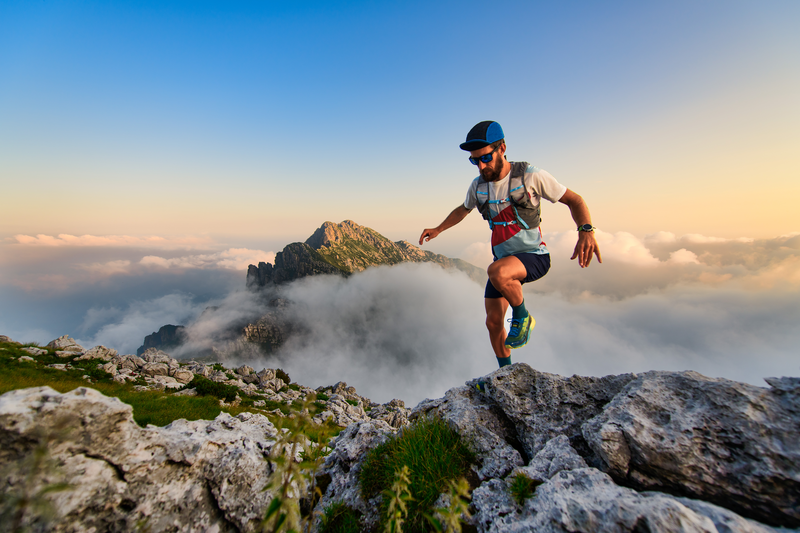
94% of researchers rate our articles as excellent or good
Learn more about the work of our research integrity team to safeguard the quality of each article we publish.
Find out more
ORIGINAL RESEARCH article
Front. Environ. Sci. , 10 March 2025
Sec. Toxicology, Pollution and the Environment
Volume 13 - 2025 | https://doi.org/10.3389/fenvs.2025.1548453
This study aimed to investigate cadmium (Cd) bioaccumulation and detoxification mechanisms in Pomacea insularum, based on specimens collected from 13 field populations in Peninsular Malaysia and transplantation experiments between polluted and unpolluted sites. Cd concentrations were analyzed in eight tissues, including the cephalic tentacle (CT), pineal sac (PS), digestive tract (DT), and shell, to determine their roles in metal uptake, storage, and excretion. The highest Cd concentration was recorded in the PS (13.5 mg/kg dry weight), while followed by the shells (5.91 mg/kg dry weight), indicating its role as a long-term sequestration site. Notably, the PS showed exceptionally high Cd accumulation, suggesting its potential as a sensitive biomarker for prolonged exposure. The transplantation study revealed that snails relocated from unpolluted to polluted sites accumulated Cd up to 5.22 mg/kg, while those transferred from polluted to unpolluted environments retained Cd between 1.00 and 6.03 mg/kg, indicating slow depuration and tissue-dependent retention. Correlation and regression analyses demonstrated significant interactions among soft tissues, with filtering and digestive organs playing a primary role in detoxification, while calcified structures contributed to long-term Cd sequestration. These findings highlight P. insularum as an effective biomonitor for assessing environmental Cd contamination and bioavailability, providing insights into both short-term exposure and long-term metal storage. Overall, this study reinforces the suitability of P. insularum in biomonitoring programs by demonstrating its ability to accumulate and retain Cd across different human activities. The exceptionally high Cd retention in specific tissues, particularly the PS, highlighting the importance of targeted tissue analysis for pollution assessment. Integrating P. insularum into long-term monitoring strategies can provide valuable data on contamination trends, aiding environmental management and freshwater ecosystem conservation.
Cadmium (Cd) is a persistent and highly toxic heavy metal that poses significant ecological and human health risks due to its widespread presence in aquatic environments, primarily from industrial discharge, mining activities, and agricultural runoff. Once introduced into water bodies, Cd remains in circulation for extended periods, accumulating in sediments and aquatic organisms, where it may biomagnify through the food chain (Maher et al., 2016; Artalina and Takarina, 2019; Astani et al., 2023; Emami et al., 2024). Elevated Cd concentrations have been reported in freshwater ecosystems worldwide, with detrimental effects on biodiversity, aquatic food webs, and public health due to its non-biodegradable nature and strong affinity for biological tissues (Simonyi-Poirier et al., 2003; Vidyalakshmi et al., 2024). As a result, the assessment of Cd bioaccumulation in aquatic species is critical for environmental monitoring and pollution management, particularly using bioindicator species capable of accumulating and retaining metals in measurable quantities (Taylor and Maher, 2006; Sabri et al., 2014; Ubrihien et al., 2017).
The freshwater gastropods have been extensively studied as bioindicators of heavy metal pollution due to their sedentary nature, high bioaccumulation potential, and sensitivity to environmental contaminants. Previous research has shown that various mollusc species accumulate Cd in metabolically active tissues such as the digestive gland, kidney, gills, and digestive caecum, with detoxification mechanisms varying among species (McGeer et al., 2011). Among these, Pomacea insularum has been recognized as a particularly robust biomonitor due to its wide distribution, adaptability to polluted environments, and efficient metal sequestration mechanisms. Cd bioaccumulation in P. insularum follows a species-specific pattern, where soft tissues primarily serve as sites for metal uptake and detoxification, while calcified structures such as shells and opercula to store detoxified Cd in order to minimize its toxic impact on vital organs (Yap et al., 2009; Zhai et al., 2017; Pedrini-Martha et al., 2021). While prior studies have explored Cd accumulation in molluscs, the specific roles of different tissues in detoxification, excretion, and long-term sequestration remain insufficiently understood, particularly under fluctuating contamination levels (Aroonsrimorakot et al., 2017; Pinkina et al., 2022).
The bioaccumulation and detoxification of Cd involve complex physiological processes, including protein binding, cellular sequestration, and transfer to excretable or biologically inactive forms. Metallothioneins, small cysteine-rich proteins, play a crucial role in Cd detoxification by binding to metal ions and facilitating their storage in less toxic forms within cellular compartments (Gnatyshyna et al., 2023). However, the tissue-specific variations in Cd processing, particularly how different organs contribute to overall metal regulation in P. insularum, remain underexplored. Understanding these physiological adaptations is essential for assessing the species’ effectiveness in biomonitoring and for developing more comprehensive models of Cd bioaccumulation and detoxification in freshwater ecosystems (Abdel Gawad, 2018; Banerjee et al., 2023).
This study aimed to investigate Cd bioaccumulation patterns in P. insularum by analyzing metal concentrations in eight different tissues across 13 field populations in Peninsular Malaysia and in transplanted specimens between polluted and unpolluted sites. By examining the tissue-specific roles in Cd uptake, detoxification, and storage, this study provides new insights into the mechanisms governing metal accumulation in freshwater gastropods.
The snails P. insularum and their habitat surface sediments (0–10 cm) were collected from 13 sampling sites in the rivers, ponds and lakes Peninsular Malaysia, representing a range of freshwater environments with varying levels of heavy metal contamination (Figure 1; Table 1). Sampling sites were selected based on proximity to potential sources of Cd pollution, including agricultural runoff and industrial discharge.
Figure 1. Sampling sites of Pomacea insularum and their habitat surface sediments in Peninsular Malaysia.
The snails were collected using hand-picking methods and were immediately placed in clean, labelled clean plastic bags. The surface sediments were collected using clean stainless steel scope. The specimens were transported to the laboratory on ice and kept in the freezer at −20°C until further analysis.
Transplantation experiments with the gastropod P. insularum were conducted at two selected sites: Juru River and UPM Lake. Juru River, classified as a polluted site, receives anthropogenic inputs from nearby factories and construction areas (Mat and Maah, 1994). In contrast, UPM Lake was considered an unpolluted site as it does not receive anthropogenic contaminants.
At least 100 individuals of snail P. insularum individuals (2.8–3.5 cm) were collected from UPM Lake and maintained in the laboratory for 24 h in five net cages (32 cm × 24 cm), each containing 20 snails. These cages were then placed in Juru River to initiate the experiment. Simultaneously, at least 100 individuals of native snails P. insularum from Juru River were collected and transplanted to UPM Lake. For the accumulation study, 10–20 snails P. insularum were retrieved from Juru River at weeks 1, 3, and 7 for Cd analysis. Similarly, for the depuration study, snails P. insularum were collected at the same intervals and analyzed for Cd depuration.
In the laboratory, the individual of snails from each sampling site were thawed and each specimen was carefully dissected to obtain eight distinct tissues: cephalic tentacle (CT), mantle, pineal sac (PS), remaining soft tissues (REM), digestive tract (DT), foot, operculum, and shell. Each tissue was rinsed with deionized water to remove external contaminants and then blotted dry with filter paper. The snail tissues and surface sediments were weighed and then dried at 105°C until a constant weight was achieved. The dried sediment samples were sieved using 63 µm siever. The dried snail tissues and sediment samples were homogenized and stored in acid-washed polyethylene containers until further analysis (Edward et al., 2010; Yap et al., 2010).
The sieved sediment samples and the dried samples from each pooled tissue type were analysed for Cd in triplicate samples. For the tissue samples, they were digested concentrated nitric acid (HNO3) (grade BDH; 69%), while for the sediment samples, they were digested in concentrated nitric acid (HNO3; Grade BDH, 69%) and perchloric acid (HClO4; Grade BDH, 60%). They were digested in 40°C for 1 h and then the temperature was increased to 140°C for 3 h. After digestion, the samples were filtered and diluted with deionized water to a final volume of 40 mL. Later, they were determined using an air-acetylene flame Atomic Absorption Spectrophotometry (AAS) AAnalyst Model 800. Calibration standards were prepared using commercially available Cd standards, and quality control was ensured by including certified reference materials for sediments (with acceptable recovery) and blank samples in each batch of analyses.
Statistical analyses were performed using NCSS software (NCSS, 2024). Descriptive statistics were calculated for each tissue’s Cd concentration. Pearson’s correlation coefficients were constructed to examine the relationships between Cd levels in different tissues, and habitat surface sediment; multiple regression analyses were conducted to determine the predictors of Cd concentrations in various tissues. Factor analysis with Varimax rotation was performed to identify underlying patterns of Cd accumulation across tissues and to group tissues based on their Cd handling characteristics. Graphs were generated using KaleidaGraph version 3.08 (November 1996). Analysis of variance (ANOVA) was used to compare mean metal concentrations, followed by Newman-Keuls multiple comparison tests to identify significant differences.
The concentrations of Cd in various tissues of P. insularum collected from different locations across Peninsular Malaysia exhibit significant spatial variations and bioaccumulative patterns. Figure 2 illustrates these variations, highlighting that the DT consistently exhibited the highest Cd concentrations across all sampling sites, followed by the shell, mantle, and operculum. Among the study locations, Melaka-2 recorded the highest Cd concentrations in most tissues, particularly in the DT, which reached approximately 9.5 mg/kg dry weight. This was followed by Subang-2 and Melaka-1, where DT concentrations exceeded 6.0 mg/kg dry weight.
Figure 2. Concentrations (mean ± SE, mg/kg dry weight) of Cd in the different tissues of Pomacea insularum, and their habitat surface sediments, collected from Peninsular Malaysia.
The shell, serving as a long-term storage site for metals, also exhibited high Cd concentrations, particularly in samples from Melaka-2, Melaka-1, and Subang-2, with values ranging from 5.5 to 7.5 mg/kg dry weight. In contrast, the foot and REM displayed relatively lower Cd concentrations, mostly between 1.0 and 3.0 mg/kg dry weight across all sampling sites. The operculum and CT also showed moderate levels of Cd accumulation, particularly in samples from Kelana-2 and Subang-1, which recorded concentrations between 2.5 and 3.5 mg/kg dry weight.
A comparison between Cd concentrations in P. insularum tissues and their corresponding habitat surface sediments indicates that metal accumulation in the gastropod follows a bioaccumulative pattern. The highest Cd sediment concentrations were found in Subang-2 and Kelana-2, where values reached up to 5.0 mg/kg dry weight. Notably, the PS exhibited extremely high Cd concentrations, particularly in samples from Subang-2, where levels reached approximately 60 mg/kg dry weight, indicating significant environmental contamination. Across all sites, DT consistently contained the highest Cd concentrations, followed by structural tissues such as the shell and mantle.
Table 2 provides overall statistics of Cd concentrations (mg/kg dry weight) in eight tissues of P. insularum collected from 13 sites across Peninsular Malaysia. The PS displayed the highest mean Cd concentration at 13.5 mg/kg dry weight with a large standard error, indicating significant variability in Cd bioaccumulation, with concentrations ranging from 2.32 to 69.4 mg/kg dry weight. This high variability suggests that the PS is highly exposed to fluctuating environmental Cd levels. The shell and DT also exhibited substantial Cd concentrations, with means of 5.91 and 3.58 mg/kg dry weight, respectively. The operculum had a relatively lower mean Cd concentration at 1.51 mg/kg dry weight, reflecting its role in long-term Cd storage, although at lower concentrations compared to soft tissues.
Table 2. Overall statistics of concentrations (mean ± SE, mg/kg dry weight) of Cd in the different tissues of Pomacea insularum, and their habitat surface sediments, collected from Peninsular Malaysia. N = 13.
The REM, CT, foot, and mantle displayed moderate Cd accumulation, with mean concentrations of 2.12, 2.11, 1.86, and 2.44 mg/kg dry weight, respectively. The mantle and DT serve important roles in both nutrient absorption and metal detoxification, while the REM functions as an intermediary tissue in Cd transport and storage. Spatially, the highest Cd accumulation in P. insularum tissues was observed in gastropods collected from Melaka-2, Melaka-1, and Subang-2, suggesting a strong link to anthropogenic sources of contamination. In contrast, samples from Kedah-1 and Kedah-2 exhibited the lowest Cd concentrations in most tissues, indicating relatively lower levels of environmental Cd exposure.
The correlation coefficients of Cd concentrations between different tissues of P. insularum and their habitat surface sediments are presented in Table 3. The results indicate significant inter-tissue correlations, with certain tissues exhibiting strong relationships, suggesting shared physiological or environmental influences on Cd accumulation.
Table 3. Correlation coefficients of Cd levels between in the eight parts of Pomacea insularum, and their habitat surface sediments (SED) collected from 13 sites in Peninsular Malaysia.
Among the soft tissues, the strongest positive correlation was observed between the foot and REM (r = 0.89, P < 0.05), followed by the foot and shell (r = 0.62, P < 0.05), and between the mantle and REM (r = 0.72, P < 0.05). The mantle also showed a strong positive correlation with the CT (r = 0.55, P < 0.05) and the operculum (r = 0.65, P < 0.05), suggesting that these tissues may exhibit similar Cd accumulation patterns. The DT displayed a moderate correlation with the cephalic tentacle (r = 0.56, P < 0.05) but showed weak or negative correlations with other tissues.
The correlation analysis between P. insularum tissues and habitat surface sediments revealed generally weak or negative associations. The shell and SED exhibited a negative correlation (r = −0.57, P < 0.05), as did the REM (r = −0.564, P < 0.05), mantle (r = −0.71, P < 0.05), and operculum (r = −0.70, P < 0.05). Conversely, the PS showed a significant positive correlation with the SED (r = 0.52, P < 0.05), indicating that Cd accumulation in this tissue may be directly influenced by environmental exposure.
The shell exhibits a strong correlation with the operculum (R = 0.76), suggesting that both calcified structures may be involved in long-term Cd storage. Interestingly, the CT has lower correlations with most other tissues, particularly the operculum (R = −0.30), suggesting that it accumulates Cd through different pathways, likely due to its direct exposure to environmental Cd sources. The REM also shows strong correlations with the operculum (R = 0.73). The correlation matrix provides insights into how Cd is distributed among tissues, with certain tissues exhibiting stronger bioaccumulation relationships than others.
Table 4 presents the results of a factor analysis performed on the Cd levels across eight parts of P. insularum, identifying four factors that explain the variation in Cd accumulation. The shell has the highest loading on Factor 1 (0.463), indicating its primary role in Cd storage. The CT, with a high loading on Factor 2 (0.820), suggests that this tissue is a key site for direct Cd uptake from the environment. The foot and mantle, both with strong loadings on Factor 3, reflect their shared role in Cd accumulation through filtration and nutrient absorption.
Table 4. Factor Structure Summary after Varimax Rotation based on Cd levels in the eight parts Pomacea insularum collected from 13 sites in Peninsular Malaysia. N = 39.
The operculum, with a high loading on Factor 4 (0.531), points to its distinct role in long-term Cd storage, similar to the shell. The REM and DT show moderate loadings across multiple factors, indicating their intermediary roles in Cd transport and bioaccumulation. The factor analysis reveals a clear division between tissues involved in direct Cd uptake (e.g., CT, foot) and those responsible for long-term storage (e.g., shell, operculum), highlighting the complexity of Cd accumulation dynamics in P. insularum.
Table 5 provides the results of multiple regression analyses, showing the predictors of Cd levels in selected tissues of P. insularum. The shell serves as a significant predictor of Cd levels in several other tissues, including the mantle (b = 4.46, P < 0.05) and operculum (b = 1.64, P < 0.05), indicating that the shell plays a central role in the overall Cd accumulation in the organism. The mantle also serves as a strong predictor for Cd levels in other tissues, particularly the foot (b = 4.39, P < 0.05), reflecting its involvement in Cd processing and transfer.
Table 5. Multiple regression analysis output on the Cd concentrations in selected part with their predictors of levels in the other seven parts of Pomacea insularum collected from 13 sites in Peninsular Malaysia. N = 39. Values in yellow are significant at P < 0.05.
The PS shows strong predictive capacity for Cd levels in the foot (b = 15.09, P < 0.05). The REM, with an R2 of 0.99, serve as strong predictors for Cd levels in the operculum and mantle, highlighting their intermediary role in Cd bioaccumulation. These regression models provide insights into how Cd levels in certain tissues can be used to predict Cd concentrations in other parts of P. insularum, reinforcing the close interactions between tissues involved in filtration, nutrient processing, and storage.
Therefore, the results from Tables 2–5 show that Cd accumulation in P. insularum is characterized by high variability across tissues, with the CT, operculum, and PS showing the highest Cd concentrations. The shell and other calcified structures serve as long-term storage sites, while the mantle, foot, and DT are key in Cd processing and bioavailability. The strong correlations and regression models highlight the interconnectedness of these tissues in managing Cd exposure, providing a comprehensive.
The accumulation of Cd concentrations in the different tissues of P. insularum following transplantation from an unpolluted site (UPM Lake) to a polluted site (Juru River) over 7 weeks is presented in Table 6. The results indicate a progressive increase in Cd levels across all tissues, with varying rates of accumulation. Among the tissues analyzed, the DT exhibited the highest increase in Cd concentration, starting from 1.33 mg/kg dry weight at week 0–3.44 mg/kg dry weight at week 7, reflecting a 159% increase. This rapid accumulation in the DT is expected due to its role in processing ingested food and environmental particulates, making it a key organ for assessing short-term Cd exposure.
Table 6. Accumulation of Cd concentrations (mean ± SE, mg/kg dry weight) in the different tissues of Pomacea insularum after 7 weeks transplanted from UPM Lake (unpolluted site) to Juru River (polluted site).
The mantle also displayed a substantial increase in Cd concentration, rising from 1.28 mg/kg dry weight at week 0–2.82 mg/kg dry weight at week 7, with an accumulation percentage (AP) of 120%. The PS, a tissue closely associated with detoxification processes, showed a 52.2% increase, rising from 3.43 mg/kg dry weight at week 0–5.22 mg/kg dry weight at week 7. The PS’s relatively high accumulation highlights its role in metal sequestration and its potential as a biomonitoring tissue reflecting environmental contamination.
The CT and foot also demonstrated significant increases in Cd concentrations, reaching 47.2% and 60.0% accumulation, respectively. The foot, primarily involved in locomotion and exposure to contaminated sediments, showed a steady increase from 1.30 mg/kg dry weight at week 0–2.08 mg/kg dry weight at week 7. The CT, which is in direct contact with water, exhibited gradual accumulation over the study period, suggesting its suitability for detecting dissolved Cd exposure. The shell displayed the lowest Cd accumulation rate (14.7%), increasing from 5.25 mg/kg dry weight at week 0–6.02 mg/kg dry weight at week 7. Given that the shell acts as a long-term storage site for metals, its relatively slow rate of accumulation aligns with its function in chronic metal sequestration.
The trend of Cd accumulation across all tissues was characterized by an initial increase in the first week, followed by a more pronounced rise from the third to the seventh week. In particular, the DT, mantle, and PS exhibited the steepest accumulation rates, suggesting that these tissues are highly responsive to environmental Cd contamination. The significant differences in accumulation patterns, as indicated by distinct alphabetical groupings (A, B, and C) in the statistical analysis, confirm that Cd bioaccumulation occurs at different rates depending on tissue type and function.
The depuration of Cd concentrations in various tissues of P. insularum after transplantation from a polluted site (Juru River) to an unpolluted site (UPM Lake) over 7 weeks is presented in Table 7. The results indicate varying degrees of Cd elimination across different tissues, with depuration percentages (DP) ranging from 6.45% in the shell to 67.8% in the DT. Among all tissues, the DT exhibited the highest depuration rate (67.8%), decreasing from 3.60 mg/kg dry weight at week 0–1.16 mg/kg dry weight at week 7. This significant reduction in Cd levels suggests that the DT is highly responsive to metal elimination, likely due to its dynamic metabolic activity and direct role in food digestion and excretion. The depuration process was most pronounced in the first 3 weeks, followed by a more gradual decrease in Cd levels towards the seventh week.
Table 7. Depuration of Cd concentrations (mean ± SE, mg/kg dry weight) in the different tissues of Pomacea insularum after 7 weeks transplanted from Juru River (polluted site) to UPM Lake (unpolluted site).
Similarly, the CT and mantle also demonstrated notable Cd depuration, with reductions of 60.0% and 59.5%, respectively. The CT Cd concentration declined from 2.50 mg/kg dry weight at week 0–1.00 ± 0.04 mg/kg dry weight at week 7, while the mantle showed a reduction from 3.28 mg/kg dry weight to 1.33 mg/kg dry weight over the same period. These results suggest that these tissues are actively involved in metal regulation, likely through excretion or tissue turnover mechanisms.
PS exhibited a 47.8% decrease in Cd levels, declining from 6.03 mg/kg dry weight to 3.15 mg/kg dry weight. Although the PS demonstrated a slower rate of depuration compared to the DT, its significant reduction indicates its ability to regulate metal detoxification over time. The operculum and foot tissues also showed moderate Cd depuration rates, with reductions of 47.9% and 30.0%, respectively. The foot Cd concentration dropped from 2.90 mg/kg dry weight at week 0–2.03 mg/kg dry weight at week 7, while the operculum showed a decrease from 2.57 mg/kg dry weight to 1.34 mg/kg dry weight. These tissues, which are in direct contact with the external environment, exhibited slower Cd elimination, possibly due to their structural roles and lower metabolic turnover.
In contrast, the shell exhibited the lowest depuration percentage (6.45%), with Cd levels decreasing only slightly from 5.58 mg/kg dry weight at week 0–5.22 mg/kg dry weight at week 7. This finding aligns with previous research indicating that the shell serves as a long-term storage site for metals, where Cd is incorporated into calcium carbonate structures and remains relatively stable over time. The depuration process varied across different tissues over the 7-week period. While the DT, CT, and mantle exhibited rapid Cd elimination within the first 3 weeks, the PS and operculum displayed a more gradual decline in Cd levels. The shell, due to its mineralized structure, showed minimal depuration, reinforcing its role as a long-term metal repository.
Overall, the results indicate that soft tissues such as the DT, CT, and mantle are highly responsive to depuration processes, making them suitable indicators for assessing short-term reductions in metal contamination, while the shell provides long-term insights into historical metal exposure. These findings further support the use of P. insularum as a biomonitoring species for tracking Cd contamination and its elimination dynamics in aquatic environments.
The spatial distribution of Cd concentrations across different sampling sites in Peninsular Malaysia highlights significant environmental variations in metal contamination. The highest Cd accumulation was recorded in P. insularum collected from Melaka-2, Melaka-1, and Subang-2, with the DT and shell showing the most pronounced levels of bioaccumulation. The elevated Cd concentrations in these specific sites suggest that these aquatic environments are exposed to persistent Cd contamination, likely originating from anthropogenic sources such as industrial effluents, urban discharge, and agricultural runoff. Notably, in Melaka-2, the Cd concentration in the DT reached approximately 9.5 mg/kg dry weight, significantly exceeding levels observed in other tissues. Similarly, high concentrations in the shell from Melaka-2 and Subang-2 (ranging from 5.5 to 7.5 mg/kg dry weight) indicate long-term exposure to Cd contamination (Yap et al., 2009).
The DT exhibited the highest Cd concentration among all tissues across multiple sites, reaffirming its role as a primary organ for metal absorption and accumulation. Since the DT is responsible for processing ingested food and environmental particulates, the elevated Cd levels in this tissue suggest that the metal primarily enters the gastropods through dietary intake, which could include periphyton, detritus, or organic sediments contaminated with Cd. The relatively lower concentrations observed in other soft tissues such as the foot and operculum indicate that while these tissues do accumulate Cd, their accumulation rates are slower compared to the DT, which acts as an immediate repository of ingested metals (Campoy-Diaz et al., 2018; Huang et al., 2018).
An interesting observation is the strong positive correlation between Cd levels in the PS and sediment Cd concentrations. This suggests that the pineal sac may serve as a tissue that directly reflects environmental Cd contamination, particularly in sediments. The significant accumulation in the PS tissue across contaminated sites, particularly in Subang-2 and Kelana-2, further suggests that this organ may be particularly sensitive to metal exposure and could serve as an early indicator of environmental contamination (Mero et al., 2019; Qiu et al., 2025).
The high Cd levels in the shell, mantle, and operculum at sites such as Melaka-2 and Subang-2 provide additional evidence of chronic exposure to Cd contamination in these environments. These structural tissues are known to incorporate heavy metals into their mineralized structures over extended periods, making them valuable indicators of long-term pollution trends. The significant accumulation of Cd in these tissues suggests that P. insularum at these sites has been exposed to persistent Cd contamination, either through waterborne exposure or trophic transfer from contaminated food sources (Yap et al., 2009; Zhai et al., 2017).
In contrast, sites such as Kedah-1 and Kedah-2 exhibited the lowest Cd concentrations across most tissues, suggesting lower levels of environmental Cd contamination. The marked difference in accumulation patterns between high-contamination sites such as Melaka-2 and lower-contamination sites such as Kedah-1 supports the conclusion that local environmental factors, including pollution sources and sediment interactions, play a critical role in influencing Cd bioavailability and uptake in P. insularum.
The correlation analysis of Cd concentrations among different tissues provides critical insights into the suitability of specific tissues for biomonitoring purposes. The DT, which consistently exhibited the highest Cd concentrations, emerges as a primary biomonitoring tissue for detecting recent Cd exposure. The moderate correlation between the DT and CT suggests that these tissues may share similar exposure routes, likely via ingestion of contaminated particles and food sources. However, the relatively weak correlation between DT and environmental sediments indicates that Cd accumulation in the DT is not solely influenced by direct environmental exposure but rather through trophic transfer mechanisms (Krupnova et al., 2018; Liu et al., 2022).
The shell and mantle also play a crucial role in biomonitoring, particularly for assessing long-term metal exposure. The shell, which serves as a repository for metal sequestration, exhibited strong correlations with other structural tissues, such as the foot and mantle. This suggests that these tissues function as long-term indicators of Cd bioaccumulation, retaining metals absorbed over extended periods. The mantle, in particular, demonstrated the highest inter-tissue correlations, particularly with the foot and operculum, reinforcing its potential as a reliable biomonitoring tissue (Abdel Gawad, 2018; Banerjee et al., 2023).
The negative correlations between Cd levels in habitat sediments and structural tissues, such as the mantle, operculum, and shell, indicate that Cd accumulation in these tissues is not a direct function of sediment metal concentrations. This suggests that Cd uptake in P. insularum is regulated by physiological processes, and that structural tissues reflect metal bioavailability in the water column rather than direct sediment contact. This finding is significant as it underscores the importance of analyzing multiple tissues to gain a comprehensive understanding of Cd exposure pathways (Chukaeva and Petrov, 2023; Mero et al., 2019; Qiu et al., 2025).
A notable exception is the PS, which exhibited a strong positive correlation with sediment Cd levels. This suggests that the PS may be a key biomonitoring tissue for assessing direct environmental contamination, particularly in benthic habitats where Cd may be more bioavailable due to sediment interactions. The fact that the PS Cd concentrations were highest in sites with elevated sediment contamination, such as Subang-2 and Kelana-2, further supports its utility as an environmental biomonitoring tissue (Yap et al., 2009).
From a biomonitoring perspective, these findings highlight the complementary roles of different tissues in assessing Cd contamination in aquatic ecosystems. The DT is best suited for detecting recent Cd exposure through dietary intake, while the shell and mantle serve as indicators of long-term bioaccumulation trends. The PS emerges as a potential sentinel tissue for detecting sediment-bound Cd contamination, making it a particularly valuable tissue for site-specific pollution assessment. Given these distinct bioaccumulation patterns, a multi-tissue approach is recommended for future biomonitoring studies involving P. insularum, as it provides a more comprehensive assessment of Cd contamination across both short-term and long-term exposure pathways (Pedrini-Martha et al., 2021).
Overall, these results reinforce the use of P. insularum as a reliable biomonitoring species for assessing Cd pollution in aquatic environments. The high sensitivity of DT to Cd exposure, the long-term accumulation potential of the shell and mantle, and the strong correlation between PS and sediment Cd levels collectively provide a robust framework for monitoring environmental contamination. Future research should further explore these relationships in different aquatic habitats and under varying environmental conditions to enhance the understanding of metal bioaccumulation dynamics in gastropods.
The results of Cd accumulation and depuration in P. insularum demonstrate its strong potential as a biomonitoring species for metal contamination in aquatic environments. From an ecotoxicological perspective, P. insularum exhibited substantial Cd uptake when exposed to a polluted environment (Juru River), followed by significant depuration when transferred to a cleaner habitat (UPM Lake). These findings highlight the gastropod’s ability to effectively reflect environmental Cd levels through its bioaccumulation and elimination dynamics (Rainbow, 2002).
Cd accumulation was evident in all tissues, with the DT showing the highest increase during the 7-week exposure period, followed by the mantle and PS. The high Cd bioaccumulation in the DT aligns with its physiological function as the primary organ for digestion and absorption, making it highly responsive to changes in environmental metal concentrations. This rapid accumulation in soft tissues indicates that P. insularum is sensitive to Cd exposure, supporting its role as a biomonitoring organism in ecotoxicological studies. The DT exhibited the highest Cd accumulation during exposure (159%) and the most efficient depuration (67.8%), making it the most responsive tissue to environmental Cd fluctuations. Since Cd enters organisms primarily through dietary intake and water exposure, the DT serves as a critical organ for monitoring recent Cd exposure, particularly in environments with fluctuating pollution levels (Gunawan et al., 2020).
The mantle also exhibited substantial Cd accumulation (120%) and high depuration (59.5%), reinforcing its role as an effective biomonitoring tissue. As the mantle plays a vital role in shell formation and structural maintenance, it integrates Cd accumulation over an extended period while remaining responsive to environmental changes (Yap et al., 2009). Following transplantation to the unpolluted site, the depuration results further reinforce the validity of using P. insularum as a biomonitor, as the DT demonstrated the most efficient Cd elimination, followed by the mantle and CT. These depuration trends suggest that P. insularum possesses a well-developed physiological mechanism for metal regulation, possibly through excretion, detoxification, or redistribution processes. Such characteristics are crucial for an effective biomonitoring species, as they allow for distinguishing between chronic and transient metal exposures (Huang et al., 2018).
Moreover, the varying rates of accumulation and depuration across tissues indicate that P. insularum can provide insights into both short-term and long-term metal contamination. Soft tissues, such as the DT and mantle, rapidly respond to environmental Cd fluctuations, making them useful indicators of recent pollution events. In contrast, the shell exhibited the lowest accumulation (14.7%) and minimal depuration (6.45%), highlighting its role as a long-term repository for Cd exposure. This distinction enhances the robustness of P. insularum as a biomonitor, allowing researchers to assess both acute metal exposure and historical contamination trends (Yap et al., 2009).
Therefore, the DT is the most accurate biomonitoring tissue for short-term Cd exposure, while the PS and mantle provide valuable insights into long-term contamination and bioavailability. A multi-tissue approach is recommended for future biomonitoring studies, as it allows for a comprehensive assessment of metal contamination in aquatic ecosystems. By integrating tissues with different accumulation and depuration characteristics, researchers can obtain a clearer picture of both immediate and historical Cd pollution dynamics, further strengthening the role of P. insularum as a key biomonitoring species in freshwater environments (Gunawan et al., 2020). Its widespread presence in tropical aquatic systems and ability to reflect environmental metal fluctuations make it an excellent candidate for monitoring heavy metal pollution in freshwater ecosystems.
The PS, which demonstrated high Cd accumulation during exposure and moderate depuration (47.8%), serves as a complementary biomonitoring tissue, particularly for assessing metal storage and detoxification mechanisms. The PS displayed a significant correlation with sediment Cd levels, indicating that it may be a useful indicator of Cd bioavailability from the surrounding environment. This suggests that the PS could provide valuable insights into the bioaccessibility of sediment-bound metals and their potential impacts on aquatic organisms.
The PS of P. insularum exhibit elevated (Cd levels, likely due to the absence of an excretion mechanism within this tissue, the Cd entering the metabolically available pool can be utilized for essential functions or stored in a detoxified form if in excess (Rainbow, 2002). However, in the case of Cd, a non-essential and potentially harmful metal, the PS follows a pathway lacking excretion, resulting in continuous accumulation and storage in the detoxified compartment (Pedrini-Martha et al., 2021; Gonçalves et al., 2016). This storage capacity within the PS, devoid of effective excretion, underscores the tissue’s function as a primary repository for non-essential metals, highlighting the physiological strategies that allow P. insularum to endure Cd exposure in contaminated environments (Dhara et al., 2017).
The absence of excretion from the PS facilitates the continuous buildup of Cd, making it one of the primary tissues responsible for Cd storage in P. insularum (Da et al., 2024). Cd is absorbed from the surrounding environment, primarily through the ingestion of contaminated sediments and water. As Cd enters the metabolically available pool, any amount not immediately detoxified or utilized accumulates within the PS without being excreted. This pattern mirrors the accumulation of non-essential metals in other freshwater organisms, where detoxification is prioritized over excretion, as documented in other molluscs (Gnatyshyna et al., 2023; Rainbow, 2002).
Given the PS’s function as a regulatory organ, its high factor loading in the analysis further emphasizes its significance in the organism’s strategy for managing Cd exposure. Acting as a long-term repository, the PS ensures that Cd concentrations in critical tissues like the gills or muscle remain non-toxic, reducing the risk of interference with vital metabolic functions (Pedrini-Martha et al., 2021; Pinkina et al., 2022). In the absence of an excretion mechanism, the PS sequesters Cd in a biologically inactive form, likely bound to metallothioneins or stored within cellular granules, as observed in similar detoxification mechanisms among other molluscs (Rainbow, 2002).
The accumulation of Cd in the PS without excretion resembles the patterns observed for other toxic metals, such as Zn and Cu, in organisms that rely more on detoxification than excretion. This strategy prevents Cd from reaching metabolically active tissues, where it could disrupt enzymatic processes or metabolic functions, thus protecting the organism’s health in Cd-contaminated environments (Karakaş and Otludil, 2020). However, the lack of excretion also poses a risk for P. insularum in environments with high Cd levels, as continued exposure could lead to concentrations exceeding the tissue’s detoxification capacity, although it remains a critical protection mechanism for more sensitive tissues (Dvorak et al., 2019; Owojori et al., 2022).
Conversely, the shell exhibited the lowest Cd accumulation and depuration rates, suggesting that it is not a suitable tissue for detecting recent Cd exposure. While the shell retains metals over long periods, its slow accumulation dynamics make it less effective for assessing short-term contamination. Instead, the shell could be used as a secondary biomonitor for evaluating historical pollution trends (Reolid et al., 2024).
Cd accumulation in the P. insularum shell plays a critical role in managing metal toxicity, functioning as a long-term storage site for detoxified metals. The regulatory model in Figure 3 shows the process by which metals like Cd are absorbed and managed within soft tissues, where they remain metabolically available until detoxified and stored in a non-toxic form (Rainbow, 2002). While the model effectively describes the regulatory processes in soft tissues, it does not entirely account for the passive storage role of the shell (Glevitzky et al., 2019; Pedrini-Martha et al., 2020; Pedrini-Martha et al., 2021).
Figure 3. The conceptual model of Cd accumulation pattern of the shells of apple snail Pomacea insularum. Note: This diagram is generated using Napkin AI with modification, accessed on 10 January 2025.
Calcified tissues like the shell and operculum act as long-term storage sites for Cd, where accumulation primarily occurs without excretion. The shell sequesters Cd in an inactive form, thus removing it from the organism’s metabolic processes and preventing Cd toxicity in more sensitive tissues (Reátegui-Zirena et al., 2017). The operculum, with a mean concentration of 5.91 mg/kg, similarly functions as a storage site for detoxified Cd, effectively supporting the overall detoxification strategy of the organism (Menon et al., 2023). Banerjee et al. (2023) showed that P. maculata operculum effectively accumulates toxic metals, making it a promising bioindicator for assessing freshwater pollution and monitoring ecosystem health over time (Marimoutou et al., 2023).
The shell, acting as a passive repository, sequesters excess Cd in an inert form over time. The high Cd levels in the shell compared to soft tissues highlight its essential role in metal detoxification and long-term storage, thus preventing Cd from accumulating in metabolically active tissues, where it could disrupt cellular processes (Reátegui-Zirena et al., 2017). This storage in the calcium carbonate matrix of the shell stabilizes Cd, isolating it from the organism’s physiological functions (Gnatyshyna et al., 2023). Unlike soft tissues that actively regulate Cd through excretion, the shell lacks excretory pathways, meaning that Cd incorporated into its structure remains indefinitely (Huang et al., 2018).
The absence of an excretion mechanism in the shell enhances its function as a long-term detoxification site, contrasting with tissues like the DT, which can transfer or excrete Cd as needed. This permanent sequestration in the shell reflects the organism’s cumulative exposure to Cd over its lifespan, offering a historical record of environmental contamination (De Silva et al., 2023). Consequently, while the model in Figure 3 is relevant for understanding Cd regulation in soft tissues, it does not capture the shell’s role in permanent storage, underscoring the complementary functions of soft and hard tissues in metal management (Nigariga et al., 2023).
Cd bioavailability in P. insularum is influenced by interactions among soft tissues, notably the mantle, foot, and DT. The high correlation between the mantle and foot (R = 0.92) indicates that these tissues share similar pathways for Cd absorption, likely linked to their roles in nutrient and metal processing. The mantle, as a central tissue, has a predictive capacity for Cd levels in other tissues, including the foot. This suggests that the mantle absorbs Cd from the environment or diet and redistributes it to other tissues for detoxification or storage (De Silva et al., 2023). The foot demonstrated moderate bioaccumulation and depuration rates, making them useful but less reliable indicators compared to the DT, PS, and mantle. The foot, being in direct contact with sediments, may reflect environmental Cd exposure, but its lower accumulation and slower depuration suggest that it is not as sensitive as other soft tissues.
The foot of P. insularum exhibits relatively low Cd accumulation compared to other tissues, likely due to its role in excreting excess Cd. In Figure 4, metals like Cd that enter the metabolically available pool may be utilized for biological functions or detoxified and stored when present in excess (Rainbow, 2002). For the foot, an excretion pathway allows for the removal of Cd from the tissue, preventing accumulation and maintaining homeostasis (Karakaş and Otludil, 2020).
Figure 4. The conceptual model of Cd accumulation pattern of the apple snail Pomacea insularum showing net accumulation of Cd (a non-essential metal) with some excretion of Cd accumulated in detoxified form (Rainbow, 2002). Note: This diagram is generated using Napkin AI with modification, accessed on 10 January 2025.
Given the foot’s direct interaction with sediment, it remains in constant exposure to Cd, yet effective excretion ensures Cd does not reach toxic levels, as shown by the lower concentrations in this tissue relative to others like the DT (Pedrini-Martha et al., 2021). This excretory role aligns with findings in other molluscs and crustaceans, where certain tissues specialize in both metal absorption and excretion to limit long-term storage of potentially harmful elements like Cd (Rainbow, 2002; Dhara et al., 2017). The ability of the foot to expel excess Cd plays a crucial role in the organism’s metal regulation strategy, contributing to P. insularum’s resilience in contaminated environments (Pinkina et al., 2022).
The foot, being in direct contact with both sediment and water, serves as a primary interface for environmental metal absorption. However, unlike tissues primarily involved in long-term Cd detoxification, such as the PS, the foot possesses an effective mechanism for excreting Cd, as illustrated by the process in the regulation model where stored metals in detoxified form are expelled from the organism (Gonçalves et al., 2016). This excretion capability allows the foot to regulate internal Cd concentrations effectively, ensuring that any absorbed Cd does not remain in the tissue for extended periods. This mechanism reflects adaptive metal regulation commonly seen in molluscs that rely on excretion as a detoxification strategy (Gnatyshyna et al., 2023).
The relatively low Cd concentration in the foot, as shown in statistical data, reflects a balance between metal absorption and excretion. As Cd enters the available pool, the foot promptly detoxifies and excretes excess metal, keeping levels below toxic thresholds (Rainbow, 2002). This regulatory process prevents the foot from serving as a long-term storage site for Cd, as observed in other tissues like the DT or mantle, which tend to accumulate Cd due to limited excretory pathways (Owojori et al., 2022; Pedrini-Martha et al., 2020). The foot’s ability to regulate Cd aligns with its physiological roles, including movement and environmental interaction, ensuring that the organism’s mobility and health are not compromised by metal accumulation (Reátegui-Zirena et al., 2017).
The foot’s role in Cd excretion is integral to P. insularum’s overall strategy for managing metal exposure, reducing its physiological burden by preventing Cd buildup in metabolically active tissues. By diverting Cd from the metabolically available pool to detoxified storage and eventual excretion, the foot helps protect the organism from Cd toxicity, supporting its survival in contaminated environments (Rainbow, 2002).
Based on Table 8, the findings from this study align with previously reported Cd accumulation patterns in Pomacea species, emphasizing their capacity as biomonitors of heavy metal contamination in freshwater ecosystems. Compared to the polluted Juru River field samples of P. insularum (Yap et al., 2009), which recorded a Cd range of 2.01–5.40 mg/kg, the present study found slightly lower Cd concentrations in P. insularum transplanted from unpolluted to polluted sites (1.28–5.22 mg/kg) and those transplanted from polluted to unpolluted sites (1.00–6.03 mg/kg). The slight variations could be attributed to differences in pollution sources, exposure duration, and environmental conditions influencing Cd bioavailability.
Additionally, field-collected P. canaliculata samples from Lampung Province, Indonesia (Gunawan et al., 2020) showed a considerably lower Cd accumulation (0.03 mg/kg, wet weight unspecified), indicating lower environmental contamination in that region or species-specific differences in Cd uptake. In contrast, experimental exposure studies (Huang et al., 2018; Qiu et al., 2025) demonstrated significantly higher Cd concentrations in P. canaliculata under controlled laboratory conditions. Specifically, Qiu et al. (2025) reported an extreme Cd accumulation of 17.4 mg/kg after 16 days of exposure to 10 μg/L Cd, suggesting that laboratory conditions with continuous exposure may facilitate greater metal uptake compared to fluctuating natural environments. Similarly, the hepatopancreas of P. canaliculata in Huang et al.’s (2018) study accumulated Cd levels between 0.64 and 3.98 mg/kg, which is comparable to the range observed in P. insularum from this study.
A particularly novel finding from this study is the high Cd concentration in the pineal sac of P. insularum, which peaked at 13.5 mg/kg, with the overall maximum Cd concentration in field-collected samples reaching 3.58 mg/kg. This value surpasses the 3.70 mg/kg observed in unpolluted UPM Lake field samples (Yap et al., 2009), suggesting that even within unpolluted environments, P. insularum can accumulate significant Cd levels in specific tissues. The ability of the pineal sac to retain high Cd concentrations may indicate a potential storage mechanism or an underexplored detoxification pathway in gastropods, warranting further histological and physiological investigations.
The transplantation experiments in this study provide further insights into the dynamic nature of Cd accumulation and depuration. While P. insularum transplanted to polluted sites accumulated Cd to levels comparable with previously reported studies, those relocated to unpolluted sites still retained measurable Cd concentrations (1.00–6.03 mg/kg). This suggests that depuration processes may vary depending on tissue type and exposure history, reinforcing the importance of long-term monitoring to assess recovery potential in decontaminated environments.
This study examined Cd accumulation across 13 field populations of P. insularum in Peninsular Malaysia, revealing significant variation in metal uptake among different sites. The highest recorded Cd concentration in the PS based on the field-collected samples, highlighting its potential as a sensitive biomarker for long-term exposure. These findings indicate that even in less contaminated environments, P. insularum can accumulate substantial Cd levels, emphasizing the importance of continuous environmental monitoring. Tissue-specific Cd distribution patterns showed that soft tissues, including the CT, PS, and DT, retained the highest Cd levels due to their direct exposure to contaminants. Meanwhile, calcified structures like the shell and operculum functioned as long-term detoxification sites, sequestering Cd in biologically inactive forms to minimize toxicity. The correlation between different tissues suggests a coordinated internal regulation of Cd, with the mantle and foot acting as intermediaries in metal processing.
The transplantation study further demonstrated the dynamic nature of Cd bioaccumulation and depuration in P. insularum. Snails transplanted from unpolluted to polluted sites accumulated Cd concentrations confirmed their responsiveness to environmental contamination. Conversely, those relocated from polluted to unpolluted sites retained Cd levels indicated that depuration is a slow and tissue-dependent process. These results suggest that once Cd is integrated into soft tissues, particularly in metabolically active organs, its clearance remains incomplete over the study period. The persistence of Cd even after relocation reinforces the use of P. insularum as a biomonitor, providing insights into both short-term and historical pollution exposure. Moving forward, further investigations into metal detoxification mechanisms, particularly in calcified structures, could enhance our understanding of Cd dynamics in freshwater ecosystems. Incorporating P. insularum into long-term biomonitoring programs will enable environmental managers to assess contamination trends, identify pollution sources, and implement effective conservation measures to protect aquatic biodiversity.
The raw data supporting the conclusions of this article will be made available by the authors, without undue reservation.
The manuscript presents research on animals that do not require ethical approval for their study.
CY: Conceptualization, Data curation, Formal Analysis, Investigation, Methodology, Project administration, Supervision, Writing–original draft, Writing–review and editing. KA-M: Funding acquisition, Resources, Software, Validation, Visualization, Writing–review and editing.
The author(s) declare that financial support was received for the research, authorship, and/or publication of this article. We acknowledge the research funding from the Putra Grant (Vote no.: 9752600), granted by Universiti Putra Malaysia.
The authors thank the undergraduate Mr. Pang, B.H. for her laboratory work.
The authors declare that the research was conducted in the absence of any commercial or financial relationships that could be construed as a potential conflict of interest.
The authors declare that Generative AI was partly (about 25%) used in the creation of this manuscript. Napkin AI was used to create Figures 3, 4 and Grammarly was used to check and improve the language.
All claims expressed in this article are solely those of the authors and do not necessarily represent those of their affiliated organizations, or those of the publisher, the editors and the reviewers. Any product that may be evaluated in this article, or claim that may be made by its manufacturer, is not guaranteed or endorsed by the publisher.
Abdel Gawad, S. S. (2018). Concentrations of heavy metals in water, sediment, and mollusk gastropod Lanistes carinatus from Lake Manzala, Egypt. Egypt. J. Aquatic Res. 44 (2), 77–82. doi:10.1016/j.ejar.2018.05.001
Aroonsrimorakot, S., Sakulkiatpanya, T., and Muangkun, S. (2017). “Heavy metal concentration in the components of golden apple snail (Pomacea canaliculata) and pond snail (Filopaludina martensi),” in Proceedings of the conference. Available at: https://api.semanticscholar.org/CorpusID:103511405.
Artalina, D., and Takarina, N. D. (2019). Metals content in edible gastropod from blanakan silvofishery ponds. J. Phys. Conf. Ser. 1245 (1), 012032. doi:10.1088/1742-6596/1245/1/012032
Astani, M., Moradi, A. M., and Mostafavi, P. G. (2023). Using biomarkers to assess the toxicity of environmental concentrations of heavy metals to the marine gastropod Thais mutabilis. Regional Stud. Mar. Sci. 59, 102803. doi:10.1016/j.rsma.2022.102803
Banerjee, S., Deoli, N. T., Whitlow, H. J., and Klerks, P. L. (2023). Invasive or biomonitoring species? Use of Pomacea maculata operculum as a tool to determine metal pollutants: a micro-PIXE investigation. Water, Air, and Soil Pollut. 234, 353. doi:10.1007/s11270-023-06327-3
Campoy-Diaz, A. D., Arribére, M. A., Guevara, S. R., and Vega, I. A. (2018). Bioindication of mercury, arsenic, and uranium in the apple snail Pomacea canaliculata (Caenogastropoda, Ampullariidae): bioconcentration and depuration in tissues and symbiotic corpuscles. Chemosphere 196, 196–205. doi:10.1016/j.chemosphere.2017.12.145
Chukaeva, M., and Petrov, D. (2023). Assessment and analysis of metal bioaccumulation in freshwater gastropods of urban river habitats, Saint Petersburg (Russia). Environ. Sci. Pollut. Res. 30 (3), 7162–7172. doi:10.1007/s11356-022-21955-8
Da, Y.-M., Li, S.-S., Li, Y.-Q., Deng, L.-Y., Li, M.-J., Huang, T., et al. (2024). Effects of cadmium on the intestinal health of the snail Bradybaena ravida Benson. Ecotoxicology 33 (8), 849–858. doi:10.1007/s10646-024-02783-7
De Silva, N. A. L., Marsden, I. D., Gaw, S., and Glover, C. N. (2023). Physiological and biochemical responses of the estuarine pulmonate mud snail, Amphibola crenata, sub-chronically exposed to waterborne cadmium. Aquat. Toxicol. 256, 106418. doi:10.1016/j.aquatox.2023.106418
Dhara, K., Saha, N. C., and Maiti, A. K. (2017). Studies on acute and chronic toxicity of cadmium to freshwater snail Lymnaea acuminata (Lamarck) with special reference to behavioral and hematological changes. Environ. Sci. Pollut. Res. 24 (35), 27326–27333. doi:10.1007/s11356-017-0349-8
Dvorak, M., Schnegg, R., Niederwanger, M., Pedrini-Martha, V., Ladurner, P., Lindner, H., et al. (2019). Cadmium pathways in snails follow a complementary strategy between metallothionein detoxification and auxiliary inactivation by phytochelatins. Int. J. Mol. Sci. 21 (1), 7. doi:10.3390/ijms21010007
Edward, F. B., Yap, C. K., and Tan, S. G. (2010). Interspecific variation of heavy metal concentrations in the different tissues of tropical intertidal gastropods from Malaysia. Toxicol. Environ. Chem. 92 (6), 1121–1134. doi:10.1080/02772240903252165
Emami, H., Abtahi, B., and Shokri, M. R. (2024). Heavy metal bioaccumulation (Ni, V, and Hg) in soft tissues of crustaceans, bivalves, and gastropods: a case study on the Northern Persian Gulf. Casp. J. Environ. Sci. 22 (2), 255–265. doi:10.22124/CJES.2023.7212
Glevitzky, M., Dumitrel, G.-A., Glevitzky, I., and Popa, M. (2019). Study on contamination of snail soft tissues (Helix pomatia) with cadmium in Sibiu County, Romania. J. Environ. Prot. Ecol. 20 (2), 599–607.
Gnatyshyna, L., Khoma, V., Martinyuk, V., Matskiv, T., Pedrini-Martha, V., Niederwanger, M., et al. (2023). Sublethal cadmium exposure in the freshwater snail Lymnaea stagnalis meets a deficient, poorly responsive metallothionein system while evoking oxidative and cellular stress. Comp. Biochem. Physiology Part - C Toxicol. Pharmacol. 263, 109490. Article 109490. doi:10.1016/j.cbpc.2022.109490
Gonçalves, S. F., Davies, S. K., Bennett, M., Raab, A., Feldmann, J., Kille, P., et al. (2016). Sub-lethal cadmium exposure increases phytochelatin concentrations in the aquatic snail Lymnaea stagnalis. Sci. Total Environ. 568, 1054–1058. doi:10.1016/j.scitotenv.2016.06.149
Gunawan, A., Achyani, A., Muhfahroyin, M., Sulistiani, W. S., and Widowati, H. (2020). “The processing of golden snail (Pomacea canaliculata) to reduce levels of heavy metal lead (Pb) and cadmium (Cd),” in Proceedings of the conference. Available at: https://api.semanticscholar.org/CorpusID:230530407.
Huang, F., Peng, L., Zhang, J., Lin, W., and Chen, S. (2018). Cadmium bioaccumulation and antioxidant enzyme activity in hepatopancreas, kidney, and stomach of invasive apple snail Pomacea canaliculata. Environ. Sci. Pollut. Res. 25 (19), 18682–18692. doi:10.1007/s11356-018-2092-1
Karakaş, S. B., and Otludil, B. (2020). Accumulation and histopathological effects of cadmium on the great pond snail Lymnaea stagnalis Linnaeus, 1758 (Gastropoda: pulmonata). Environ. Toxicol. Pharmacol. 78, 103403. Article 103403. doi:10.1016/j.etap.2020.103403
Krupnova, T. G., Mashkova, I. V., Kostryukova, A. M., Schelkanova, E. E., and Gavrilkina, S. V. (2018). Gastropods as potential biomonitors of contamination caused by heavy metals in South Ural lakes, Russia. Ecol. Indic. 95, 1001–1007. doi:10.1016/j.ecolind.2017.12.005
Liu, X., Yu, S., Chen, P., Hong, B., Zhang, Y., Lin, X., et al. (2022). Metal loadings in estuarine bivalve and gastropod shellfish in response to socioeconomic development in watershed. Mar. Environ. Res. 176, 105593. Article 105593. doi:10.1016/j.marenvres.2022.105593
Maher, W., Maher, N., Taylor, A., Krikowa, F., Ubrihien, R., and Mikac, K. M. (2016). The use of the marine gastropod Cellana tramoserica as a biomonitor of metal contamination in nearshore environments. Environ. Monit. Assess. 188 (7), 391. doi:10.1007/s10661-016-5380-6
Marimoutou, M., Oriot, J., Baldoni-Andrey, P., Bareille, G., Boullemant, A., Gelber, C., et al. (2023). Metal localisation in gastropod shells: new insights from mass spectrometry techniques. Chemosphere 344, 140375. doi:10.1016/j.chemosphere.2023.140375
Mat, I., and Maah, M. J. (1994). An assessment of trace metal pollution in the mudflats of Kuala Selangor and Batu Kawan, Malaysia. Mar. Pollut. Bull. 28 (8), 512–514. doi:10.1016/0025-326X(94)90526-6
McGeer, J. C., Niyogi, S., and Smith, D. S. (2011). “Cadmium,”. Fish physiology. Editors C. M. Wood, A. P. Farrell, and C. J. Brauner (Part B: Academic Press), 31, 125–184. doi:10.1016/S1546-5098(11)31025-4
Menon, M., Mohanraj, R., Vb, J., and Prasath, R. V. A. (2023). Bioaccumulation of heavy metals in a gastropod species at the Kole wetland agroecosystem, a Ramsar site. J. Environ. Manag. 329, 117027. Article 117027. doi:10.1016/j.jenvman.2022.117027
Mero, M., Pernía, B., Ramírez-Prado, N., Bravo, K., Ramírez, L., Larreta, E., et al. (2019). Concentration of cadmium in water, sediments, Eichhornia crassipes, and Pomacea canaliculata in the Guayas (Ecuador) River and tributaries. Rev. Int. Contam. Ambient. 35 (3), 623–640. doi:10.20937/RICA.2019.35.03.09
Nigariga, P., Saren, D., Varma, R., and Sugumar, V. (2023). Assessment of trace metal bioaccumulation on the shells of edible gastropod Chicoreus ramosus and Hemifusus pugilinus. Environ. Monit. Assess. 195 (5), 608. doi:10.1007/s10661-023-11130-y
Owojori, O. J., Awodiran, M., Ayanda, O. E., and Jegede, O. O. (2022). Toxicity and accumulation of lead and cadmium in the land snail Archachatina papyracea in a tropical Alfisol from Southwestern Nigeria. Environ. Sci. Pollut. Res. 29 (29), 44917–44927. doi:10.1007/s11356-022-18947-z
Pedrini-Martha, V., Köll, S., Dvorak, M., and Dallinger, R. (2020). Cadmium uptake, MT gene activation and structure of large-sized multi-domain metallothioneins in the terrestrial door snail Alinda biplicata (Gastropoda, Clausiliidae). Int. J. Mol. Sci. 21 (5), 1631. doi:10.3390/ijms21051631
Pedrini-Martha, V., Schnegg, R., Schäfer, G. G., Lieb, B., Salvenmoser, W., and Dallinger, R. (2021). Responsiveness of metallothionein and hemocyanin genes to cadmium and copper exposure in the garden snail Cornu aspersum. J. Exp. Zoology Part A Ecol. Integr. Physiology 335 (2), 228–238. doi:10.1002/jez.2425
Pinkina, T., Zymaroieva, A., and Fedoniuk, T. (2022). Cadmium impact on the growth and survival rate of great pond snail (Lymnaea stagnalis) in the chronic experiment. Biologia 77 (3), 749–756. doi:10.1007/s11756-022-01015-9
Qiu, M., Bi, X., Liu, Y., Li, H., Li, D., and Chen, G. (2025). Toxicology effects of cadmium in Pomacea canaliculata: accumulation, oxidative stress, microbial community, and transcriptome analysis. Int. J. Mol. Sci. 26 (2), 751. doi:10.3390/ijms26020751
Rainbow, P. S. (2002). Trace metal concentrations in aquatic invertebrates: why and so what? Environ. Pollut. 120 (3), 497–507. doi:10.1016/s0269-7491(02)00238-5
Reátegui-Zirena, E. G., French, A. D., Klein, D. M., and Salice, C. J. (2017). Cadmium compartmentalization in the pulmonate snail Lymnaea stagnalis: improving our understanding of exposure. Archives Environ. Contam. Toxicol. 72 (4), 575–585. doi:10.1007/s00244-017-0407-8
Reolid, M., Boateng, M. C., and Mahu, E. (2024). Geochemical fractionation of trace elements in ostreid and gastropod shells: a potential proxy for heavy metal pollution in Ghana’s coastal environment. Estud. Geol. 80 (1), 1085. doi:10.3989/egeol.45247.1085
Sabri, S., Said, M. I. M., and Azman, S. (2014). Lead (Pb) and zinc (Zn) concentrations in marine gastropod Strombus canarium in Johor coastal areas. Malays. J. Anal. Sci. 18 (1), 37–42.
Simonyi-Poirier, M., Goulet, R. R., and Pick, F. R. (2003). The use of periphyton and the freshwater gastropod Helisoma trivolvis to assess dissolved metal retention by a constructed wetland. Rev. Sci. l'Eau 16 (2), 237–254. doi:10.7202/705506ar
Taylor, A., and Maher, W. (2006). The use of two marine gastropods, Austrocochlea constricta and Bembicium auratum, as biomonitors of zinc, cadmium, and copper exposure: effect of tissue distribution, gender, reproductive state, and temporal variation. J. Coast. Res. 22 (2), 298–306. doi:10.2112/05-0601.1
Ubrihien, R. P., Taylor, A. M., and Maher, W. A. (2017). Bioaccumulation, oxidative stress, and cellular damage in the intertidal gastropod Bembicium nanum exposed to a metal contamination gradient. Mar. Freshw. Res. 68 (5), 922–930. doi:10.1071/MF16026
Vidyalakshmi, D., Yesudas, A., Sivan, G., Akhil Prakash, E., and Priyaja, P. (2024). Heavy metal accumulation analysis using bivalve, sponge, sea urchin, and gastropod species as bioindicators. Mar. Pollut. Bull. 202, 116374. Article 116374. doi:10.1016/j.marpolbul.2024.116374
Yap, C. K., Edward, F. B., Pang, B., Ismail, A., Tan, S. G., and Jambari, H. A. (2009). Distribution of heavy metal concentrations in the different soft tissues of the freshwater snail Pomacea insularum (D’Orbigny, 1839; Gastropoda), and sediments collected from polluted and unpolluted sites from Malaysia. Toxicol. and Environ. Chem. 91, 17–27. doi:10.1080/02772240802010904
Yap, C. K., Hisyam, M. N. D., Edward, F. B., Cheng, W. H., and Tan, S. G. (2010). Concentrations of heavy metal in different parts of the gastropod Faunus ater collected from intertidal areas of Peninsular Malaysia. Pertanika J. Trop. Agric. Sci. 33 (1), 45–60.
Keywords: Cd bioaccumulation, Pomacea insularum, detoxification, freshwater biomonitoring, environmental contamination
Citation: Yap CK and Al-Mutairi KA (2025) Cadmium bioaccumulation and detoxification mechanisms in Pomacea insularum: implications for biomonitoring in freshwater ecosystems. Front. Environ. Sci. 13:1548453. doi: 10.3389/fenvs.2025.1548453
Received: 19 December 2024; Accepted: 21 February 2025;
Published: 10 March 2025.
Edited by:
Fayuan Wang, Qingdao University of Science and Technology, ChinaReviewed by:
Titus Chinedu Egbosiuba, Missouri University of Science and Technology, United StatesCopyright © 2025 Yap and Al-Mutairi. This is an open-access article distributed under the terms of the Creative Commons Attribution License (CC BY). The use, distribution or reproduction in other forums is permitted, provided the original author(s) and the copyright owner(s) are credited and that the original publication in this journal is cited, in accordance with accepted academic practice. No use, distribution or reproduction is permitted which does not comply with these terms.
*Correspondence: Chee Kong Yap, eWFwY2hlZUB1cG0uZWR1Lm15
Disclaimer: All claims expressed in this article are solely those of the authors and do not necessarily represent those of their affiliated organizations, or those of the publisher, the editors and the reviewers. Any product that may be evaluated in this article or claim that may be made by its manufacturer is not guaranteed or endorsed by the publisher.
Research integrity at Frontiers
Learn more about the work of our research integrity team to safeguard the quality of each article we publish.