypti has been established since the end of the last century, and outbreaks of Aedes-transmitted arboviruses have been reported since 2009 (15).
Ae. albopictus is not yet established (although recently locally introduced) in United Kingdom (35, 53, 54). In the Netherlands, evidence of introduction and even local reproduction of Aedes aegypti and Aedes albopictus has been found around Schiphol Airport (54, 55). The first specimen of Ae. albopictus in Belgium was found in 2000, it is now regularly introduced (36, 56) and some specimens survive the Belgian winter (57). See also Figure 1 for the current and past distributions of Aedes albopictus in mainland Europe.
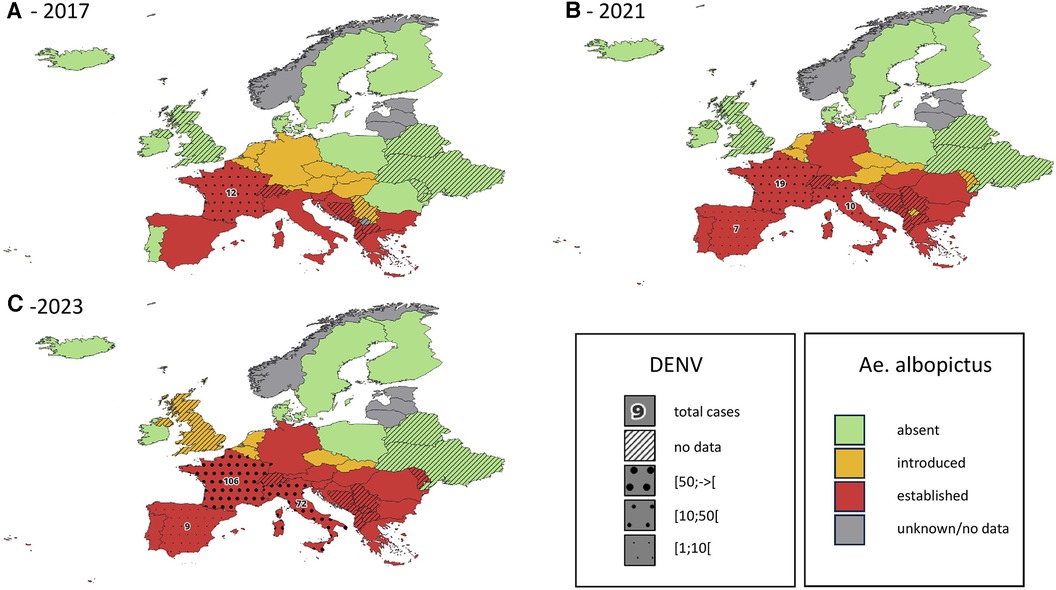
Figure 1. Introduction status of the invasive mosquito species aedes albopictus in Europe, in 2017 (A), 2021 (B) and 2023 (C) cumulative reported autochtonous dengue cases are indicated on the same map for the period 2014-2017 (A), 2018-2021 (B) and 2022-2023 (C) data regarding DENV cases are only available for countries belonging to the EEA. Data obtained from the European Centre for Diseases Prevention and Control (1–4).
2.4 Other Aedes mosquitoes
Ae. japonicus and Ae. koreica are other potentially invasive Aedes mosquitoes that could cause health issues. There is evidence of competence of Ae. japonicus for the transmission of arboviruses such as dengue, chikungunya and Zika virus, in laboratory setting (58, 59). These two mosquitoes are now widely established in Europe (60). Their health importance is unknown to date, and their field transmission competence has not been established in Europe (58, 59).
3 Aedes-borne diseases
The main arboviruses transmitted by Aedes aegypti (and to varying extents by Ae. albopictus) present similar epidemiological features and clinical syndromes.
Most of the time, the natural course of diseases caused by these viruses consists in the succession of a short incubation phase, a febrile phase, and a defervescence phase (61, 62). The defervescence phase progresses within a week either towards recovery or towards a more severe disease (61, 62). Seroconversion (IgM) is generally observed one week after symptoms onset (61). However, cross-reactivity between different flaviviruses can make distinction complicated, particularly in cases of pre-exposure (infection or vaccination) (63). Direct detection by PCR is effective but limited to the first days of the disease (61).
3.1 Chikungunya
Chikungunya virus (CHIKV), a togaviridae, is transmitted by Aedes spp mosquitoes, especially Ae. aegypti and Ae. albopictus. Four lineages are currently described, all of which belonging to the same serotype (64). CHIKV was first isolated from a patient in Tanzania in 1952 (65). Its natural cycle, originally described in Asia and the Indian Pacific Islands is sylvatic, with numerous vertebrates (notably non-human primates, bats and ectothermic vertebrates) as reservoirs (66, 67). However, epidemics are characterized by the circulation of the virus in an urban cycle, with a transmission between mosquitoes and humans, the latter serving as reservoirs (66). Occasionally the virus can also be transmitted directly from human to human, through contact with bodily fluids of highly viremic individuals (68).
Chikungunya disease is classically characterized by a sudden onset of high fever, severe arthralgia (often with swelling) and a rash (61, 69). Full recovery is usual within ten days but joint pain can persist in up to 25% of the patients and become chronic (69–71). Severe disease, with neurological, renal, cardiac or metabolic involvement is rare and 1 death per 1,000 cases is observed, mainly among comorbid patients (72).
Chikungunya remained relatively unknown until the early 2000s. In 2004, an epidemic started in Kenya and spread in 2005—2006 to the islands of the Indian Ocean, notably the Reunion Island (66). The epidemic that occurred in Reunion was striking due to its intensity and the fact that its main vector was Ae. albopictus, which was previously thought to be only a secondary vector (73). These two characteristics were partially explained by the A226V mutation altering a membrane fusion glycoprotein (74). This mutation makes Ae. albopictus slightly more competent than Ae. aegypti for the E1-A226V variant, which rapidly constituted the main variant in Reunion (75, 76, 77). Another important cause of the severity of the Reunion epidemic was the immunologically naive nature of the population to this arbovirus (48).
Since then, other significant outbreaks have occurred around the world, including several in Europe. In 2007, the first Chikungunya outbreak in Europe was recorded in Italy (34, 78). In the following years, a few indigenous cases were reported in France, often associated with extreme climatic events (34, 51, 79, 80). If the CHIKV strain with the E1-A226V mutation shows, in most studies, better transmissibility by Ae. albopictus, it is not the only strain transmitted in Europe by this vector (81). Indeed, a strain without this mutation caused an epidemic in Italy in 2017 (82), during which the competence of Ae. albopictus was similar to the 2007 CHIKV-E1-A226V outbreak (83).
As discussed previously, the competent vector Ae. albopictus is already found in northern Europe. Furthermore, the incubation of CHIKV in Ae. albopictus can occur at temperatures as low as 18°C, resulting in a high transmission rate (84). Therefore, the climate in some parts of northern Europe is already conducive to a Chikungunya outbreak in summer (25, 26). In the coming decades, temperature conditions are expected to allow the transmission of Chikungunya in northern Europe for 3 months per year (85).
3.2 Dengue
Dengue virus (DENV), a flavivirideae, was first isolated in 1943 (86). There are four serotypes, initially isolated in Asia and Oceania, which now circulate widely throughout the world, mainly in Asia, Africa and America (87, 90). Its enzootic cycle involves specific sylvatic strains circulating between non-human primates and arboreal mosquitoes (sylvatic cycle). On the other hand, the epidemic circulation of urban DENV (urban cycle) is classically described as exclusively depending on the human host and (peri-)urban mosquitoes' vector Aedes aegypti and Ae. Albopictus (86). However, spillovers from the enzootic cycle are regularly documented and four of these cross-over species transmission events are thought to be the origin of the four current urban serotypes of dengue (88). Moreover, there is recent evidence supporting the fact that other vertebrates can be infected and their role as urban reservoirs can't be ruled out (89). These findings suggest that the role of the animal-human interface in the present and future (re)emergence of dengue shouldn't be overlooked.
The disease caused by this virus is dengue fever, a usually mild disease with a spontaneous favourable evolution within 1–2 weeks (90). However, a more serious disease is also observed, severe dengue, preferentially affecting children before the age of 15, and fatal in 20% of cases in the absence of appropriate supporting care (91). Severe dengue occurs preferentially in areas of coexistence of different subtypes, and the main causal mechanism is linked to sequential infection by two different subtypes, the previous antibodies forming antibody-virus complexes during the next infection (92). However, the antibody not being specific to the subtype fails to inactivate the virus and leads to the antibody-dependent enhancement of the viral replication, increasing the risk of sepsis (92).
Dengue has undergone the most dramatic increase among infectious diseases during the last 50 years, with a 30-fold increase (93). Evidence already exists in Asia of the impact of climate change on its distribution (14). Models predict an increase in the endemic area of dengue, an extension of the transmission season and a shift toward a younger age at secondary infection by dengue under the effect of climate change (27, 94).
Although Ae. aegypti is historically considered the main vector of dengue, the role of Aedes albopictus in the transmission of dengue is increasingly recognized. Its involvement in previous epidemics in temperate zones has been proven (95). Some studies even consider that its robustness and lifespan, make Ae. albopictus a more competent vector than Ae. aegypti for DENV (96). The optimum temperature for dengue transmission depends on the vector concerned (97). Ae. albopictus is competent for dengue transmission at temperatures as low as 21°C (with an optimum around 30°C) (98). In this interval, increasing temperature decreases the incubation period and increases the rate of transmission (99).
In mainland France where Ae. albopictus is established, autochthonous transmission of DENV has been observed since 2010 (51, 100, 101). This transmission mode remains confined to small self-limited outbreaks of a few individuals, even if 2022 summer has seen a dramatic increase of these events with 65 indigenous cases (more than the total from 2010 to 2021) (102, 103). Figure 1 shows the cumulative reported autochthonous dengue cases in countries of the European Union, in parallel with the distribution of Ae. albopictus.
Given the projections regarding the likely establishment of Ae. albopictus in the short term in northern Europe, the rather moderate temperatures allowing transmission of dengue by this mosquito, observations already reported in neighboring countries, and inevitable introductions of DENV (via infected people), it therefore seems probable that dengue epidemics will occur in the coming decades in northern Europe.
4 Discussion
Aedine mosquitoes, and in particular Aedes albopictus, have shown a recent large geographical expansion, becoming established in regions ever further north of the European continent. Ae. albopictus is considered as one of the more threatening invasive species in the world (104). Even under current climatic conditions, it is expected that the mosquito will continue its dissemination across the European continent in the next years through human-mediated spread, considering its fundamental niche already includes most of this territory (23, 49). Afterwards, as the suitability of European climate for this mosquito is expected to continually increase in the next decades, its distribution will likely include northern Europe by the end of the century (49).
Both Ae. albopictus and Ae. aegypti are vectors of human health threatening viruses, among which dengue and chikungunya are of particular concern. Data from other regions of the world show that the first epidemics of Aedes-borne viruses were observed between 5 and 15 years after the establishment of Ae. albopictus (49). On the American continent, the existence of competent vectors for ZIKV and CHIKV allowed the introduction of these two viruses in the 2010s. It resulted in large epidemics in the following years and ultimately the endemic establishment of these diseases (105, 106). The first European data tend to confirm this pattern, with an important increase of autochthonous DENV cases in countries where Ae. albopictus has been well established for several years (see Figure 1).
These data converge to demonstrate the probability that large arboviruses epidemics could occur in northern Europe in next decades. They also tend to support the need to act on different levels to prevent these arboviruses from posing a serious public health threat in the coming decades.
Entomological surveillance of invasive mosquitoes species already exists and is coordinated at European level (107). However, on the field, some disparities exist in the degree and methods of surveillance around high-risk introduction routes (54). Resources to strengthen and improve coordination of entomological surveillance could therefore help to prevent the dissemination, especially of Ae. albopictus, in the already climatically suitable European regions. Furthermore, several initiatives have been taken to locally control the mosquito population, including eradication programs for Ae. aegypti in the Mediterranean (92) or the use of larvicides to control introduced populations in Belgium (36).
Despite the efforts put in the surveillance and control of invasive mosquitoes, it is likely that Ae. albopictus will finally become established in large parts of Europe, and some resources should be allocated to the next steps of the risk mitigation (49). One of these next steps have to be the increase of knowledge and awareness concerning arboviral diseases among healthcare workers (108, 109). Furthermore, an epidemiologic monitoring as soon as the vector is established could be effectively done by an entomo-virological surveillance followed by serologic surveys (110). Serological surveys are useful to monitor the level of viral circulation and take timely mitigation measures (111). They also show that, among travellers, the circulation of dengue is underestimated (112). However, the serological diagnosis of arboviruses is still a challenge in many ways, due to laboratory lack of preparedness and the technical challenge posed by cross-reactivity among arboviruses (113, 114). Efforts to improve laboratory preparedness, physician awareness and to develop accurate and cost-effective diagnostic tools would therefore help assess the current circulation of these arboviruses and prepare to respond in a timely manner to a future outbreak.
Finally, when basing assumptions on climate change modeling scenarios, it must not be neglected that the more unfavorable the mitigation scenario, the greater the uncertainty regarding temperature increases and climate extremes (1). Preparing for the inevitable consequences of the climate change should therefore not make us forget the crucial importance of limiting its (potentially unpredictable) impact by continuing efforts to reduce greenhouse gases and mitigate other human-mediated global changes.
Author contributions
JL: Conceptualization, Writing – original draft, Writing – review & editing. DD: Writing – review & editing. M-PH: Writing – review & editing. GD: Conceptualization, Writing – review & editing.
Funding
The author(s) declare financial support was received for the research, authorship, and/or publication of this article.
GD is a postdoctoral clinical master specialist for the Belgian National Fund for Scientific Re-search.
Acknowledgments
The authors thank Anne-Laure Lenoir (University of Liège, Belgium) for her insights from the early stages of this project. They also thank Haroun Jedidi and Arnaud Bourdoux for their careful proofreading and comments on the manuscript.
Conflict of interest
The authors declare that the research was conducted in the absence of any commercial or financial relationships that could be construed as a potential conflict of interest.
Publisher's note
All claims expressed in this article are solely those of the authors and do not necessarily represent those of their affiliated organizations, or those of the publisher, the editors and the reviewers. Any product that may be evaluated in this article, or claim that may be made by its manufacturer, is not guaranteed or endorsed by the publisher.
References
1. AR6 Synthesis Report: Climate Change 2023. Available at: https://www.ipcc.ch/report/ar6/syr/ (Cited June 6, 2023).
2. Rossati A. Global warming and its health impact. Int J Occup Environ Med. (2016) 8(1):7–20. doi: 10.15171/ijoem.2017.963
3. Romanello M, Di Napoli C, Drummond P, Green C, Kennard H, Lampard P, et al. The 2022 report of the lancet countdown on health and climate change: health at the mercy of fossil fuels. Lancet. (2022) 400(10363):1619–54. doi: 10.1016/S0140-6736(22)01540-9
4. Jones KE, Patel NG, Levy MA, Storeygard A, Balk D, Gittleman JL, et al. Global trends in emerging infectious diseases. Nature. (2008) 451(7181):990–3. doi: 10.1038/nature06536
5. Jánová E. Emerging and threatening vector-borne zoonoses in the world and in Europe: a brief update. Pathog Glob Health. (2019) 113(2):49–57. doi: 10.1080/20477724.2019.1598127
6. Eisen L, Monaghan AJ, Lozano-Fuentes S, Steinhoff DF, Hayden MH, Bieringer PE. The impact of temperature on the bionomics of Aedes (Stegomyia) aegypti, with special reference to the cool geographic range margins. J Med Entomol. (2014) 51(3):496–516. doi: 10.1603/ME13214
7. Beck-Johnson LM, Nelson WA, Paaijmans KP, Read AF, Thomas MB, Bjørnstad ON. The effect of temperature on anopheles mosquito population dynamics and the potential for malaria transmission. PLoS One. (2013) 8(11):e79276. doi: 10.1371/journal.pone.0079276
8. Hopp MJ, Foley JA. Global-Scale relationships between climate and the dengue fever vector, Aedes aegypti. Clim Change. (2001) 48(2):441–63. doi: 10.1023/A:1010717502442
9. Ogden NH, Lindsay LR. Effects of climate and climate change on vectors and vector-borne diseases: ticks are different. Trends Parasitol. (2016) 32(8):646–56. doi: 10.1016/j.pt.2016.04.015
10. Ma J, Guo Y, Gao J, Tang H, Xu K, Liu Q, et al. Climate change drives the transmission and spread of vector-borne diseases: an ecological perspective. Biology (Basel). (2022) 11(11):1628. doi: 10.3390/biology11111628
11. Kramer LD. Complexity of virus—vector interactions. Curr Opin Virol. (2016) 21:81–6. doi: 10.1016/j.coviro.2016.08.008
12. Mordecai EA, Caldwell JM, Grossman MK, Lippi CA, Johnson LR, Neira M, et al. Thermal biology of mosquito-borne disease. Ecol Lett. (2019) 22(10):1690–708. doi: 10.1111/ele.13335
13. Kilpatrick AM, Meola MA, Moudy RM, Kramer LD. Temperature, viral genetics, and the transmission of west Nile virus by culex pipiens mosquitoes. PLoS Pathog. (2008) 4(6):e1000092. doi: 10.1371/journal.ppat.1000092
14. Kulkarni MA, Duguay C, Ost K. Charting the evidence for climate change impacts on the global spread of malaria and dengue and adaptive responses: a scoping review of reviews. Global Health. (2022) 18:1. doi: 10.1186/s12992-021-00793-2
15. Robert MA, Stewart-Ibarra AM, Estallo EL. Climate change and viral emergence: evidence from aedes-borne arboviruses. Curr Opin Virol. (2020) 40:41–7. doi: 10.1016/j.coviro.2020.05.001
16. Daniel M, Danielová V, Kříž B, Jirsa A, Nožička J. Shift of the tick Ixodes ricinus and tick-borne encephalitis to higher altitudes in central Europe. Eur J Clin Microbiol Infect Dis. (2003) 22(5):327–8. doi: 10.1007/s10096-003-0918-2
17. Lindgren E, Tälleklint L, Polfeldt T. Impact of climatic change on the northern latitude limit and population density of the disease-transmitting European tick Ixodes ricinus. Environ Health Perspect. (2000) 108(2):119–23. doi: 10.1289/ehp.00108119
18. Ansari H, Shahbaz B, Izadi S, Zeinali M, Tabatabaee SM, Mahmoodi M, et al. Crimean-Congo hemorrhagic fever and its relationship with climate factors in southeast Iran: a 13-year experience. J Infect Dev Count. (2014) 8(06):749–57. doi: 10.3855/jidc.4020
19. Andersen LK, Davis MDP. Climate change and the epidemiology of selected tick-borne and mosquito-borne diseases: update from the international society of dermatology climate change task force. Int J Dermatol. (2017) 56(3):252–9. doi: 10.1111/ijd.13438
20. Van de Vuurst P, Escobar LE. Climate change and infectious disease: a review of evidence and research trends. Infect Dis Poverty. (2023) 12(1):51. doi: 10.1186/s40249-023-01102-2
21. Sadeghieh T, Waddell LA, Ng V, Hall A, Sargeant J. A scoping review of importation and predictive models related to vector-borne diseases, pathogens, reservoirs, or vectors (1999–2016). PLoS One. (2020) 15(1):e0227678. doi: 10.1371/journal.pone.0227678
22. Ogden NH, Maarouf A, Barker IK, Bigras-Poulin M, Lindsay LR, Morshed MG, et al. Climate change and the potential for range expansion of the lyme disease vector Ixodes scapularis in Canada. Int J Parasitol. (2006) 36(1):63–70. doi: 10.1016/j.ijpara.2005.08.016
23. Laporta GZ, Potter AM, Oliveira JFA, Bourke BP, Pecor DB, Linton YM. Global distribution of Aedes aegypti and Aedes albopictus in a climate change scenario of regional rivalry. Insects. (2023) 14(1):49. doi: 10.3390/insects14010049
24. Ryan SJ, Carlson CJ, Mordecai EA, Johnson LR. Global expansion and redistribution of Aedes-borne virus transmission risk with climate change. PLoS Negl Trop Dis. (2019) 13(3):e0007213. doi: 10.1371/journal.pntd.0007213
25. Ravasi D, Parrondo Monton D, Guidi V, Flacio E. Evaluation of the public health risk for autochthonous transmission of mosquito-borne viruses in southern Switzerland. Med Vet Entomol. (2020) 34(2):244–50. doi: 10.1111/mve.12421
26. Solimini AG, Manica M, Rosà R, della Torre A, Caputo B. Estimating the risk of dengue, chikungunya and Zika outbreaks in a large European city. Sci Rep. (2018) 8:16435. doi: 10.1038/s41598-018-34664-5
27. Naish S, Dale P, Mackenzie JS, McBride J, Mengersen K, Tong S. Climate change and dengue: a critical and systematic review of quantitative modelling approaches. BMC Infect Dis. (2014) 14(1):1–14. doi: 10.1186/1471-2334-14-167
28. Liu-Helmersson J, Quam M, Wilder-Smith A, Stenlund H, Ebi K, Massad E, et al. Climate change and Aedes vectors: 21st century projections for dengue transmission in Europe. EBioMedicine. (2016) 7:267–77. doi: 10.1016/j.ebiom.2016.03.046
29. Roehrig JT. West Nile virus in the United States — a historical perspective. Viruses. (2013) 5(12):3088–108. doi: 10.3390/v5123088
30. Conway MJ, Colpitts TM, Fikrig E. Role of the vector in arbovirus transmission. Annu Rev Virol. (2014) 1(1):71–88. doi: 10.1146/annurev-virology-031413-085513
31. Näslund J, Ahlm C, Islam K, Evander M, Bucht G, Lwande OW. Emerging mosquito-borne viruses linked to Aedes aegypti and Aedes albopictus: global status and preventive strategies. Vector-Borne Zoonotic Dis. (2021) 21(10):731–46. doi: 10.1089/vbz.2020.2762
32. Miranda MÁ, Barceló C, Arnoldi D, Augsten X, Bakran-Lebl K, Balatsos G, et al. AIMSurv: first pan-European harmonized surveillance of Aedes invasive mosquito species of relevance for human vector-borne diseases. GigaByte. (2022) 2022:gigabyte57. doi: 10.46471/gigabyte.57
33. European Centre for Disease Prevention and Control. Guidelines for the surveillance of invasive mosquitoes in Europe. LU: Publications Office (2012). Available at: https://data.europa.eu/doi/10.2900/61134 (Cited May 29, 2023).
34. Tomasello D, Schlagenhauf P. Chikungunya and dengue autochthonous cases in Europe, 2007–2012. Travel Med Infect Dis. (2013) 11(5):274–84. doi: 10.1016/j.tmaid.2013.07.006
35. Aedes albopictus—current known distribution: August 2023 (2023). Available at: https://www.ecdc.europa.eu/en/publications-data/aedes-albopictus-current-known-distribution-august-2023 (Cited October 23, 2023).
36. Deblauwe I, De Wolf K, De Witte J, Schneider A, Verlé I, Vanslembrouck A, et al. From a long-distance threat to the invasion front: a review of the invasive Aedes mosquito species in Belgium between 2007 and 2020. Parasit Vectors. (2022) 15:206. doi: 10.1186/s13071-022-05303-w
37. Medlock JM, Hansford KM, Versteirt V, Cull B, Kampen H, Fontenille D, et al. An entomological review of invasive mosquitoes in Europe. Bull Entomol Res. (2015) 105(6):637–63. doi: 10.1017/S0007485315000103
38. Paupy C, Delatte H, Bagny L, Corbel V, Fontenille D. Aedes albopictus, an arbovirus vector: from the darkness to the light. Microbes Infect. (2009) 11(14):1177–85. doi: 10.1016/j.micinf.2009.05.005
39. Thomas SM, Obermayr U, Fischer D, Kreyling J, Beierkuhnlein C. Low-temperature threshold for egg survival of a post-diapause and non-diapause European aedine strain, aedes albopictus (Diptera: culicidae). Parasit Vectors. (2012) 5(1):100. doi: 10.1186/1756-3305-5-100
40. Li Y, Kamara F, Zhou G, Puthiyakunnon S, Li C, Liu Y, et al. Urbanization increases aedes albopictus larval habitats and accelerates mosquito development and survivorship. PLoS Negl Trop Dis. (2014) 8(11):e3301. doi: 10.1371/journal.pntd.0003301
41. Benelli G, Wilke ABB, Beier JC. Aedes albopictus (Asian tiger mosquito). Trends Parasitol. (2020) 36(11):942–3. doi: 10.1016/j.pt.2020.01.001
42. Cunze S, Kochmann J, Koch LK, Klimpel S. Aedes albopictus and its environmental limits in Europe. PLoS One. (2016) 11(9):e0162116. doi: 10.1371/journal.pone.0162116
43. Kamal M, Kenawy MA, Rady MH, Khaled AS, Samy AM. Mapping the global potential distributions of two arboviral vectors Aedes aegypti and ae. Albopictus under changing climate. PLoS One. (2018) 13(12):e0210122. doi: 10.1371/journal.pone.0210122
44. Carvalho BM, Rangel EF, Vale MM. Evaluation of the impacts of climate change on disease vectors through ecological niche modelling. Bull Entomol Res. (2017) 107(4):419–30. doi: 10.1017/S0007485316001097
45. Aedes aegypti—current known distribution: August 2023 (2023). Available at: https://www.ecdc.europa.eu/en/publications-data/aedes-aegypti-current-known-distribution-august-2023 (Cited November 10, 2023).
46. Yang HM, Macoris MLG, Galvani KC, Andrighetti MTM, Wanderley DMV. Assessing the effects of temperature on the population of Aedes aegypti, the vector of dengue. Epidemiol Infect. (2009) 137(8):1188–202. doi: 10.1017/S0950268809002040
47. Yang HM, Macoris MLG, Galvani KC, Andrighetti MTM. Follow up estimation of Aedes aegypti entomological parameters and mathematical modellings. Biosystems. (2011) 103(3):360–71. doi: 10.1016/j.biosystems.2010.11.002
48. Christophers R. Aedes Aegypti (L.) the Yellow Fever Mosquito—It’s Life History, Bionomics and Structure. London: Cambridge University Press. (1960). p. 474–5.
49. Kraemer MUG, Reiner RC, Brady OJ, Messina JP, Gilbert M, Pigott DM, et al. Past and future spread of the arbovirus vectors Aedes aegypti and aedes albopictus. Nat Microbiol. (2019) 4(5):854–63. doi: 10.1038/s41564-019-0376-y
50. Wint W, Jones P, Kraemer M, Alexander N, Schaffner F. Past, present and future distribution of the yellow fever mosquito Aedes aegypti: the European paradox. Sci Total Environ. (2022) 847:157566. doi: 10.1016/j.scitotenv.2022.157566
51. Jourdain F, Roiz D, de Valk H, Noël H, L’Ambert G, Franke F, et al. From importation to autochthonous transmission: drivers of chikungunya and dengue emergence in a temperate area. PLoS Negl Trop Dis. (2020) 14(5):e0008320. doi: 10.1371/journal.pntd.0008320
52. Direction Générale de la Santé. Ministère de la Santé et de la Prévention. Cartes de présence du moustique tigre (Aedes albopictus) en France métropolitaine (2023). Available at: https://sante.gouv.fr/sante-et-environnement/risques-microbiologiques-physiques-et-chimiques/especes-nuisibles-et-parasites/article/cartes-de-presence-du-moustique-tigre-aedes-albopictus-en-france-metropolitaine (Cited October 23, 2023).
53. Medlock JM, Hansford KM, Vaux AGC, Cull B, Gillingham E, Leach S. Assessment of the public health threats posed by vector-borne disease in the United Kingdom (UK). Int J Environ Res Public Health. (2018) 15(10):2145. doi: 10.3390/ijerph15102145
54. Ibáñez-Justicia A, Smitz N, den Hartog W, van de Vossenberg B, De Wolf K, Deblauwe I, et al. Detection of exotic mosquito species (Diptera: culicidae) at international airports in Europe. Int J Environ Res Public Health. (2020) 17(10):3450. doi: 10.3390/ijerph17103450
55. Ibañez-Justicia A, Gloria-Soria A, den Hartog W, Dik M, Jacobs F, Stroo A. The first detected airline introductions of yellow fever mosquitoes (Aedes aegypti) to Europe, at schiphol international airport, The Netherlands. Parasit Vectors. (2017) 10:603. doi: 10.1186/s13071-017-2555-0
56. Schaffner F, Van Bortel W, Coosemans M. First record of Aedes (stegomyia) albopictus in Belgium. J Am Mosq Control Assoc. (2004) 20(2):201–3.15264633
57. Institut Belge de Santé. Sciensano. Le moustique tigre survit à l’hiver belge. Available at: https://www.sciensano.be/fr/coin-presse/le-moustique-tigre-survit-a-lhiver-belge (Cited October 23, 2023).
58. Martinet JP, Ferté H, Failloux AB, Schaffner F, Depaquit J. Mosquitoes of North-Western Europe as potential vectors of arboviruses: a review. Viruses. (2019) 11(11):1059. doi: 10.3390/v11111059
59. Schaffner F, Medlock JM, Van Bortel W. Public health significance of invasive mosquitoes in Europe. Clin Microbiol Infect. (2013) 19(8):685–92. doi: 10.1111/1469-0691.12189
60. Barzon L. Ongoing and emerging arbovirus threats in Europe. J Clin Virol. (2018) 107:38–47. doi: 10.1016/j.jcv.2018.08.007
61. Eckerle I, Briciu VT, Ergönül Ö, Lupşe M, Papa A, Radulescu A, et al. Emerging souvenirs—clinical presentation of the returning traveller with imported arbovirus infections in Europe. Clin Microbiol Infect. (2018) 24(3):240–5. doi: 10.1016/j.cmi.2018.01.007
62. Cleton N, Koopmans M, Reimerink J, Godeke GJ, Reusken C. Come fly with me: review of clinically important arboviruses for global travelers. J Clin Virol. (2012) 55(3):191–203. doi: 10.1016/j.jcv.2012.07.004
63. Johnson BW, Kosoy O, Martin DA, Noga AJ, Russell BJ, Johnson AA, et al. West Nile virus infection and serologic response among persons previously vaccinated against yellow fever and Japanese encephalitis viruses. Vector-Borne Zoonotic Dis. (2005) 5(2):137–45. doi: 10.1089/vbz.2005.5.137
64. Petersen LR, Powers AM. Chikungunya: epidemiology. F1000Research. (2016). Available at: https://f1000research.com/articles/5-82 (Cited June 3, 2023).
65. Robinson MC. An epidemic of virus disease in southern province, tanganyika territory, in 1952-53. I. Clinical features. Trans R Soc Trop Med Hyg. (1955) 49(1):28–32. doi: 10.1016/0035-9203(55)90080-8
66. Pialoux G, Gaüzère BA, Jauréguiberry S, Strobel M. Chikungunya, an epidemic arbovirosis. Lancet Infect Dis. (2007) 7(5):319–27. doi: 10.1016/S1473-3099(07)70107-X
67. Bosco-Lauth AM, Hartwig AE, Bowen RA. Reptiles and amphibians as potential reservoir hosts of chikungunya virus. Am J Trop Med Hyg. (2018) 98(3):841–4. doi: 10.4269/ajtmh.17-0730
68. Parola P, de Lamballerie X, Jourdan J, Rovery C, Vaillant V, Minodier P, et al. Novel chikungunya virus variant in travelers returning from Indian ocean islands. Emerg Infect Dis. (2006) 12(10):1493–9. doi: 10.3201/eid1210.060610
69. Taubitz W, Cramer JP, Kapaun A, Pfeffer M, Drosten C, Dobler G, et al. Chikungunya fever in travelers: clinical presentation and course. Clin Infect Dis. (2007) 45(1):e1–4. doi: 10.1086/518701
70. Lakshmi V, Neeraja M, Subbalaxmi MVS, Parida MM, Dash PK, Santhosh SR, et al. Clinical features and molecular diagnosis of chikungunya fever from south India. Clin Infect Dis. (2008) 46(9):1436–42. doi: 10.1086/529444
71. Rodríguez-Morales AJ, Cardona-Ospina JA, Fernanda Urbano-Garzón S, Sebastian Hurtado-Zapata J. Prevalence of post-chikungunya infection chronic inflammatory arthritis: a systematic review and meta-analysis. Arthritis Care Res (Hoboken). (2016) 68(12):1849–58. doi: 10.1002/acr.22900
72. de Brito CAA. Alert: severe cases and deaths associated with chikungunya in Brazil. Rev Soc Bras Med Trop. (2017) 50:585–9. doi: 10.1590/0037-8682-0479-2016
73. Enserink M. Massive outbreak draws fresh attention to little-known virus. Science. (2006) 311(5764):1085–1085. doi: 10.1126/science.311.5764.1085a
74. Schuffenecker I, Iteman I, Michault A, Murri S, Frangeul L, Vaney MC, et al. Genome microevolution of chikungunya viruses causing the Indian ocean outbreak. PLoS Med. (2006) 3(7):e263. doi: 10.1371/journal.pmed.0030263
75. Tsetsarkin KA, Vanlandingham DL, McGee CE, Higgs S. A single mutation in chikungunya virus affects vector specificity and epidemic potential. PLoS Pathog. (2007) 3(12):e201. doi: 10.1371/journal.ppat.0030201
76. Vazeille M, Moutailler S, Coudrier D, Rousseaux C, Khun H, Huerre M, et al. Two chikungunya isolates from the outbreak of la reunion (Indian ocean) exhibit different patterns of infection in the mosquito, aedes albopictus. PLoS One. (2007) 2(11):e1168. doi: 10.1371/journal.pone.0001168
77. Dubrulle M, Mousson L, Moutailler S, Vazeille M, Failloux AB. Chikungunya virus and Aedes mosquitoes: saliva is infectious as soon as two days after oral infection. PLoS One. (2009) 4(6):e5895. doi: 10.1371/journal.pone.0005895
78. Rezza G, Nicoletti L, Angelini R, Romi R, Finarelli A, Panning M, et al. Infection with chikungunya virus in Italy: an outbreak in a temperate region. Lancet. (2007) 370(9602):1840–6. doi: 10.1016/S0140-6736(07)61779-6
79. Roiz D, Boussès P, Simard F, Paupy C, Fontenille D. Autochthonous chikungunya transmission and extreme climate events in southern France. PLoS Negl Trop Dis. (2015) 9(6):e0003854. doi: 10.1371/journal.pntd.0003854
80. Delisle E, Rousseau C, Broche B, Leparc-Goffart I, L’Ambert G, Cochet A, et al. Chikungunya outbreak in Montpellier, France, September to October 2014. Eurosurveillance. (2015) 20(17):21108. doi: 10.2807/1560-7917.ES2015.20.17.21108
81. Vega-Rua A, Zouache K, Caro V, Diancourt L, Delaunay P, Grandadam M, et al. High efficiency of temperate Aedes albopictus to transmit chikungunya and dengue viruses in the southeast of France. PLoS One. (2013) 8(3):e59716. doi: 10.1371/journal.pone.0059716
82. Venturi G, Luca MD, Fortuna C, Remoli ME, Riccardo F, Severini F, et al. Detection of a chikungunya outbreak in central Italy, August to September 2017. Eurosurveillance. (2017) 22(39):17. doi: 10.2807/1560-7917.ES.2017.22.39.17-00646
83. Fortuna C, Toma L, Remoli ME, Amendola A, Severini F, Boccolini D, et al. Vector competence of Aedes albopictus for the Indian ocean lineage (IOL) chikungunya viruses of the 2007 and 2017 outbreaks in Italy: a comparison between strains with and without the E1:a226V mutation. Eurosurveillance. (2018) 23(22):1800246. doi: 10.2807/1560-7917.ES.2018.23.22.1800246
84. Heitmann A, Jansen S, Lühken R, Helms M, Pluskota B, Becker N, et al. Experimental risk assessment for chikungunya virus transmission based on vector competence, distribution and temperature suitability in Europe, 2018. Euro Surveill. (2018) 23(29):1800033. doi: 10.2807/1560-7917.ES.2018.23.29.1800033
85. Fischer D, Thomas SM, Suk JE, Sudre B, Hess A, Tjaden NB, et al. Climate change effects on chikungunya transmission in Europe: geospatial analysis of vector’s climatic suitability and virus’ temperature requirements. Int J Health Geogr. (2013) 12:51. doi: 10.1186/1476-072X-12-51
86. Gubler DJ. Dengue and dengue hemorrhagic fever. Clin Microbiol Rev. (1998) 11(3):480–96. doi: 10.1128/CMR.11.3.480
87. Messina JP, Brady OJ, Golding N, Kraemer MUG, Wint GRW, Ray SE, et al. The current and future global distribution and population at risk of dengue. Nat Microbiol. (2019) 4(9):1508–15. doi: 10.1038/s41564-019-0476-8
88. Vasilakis N, Cardosa J, Hanley KA, Holmes EC, Weaver SC. Fever from the forest: prospects for the continued emergence of sylvatic dengue virus and its impact on public health. Nat Rev Microbiol. (2011) 9(7):532–41. doi: 10.1038/nrmicro2595
89. Gwee SXW, St John AL, Gray GC, Pang J. Animals as potential reservoirs for dengue transmission: a systematic review. One Health. (2021) 12:100216. doi: 10.1016/j.onehlt.2021.100216
90. Cobra C, Rigau-Pérez JG, Kuno G, Vomdam V. Symptoms of dengue fever in relation to host immunologic response and virus serotype, Puerto Rico, 1990–1991. Am J Epidemiol. (1995) 142(11):1204–11. doi: 10.1093/oxfordjournals.aje.a117579
91. Alejandria MM. Dengue haemorrhagic fever or dengue shock syndrome in children. BMJ Clin Evid. (2015) 2015:0917. PMID: 25860404 PMCID: PMC439284225860404
92. Halstead SB. Pathogenesis of dengue: challenges to molecular biology. Science. (1988) 239(4839):476–81. doi: 10.1126/science.239.4839.476
93. Huang YJS, Higgs S, Vanlandingham DL. Emergence and re-emergence of mosquito-borne arboviruses. Curr Opin Virol. (2019) 34:104–9. doi: 10.1016/j.coviro.2019.01.001
94. Jetten TH, Focks DA. Potential changes in the distribution of dengue transmission under climate warming. Am J Trop Med Hyg. (1997) 57(3):285–97. doi: 10.4269/ajtmh.1997.57.285
95. Kobayashi D, Murota K, Fujita R, Itokawa K, Kotaki A, Moi ML, et al. Dengue virus infection in aedes albopictus during the 2014 autochthonous dengue outbreak in Tokyo metropolis, Japan. Am J Trop Med Hyg. (2018) 98(5):1460–8. doi: 10.4269/ajtmh.17-0954
96. Brady OJ, Golding N, Pigott DM, Kraemer MUG, Messina JP, Reiner RC Jr, et al. Global temperature constraints on Aedes aegypti and ae. Albopictus persistence and competence for dengue virus transmission. Parasit Vectors. (2014) 7(1):338. doi: 10.1186/1756-3305-7-338
97. Mordecai EA, Cohen JM, Evans MV, Gudapati P, Johnson LR, Lippi CA, et al. Detecting the impact of temperature on transmission of Zika, dengue, and chikungunya using mechanistic models. PLoS Negl Trop Dis. (2017) 11(4):e0005568. doi: 10.1371/journal.pntd.0005568
98. Xiao FZ, Zhang Y, Deng YQ, He S, Xie HG, Zhou XN, et al. The effect of temperature on the extrinsic incubation period and infection rate of dengue virus serotype 2 infection in Aedes albopictus. Arch Virol. (2014) 159(11):3053–7. doi: 10.1007/s00705-014-2051-1
99. Alto BW, Bettinardi D. Temperature and dengue virus infection in mosquitoes: independent effects on the immature and adult stages. Am J Trop Med Hyg. (2013) 88(3):497–505. doi: 10.4269/ajtmh.12-0421
100. Ruche GL, Souarès Y, Armengaud A, Peloux-Petiot F, Delaunay P, Desprès P, et al. First two autochthonous dengue virus infections in metropolitan France, September 2010. Eurosurveillance. (2010) 15(39):19676. doi: 10.2807/ese.15.39.19676-en
101. Marchand E, Prat C, Jeannin C, Lafont E, Bergmann T, Flusin O, et al. Autochthonous case of dengue in France, October 2013. Eurosurveillance. (2013) 18(50):20661. doi: 10.2807/1560-7917.ES2013.18.50.20661
102. Cochet A, Calba C, Jourdain F, Grard G, Durand GA, Guinard A, et al. Autochthonous dengue in mainland France, 2022: geographical extension and incidence increase. Euro Surveill. (2022) 27(44):2200818. doi: 10.2807/1560-7917.ES.2022.27.44.2200818
103. Succo T, Leparc-Goffart I, Ferré JB, Roiz D, Broche B, Maquart M, et al. Autochthonous dengue outbreak in nîmes, south of France, July to September 2015. Eurosurveillance. (2016) 21(21):30240. doi: 10.2807/1560-7917.ES.2016.21.21.30240
104. GISD. Available at: http://www.iucngisd.org/gisd/100_worst.php (Cited 2023 October 4).
105. Bortel WV, Dorleans F, Rosine J, Blateau A, Rousset D, Matheus S, et al. Chikungunya outbreak in the Caribbean region, December 2013 to march 2014, and the significance for Europe. Eurosurveillance. (2014) 19(13):20759. doi: 10.2807/1560-7917.ES2014.19.13.20759
106. Faria NR, Azevedo RSS, Kraemer MUG, Souza R, Cunha MS, Hill SC, et al. Zika Virus in the Americas: early epidemiological and genetic findings. Science. (2016) 352(6283):345–9. doi: 10.1126/science.aaf5036
107. European Centre for Disease Prevention and Control. Organisation of vector surveillance and control in Europe. LU: Publications Office (2021). Available at: https://data.europa.eu/doi/10.2900/853486 (Cited October 9, 2023).
108. Giang HTN, Sayed AM, Dang T, Iqtadar S, Tuan NM, Khiem NT, et al. Survey of knowledge, attitude and practice of healthcare professionals on dengue transmission, diagnosis and clinical classification. BMC Infect Dis. (2021) 21:1130. doi: 10.1186/s12879-021-06816-y
109. Riccò M, Zaniboni A, Satta E, Ranzieri S, Cerviere MP, Marchesi F, et al. West Nile virus infection: a cross-sectional study on Italian medical professionals during summer season 2022. Trop Med Infect Dis. (2022) 7(12):404. doi: 10.3390/tropicalmed7120404
110. Leandro AS, Lopes RD, Amaral Martins C, Delai RM, Villela DAM, Maciel-de-Freitas R. Entomo-virological surveillance followed by serological active survey of symptomatic individuals is helpful to identify hotspots of early arbovirus transmission. Front Public Health. (2022) 10:1024187. doi: 10.3389/fpubh.2022.1024187
111. Pem-Novosel I, Vilibic-Cavlek T, Gjenero-Margan I, Kaic B, Babic-Erceg A, Merdic E, et al. Dengue virus infection in Croatia: seroprevalence and entomological study. New Microbiologica. (2015) 38(1):97–100.25821943
112. Allwinn R, Hofknecht N, Doerr HW. Dengue in travellers is still underestimated. Intervirology. (2008) 51(2):96–100. doi: 10.1159/000131667
113. Reusken CB, Ieven M, Sigfrid L, Eckerle I, Koopmans M. Laboratory preparedness and response with a focus on arboviruses in Europe. Clin Microbiol Infect. (2018) 24(3):221–8. doi: 10.1016/j.cmi.2017.12.010
Keywords: one health, Aedes albopictus, chikungunya (CHIKV), dengue (DENV), emerging disease, Northern Europe, arbovirosis
Citation: Laverdeur J, Desmecht D, Hayette M-P and Darcis G (2024) Dengue and chikungunya: future threats for Northern Europe?. Front. Epidemiol. 4:1342723. doi: 10.3389/fepid.2024.1342723
Received: 22 November 2023; Accepted: 2 January 2024;
Published: 15 January 2024.
Edited by:
Ali Davod Parsa, Anglia Ruskin University, United KingdomReviewed by:
Mariana Alejandra Rivero, Universidad Nacional del Centro de Buenos Aires, Argentina© 2024 Laverdeur, Desmecht, Hayette and Darcis. This is an open-access article distributed under the terms of the Creative Commons Attribution License (CC BY). The use, distribution or reproduction in other forums is permitted, provided the original author(s) and the copyright owner(s) are credited and that the original publication in this journal is cited, in accordance with accepted academic practice. No use, distribution or reproduction is permitted which does not comply with these terms.
*Correspondence: Justine Laverdeur justinelaverdeur@gmail.com