- 1LIFE Di Center, Faculty of Psychology, Chulalongkorn University, Bangkok, Thailand
- 2Department of Psychological Medicine, The University of Auckland, Auckland, New Zealand
- 3Research Institute for Health Sciences, Chiang Mai University, Chiang Mai, Thailand
- 4Faculty of Public Health, Chiang Mai University, Chiang Mai, Thailand
- 5School of Public Health, Rutgers University, Piscataway, NJ, United States
- 6Gangarosa Department of Environmental Health, Rollins School of Public Health, Emory University, Atlanta, GA, United States
- 7Department of Population and Public Health Sciences, Keck School of Medicine, University of Southern California, Los Angeles, CA, United States
- 8Environmental and Occupational Health Science Institute, Rutgers University, Piscataway, NJ, United States
Introduction: Organophosphate (OP) insecticides are among the most abundantly used insecticides worldwide. Thailand ranked third among 15 Asian countries in its use of pesticides per unit hectare and fourth in annual pesticide use. More than 40% of Thai women of childbearing age work on farms where pesticides are applied. Thus, the potential for pregnant women and their fetuses to be exposed to pesticides is significant. This study investigated the relationship between early, mid, and late pregnancy maternal urine concentrations of OP metabolites and infant neural integrity at 5 weeks of age.
Method: We enrolled women employed on farms from two antenatal clinics in the Chiang Mai province of northern Thailand. We collected urine samples monthly during pregnancy, composited them by early, mid and late pregnancy and analyzed the composited samples for dialkylphosphate (DAP) metabolites of OP insecticides. At 5 weeks after birth, nurses certified in use of the NICU Network Neurobehavioral Scale (NNNS) completed the evaluation of 320 healthy infants. We employed generalized linear regression, logistic and Poisson models to determine the association between NNNS outcomes and DAP concentrations. All analyses were adjusted for confounders and included creatinine as an independent variable.
Results: We did not observe trimester specific associations between DAP concentrations and NNNS outcomes. Instead, we observed statistically significant inverse associations between NNNS arousal (β = −0.10; CI: −0.17, −0.002; p = 0.0091) and excitability [0.79 (0.68, 0.92; p = 0.0026)] among participants with higher average prenatal DAP concentrations across pregnancy. We identified 3 NNNS profiles by latent profile analysis. Higher prenatal maternal DAP concentrations were associated with higher odds of being classified in a profile indicative of greater self-regulation and attention, but arousal and excitability scores below the 50th percentile relative to US normative samples [OR = 1.47 (CI: 1.05, 2.06; p = 0.03)]. Similar findings are also observed among infants with prenatal exposure to substances of abuse (e.g., methamphetamine).
Discussion: Overall, the associations between prenatal DAP concentrations and NNNS summary scores were not significant. Further evaluations are warranted to determine the implications of low arousal and excitability for neurodevelopmental outcomes of attention and memory and whether these results are transitory or imply inadequate responsivity to stimulation among children as they develop.
Introduction
Organophosphate (OP) insecticides are among the most abundantly used insecticides worldwide that tend to be used in greater amounts and with fewer restrictions in low- and middle-income countries (LMICs) despite their well-documented acute neurotoxic effects (1). Globally, Thailand is one of the top countries in agricultural pesticide use (2). The country continues to increase its annual import of pesticides for agriculture with OP insecticides being listed among the most imported insecticide used to control pests for crops such as vegetables, rice, and flowers (3). Women in their childbearing years comprise ~40% of the workforce on Thai farms (4). Thus, for those women working on farms where pesticides are used, the potential for their fetus to be exposed to pesticides during the prenatal period is significant (5).
The primary mode of OP insecticide acute toxicity is by binding to acetylcholinesterase (AChE) thus preventing the breakdown of the neurotransmitter acetylcholine. Other non-cholinergic effects on the nervous system such as altered neuronal proliferation and impaired cognition have also been reported after low level exposures to OP insecticides. Previous human and animal studies have reported that neurotoxic insecticides cross the placental barrier and enter into the fetal blood stream (6, 7). Because the fetal detoxification process is immature, insecticide exposures during critical windows of fetal development produce lasting disruption of neurulation, neuron proliferation, neural migration, myelination, and synaptogenesis with specific sensitivity of the serotonin and dopamine systems as reported in animal models (8–10).
Although inconsistencies exist, epidemiologic studies from four US birth cohorts show that early indicators of growth and later cognitive development are adversely affected by prenatal insecticide exposure even without evidence of AChE inhibition during pregnancy (11–20). For example, lower birth weight and length, and shorter gestation were linked to maternal OP exposure (16, 19, 21) and, of note, infants of mothers whose paraoxonase status dictated slow OP insecticide metabolism exhibited smaller head circumference (20). In addition, studies document that increased concentrations of OP exposure in utero result in reduced fetal length and weight estimated from ultrasound at 20 weeks gestation. Neurodevelopmental deficits in neonates such as abnormal reflexes have also been noted in several studies (14, 22–24). A birth cohort study in China reported that high exposure of pregnant women to OP insecticides was the predominant risk factor for a lower summary score on the Neonatal Behavioral Neurologic Assessment (25). More recently, results from a cohort study of prenatal exposure to multiple pesticides in Chinese infants showed that auditory brainstem response (ABR) processing was slower in infants with greater prenatal pesticide exposure, indicating impaired neuromaturation (26). In addition to the effects on birth outcomes and neural integrity in the first few weeks of life, total diethylalkylphosphate (DEAP) and total dialkylphosphate (DAP) metabolite concentrations, measured at 28 weeks gestation, were significantly associated with reduced motor composite scores on the Bayley Scales of Infant Development III administered at 5 months (27).
Although several birth cohort studies investigating the associations of OP insecticide exposure on birth outcomes and neurodevelopment have been conducted, characterization of exposure in each trimester of pregnancy has been difficult to accomplish. For example, previous birth cohort studies relied upon a single measurement of OP exposure throughout the pregnancy (22, 28). In addition, with one exception, previous studies used the Brazelton Neonatal Behavioral Assessment Scale (BNBAS) to assess infant neural integrity (29). Although widely used, BNBAS examiners are trained to elicit optimal performance of the infant rather than a more objective assessment of the infant's ability to organize their own behavior. Additionally, norms are not available for the BNBAS partly due to variability among examiners in seeking optimal infant performance (30). Of note, one study using the NICU Network Neurobehavioral Scale (NNNS) to evaluate the effect of OP insecticides on neurological development reported no detrimental effects of gestational exposure to OP insecticides on neurobehavioral outcomes among 5-week old infants. Rather, the study found that higher creatinine-corrected urinary concentrations of diethylphosphate metabolite were associated with improved attention, reduced lethargy and hypotonia among infants while higher creatinine-corrected urinary concentrations of total DAP metabolites were associated with fewer signs of autonomic stress (31). However, this study obtained spot urine samples at two-points during 3 trimesters of pregnancy which could contribute to exposure misclassification (31, 32).
The aims of the present study were to investigate the associations of OP insecticide exposure during each trimester of pregnancy and summed across pregnancy on the neural integrity of Thai infants. Our study was intended to address gaps in the existing literature by capturing OP metabolites during early, mid and late pregnancy and employing an objective measure of infant neural integrity at 5 weeks of age after resolution of birth trauma. Determining specific windows of vulnerability during gestation could inform prevention measures to reduce immediate and life-long adverse outcomes.
Methods
Participants and recruitment
The SAWASDEE study is a prospective birth cohort designed to examine the impact of prenatal, occupational exposure to OP insecticides on infant neurodevelopment. Between July 30 and June 30, 1,290 pregnant women who presented at Chom Thong or Muang Chum Tambon (Fang) hospitals, in Chiang Mai province, Thailand for their first antenatal care were screened by trained nurses. A total of 394 pregnant women who met the inclusion criteria listed in Table 1 were recruited for the study. Informed consent was obtained from all study participants prior to enrollment. This study was reviewed and approved by the Institutional Review Board at Emory University (with Rutgers University reliance) and the Ethical Review Board at Chiang Mai University (with Chulalongkorn University reliance). We excluded 61 pregnant women for the following reasons: miscarriage (n = 21), blighted ovum (n = 7), no longer receiving care at participating clinics (n = 11), illness (e.g., diabetes, thyroid, hepatitis) (n = 6), loss to follow-up or drop-out (n = 6), pregnancy complications (e.g., ectopic, fetal abnormality) (n = 4), twin pregnancy (n = 3), GA >20 weeks by ultrasound (n = 2), and substance abuse (n = 1).
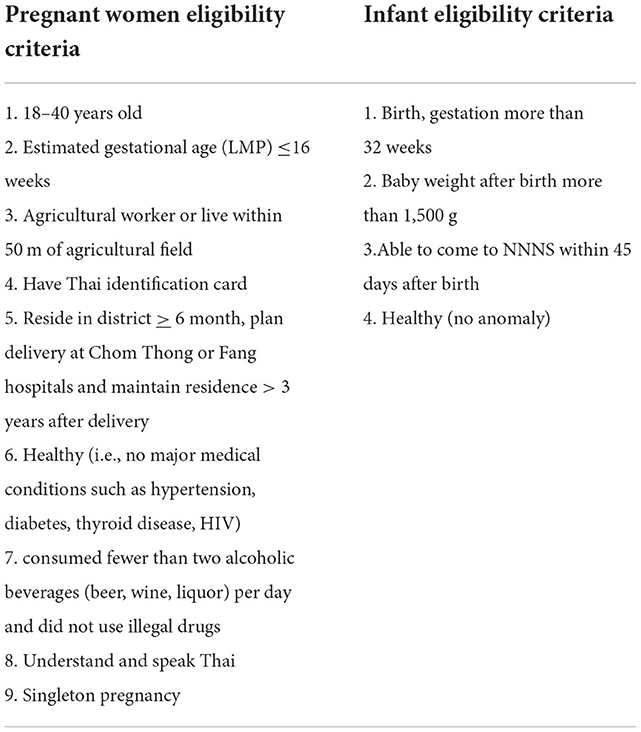
Table 1. Eligibility criteria for pregnant women and Infants, study of Asian women and their offspring's' development and environmental exposure (2017–2019).
A total of 333 pregnant women delivered live infants. Infants with the following conditions were excluded: GA < 32 weeks (n = 1), birth weight < 30 gm (n = 2), birth defect (i.e., prefrontal brain damage, meningomyelocele, congenital heart disease; n = 3), significant developmental delay (i.e., motor developmental delay at 6 months), hydrocephalus (n = 2), loss to follow-up (n = 1), and moved (n = 1). Three eligible participants did not complete the NNNS but remained in the study for subsequent neurodevelopmental tests. The final sample of 320, healthy 5-week old infants were administered the NNNS at the SAWASDEE clinic.
Interview, medical record abstraction, and questionnaires
Trained, registered nurses recruited pregnant women and administered an intake questionnaire to determine study eligibility in community and district hospitals. After informed consent, trained research assistants administered a structured questionnaire to collect the following demographic, medical, and occupational information: age, education, marital status, ethnicity, primary language (Thai/non-Thai), parity/gravidity, monthly income, work history, pesticide use, drug and alcohol use, smoking history (mother and father), acute and chronic medical diagnoses, medication use, vitamins, and stressors (e.g., abuse, disasters, death in family). This questionnaire was repeated at mid and late pregnancy to determine changes in medical or occupational information. Maternal weight gain as a surrogate for nutritional status was based on the change in weight from the intake visit during the first trimester to mother's weight at delivery. Because it is difficult to determine income among farm workers in LMIC settings, we developed an Observation of Assets Questionnaire (assets questionnaire) as an alternate measure of income (33, 34). Participants completed the assets questionnaire which was factor analyzed, yielding 3 primary factors: (1) Housing Quality: items rated to reflect quality of building materials and utilities (e.g., electricity, flooring); (2) Safety of home and neighborhood: participant perceptual ratings of home and neighborhood, and (3) Asset value: total value of appliances, farm equipment, vehicles, and land/home ownership. The Test of Non-verbal Intelligence (TONI-IV) was completed by participants as a language free assessment of intellectual ability (35). The study nurse abstracted the following data from medical records at delivery: sex, birth weight and length, head circumference, delivery type, Apgar at 1 and 5 min, and gestational age. All variables from these documents were evaluated as confounders in final statistical models.
OP insecticide exposure assessment
Urine samples were obtained up to 6 times during pregnancy at each antenatal care visit. Samples were collected in 100-ml polypropylene urine collection containers and were aliquoted and stored in a −20°C freezer until analysis. To characterize exposure across pregnancy while keeping the analytic burden low, urine samples were composited using equal volumes to create early- (0–14 weeks gestation), mid- (>14 weeks through 27 weeks gestation) and late- (>27 weeks gestation) pregnancy samples that roughly corresponded to trimester. Details about sample collection, composite scheme, and DAP metabolite descriptive statistics including intraclass correlation coefficients and spearman correlations of trimester samples can be found in Baumert et al. (5).
Composited urine samples were analyzed for 6 DAP metabolites using a previously validated method which was also cross-validated by gas chromatography-mass spectrometry (36). All samples were randomized using a Fisher-Yates shuffling algorithm prior to analysis to reduce potential batch effects (37, 38). Briefly, 5 mL of urine was spiked with dibutylphosphate (DBP) as a surrogate internal standard, then acidified with hydrochloric acid. The acidified urine was extracted with ethyl acetate: acetone then the DAP metabolites were derivatized to their pentafluorobenzyl phosphate esters. The target DAP derivatives were isolated from the reaction mixture using a hexane extraction. The concentrated extract was analyzed by gas chromatography-flame photoionization detection. Data were quantified using a continuing calibration curve normalizing on the DBP internal standard area. Quality control (QC) samples were derived from 2 separated urine pools: unspiked pooled urine (low concentration QC pool) and spiked pooled urine where DAP metabolites were spiked into the pool at various concentrations (high concentration QC pool). Two blank samples, four QC samples and calibrants were analyzed concurrently with unknown samples in each analytical run. The limits of detection (LOD) were 5 ng/mL (dimethylphosphate; DMP), 1 ng/mL (dimethylthiophosphate; DMTP), 0.5 ng/mL (dimethyldithiophosphate; DMDTP), 1 ng/mL (DEP), 0.125 ng/mL (diethylthiophosphate; DETP), and 0.25 ng/mL (diethyldithiophosphate; DEDTP) and the relative recoveries ranged from 94 to 119%. Relative standard deviations of the QC pools ranged from 4.5 to 12.6% (36). The laboratory also participated in the proficiency testing program administered by the German External Quality Assessment Scheme.
Because of physiologic change during pregnancy, the validity of using creatinine correction for maternal samples has been debated (17, 19, 21, 39). Nevertheless, we chose this approach because it has been used more often in epidemiological studies of pesticide related neurodevelopment. Thus, we can compare our results with other studies directly. In addition and as stated in O'Brien et al. (40), there is no universally accepted measure of urinary dilution. Creatinine was measured by diluting urine samples 1,000-fold with water after spiking with its isotopically labeled analog. Diluted samples were analyzed by liquid chromatography tandem mass spectrometry coupled with electrospray ionization (41). During the analysis of creatinine, a certified reference material (SRM) obtained from the National Institute of Standards and Technology (NIST) was included (NIST SRM 3667).
NICU network neurobehavioral scale
Five weeks after birth, maternal and infant participants came to SAWASDEE clinics where the research assistant measured infant participants' weight, length, and head circumference. Maternal participants completed the Depression Anxiety Stress Scale (DASS) (42–44). Early infant neurologic function, behavior, and signs of stress were measured using the NNNS, a tool developed as an assessment for the at-risk infant such as those born prematurely or prenatally exposed to neurotoxic substances. The NNNS measure is appropriate for infants gestational age 30–46 weeks (corrected for conceptional age (45). The NNNS has strong psychometric qualities with good internal and concurrent validity (46). Study nurses, blind to infant exposure and certified by a Brown University certified trainer, administered the NNNS while the mother observed the exam. During the assessment period, nurses met monthly for reliability checks.
We administered the NNNS in a quiet, temperature-controlled room. The test began with a baseline observation of respiration, color, and tone. If the infant was asleep, a sequence of habituation items was presented to measure the infant's ability to process visual, auditory, and tactile stimuli, and to protect sleep. The habituation package is often omitted due to the sleep requirement. Examination of primitive reflexes, as well as passive and active tone ensues, followed by social interaction components and an assessment of attention. Additional neurological items were completed, followed by a post-exam observation of respiration, color, and tone to end the assessment. Summarization of NNNS raw data results in scores on 13 dimensions: habituation, attention, arousal, self-regulation, special handling needed from the examiner to assist the infant through the exam, quality of movement, excitability, lethargy, non-optimal reflexes, asymmetrical reflexes, hypertonicity, hypotonicity, and stress/abstinence (45).
Statistical analysis
Frequencies and percentages or means, standard deviations and range are used to describe the distributions of categorical or continuous covariates, respectively, overall and stratified by location. DAP concentrations were converted into summed DAP concentrations, consisting of ∑DEAP, ∑DMAP, and ∑DAP applying the following formulas: ∑DEAP = (DEP/154) + (DETP/170) + (DEDTP/186); ∑DMAP = (DMP/126) + (DMTP/142) + (DMDTP/158); ∑DAP = ∑DMAP + ∑DEAP. Summed DAP concentrations were created from the composited urine samples to represent early, middle, and late pregnancy period for each subject. The average summed DAP concentration was calculated by combining the summed DAP concentrations obtained from each composited urine sample and dividing by the number of composited urine samples (n = 3). The mean ∑DAP variable, reported in μmol/L unit, was included in the model to represent average OP exposure across pregnancy for each individual subject (5). These summed DAP concentrations were treated as continuous variables in statistical models.
The distributions of NNNS outcomes are summarized and expressed as means, standard deviations, and percentiles. Because we administered the habituation package to < 50% of our sample (N = 125) due to the sleep requirement, scores for habituation were omitted from further analysis. We used regression models to determine the association between NNNS outcomes and summed DAP concentrations. In these models, the heavily right-skewed summed DAP concentrations were log-transformed to reduce outsized influence of the higher values on estimated regression parameters. NNNS measures that were continuous (habituation, attention, handling, self-regulation, arousal, lethargy, quality of movement, and stress abstinence) were evaluated using standard linear regression, while counts (excitability, non-optimal reflexes, and asymmetric reflexes) were evaluated with Poisson regression and binary measures (hyper- and hypotonicity) with logistic regression. As such, standardized regression coefficients (equivalent to partial correlations) estimated the effect of summed DAP concentrations on continuous NNNS measures, risk ratios the effects on count measures, and odds ratios the effects on binary measures. Effects were estimated adjusting for creatinine concentrations and then adding other potential confounders. Potential confounders were identified from the demographic information available (see Table 1) as those that were associated with at least one of the NNNS measures and summed DAP concentrations at the 0.10 significance level.
In cases of significant associations, the means, standard deviations, and percentiles of NNNS measures were calculated for low (0–25th percentile), medium (25–75th percentile), and high (>75th percentile) exposure.
Latent Profile Analysis (LPA) using Mplus 7 statistical package identified groups of infants with similar response patterns. This parametric analysis accounted for the distributions of each of the NNNS measures, specifically, normal for continuous, Poisson for count, and logistic for binary (47). The validity of the resulting groupings was confirmed with non-parametric cluster analysis performed on the standardized scores iteratively using Euclidian distances to identify clusters. Logistic regression evaluated the association of pesticide concentrations on profile membership, adjusting for potential confounders.
All analyses except LPA were performed using SAS software, Version 9.4 of the SAS System for Windows (© 2016, SAS Institute Inc).
Results
Table 2 is a summary of the study participant demographics and values for summed DAP concentrations for the entire study sample and separately for each location (Chom Thong; Fang) from which participants were recruited. As can be seen in Table 2, participants from these locations differed on several demographic and exposure variables. Most of our mothers spoke Thai and were born in Thailand. However, 98% of participants from Chom Thong were born in Thailand while 60% of mothers from Fang district were born in Myanmar. Most of our sample was married and did not report a history of abuse, intimate partner violence, alcohol or recreational drug use during pregnancy. Mother and father education and family income were lower among Fang compared to Chom Thong participants. Most infants had vaginal birth with similar gestational age, weight, length, head circumference and Apgar scores across sites. The highest summed DAP concentrations were observed for participants from Fang.
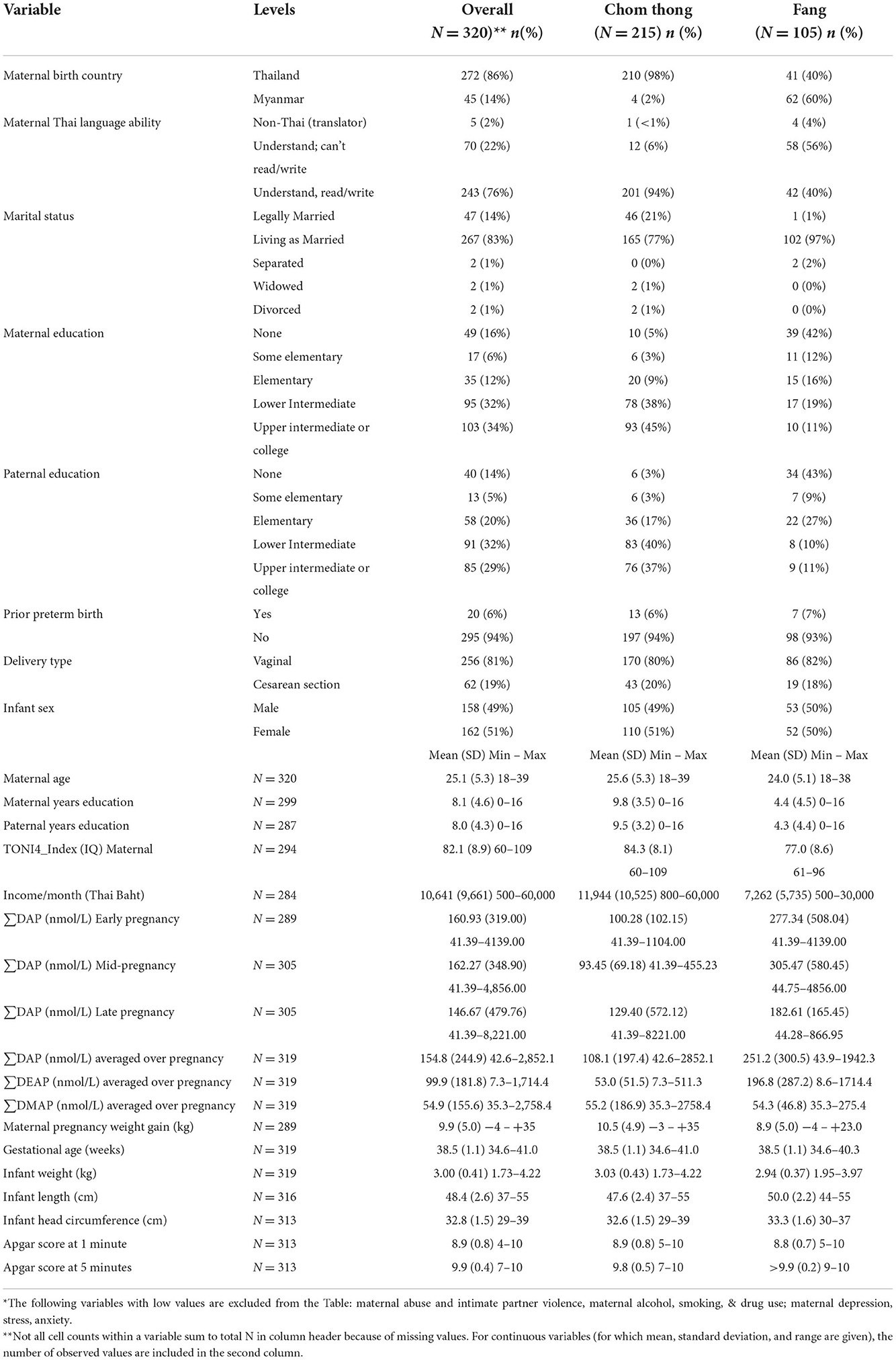
Table 2. Overall and location specific family and infant demographics and ∑DAP values, study of Asian women and their offsprings' development and environmental exposure (2017–2019)*.
OP Metabolites as predictors of NNNS Summary scores.
Table 3 displays descriptive statistics for the NNNS summary scores for all participants followed by Table 4 showing the associations between OP metabolites and NNNS summary scores. The following variables associated with at least one of the 12 NNNS scores and summed DAP concentrations at p ≤ 0.10 were included as confounders in models to predict outcomes: father's education, infant sex, amenities/appliances in home, having at least one previous preterm birth, gestational age, and maternal TONI-IV. Creatinine was included in models as an independent variable. Initially, we evaluated the effects of ∑DAP concentrations on NNNS summary scales for each trimester. We observed lower arousal among infants with higher ∑DAP concentrations during each trimester and less excitability for the second and third trimester exposures for higher ∑DAP concentrations (data not shown). Because we did not find a consistent effect of trimester specific ∑DAP concentrations, the remainder of our analyses used average ∑DAP concentrations across pregnancy.
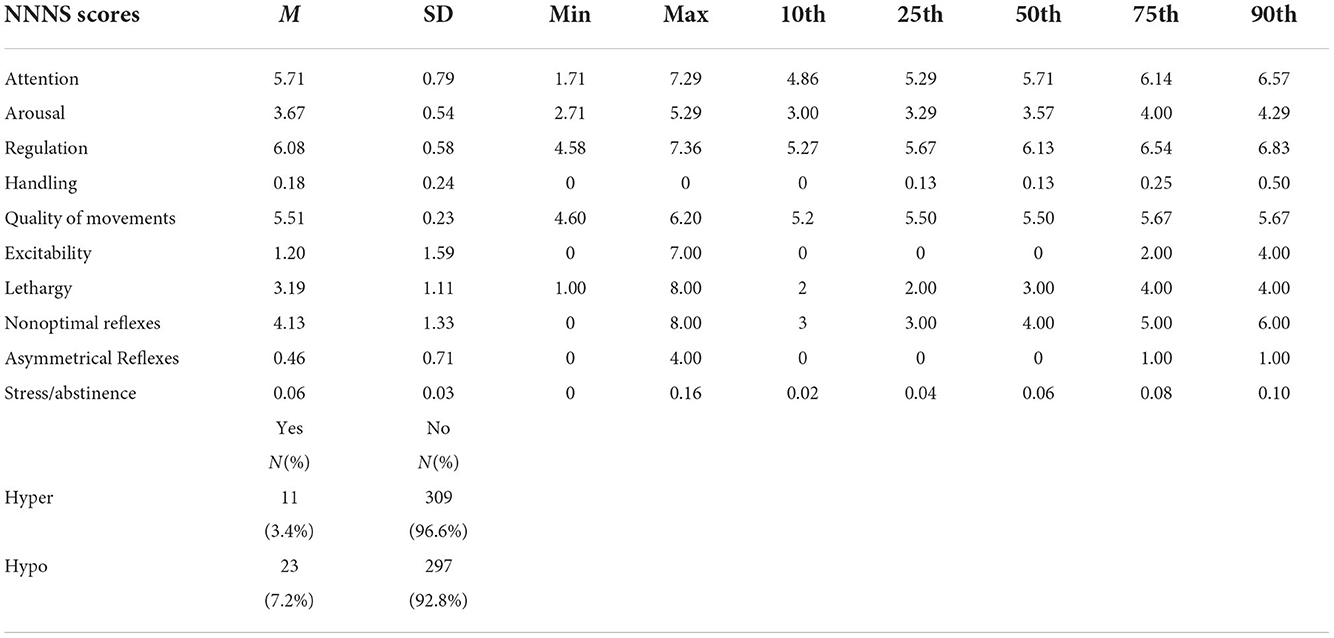
Table 3. Descriptive statistics for NNNS summary scores, study of Asian women and their offsprings' development and environmental exposure (2017–2019).
We observed a statistically significant inverse association between NNNS arousal and log values of both ∑DAP [β = −0.10 (CI: −0.17, −0.02) p = 0.0091] and ∑DEAP but not ∑DMAP concentrations. Participants had a significantly lower risk of excitability with higher prenatal ∑DAP concentrations [0.79** (0.68, 0.92) p = 0.0026] and higher ∑DMAP and ∑DEAP concentrations (see Table 4). To explore sex differences for the associations of OP insecticides on NNNS outcomes, we performed the same regression analyses stratified by infant sex and controlling for covariates and creatinine correction. We observed that lower excitability and arousal with higher ∑DAP concentrations were not different for males and females (data not shown).
To explore further the associations of ∑DAP concentrations on infant arousal and excitability, we grouped participants based on high, medium and low prenatal ∑DAP concentrations (Table 5). Relative to a US normative sample of infants examined at 4 weeks old, our participants' average NNNS summary scores were within the normative range, defined as between the 10 and 90th percentile. However, mean arousal and excitability scores were below the normative 10th percentile for participants who had high ∑DAP concentrations (48). This low arousal and excitability could be indicative of non-optimal development at 1 month. Neither arousal nor excitability changed significantly from birth to 1 month in a US normative sample (48), suggesting that lower arousal and excitability may remain stable. Moreover, our scores for these scales were also below the 10th percentile observed by Fink (49) in a larger US normative sample evaluated at birth.
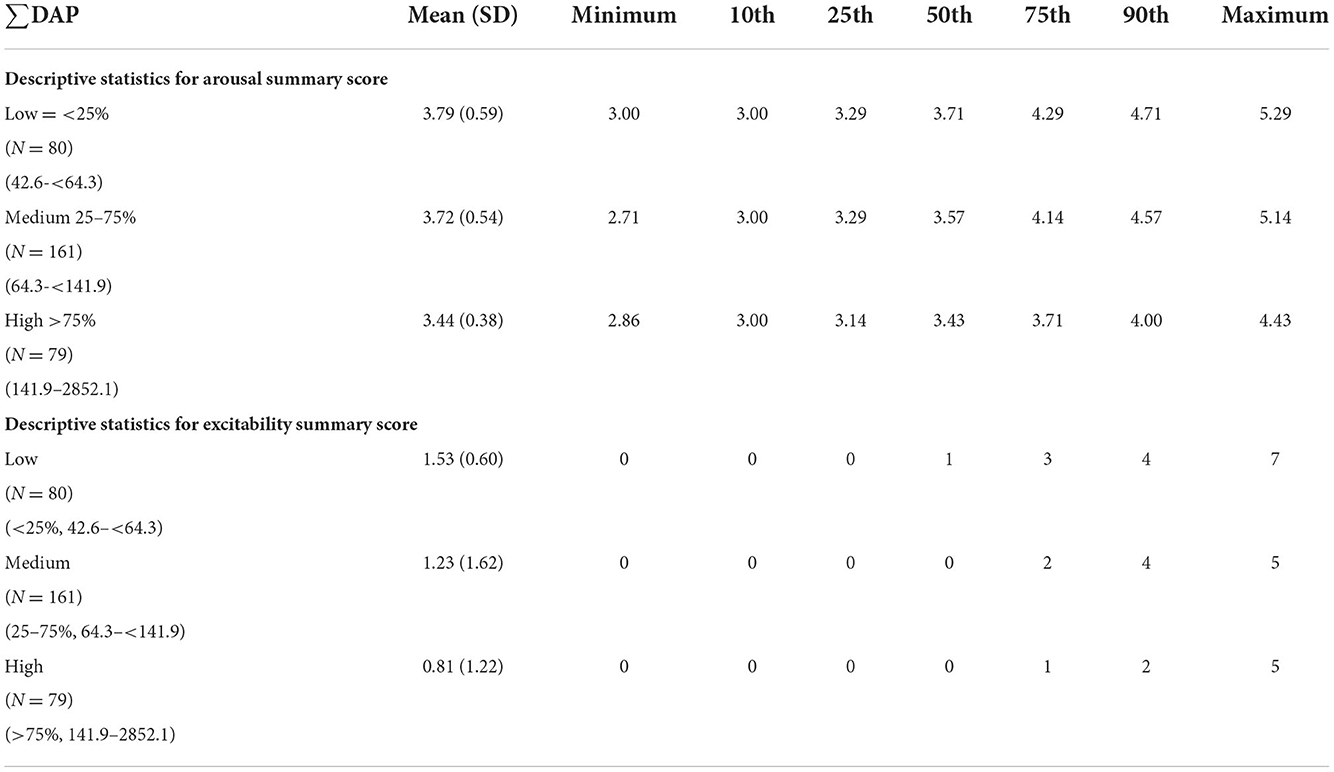
Table 5. NNNS arousal and excitability summary scores in high, medium, and low ∑DAP concentration categories.
LPA of NNNS summary scales identified 3 profiles: Profile 1 (N = 183): low need for handling, self regulated, better attention, and low arousal/excitability; Profile 2 (N = 75): need for handling, and moderate levels of arousal, self regulation and excitability; Profile 3 (N = 62): higher need for handling, low attention and self-regulation, high arousal and excitability and greater stress/abstinence (see Figure 1). We compared summary scores across profiles and found significant differences for each summary scale, validating the independence of the profiles (Supplementary Table S1).
Overall higher ∑DAP concentrations conferred lower odds of being classified in Profile 3, characterized as infants with lower attention and self-regulation and higher arousal and excitability [Profile 1: OR = 0.55 (CI: 0.34–0.86) p = 0.012]. Moreover, overall higher ∑DAP concentrations increased the odds ratio for Profile 1, identified as more self regulated, better attention, but lower excitability and arousal [Profile 1: OR = 1.47 (CI: 1.05–2.06) p = 0.03]. ∑DEAP metabolites reflecting chlorpyrifos exposure were responsible for the associations with NNNS profiles while ∑DMAP metabolites were not associated with specific profiles. Higher ∑DAP metabolites in the first and second trimester predicted lower odds of being in Profile 3, reflecting higher excitability and arousal, and greater odds of being categorized in Profile 1, reflecting higher self-regulation and lower excitability (see Supplementary Tables S2a–c). ∑DAP concentrations measured in the third trimester did not impact the odds ratio for any profile.
Discussion
Although one aim of our study was to determine trimester specific susceptibility, we did not find consistent associations with NNNS scales and ∑DAPs for each trimester. We observed, however, an inverse association between prenatal urinary DAP concentrations averaged across pregnancy and NNNS summary scales for arousal and excitability among 5 week old Thai infants. These infants exhibited lower levels of fussing, crying and associated motor activity throughout the examination (i.e., arousal) and lower levels of motor, state, and physiological reactivity and irritability (i.e., excitability) (50). DEAP metabolites reflecting exposure to pesticides such as chlorpyrifos but not dimethylalkylphosphate metabolites (e.g., malathion) were the OP insecticides predictive of low arousal while both DEAP and DMP metabolites were associated with low excitability. For infants whose maternal metabolite concentrations were >the 75th percentile, over 50 % of those infants scored zero on excitability and 50% scored below the normative value for arousal (48, 49). Arousal and excitability scores that are extremely high or low may confer risk for achieving neurodevelopmental milestones. In contrast, profile analysis did not reveal a significant association between higher prenatal ∑DAP concentrations and Profile 3, a higher risk profile reflecting lower attention, self-regulation and higher arousal and excitability. Instead, infants with higher prenatal OP insecticide exposure, particularly during the first and second trimester were more likely to be within Profile 1, reflecting better attention and self-regulation but lower arousal and excitability. These disparate results imply that higher OP insecticide exposure for some infants may be protective based on better attention and self-regulation. Future analyses of neurodevelopmental measures of visual attention, processing speed, and memory administered at 4 and 7 months will test the neurodevelopmental implications of low excitability and arousal observed at 5 weeks.
OP insecticides inhibit AChE, the enzyme that breaks down the neurotransmitter, acetylcholine (Ach), and is found in both the peripheral and central nervous systems (51). The acute effects of OP insecticide poisoning are well known, but whether neurodevelopmental effects are observed among infants and children in the absence of AChE inhibition evidence remains controversial. Several previous US birth cohort studies have shown mixed results of prenatal OP insecticide exposure on early infant behaviors. In US birth cohorts of 2–3 day old infants evaluated with the NBAS, higher concentrations of DAPs during pregnancy were associated with more abnormal reflexes (14, 22). Yolton et al. (31), however, observed that 5 week old US infants with higher prenatal exposure to OP insecticides producing DEAP metabolites had improved attention, lower lethargy and less hypotonia while ∑DAP concentrations were associated with fewer signs of autonomic distress. These disparate findings may be due to assessments performed a few days after birth relative to 5 weeks of age when the trauma of birth is resolved. However, it is also noteworthy that ∑DAP concentrations vary significantly among birth cohorts which is also likely to contribute to variability in outcomes. For example, the concentrations observed in our occupational cohort were higher than previous US birth cohorts from California (CHAMACOS: 111.7 nmol/g creatinine), Cincinnati (HOME:76.17 nmol/g creatinine), and New York (Columbia: 39.16 nmol/g creatinine; Mount Sinai:73.8 nmol/g creatinine) (7, 19, 20, 52).
Overall, our NNNS profile analysis reveals that higher ∑DAPs are associated with a profile reflecting better self regulation and attention, and less need for handling but lower arousal and excitability. This outcome may be consistent with Yolton et al. (31) who reported no detrimental effects of OP exposure during gestation and also observed improved attention and reduced lethargy. However, our observation of a lower level of arousal and excitability while potentially reflecting stability in behavior, very low arousal and excitability can also suggest that infants may not respond adequately to stimulation. Our findings show similarity with other studies of infants exposed prenatally to drugs of abuse and implicate effects on the dopaminergic and serotonergic systems. For example, infants exposed prenatally to methamphetamine (MA) exhibited lower arousal and excitability along with poorer quality of movement, and more total stress/abstinence (53). Prenatal cocaine exposure also resulted in lower arousal and inability to self-regulate among 1 month old infants evaluated with the NNNS (54). A study of African American infants with prenatal exposures to the stimulant nicotine (higher cotinine) exhibited decreased arousal and excitability along with increased self-regulation and hypotonicity in contrast to white infants who exhibited the opposite effect (55). African American infants were less responsive during the examination suggesting reduced neurobehavioral activation (55).
Rodent studies of MA exposure suggest toxic effects on dopaminergic and serotonergic neurons, both systems also shown to be sensitive to OP insecticide exposure in animal models (8–10). However, findings for drugs of abuse are not universal. For example, prenatal, self-report exposure to cocaine, alcohol, tobacco, and marijuana were predictive of increased arousal and excitability, hypertonia, poor quality of movement, self-regulation and attention (56). The latter study, however, was based largely on self-report rather than biomarkers of exposures.
Scores for excitability and arousal among OP insecticide exposed infants in the present study were lower than infants with prenatal exposure to drugs of abuse and for premature infants reported to have low excitability scores (57). However, comparisons to US normative data for the NNNS may not be applicable for Thai infants. Nevertheless, we have a relatively large sample size and have taken into account a full suite of potential confounders to evaluate the effects of prenatal OP insecticide exposures. Future validation of our NNNS results for neurodevelopmental outcomes will await analysis of neurodevelopmental measures, including measures of attention, memory, executive function and overall cognitive and motor development up to 3 years of age.
Data availability statement
The raw data supporting the conclusions of this article will be made available by the authors, without undue reservation.
Ethics statement
This study was reviewed and approved by the Institutional Review Board at Emory University (with Rutgers University reliance) and the Ethical Review Board at Chiang Mai University (with Chulalongkorn University reliance). The patients/participants provided their written informed consent to participate in this study.
SAWASDEE birth cohort investigative team
Dana Boyd Barr, Nancy Fiedler, Tippawan Prapamontol, Panrapee Suttiwan, Warangkana Naksen, Parinya Panuwet, P. Barry Ryan, Kyle Steenland, Melissa M. Smarr, Brittney O. Baumert, Priya D'Souza, Grace E. Lee, Volha Yakimavets, Tamaria Hawkins, Margaret Sullivan, Pamela Ohman-Strickland, Mark G. Robson, Sompoch Iamsupasit, Wattasit Siriwong, Kathryn J. Barr, Rachel Greenwald, Carol Cheatham, Ampica Mangklabruks, Tanyaporn Kerdnoi, Namtip Srirak, Surat Hongsibsong, Supattra Sittiwang, Pimjuta Nimmapirat, Wathoosiri Promduang, Natabhol Jayakittivaraloes, Nattawadee Promkam, Sureewan Pingwong, Kewalin Kunsupa, Anchana Kantasri, Kritsanachai Kaewthit, Anchalee Wongkampaun, Nathaporn Thongjan, Kanyapak Kohsuwan, Ranuka Dawandee, Maytinee Chaimidchid, Sasiwimon Soonsawat, Kingpaka Sritongkom, Sakawrat Namakunna, and Soythong Pinasu.
Author contributions
SS is responsible for the conduct of the study to include design, execution, and analysis of results. All authors contributed to the article and approved the submitted version.
Funding
This study was funded by NIH/NIEHS and Fogarty Grants R01ES026082, R21ES01546501, and R21ES018722 and NIH/NIEHS Grants P30ES019776, T32ES012870 (BB), R01ES029212, and P30ES005022.
Acknowledgments
We appreciate the contributions of Chiang Mai and Chulalongkorn Universities and other team members in support of our research and the Thai families who participated in our study. The study required significant time on the part of our participants to bring their children to each research session over a 3-year period.
Conflict of interest
The authors declare that the research was conducted in the absence of any commercial or financial relationships that could be construed as a potential conflict of interest.
Publisher's note
All claims expressed in this article are solely those of the authors and do not necessarily represent those of their affiliated organizations, or those of the publisher, the editors and the reviewers. Any product that may be evaluated in this article, or claim that may be made by its manufacturer, is not guaranteed or endorsed by the publisher.
Supplementary material
The Supplementary Material for this article can be found online at: https://www.frontiersin.org/articles/10.3389/fepid.2022.1039922/full#supplementary-material
References
1. Grube A, Donaldson D, Kiely T, Wu L. Pesticides Industry Sales Usage: 2006 2007 Market Estimates. (2011). Available from: https://www.epa.gov/pesticides/pesticides-industry-sales-and-usage-2006-and-2007-market-estimates (accessed January 1, 2013).
2. FAO/WHO. Pesticide Residues in Food 2018—Report 2018—Joint FAO/WHO Meeting in Pesticide Residues (2019).
3. Panuwet P, Siriwong W, Prapamontol T, Ryan PB, Fiedler N, Robson MG, et al. Agricultural pesticide management in thailand: situation and population health risk. Environ Sci Policy. (2012) 17:72–81. doi: 10.1016/j.envsci.2011.12.005
4. The National Statistical Office. 2013 Agricultural Census Northern Region. (2014). Available online at: http://www.nso.go.th/sites/2014en/Pages/Census/Agricultural%20Census/2013/northern%20region/Full%20Report.pdf
5. Baumert BO, Fiedler N, Prapamontol T, Naksen W, Panuwet P, Hongsibsong S, et al. Urinary concentrations of dialkylphosphate metabolites of organophosphate pesticides in the stud of Asian women and their offpring's development and environmental exposures (SAWSDEE). Environ Int. (2022) 158. doi: 10.1016/j.envint.2021.106884
6. Whyatt R, Barr DB. Measurement of organophosphate metabolites in postpartum meconium as a potential biomarker of prenatal exposure: a validation study. Environ Health Perspect. (2001) 109:417–20. doi: 10.1289/ehp.01109417
7. Whyatt RM, Barr DB, Camann DE, Kinney PL, Barr JR, Andrews HF, et al. Contemporary-use pesticides in personal air samples during pregnancy and blood samples at delivery among urban minority mothers and newborns. Environ Health Perspect. (2003) 111:749–56. doi: 10.1289/ehp.5768
8. Aldridge JE, Levin ED, Seidler FJ, Slotkin TA. Developmental exposure of rats to chlorpyrifos leads to behavioral alterations in adulthood, involving serotonergic mechanisms and resembling animal models of depression. Environ Health Perspect. (2005) 113:527–531. doi: 10.1289/ehp.7867
9. Chanda SM, Pope CN. Neurochemical and neurobehavioral effects of repeated gestational exposure to chlorpyrifos in maternal and developing rats. Pharmacol Biochem Behav. (1996) 53:771–6. doi: 10.1016/0091-3057(95)02105-1
10. Karen DJ, Li W, Harp PR, Gillette JS, Bloomquist JR. Striatal dopaminergic pathways as a target for the insecticides permethrin and chlorpyrifos. Neurotoxicology. (2001) 22:811–7. doi: 10.1016/S0161-813X(01)00063-8
11. Bouchard MF, Chevrier J, Harley KG, Kogut K, Vedar M, Calderon N, et al. Prenatal exposure to organophosphate pesticides and IQ in 7-year-old children. Environ Health Perspect. (2011) 119:1189–95. doi: 10.1289/ehp.1003185
12. Eskenazi B, Marks AR, Bradman A, Harley K, Barr DB, Johnson C, et al. Organophosphate pesticide exposure and neurodevelopment in young Mexican-American children. Environ Health Perspect. (2007) 115:792–8. doi: 10.1289/ehp.9828
13. Marks AR, Harley K, Bradman A, Kogut K, Barr DB, Johnson C, et al. Organophosphate pesticide exposure and attention in young Mexican-American children: the CHAMACOS study. Environ Health Perspect. (2010) 118:1768–74. doi: 10.1289/ehp.1002056
14. Young JG, Eskenazi B, Gladstone EA, Bradman A, Pedersen L, Johnson C, et al. Association between in utero organophosphate pesticide exposure and abnormal reflexes in neonates. Neurotoxicology. (2005) 26:199–209. doi: 10.1016/j.neuro.2004.10.004
15. Rauh V, Arunajadai S, Horton M, Perera F, Hoepner L, Barr DB, et al. Seven-year neurodevelopmental scores and prenatal exposure to chlorpyrifos, a common agricultural pesticide. Environ Health Perspect. (2011) 119:1196–201. doi: 10.1289/ehp.1003160
16. Whyatt RM, Rauh V, Barr DB, Camann DE, Andrews HF, Garfinkel R, et al. Prenatal insecticide exposures and birth weight and length among an urban minority cohort. Environ Health Perspect. (2004) 112:1125–32. doi: 10.1289/ehp.6641
17. Engel SM, Wetmur J, Chen J, Zhu CB, Barr DB, Canfield RL, et al. Prenatal exposure to organophosphates, paraoxonase 1, and cognitive development in childhood. Environ Health Perspect. (2011) 119:1182–8. doi: 10.1289/ehp.1003183
18. Rauh VA, Perera FP, Horton MK, Whyatt RM, Bansal R, Hao X, et al. Brain anomalies in children exposed prenatally to a common organophosphate pesticide. Proc Natl Acad Sci U S A. (2012) 109:7871–6. doi: 10.1073/pnas.1203396109
19. Rauch SA, Braun JM, Barr DB, Calafat AM, Khoury J, Montesano AM, et al. Associations of prenatal exposure to organophosphate pesticide metabolites with gestational age and birth weight. Environ Health Perspect. (2012) 120:1055–60. doi: 10.1289/ehp.1104615
20. Berkowitz GS, Wetmur JG, Birman-Deych E, Obel J, Lapinski RH, Godbold JH, et al. In utero pesticide exposure, maternal paraoxonase activity, and head circumference. Environ Health Perspect. (2004) 112:388–391. doi: 10.1289/ehp.6414
21. Eskenazi B, Harley K, Bradman A, Weltzien E, Jewell NP, Barr DB, et al. Association of in utero organophosphate pesticide exposure and fetal growth and length of gestation in an agricultural population. Environ Health Perspect. (2004) 112:1116–24. doi: 10.1289/ehp.6789
22. Engel SM, Berkowitz GS, Barr DB, Teitelbaum SL, Siskind J, Meisel SJ, et al. Prenatal organophosphate metabolite and organochlorine levels and performance on the Brazelton Neonatal Behavioral Assessment Scale in a multiethnic pregnancy cohort. Am J Epidemiol. (2007) 165:1397–404. doi: 10.1093/aje/kwm029
23. Muñoz-Quezada MT, Lucero BA, Barr DB, Steenland K, Levy K, Ryan PB, et al. Neurodevelopmental effects in children associated with exposure to organophosphate pesticides: a systematic review. Neurotoxicology. (2013) 39:158–68. doi: 10.1016/j.neuro.2013.09.003
24. Wroblewska L, Kitada T, Endo K, Siciliano V, Stillo B, Saito H, et al. Mammalian synthetic circuits with RNA binding, proteins for RNA-only delivery. HHS Public Access Nat Biotechnol. (2016) 33:839–841. doi: 10.1038/nbt.3301
25. Zhang Y, Han S, Liang D, Shi X, Wang F, Liu W, et al. Prenatal exposure to organophosphate pesticides and neurobehavioral development of neonates: a birth cohort study in Shenyang, China. PLoS ONE. (2014) 9:e88491. doi: 10.1371/journal.pone.0088491
26. Sturza J, Silver MK, Xu L, Li M, Mai X, Xia Y, et al. Prenatal exposure to multiple pesticides is associated with auditory brainstem response at 9 months in a cohort study of Chinese infants. Environ Int. (2016) 92:478–85. doi: 10.1016/j.envint.2016.04.035
27. Kongtip P, Techasaensiri B, Nankongnab N, Adams J, Phamonphon A, Surach A, et al. The impact of prenatal organophosphate pesticide exposures on thai infant neurodevelopment. Int J Environ Res Public Health. (2017) 14:570. doi: 10.3390/ijerph14060570
28. Rauh VA, Garfinkel R, Perera FP, Andrews HF, Hoepner L, Barr DB, et al. Impact of prenatal chlorpyrifos exposure on neurodevelopment in the first 3 years of life among inner-city children. Pediatrics. (2006) 118:e1845–59. doi: 10.1542/peds.2006-0338
30. Tronick EA. Grandchild of the NBAS: the NICU network neurobehavioral scale (NNNS) a review of the research using the NNNS. J Child Adolesc Psychiatry Nurs. (2013) 26:193–203. doi: 10.1111/jcap.12042
31. Yolton K, Xu Y, Sucharew H, Succop P, Altaye M, Popelar A, et al. Impact of low-level gestational exposure to organophosphate pesticides on neurobehavior in early infancy: a prospective study. Environ Health. (2013) 12:79. doi: 10.1186/1476-069X-12-79
32. Barkoski J, Bennett D, Tancredi D, Barr DB, Elms W, Hertz-Picciotto I. Variability of urinary pesticide metabolite concentrations during pregnancy in the MARBLES study. Environ Res. (2019) 165:400–9. doi: 10.1016/j.envres.2018.05.002
33. Clarke R. Measuring Wealth Across Seven Thai Communities. WeD Working Paper 17, University of Bath (2006).
34. Howe L. Measuring socio-economic position for epidemiologic studies in low-and middle-income countries: a methods of measurement in epidemiology paper. Int J Epidemiol. (2012) 41:871–86. doi: 10.1093/ije/dys037
35. Ritter N, Kilinc E, Navruz B, Bae Y. Test of Nonverbal Intelligence-4 (TONI-4). J Psychoeduc Assess. (2011) 29:484–8. doi: 10.1177/0734282911400400
36. Prapamontol T, Sutan K, Laoyang S, Hongsibsong S, Lee G, Yano Y, et al. Cross validation of gas chromatography-flame photometric detection and gas chromatography-mass spectrometry methods for measuring dialkylphosphate metabolites of organophosphate pesticides in human urine. Int J Hyg Environ Health. (2014) 217:554–66. doi: 10.1016/j.ijheh.2013.10.005
37. Knuth DE. The Art of Computer Programming (Vol. 3). London, United Kingdom: Pearson Education (1997).
38. Toutenburg H, et al. Statistical tables for biological, agricultural and medical research. Biometrische Zeitschrift. (1971) 13:285–285. doi: 10.1002/bimj.19710130413
39. Bradman A, Kogut K, Eisen EA, Jewell NP, Quirós-Alcalá L, Castorina R, et al. (2013). Variability of organophosphorous pesticide metabolite levels in spot and 24-hr urine samples collected from young children during 1 week. Environ Health Perspect. (2013) 121:118–24. doi: 10.1289/ehp.1104808
40. O'Brien KM, Upson K, Buckley JP. Lipid and creatinine adjustment to evaluate health effects of environmental exposures. Curr Environ Health Rep. (2017) 4:44–50. doi: 10.1007/s40572-017-0122-7
41. Kwon W, Kim JY, Suh S, In MK. Simultaneous determination of creatinine and uric acid in urine by liquid chromatography tandem mass spectrometry with polarity switching electrospray ionization. Forensic Sci Int. (2012) 221:57–64. doi: 10.1016/j.forsciint.2012.03.025
42. Brown TA, Chorpita BF, Korotitsch W, Barlow DH. Psychometric properties of the depression anxiety stress scales (DASS) in clinical samples. Behav Res and Ther. (1997) 35:79–89. doi: 10.1016/S0005-7967(96)00068-X
43. Lovibond PF, Lovibond SH. The structure of negative emotional states comparison of the depression anxiety stress scales (DASS) with the beck depression and anxiety inventories. Behav Res Ther. (1995) 33:335–343. doi: 10.1016/0005-7967(94)00075-U
44. Oei TPS, Sawang S, Goh YW, Mukhtar F. Using the depression anxiety stress scale 21 (DASS-21) across cultures. Int J Psychol. (2013) 48:1018–1029. doi: 10.1080/00207594.2012.755535
45. Lester BM, Andreozzi-Fontaine L, Tronick E, Bigsby R. Assessment and evaluation of the high risk neonate: The NICU network neurobehavioral scale. J Visual Exp. (2014) 90:1–9. doi: 10.3791/3368
46. Noble Y, Boyd R. Neonatal assessments for the preterm infant up to 4 months corrected age: a systematic review. Dev Med Child Neurol. (2012) 54:129–39. doi: 10.1111/j.1469-8749.2010.03903.x
47. Muthen LK, Muthen BO. Mplus User's Guide (8th ed.) (1998–2017). Los Angeles, CA: Muthen and Muthen.
48. Provenzi. NICU network neurobehavioral scale: 1 month normative data and variation from birth to 1 month. Pediatr Res. (2018) 83:1104–9. doi: 10.1038/pr.2018.25
49. Fink NS. Healthy newborns neurobehavior: Norms and relations to medical and demographic factors. J Pediatr. (2012) 161:1073–9. doi: 10.1016/j.jpeds.2012.05.036
50. Wouldes TA, Woodward LJ. Neurobehavior of newborn infants exposed prenatally to methadone and identification of a neurobehavioral profile linked to poorer neurodevelopmental outcomes at age 24 months. PLoS ONE. (2020) 15:e0240905. doi: 10.1371/journal.pone.0240905
51. Kamanyire R, Karalliedde L. Organophosphate toxicity and occupational exposure. Occup Med. (2004) 54:69–75. doi: 10.1093/occmed/kqh018
52. Bradman A, Eskenazi B, Barr DB, Bravo R, Castorina R, Chevrier J, et al. Organophosphate urinary metabolite levels during pregnancy and after delivery in women living in an agricultural community. Environ Health Perspect. (2005) 113:1802–7. doi: 10.1289/ehp.7894
53. LaGasse LL, Wouldes T, Newman E, Smith LM, Shah RZ, Derauf C, et al. Prenatal methamphetamine exposure and neonatal neurobehavioral outcome in the USA and New Zealand. Neurotoxicol Teratol. (2011) 33:166–75. doi: 10.1016/j.ntt.2010.06.009
54. Lester BM, Tronick EZ, LaGasse L, Seifer R, Bauer CR, Shankaran S, et al. The maternal lifestyle study: Effects of substance exposure during pregnancy on neurodevelopmental outcome in 1-month-old infants. Pediatrics. (2002) 110:1182–92. doi: 10.1542/peds.110.6.1182
55. Yolton K, Khoury J, Xu Y, Succop P, Lanphear B, Bernert JT, et al. Low level prenatal exposure to nicotine and infant neurobehavior. Neurotoxicol Teratol. (2009) 31:356–63. doi: 10.1016/j.ntt.2009.07.004
56. Liu J, Bann C, Lester B, Tronick E, Das A, Lagasse L, et al. Neonatal neurobehavior predicts medical and behavioral outcome. Pediatrics. (2010) 125:e90–8. doi: 10.1542/peds.2009-0204
Keywords: organophosphates, NICU network neurobehavioral scale (NNNS), newborn, Thailand, farming, insecticides
Citation: Sittiwang S, Nimmapirat P, Suttiwan P, Promduang W, Chaikittipornlert N, Wouldes T, Prapamontol T, Naksen W, Promkam N, Pingwong S, Breckheimer A, Cadorett V, Panuwet P, Barr DB, Baumert BO, Ohman-Strickland P and Fiedler N (2022) The relationship between prenatal exposure to organophosphate insecticides and neurodevelopmental integrity of infants at 5-weeks of age. Front. Epidemiol. 2:1039922. doi: 10.3389/fepid.2022.1039922
Received: 13 September 2022; Accepted: 14 November 2022;
Published: 14 December 2022.
Edited by:
Deborah Watkins, University of Michigan, United StatesReviewed by:
Lidia Minguez Alarcon, Department of Medicine and Harvard Medical School, United StatesKimberly Yolton, Cincinnati Children's Hospital Medical Center, United States
Copyright © 2022 Sittiwang, Nimmapirat, Suttiwan, Promduang, Chaikittipornlert, Wouldes, Prapamontol, Naksen, Promkam, Pingwong, Breckheimer, Cadorett, Panuwet, Barr, Baumert, Ohman-Strickland and Fiedler. This is an open-access article distributed under the terms of the Creative Commons Attribution License (CC BY). The use, distribution or reproduction in other forums is permitted, provided the original author(s) and the copyright owner(s) are credited and that the original publication in this journal is cited, in accordance with accepted academic practice. No use, distribution or reproduction is permitted which does not comply with these terms.
*Correspondence: Nancy Fiedler, bmZpZWRsZXImI3gwMDA0MDtlb2hzaS5ydXRnZXJzLmVkdQ==
†These authors have contributed equally to this work
‡These authors share senior authorship