- 1Department of Environmental Health Sciences, University of Michigan School of Public Health, Ann Arbor, MI, United States
- 2Department of Communication Sciences and Disorders, Northeastern University, Boston, MA, United States
- 3Department of Epidemiology and Biostatistics, University of Georgia, Athens, GA, United States
- 4UPR Medical Sciences Campus, University of Puerto Rico Graduate School of Public Health, San Juan, Puerto Rico
- 5College of Engineering, Northeastern University, Boston, MA, United States
Background/Aim: Infant non-nutritive suck (NNS) has been used as an early marker of neonatal brain function. Although there is an established relationship between prenatal exposure to certain metals and brain development, the association between metal exposure and NNS has not been explored. Therefore, in this study we assessed associations between maternal urinary metal(loid) concentrations and NNS measurements among infants from the Puerto Rico PROTECT birth cohort. We hypothesized that maternal urinary metal(loid) concentrations are significantly associated with infant NNS measures in a sex-dependent manner.
Methods: We measured urinary concentrations of 14 metal(loid)s in pregnant women at up to three time points in pregnancy. The geometric mean of each metal(loid) for each pregnant woman was calculated and used as an exposure measurement across gestation. NNS measurements (duration, frequency, amplitude, bursts/min, cycles/burst, cycles/min) were collected from infants between 4 and 6 (±2 weeks) weeks of age using our custom research pacifier. Linear regression was used to estimate associations between urinary metal(loid) concentrations across pregnancy and continuous NNS variables. Sex-specific effects were estimated using interaction terms between NNS variables and infant sex.
Results: We observed significant positive associations between mercury, manganese, and tin with NNS duration (mercury: %Δ = 1.08, 95% CI: 0.42, 1.74; manganese: %Δ = 0.67, 95% CI: 0.15, 1.20; tin: %Δ = 0.83, 95% CI: 0.17, 1.49) and NNS cycles/burst (mercury: %Δ = 1.85, 95% CI: 0.58, 3.11; manganese: (%Δ = 1.37, 95% CI: 0.40, 2.34; tin: %Δ = 1.68, 95% CI: 0.46, 2.91). Furthermore, the association between NNS cycles/min with cadmium (%Δ = 8.06, 95% CI: 3.33, 12.78), manganese (%Δ = 4.44, 95% CI: 1.40, 7.47), and tin (%Δ = 4.50, 95% CI: 0.81, 8.18) were in the opposite direction from its association with zinc (%Δ = −9.30, 95% CI: −14.71, −3.89), as well as with copper (%Δ = −6.58, 95% CI: −12.06, −1.10). For the sex-stratified analysis, the negative associations between metal(loid)s and NNS duration were predominantly driven by male infants; however, the negative associations between metal(loid)s and NNS bursts/min were mainly driven by female infants.
Conclusion: We observed significant associations between prenatal metal(loid) exposure and NNS measurements among infants from the ongoing Puerto Rico PROTECT cohort. Similar to previous studies that have demonstrated associations between NNS and subsequent neurodevelopment, this study highlights the potential of NNS as a quantitative index to measure altered neurodevelopment from prenatal metal(loid) exposures. We believe this study will inform future efforts aimed at reducing health risks related to early life metal exposures, such as developing early identification of metal-induced adverse outcomes in child neurodevelopment.
Introduction
Neurofunction is often assessed at an early stage of life, as infancy is a critical period for brain development. Unfortunately, many common neurodevelopmental tests administered during infancy tend to be relatively unsuccessful at predicting future neurobehavioral function (1–3). Notably, Anderson et al. observed significant over- and under- estimation of developmental delays in two-year-old Australian children using the Bayley Scales of Infant Development 3rd edition (BSID-III) (1, 2). Therefore, better technology to assess infant brain development is greatly needed.
Non-nutritive suck (NNS) is an emerging novel tool for environmental epidemiology and could be used as an early marker of central nervous system (CNS) function (4–6). NNS is defined as sucking without any nutrients being delivered. NNS is controlled by the brainstem and generally starts in utero at around 15 weeks of gestation (7). Several studies have demonstrated a link between infant NNS patterning and subsequent development (8–12), providing evidence that NNS could be used as an indicator of exposure-related disruptions in CNS function in infants.
Recently, Zimmerman et al. observed significant associations between prenatal phthalate exposure and NNS among infants from PROTECT (n = 280) (13). This study highlighted the potential of NNS as a novel tool for early detection of prenatal environmental exposure-induced adverse effects on neurodevelopment. Other common neurotoxic environmental exposures are metals. Although metal-induced adverse child neurodevelopment is well established (14–17), the clear relationship between infant NNS and prenatal metal exposure is yet to be explored. Furthermore, the evidence for sex-specific toxicological effects is mounting (18–22); however, the clear understanding of the role of infant sex on metal-induced child neurodevelopmental impairment remains limited.
The overarching goal of this study was to investigate associations between prenatal metal(loid)s exposure and child neurodevelopment, using NNS measurements, among infants from the ongoing Puerto Rico PROTECT birth cohort. We hypothesized that maternal urinary metal(loid) concentrations are significantly associated with the NNS measures in an infant sex-dependent manner. We believe this study will inform future efforts aimed at developing early identification of metal exposure-induced adverse outcomes in child neurodevelopment and contribute to facilitating proactive medical care.
Methods
This study sample is a subset of the Puerto Rico PROTECT cohort. The PROTECT cohort began recruitment in 2010 through funding from the National Institute of Environmental Health Sciences Superfund Research Program. Each pregnant woman participated in a total of up to three study visits (18 ± 2 weeks, 22 ± 2 weeks, and 26 ± 2 weeks of gestation). Inclusion criteria for recruitment included: participant age between 18 and 40 years; residence in the Northern Karst aquifer region; disuse of oral contraceptives three months before pregnancy; disuse of in vitro fertilization; and no indication in medical records for major obstetrical complications, including pre-existing diabetes. This study was approved by the research and ethics committees of the University of Michigan School of Public Health, University of Puerto Rico, Northeastern University, and participating hospitals and clinics. All methods reported in this study were performed in accordance with relevant guidelines and regulations imposed by those institutions. All study participants provided full informed consent prior to participation.
From 2017 to 2019, children born to PROTECT mothers were then recruited into the Center for Research on Early Childhood Exposure and Development in Puerto Rico, or CRECE study (23). In order to be included in the present analysis, infants were required to be born full-term (≥37 weeks' gestation), and have both an NNS measurement sampled in infancy and maternal urinary metal(loid)s concentrations from at least one urine sample collected during pregnancy.
Urinary metal(loid)s measurements
The samples were collected at up to three study visits per participant (18 ± 2 weeks, 22 ± 2 weeks, and 26 ± 2 weeks' of gestation). Spot urine samples were collected in sterile polypropylene cups, divided into aliquots, frozen at −8°C, and shipped on dry ice to NSF International (Ann Arbor, MI, USA) for analysis. Concentrations of 14 metal(loid)s were measured in urine: arsenic (As), barium (Ba), cadmium (Cd), cobalt (Co), cesium (Cs), copper (Cu), mercury (Hg), manganese (Mn), molybdenum (Mo), nickel (Ni), selenium (Se), tin (Sn), thallium (Tl), and zinc (Zn). Metal(loid) concentrations were measured using inductively coupled plasma mass spectrometry (ICPMS) as described previously (24). Urinary specific gravity (SG) was measured at the University of Puerto Rico Medical Sciences Campus using a hand-held digital refractometer (Atago Co., Ltd., Tokyo, Japan) as an indicator of urine dilution.
Infant NNS measurements
Infants born to PROTECT mothers and enrolled in CRECE attended a follow-up study visit at 4–6 weeks (±2 weeks) weeks of age for measurements of NNS in our clinic in Manati, Puerto Rico. To assess NNS, we utilized our custom research pacifier, which yields quantitative NNS data in real-time and the collection process is described in previous studies (13, 25–28). Briefly, once the device was calibrated, the parents were instructed to hold the infant and offer the pacifier to their child for approximately five minutes. All data were analyzed using LabChart software (ADInstruments). NNS bursts were manually selected using the following criteria: bursts containing two or more suck cycles, each suck cycle's amplitude over 1 cm H2O, and a new burst if there is a break of >1,000 ms between cycles. These criteria are consistent with previous studies examining NNS in young infants (25, 26, 28, 29). After manually selecting each suck sample, they were entered into a custom NNS Burst macro for processing of the following burst variables: duration (s), frequency (Hz), amplitude (cmH2O), bursts/min, cycles/burst, cycles/min.
Statistical analysis
We summarized the distribution of urinary metal(loid) concentrations across pregnancy, calculating geometric means, standard deviations, and select percentiles. Urinary metal(loid) concentrations were log-normally distributed and thus were natural log-transformed for all subsequent analyses. We also calculated distributions of NNS outcome measures for all term infants (n = 116). NNS measures were approximately normally distributed, and thus not transformed prior to analyses. We used multiple linear regression to estimate associations between individual urinary metal(loid) concentrations across pregnancy and continuous NNS variables. Resulting models adjusted for maternal age at assessment, infant sex, birth weight, and SG. Effect estimates were calculated as the percent change in NNS outcome measure compared to the population median per interquartile range (IQR) increase in urinary metal(loid) concentration. The significance level was set to alpha = 0.05.
We then conducted sensitivity analyses to explore possible effect modification by infant sex on associations between urinary metal(loid) concentrations and NNS measures. We first included metal*sex interaction terms in regression models, and if significant (p-value for interaction term <0.2), we then stratified regression models by infant sex.
Results
The demographic and health characteristics of the study participants are presented in Table 1. The mean age of the mother participants was 28.8 years, approximately 60% had earned tertiary education, the majority (82.5%) had an annual household income of less than $50,000, and 74.8% were employed. Most participants had never smoked (93.0%) and did not drink during pregnancy (97.4%). Infant sex was evenly distributed among female (n = 59, 50.9%) and male infants (n = 57, 49.1%). The whole PROTECT cohort demographic and health characteristics are also presented for comparison in Table 1. The notable differences between the groups include maternal age, education, smoking status, and alcohol use. The mean age of the overall PROTECT cohort was 26.8 years, less than 50% had earned tertiary education, 82.8% of the participants had never smoked, and 94.8% of the participants did not drink during pregnancy.
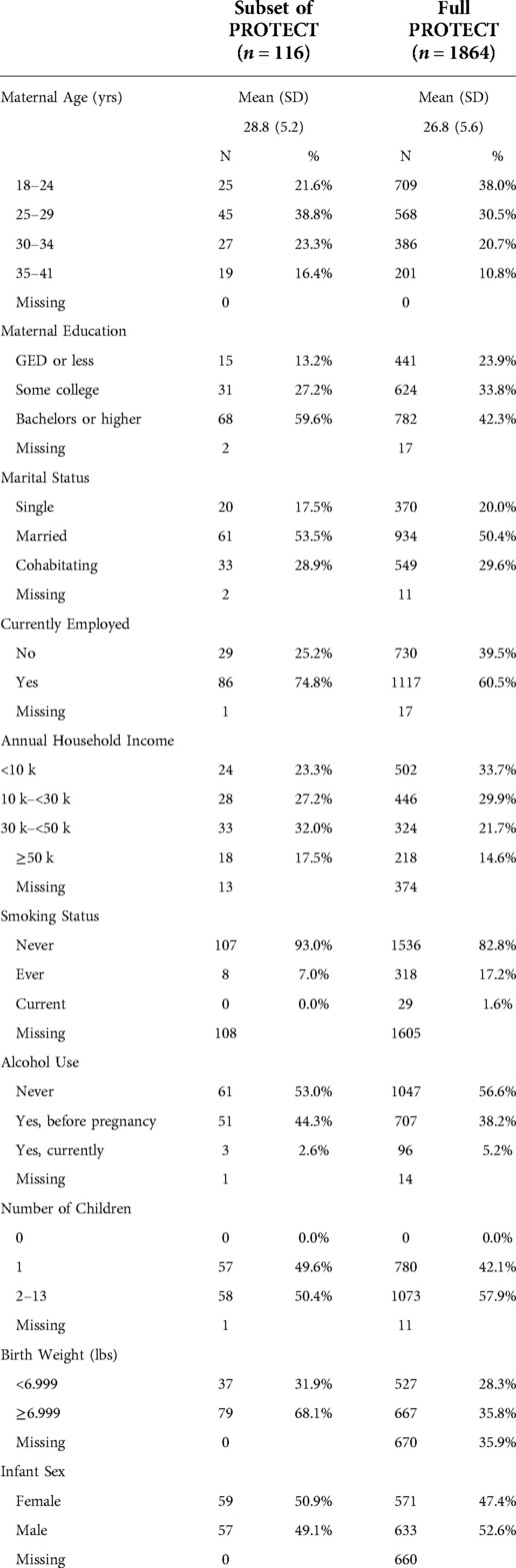
Table 1. Demographic and other relevant health information on 116 pregnant women, compared to the full PROTECT cohort.
Table 2 shows the distributions of maternal urinary metal(loid) concentrations, including As, Ba, Cd, Co, Cs, Cu, Hg, Mn, Mo, Ni, Se, Sn, Tl, and Zn. The majority of metal(loid)s analyzed were above the LOD in at least 80% of samples. The only exceptions were Cd (62.7% at visit 1 and 57.5% at visit 3), Mn (69.0% at visit 3), and Tl (77.8% at visit 1 and 65.9% at visit 3). NNS measurements were available for 116 term infants, with means and standard deviations (SD) according to sociodemographic variables shown in Table 3. On average, infants from the cohort had an NNS duration of 6.51 s, a frequency of 1.90 Hz, and a height of 16.93 CmH2O with 6.23 bursts/minute, 12.11 cycles/burst, and 62.78 cycles/minute.
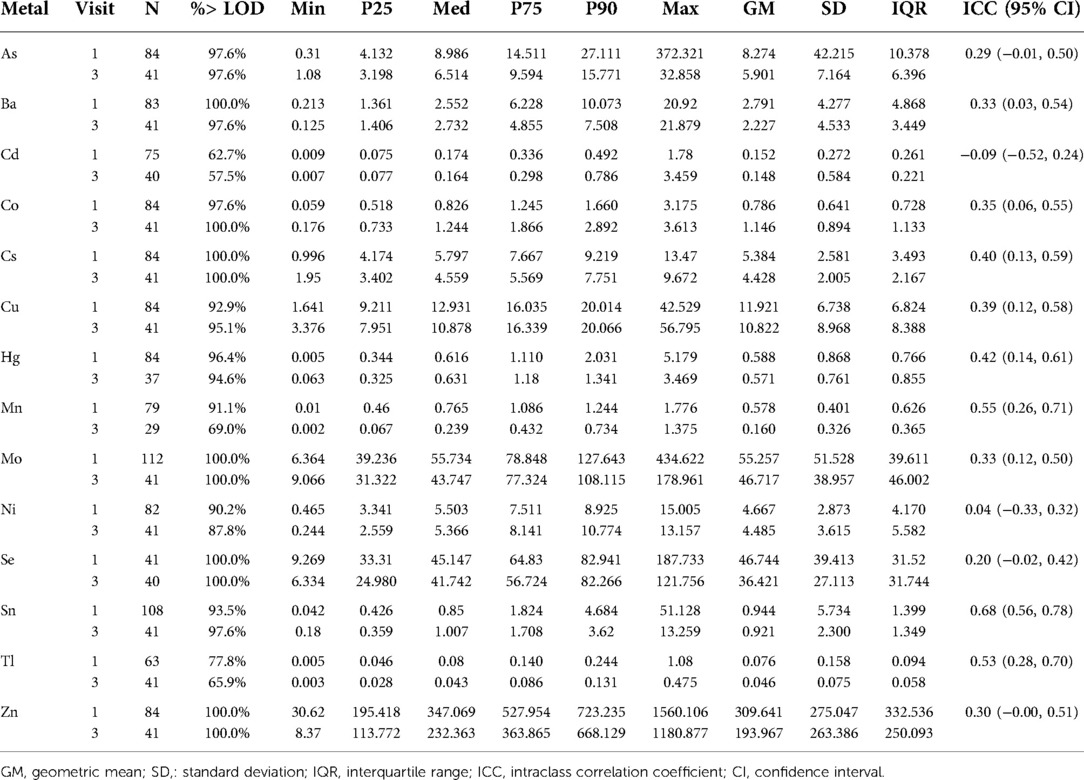
Table 2. Distributions of urinary metal concentrations (ng/ml), by study visit, among 116 women in PROTECT.
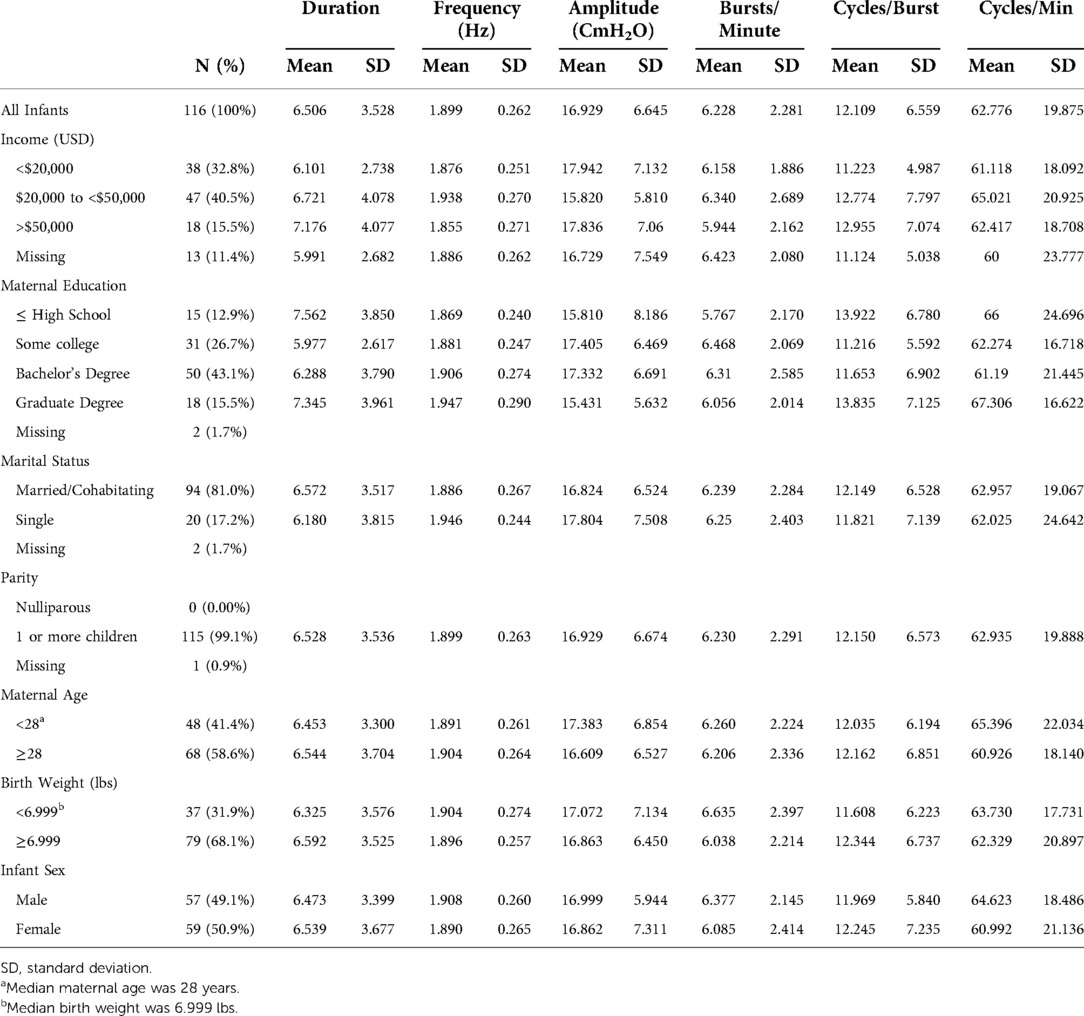
Table 3. NNS measurements among PROTECT infants according to sociodemographic characteristics (n = 116).
The associations between maternal urinary metal(loid) concentrations and NNS measurements are shown in Supplementary Table S1. After adjustment for infant sex, birth weight, and urinary SG, an IQR increase in Hg concentrations across pregnancy was associated with a 1.08% increase in NNS duration (95% CI: 0.42, 1.74) and 1.85% increase in cycles/burst (95% CI: 0.59, 3.11). Further, Mn concentrations were associated with 0.67% increase (95% CI: 0.15, 1.20) in duration, 1.37% increase (95% CI: 0.40, 2.34) in cycles/burst, and 4.44% increase (95% CI: 1.40, 7.47) in cycles/min. Similar to Mn, Sn concentrations were associated with 0.83% increase (95% CI: 0.17, 1.49) in duration, 1.68% increase (95% CI: 0.46, 2.91), and 4.50% increase (95% CI: 0.81, 8.18) in cycles/min. Also, an IQR increase in Cd concentrations was associated with an 8.06% increase (95% CI: 3.33, 12.78) in cycles/min. Conversely, Zn (%Δ = −9.30, 95% CI: −14.71, −3.89) was negatively associated with cycles/min.
We observed infant sex-specific differences in the associations between urinary metal(loid) concentrations and NNS measures shown in Figure 1 (corresponding effect estimates and confidence intervals are shown in Supplementary Table S2). Among the significant associations (p-value > 0.2), we observed negative associations between metal(loid)s (As, Ba, Cs, Cu, and Ni) concentrations and NNS duration predominantly in male infants. In contrast, we observed negative associations between the metal(loid)s and NNS bursts/min mainly in female infants (Figure 1 and Supplementary Table S2).
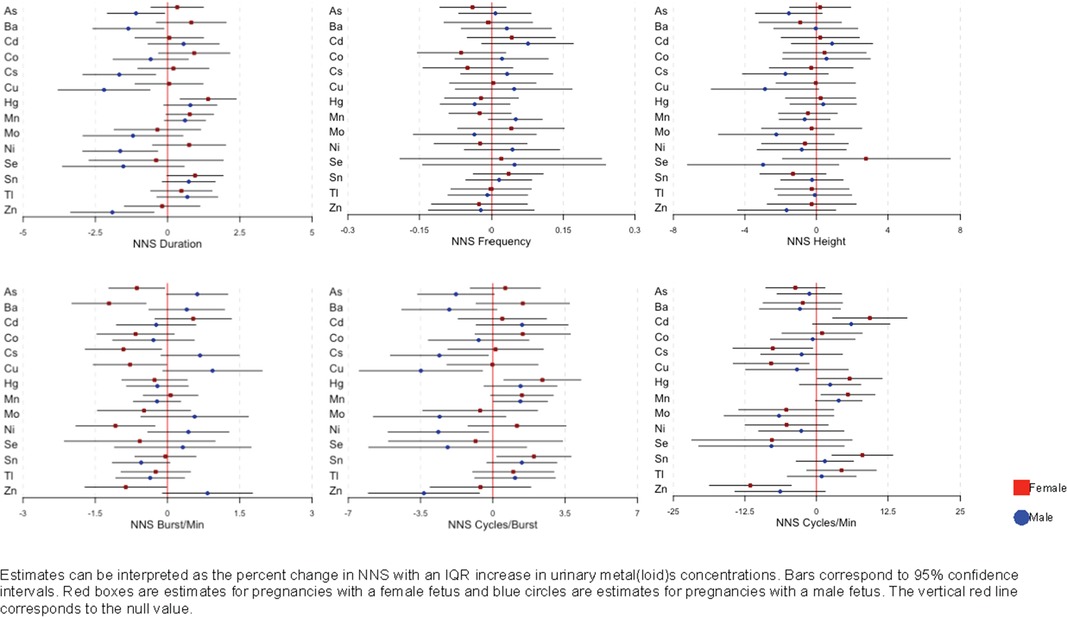
Figure 1. Associations and 95% confidence intervals between maternal urinary metal(loid)s concentrations and NNS, by infant sex, among 116 women in PROTECT, adjusted for urinary specific gravity, maternal age at assessment, infant sex, and birth weight.
Discussion
The pregnant mothers in the ongoing Puerto Rico PROTECT birth cohort are exposed to a variety of environmental contaminants, including metals. The associations between metal exposure and neurodevelopment are well-established (14–17); however, the clear relationship between infant NNS and prenatal metal exposure is understudied. Here, our current study observed the associations between prenatal metal(loid)s exposure and infant NNS among participants in PROTECT, as well as infant sex-specific differences in the associations.
In this study, we observed significant associations between NNS and maternal urinary metal(loid) concentrations of the study participants in the PROTECT cohort. More specifically, positive associations were observed between Hg with NNS duration and cycles/burst, Mn with duration, cycles/burst, and cycles/min, and Cd with cycles/min. Previous studies have demonstrated that infant NNS is linked to subsequent neurodevelopment, such as verbal intelligence, language, and motor skills (11, 12). Consistently, Martens et al. observed that infants with more cycles/burst and cycles/min with longer burst duration have lower cognitive developmental scores at 12 months (±2 weeks) (n = 50) (30). Together, our observations suggest that urinary concentrations of these metals may be associated with poor neurodevelopmental outcomes. Unlike Hg and Cd, Mn is an essential metal; therefore, deficient or excessive levels of Mn could result in biological dysfunction. Specifically, excessive Mn exposure can result in neurodevelopmental impairment (31–34). For example, Claus Henn et al. observed adverse effects on infant neurodevelopment from early-life both low and high Mn exposures, emphasizing the dual role of Mn as an essential metal and a toxicant (31). Hg and Cd are also well-established neurotoxic metals, and there is mounting evidence that suggests associations between prenatal exposure to these metals and neurodevelopment in children (35–40). Unlike the other urinary metal(loid)s we measured, Zn had a negative association with NNS cycles/min. Similar to Mn, Zn is also an essential metal, and excess levels of Zn can be neurotoxic (41). Although Mn and Zn have similar chemical properties, their differences in liganding properties may partially explain the opposite associations between Mn and Zn with NNS (42); however, more studies are needed to substantiate the present observation. Together, our observations suggest that prenatal metal(loid)s exposures may alter NNS patterning in full-term infants. Future studies are warranted to obtain a clear understanding of the relationship between infant NNS patterning and subsequent neurodevelopment, as well as to establish typical NNS scores during infancy and across populations.
We also observed infant sex-specific differences in associations between NNS and urinary metal(loid) concentrations. Specifically, we observed significant negative associations between NNS duration and metal(loid) concentrations (As, Ba, Cs, Cu, and Ni) predominantly in male infants; however, we observed significant negative associations between NNS bursts/min and the metal(loid) concentrations mainly in female infants. As mentioned above, in a previous study, lower cognitive developmental scores were observed in infants with more cycles/burst (30). Though it did not reach statistical significance, we observed positive associations between NNS cycles/burst and the metal(loid) concentrations predominantly in female infants. Together, our observations suggest that female infants may be more susceptible to poor neurodevelopmental outcomes from prenatal metal exposures. Because As, Ba, Cs, Cu, and Ni are strongly associated with NNS duration and bursts/min in an infant sex-dependent manner, it is possible that these metal(loid)s affect infant NNS patterning by modulating the levels of sex hormones. Several studies have demonstrated that metals, including As and Cu, can modulate the levels of free sex hormones (43–45), which can directly affect cognitive functions. Consistently, Martens et al. have demonstrated that early NNS is linked to an early cognitive screener (30). However, due to limited studies on different types and levels of hormones between sex, more studies are necessary to be more conclusive in determining the effect of prenatal metal(loid) exposures on sex hormonal disturbance-induced cognitive impairment between sexes.
There are important limitations in our study to note. One limitation of our study is the modest sample size. Therefore, future investigations with a larger sample size could provide a better understanding of prenatal metal(loid)s exposures and infant NNS associations. Further, metal concentrations in prenatal urine are a measure of gestational exposure when NNS patterning is developing; however, collection of infant urine samples for biomarkers of postnatal metals exposure could be valuable in future studies. Lastly, our observations may not be generalizable to other populations due to our study focus on an underrepresented community in the U.S.
Despite these limitations, it is important to highlight the strengths of our study. This study is the first to investigate the associations between infant NNS and prenatal metal(loid) exposures. Notably, we observed infant sex-specific differences in the associations between maternal urinary metal(loid) concentrations and infant NNS patterning. This observation highlights the importance of considering infant sex as an independent factor in studying child neurodevelopment. It is also important to highlight that despite the modest sample size, we observed significant associations between maternal urinary metal(loid) concentrations and infant NNS, further emphasizing the need to assess the associations with a larger sample size across populations and geographical regions. Additionally, we conducted this preliminary analysis in an established birth cohort among an underrepresented population of pregnant women at risk for elevated environmental exposures. Results from these data are valuable as they contribute to improving current public health policy, as well as the overall quality of population health by promoting future research activities to both underserved and general populations.
Conclusions
In this exploratory study we present new information that may contribute to our understanding of prenatal metal exposure-induced adverse effects on child neurodevelopment. We observed significant associations between infant NNS and urinary metal(loid) concentrations in the PROTECT cohort. We also reported the modifying effects of infant sex on the associations between NNS and urinary metal(loid)s concentrations. Taken together, these findings highlight the relationship between prenatal metal(loid) exposures and altered infant NNS patterning, which may provide more targeted strategies to develop early detection tools for adverse child neurodevelopment. Further, this study warrants future work to explore the biological mechanisms by which prenatal metal(loid) exposures might influence infant NNS patterning.
Acknowledgments
We thank the nurses and research staff who participated in cohort recruitment and follow up, as well as the Federally Qualified Health Centers (FQHC) and clinics in Puerto Rico who facilitated participant recruitment, including Morovis Community Health Center (FQHC), Prymed: Ciales Community Health Center (FQHC), Camuy Health Services, Inc. (FQHC), and the Delta OBGyn (Prenatal Clinic).
Data availability statement
The raw data supporting the conclusions of this article will be made available by the authors, without undue reservation.
Ethics statement
The studies involving human participants were reviewed and approved by University of Michigan School of Public Health University of Puerto Rico Northeastern University. The patients/participants provided their written informed consent to participate in this study
Author contributions
C.K.: Investigation; Writing- original draft; Formal analysis. E.Z.: Conceptualization; Writing- review and editing; Funding acquisition. G.H.M: Data acquisition. Z.R.: Data curation; Project administration. C.M.V.: Project administration; Funding acquisition. A.A.: Conceptualization; Funding acquisition. J.F.C.: Conceptualization, Funding acquisition. J.D.M.: Conceptualization; Funding acquisition; Supervision. D.J.W.: Conceptualization; Writing- review and editing; Funding acquisition; Supervision. All authors contributed to the article and approved the submitted version.
Funding
This study was supported by the Superfund Research Program of the National Institute of Environmental Health Sciences, National Institutes of Health (P42ES017198). Additional support was provided from NIEHS grant numbers T32ES007062, P50ES026049, and P30ES017885 and the Environmental influences on Child Health Outcomes (ECHO) program grant number UH3OD023251.
Conflict of interest
The authors declare that the research was conducted in the absence of any commercial or financial relationships that could be construed as a potential conflict of interest.
Publisher's note
All claims expressed in this article are solely those of the authors and do not necessarily represent those of their affiliated organizations, or those of the publisher, the editors and the reviewers. Any product that may be evaluated in this article, or claim that may be made by its manufacturer, is not guaranteed or endorsed by the publisher.
Supplementary material
The Supplementary Material for this article can be found online at: https://www.frontiersin.org/articles/10.3389/fepid.2022.1057515/full#supplementary-material.
References
1. Anderson PJ, De Luca CR, Hutchinson E, Roberts G, Doyle LW, G. Victorian Infant Collaborative. Underestimation of developmental delay by the new Bayley-III Scale. Arch Pediatr Adolesc Med. (2010) 164(4):352–6. doi: 10.1001/archpediatrics.2010.20
2. Anderson PJ, Burnett A. Assessing developmental delay in early childhood - concerns with the Bayley-III scales. Clin Neuropsychol. (2017) 31(2):371–81. doi: 10.1080/13854046.2016.1216518
3. Spencer-Smith MM, Spittle AJ, Lee KJ, Doyle LW, Anderson PJ. Bayley-III Cognitive and Language Scales in Preterm Children. Pediatrics. (2015) 135(5):e1258–65. doi: 10.1542/peds.2014-3039
4. Zimmerman E, Foran M. Patterned auditory stimulation and suck dynamics in full-term infants. Acta Paediatr. (2017) 106(5):727–32. doi: 10.1111/apa.13751
5. Zimmerman E, Forlano J, Gouldstone A. Not All Pacifiers Are Created Equal: a Mechanical Examination of Pacifiers and Their Influence on Suck Patterning. Am J Speech Lang Pathol. (2017) 26(4):1202–12. doi: 10.1044/2017_AJSLP-16-0226
6. Pineda R, Dewey K, Jacobsen A, Smith J. Non-Nutritive Sucking in the Preterm Infant. Am J Perinatol. (2019) 36(3):268–76. doi: 10.1055/s-0038-1667289
7. Humphrey T. The development of human fetal activity and its relation to postnatal behavior. Adv Child Dev Behav. (1970) 5:1–57. doi: 10.1016/S0065-2407(08)60464-4
8. Medoff-Cooper B, Ray W. Neonatal sucking behaviors. Image J Nurs Sch. (1995) 27(3):195–200. doi: 10.1111/j.1547-5069.1995.tb00858.x
9. Malas K, Trudeau N, Chagnon M, McFarland DH. Feeding-swallowing difficulties in children later diagnosed with language impairment. Dev Med Child Neurol. (2015) 57(9):872–9. doi: 10.1111/dmcn.12749
10. Malas K, Trudeau N, Giroux MC, Gauthier L, Poulin S, McFarland DH. Prior History of Feeding-Swallowing Difficulties in Children With Language Impairment. Am J Speech Lang Pathol. (2017) 26(1):138–45. doi: 10.1044/2016_AJSLP-15-0171
11. Wolthuis-Stigter MI, Luinge MR, da Costa SP, Krijnen WP, van der Schans CP, Bos AF. The association between sucking behavior in preterm infants and neurodevelopmental outcomes at 2 years of age. J Pediatr. (2015) 166(1):26–30. doi: 10.1016/j.jpeds.2014.09.007
12. Wolthuis-Stigter MI, Da Costa SP, Bos AF, Krijnen WP, Van Der Schans CP, Luinge MR. Sucking behaviour in infants born preterm and developmental outcomes at primary school age. Dev Med Child Neurol. (2017) 59(8):871–7. doi: 10.1111/dmcn.13438
13. Zimmerman E, Watkins DJ, Huerta-Montanez G, Rosario Pabon Z, Feric Z, Manjourides J, et al. Associations of gestational phthalate exposure and non-nutritive suck among infants from the Puerto Rico Testsite for Exploring Contamination Threats (PROTECT) birth cohort study. Environ Int. (2021) 152:106480. doi: 10.1016/j.envint.2021.106480
14. Frye RE, Cakir J, Rose S, Delhey L, Bennuri SC, Tippett M, et al. Early life metal exposure dysregulates cellular bioenergetics in children with regressive autism spectrum disorder. Transl Psychiatry. (2020) 10(1):223. doi: 10.1038/s41398-020-00905-3
15. Sanders AP, Claus Henn B, Wright RO. Perinatal and Childhood Exposure to Cadmium, Manganese, and Metal Mixtures and Effects on Cognition and Behavior: a Review of Recent Literature. Curr Environ Health Rep. (2015) 2(3):284–94. doi: 10.1007/s40572-015-0058-8
16. Shah-Kulkarni S, Lee S, Jeong KS, Hong YC, Park H, Ha M, et al. Prenatal exposure to mixtures of heavy metals and neurodevelopment in infants at 6 months. Environ Res. (2020) 182:109122. doi: 10.1016/j.envres.2020.109122
17. Sarigiannis DA, Papaioannou N, Handakas E, Anesti O, Polanska K, Hanke W, et al. Neurodevelopmental exposome: the effect of in utero co-exposure to heavy metals and phthalates on child neurodevelopment. Environ Res. (2021) 197:110949. doi: 10.1016/j.envres.2021.110949
18. Vahter M, Akesson A, Liden C, Ceccatelli S, Berglund M. Gender differences in the disposition and toxicity of metals. Environ Res. (2007) 104(1):85–95. doi: 10.1016/j.envres.2006.08.003
19. Llop S, Lopez-Espinosa MJ, Rebagliato M, Ballester F. Gender differences in the neurotoxicity of metals in children. Toxicology. (2013) 311(1-2):3–12. doi: 10.1016/j.tox.2013.04.015
20. Rechtman E, Curtin P, Papazaharias DM, Renzetti S, Cagna G, Peli M, et al. Sex-specific associations between co-exposure to multiple metals and visuospatial learning in early adolescence. Transl Psychiatry. (2020) 10(1):358. doi: 10.1038/s41398-020-01041-8
21. Gochfeld M. Framework for gender differences in human and animal toxicology. Environ Res. (2007) 104(1):4–21. doi: 10.1016/j.envres.2005.12.005
22. Signes-Pastor AJ, Doherty BT, Romano ME, Gleason KM, Gui J, Baker E, et al. Prenatal exposure to metal mixture and sex-specific birth outcomes in the New Hampshire Birth Cohort Study. Environ Epidemiol. (2019) 3(5):e068. doi: 10.1097/EE9.0000000000000068
23. Manjourides J, Zimmerman E, Watkins DJ, Carpenito T, Velez-Vega CM, Huerta-Montanez G, et al. Cohort profile: center for Research on Early Childhood Exposure and Development in Puerto Rico. BMJ Open. (2020) 10(7):e036389. doi: 10.1136/bmjopen-2019-036389
24. Kim SS, Meeker JD, Carroll R, Zhao S, Mourgas MJ, Richards MJ, et al. Urinary trace metals individually and in mixtures in association with preterm birth. Environ Int. (2018) 121(Pt 1):582–90. doi: 10.1016/j.envint.2018.09.052
25. Barlow SM, Burch M, Venkatesan L, Harold M, Zimmerman E. Frequency Modulation and Spatiotemporal Stability of the sCPG in Preterm Infants with RDS. Int J Pediatr. (2012) 2012:581538. doi: 10.1155/2012/581538
26. Estep M, Barlow SM, Vantipalli R, Finan D, Lee J. Non-Nutritive Suck Parameter in Preterm Infants with RDS. J Neonatal Nurs. (2008) 14(1):28–34. doi: 10.1016/j.jnn.2007.12.005
27. Poore M, Zimmerman E, Barlow SM, Wang J, Gu F. Patterned orocutaneous therapy improves sucking and oral feeding in preterm infants. Acta Paediatr. (2008) 97(7):920–7. doi: 10.1111/j.1651-2227.2008.00825.x
28. Martens A, Hines M, Zimmerman E. Changes in non-nutritive suck between 3 and 12 months. Early Hum Dev. (2020) 149:105141. doi: 10.1016/j.earlhumdev.2020.105141
29. Poore M, Barlow SM, Wang J, Estep M, Lee J. Respiratory treatment history predicts suck pattern stability in preterm infants. J Neonatal Nurs. (2008) 14(6):185–92. doi: 10.1016/j.jnn.2008.07.006
30. Martens A, Hines M, Zimmerman E. Non-nutritive suck in full-term infants and developmental outcomes at 12 months. Washington D.C: Poster presented virtually at the American Speech Language and Hearing Convention (2021).
31. Claus Henn B, Ettinger AS, Schwartz J, Tellez-Rojo MM, Lamadrid-Figueroa H, Hernandez-Avila M, et al. Early postnatal blood manganese levels and children's neurodevelopment. Epidemiology. (2010) 21(4):433–9. doi: 10.1097/EDE.0b013e3181df8e52
32. Balachandran RC, Mukhopadhyay S, McBride D, Veevers J, Harrison FE, Aschner M, et al. Brain manganese and the balance between essential roles and neurotoxicity. J Biol Chem. (2020) 295(19):6312–29. doi: 10.1074/jbc.REV119.009453
33. Bouabid S, Tinakoua A, Lakhdar-Ghazal N, Benazzouz A. Manganese neurotoxicity: behavioral disorders associated with dysfunctions in the basal ganglia and neurochemical transmission. J Neurochem. (2016) 136(4):677–91. doi: 10.1111/jnc.13442
34. Horning KJ, Caito SW, Tipps KG, Bowman AB, Aschner M. Manganese Is Essential for Neuronal Health. Annu Rev Nutr. (2015) 35:71–108. doi: 10.1146/annurev-nutr-071714-034419
35. Lederman SA, Jones RL, Caldwell KL, Rauh V, Sheets SE, Tang D, et al. Relation between cord blood mercury levels and early child development in a World Trade Center cohort. Environ Health Perspect. (2008) 116(8):1085–91. doi: 10.1289/ehp.10831
36. Jedrychowski W, Jankowski J, Flak E, Skarupa A, Mroz E, Sochacka-Tatara E, et al. Effects of prenatal exposure to mercury on cognitive and psychomotor function in one-year-old infants: epidemiologic cohort study in Poland. Ann Epidemiol. (2006) 16(6):439–47. doi: 10.1016/j.annepidem.2005.06.059
37. Kim B, Shah S, Park HS, Hong YC, Ha M, Kim Y, et al. Adverse effects of prenatal mercury exposure on neurodevelopment during the first 3 years of life modified by early growth velocity and prenatal maternal folate level. Environ Res. (2020) 191:109909. doi: 10.1016/j.envres.2020.109909
38. Kupsco A, Estrada-Gutierrez G, Cantoral A, Schnaas L, Pantic I, Amarasiriwardena C, et al. Modification of the effects of prenatal manganese exposure on child neurodevelopment by maternal anemia and iron deficiency. Pediatr Res. (2020) 88(2):325–33. doi: 10.1038/s41390-020-0754-4
39. Kippler M, Bottai M, Georgiou V, Koutra K, Chalkiadaki G, Kampouri M, et al. Impact of prenatal exposure to cadmium on cognitive development at preschool age and the importance of selenium and iodine. Eur J Epidemiol. (2016) 31(11):1123–34. doi: 10.1007/s10654-016-0151-9
40. Ma C, Iwai-Shimada M, Nakayama SF, Isobe T, Kobayashi Y, Tatsuta N, et al. Association of prenatal exposure to cadmium with neurodevelopment in children at 2 years of age: the Japan Environment and Children's Study. Environ Int. (2021) 156:106762. doi: 10.1016/j.envint.2021.106762
41. Mizuno D, Kawahara M. The molecular mechanisms of zinc neurotoxicity and the pathogenesis of vascular type senile dementia. Int J Mol Sci. (2013) 14(11):22067–81. doi: 10.3390/ijms141122067
42. Bock CW, Katz AK, Markham GD, Glusker JP. Manganese as a Replacement for Magnesium and Zinc: functional Comparison of the Divalent Ions. J Am Chem Soc. (1999) 121(32):7360–72. doi: 10.1021/ja9906960
43. De Craemer S, Croes K, van Larebeke N, De Henauw S, Schoeters G, Govarts E, et al. Metals, hormones and sexual maturation in Flemish adolescents in three cross-sectional studies (2002-2015). Environ Int. (2017) 102:190–9. doi: 10.1016/j.envint.2017.02.014
44. Wu T, Buck GM, Mendola P. Blood lead levels and sexual maturation in U.S. girls: the Third National Health and Nutrition Examination Survey, 1988-1994. Environ Health Perspect. (2003) 111(5):737–41. doi: 10.1289/ehp.6008
Keywords: prenatal exposure, child neurodevelopment, metals, pregnancy, Puerto Rico
Citation: Kim C, Zimmerman E, Huerta-Montañez G, Rosario-Pabón ZY, Vélez-Vega CM, Alshawabkeh AN, Cordero JF, Meeker JD and Watkins DJ (2022) Associations between biomarkers of prenatal metals exposure and non-nutritive suck among infants from the PROTECT birth cohort in Puerto Rico. Front. Epidemiol. 2:1057515. doi: 10.3389/fepid.2022.1057515
Received: 29 September 2022; Accepted: 15 November 2022;
Published: 1 December 2022.
Edited by:
Kai Chen, Yale University, United StatesReviewed by:
Marisol Castillo-Castrejon, University of Oklahoma Health Sciences Center, United StatesRajendra Prasad Parajuli, Tribhuvan University, Nepal
© 2022 Kim, Zimmerman, Huerta-Montañez, Rosario-Pabón, Vélez-Vega, Alshawabkeh, Cordero, Meeker and Watkins. This is an open-access article distributed under the terms of the Creative Commons Attribution License (CC BY). The use, distribution or reproduction in other forums is permitted, provided the original author(s) and the copyright owner(s) are credited and that the original publication in this journal is cited, in accordance with accepted academic practice. No use, distribution or reproduction is permitted which does not comply with these terms.
*Correspondence: Deborah J. Watkins debjwat@umich.edu
Specialty Section: This article was submitted to Occupational and Environmental Epidemiology, a section of the journal Frontiers in Epidemiology